- 1School of Life and Health Science, Anhui Science and Technology University, Bengbu, China
- 2College of Life science, Anhui Agricultural University, Hefei, China
Plants can naturally interact with beneficial rhizobacteria to mediate defense responses against foliar pathogen infection. However, the mechanisms of rhizobacteria-mediated defense enhancement remain rarely clear. In this study, beneficial rhizobacterial strain Pseudomonas fluorescens DN16 greatly increased the resistance of cucumber plants against Botrytis cinerea infection. RNA-sequencing analyses showed that several polyamine-associated genes including a thermospermine (TSpm) synthase gene (CsACL5) and polyamine catabolic genes (CsPAO1, CsPAO5, and CsCuAO1) were notably induced by DN16. The associations of TSpm metabolic pathways with the DN16-mediated cucumber defense responses were further investigated. The inoculated plants exhibited the increased leaf TSpm levels compared with the controls. Accordantly, overexpression of CsACL5 in cucumber plants markedly increased leaf TSpm levels and enhanced defense against B. cinerea infection. The functions of TSpm catabolism in the DN16-mediated defense responses of cucumber plants to B. cinerea were further investigated by pharmacological approaches. Upon exposure to pathogen infection, the changes of leaf TSpm levels were positively related to the enhanced activities of polyamine catabolic enzymes including polyamine oxidases (PAOs) and copper amine oxidases (CuAOs), which paralleled the transcription of several defense-related genes such as pathogenesis-related protein 1 (CsPR1) and defensin-like protein 1 (CsDLP1). However, the inhibited activities of polyamine catabolic enzymes abolished the DN16-induced cucumber defense against B. cinerea infection. This was in line with the impaired expression of defense-related genes in the inoculated plants challenged by B. cinerea. Collectively, our findings unraveled a pivotal role of TSpm catabolism in the regulation of the rhizobacteria-primed defense states by mediating the immune responses in cucumber plants after B. cinerea infection.
Introduction
Plants as sessile organisms inevitably suffer from biotic and abiotic stresses. Unlike animals, plants cannot avoid these stressful factors, which seriously impact plant growth and development, and the biomass of agricultural crops. During long-term evolution, a series of highly complicated and coordinated strategies has been developed to tolerate various biotic stresses, involving activation of signaling cascades that results in a wide range of stress responses. These adaptive stress responses have well been manifested by diverse biochemical and physiological alterations, such as the biosynthesis of terpenes, pathogenesis-related proteins, and polyamines (PAs; Walters, 2003; van Loon et al., 2006; Block et al., 2019).
Polyamines including putrescine (Put), spermidine (Spd), spermine (Spm), and thermospermine (TSpm) are widely found in diverse plant species, which are important for plant physiological and metabolic processes (Shi and Chan, 2014). The Put can be synthesized via the catalysis of the ornithine decarboxylase and arginine decarboxylase. Subsequently, the Spd synthase promotes the conversion of Put into Spd. The Spd can be successively converted into both the Spm and TSpm by the Spm and TSpm synthase, respectively (Fuell et al., 2010; Kim et al., 2014). The TSpm exists in the whole plant kingdom, whereas the Spm is only found in diverse angiosperms (Takano et al., 2012). In Arabidopsis, the xylem-specific ACL5 gene is essential for the synthesis of TSpm, which participates in the regulation of vascular development by hindering the premature death of xylem elements (Kakehi et al., 2018). The functions of TSpm have been found to be associated with the modulation of diverse processes such as cell wall patterning, cell death, and xylem cell morphology (Muñiz et al., 2008; Shinohara et al., 2019; Vuosku et al., 2019). Furthermore, high-level TSpm contributes to enhancing the tolerance of Arabidopsis plants to Pseudomonas viridiflava, whereas the acl5 mutants deficient in the synthesis of TSpm exhibit the increased sensitivity to the pathogen attacks compared with the wild type (WT) plants (Marina et al., 2013). Accordantly, silencing of GhACL5 reduces the levels of TSpm in cotton, thereby increasing the susceptibility to Verticillium dahlia (Mo et al., 2015a).
In plants, the catabolism of cellular PAs can be modulated by the PA oxidases (PAOs) and copper amine oxidases (CuAOs) under abiotic and biotic stress conditions (Cona et al., 2006; Wang et al., 2009). In many cases, biotic stress can induce the synthesis of PA synthesis and further trigger its catabolism (Angelini et al., 2010). Microbial pathogens have also been shown to induce the synthesis and oxidation of PAs in the tobacco leaf apoplast (Marina et al., 2008). Upon exposure to biotic stress, the metabolic homeostasis of PA participates in regulating defense responses in plants (Takahashi et al., 2004; Gonzalez et al., 2011). Spm can function as a key signaling molecule that provokes defense responses in both the virus‐ and P. viridiflava-infected plants (Gonzalez et al., 2011). However, high-level Spd can enhance the susceptibility of tomato plants to Botrytis cinerea by attenuating the ET-mediated signaling pathways (Nambeesan et al., 2012). PAOs can catalyze the conversion of Spd and Spm into 1,3-diaminopropane, H2O2, and the corresponding aldehyde (Rea et al., 2002). In Arabidopsis, several PAOs promote the back conversion of Spm to Put, accompanied by generating 3-aminopropanal and H2O2 (Moschou et al., 2008). Many studies have indicated that the oxidation of PAs can impact cell-wall maturation, lignification and reinforce cell wall, leading to the increased resistance against pathogen infection (Cona et al., 2006). Overexpression of the PAO genes in tobacco plants induces systemic acquired resistance and enhances the cell-wall-based defenses, conferring the increased resistance against bacterial and fungal pathogens (Moschou et al., 2009). It is increasingly evidenced that the oxidation of PAs in plants by PAOs and CuAOs is correlated with plant disease resistance (Hatmi et al., 2014, 2015). Repression of the PAO and CuAO activities in the stressed-treated grapevine leaves abolishes the activation of defense systems against B. cinerea infection.
It has previously been indicated that interaction of plant roots with soil microorganisms can mediate plant growth and development, and adaptive responses under adverse environments (Nadeem et al., 2014; Fahad et al., 2015). Several rhizobacteria strains establish mutualistic relationships with the hosts to benefit two parties (Zhou et al., 2019). Beneficial bacteria habituated in plant rhizosphere are frequently called as plant growth promoting rhizobacteria (PGPR), which help the host inhibit phytopathogens, promote plant growth and survive under adverse conditions (Lugtenberg and Kamilova, 2009). Many studies have reported that several PGPR strains can augment the levels of PAs in host plants by upregulating the expression of PA biosynthetic genes or direct secretion of PAs, which confers the enhanced abiotic stress tolerance in plants (Zhou et al., 2016; Sen et al., 2018). However, it remains elusive whether rhizobacteria can mediate the metabolic pathways of PAs in plants and how its metabolic alterations are correlated with host immune responses.
In this study, inoculation with P. fluorescens DN16 increased leaf TSpm levels in cucumber plants, which were positively associated with the transcription levels of CsACL5, as evidenced by the RNA-sequencing data. We further assessed the impacts of DN16 on the levels of TSpm and the activities of PA catabolic enzymes. A pharmacological approach was also applied to assess the roles of DN16-mediated TSpm catabolism in the regulation of cucumber resistance against B. cinerea infection.
Materials and Methods
Plant Materials and Microbial Culture
Seeds of the cucumber (Cucumis sativus L.) inbred line NK23 were sterilized with 0.1% HgCl2 followed by rinsing with sterile water and were then cultured in 1/2 MS medium. Seven-day-old cucumber seedlings were transferred into sterile soil and placed in a plant growth chamber at 16-h day (25°C)/8-h night (23°C) cycle.
The bacterial strain tested in this study was P. fluorescens DN16, which was isolated from the rhizospheric soil of 2-month-old cucumber plants. DN16 was cultured in liquid potato dextrose agar (PDA) medium at 28°C. The cultures were centrifuged at 6000 rpm for 5 min and resuspended in sterile PBS solution (0.02 M, pH 7.2). The collected cells were then adjusted to the concentration of 1 × 108 CFU ml−1 for next experiments.
For assays of pathogen infection, the foliar pathogen B. cinerea was cultured on PDA agar medium at 28°C for 2 weeks. Spore suspensions of B. cinerea were harvested with the solution (0.05 M KH2PO4 and 6 mM glucose) and 0.01% (v/v) Tween 20, adjusting to 2 × 105 spores ml−1 (Curvers et al., 2010).
