- 1College of Horticulture Science and Engineering, Shandong Agricultural University, Tai’an, China
- 2Collaborative Innovation Center of Fruit & Vegetable Quality and Efficient Production in Shandong, Tai’an, China
- 3Key Laboratory of Biology and Genetic Improvement of Horticultural Crops in Huanghuai Region, Ministry of Agriculture and Rural Affairs, Tai’an, China
- 4State Key Laboratory of Crop Biology, Tai’an, China
The active regulation of the plant growth environment is a common method for optimizing plant yield and quality. In horticulture today, light quality control is carried out using photo-selective nets or membranes to improve the yield and quality of cultivated plants. In the present study, with natural light as the control (CK), we tested different photo-selective nets (white, WN; blue, BN; green, GN; yellow, YN; and red, RN) with 30% shade for characteristics of growth, development, quality, yield, photosynthesis, and chlorophyll fluorescence, considering the antioxidant system, as well as the influence of element absorption and transformation of green onion (Allium fistulosum L.) plants at different growth stages. We found that plants under BN and WN have greater height and fresh weight than those of plants under the other nets. Plants under the BN treatment had the highest quality, yield, photosynthetic pigment content, net photosynthetic rate, transpiration rate, and stomatal conductance, whereas the intercellular CO2 concentration was the highest in plants in the YN treatment. The photosynthesis noon break phenomenon was significantly lower in plants with covered photo-selective nets than in CK plants. NPQ was the highest in the YN treatment, and Fv/Fm, ΦPSII, and qP among the plants in the other treatments were different; from highest to lowest, they were as follows: BN > WN > CK > RN > GN > YN. The active oxygen content of green onion leaves in the BN treatment was significantly lower than that in the other treatments, and their key enzyme activity was significantly increased. BN also improved the absorption and transformation of elements in various organs of green onion.
Introduction
Light is an indispensable environmental factor in the life cycle of plants and is the main factor driving plant photosynthesis (Das, 2004). Irradiation quality, quantity, and photoperiod can regulate plant growth and development, trigger physiological and morphological reactions, and affect their secondary metabolism (Kurepin et al., 2007; Jackson, 2009; Wang et al., 2018a; Yangen et al., 2019). Under appropriate light conditions, plants can increase their CO2 fixation capacity and net photosynthetic rate by increasing the absorption of light energy and electron transfer rate (Yang et al., 2018). However, excessive light inhibits photosynthesis and can even lead to the photooxidation of photosynthetic organs (Wang et al., 2018b). The response of different species to light varies greatly (Lusk and Pozo, 2002).
The effects of global warming have already begun to have a significant impact on our environment, including an increase in atmospheric CO2 concentration, which may change the internal quality of vegetable products or lead to a decrease in photosynthesis rate (Bisbis et al., 2018). Among others, the challenges brought upon by global warming include an increase in air temperature (AT) and solar radiation intensity (Hu et al., 2018). For this reason, active manipulation of the plant growth environment is often used to optimize plant yield and quality (Dueck et al., 2016). With the adjustment of industrial structure and the continuous development of facility agriculture, photo-selective nets or films have been increasingly used in crop greenhouse technology to control the quality and quantity of ambient light to improve the yield, quality, and phytochemical composition of cultivated plants and protect them against pests and physical damage (Nissim-Levi et al., 2014; Selahle et al., 2014; Carvalho et al., 2016; Abbasnia Zare et al., 2019).
Photo-selective nets are a covering net material developed by the Israeli Agricultural Research Organization, Volcani Center, and Polysack Plastics Industries in Israel in the 1990s (Shahak et al., 2004). Compared with ordinary nets, certain pigments or light scattering and reflection aids are added to photo-selective nets in the production process, which is why the light quality changes after passing through the net. These nets can change the spectral composition of light, increase the proportion of scattered light (Shahak, 2008), and change the quality and quantity of light, as well as affect the airflow, temperature, and humidity.
Numerous studies have shown that different colors of photo-selective nets have different effects on plant growth, as well as that different plants have distinct responses to photo-selective nets. Compared to the use of black nets, the use of red nets led to an increase in the production of sweet peppers (Shahak, 2008; Darázsi, 2014). Furthermore, the pearl color net increased the number of branches of the pot plant Myrtus communis (Ada et al., 2015). However, research on Pittosporum tobira showed that the number of branches on stems increased most obviously under red nets, followed by gray nets, whereas it increased the least under black and blue nets (Stamps and Chandler, 2008). Experiments on basil plants showed that after covering the plants with red and blue nets (shading level 50%) for 90–120 days, leaf thickness and stomata density decreased compared to those in plants grown in the open field (Costa et al., 2010). Compared with red and black nets, blue nets increased the biomass of Phalaenopsis leaves and roots (Leite et al., 2008).
Green onion (Allium fistulosum L.) is often consumed as a vegetable or spice condiment and has high nutritional value (Gao et al., 2021). As the most important seasoned vegetable in Asia during summer, its seedlings are easily affected by high light. If the light is too strong, the leaf fiber increases, and the leaf body ages, which reduces its edible value and quality. Therefore, it is necessary to assess the growth of green onion during midsummer. In our experiment, through studying photosynthesis, chlorophyll fluorescence characteristics, resistance, quality, and yield of green onion leaves under photo-selective net conditions, we gained an in-depth understanding of the photosynthesis reaction mechanism of green onion under different spectral conditions and shades, which provides a theoretical basis for the cultivation of green onion in summer.
Materials and Methods
Plant Material and Sample Preparation
The experiment was conducted in the experimental field of Shandong Agricultural University (SDAU, N361.8°, E117.1°), Tai’an City, China, in 2019 based on a preliminary test in 2018. Green onion (Allium fistulosum L.) variety “Yuanzang” was originally obtained from the Tai’an Taishan Seed Industry Technology Co., Ltd. The seeds were sown on March 20, and the seedlings were about 40 cm high on June 25. They were moved to a planting ditch when they had four unfolded true leaves. The test soil was loamy with a pH of 6.72 and organic matter content of 11.09 g/kg.
In cultivation, row spacing was 80 cm, and plant spacing was 3.5–4 cm. A tent covering method was adopted for plant cultivation, that is, photo-selective nets (Fuzhou Kangsheng Knitting Textile Co., Ltd.) were placed directly on the support structure upper part of the green onion. The support structures were 2 m high, 10 m wide, and 20 m long.
The experiment involved a total of six treatments. Natural light was used as a control (CK), and the five treatments included the five colors of the photo-selective nets: white (WN), blue (BN), green (GN), yellow (YN), and red (RN; shading level 30 ± 3%). The nets were arranged randomly, and each experiment was repeated three times. The photosynthetic active radiation (PAR) of each photo-selective net was recorded with HOBO Data Loggers (MicroDAQ.com, Ltd., NH, United States) to calculate the shading level = PARNET/PARCK (Table 1; Figure 1A). The photo-selective nets were covered when the plants were being planted, and each treatment was managed in accordance with conventional production technology measures. Green onion plants were harvested on July 25, August 25, September 25, and October 25.
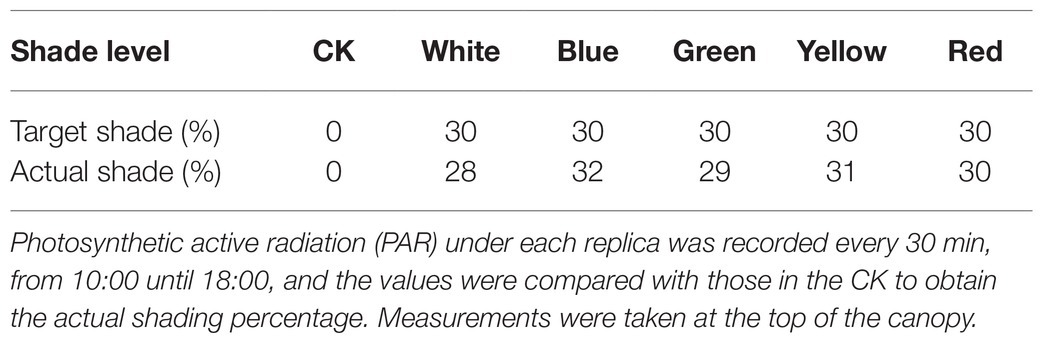
Table 1. Actual and target shade readings (%) for control (CK), white, blue, green, yellow, and red photo-selective netting.
