- Department of Ecology, College of Applied Meteorology, Nanjing University of Information Science and Technology, Nanjing, China
Investigating the diurnal and seasonal variations of plant photosynthetic performance under future atmospheric CO2 conditions is essential for understanding plant adaptation to global change and for estimating parameters of ecophysiological models. In this study, diurnal changes of net photosynthetic rate (Anet), stomatal conductance (gs), and photochemical efficiency of PSII (Fv′/Fm′) were measured in two rice cultivars grown in the open-top-chambers at ambient (∼450 μmol mol–1) and elevated (∼650 μmol mol–1) CO2 concentration [(CO2)] throughout the growing season for 2 years. The results showed that elevated (CO2) greatly increased Anet, especially at jointing stage. This stimulation was acclimated with the advance of growing season and was not affected by either stomatal limitations or Rubisco activity. Model parameters in photosynthesis model (Vcmax, Jmax, and Rd) and two stomatal conductance models (m and g1) varied across growing stages and m and g1 also varied across (CO2) treatments and cultivars, which led to more accurate photosynthesis and stomatal conductance simulations when using these cultivar-, CO2-, and stage- specific parameters. The results in the study suggested that further research is still needed to investigate the dominant factors contributing to the acclimation of photosynthetic capacity under future elevated CO2 conditions. The study also highlighted the need of investigating the impact of other environmental, such as nitrogen and O3, and non-environmental factors, such as additional rice cultivars, on the variations of these parameters in photosynthesis and stomatal conductance models and their further impacts on simulations in large scale carbon and water cycles.
Introduction
Under the influence of human activities, atmospheric CO2 concentration [(CO2)] has been increasing to 415 μmol mol–1 in 2019 since the Industrial Revolution, before which the (CO2) was steadily maintained at about 280 μmol mol–1 (National Oceanic and Atmospheric Administration NOAA, 2019). It would possibly reach up to 936 μmol mol–1 in 2100 under RCP8.5 scenario (IPCC, 2014). Rice (Oryza sativa L.) has been one of the most important crops for human beings since the 1900s, especially in Asia and in the tropical and subtropical regions of Africa. Rice has the third largest planting area in the world and has been the main source of daily food for nearly half of the world’s population. With Global (CO2) rising and the increase of global population, it is critical to investigate and predict the responsive changes of the physiology and growth of rice to the increasing (CO2).
As the essential substrate for photosynthesis, the increase of (CO2) will stimulate photosynthesis and increase the biomass production and yield (Ainsworth and Long, 2005; Ainsworth and Rogers, 2007; Brito et al., 2020). Soil-Plant-Atmosphere-Research (SPAR) showed that rising (CO2) from 350 to 700 μmol mol–1 increased rice growth, grain yield and canopy photosynthesis and increased the final aboveground biomass by 29% with sufficient irrigation (Baker and Allen, 2005). The increase of air temperature at future elevated (CO2) made the CO2 effect on photosynthesis more complicated. Leaf photosynthetic rate (A) of two species, rice and soybean, were both increased under (CO2) enrichment, but this enhancement was reduced when the air temperature increased by 3°C (Vu et al., 1997). Rising (CO2) from 370 to 700 μmol mol–1 could offset the negative effect on photosynthesis due to 3.0–3.9°C warming, but had larger negative effect on photosynthetic carboxylation capacity in warming condition compared with ambient air temperature (Lamba et al., 2018). The combination of rising (CO2) and temperature increased the photosynthesis but decreased yield for rice (Cai et al., 2016). The yield reduction under the combination of 200 μmol mol–1 above ambient (CO2) and 1°C warming was reported in another research and the decrease of spikelet density might be the dominant factor (Wang W. et al., 2018).
Stomatal function is an important factor affecting photosynthesis and transpiration of plants. Lower stomatal conductance (gs) and transpiration rate (TR), therefore higher water use efficiency (WUE) due to elevated (CO2) were reported in varieties of species (Wand et al., 1999; Medlyn et al., 2001; Ainsworth and Rogers, 2007; Shimono and Bunce, 2009). Free air concentration enrichment (FACE) experiments showed an average increase of 23% in yield and a 25% reduction in gs when (CO2) increased from 360 to 627 μmol mol–1 (Ainsworth, 2008). FACE experiments in Iwate, Japan, indicated that raising (CO2) by 200 μmol mol–1 significantly decreased gs by 23% on average and doubling (CO2) made transpiration of rice reduced by 45 and 41% at full heading stage and mid-ripening stage, respectively (Shimono and Bunce, 2009; Shimono et al., 2010). Another research showed that lower gs of wheat grown under ambient (CO2) was observed compared with that under elevated (CO2) when ammonium fertilization was supplied as the sole N source, and this phenomenon disappeared when plants were set under nitrate nutrition (Torralbo et al., 2019). And EucFACE in 2017 found no significant influence on gs for one dominant C3 grass and two sympatric C3 forbs due to increasing (CO2) by 150 μmol mol–1 (Pathare et al., 2017). Therefore, the regulation of stomatal conductance by elevated CO2 could vary greatly depending on other environmental factors.
Responses of plant photosynthesis to short-term changes in environmental factors can be reflected through diurnal variations in photosynthesis. The maximum of Anet could be observed at 9 a.m. in tea [Camellia sinensis (L.) O. Kuntze] (Mohotti and Lawlor, 2002). An Conviron environmental chamber experiment for drought-tolerant plant Elaeagnus umbellata showed that Anet and gs both reached the maximum rates early in the day (around 10 a.m.) and the daily average levels decreased significantly during times of drought stress (Naumann et al., 2010). Research for maize showed that the maximum of Anet and gs occurred from 12 p.m. to 15 p.m. among two N fertilized and non-fertilized treatments, and the difference of Anet was significant due to N treatment when the light intensity was high, while the influence was found minimal on gs and Ci (Peng et al., 2014). FACE research focused on maize found no significant difference in the diurnal variation of Anet at ambient and elevated (CO2) in the early growing stage, but the difference could be observed in the late growing stage (Markelz et al., 2011). Depression of net CO2 assimilation occurred around noon due to stomatal closure and the decrease of intercellular CO2 (Ci) and elevated (CO2) could either significantly enhance the assimilation ability or weaken the midday depression (Nijs et al., 1992; Špunda et al., 2005). However, when C3 plants were exposed to elevated (CO2) for an extended period of time, the accelerated rate of photosynthesis often cannot be maintained, a phenomenon called photosynthetic acclimation to elevated (CO2) (Van Oosten and Besford, 1996). Ignoring this acclimation would possibly overestimate the magnitude of photosynthetic stimulation of elevated (CO2) concentrations compared with what was expected with the influence of short-term elevated (CO2) (Dusenge et al., 2019). Photosynthetic acclimation was usually associated with the down-regulation of stomatal conductance, Rubisco amount or activity, nitrogen concentrations or sink strength under elevated CO2 conditions (Ainsworth et al., 2004; Leakey et al., 2009; Rogers et al., 2017). For plants holding nitrogen-fixing bacteria, reduction of photosynthesis will be effectively alleviated under long-term elevated (CO2), especially under nitrogen limitation (Rho et al., 2020). Few studies have investigated the diurnal variations of photosynthetic characteristics of rice throughout the growing season. Whether the effects varied across different cultivars and growing stages requires systematic measurements of the diurnal variation of photosynthesis and stomatal conductance throughout the growing season to shed lights on both the short- and long- term effect of CO2 on photosynthetic characteristics.
Understanding and predicting large-scale carbon, water, and energy cycles requires accurate simulations in leaf photosynthesis and stomatal activities (Laisk et al., 2005). The Farquhar-von Caemmerer–Berry model (FvCB model), Ball, Woodrow and Berry (BWB model), and Medlyn model (MED model) are widely used photosynthetic and stomatal models to simulate the photosynthesis and stomatal conductance at the leaf level (Farquhar et al., 1980; Ball et al., 1987; Medlyn et al., 2012). The parameters of these models (Vcmax, Jmax, and Rd from the FvCB model, m and g0 from BWB model, g1 and g0 from MED model) are valuable for large-scale simulations and represent important physiological traits that determine plant photosynthetic potential and water-use efficiency. How do photosynthetic parameters of Vcmax, Jmax, and Rd and stomatal slope parameters vary among different rice cultivars and under different CO2 conditions require further study and analysis. Whether using cultivar-specific or environment-specific, instead of generic, model parameters can increase the accuracy of both photosynthesis and stomatal conductance simulations needs further investigation too.
In this study, diurnal changes of net photosynthetic rate (Anet), stomatal conductance (gs), intercellular CO2 concentration (Ci) and chlorophyll a fluorescence characteristics (photochemical efficiency of PSII, Fv′/Fm′; actual photochemical efficiency of PSII, ΦPSII; photochemical quenching, qP) were measured in two rice cultivars grown in the open-top-chambers at ambient (∼450 μmol mol–1) and elevated (∼650 μmol mol–1) CO2 concentrations throughout the growing season for 2 years. Seasonal variations of Vcmax, Jmax, and Rd and stomatal slope parameters were determined by Anet-Ci measurements at different growing stages. The objectives of this study were: (1) to investigate the diurnal and seasonal variations of rice photosynthetic performance under future atmospheric CO2 conditions; (2) to compare the diurnal and seasonal variations across two rice cultivars; (3) to test whether the photosynthetic and stomatal conductance models are effective under elevated CO2 conditions and whether using cultivar-, CO2- and stage- specific parameters can improve the accuracy of the photosynthesis and stomatal conductance simulations. Specifically, we hypothesized that (1) the positive effects of elevated (CO2) observed in the earlier growing season will decrease in the later growing season; (2) the extent of photosynthetic acclimation will be smaller for the more CO2-responsive cultivar; (3) the cultivar-, CO2-, and stage- specific parameters will increase the accuracy of the photosynthetic and stomatal conductance models.