Generation of Transgenic Cucumber Plants
To construct CsACL5-overexpressing vector, cDNA sequence of CsACL5 was amplified and ligated into the pMD18-T vector (Takara, Japan). After sequencing, the cDNA sequence of CsACL5 was digested and introduced into the plant binary vector pCAMBIA1300. To generate RNAi-CsACL5 vector, the cDNA fragment of CsACL5 was amplified and inserted into the intermediated vector pHANNIBAL with the sense direction by digestion of XhoI and EcoRI and antisense direction by digestion of HindIII and XbaI, respectively. Subsequently, the pHANNIBA-CsACL5 vector was digested by NotI and ligated into the pART27 to form the recombinant plasmid RNAi-CsACL5. Agrobacterium tumefaciens EHA105 harboring the above constructed plasmids were transformed into cucumber plants as described by Wang et al. (2014).
Pathogen Infection Tests
Assays of P. fluorescens DN16-induced disease resistance were carried out, in which 7-day-old cucumber seedlings grown in 1/2 MS media were transferred into plastic pots containing sterile soils and grown for 4 weeks. Then, cell suspensions of DN16 were collected and poured into the soil (5 × 107 CFU g−1 soil), and the control treatments were inoculated with sterile PBS solution (0.02 M, pH 7.2) for 5 days. Then, the control and inoculated plants were subjected to in vitro and in vivo pathogen infection assays (El Oirdi et al., 2011). For in vitro tests, 5 μl spore suspensions of B. cinerea were dipped on detached leaves. At 5 days post infection (dpi), lesion diameters were determined. For in vivo tests, the leaves were sprayed with B. cinerea and placed in growth chamber at 100% relative humidity. At 7 dpi, disease index was evaluated as described recently by Jing et al. (2019). Moreover, the ratio of BcActin to CsActin was calculated for determining the biomass of B. cinerea as reported by Hu et al. (2018).
Histochemical Detection and Determination of Fv/Fm
In vivo localization of dead cells and H2O2 were detected in cucumber leaves using trypan blue and diaminobenzidine (DAB) staining, respectively (Daudi and O’Brien, 2012; Tian et al., 2018). To analyze pathogen-induced cell death, leaf tissues separated from both the pathogen-infected control and inoculated plants were incubated in the trypan blue staining solution for 45 min, followed by rinsing with distilled water five times and bleaching with 75% ethanol. To observe the production of H2O2, harvested leaves were immediately immersed in the DAB staining solution for 6 h, followed by bleaching with 75% ethanol. In addition, the values of Fv/Fm were determined by a Portable Photosynthesis System CIRAS-2.
Analyses of RNA Sequencing and Quantitative Real-Time PCR
Five-week-old cucumber plants grown in sterile soils were inoculated with or without DN16 at the density of 5 × 107 CFU g−1 soil for 5 days, and the control and inoculated leaves were then sprayed with spore suspension of B. cinerea (2 × 105 spore ml−1). Universal RNA Extraction Kit (Takara, Japan) was applied to isolate total RNAs from the control and inoculated leaves at different time points post pathogen infection. The quality and concentration of RNA samples were spectrophotometericaly detected and the contaminated DNA was digested. To conduct RNA-Seq analyses, these RNA samples from the leaves at 0, 24, and 48 hpi were used to construct cDNA libraries, and three biological replicates were carried out. Low-quality and adapter sequences were then removed from raw data that were deposited in the NCBI SRA database (no. PRJNA686802), and were then aligned to the database.1 Compared with the controls, several DEGs were screened from the leaves of DN16-inoculated plants at a cutoff of FDR value <0.05 and │log2 ratio ≥ 1.5│, and were enriched by the GO terms and KEGG pathways (Zhang et al., 2016). Furthermore, quantitative PCR (qPCR) analyses were conducted with at least three biological replicates. qPCR reactions were performed with the SYBR Premix Ex TaqTM II kit (TaKaRa, Japan) in an ABI7500 machine. Gene expression in cucumber leaves was analyzed by qPCR and the CsActin gene was used as an internal control for normalizing their transcription (Yang et al., 2019). Gene expression was analyzed using the 2−ΔΔCt method. The information of primers was shown in Supplementary Table S1.
Determination of PAs
The content of PAs was quantified by HPLC as reported previously by Mo et al. (2015b). Briefly, leaf tissues (0.5 g) were ground and mixed in 10 ml of ice-cold 5% perchloric acid for 1 h, and then centrifuged at 12000 rpm for 15 min at 4°C. The supernatants (0.5 ml) were mixed with 1 ml of 2 N NaOH and 7 μl of benzoyl chloride, followed by reacting for 30 min at 37°C. After that, 1.5 ml of saturated NaCl and diethyl ether was added into the mixture and was then centrifuged for 10 min at 1000 rpm. One milliliter of organic solvent phase was collected and dissolved in 100 μl of methanol and filtered by 0.22 μm microfiltration membrane. Finally, the methanol solution was detected by HPLC as reported by Mo et al. (2015b).
Analyses of PAO and CuAO Activities and Pharmacological Treatments
Assays of leaf PAO and CuAO activities were carried out as reported previously by Hatmi et al. (2015). Leaf tissues (0.5 g) was homogenized in 1 ml of 0.25 M phosphate buffer solution, and then centrifuged at 12000 rpm for 5 min at 4°C. The supernatants were used to detect the activities of PAOs and CuAOs as described previously by Cona et al. (2006). In addition, to inhibit the activities of PA catabolic enzymes, the leaves of whole plants were pretreated with the CuAO inhibitor aminoguanidine (AG, 5 mM) or the PAO inhibitor β-hydroxyehtylhydrazine (HEH, 10 mM). After 3 days of treatments, these plants were subjected to foliar treatment with spore suspension of B. cinerea (2 × 105 spore ml−1).
Statistical Analysis
The data were analyzed with SPSS software, and all the data were indicated as the means ± SD from three biological repeats and analyzed statistically using Duncan’s multiple range tests (p < 0.05).
Results
P. fluorescens DN16 Induces Cucumber Resistance Against B. cinerea Infection
To examine whether P. fluorescens DN16-induced systemic resistance (ISR) was effective against the foliar pathogen B. cinerea, 5-week-old cucumber plants grown in sterile soils were treated with cell suspension of DN16 for 5 days, followed by challenging with B. cinerea. In the detached leaf tests, leaves of non-inoculated (control) plants exhibited larger lesion diameters and necrotic tissues at 5 dpi (Figure 1A). In contrast, leaves of DN16-inoculated plants displayed disease-resistant phenotypes, as reflected by smaller lesion diameters (Figures 1A,B). In addition, the inoculated plants had higher proportions of fewer small lesions (lesion diameter < 5 mm) than the controls (Figure 1C).