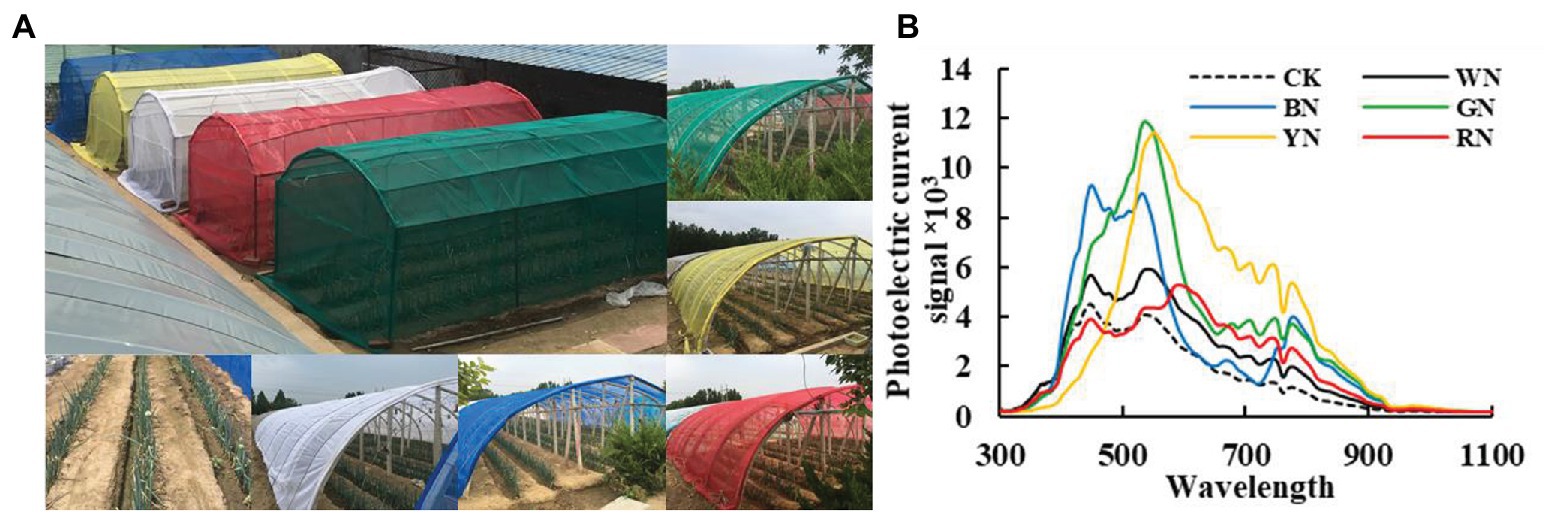
Figure 1. (A) Green onion cultivation under different photo-selective net conditions. (B) Spectral characteristics of each treatment. CK, control; BN, blue photo-selective nets; YN, yellow; WN, white; GN, green; and RN, red.
Spectral Irradiance Transmitted Through Photo-Selective Nets
In clear weather, the spectral characteristics of different photo-selective nets were measured using a UniSpec-SC spectrum analyzer (PP-SYSTEMS, United Kingdom), with a bandwidth of 300–1,100 nm and a 3.3 nm scanning interval. The spectral characteristics of each treatment are shown in Figure 1B.
Analytical Methods
Growth Parameter, Yield, and Quality Evaluation
Plant samples were taken at the seedling stage, pseudo-stem formation stage, pseudo-stem expansion stage, and harvest stage. Three plants were selected from each treatment, and the plant height, stem diameter, leaf, and pseudo-stem fresh weight (FW), and dry weight (DW) were determined. At harvest time, plants were weighted to determine yield per plant. Supplementary Text S1 describes in detail the determination method of soluble sugar, cellulose, soluble protein, free amino acid, and vitamin C (Vc) contents.
Photosynthetic Pigments and Gas Exchange System
Chlorophyll (Chl) and carotenoids (CAR) were extracted and quantified using the acetone extraction colorimetric method described in Gilmore and Yamamoto (1991). First, 0.2 g of the leaf was weighted into the test tube. Twenty milliliters of 80% acetone were added and extracted in the dark for 24 h until the leaves turned white. Then, UV spectrophotometers with wavelengths set at 663 nm, 646 nm, and 470 nm were used for colorimetry. Based on the method described in Gao et al. (2020), we used the Li-6,800 portable photosynthetic apparatus (Li-COR, United States) to measure the net photosynthetic rate (Pn), stomatal conductance (Gs), intercellular CO2 concentration (Ci), and transpiration rate (Tr) of the third functional leaf on July 25, August 25, September 25, and October 25, and the diurnal variation in photosynthetic parameters during the pseudo-stem formation stage. Throughout the experiment, leaves of the same ontogeny were evaluated for three plants, every 2 h from 7:00 to 17:00 h (Tucci et al., 2010).
Chlorophyll Fluorescence
Fluorescence parameters such as initial fluorescence (Fo), maximum fluorescence (Fm), initial fluorescence (Fo'), and maximum fluorescence (Fm') were measured using an FMS-2 portable fluorometer (Hansatech, United Kingdom). The maximum photochemical efficiency (Fv/Fm), actual photochemical efficiency (ΦPSII) = (Fm'-Fs)/Fm', photochemical quenching coefficient (qP) = (Fm'-Ft)/(Fm'-Fo'), and non-photochemical quenching coefficient (NPQ) = 1-(Fm'-Fo')/(Fm-Fo) were calculated as well, the light intensity used to calculate the yield of NPQ and ΦPSII was 1,200 μmol·m−2·s−1 (Genty et al., 1989; Roháček, 2002).
Antioxidant System
The third functional leaves of green onion plants were selected for the assessment of the antioxidant system, including antioxidant substances and antioxidant-related enzyme activities. Malondialdehyde (MDA) content is an index used for evaluating oxidative damage and is determined by the thiobarbituric acid method (Wada et al., 2011). We used nitro blue tetrazolium (NBT) to detect the accumulation of superoxide (O2−; Xia et al., 2009). The H2O2 content was determined according to Patterson et al. (1984). The assay was based on the absorbance change of the titanium peroxide complex at 415 nm. The enzyme solution was extracted according to the method described in Gong et al. (2014). The antioxidant system was evaluated by measuring the activity of superoxide dismutase (SOD), peroxidase (POD), and catalase (CAT; Roldán et al., 2008; Chapman et al., 2019).
Element Contents
During the harvest period, the roots, stems, and leaves of green onion plants were washed three times with deionized water, dried at 105°C for 15 min, and then dried to a constant weight at 70°C. From each sample, 0.2 g were ground and digested with H2SO4-H2O2, and a discrete auto-analyzer (Smartchem200, Alliance, France) was used to determine N and P contents. An inductively coupled plasma optical emission spectrometer (ICP-OES; iCAP-7,400, Thermo Scientific, United States) was used to determine the potassium content in the solution.
Data Analysis
In the present study, all plants were randomly sampled. The data were processed, plotted, and statistically analyzed in Excel 2016 and DPS software package (DPS for Windows, 2009). The differences among treatments were tested using Duncan’s new multiple range test at a significance level of p ≤ 0.05.
Results
The Shading Level and Transmission Spectrum Characteristics of Different Photo-Selective Nets
Figure 1A shows the cultivation of green onion under different photo-selective net conditions. We used PAR measurements to calculate the shadow transmittance (vs. non-netted controls). We found that compared with CK, the actual shading level of WN, BN, GN, YN, and RN were 28, 32, 29, 31, and 30%, respectively (Table 1). As shown in Figure 1B, the transmission spectra of each colored photo-selective net were different. The proportion of blue and green light in BN was significantly higher than in other treatments, the proportion of green and yellow light in GN was significantly higher than other treatments, especially green light, and the proportion of green, yellow, and red light in YN was significantly increased, and the proportion of red light in RN increased.
Effects of Photo-Selective Nets on the Growth of Green Onion
Different light qualities and light intensities had different effects on the growth of plants at different growth stages. Table 2 shows the influence of different treatments on the growth of plants in different growth stages. There was no significant difference in plant growth among the treatments at the seedling stage. However, at the pseudo-stem formation stage, with the increase in plant height and stem diameter, the differences among the different treatments became significant. The plant height in BN and WN treatments increased by 9.74 and 3.38%, respectively, whereas in RN, GN, and YN treatments, it decreased by 2.34, 4.25, and 7.21%, respectively, compared with that in CK. The stem diameter in BN and WN treatments increased by 8.56 and 4.10%, respectively, whereas in RN, GN, and YN, it decreased by 5.96, 10.10, and 14.31%, respectively, compared with that in CK. The leaf fresh weight in BN and WN treatments increased by 6.71 and 1.57%, respectively, whereas in RN, GN, and YN, it decreased by 4.18, 5.41, and 10.87%, respectively, compared with that in CK.
At the pseudo-stem expansion stage, the pseudo-stem fresh weight varied significantly among the treatments. In BN and WN, it increased by 15.05 and 7.43%, respectively, compared with that in CK. Therefore, BN and WN treatments promoted the growth of green onion. At the harvest stage, the final aboveground fresh weight of plants in BN and WN treatments increased by 14.18 and 3.42%, respectively, whereas that in RN, GN, and YN treatments decreased by 5.21, 12.32, and 17.69%, respectively, compared with that in CK (Table 2). By measuring the height of the green onion plant, stem diameter, and the fresh weight of the plant, we found that from the best to the worst, the growth status of plants in the five treatments was BN > WN > RN > GN > YN (Figure 2).