Materials and Methods
Site Description
The study site was located in the agrometeorological experimental station of Nanjing University of Information Science and Technology, in Nanjing, Jiangsu province of China (32°16′N, 118°86′E). The climate in this region characterizes subtropical monsoon season, with the annual average precipitation of 1,100 mm, the average temperature in recent years of 15.6°C and the average annual frost-free period of 237 days. The soil texture in the tillage layer was loamy clay and the clayey content was 26.1%. The bulk density of 0–20 cm soil was 1.57 g⋅cm–3 and the pH (H2O) value were 6.3. The organic carbon and total nitrogen content were 11.95 and 1.19 g⋅kg–1, respectively.
Experimental Design
Open top chambers (OTC) were used in the experiment to simulate elevated (CO2) treatments and description was provided in Supplementary Material 1. The OTCs are regular octagonal prisms with a diameter of 3.75 m, a height of 3 m, and a base area of 10 m2. There were two (CO2) treatments, ambient [a(CO2)] and elevated [e(CO2)], each with four replicative chambers. The treatment of elevated (CO2) started from the turning green stage and lasted to the end of growing season. The (CO2) concentration in the OTCs was controlled with an automatic control platform, composed of CO2 sensors, gas-supplying devices and automatic control system. Three wind-blowing fans were placed in each chamber to make the CO2 gas in the chamber evenly distributed and the top of the OTC is designed with an opening inclined 45° inward to avoid the rapid loss of CO2 gas. The CO2 sensor fed back the surrounding CO2 concentration information in the chambers to the automatic control system every 2 s. Our experiment was performed from 2019 to 2020 to make sure that the trend we observed was not a random impact. The daytime (CO2) averaged over the growing season was 641 ± 43 and 631 ± 39 μmol mol–1 in elevated (CO2) chambers and 478 ± 34 and 485 ± 33 μmol mol–1 in ambient (CO2) chambers in 2019 and 2020, respectively. The nighttime (CO2) was 667 ± 27 and 673 ± 24 μmol mol–1 in elevated (CO2) chambers and 537 ± 49 and 550 ± 36 μmol mol–1 in ambient (CO2) chambers.
Two rice cultivars, Yangdao6, a more CO2-responsive indica cultivar and Wuyunjing30, a less CO2-responsive japonica cultivar, as indicated in previous studies (Zhu et al., 2015; Tao and Wang, 2021; Wang et al., 2021), were selected in this study. Seedlings of each rice cultivar were transplanted into the eight OTCs on June 19, 2019 and June 16, 2020, and harvested on October 25, 2019 and October 28, 2020, respectively. The spacing of the hills was 16.7 cm × 25 cm (equivalent to 24 hills m–2). During the whole growing season, sufficient supplies of water and fertilizer were maintained.
Photosynthetic Measurements
Gas exchange characteristics (including net photosynthetic rate and stomatal conductance) were measured with a portable infrared gas analyzer (LI-COR 6400LCF; LI-COR, Lincoln, NE, United States) on one randomly selected and fully expanded healthy leaf of each cultivar from each chamber. The measurement was taken at jointing, booting, heading, grain-filling and maturity stage on July 24, August 18, September 8, September 28 and October 13 in 2019, and July 25, August 24, September 7, September 24 and October 20 in 2020, respectively, which were confirmed by observation and previous research (Counce et al., 2000; IRRI, 2013). During diurnal measurements, the (CO2) of the leaf chambers were set according to the OTC conditions and the temperature and light levels were set as the environmental conditions. Photochemical efficiency of PSII in light-adapted leaves (Fv′/Fm′) and photochemical quenching (qP) were measured using a Licor 6400-40 Leaf Chamber Fluorometer. The photosynthesis-CO2 response (Anet–Ci) curves were measured on the next day after the diurnal measurements were taken. During measurements, leaves were acclimated for 30–60 min before adjusting the CO2 concentrations. Thereafter, CO2 concentration was decreased in six steps (400, 300, 230, 150, 90, and 50 μmol mol–1 CO2) and then increased in four steps (400, 600, 800, and 1,000 μmol mol–1 CO2). Leaf temperature was controlled at 35, 35, 35, 30, and 25°C when Anet–Ci curve measurement was conducted at jointing, booting, heading, grain-filling, and maturity stage, respectively, and photosynthetic photon flux density (PPFD) was maintained at 2,000 μmol m–2 s–1 all the time because the light level is close to the midday light level in the region.
Assessing the Photosynthetic and Stomatal Conductance Parameters
Anet-Ci curves were fit to the FvCB models (Equations 1, 2) to solve the photosynthetic parameters including maximum ribulose 1⋅5-bisphosphate carboxylase/oxygenase (Rubisco) carboxylation rate (Vcmax, μmol m–2 s–1), potential light saturated electron transport rate (Jmax, μmol m–2 s–1), and leaf dark respiration (Rd, μmol m–2 s–1), respectively. Anet-Ci curves were then fit to the BWB (Equation 3) and Medlyn (Equation 4) models to solve the parameters of m and g1 (Wolz et al., 2017; Wang D. et al., 2018).
where Ci is the intercellular (CO2), O is the oxygen concentration, Γ∗ is the photosynthetic CO2 compensation point without dark respiration, KC and KO are the Michaelis–Menten constants for CO2 and O2 and can be found in Bernacchi et al. (2001). Equation 1 can be used if Anet is limited by Rubisco activity [low (CO2)] and if Anet is limited by the regeneration of ribulose-1,5-bisphosphate (RuBP) at high (CO2), it can be determined as follows:
where a and b are model parameters and are given as 4.0 and 8.0, respectively (Bernacchi et al., 2003); J is the photosynthetic electron transport rate and can be used to estimate Jmax with the non-rectangular hyperbolic model (von Caemmerer, 2000). Values of both Vcmax and Jmax would be corrected to those at 25°C after the estimation by using Arrhenius equation provided from Bernacchi et al. (2003).
For each leaf, a linear least squares regression of Equations 3 or 4 was used to estimate the intercept and slope parameters of the BWB and MED model, respectively. Biologically, the slope parameter of each model represents the sensitivity of gs to changes in Anet, Ca, and atmospheric water status and will be the focus of this analysis. A term for the y intercept of each model algorithm (g0) can be used to describe variation in minimum gs. Only simulations that provided a regression between modeled and observed stomatal conductance with an R2 > 0.8 were included in further analyses (Wolz et al., 2017).
where gs is stomatal conductance (mol m–2 s–1), A is the net rate of photosynthetic CO2 uptake (μmol m–2 s–1), h is atmospheric relative humidity (unitless), Ca is the atmospheric CO2 concentration at the leaf surface (μmol mol–1), g0 is the y-axis intercept and m is the slope of the line.
where D is the atmospheric vapor pressure deficit (kPa) and g1 is the model parameter related to the slope of the line. Previous study showed that whether the value of g0 is 0 does not significantly affect the linear and non-linear relationship in Equations 3, 4 (Leakey et al., 2006), so we set the value of g0 to 0 in order to focus on the variations of the stomatal slope.
The diurnal measurements of Anet and gs were used to validate the coupled FvCB photosynthetic model and BWB and MED stomatal conductance models (Wolz et al., 2017). Specific (cultivar-, CO2- and stage- specific) photosynthetic and stomatal parameters and generic parameters (the average values across all the groups) were used to test whether the specific parameters will increase the accuracy of the models.
Statistical Analysis
Four-way analysis of variance (ANOVA) was used to test the fixed effects of years, cultivars, growing stages, CO2 treatments and their interactions on Anet, gs, Fv′/Fm′, Vcmax, Jmax, Rd and stomatal slope parameters. Post hoc Tukey’s HSD tests were conducted on specific contrasts to examine significant treatment effects among groups. General linear models (GLM) were used to assess the relationship between observed and modeled parameters. For all the analysis, the normality of the residuals was tested using the Shapiro–Wilk test. All statistical testes were considered significant at P ≤ 0.05. Mean values of each variable were expressed with their standard error (SE). All analyses were conducted in R with package “plantecophys” and all figures were drawn with the “ggplot” function in package “tidyverse” (R 3.6.31).
Results
Diurnal and Seasonal Variations in Environmental Parameters
The photosynthetic active radiation (PAR) usually reached its maximum from 10 a.m. to 13 p.m. (Figure 1). The maximum values of PAR varied on the measuring date across the growing season, with 1,809.96, 1,619.51, 1,519.59, 1,574.90, and 1,489.53 μmol m–2 s–1 at jointing, booting, heading, grain-filling and maturity stages in 2019 and 2,019.40, 1,919.95, 1,850.80, 1,940.95, and 1,701.12 μmol m–2 s–1 in 2020, respectively. Diurnal variations of air temperature (Tair) and vapor pressure deficit (VPD) followed the similar trend as PAR. The maximal Tair were 38.03, 32.91, 32.97, 31.06, and 25.28°C in 2019 and 34.50, 37.71, 34.62, 28.92, and 22.57°C in 2020, while those of VPD were 2.09, 1.96, 2.02, 1.72, and 1.41 kPa in 2019 and 2.29, 2.42, 2.18, 1.98, and 1.42 kPa in 2020 on the measuring date at five growing stages. Tair, VPD and PAR varied significantly across the growing season.