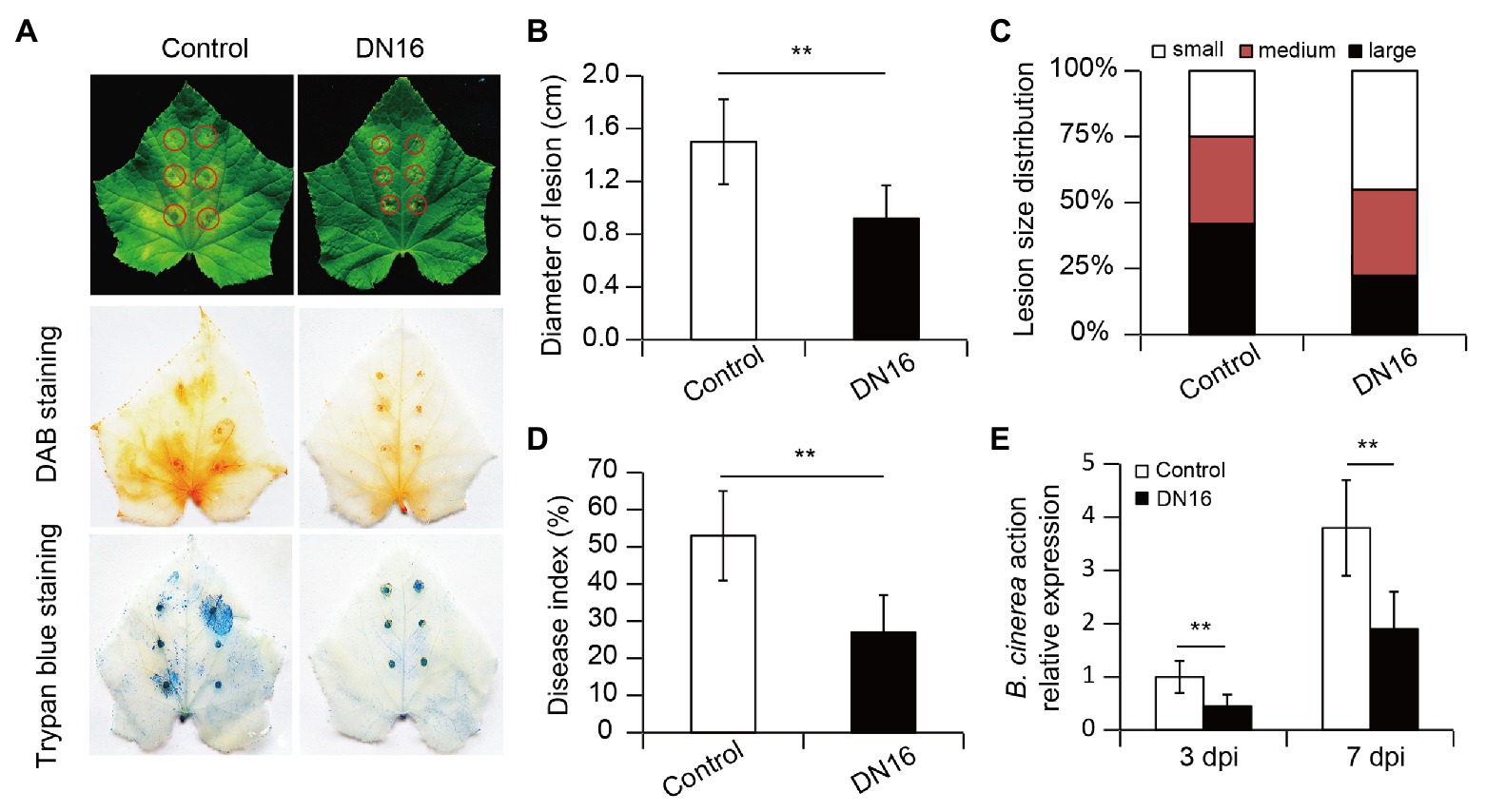
Figure 1. Effects of Pseudomonas fluorescens DN16 inoculation on the resistance of cucumber plants against Botrytis cinerea. Five-week-old cucumber plants were treated with or without DN16 for 5 days. Then, these plants were challenged with the foliar pathogen B. cinerea. (A) Lesion symptoms of detached leaves at 5 days post infection (dpi), and diaminobenzidine (DAB) and trypan blue staining of pathogen-infected leaves. (B) Lesion diameters of detached leaves. (C) Lesion distribution: small (LD < 5 mm), medium (5 mm < LD < 10 mm), and large (LD > 10 mm). (D) Both the control and inoculated leaves were sprayed with B. cinerea for 7 days, and disease index was calculated. (E) Relative expression of BcActin was examined at 3 and 7 dpi, respectively. The data represented the means ± SD from three biological repeats and significant differences were examined by student’s t-test at **p < 0.01.
The production of H2O2 and cell death in the leaves was further examined by DAB and trypan blue staining, respectively. As shown in Figure 1A, the leaves of the inoculated plants accumulated lower H2O2 levels than those of the controls. The inoculated leaves also exhibited markedly less cell death than the control leaves. In the whole-plant inoculation assays, both the control and inoculated leaves were treated with B. cinerea. At 7 dpi, the inoculated plants had lower disease index than the controls (Figure 1D). Moreover, qPCR analyses showed that the inoculated leaves had lower BcActin transcripts than the controls at 3 and 7 dpi (Figure 1E), which was in accordance to the observed reduction of disease occurrence.
To rule out the probability that the DN16-induced disease resistance was resulted from direct impacts of DN16 on B. cinerea, potentially transferring of root-inoculated bacteria into leaf tissues was evaluated by culturing leaf extracts from the DN16-inoculated plants onto selective KB agar plates. However, DN16 was not detected in the inoculated leaves (data not shown). In combination with the inability of DN16 to antagonize the growth of B. cinerea, our data indicated that the DN16-induced systemic resistance was not attributable to microbial antagonism but rather activation of the defense systems of plant itself.
Transcriptome Analyses of the DN16-Inoculated Plants Infected by B. cinerea
To unravel the molecular mechanisms of DN16-mediated ISR in cucumber plants, DEGs were identified by comparing analyses of the control and inoculated plants, which were markedly induced by DN16. Herein, 5-week-old cucumber plants cultivated in the sterile soils were treated with DN16 for 5 days, a total of 933 DEGs were screened, including 284 downregulated and 649 upregulated DEGs (Supplementary Table S2). KEGG pathway enrichment analysis revealed a total of 20 enriched pathways, involving metabolic pathways, biosynthesis of secondary metabolites, phenylpropanoid biosynthesis, and other pathways (Figure 2A). GO enrichment analysis for these DEGs was categorized into biological process, cell component, and molecular function (Figure 2B). The enriched DEGs were assigned to multiple processes, such as metabolic processes, cellular process, and biological regulation, and the majority of the enriched DEGs belonged to the metabolic process.
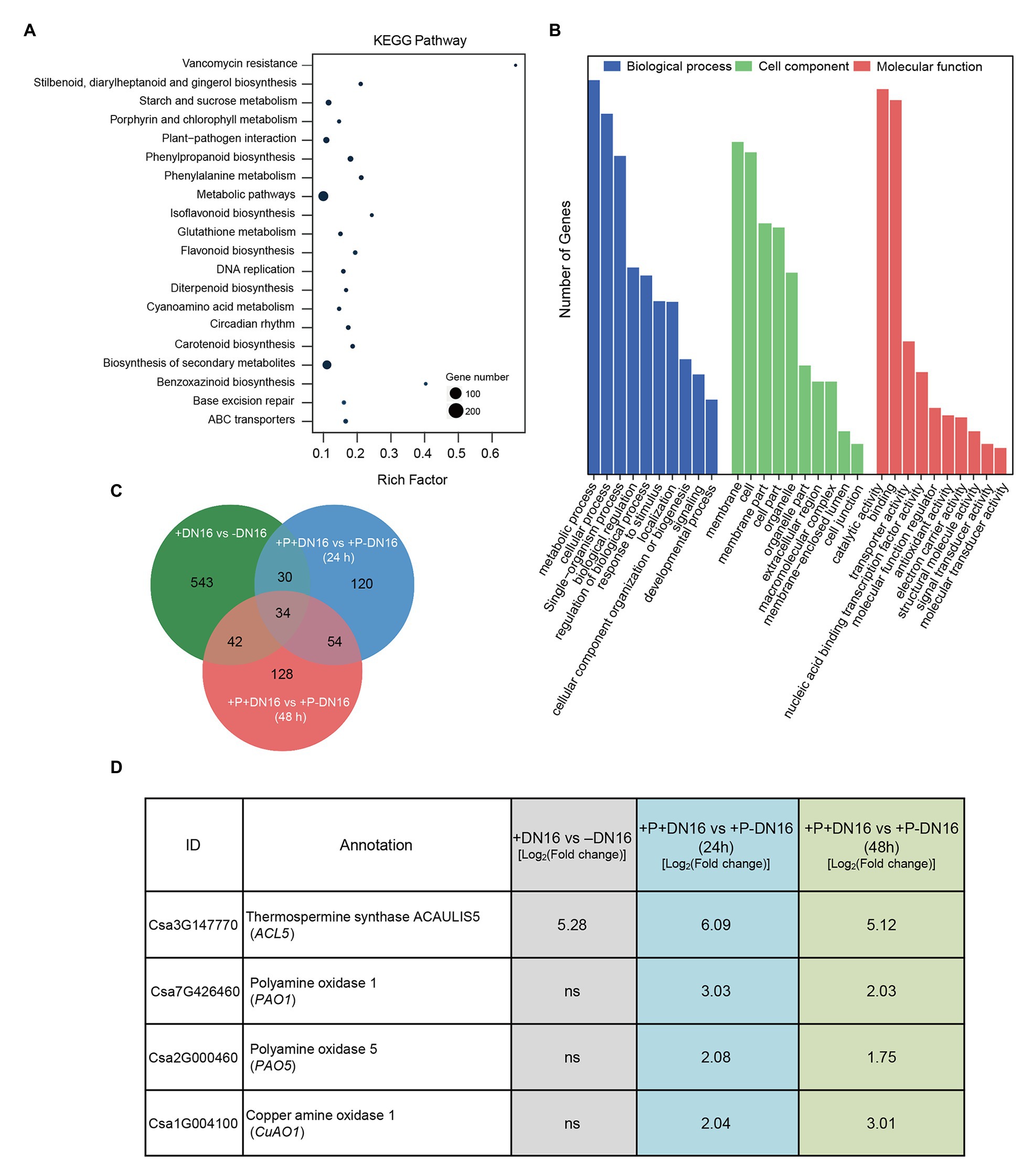
Figure 2. Identification of differentially expressed genes (DEGs) in cucumber plants in response to P. fluorescens DN16 and/or B. cinerea. Five-week-old cucumber plants were treated with or without DN16 for 5 days. Then, both the control and inoculated leaves were sprayed with B. cinerea. The leaves at 0, 24, and 48 dpi were harvested for the RNA-Seq analyses. (A) KEGG pathway and (B) GO enrichment analyses of DEGs at 0 dpi. (C) Venn diagrams of shared DEGs among different experimental groups. (D) Expression profiles of four polyamines (PA)-related genes (CsACL5, CsPAO1, CsPAO5, and CsCuAO1) in both the control and inoculated leaves challenged with or without B. cinerea infection.