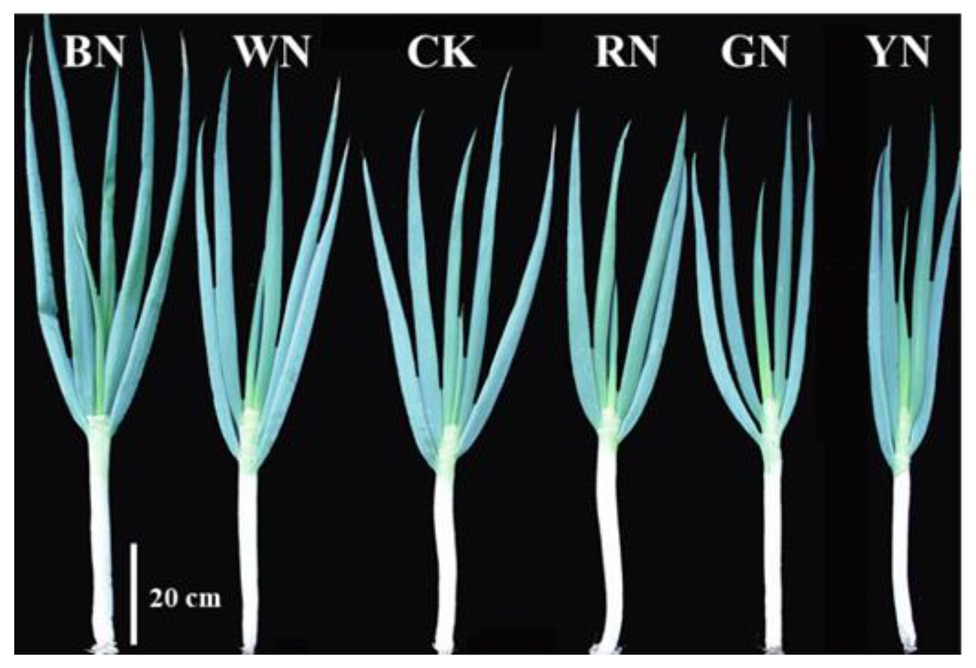
Figure 2. The morphology of green onion plants under different photo-selective net treatments at the harvest stage. Scale bar = 20 cm. BN, blue photo-selective nets; WN, white; CK, control; RN, red; GN, green; and YN, yellow.
Effects of Photo-Selective Nets on the Yield and Quality of Green Onion
As shown in Table 3, we calculated the yield per 1000 m2 under different treatments, and the results showed that there was a significant difference in the yield of green onion among different treatments. Compared with the yield of CK plants, the yield of plants in BN and WN treatments increased by 14.54 and 3.77%, respectively, whereas the yields of plants in RN, GN, and YN treatments decreased by 5.47, 12.39, and 16.47%, respectively. According to quality evaluation, there was no significant difference in soluble sugar and crude cellulose content among the plants in different treatments. The contents of dry matter, soluble proteins, free amino acids, and Vc from highest to lowest were BN > WN > CK > RN > GN > YN. The dry matter, soluble protein, free amino acids, and Vc contents in plants in the BN treatment increased by 9.20, 24.83, 23.91, and 30.08%, respectively, compared with those in CK plants (Table 4).
Effects of Photo-Selective Nets on Photosynthetic Pigments and Gas Exchange System of Green Onion
The chlorophyll and carotenoid contents of plants in BN and WN treatments were significantly higher than those of CK plants at different growth stages, whereas, in plants in RN, GN, and YN treatments, these contents were significantly lower than those in CK plants (Figure 3). During the experiment, the chlorophyll and carotenoid contents in green onion leaves increased at first and then decreased. The highest chlorophyll content was recorded on September 25th; compared to the chlorophyll content in CK plants, the plants in BN and WN treatments had 18.87 and 10.38% higher chlorophyll content, whereas the plants in RN, GN, and YN treatments had 4.25, 12.74, and 20.75% lower chlorophyll content, respectively (Figure 3A). Therefore, both BN and WN treatments increased the pigment content in green onion leaves. The trend of change in carotenoid content was consistent with the trend of change in chlorophyll content (Figure 3B).
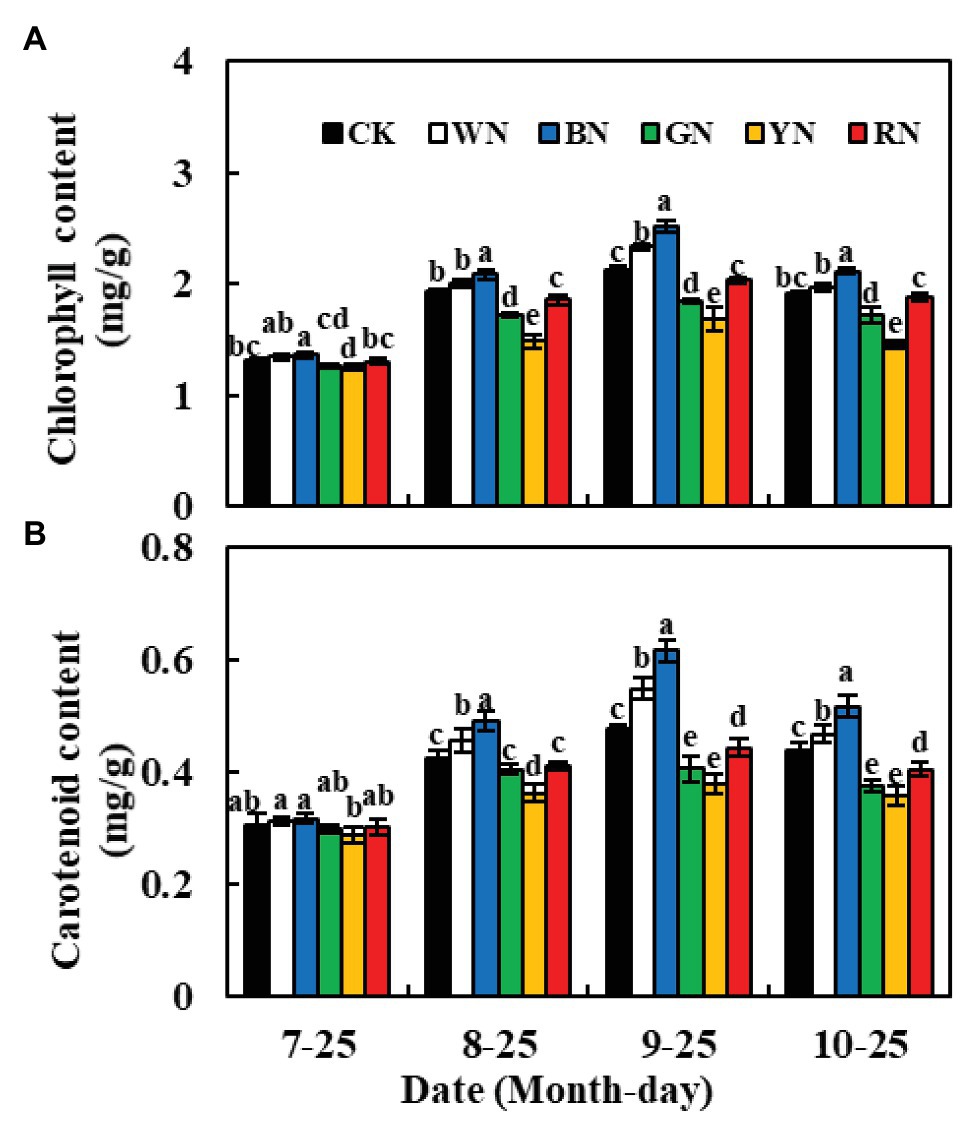
Figure 3. Effect of photo-selective nets on the photosynthetic pigment contents of green onion leaves at different growth stages. (A) Chlorophyll content, (B) carotenoid content. CK, control; WN, white photo-selective nets; BN, blue; GN, green; YN, yellow; and RN, red. Values are average ± SD, n = 18. Error bars indicate standard errors. Different letters (a, b, c, d, and e) in the same column indicate significant differences among treatments at p ≤ 0.05 according to Duncan’s new multiple range test.
Figure 4 shows the effect of photo-selective nets on the gas exchange parameters of green onion leaves at different growth stages. We found that both Tr and Gs showed a trend of increasing at first and then decreasing during the growth process. The differences in Tr and Gs among the treatments were significant at the pseudo-stem formation stage and reached the highest level at the pseudo-stem expansion stage, Tr and Gs were the highest in the BN treatment (Figures 4B,C). In the pseudo-stem expansion stage on September 25th, the Pn of plants in BN and WN treatments increased by 10.86 and 6.90%, respectively, compared with that in CK, whereas in plants in RN, GN, and YN treatments, it decreased by 4.91, 10.58, and 15.12%, respectively, compared with that in CK (Figure 4A). Ci had the opposite trend than Pn, Tr, and Gs. The Ci was the highest in YN and the lowest in BN, which indicated that BN consumed more CO2, thus promoting Pn (Figure 4D).