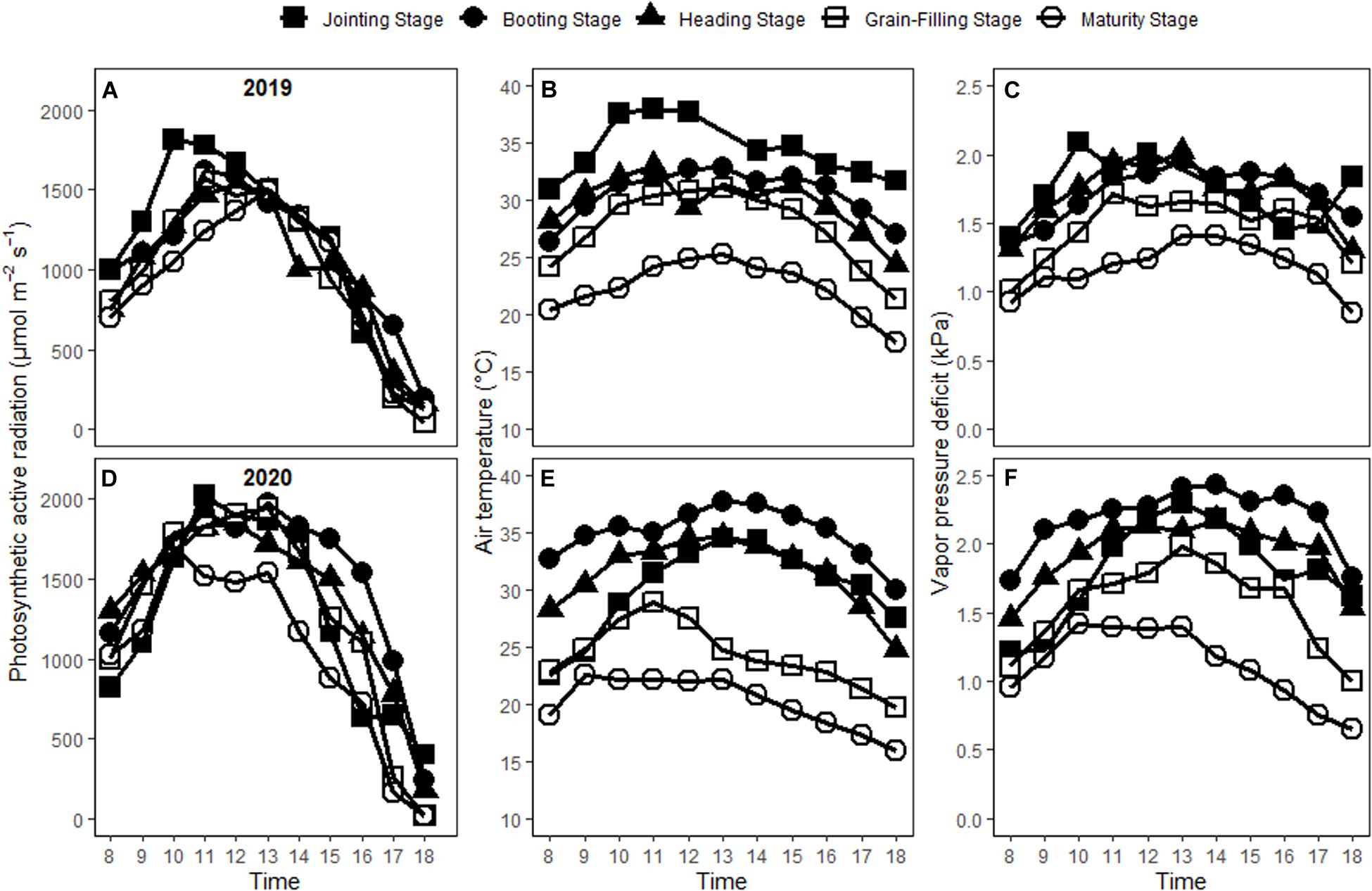
Figure 1. Changes of photosynthetic active radiation (A,D), air temperature (B,E), and vapor pressure deficit (C,F) with time on the measuring date at five different growing stages in 2019 and 2020.
Diurnal and Seasonal Variations in Anet and gs
In most cases, Anet increased to the maximum around noon and decreased in the afternoon and the maximum value appeared no later than 14 p.m. (Figure 2). The midday depression of Anet was observed on some of the measuring dates for both the cultivars and (CO2) treatments. On average, elevated (CO2) significantly stimulated Anet of both cultivars by 34.19% in 2019 and 47.65% in 2020 in the whole growing season, which was consistent with the impact of elevated (CO2) on intercellular (CO2) (Supplementary Material 2). The greatest stimulation of elevated (CO2) on Anet could be observed around midday at jointing stage (54.35% in 2019 and 56.12% in 2020 for Wuyunjing30; 57.95% in 2019 and 61.50% in 2020 for Yangdao6), and the enhancement of Anet of each cultivar due to elevated (CO2) at the following four growing stages were much lower than that at this stage in 2 years (Supplementary Material 3). Anet decreased significantly with the advance of growing season and there were significant (CO2) × growing stage effect on Anet (Table 1 and Supplementary Material 4). The more CO2-responsive cultivar Yangdao6 had higher Anet than Wuyunjin30 and the two cultivars responded similarly to elevated (CO2) (Supplementary Material 5).
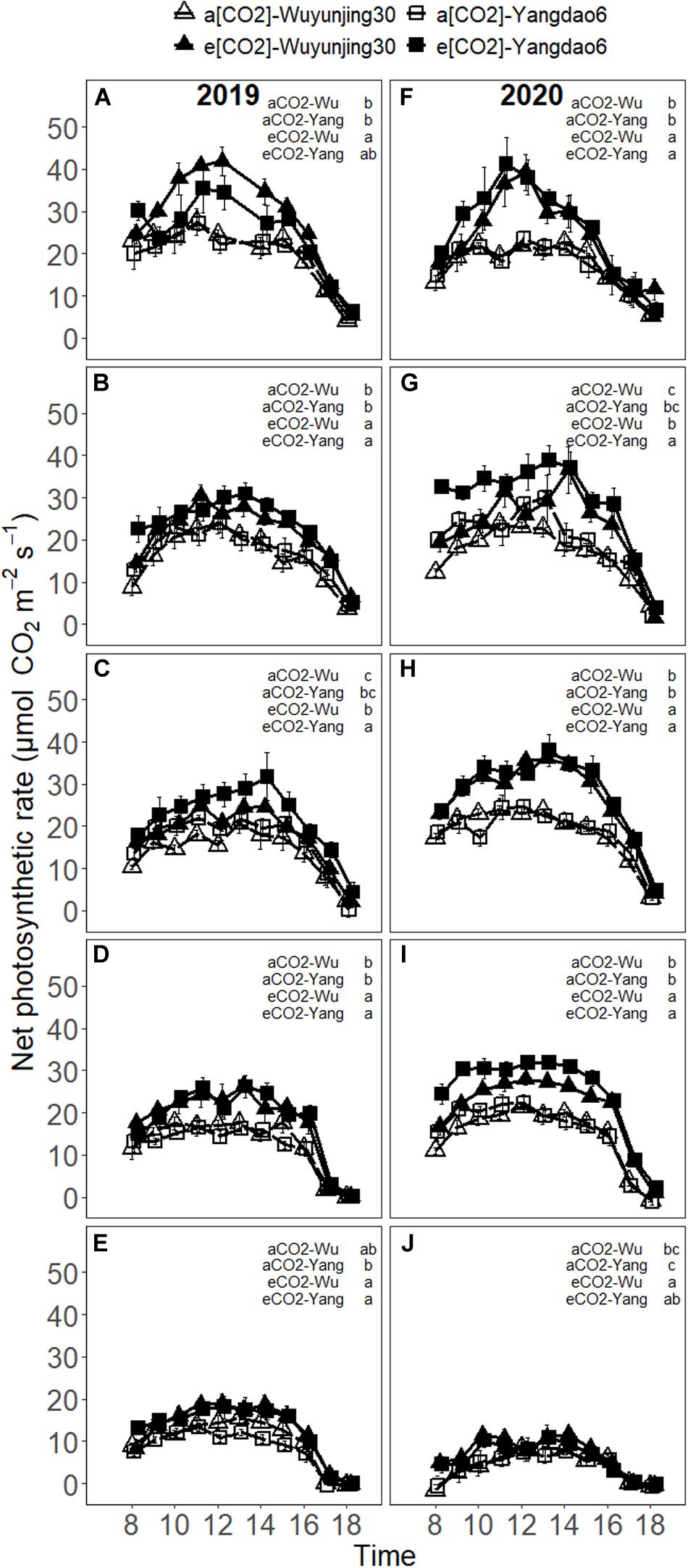
Figure 2. Diurnal variations in net photosynthetic rate (Anet) of rice cultivar Wuyunjing30 and Yangdao6 grown at ambient and elevated (CO2) at jointing (A,F), booting (B,G), heading (C,H), grain-filling (D,I), and maturity (E,J) stages in 2019 and 2020. Values were expressed as means ± standard errors form. Values of Anet under four (CO2) × cultivar treatments at 13 p.m. at jointing stage in 2019 were deleted because of the abnormal values found by examination. Statistical analyses of multiple comparisons for five growing stages in 2 years were shown in each panels of the figure in the form of lowercase letters.
The values of gs remained relatively high before 15 p.m. and started to decrease after that (Figure 3). In 2019, gs under all (CO2) × cultivars tended to decrease significantly with the advance of growing season, while a slight recovery of gs beginning at heading stage was observed for Wuyunjing30 under both (CO2) treatments (Supplementary Material 4). In 2020, gs of Yangdao6 under both (CO2) treatments slightly increased from jointing to booting stage, while that of Wuyunjing30 decreased or remained stable during this period, and gs always decreased from heading to maturity stage. Seasonal variations in gs synchronized well with those in Anet, especially in 2020. Elevated (CO2) had no significant effect on gs (Table 1 and Supplementary Material 3). The values of gs of cultivar Yangdao6 were higher than those of Wuyunjing30 (Supplementary Material 5).