After 5 days of inoculation, the cucumber plants were subjected to foliar treatment with B. cinerea. A total of 681 and 519 DEGs were screened at 24 (Supplementary Table S3) and 48 hpi (Supplementary Table S4), respectively, which were distinctly induced in the pathogen-infected plants colonized by DN16. As shown in Figure 2C, Venn diagram revealed that 34 upregulated DEGs were shared in the DN16-inoculated plants, which was likely related to the DN16-mediated disease resistance (Supplementary Table S5). Among these shared DEGs, the expression of a thermospermine synthase ACL5 gene (CsACL5) was induced by DN16. Moreover, 54 upregulated DEGs were significantly enriched in the inoculated plants at 24 and 48 hpi. Among these DEGs, several pathogen defense-related genes including pathogenesis-related protein 1 (CsPR1), defensin-like protein 1 (CsDLP1), and PA catabolic genes including polyamine oxidase 1 (CsPAO1), polyamine oxidase 5 (CsPAO5), and copper amine oxidase 1 (CsCuAO1) were significantly enriched in the pathogen-infected plants colonized by DN16 (Figure 2D). qPCR analyses showed that these PA-related genes were significantly affected in the inoculated plants, which was in line with the data of RNA-Seq (Supplementary Table S6).
Induction of CsACL5 by DN16 Contributed to Increased Disease Resistance
As shown in Figure 3A, compared with the controls, the cucumber plants exhibited a marked increase of leaf TSpm levels after 3 days of DN16 inoculation. The levels of TSpm were gradually decreased in the inoculated leaves followed by the time delay of pathogen infection. After 3 days of pathogen infection, the inoculated plants displayed no marked discrepancy with the controls (Figure 3B). As mentioned above, RNA-Seq data revealed that the inoculation with DN16 induced a remarkable elevation of CsACL5 transcripts in the cucumber leaves. To verify whether upregulation of CsACL5 contributed to the improved host resistance against B. cinerea, the WT plants were transformed with CsACL5 driven by the CaMV 35S promoter. Two independent T2 lines (ACL5ox-L2 and -L8) were used in this study, which exhibited exceedingly high expression of CsACL5 (Figure 3C). Accordantly, the two 35S::CsACL5 lines had higher leaf TSpm levels than the WT plants (Figure 3D). We further assessed the impacts of CsACL5 overexpression on disease resistance. At 7 dpi, the 35S::CsACL5 lines exhibited a notable reduction in disease index compared with the WT plants (Figure 3E). The 35S::CsACL5 lines also displayed less photosynthetic damages than the WT plants upon exposure to pathogen infection, as reflected by higher values of ФPSII in the transgenic lines (Figure 3F). qPCR analyses revealed that the transcription of BcActin was markedly lower in the leaves of the 35S::CsACL5 lines than the WT plants (Figure 3G). Consistently, in the detached leaf pathogen tests, overexpression of CsACL5 enhanced cucumber resistance against B. cinerea infection, displaying smaller lesion diameters (Figures 3H,I). These findings implied that high-level transcription of CsACL5 conferred the increased disease resistance in cucumber plants.
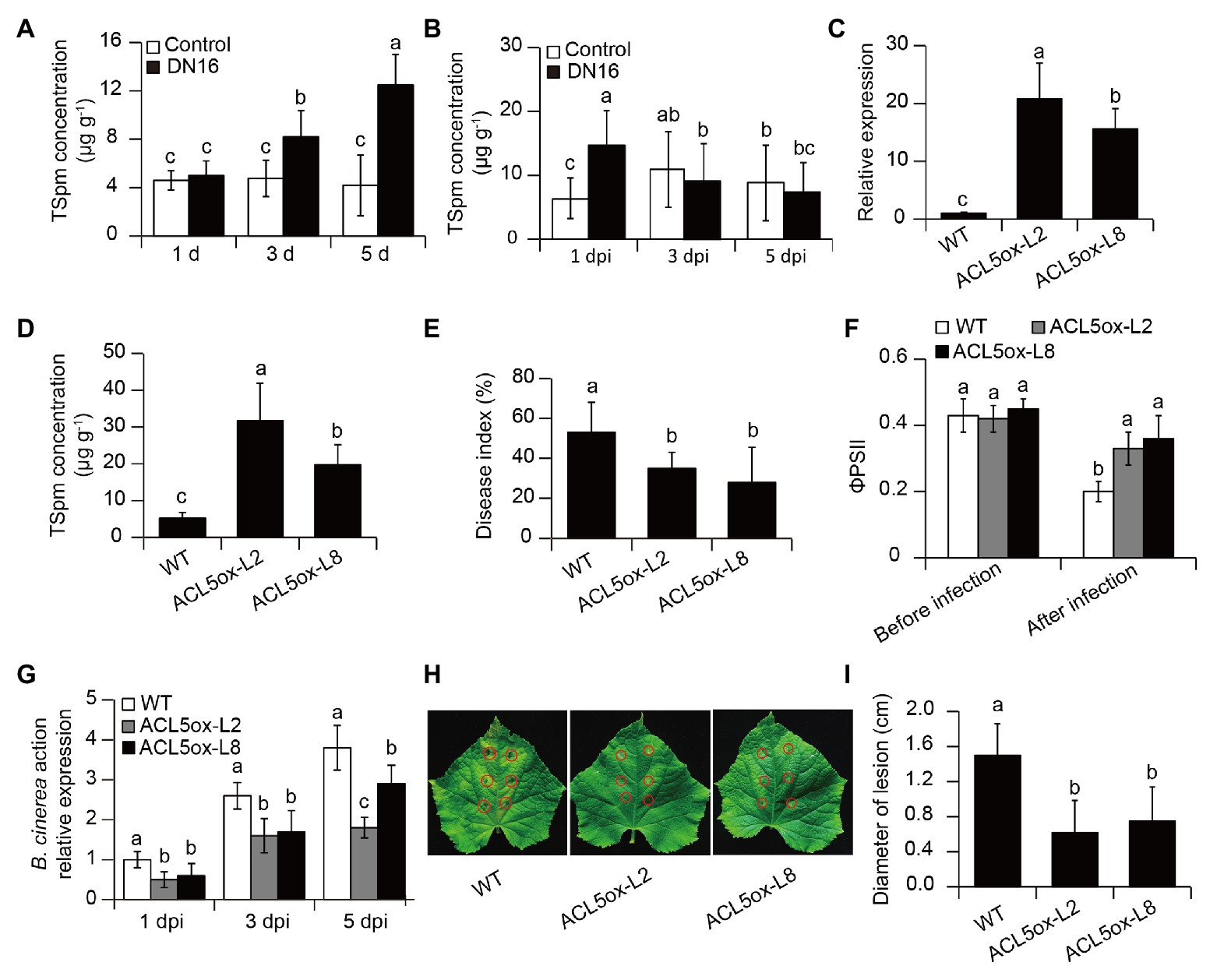
Figure 3. The contribution of thermospermine (TSpm) to P. fluorescens DN16-induced defense against B. cinerea infection. Five-week-old cucumber plants were treated with or without DN16, followed by B. cinerea infection. (A) After 1, 3, and 5 days of inoculation, the levels of TSpm were quantified in both the control and inoculated leaves. (B) The levels of TSpm in the control and inoculated leaves at 1, 3, and 5 dpi. (C) The expression of CsACL5 and (D) leaf TSpm levels in both the wild type (WT) and two transgenic lines (ACL5ox-L2 and -L8). Five-week-old cucumber plants were treated with B. cinerea, and disease index (E), ΦPSII (F), and the expression of BcActin (G) were determined, respectively. (H) Lesion symptoms and (I) diameters of detached leaves at 5 dpi. The data represented the means ± SD from three biological repeats and different letters indicated significant differences using Duncan’s multiple range tests (p < 0.05).