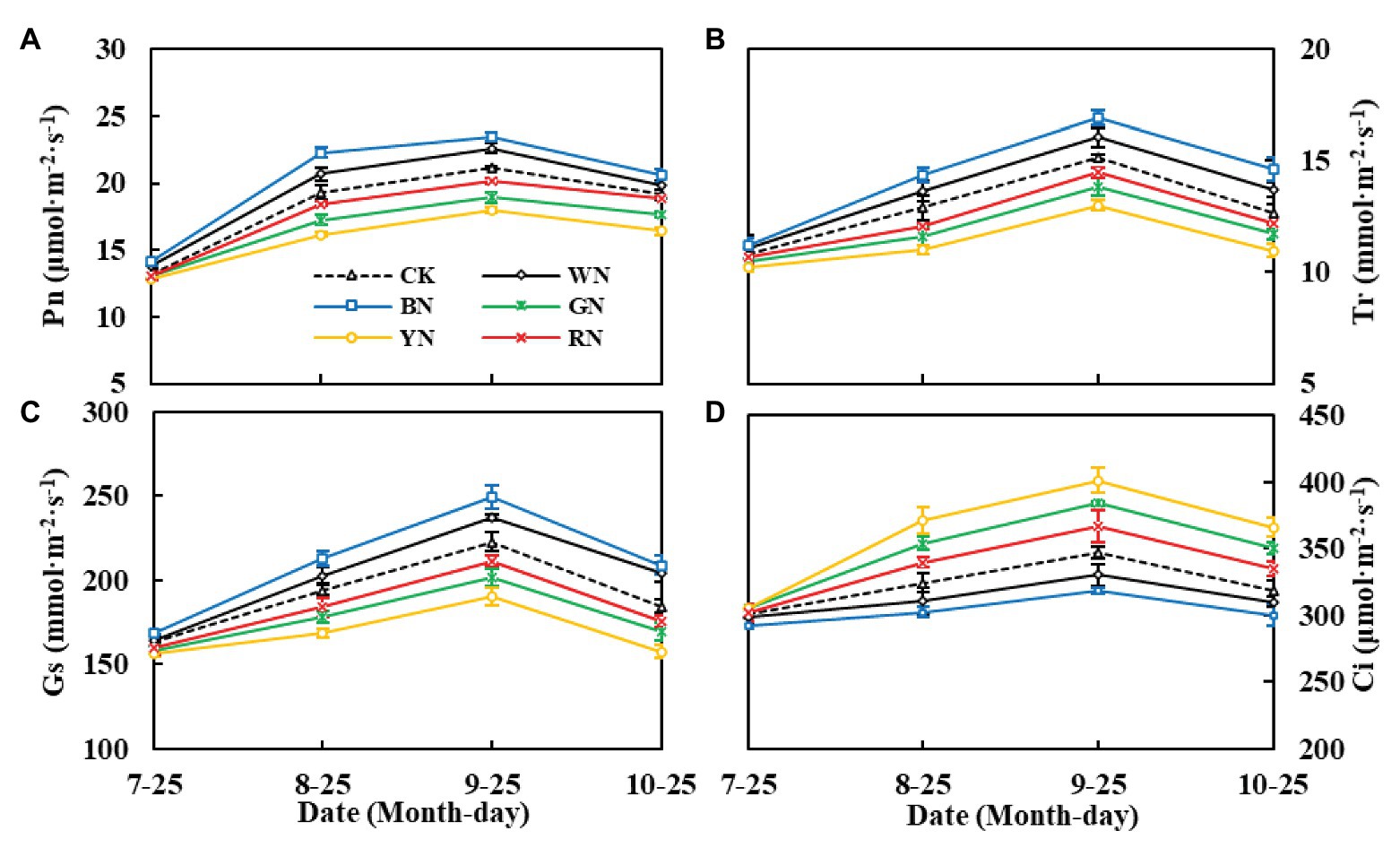
Figure 4. Effect of photo-selective nets on the photosynthetic parameters of green onion leaves at different growth stages. (A) Net photosynthetic rate (Pn), (B) transpiration rate (Tr), (C) stomatal conductance (Gs), (D) intercellular CO2 concentration (Ci). CK, control; WN, white photo-selective nets; BN, blue; GN, green; YN, yellow; and RN, red. Values are average ± SD, n = 18. Error bars indicate standard errors.
By analyzing the diurnal variation in photosynthesis in the green onion during the pseudo-stem formation stage (Figures 5A–D), we found that the differences among the treatments were consistent with the trend in the growth stage, with plants in the BN treatment being the highest in Pn, Tr, and Gs. During the course of 1 day, the Pn change trend was of the “M” type: the peak values were recorded at 11:00 and 15:00, the valley was recorded at 13:00, and the “photosynthesis noon break phenomenon” (Tucci et al., 2010) in CK plants at 13:00 was more severe than that in plants under the other treatments (Figure 5A). Compared with that in CK plants, the Pn of plants in BN, WN, and RN treatments increased by 28.60, 18.79, and 6.46%, respectively. Therefore, covering with photo-selective nets could enhance the hardiness of the green onion leaves, improve the leaf net photosynthetic rate, and reduce the occurrence of “photosynthesis noon break phenomenon.” The diurnal variation in Tr and Gs of green onion leaves in different treatments showed a single peak curve. There was a significant difference in Tr and Gs between the treatments at 11:00, and Tr peaked at 13:00 (Figure 5B). The diurnal variation in Gs with the trend of Pn reached the maximum at 11:00 and then declined (Figure 5C). The diurnal variation in Ci was characterized by the highest values in the morning, followed by those in the afternoon, and the lowest values at noon, which was essentially the opposite of Pn (Figure 5D).
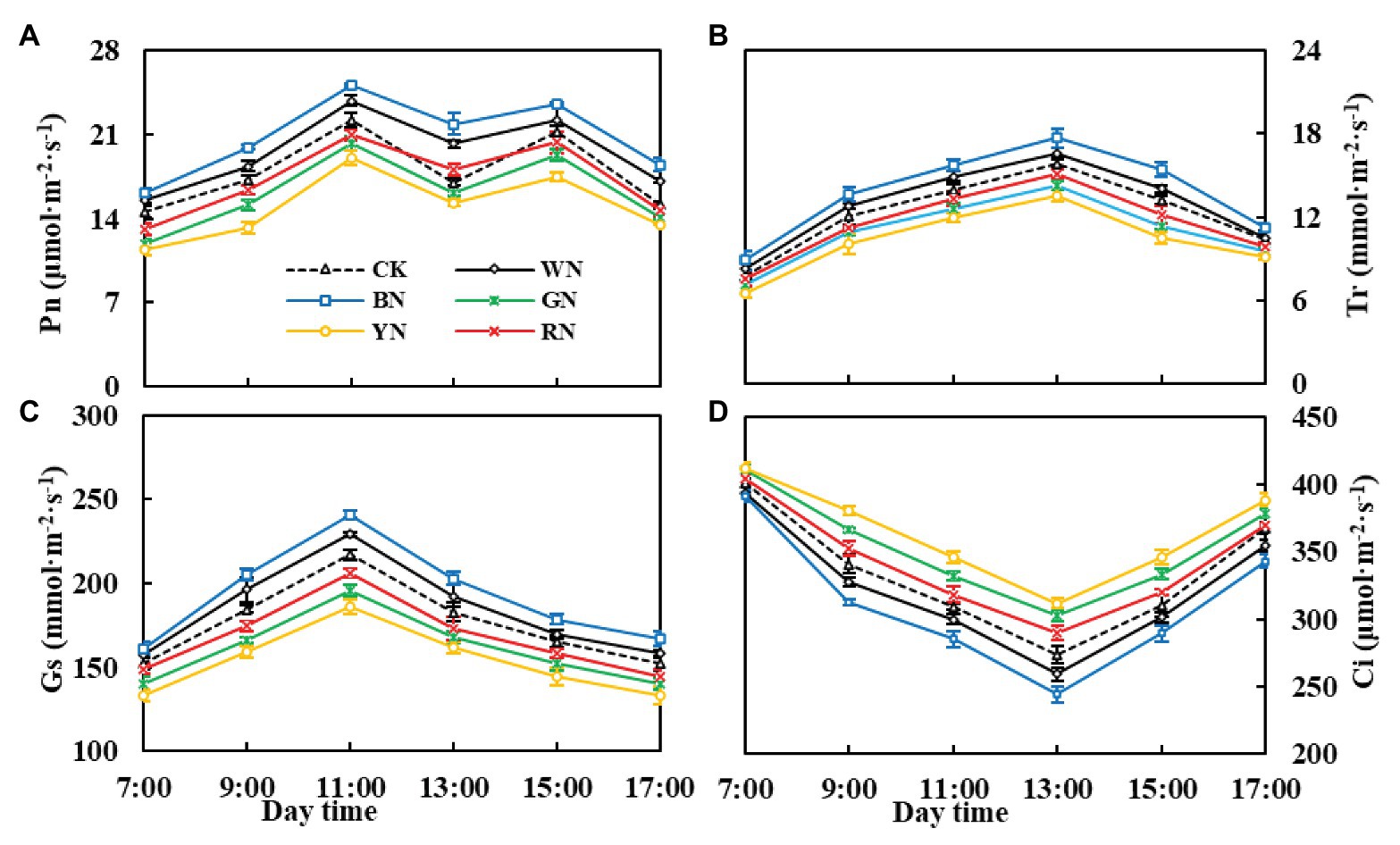
Figure 5. The effect of photo-selective nets on diurnal variation in photosynthetic parameters of green onion leaves during the pseudo-stem formation stage. (A) Net photosynthetic rate (Pn), (B) transpiration rate (Tr), (C) stomatal conductance (Gs), (D) intercellular CO2 concentration (Ci). CK, control; WN, white photo-selective nets; BN, blue; GN, green; YN, yellow; and RN, red. Values are average ± SD, n = 18. Error bars indicate standard errors.