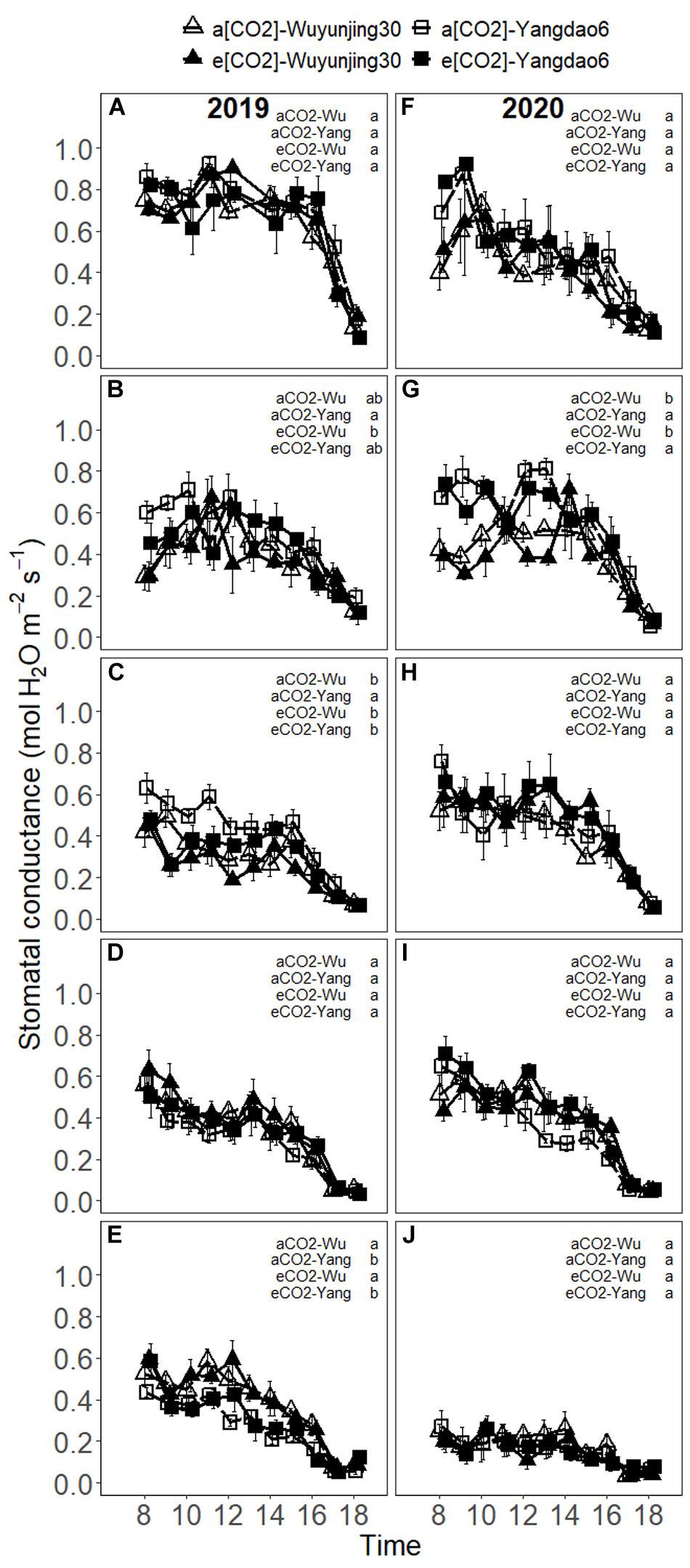
Figure 3. Diurnal variations in stomatal conductance (gs) of rice cultivar Wuyunjing30 and Yangdao6 grown at ambient and elevated (CO2) at jointing (A,F), booting (B,G), heading (C,H), grain-filling (D,I), and maturity (E,J) stages in 2019 and 2020. Values were expressed as means ± standard errors form. Values of gs under four (CO2) × cultivar treatments at 13 p.m. at jointing stage in 2019 were deleted because of the abnormal values found by examination. Statistical analyses of multiple comparisons for five growing stages in 2 years were shown in each panels of the figure in the form of lowercase letters.
Diurnal and Seasonal Variations in Fv′/Fm′, ΦPSII, and qP
The values of Fv′/Fm′, ΦPSII, and qP decreased to the minimum around noon and recovered to normal or even higher levels under each treatment (Figure 4 and Supplementary Materials 6, 7). Fv′/Fm′ increased with the advance of growing season and reached the maximum at heading stage or grain-filling stage, and decreased at maturity stage in 2019 (Supplementary Material 4). In 2020, Fv′/Fm′ decreased to the minimum value at booting stage at first, then increased to the maximum value at heading or grain-filling stage, and decreased till the end of the growth period. On average, elevated (CO2) significantly increased Fv′/Fm′ of both cultivars by 6.92% in 2019 and 6.41% in 2020 across the growth season, while qP was decreased by 4.30% in 2019 and 3.97% in 2020, respectively (Table 1). The greatest stimulation influences on Fv′/Fm′ due to elevated (CO2) at midday were 21.39% at heading stage in 2019 and 26.80% at the same stage in 2020 for Wuyunjing30, and 16.18% at maturity stage in 2019 and 29.27% at grain-filling stage in 2020 for Yangdao6 (Supplementary Material 3). The values of Fv′/Fm′ of cultivar Yangdao6 were significantly higher than those of Wuyunjing30 (Supplementary Material 5).
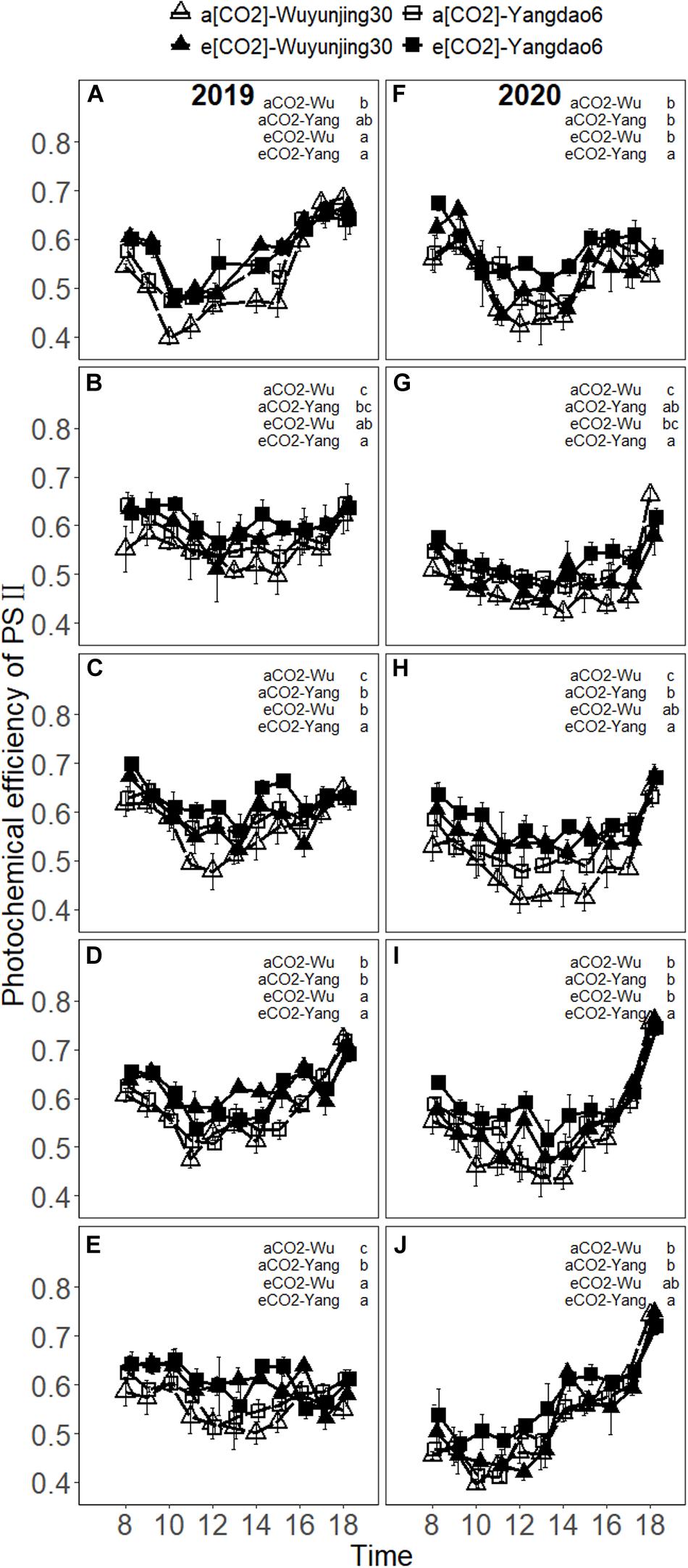
Figure 4. Diurnal variations in photochemical efficiency of PSII (Fv′/Fm′) of rice cultivar Wuyunjing30 and Yangdao6 grown at ambient and elevated (CO2) at jointing (A,F), booting (B,G), heading (C,H), grain-filling (D,I), and maturity (E,J) stages in 2019 and 2020. Values were expressed as means ± standard errors form. Values of Fv′/Fm′ under four (CO2) × cultivar treatments at 13 p.m. at jointing stage in 2019 were deleted because of the abnormal values found by examination. Statistical analyses of multiple comparisons for five growing stages in 2 years were shown in each panels of the figure in the form of lowercase letters.
Seasonal Variations of Photosynthetic and Stomatal Parameters
For most treatments, Vcmax and Jmax reached the maximum at heading stage and decreased to the minimum at maturity stage (Table 2), which could also be observed in Anet-Ci curves (Supplementary Material 8). Values of Vcmax at booting stage were higher than those at jointing stage only for Yangdao6 in 2019. Values of Jmax at booting stage were lower than those at jointing stage for Wuyunjing30 grown at ambient and elevated (CO2) and Yangdao6 grown at elevated (CO2) in 2019. The values of Rd increased to the maximum at grain-filling or maturity stage. There is no significant influence of (CO2) or cultivar or the interaction on Vcmax, Jmax, and Rd (Table 2), and Anet-Ci curves showed no differences of three parameters among four (CO2) × cultivar treatments, either (Supplementary Material 9). The values of m and g1 were highest at maturity stage across (CO2) × cultivar treatments. On average, elevated (CO2) significantly decreased m and g1 of both cultivars by 7.62 and 9.82% respectively. The values of m and g1 of more CO2-responsive cultivar Yangdao6 was 10.75 and 10.87% lower than those of less CO2-responsive cultivar Wuyunjing30, respectively.