To further ascertain whether induction of CsACL5 transcripts in cucumber plants by DN16 was responsible for the DN16-mediated disease resistance, the RNAi-CsACL5 vector was introduced into the WT plants. qPCR analyses revealed a marked decrease in the transcription of CsACL5 of approximate 60% in two CsACL5-silenced lines (ACL5s-L3 and -L5) compared with the WT plants (Figure 4A). Consistently, leaf TSpm levels were significantly reduced in the CsACL5-silenced lines compared with the WT plants (Figure 4B). The inoculation with DN16 was not able to considerably augment the levels of TSpm in the leaves of the CsACL5-silenced lines (Figure 4B). Furthermore, we examined the effects of CsACL5 silencing on the DN16-mediated ISR. As shown in Figure 4C, silencing of CsACL5 greatly weakened the DN16-induced cucumber resistance against B. cinerea infection. At 7 dpi, the CsACL5-silenced lines displayed the increased disease index compared with the WT plants. qPCR analyses showed that the expression of BcActin was distinctly less in the leaves of the CsACL5-silenced lines than the WT plants (Figure 4D). Accordantly, detached leaves of the CsACL5-silenced lines exhibited more serious necrotic tissues and larger lesion diameters than the WT plants at 5 dpi (Figures 4E,F). Additionally, the WT plants had lower proportions of fewer small lesions (lesion diameter < 5 mm) than the CsACL5-silenced lines (Figure 4G).
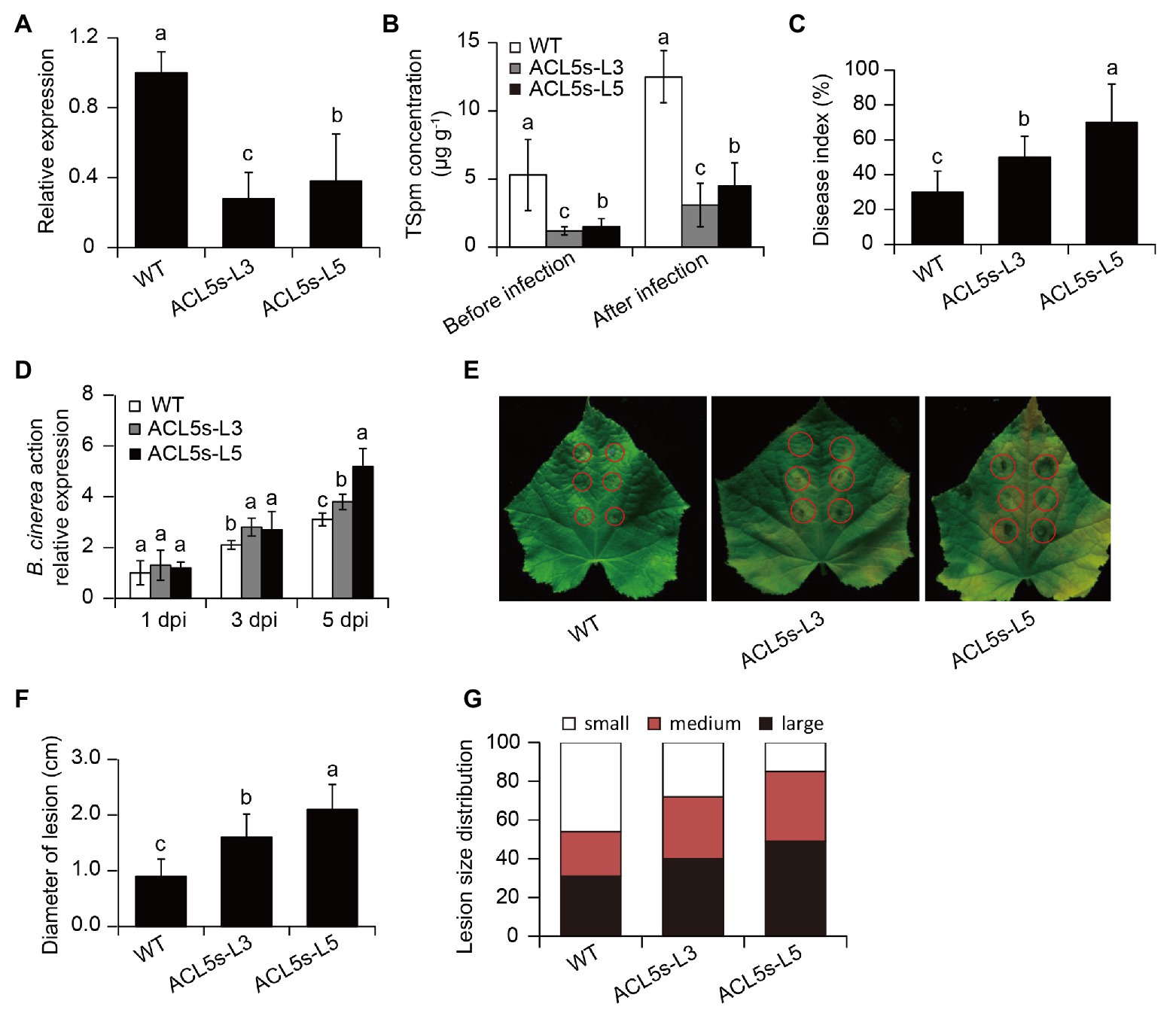
Figure 4. Silencing of CsACL5 increased the susceptibility of cucumber plants to B. cinerea infection. (A) The expression of CsACL5 in both the WT and two transgenic leaves (ACL5s-L3 and -L5). (B) Leaf TSpm content in both the WT and two transgenic lines before pathogen infection or after 5 days of pathogen infection. Five-week-old WT and transgenic lines were sprayed with B. cinerea, and (C) disease index and (D) the expression of BcActin were calculated at 1, 3, and 5 dpi, respectively. (E) Lesion symptoms, (F) diameters, and (G) size distribution of detached leaves at 5 dpi. The data represented the means ± SD from three biological repeats and different letters indicated significant differences Duncan’s multiple range tests (p < 0.05).
PA Catabolism Is Essential for DN16-Induced Resistance Against B. cinerea
Pathogen-mediated activation of defense responses is concomitant with the stimulated oxidation of cellular PAs in plants (Hatmi et al., 2014, 2015). The increased disease resistance is positively associated with the promoted PA catabolism in the pathogen-infected plants. In this study, the RNA-Seq data mentioned above showed that the transcription of PA catabolic genes (CsPAO1, CsPAO5, and CsCuAO1) was remarkably upregulated in the pathogen-infected leaves, but their expression was observably higher in the inoculated leaves. Furthermore, the PAO activities were measured in both the pathogen-infected control and inoculated leaves in the presence or absence of DN16. Before pathogen infection, there were no significant differences in the PAO and CuAO activities between the control and inoculated plants (Figure 5A). Upon exposure to B. cinerea infection, the activities of PAOs and CuAOs were progressively elevated followed by the time delay of pathogen infection. However, the inoculated plants had higher PA catabolic enzymatic activities than the controls (Figure 5B).
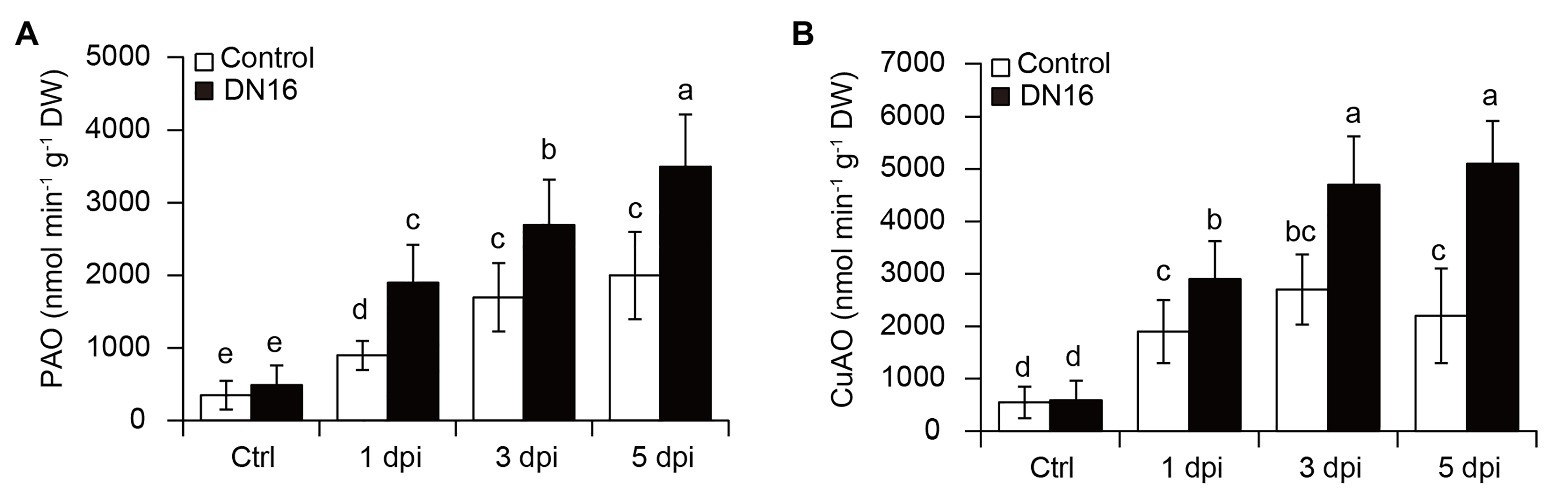
Figure 5. Changes of copper amine oxidase (CuAO) and polyamine oxidase (PAO) activities in both the control and DN16-inoculated leaves in response to B. cinerea infection. Detached leaves were treated with B. cinerea at the indicated times. (A) The activities of PAOs and (B) CuAOs were quantified in both the non-pathogen‐ (Ctrl) and pathogen-infected leaves in the absence or presence of DN16. The data represented the means ± SD from three biological repeats and different letters indicated significant differences using Duncan’s multiple range tests (p < 0.05).