Effects of Photo-Selective Nets on Chlorophyll Fluorescence of Green Onion
Furthermore, photo-selective nets significantly affected the chlorophyll fluorescence parameters of green onion leaves. From highest to lowest, the Fv/Fm, qP, and ФPSII of each treatment were BN > WN > CK > RN > GN > YN. Moreover, throughout the growth period, from the seedling stage to the pseudo-stem expansion stage, the parameters showed an upward trend, and the indicators from the pseudo-stem expansion stage to the harvest stage declined, whereas the trend of NPQ was the opposite (Figures 6A–D). In the pseudo-stem expansion stage, compared with that in CK plants, the Fv/Fm of plants in BN and WN treatments increased by 4.88 and 1.84%, whereas in RN, GN, and YN, it decreased by 2.33, 5.14, and 7.47%, respectively. The results showed that BN and WN improved the light energy conversion efficiency of the PSII reaction center and enhanced the actual light energy capture efficiency when this reaction center was partially closed, whereas YN and GN increased the heat dissipation of green onion plants.
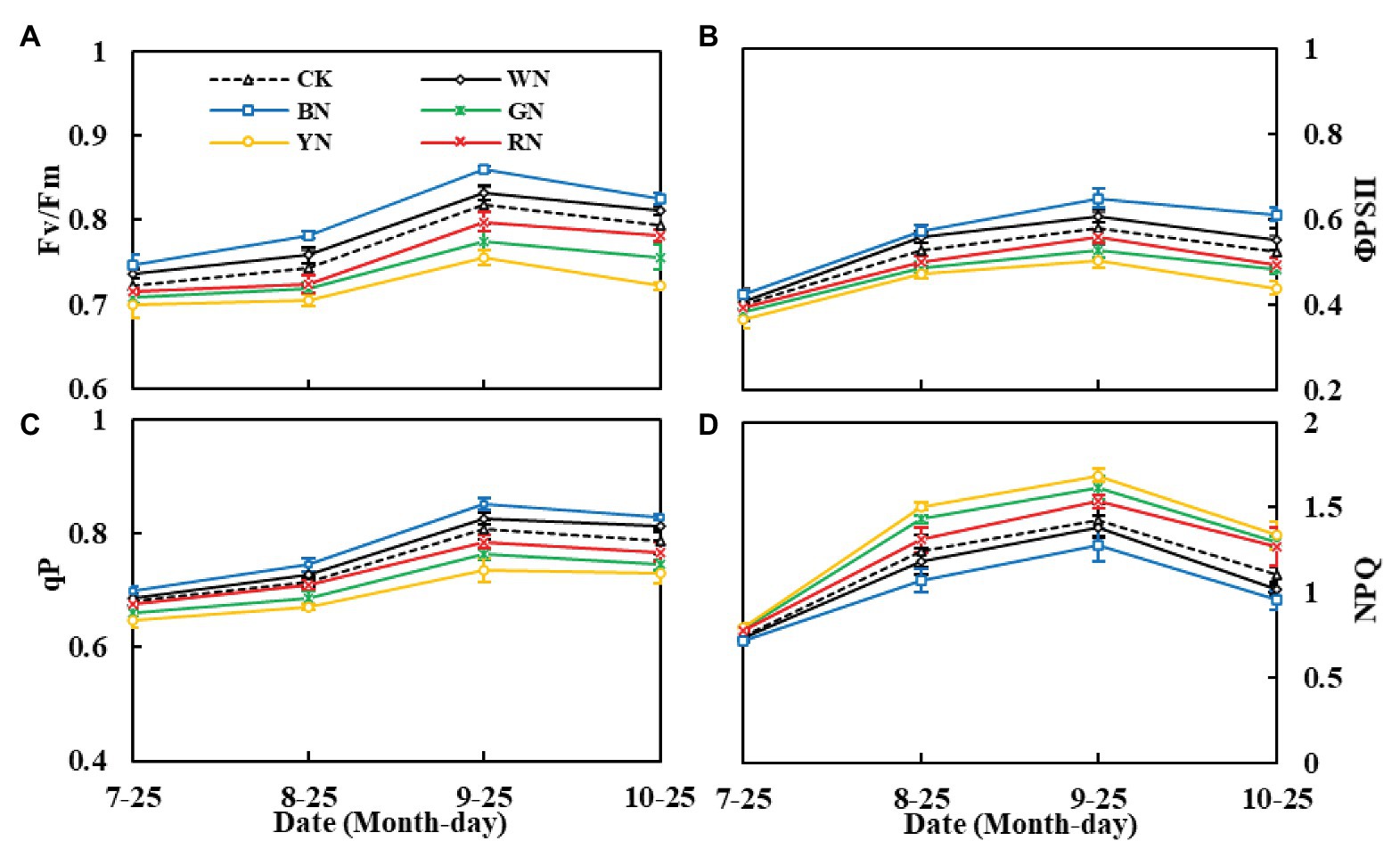
Figure 6. The effect of photo-selective nets on the fluorescence parameters of green onion leaves at different growth stages. (A) Maximum photochemical efficiency of PSII under dark adaptation (Fv/Fm), (B) actual photochemical efficiency (ΦPSII), (C) photochemical quenching coefficient (qP), (D) non-photochemical quenching coefficient (NPQ). CK, control; WN, white photo-selective nets; BN, blue; GN, green; YN, yellow; and RN, red. Values are average ± SD, n = 18. Error bars indicate standard errors.
Effects of Photo-Selective Nets on the Antioxidant System of Green Onion
As shown in Figure 7, the level of reactive oxygen species (ROS) in the leaves of plants under photo-selective nets continued to increase with the increase in plant growth. The levels of ROS in YN, GN, and RN treatments were significantly higher than those in the CK treatment (Figures 7A,B), the MDA content was consistent with the trend of ROS levels (Figure 7C). In plants, superoxide dismutase (SOD) is an important protective enzyme for removing ROS. It can effectively remove excess O2− and decrease its peroxidation effect on membrane lipids. In the present study, the activity of SOD in green onion leaves showed an upward trend followed by a downward trend during the experiment, with the highest activity on September 25. At that time, the SOD activity in the leaves of plants in BN and WN treatments was 13.34 and 4.85% higher than that in the leaves of CK plants, respectively, and in the leaves of plants in RN, GN, and YN treatments, it was 4.50, 10.90, and 18.46% lower than that in the leaves of CK plants, respectively. The trend of POD and CAT activity was similar to that of SOD activity (Figures 7D–F). BN and WN reduced the accumulation of ROS by increasing the activity of SOD, POD, and CAT to further decrease MDA levels in leaves.
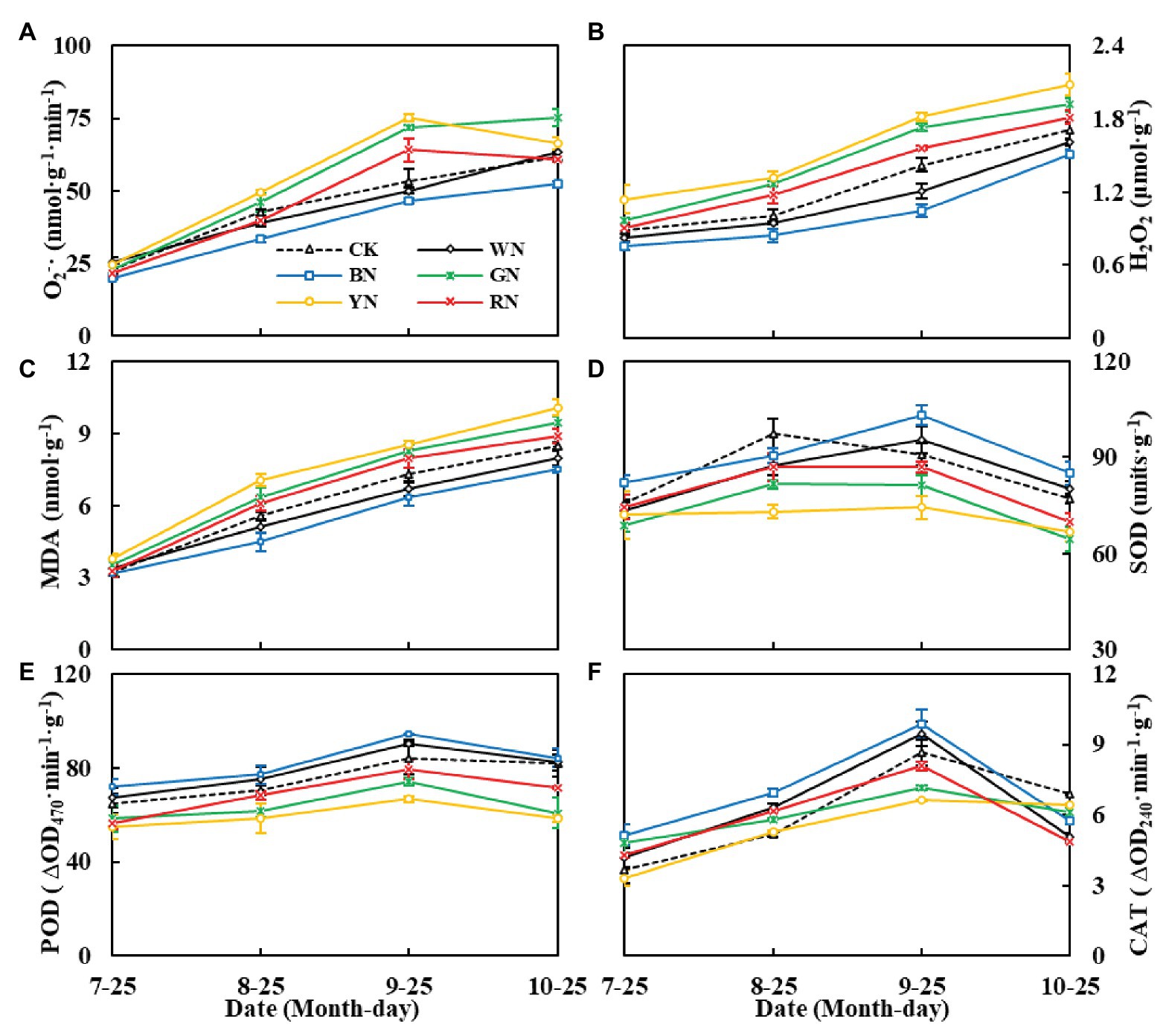
Figure 7. The effect of photo-selective nets on reactive oxygen, malondialdehyde (MDA) contents, and antioxidant enzyme activities of green onion leaves at different growth stages. (A) O2− (superoxide anion), (B) H2O2, (C) MDA content, (D) SOD activity, (E) POD activity, (F) CAT activity. CK, control; WN, white photo-selective nets; BN, blue; GN, green; YN, yellow; and RN, red. Values are average ± SD, n = 18. Error bars indicate standard errors.