The Photosynthesis and Stomatal Conductance Simulations
The predicted values of Anet and gs correlated well with the measured ones across all treatments no matter whether BWB or MED was used or specific or generic model parameters were selected (Figures 5, 6). The square of Pearson correlation coefficient (r2) between predicted and measured values was used to describe the simulation accuracy in our study. We found that the accuracy of photosynthetic simulations was higher when using the specific parameters (r2 = 0.83) than using the generic ones (r2 = 0.66). The moderate improvement of gs simulations was achieved using specific parameters (r2 = 0.45 for BWB and 0.47 for MED model), compared with using generic parameters (r2 = 0.41 for BWB and 0.37 for MED models).
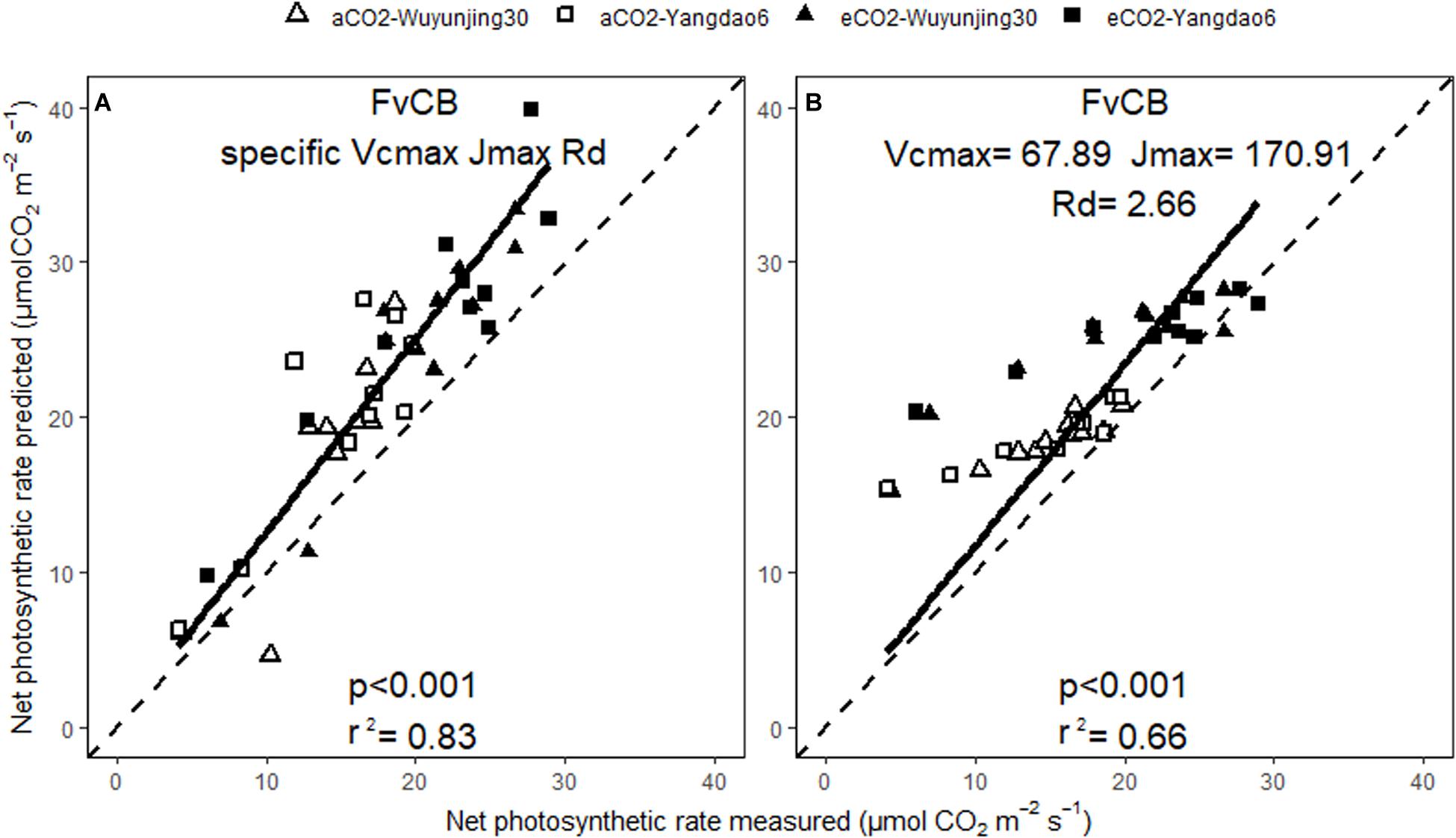
Figure 5. Relationships between the measured and simulated daily average net photosynthetic rate for the two rice cultivars at ambient and elevated (CO2) throughout the growing season. Net photosynthetic rate was simulated with FvCB model using either specific model parameters (A) or generic model parameters (B). p value of the correlation analysis and the square of Pearson correlation coefficient (r2) are given.
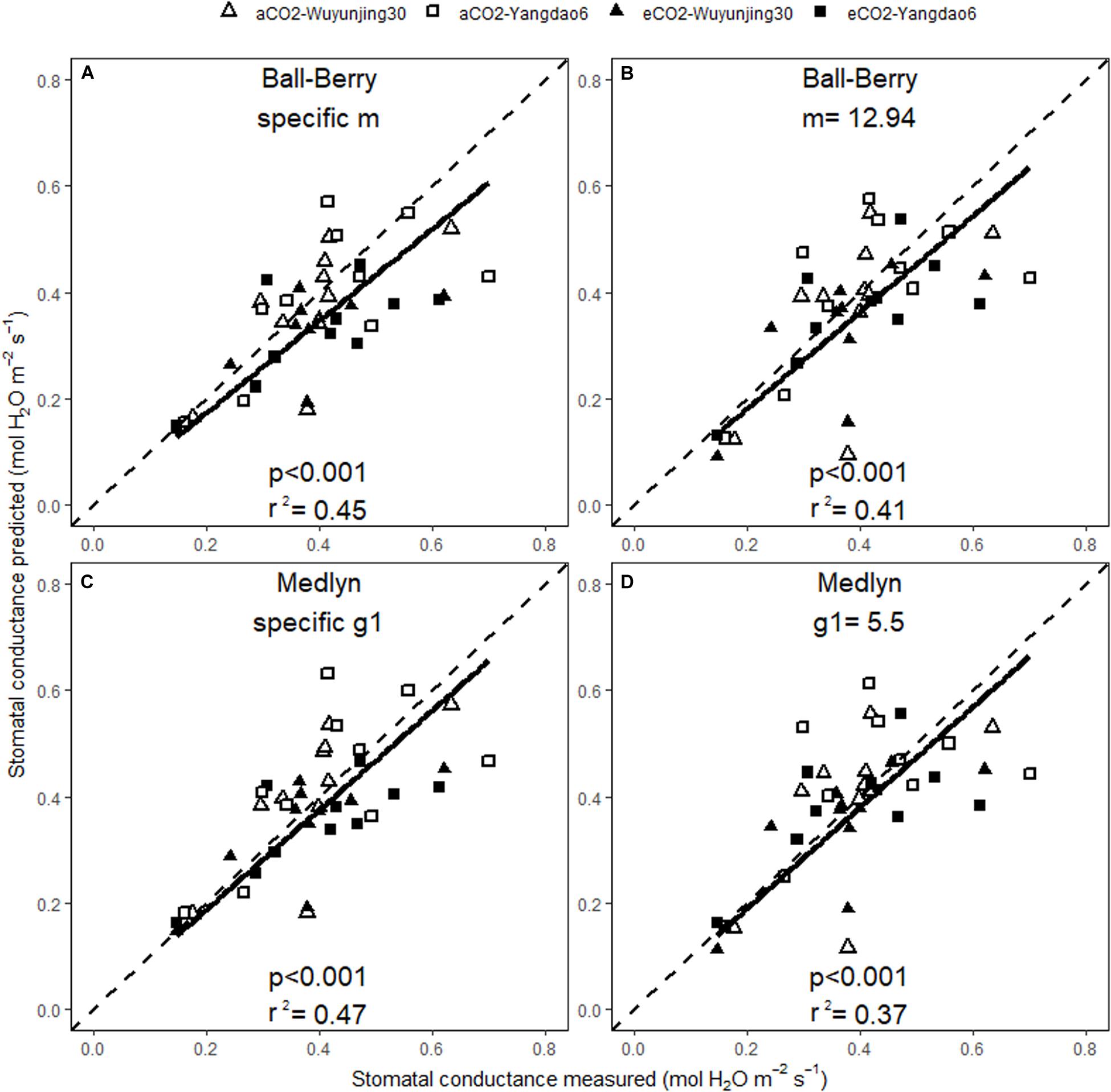
Figure 6. Relationships between the measured and simulated daily average stomatal conductance for the two rice cultivars at ambient and elevated (CO2) throughout the growing season. Stomatal conductance was simulated with BWB (A,B) or MED models (C,D), using either specific model parameters (A,C) or generic model parameters (B,D). p value of the correlation analysis and the square of Pearson correlation coefficient (r2) are given.
Discussion
Individual studies and meta-analysis have investigated the general tendency of elevated CO2 effects on plant physiology and production (Ainsworth and Long, 2005; Ainsworth, 2008; Wang et al., 2012). However, it remains unclear how the effects of elevated CO2 varied under short- and long- term treatment and across different rice cultivars. In this study, we took the diurnal and Anet-Ci photosynthetic measurements throughout the growing season for 2 years. Overall, we found that (1) the diurnal variation of net photosynthetic rate was mainly affected by the dynamic of photosynthetic active radiation and air temperature and the midday depression of net photosynthetic rate observed in most cases was caused by both stomatal and non-stomatal factors; (2) CO2 had a positive effect on net photosynthetic rate and the effects decreased after the booting stage and the degree of the acclimation did not vary between the two tested cultivars; (3) the photosynthetic and stomatal conductance models proved to be effective under elevated CO2 conditions and using specific parameters greatly improved the accuracy of the photosynthetic simulations and moderately improved that of stomatal conductance simulations.