We further examined whether the oxidation of TSpm was involved in the DN16-induced cucumber resistance against B. cinerea. For this purpose, both the control and inoculated plants were pre-treated with the PAO inhibitor HEH for 3 days, and were then infected with B. cinerea. The disease-resistant ability of plants was evaluated by observing the necrosis on the pathogen-infected leaves at 5 dpi. As shown in Figure 6A, the inoculation with DN16 enhanced leaf resistance against B. cinerea infection. The diameters of necrotic lesion were significantly smaller in the DN16-inoculated leaves than the controls (Figure 6B). However, foliar treatment with HEH resulted in the increased necrotic lesions. Accordantly, HEH treatment fully abrogated the DN16-induced disease resistance, displaying higher disease index (Figure 6C). Overexpression of CsACL5 also failed to the increased disease resistance of cucumber plants pre-treated with HEH. Moreover, qPCR analyses showed that the transcription levels of BcActin were remarkably higher in the HEH-treated leaves than the control leaves. Our findings suggested a pivotal role of TSpm oxidation in mediating the defense of cucumber plants against B. cinerea infection.
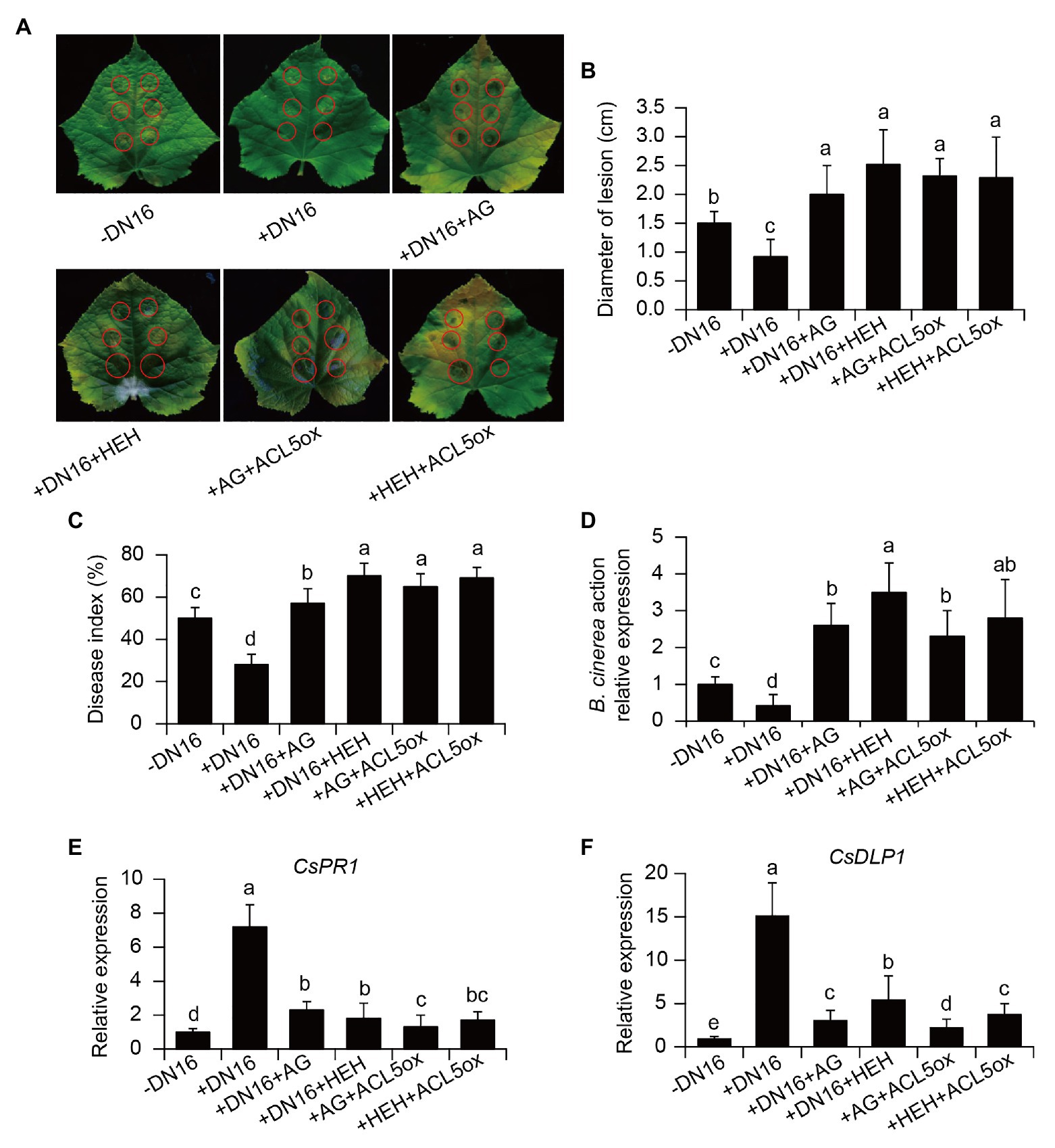
Figure 6. Effects of PA oxidation on the DN16-induced defense against B. cinerea. The control, inoculated, and ACL5-overexpressing plants were pre-treated with aminoguanidine (AG) or β-hydroxyehtylhydrazine (HEH) for 24 h. These plants were then subjected to B. cinerea infection. (A) Lesion symptoms and (B) diameters of detached leaves at 5 dpi. Disease index (C), the expression of BcActin (D), CsPR1 (E), and CsDLP1 (F) were determined in cucumber plants at 7 dpi. Control, −DN16; DN16-inoculated plants, +DN16; DN16-inoculated plants treated with AG (+DN16+AG) or HEH (+DN16+HEH); ACL5-overexpressing plants treated with AG (+AG+ACL5ox) or HEH (+HEH+ACL5ox). The data represented the means ± SD from three biological repeats and different letters indicated significant differences using Duncan’s multiple range tests (p < 0.05).
The PAO-mediated oxidation of TSpm is associated with the production of Put, which can be further oxidized by CuAOs. This indicated that the DN16-induced resistance to B. cinerea was likely resulted from the CuAO-mediated Put oxidation. As shown in Figures 6A–D, the function of CuAOs in the DNA16-mediated ISR was verified by the data that the CuAO inhibitor AG abrogated the DN16-induced cucumber resistance against pathogen infection. Similarly, overexpression of CsACL5 could not increase the resistance of cucumber plants against pathogen invasion when the plants were pre-treated with AG. In addition, pre-treatment with the AG or HEH failed to considerably elevate the transcription of defense-related genes including CsPR1 and CsDLP1 in both the DN16-inoculated and CsACL5-overexpressing plants after pathogen infection (Figures 6E,F). Therefore, these data indicated that the DN16-mediated enhancement of defense responses was closely related to the PAO‐ and CuAO-mediated oxidation of PAs.
Discussion
The present study showed that beneficial rhizobacterial strain P. fluorescens DN16 remarkably stimulated the synthesis of TSpm and its catabolism with obvious ramifications for the interactions between the foliar pathogen B. cinerea and cucumber plants. Specifically, the DN16-inoculated plants accumulated higher leaf TSpm levels than the controls. The transcription levels of the CsACL5 gene, encoding a putative TSpm synthase, were notably induced in the cucumber plants colonized by DN16. The increased TSpm level was positively correlated with upregulation of CsACL5 transcripts in the leaves of the inoculated plants. Suppression of B. cinerea infection in the inoculated plants with higher level of TSpm implied that this tetraamine conferred the enhanced host resistance against this pathogen. This hypothesis was further favored by the increased tolerance to B. cinerea displayed by the CsACL5-overexpressing lines. Moreover, the inoculated plants also displayed higher transcription of PA catabolic genes than the controls. Repression of the PAO and CuAO activities distinctly abrogated the DN16-mediated ISR in cucumber plants, indicating that the abilities of PA catabolic enzymes to oxidize TSpm were responsible for the TSpm-mediated defense responses in the DN16-inoculated plants. Our findings were in accordance with the data of a previous study, in which the PAO-mediated TSpm catabolism participates in modifying Arabidopsis defense against B. cinerea infection (Marina et al., 2013).