Effects of Photo-Selective Nets on the Nutrient Content of Green Onion
Macroelements refer to the essential nutrients required for normal plant growth and development. In our experiment, we mainly determined the contents of N, P, and K in the roots, pseudo-stems, and leaves of green onion during the harvest stage. We found that the balance of nutrients in plants was significantly different under different photo-selective nets. Figure 8 summarizes the contents of various mineral elements in the roots, stems, and leaves of green onion under different treatments. We found that the nitrogen content in roots, pseudo-stems, and leaves was the highest under the BN treatment, and the trend among treatments was BN > WN > CK > RN > GN > YN. The changing trend of phosphorus and potassium content was consistent with nitrogen content.
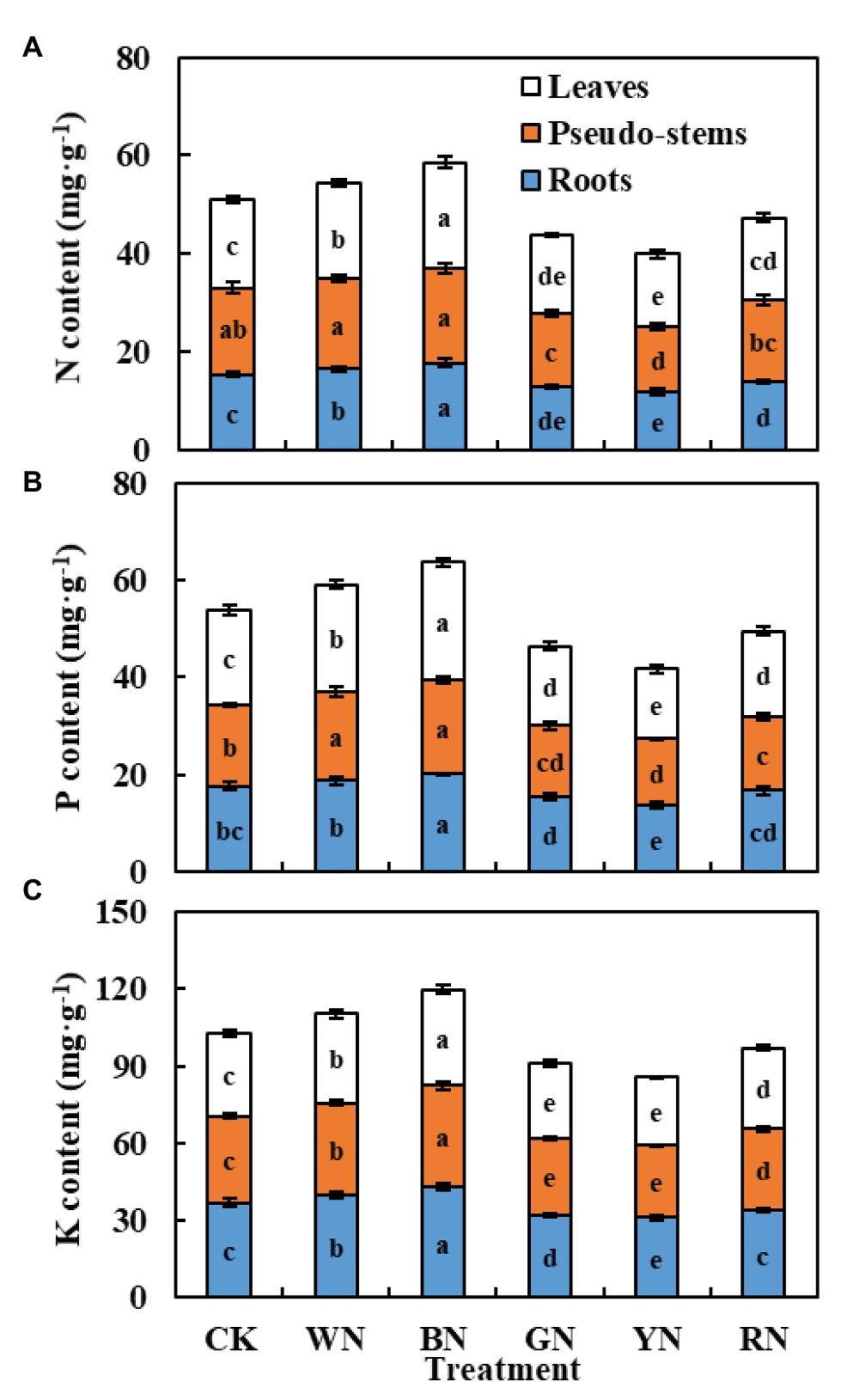
Figure 8. The effect of photo-selective nets on element content of green onion leaves, pseudo-stems, and roots during the harvest stage. (A) N content, (B) P content, (C) K content. CK, control; WN, white photo-selective nets; BN, blue; GN, green; YN, yellow; and RN, red. Values are average ± SD, n = 18. Error bars indicate standard errors. Different letters (a, b, c, d, and e) in the same column indicate significant differences among treatments at p ≤ 0.05 according to Duncan’s new multiple range test.
Discussion
Photo-selective nets are considered to prevent excessive sun radiation, improve soil moisture, stabilize air temperature, and promote normal plant growth. They can also change the radiation spectrum available to plants, which results in metabolic adjustment of the photosynthesis system (Sabino et al., 2016), which may help improve the plant growth characteristics evaluated in the present study. In a study carried out in Hungary using colored shading nets on peppers, the temperature changed relatively little in response to the nets, and it decreased as the shading level increased (Ombódi et al., 2015). It was reported that coffee trees planted under red nets have higher biomass than those planted under other types of shade nets (Henrique et al., 2011). This may be because red shading nets allowed the plants to absorb more photons of red wavelength, which may be beneficial for increasing the activity of photosynthetic pigments, resulting in increased photosynthetic assimilation (Yu et al., 2017). In our experiment, plant height, stem diameter, and aboveground fresh weight of plants under BN and WN treatments were significantly higher than those in plants under CK, RN, GN, and YN treatments (Table 2). This was inconsistent with the results of previous research, suggesting possible species-specific variation. It is possible to modify desirable plant growth characteristics by combining light scattering and spectral manipulation.
Light conditions have an important impact on the quality and yield of vegetable products (Fu et al., 2017). Red and pearl nets have been shown to improve the quality of tomato fruits (Ilić et al., 2012, 2015). Compared with traditional black nets, light adjustment and microclimate conditions under pearl nets provided higher crop yields (Ilić et al., 2011). Pearl nets not only improved the yield and quality of sweet peppers but also improved the postharvest shelf life of sweet pepper fruits (Selahle et al., 2015; Díaz-Pérez et al., 2020). In our experiment, BN increased the content of dry matter, soluble proteins, free amino acids, Vc, and soluble sugars (Table 4). This may be because BN improved the yield (Table 3) by improving the water use efficiency and absorption and conversion of various elements (Figure 8).
Chlorophyll is the basis for photosynthesis in plants. The chlorophyll content of plants in BN and WN treatments was significantly higher than that of CK plants. Ilić et al. (2017a) also found that the total chlorophyll content of leaves of lettuce cultivated under blue and black nets was higher than that of leaves of lettuce cultivated under other nets. Carotenoids act as antenna pigments and transmit the absorbed light energy to chlorophyll for further photochemical reaction, and they also serve to protect the chlorophylls from too much light or the wrong light wavelengths and thus act as a selective filter (Ilić et al., 2017b). This may be the reason why Pn was higher in plants under the BN treatment than in plants under the other treatments. Blue light has been proven to promote stomata opening (Inoue et al., 2008). In our experiment, the Gs of plants in the BN treatment were significantly higher than those of plants in the other treatments, which may also be the reason for the higher Tr. Plants with higher Pn consumed more CO2, which led to a decrease in the leaf Ci concentration (Figure 4). The increase in Tr compared to the Tr of CK plants indicated that BN could improve the absorption efficiency of nutrients by plants and allocate more nutrients to the aboveground organs. Increased Gs can lead to an increase in Ci concentration, which is beneficial for improving the photosynthetic activity of leaves and promoting the increase in Pn. From the diurnal variation in photosynthetic parameters (Figure 5), it can be seen that covering the plants with BN reduced the noon photosynthetic inhibition. This may be because BN can improve plant performance by reducing the noon photosynthetic inhibition caused by high temperatures and stomatal closure (Raveh et al., 2003).