In general, values of Anet were lower in the early morning and the late afternoon and the maximum occurred around noon. These unimodal or bimodal patterns were consistent with the dynamics of PAR and Tair across growing stages. In contrary, although the decrease of gs was synchronous with that of Anet in the afternoon, the variation of gs in the morning could not reflect the dynamic of Anet in this period. Therefore, light intensity as well as air temperature, rather than stomatal functions, were the dominant factors affecting the diurnal variation of Anet on daily scale (Wen et al., 1998). The midday depression of Anet was observed in most cases in these 2 years and the synchronous decline of gs could also be found when midday depression of Anet occurred, which was consistent with previous studies (Farquhar and Sharkey, 1982; Panda, 2011; Koyama and Takemoto, 2014). Short-term changes of Ci should be considered when determining whether stomatal or non-stomatal limitation caused the midday depression (Farquhar and Sharkey, 1982). Stomatal limitation would be the major factor if the reduction of Ci and gs was synchronously observed when the midday depression of Anet occurred. In contrary, if Ci kept at the stable value or even increased, non-stomatal limitation should be taken into account. In our research, for example, rapid decrease of Ci could be observed under the situation of photosynthetic midday depression for Wuyunjing30 under both ambient and elevated (CO2) at heading stage in 2019. But Ci changed little or remained unchanged, for Wuyunjing30 and Yangdao6 under ambient (CO2) at jointing stage in 2020. Meanwhile, we noticed the decrease of Fv′/Fm′ and qP around noon for each cultivar at both the (CO2) treatments and they recovered to normal or even higher values at late afternoon, proving the existence of reversible photoinhibition in rice throughout the growth season (Adams and Demmig-Adams, 1992; Demmig-Adams and Adams, 1992). Liu et al. (2020) demonstrated that the midday depression of Anet in leaves of Drepanostachyum luodianense was mainly caused by non-stomatal limitation in April, but caused by stomatal limitation in July. And in September, stomatal limitation was the dominant factor leading to the Anet depression from 10 a.m. to 12 p.m. while non-stomatal limitation dominated the depression from 12 p.m. to 13 p.m. Therefore, the midday depression of photosynthesis was caused by both the stomatal and non-stomatal limitations such as photoinhibition, and the dominant factor changed depending on the surrounding environment. While the dynamics of major environmental factors such as PAR and air temperature affected the overall trend of the diurnal variation of rice photosynthesis, stomatal closure and photoprotection of plants responding to high light intensity and other non-stomatal limitations were the dominant factors affecting the midday decrease of the photosynthesis.
The stimulation of elevated (CO2) on plant photosynthesis has been widely reported in recent decades, but the magnitude of the stimulation varied greatly depending on species, plant functional types (PFTs) and other environmental factors (Ainsworth and Long, 2005; Wang et al., 2012). In this study, elevated (CO2) enhanced Anet of rice by 34.19 and 47.65% on average throughout the growing season in 2019 and 2020, respectively, which was comparable to what was reported 33% increase in the meta-analysis studies (Wand et al., 1999; Ainsworth and Long, 2005). Higher intercellular CO2 concentration (Ci) and photochemical efficiency of PSII (Fv′/Fm′) were the main factors for the stimulation on Anet of rice at elevated (CO2). Photosynthetic acclimation to elevated (CO2) was found in crop varieties with limited sink size (Thilakarathne et al., 2015; Ruiz-Vera et al., 2017), but not in those with many large leaves (Ruiz-Vera et al., 2017) or high tillering potentials (Tausz-Posch et al., 2015). The enhancement of Anet due to elevated (CO2) was greatest at jointing stage in 2 years, but was weakened afterward and even disappeared at maturity stage in 2020, which suggested the occurrence of photosynthetic acclimation at elevated (CO2) and it could happen at the early growing season. We did not observe the less enhancement by elevated (CO2) on Fv′/Fm′ and qP at the following growing stages compared with jointing stage or any significant influence of elevated (CO2) on gs, Vcmax and Jmax which could explain the early season photosynthetic acclimation. Therefore, the down-regulation of stomatal and Rubisco enzyme functions might not be the only reasons for the photosynthetic acclimation of rice at elevated (CO2) (Chen et al., 2005). A more frequent and detailed measurement in the early growing season was required to fully understand the evident but decreased elevated CO2 effect on the net photosynthesis. The elevated CO2 effect on rice roots growth, morphology and physiology was also under investigation in this study to further discover whether the photosynthetic acclimation of rice was the added-up effects from both the above- and below- ground physiological changes. Besides, there was no significant difference of the photosynthetic acclimation between more CO2-responsive cultivar Yangdao6 and less CO2-responsive cultivar Wuyunjing30 in terms of the occurrence and the extent of the acclimation, even though higher Anet and gs were observed in Yangdao6 compared with Wuyunjing30 throughout the growing season, which was consistent with previous studies (Zhu et al., 2015).
Model parameters in FvCB model (Vcmax, Jmax, and Rd) and stomatal slope parameters in BWB model (m) and MED model (g1) varied across growing stages. The decrease of Vcmax and Jmax could be the reason for the decline of photosynthetic capacity with the advance of growth period. Vcmax, Jmax, and Rd varied not only among different species (Wullschleger, 1993), but also within single species in different environments (Bunce, 2000; Medlyn et al., 2002a; Medlyn et al., 2002b). Elevated (CO2) did not lead to significant changes of Vcmax and Jmax in our research. The response of Vcmax, Jmax, and Rd in C3 plants grown at elevated (CO2) were reported to be quite different and both stimulation and inhibition of elevated (CO2) effects had been reported in recent years (Sims et al., 1998a; Sims et al., 1998b; Ellsworth et al., 2004). Elevated (CO2) treatment as short as 7 days increased Vcmax and Jmax for cucumber by 12.0 and 14.7% without drought stresses, but the enhancement was no longer significant under mild and severe drought stresses (Liu et al., 2018). From our ANOVA analysis, Vcmax, Jmax, and Rd of rice did not vary significantly across cultivars, suggesting that photosynthetic simulations could use similar parameters at least between the two tested cultivars. For stomatal conductance models, previous research has reported significant correlation between stomatal slope parameters and environmental factors such as temperature, moisture and season and plant physiological factors such as wood density (Lin et al., 2015). And recent research has also indicated the variability of stomatal slopes in plants at species level (Wolz et al., 2017). FACE research reported no stomatal acclimation for soybean at elevated (CO2) (Leakey et al., 2009). However, the Ball–Berry slope was significantly different between ambient and elevated (CO2) grown wheat (Tausz-Posch et al., 2013). In our research, stomatal slopes (m and g1) varied across both (CO2) treatments and cultivars, demonstrating that variability of m and g1 was caused not only by the diversity of plant function groups but also by the diversity of physiological characteristics among cultivars within a single species.
Higher accuracy of simulation using specific model parameters in both photosynthesis model (Vcmax, Jmax, and Rd) and stomatal conductance model (m and g1) compared with using generic parameters was achieved in this study, suggesting that future simulations in large scale carbon and water cycles should take account of the variations of model parameters across environmental and non-environmental factors. Though we did not observe a significant impact of elevated (CO2) on Vcmax and Jmax, it should be noted that not considering the impact of mesophyll conductance (gm) might lead to the potential increased systematic errors of determining Vcmax and Jmax (Singsaas et al., 2003). And the possibility that the differences of model parameters of rice will change under other environmental conditions should not be ruled out too. Therefore, further research focusing on the diversity of model parameters are needed to confirm the existence of (CO2), cultivar and growing stage variation of photosynthesis and stomatal conductance model parameters of rice and other plant species.
In conclusion, diurnal variations of net photosynthetic rate of rice showed unimodal or bimodal patterns, which was mainly influenced by light intensity and air temperature. Meanwhile, the closure of stomata and the photoinhibition around noon were dominant factors for the short-term midday depression of net photosynthetic rate. Elevated (CO2) greatly increased net photosynthetic rate at jointing stage. This stimulation was acclimated with the advance of growing season and was not affected by either stomatal limitation or Rubisco activity. Model parameters in photosynthesis model (Vcmax, Jmax, and Rd) and two stomatal conductance models (m and g1) varied across growing stages and m and g1 also varied across (CO2) treatments and cultivars, which led to more accurate photosynthesis and stomatal conductance simulations when using these specific parameters. The results in the study suggested that further researches are still needed to investigate the dominant factors contributing to the acclimation of photosynthetic capacity under future elevated CO2 conditions. The study also highlighted the need of investigating the impact of other environmental, such as nitrogen and O3, and non-environmental factors, such as additional rice cultivars, on the variations of the model parameters in photosynthesis and stomatal conductance models and the further impacts on simulations in large scale carbon and water cycles.
Data Availability Statement
The raw data supporting the conclusions of this article will be made available by the authors, without undue reservation.
Author Contributions
DW conceived the idea and led the study. YM and YC designed the experiment. YM, YC, and HW measured all the data used for this study. YM analyzed the data and wrote the manuscript with the critical suggestions by DW. All authors contributed to the article and approved the submitted version.
Funding
Funding for this research was provided by Nanjing University of Information Science and Technology (2013r115), Jiangsu Distinguished Professor Scholarship, Jiangsu Six Talent Peaks (R2016L15), the Jiangsu Natural Science Foundation (BK20150894), and the National Natural Science Foundation of China (31500503 and 31770485) through DW.
Conflict of Interest
The authors declare that the research was conducted in the absence of any commercial or financial relationships that could be construed as a potential conflict of interest.