Thermospermine is a major type of PAs widely present in plant kingdom known to induce defense responses in plants (Jiménez-Bremont et al., 2014). TSpm exogenously supplied can enhance the resistance of Arabidopsis plants against P. viridiflava, which is also similar to the observation for Arabidopsis plants ectopically expressing AtACL5 (Marina et al., 2013). Mount evidence has indicated that genetic manipulation of ACL5 homologous genes or the increased TSpm levels can enhance defense responses in plants (Sagor et al., 2012; Mo et al., 2015a). Silencing of GhACL5 reduces the levels of TSpm and increases the susceptibility of plants to V. dahlia. In this study, it was worth noting that the transcription levels of CsACL5 were markedly increased in the DN16-inoculated plants. Consistently, the inoculated plants accumulated more leaf TSpm content than the controls. Similarly, overexpression of GhACL5 can increase the levels of TSpm in Arabidopsis plants (Mo et al., 2015a). We further verified whether the DN16-induced expression of CsACL5 contributed to the increased disease resistance in cucumber plants. Our results showed that overexpression of CsACL5 in cucumber plants led to higher accumulation of TSpm and stronger resistance to B. cinerea. Conversely, silencing of CsACL5 abolished the DN16-induced resistance of plants to B. cinerea infection. Collectively, these results indicated that the expression levels of CsACL5 were positively associated with the disease-resistant ability of cucumber plants to B. cinerea infection.
It has recently been indicated that overexpression of AtACL5 lead to no obvious changes of the TSpm levels in Arabidopsis plants compared with the controls, but enhances disease resistance, which is primarily attributable to activation of PAOs that can mediate the catabolism of TSpm (Marina et al., 2013). Interestingly, upon exposure to pathogen infection, there was no marked discrepancy in the leaf TSpm levels between the control and DN16-inoculated plants challenged with B. cinerea in spite of higher TSpm levels in the inoculated plants without pathogen infection. This finding prompted us to speculate that the attacks of B. cinerea may trigger the expression of several genes involved in the PA catabolism, thereby promoting the catabolism of TSpm. The enhanced transcription of CsPAO1, CsPAO5, and CsCuAO1 displayed by the pathogen-infected plants inoculated with DN16 implied that reduction of leaf TSpm levels in the inoculated plants were resulted from the enhanced activities of PA catabolic enzymes, probably participating in adjusting dynamic homeostasis of TSpm. Similar results were also observed in Arabidopsis plants, in which overexpression of AtACL5 significantly upregulates the expression levels of several PAO genes including PAO1, 3, and 5, indicating that these PAOs play important roles in controlling the level of TSpm (Marina et al., 2013).
Either abiotic stress or and B. cinerea infection can also enhance the activities of PAOs and defense responses in grapevine leaves (Hussain et al., 2011; Hatmi et al., 2014, 2015). Yoda et al. (2009) have shown that the tobacco mosaic virus (TMV)-infected tobacco plants display the augmented PAO activities with higher production of apoplastic H2O2 through the PA oxidation-related pathways. The PA oxidation further contributes to the enhanced defense responses of tobacco plants against TMV infection. Apoplastic PA oxidation has previously been shown to participate in plant defense against microbial pathogens (Marina et al., 2008). Gonzalez et al. (2011) have demonstrated that the increased Arabidopsis resistance to P. viridiflava regulated by Spm is at least partially dependent on the activities of PAOs. In this study, the inoculation with DN16 failed to increase the resistance of cucumber plants against B. cinerea infection after treatment with the PAO or CuAO inhibitor, indicating that the PAO‐ and CuAO-mediated TSpm catabolism was essential for the DN16-induced resistance against B. cinerea infection. In fact, either beneficial rhizobacteria or pathogens can induce priming in plants, which has been shown to effectively provoke defense responses. A faster and stronger activation of defense responses frequently occurs in the primed plants after subsequent invasion of pathogens (Heil and Bostock, 2002; Van der Ent et al., 2009; Romera et al., 2019). Previously, volatile organic compounds can be released by caterpillar-infested plants for inducing priming protective effects for earlier and stronger activation of subsequent defenses rather than to induce a direct activation of defense responses (Ton et al., 2006). Our data revealed that the inoculation with DN16 did not largely impact the expression of most defense-related genes, but the induced defense responses were markedly enhanced in the inoculated plants infected by B. cinerea. However, the priming effects were fully abolished in the inoculated plants treated with the PAO or CuAO inhibitor. These results confirmed that the TSpm-mediated priming was a more valid defense strategy, involving the DN16-induced cucumber resistance against B. cinerea.
Conclusion
Our study has unraveled an important role of beneficial rhizobacterial strains as a part of cucumber immune systems against B. cinerea. To our knowledge, these findings provide novel evidence for the tripartite interactions between rhizobacteria, plants, and foliar pathogen. Herein, a model was provided for the DN16-induced cucumber resistance against B. cinerea (Figure 7), whereby the biosynthesis of TSpm is substantially induced by DN16, resulting in higher leaf TSpm levels. Upon exposure to B. cinerea infection, the PAO and CuAO activities can be quickly activated to promote the catabolism of TSpm in the inoculated plants, thereby effectively provoking defense responses.
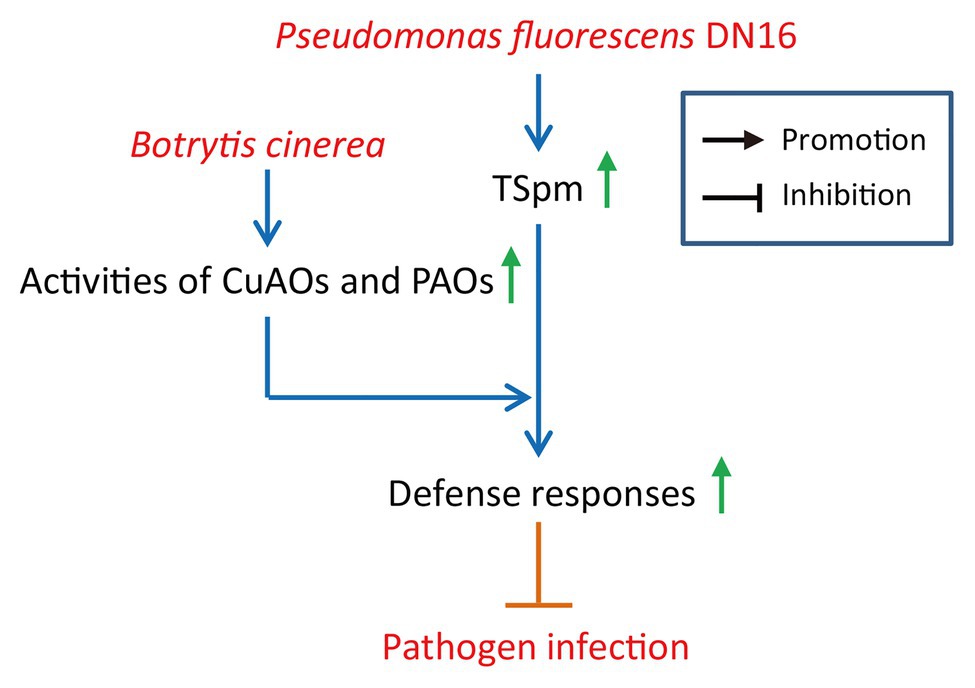
Figure 7. A proposed model for illustrating the functions of TSpm oxidation in the rhizobacteria-mediated cucumber resistance against B. cinerea. Pseudomonas fluorescens DN16 increased leaf TSpm levels in cucumber plants, but did not trigger the expression of pathogenesis-related genes, conferring a primed state of enhanced defense in cucumber plants. Upon exposure to B. cinerea, the catabolism of TSpm was quickly activated, involving the stimulation of the CuAO and PAO activities. This further contributed to the increased host resistance against pathogen infection.
Data Availability Statement
The datasets presented in this study can be found in online repositories. The names of the repository/repositories and accession number(s) can be found in the article/Supplementary Material.
Author Contributions
LQ and HD: conceptualization and supervision. LZ, NQ, and YS: investigation and formal analysis. LQ and LZ: funding acquisition. LZ and NQ: experiments. LZ, NQ, and XL: analysis of results. LZ, LQ, and XL: writing original draft. HD, YS, NQ, and LZ: review and editing. All authors contributed to the article and approved the submitted version.