In our study, taking the pseudo-stem expansion stage as an example, the Fv/Fm, ΦPSII, and qP of plants in the BN and WN treatments were significantly higher than those of CK plants, whereas Fv/Fm was significantly reduced and NPQ increased in plants in the GN and YN treatments compared to that in plants in the other treatments. This indicated that GN and YN caused a severe decrease in the photosynthesis of green onion plants and an increase in the heat dissipation in LHCII. In addition, this mainly indicated that green onion grown under GN and YN responded to photoinhibition; this can cause the production of ROS, which also explained the higher ROS content in green onion leaves in the YN treatment than in green onion leaves in the other treatments.
Oxidative stress can lead to the inhibition of photosynthesis and respiration processes, thereby inhibiting plant growth. MDA is one of the peroxidation products of cell membranes, reflecting the antioxidant capacity of plants. As important protective enzymes of the active oxygen scavenging enzyme system in plants, the increase in SOD, CAT, and POD activity could effectively prevent the accumulation of active oxygen, thereby ensuring that the MDA content was maintained at a low level, and maintaining normal plant growth. SOD is the first line of defense against oxidative stress and lipid peroxidation, catalyzing O2− to generate H2O2 and O2, whereas POD and CAT can remove H2O2 from peroxisomes and the cytoplasm (Dewir et al., 2006; Wu, 2016). In the present study, the contents of O2−, H2O2, and MDA in plants under BN and WN were lower, whereas the activities of SOD, POD, and CAT were higher than those of plants under the other photo-selective nets (Figure 7), indicating that BN and WN could effectively improve antioxidant capacity and delay the senescence of green onion plants.
Conclusion
This study found that photo-selective nets, especially blue and white nets, can affect the growth of green onion by increasing the antioxidant enzyme activity, chlorophyll content, photosynthetic performance, and element absorption and transformation capabilities of green onion. In green onion cultivation, blue nets can be used to improve plant yield and quality.
Data Availability Statement
The original contributions presented in the study are included in the article/Supplementary Material, further inquiries can be directed to the corresponding author.
Author Contributions
SG gathered samples, participated in the study design, performed data analysis, interpreted the results, and drafted the manuscript. XL and YL conducted experiments. KX conceived of the study, provided funding, and gave guidance on experimental design. KX, BC, and ZC modified the paper. All authors contributed to the article and approved the submitted version.
Funding
This work was supported by the Agricultural Fine Variety Project in Shandong Province of China (2020LZGC006).
Conflict of Interest
The authors declare that the research was conducted in the absence of any commercial or financial relationships that could be construed as a potential conflict of interest.
Acknowledgments
We would like to thank Editage1 for English language editing.
Supplementary Material
The Supplementary Material for this article can be found online at: https://www.frontiersin.org/articles/10.3389/fpls.2021.650471/full#supplementary-material
Footnotes
References
Abbasnia Zare, S. K., Sedaghathoor, S., Padasht Dahkaei, M.-N., and Hashemabadi, D. (2019). The effect of light variations by photoselective shade nets on pigments, antioxidant capacity, and growth of two ornamental plant species: marigold (Calendula officinalis L.) and violet (Viola tricolor). Cogent Food Agric. 5:1650415. doi: 10.1080/23311932.2019.1650415
Ada, N.-L., Farkash, L., Hamburger, D., Ovadia, R., Forrer, I., Kagan, S., et al. (2015). Light-scattering shade net increases branching and flowering in ornamental pot plants. J. Hortic. Sci. Biotechnol. 83, 9–14. doi: 10.1080/14620316.2008.11512340
Bisbis, M. B., Gruda, N., and Blanke, M. (2018). Potential impacts of climate change on vegetable production and product quality - a review. J. Clean. Prod. 170, 1602–1620. doi: 10.1016/j.jclepro.2017.09.224
Carvalho, S. D., Schwieterman, M. L., Abrahan, C. E., Colquhoun, T. A., and Folta, K. M. (2016). Light quality dependent changes in morphology, antioxidant capacity, and volatile production in sweet basil (Ocimum basilicum). Front. Plant Sci. 7:1328. doi: 10.3389/fpls.2016.01328
Chapman, J. M., Muhlemann, J. K., Gayomba, S. R., and Muday, G. K. (2019). RBOH-dependent ROS synthesis and ROS scavenging by plant specialized metabolites to modulate plant development and stress responses. Chem. Res. Toxicol. 32, 370–396. doi: 10.1021/acs.chemrestox.9b00028
Costa, L. C. B., Pinto, J. E. B. P., de Castro, E. M., Alves, E., Bertolucci, S. K. V., and Rosal, L. F. (2010). Effects of colored shade netting on the vegetative development and leaf structure of Ocimum selloi. Bragantia 69, 349–359. doi: 10.1590/S0006-87052010000200012
Darázsi, H. L. (2014). Influence of color net shading on quantity and quality of sweet pepper yield. Rev. Agric. Rural Dev. 3, 429–434.
Das, V. R. (2004). Photosynthesis: Regulation under varying light regimes. 1st Edn. Enfield, NH: Science Publishers, Inc.
Dewir, Y. H., Chakrabarty, D., Ali, M. B., Hahn, E. J., and Paek, K. Y. (2006). Lipid peroxidation and antioxidant enzyme activities of Euphorbia millii hyperhydric shoots. Environ. Exp. Bot. 58, 93–99. doi: 10.1016/j.envexpbot.2005.06.019
Díaz-Pérez, J. C., St John, K., Nambeesan, S. U., Kabir, M. Y., Alvarado-Chávez, J. A., and Bautista, J. (2020). Bell pepper (Capsicum annuum L.) plant growth and fruit yield as affected by colored shade nets. Acta Hortic. 1268, 289-292. doi: 10.17660/ActaHortic.2020.1268.38
Dueck, T., van Ieperen, W., and Taulavuori, K. (2016). Light perception, signaling and plant responses to spectral quality and photoperiod in natural and horticultural environments. Environ. Exp. Bot. 121, 1–3. doi: 10.1016/j.envexpbot.2015.06.012
Fu, Y., Li, H., Yu, J., Liu, H., Cao, Z., Manukovsky, N. S., et al. (2017). Interaction effects of light intensity and nitrogen concentration on growth, photosynthetic characteristics and quality of lettuce (Lactuca sativa L. Var. youmaicai). Sci. Hortic. 214, 51–57. doi: 10.1016/j.scienta.2016.11.020
Gao, S., Liu, X., Liu, Y., Cao, B., Chen, Z., and Xu, K. (2020). Photosynthetic characteristics and chloroplast ultrastructure of welsh onion (Allium fistulosum L.) grown under different LED wavelengths. BMC Plant Biol. 20:78. doi: 10.1186/s12870-020-2282-0
Gao, S., Liu, X., Liu, Y., Cao, B., Chen, Z., and Xu, K. (2021). Comparison of the effects of LED light quality combination on growth and nutrient accumulation in green onion (Allium fistulosum L.). Protoplasma. doi: 10.1007/s00709-020-01593-y [Epub ahead of print]
Genty, B., Briantais, J.-M., and Baker, N. R. (1989). The relationship between the quantum yield of photosynthetic electron transport and quenching of chlorophyll fluorescence. Biochim. Biophys. Acta 990, 87–92. doi: 10.1016/S0304-4165(89)80016-9
Gilmore, A. M., and Yamamoto, H. Y. (1991). Resolution of lutein and zeaxanthin using a non-endcapped, lightly carbon-loaded C18 high-performance liquid chromatographic column. J. Chromatogr. 543, 137–145. doi: 10.1016/S0021-9673(01)95762-0
Gong, B., Miao, L., Kong, W., Bai, J.-G., Wang, X., Wei, M., et al. (2014). Nitric oxide, as a downstream signal, plays vital role in auxin induced cucumber tolerance to sodic alkaline stress. Plant Physiol. Biochem. 83, 258–266. doi: 10.1016/j.plaphy.2014.08.004
Henrique, P. C., Alves, J. D., Deuner, S., Goulart, P. F. P., and do Livramento, D. E. (2011). Aspectos fisiológicos do desenvolvimento de mudas de café cultivadas sob telas de diferentes colorações. Pesq. Agrop. Brasileira 46, 458–465. doi: 10.1590/S0100-204X2011000500002
Hu, X., Cai, M., Yang, S., and Sejas, S. A. (2018). Air temperature feedback and its contribution to global warming. Sci. China Earth Sci. 61, 1491–1509. doi: 10.1007/s11430-017-9226-6
Ilić, S. Z., Milenković, L., Dimitrijević, A., Stanojević, L., Cvetković, D., Kevrešan, Ž., et al. (2017a). Light modification by color nets improve quality of lettuce from summer production. Sci. Hortic. 226, 389–397. doi: 10.1016/j.scienta.2017.09.009
Ilić, Z., Milenković, L., Đurovka, M., and Kapoulas, N. (2011). “The effect of color shade nets on the greenhouse climate and pepper yield” in Proceedings of the 46th Croatian and 6th International Symposium on Agriculture, Opatija Croatia; October 4, 2011, 529–532.