Supplementary Material
The Supplementary Material for this article can be found online at: https://www.frontiersin.org/articles/10.3389/fpls.2021.651606/full#supplementary-material
Footnotes
References
Adams, W. W., and Demmig-Adams, B. (1992). Operation of the xanthophyll cycle in higher plants in response to diurnal changes in incident sunlight. Planta 186, 390–398. doi: 10.1007/BF00195320
Ainsworth, E. A. (2008). Rice production in a changing climate: a meta-analysis of responses to elevated carbon dioxide and elevated ozone concentration. Glob. Chang. Biol. 14, 1642–1650. doi: 10.1111/j.1365-2486.2008.01594.x
Ainsworth, E. A., and Long, S. P. (2005). What have we learned from 15 years of free-air CO2 enrichment (FACE)? A meta-analytic review of the responses of photosynthesis, canopy properties and plant production to rising CO2. New Phytol. 165, 351–372. doi: 10.1111/j.1469-8137.2004.01224.x
Ainsworth, E. A., and Rogers, A. (2007). The response of photosynthesis and stomatal conductance to rising [CO2]: mechanisms and environmental interactions. Plant Cell Environ. 30, 258–270. doi: 10.1111/j.1365-3040.2007.01641.x
Ainsworth, E. A., Rogers, A., Nelson, R., and Long, S. P. (2004). Testing the “source–sink” hypothesis of down-regulation of photosynthesis in elevated [CO2] in the field with single gene substitutions in Glycine max. Agric. For. Meteorol. 122, 85–94. doi: 10.1016/j.agrformet.2003.09.002
Baker, J. T., and Allen, L. H. (2005). Rice growth, yield and photosynthetic responses to elevated atmospheric carbon dioxide concentration and drought. J. Crop Crop Improv. 13, 7–30. doi: 10.1300/j411v13n01_02
Ball, J. T., Woodrow, I. E., and Berry, J. A. (1987). “A model predicting stomatal conductance and its contribution to the control of photosynthesis under different environmental conditions,” in Progress in Photosynthesis Research, ed. J. Biggens (Berlin: Springer), 221–224. doi: 10.1007/978-94-017-0519-6_48
Bernacchi, C. J., Pimentel, C., and Long, S. P. (2003). In vivo temperature response functions of parameters required to model RuBP-limited photosynthesis. Plant Cell Environ. 26, 1419–1430. doi: 10.1046/j.0016-8025.2003.01050.x
Bernacchi, C. J., Singsaas, E. L., Pimentel, C., Portis, A. R. Jr., and Long, S. P. (2001). Improved temperature response functions for models of Rubisco-limited photosynthesis. Plant Cell Environ. 24, 253–259. doi: 10.1111/j.1365-3040.2001.00668.x
Brito, F. A. L., Pimenta, T. M., Henschel, J. M., Martins, S. C. V., Zsogon, A., and Ribeiro, D. M. (2020). Elevated CO2 improves assimilation rate and growth of tomato plants under progressively higher soil salinity by decreasing abscisic acid and ethylene levels. Environ. Exp. Bot. 176:104050. doi: 10.1016/j.envexpbot.2020.104050
Bunce, J. A. (2000). Acclimation of photosynthesis to temperature in eight cool and warm climate herbaceous C3 species: temperature dependence of parameters of a biochemical photosynthesis model. Photosynth. Res. 63, 59–67. doi: 10.1023/A:1006325724086
Cai, C., Yin, X., He, S., Jiang, W., Si, C., Struik, P. C., et al. (2016). Responses of wheat and rice to factorial combinations of ambient and elevated CO2 and temperature in FACE experiments. Glob. Chang. Biol. 22, 856–874. doi: 10.1111/gcb.13065
Chen, G., Yong, Z., Liao, Y., Zhang, D., Chen, Y., Zhang, H., et al. (2005). Photosynthetic acclimation in rice leaves to free-air CO2 enrichment related to both ribulose-1,5-bisphosphate carboxylation limitation and ribulose-1,5-bisphosphate regeneration limitation. Plant Cell Physiol. 46, 1036–1045. doi: 10.1093/pcp/pci113
Counce, P. A., Keisling, T. C., and Mitchell, A. J. (2000). A uniform, objective, and adaptive system for expressing rice development. Crop Sci. 40, 436–443. doi: 10.2135/cropsci2000.402436x
Demmig-Adams, B., and Adams, W. W. (1992). Photoprotection and other responses of plants to high light stress. Ann. Rev. Plant Physiol. Plant Mol. Biol. 43, 599–626. doi: 10.1146/annurev.pp.43.060192.003123
Dusenge, M. E., Duarte, A. G., and Way, D. A. (2019). Plant carbon metabolism and climate change: elevated CO2 and temperature impacts on photosynthesis, photorespiration and respiration. New Phytol. 221, 32–49. doi: 10.1111/nph.15283
Ellsworth, D. S., Reich, P. B., Naumburg, E. S., Koch, G. W., Kubiske, M. E., and Smith, S. D. (2004). Photosynthesis, carboxylation and leaf nitrogen responses of 16 species to elevated pCO2 across four free-air CO2 enrichment experiments in forest, grassland and desert. Glob. Chang. Biol. 10, 2121–2138. doi: 10.1111/j.1365-2486.2004.00867.x
Farquhar, G. D., and Sharkey, T. D. (1982). Stomatal conductance and photosynthesis. Ann. Rev. Plant Physiol. 33, 317–345. doi: 10.1146/annurev.pp.33.060182.001533
Farquhar, G. D., von Caemmerer, S., and Berry, J. A. (1980). A biochemical model of photosynthetic CO2 assimilation in leaves of C3 species. Planta 149, 78–90. doi: 10.1007/BF00386231
IPCC. (2014). “Climate change 2014: synthesis report,” in Contribution of Working Groups I, II and III to the Fifth Assessment Report of the Intergovernmental Panel on Climate Change [Core Writing Team, eds R. K. Pachauri and L. A. Meyer (Geneva: IPCC), 151.
IRRI. (2013). Standard Evaluation System (SES) for Rice. Philippines: International Rice Research Institute, P.O.Box 933, 1099 Manila. ∗.
Koyama, K., and Takemoto, S. (2014). Morning reduction of photosynthetic capacity before midday depression. Sci. Rep. 4:4389. doi: 10.1038/srep04389
Laisk, A., Eichelmann, H., Oja, V., Rasulov, B., Padu, E., Bichele, I., et al. (2005). Adjustment of leaf photosynthesis to shade in a natural canopy: rate parameters. Plant Cell Environ. 28, 375–388. doi: 10.1111/j.1365-3040.2004.01274.x
Lamba, S., Hall, M., Räntfors, M., Chaudhary, N., Linder, S., Way, D., et al. (2018). Physiological acclimation dampens initial effects of elevated temperature and atmospheric CO2 concentration in mature boreal Norway spruce. Plant Cell Environ. 41, 300–313. doi: 10.1111/pce.13079
Leakey, A. D. B., Ainsworth, E. A., Bernacchi, C. J., Rogers, A., Long, S. P., and Ort, D. R. (2009). Elevated CO2 effects on plant carbon, nitrogen, and water relations: six important lessons from FACE. J. Exp. Bot. 60, 2859–2876. doi: 10.1093/jxb/erp096
Leakey, A. D. B., Bernacchi, C. J., Ort, D. R., and Long, S. P. (2006). Long-term growth of soybean at elevated [CO2] does not cause acclimation of stomatal conductance under fully open-air conditions. Plant Cell Environ. 29, 1794–1800. doi: 10.1111/j.1365-3040.2006.01556.x
Lin, Y. S., Medlyn, B. E., Duursma, R. A., Prentice, I. C., Atkin, O. K., Barton, C. V. M., et al. (2015). Optimal stomatal behaviour around the world. Nat. Clim. Chang. 5, 459–464. doi: 10.1038/nclimate2550
Liu, B. B., Li, M., Li, Q. M., Cui, Q. Q., Zhang, W. D., Ai, X. Z., et al. (2018). Combined effects of elevated CO2 concentration and drought stress on photosynthetic performance and leaf structure of cucumber (Cucumis sativus L.) seedlings. Photosynthetica 56, 942–952. doi: 10.1007/s11099-017-0753-9
Liu, J., Huang, L., Liao, X., Tong, B., and Li, L. (2020). Relationship between diurnal changes of photosynthetic characteristics and environmental factors of Drepanostachyum luodianense in different seasons (in Chinese). J. Anhui Agric. Univ. 47, 62–69. doi: 10.13610/j.cnki.1672-352x.20200327.006
Markelz, R. J. C., Strellner, R. S., and Leakey, A. D. B. (2011). Impairment of C4 photosynthesis by drought is exacerbated by limiting nitrogen and ameliorated by elevated [CO2] in maize. J. Exp. Bot. 62, 3235–3246. doi: 10.1093/jxb/err056
Medlyn, B. E., Barton, C. V. M., Broadmeadow, M. S. J., Ceulemans, R., Angelis, P. D., Forstreuter, M., et al. (2001). Stomatal conductance of forest species after long-term exposure to elevated CO2 concentration: a synthesis. New Phytol. 149, 247–264. doi: 10.1046/j.1469-8137.2001.00028.x
Medlyn, B. E., Dreyer, E., Ellsworth, D., Forstreuter, M., Harley, P. C., Kirschbaum, M. U. F., et al. (2002a). Temperature response of parameters of a biochemically based model of photosynthesis. II. a review of experimental data. Plant Cell Environ. 25, 1167–1179. doi: 10.1046/j.1365-3040.2002.00891.x
Medlyn, B. E., Duursma, R. A., Eamus, D., Ellsworth, D. S., Prentice, I. C., Barton, C. V. M., et al. (2012). Reconciling the optimal and empirical approaches to modelling stomatal conductance. Glob. Chang. Biol. 18, 3476–3476. doi: 10.1111/j.1365-2486.2012.02790.x
Medlyn, B. E., Loustau, D., and Delzon, S. (2002b). Temperature response of parameters of a biochemically based model of photosynthesis. I. seasonal changes in mature maritime pine (Pinus pinaster Ait.). Plant Cell Environ. 25, 1155–1165. doi: 10.1046/j.1365-3040.2002.00890.x
Mohotti, A. J., and Lawlor, D. W. (2002). Diurnal variation of photosynthesis and photoinhibition in tea: effects of irradiance and nitrogen supply during growth in the field. J. Exp. Bot. 53, 313–322. doi: 10.1093/jexbot/53.367.313
Naumann, J. C., Bissett, S. N., Young, D. R., Edwards, J., and Anderson, J. E. (2010). Diurnal patterns of photosynthesis, chlorophyll fluorescence, and PRI to evaluate water stress in the invasive species, Elaeagnus umbellata Thunb. Trees 24, 237–245. doi: 10.1007/s00468-009-0394-0
Nijs, I., Impens, I., and Hecke, P. V. (1992). Diurnal changes in the response of canopy photosynthetic rate to elevated CO2 in a coupled temperature-light environment. Photosynth. Res. 32, 121–130. doi: 10.1007/BF00035946
NOAA (2019). Carbon Dioxide Levels Hit Record Peak in May. Available online at: https://research.noaa.gov/article/ArtMID/587/ArticleID/2461(accesseed June 4, 2019).