Ilić, Z. S., Milenković, L., Stanojević, L., Cvetković, D., and Fallik, E. (2012). Effects of the modification of light intensity by color shade nets on yield and quality of tomato fruits. Sci. Hortic. 139, 90–95. doi: 10.1016/j.scienta.2012.03.009
Ilić, Z. S., Milenković, L., Šunić, L., Barać, S., Mastilović, J., Kevrešan, Ž., et al. (2017b). Effect of shading by colored nets on yield and fruit quality of sweet pepper. Zemdirbyste-Agriculture 104, 53–62.
Ilić, Z. S., Milenković, L., Šunić, L., and Fallik, E. (2015). Effect of coloured shade-nets on plant leaf parameters and tomato fruit quality. J. Sci. Food Agric. 95, 2660–2667. doi: 10.1002/jsfa.7000
Inoue, S.-i., Kinoshita, T., Matsumoto, M., Nakayama, K. I., Doi, M., and Shimazaki, K.-i. (2008). Blue light-induced autophosphorylation of phototropin is a primary step for signaling. Proc. Natl. Acad. Sci. 105, 5626–5631. doi: 10.1073/pnas.0709189105
Jackson, S. D. (2009). Plant responses to photoperiod. New Phytol. 181, 517–531. doi: 10.1111/j.1469-8137.2008.02681.x
Kurepin, L. V., Emery, R. J. N., Pharis, R. P., and Reid, D. M. (2007). Uncoupling light quality from light irradiance effects in Helianthus annuus shoots: putative roles for plant hormones in leaf and internode growth. J. Exp. Bot. 58, 2145–2157. doi: 10.1093/jxb/erm068
Leite, C. A., Ito, R. M., Lee, G. T. S., Ganelevin, R., and Fagnani, M. A. (2008). “Light spectrum management using colored nets to control the growth and blooming of phalaenopsis” in International Society for Horticultural Science (ISHS) ; Leuven, Belgium, 177–184.
Lusk, C. H., and Pozo, A. D. (2002). Survival and growth of seedlings of 12 Chilean rainforest trees in two light environments: gas exchange and biomass distribution correlates. Austral Ecol. 27, 173–182. doi: 10.1046/j.1442-9993.2002.01168.x
Nissim-Levi, A., Ovadia, R., Kagan, S., and Oren-Shamir, M. (2014). Shading stock plants with photoselective nets affects the yield and rooting quality of their cuttings. J. Hortic. Sci. Biotechnol. 89, 693–699. doi: 10.1080/14620316.2014.11513139
Ombódi, A., Pék, Z., Szuvandzsiev, P., Tóthné Taskovics, Z., Kóházi-Kis, A., Kovács, A., et al. (2015). Effects of external coloured shade nets on sweet peppers cultivated in walk-in plastic tunnels. Not. Bot. Horti Agrobot. Cluj Napoca 43, 398–403. doi: 10.15835/nbha4329863
Patterson, B. D., MacRae, E. A., and Ferguson, I. B. (1984). Estimation of hydrogen peroxide in plants extracts using titanium(IV). Anal. Biochem. 139, 487–492. doi: 10.1016/0003-2697(84)90039-3
Raveh, E., Cohen, S., Raz, T., Yakir, D., Grava, A., and Goldschmidt, E. E. (2003). Increased growth of young citrus trees under reduced radiation load in a semi-arid climate1. J. Exp. Bot. 54, 365–373. doi: 10.1093/jxb/erg009
Roháček, K. (2002). Chlorophyll fluorescence parameters: the definitions, photosynthetic meaning, and mutual relationships. Photosynthetica 40, 13–29. doi: 10.1023/A:1020125719386
Roldán, A., Díaz-Vivancos, P., Hernández, J. A., Carrasco, L., and Caravaca, F. (2008). Superoxide dismutase and total peroxidase activities in relation to drought recovery performance of mycorrhizal shrub seedlings grown in an amended semiarid soil. J. Plant Physiol. 165, 715–722. doi: 10.1016/j.jplph.2007.02.007
Sabino, M., Korpan, C., Ferneda, B. G., and Silva, A. C. (2016). Crescimento de mudas de ipês em diferentes telas de sombreamento. Nat. Aust. 4, 61–65. doi: 10.14583/2318-7670.v04n02a01
Selahle, K. M., Sivakumar, D., Jifon, J., and Soundy, P. (2015). Postharvest responses of red and yellow sweet peppers grown under photo-selective nets. Food Chem. 173, 951–956. doi: 10.1016/j.foodchem.2014.10.034
Selahle, M. K., Sivakumar, D., and Soundy, P. (2014). Effect of photo-selective nettings on post-harvest quality and bioactive compounds in selected tomato cultivars. J. Sci. Food Agric. 94, 2187–2195. doi: 10.1002/jsfa.6536
Shahak, Y. (2008). “Photo-selective netting for improved performance of horticultural crops. A review of ornamental and vegetable studies carried out in Israel” in International Society for Horticultural Science (ISHS) ; Leuven, Belgium, 161–168.
Shahak, Y., Gussakovsky, E., Gal, E., and Ganelevin, R. (2004). “Crop protection and light-quality manipulation in one technology” in Proceedings of the VII th International Syposium ISHS–Acta Horticulturae. 143–151.
Stamps, R. H., and Chandler, A. L. (2008). “Differential effects of colored shade nets on three cut foliage crops.” in International Society for Horticultural Science (ISHS) ; Leuven, Belgium, 169–176.
Tucci, M. L. S., Erismann, N. M., Machado, E. C., and Ribeiro, R. V. (2010). Diurnal and seasonal variation in photosynthesis of peach palms grown under subtropical conditions. Photosynthetica 48, 421–429. doi: 10.1007/s11099-010-0055-y
Wada, M., Nagano, M., Kido, H., Ikeda, R., Kuroda, N., and Nakashima, K. (2011). Suitability of TBA method for the evaluation of the oxidative effect of non-water-soluble and water-soluble rosemary extracts. J. Oleo Sci. 60, 579–584. doi: 10.5650/jos.60.579
Wang, X., Fu, X., Chen, M., Huan, L., Liu, W., Qi, Y., et al. (2018a). Ultraviolet B irradiation influences the fruit quality and sucrose metabolism of peach (Prunus persica L.). Environ. Exp. Bot. 153, 286–301. doi: 10.1016/j.envexpbot.2018.04.015
Wang, F., Wu, N., Zhang, L., Ahammed, G. J., Chen, X., Xiang, X., et al. (2018b). Light signaling-dependent regulation of photoinhibition and photoprotection in tomato. Plant Physiol. 176:1311. doi: 10.1104/pp.17.01143
Wu, H. (2016). Effect of different light qualities on growth, pigment content, chlorophyll fluorescence, and antioxidant enzyme activity in the red alga Pyropia haitanensis (Bangiales, Rhodophyta). Biomed. Res. Int. 2016:7383918. doi: 10.1155/2016/7383918
Xia, X.-J., Wang, Y.-J., Zhou, Y.-H., Tao, Y., Mao, W.-H., Shi, K., et al. (2009). Reactive oxygen species are involved in brassinosteroid-induced stress tolerance in cucumber. Plant Physiol. 150, 801–814. doi: 10.1104/pp.109.138230
Yang, X., Xu, H., Shao, L., Li, T., Wang, Y., and Wang, R. (2018). Response of photosynthetic capacity of tomato leaves to different LED light wavelength. Environ. Exp. Bot. 150, 161–171. doi: 10.1016/j.envexpbot.2018.03.013
Yangen, F., Xiuxiu, Z., Hanyue, W., Yueyue, T., Qinzeng, X., and Lixia, Z. (2019). Effects of light intensity on metabolism of light-harvesting pigment and photosynthetic system in Camellia sinensis L. cultivar “Huangjinya”. Environ. Exp. Bot. 166:103796. doi: 10.1016/j.envexpbot.2019.06.009
Keywords: green onion (Allium fistulosum L.), photo-selective nets, photosynthesis, product quality, plant antioxidant system
Citation: Gao S, Liu X, Liu Y, Cao B, Chen Z and Xu K (2021) The Spectral Irradiance, Growth, Photosynthetic Characteristics, Antioxidant System, and Nutritional Status of Green Onion (Allium fistulosum L.) Grown Under Different Photo-Selective Nets. Front. Plant Sci. 12:650471. doi: 10.3389/fpls.2021.650471
Edited by:
Fabricio Eulalio Leite Carvalho, Corporacion Colombiana De Investigacion Agropecuaria (Agrosavia) - CI La Suiza, ColombiaReviewed by:
Peter J. Gollan, University of Turku, FinlandSzilvia Z. Tóth, Hungarian Academy of Sciences (MTA), Hungary
Copyright © 2021 Gao, Liu, Liu, Cao, Chen and Xu. This is an open-access article distributed under the terms of the Creative Commons Attribution License (CC BY). The use, distribution or reproduction in other forums is permitted, provided the original author(s) and the copyright owner(s) are credited and that the original publication in this journal is cited, in accordance with accepted academic practice. No use, distribution or reproduction is permitted which does not comply with these terms.
*Correspondence: Kun Xu, xukun@sdau.edu.cn; Zijing Chen, chenzijing@sdau.edu.cn