Panda, D. (2011). Diurnal variations in gas exchange and chlorophyll fluorescence in rice leaves: the cause for midday depression in CO2 photosynthetic rate. J. Stress Physiol. Biochem. 7, 175–186.
Pathare, V. S., Crous, K. Y., Cooke, J., Creek, D., Ghannoum, O., and Ellsworth, D. S. (2017). Water availability affects seasonal CO2-induced photosynthetic enhancement in herbaceous species in a periodically dry woodland. Glob. Chang. Biol. 23, 5164–5178. doi: 10.1111/gcb.13778
Peng, Y., Li, C., and Fritschi, F. B. (2014). Diurnal dynamics of maize leaf photosynthesis and carbohydrate concentrations in response to differential N availability. Environ. Exp. Bot. 99, 18–27. doi: 10.1016/j.envexpbot.2013.10.013
Rho, H., Doty, S. L., and Kim, S. (2020). Endophytes alleviate the elevated CO2-dependent decrease in photosynthesis in rice, particularly under nitrogen limitation. J. Exp. Bot. 71, 707–718. doi: 10.1093/jxb/erz440
Rogers, A., Medlyn, B. E., Dukes, J. S., Bonan, G., von Caemmerer, S., Dietze, M. C., et al. (2017). A roadmap for improving the representation of photosynthesis in Earth system models. New Phytol. 213, 22–42. doi: 10.1111/nph.14283
Ruiz-Vera, U. M., De Souza, A. P., Long, S. P., and Ort, D. R. (2017). The role of sink strength and nitrogen availability in the down-regulation of photosynthetic capacity in field-grown Nicotiana tabacum L. at elevated CO2 concentration. Front. Plant Sci. 8:998. doi: 10.3389/fpls.2017.00998
Shimono, H., and Bunce, J. A. (2009). Acclimation of nitrogen uptake capacity of rice to elevated atmospheric CO2 concentration. Ann. Bot. 103, 87–94. doi: 10.1093/aob/mcn209
Shimono, H., Okada, M., Inoue, M., Nakamura, H., Kobayashi, K., and Hasegawa, T. (2010). Diurnal and seasonal variations in stomatal conductance of rice at elevated atmospheric CO2 under fully open-air conditions. Plant Cell Environ. 33, 322–331. doi: 10.1111/j.1365-3040.2009.02057.x
Sims, D. A., Luo, Y., and Seemann, J. R. (1998a). Comparison of photosynthetic acclimation to elevated CO2 and limited nitrogen supply in soybean. Plant Cell Environ. 21, 945–952. doi: 10.1046/j.1365-3040.1998.00334.x
Sims, D. A., Seemann, J. R., and Luo, Y. (1998b). The significance of differences in the mechanisms of photosynthetic acclimation to light, nitrogen and CO2 for return on investment in leaves. Funct. Ecol. 12, 185–194. doi: 10.1046/j.1365-2435.1998.00194.x
Singsaas, E. L., Ort, D. R., and Delucia, E. H. (2003). Elevated CO2 effects on mesophyll conductance and its consequences for interpreting photosynthetic physiology. Plant Cell Environ. 27, 41–50. doi: 10.1046/j.0016-8025.2003.01123.x
Špunda, V., Kalina, J., Urban, O., Luis, V. C., Sibisse, I., Puértolas, J., et al. (2005). Diurnal dynamics of photosynthetic parameters of Norway spruce trees cultivated under ambient and elevated CO2: the reasons of midday depression in CO2: the reasons of midday depression in CO2 assimilation. Plant Sci. 168, 1371–1381. doi: 10.1016/j.plantsci.2005.02.002
Tao, W., and Wang, D. (2021). Effects of increased CO2 concentration on the photosynthetic physiology and root growth of indica and japonica rice. J. Nanjing Agric. Univ. 44, 27–35. doi: 10.7685/jnau.202003041
Tausz-Posch, S., Dempsey, R. W., Seneweera, S., Norton, R. M., Fitzgerald, G., and Tausz, M. (2015). Does a freely tillering wheat cultivar benefit more from elevated CO2 than a restricted tillering cultivar in a water-limited environment? Eur. J. Agron. 64, 21–28. doi: 10.1016/j.eja.2014.12.009
Tausz-Posch, S., Norton, R. M., Seneweera, S., Fitzgerald, G. J., and Tausz, M. (2013). Will intra-specific differences in transpiration efficiency in wheat be maintained in a high CO2 world? A face study. Physiol. Plant. 148, 232–245. doi: 10.1111/j.1399-3054.2012.01701.x
Thilakarathne, C. L., Tausz-Posch, S., Cane, K., Norton, R. M., Fitzgerald, G. J., Tausz, M., et al. (2015). Intraspecific variation in leaf growth of wheat (Triticum aestivum) under Australian grain free air CO2 enrichment (AGFACE): is it regulated through carbon and/or nitrogen supply? Funct. Plant Biol. 42, 299–308. doi: 10.1071/FP14125
Torralbo, F., González-Moro, M. B., Baroja-Fernández, E., Aranjuelo, I., and González-Murua, C. (2019). Differential regulation of stomatal conductance as a strategy to cope with ammonium fertilizer under ambient versus elevated CO2. Front. Plant Sci. 10:597. doi: 10.3389/fpls.2019.00597
Van Oosten, J. J., and Besford, R. T. (1996). Acclimation of photosynthesis to elevated CO2 through feedback regulation of gene expression: climate of opinion. Photosynth. Res. 48, 353–365. doi: 10.1007/BF00029468
Vu, J. C. V. Jr., Allen, L. H., Boote, K. J., and Bowes, G. (1997). Effects of elevated CO2 and temperature on photosynthesis and Rubisco in rice and soybean. Plant Cell Environ. 20, 68–76. doi: 10.1046/j.1365-3040.1997.d01-10.x
Wand, S. J. E., Midgley, G. F., Jones, M. H., and Curtis, P. S. (1999). Responses of wild C4 and C3 grass (Poaceae) species to elevated atmospheric CO2 concentration: a meta-analytic test of current theories and perceptions. Glob. Chang. Biol. 5, 723–741. doi: 10.1046/j.1365-2486.1999.00265.x
Wang, D., Heckathorn, S. A., Wang, X., and Philpott, S. M. (2012). A meta-analysis of plant physiological and growth responses to temperature and elevated CO2. Oecologia 169, 1–13. doi: 10.1007/s00442-011-2172-0
Wang, D., Ling, T., Wang, P., Jing, P., Fan, J., Wang, H., et al. (2018). Effects of 8-year nitrogen and phosphorus treatments on the ecophysiological traits of two key species on tibetan plateau. Front. Plant Sci. 9:1290. doi: 10.3389/fpls.2018.01290
Wang, W., Cai, C., Lam, S. K., Liu, G., and Zhu, J. (2018). Elevated CO2 cannot compensate for japonica grain yield losses under increasing air temperature because of the decrease in spikelet density. Eur. J. Agron. 99, 21–29. doi: 10.1016/j.eja.2018.06.005
Wang, W., He, J., Wang, Z., Gu, J., Liu, L., Zhang, W., et al. (2021). Leaf characteristics of rice cultivars with a stronger yield response to projected increases in CO2 concentration. Physiol. Plant. 171, 416–423. doi: 10.1111/ppl.13246
Wen, X., Jiang, D., Lu, Q., and Rao, L. (1998). Major Factors on diurnal changes of photosynthetic rate. Chin. J. Rice Sci. 12, 105–108. doi: 10.16819/j.1001-7216.1998.02.007
Wolz, K. J., Wertin, T. M., Abordo, M., Wang, D., and Leakey, A. D. B. (2017). Diversity in stomatal function is integral to modelling plant carbon and water fluxes. Nat. Ecol. Evol. 1, 1292–1298. doi: 10.1038/s41559-017-0238-z
Wullschleger, S. D. (1993). Biochemical limitations to carbon assimilation in C3 plants—a retrospective analysis of the A/Ci curves from 109 species. J. Exp. Bot. 44, 907–920. doi: 10.1093/jxb/44.5.907
Keywords: global change, photosynthesis, stomatal conductance, chlorophyll a fluorescence, photosynthesis model, stomatal conductance model
Citation: Miao Y, Cai Y, Wu H and Wang D (2021) Diurnal and Seasonal Variations in the Photosynthetic Characteristics and the Gas Exchange Simulations of Two Rice Cultivars Grown at Ambient and Elevated CO2. Front. Plant Sci. 12:651606. doi: 10.3389/fpls.2021.651606
Received: 10 January 2021; Accepted: 12 March 2021;
Published: 06 April 2021.
Edited by:
Fabricio Eulalio Leite Carvalho, Corporación Colombiana de Investigación Agropecuaria (Agrosavia) – CI La Suiza, ColombiaReviewed by:
James Bunce, Beltsville Agricultural Research Center, Agricultural Research Service (USDA), United StatesAna Karla M. Lobo, São Paulo State University, Brazil
Copyright © 2021 Miao, Cai, Wu and Wang. This is an open-access article distributed under the terms of the Creative Commons Attribution License (CC BY). The use, distribution or reproduction in other forums is permitted, provided the original author(s) and the copyright owner(s) are credited and that the original publication in this journal is cited, in accordance with accepted academic practice. No use, distribution or reproduction is permitted which does not comply with these terms.
*Correspondence: Dan Wang, d2FuZ2RhbkBudWlzdC5lZHUuY24=