Funding
This research was funded by the Natural Science Foundation of the Anhui Science and Technology Committee (1908085QC110), the National Undergraduate Training Program for Innovation and Entrepreneurship (201910879073), the Open Funds of the Anhui Province Key Laboratory of Farmland Ecological Conservation and Pollution Prevention (KLFECPP201802), the School-enterprise Cooperation Projects (ZHEP2019001), and the Natural Science Foundation of Education Department of Anhui province (KJ2020A0067).
Conflict of Interest
The authors declare that the research was conducted in the absence of any commercial or financial relationships that could be construed as a potential conflict of interest.
Supplementary Material
The Supplementary Material for this article can be found online at: https://www.frontiersin.org/articles/10.3389/fpls.2021.645338/full#supplementary-material
Footnotes
References
Angelini, R., Cona, A., Federico, R., Fincato, P., Tavladoraki, P., and Tisi, A. (2010). Plant amine oxidases “on the move”: an update. Plant Physiol. Biochem. 48, 560–564. doi: 10.1016/j.plaphy.2010.02.001
Block, A. K., Vaughan, M. M., Schmelz, E. A., and Christensen, S. A. (2019). Biosynthesis and function of terpenoid defense compounds in maize (Zea mays). Planta 249, 21–30. doi: 10.1007/s00425-018-2999-2
Cona, A., Rea, G., Angelini, R., Federico, R., and Tavladoraki, P. (2006). Functions of amine oxidases in plant development and defence. Trends Plant Sci. 11, 80–88. doi: 10.1016/j.tplants.2005.12.009
Curvers, K., Seifi, H., Mouille, G., de Rycke, R., Asselbergh, B., Van Hecke, A., et al. (2010). Abscisic acid deficiency causes changes in cuticle permeability and pectin composition that influence tomato resistance to Botrytis cinerea. Plant Physiol. 154, 847–860. doi: 10.1104/pp.110.158972
Daudi, A., and O’Brien, J. A. (2012). Detection of hydrogen peroxide by DAB staining in Arabidopsis leaves. Bio Protoc. 2:e263. doi: 10.21769/BioProtoc.263
El Oirdi, M., Abd El Rahman, T., Rigano, L., El Hadrami, A., Rodriguez, M. C., Daayf, F., et al. (2011). Botrytis cinerea manipulates the antagonistic effects between immune pathways to promote disease development in tomato. Plant Cell 23, 2405–2421. doi: 10.1105/tpc.111.083394
Fahad, S., Hussain, S., Bano, A., Saud, S., Hassan, S., Shan, D., et al. (2015). Potential role of phytohormones and plant growth-promoting rhizobacteria in abiotic stresses: consequences for changing environment. Environ. Sci. Pollut. Res. Int. 22, 4907–4921. doi: 10.1007/s11356-014-3754-2
Fuell, C., Elliott, K. A., Hanfrey, C. C., Franceschetti, M., and Michael, A. J. (2010). Polyamine biosynthetic diversity in plants and algae. Plant Physiol. Biochem. 48, 513–520. doi: 10.1016/j.plaphy.2010.02.008
Gonzalez, M. E., Marco, F., Minguet, E. G., Carrasco-Sorli, P., Blazquez, M. A., Carbonell, J., et al. (2011). Perturbation of spermine synthase gene expression and transcript profiling provide new insights on the role of the tetraamine spermine in Arabidopsis defense against Pseudomonas viridiflava. Plant Physiol. 156, 2266–2277. doi: 10.1104/pp.110.171413
Hatmi, S., Gruau, C., Trotel-Aziz, P., Villaume, S., Rabenoelina, F., Baillieul, F., et al. (2015). Drought stress tolerance in grapevine involves activation of polyamine oxidation contributing to improved immune response and low susceptibility to Botrytis cinerea. J. Exp. Bot. 66, 775–787. doi: 10.1093/jxb/eru436
Hatmi, S., Trotel-Aziz, P., Villaume, S., Couderchet, M., Clément, C., and Aziz, A. (2014). Osmotic stress-induced polyamine oxidation mediates defence responses and reduces stress-enhanced grapevine susceptibility to Botrytis cinerea. J. Exp. Bot. 65, 75–88. doi: 10.1093/jxb/ert351
Heil, M., and Bostock, R. M. (2002). Induced systemic resistance (ISR) against pathogens in the context of induced plant defences. Ann. Bot. 89, 503–512. doi: 10.1093/aob/mcf076
Hu, Z., Shao, S., Zheng, C., Sun, Z., Shi, J., Yu, J., et al. (2018). Induction of systemic resistance in tomato against Botrytis cinerea by N-decanoyl-homoserine lactone via jasmonic acid signaling. Planta 247, 1217–1227. doi: 10.1007/s00425-018-2860-7
Hussain, S. S., Ali, M., Ahmad, M., and Siddique, K. H. (2011). Polyamines: natural and engineered abiotic and biotic stress tolerance in plants. Biotechnol. Adv. 29, 300–311. doi: 10.1016/j.biotechadv.2011.01.003
Jiménez-Bremont, J. F., Marina, M., Guerrero-González Mde, L., Rossi, F. R., Sánchez-Rangel, D., Rodríguez-Kessler, M., et al. (2014). Physiological and molecular implications of plant polyamine metabolism during biotic interactions. Front. Plant Sci. 5:95. doi: 10.3389/fpls.2014.00095
Jing, N., Cui, J., Shi, Y., Yang, G., Zhou, X., Hou, X., et al. (2019). Tomato lncRNA23468 functions as a competing endogenous RNA to modulate NBS-LRR genes by decoying miR482b in the tomato-Phytophthora infestans interaction. Hortic. Res. 6:28. doi: 10.1038/s41438-018-0096-0
Kakehi, J., Kuwashiro, Y., Niitsu, M., and Takahashi, T. (2018). Thermospermine is required for stem elongation in Arabidopsis thaliana. Plant Cell Physiol. 49, 1342–1349. doi: 10.1093/pcp/pcn109
Kim, D. W., Watanabe, K., Murayama, C., Izawa, S., Niitsu, M., Michael, A. J., et al. (2014). Polyamine oxidase5 regulates Arabidopsis growth through thermospermine oxidase activity. Plant Physiol. 165, 1575–1590. doi: 10.1104/pp.114.242610
Lugtenberg, B., and Kamilova, F. (2009). Plant-growth-promoting rhizobacteria. Annu. Rev. Microbiol. 63, 541–556. doi: 10.1146/annurev.micro.62.081307.162918
Marina, M., Maiale, S. J., Rossi, F. R., Romero, M. F., Rivas, E. I., Gárriz, A., et al. (2008). Apoplastic polyamine oxidation plays different roles in local responses of tobacco to infection by the necrotrophic fungus Sclerotinia sclerotiorum and the biotrophic bacterium Pseudomonas viridiflava. Plant Physiol. 147, 2164–2178. doi: 10.1104/pp.108.122614
Marina, M., Sirera, F. V., Rambla, J. L., Gonzalez, M. E., Blázquez, M. A., Carbonell, J., et al. (2013). Thermospermine catabolism increases Arabidopsis thaliana resistance to Pseudomonas viridiflava. J. Exp. Bot. 64, 1393–1402. doi: 10.1093/jxb/ert012
Mo, H., Wang, X., Zhang, Y., Yang, J., and Ma, Z. (2015a). Cotton ACAULIS5 is involved in stem elongation and the plant defense response to Verticillium dahliae through thermospermine alteration. Plant Cell Rep. 34, 1975–1985. doi: 10.1007/s00299-015-1844-3
Mo, H., Wang, X., Zhang, Y., Zhang, G., Zhang, J., and Ma, Z. (2015b). Cotton polyamine oxidase is required for spermine and camalexin signalling in the defence response to Verticillium dahliae. Plant J. 83, 962–975. doi: 10.1111/tpj.12941
Moschou, P. N., Sanmartin, M., Andriopoulou, A. H., Rojo, E., SanchezSerrano, J. J., and Roubelakis-Angelakis, K. A. (2008). Bridging the gap between plant and mammalian polyamine catabolism: a novel peroxisomal polyamine oxidase responsible for a full back-conversion pathway in Arabidopsis. Plant Physiol. 147, 1845–1857. doi: 10.1104/pp.108.123802
Moschou, P. N., Sarris, P. F., Skandalis, N., Andriopoulou, A. H., Paschalidis, K. A., Panopoulos, N. J., et al. (2009). Engineered polyamine catabolism preinduces tolerance of tobacco to bacteria and oomycetes. Plant Physiol. 149, 1970–1981. doi: 10.1104/pp.108.134932
Muñiz, L., Minguet, E. G., Singh, S. K., Pesquet, E., Vera-Sirera, F., Moreau-Courtois, C. L., et al. (2008). ACAULIS5 controls Arabidopsis xylem specification through the prevention of premature cell death. Development 135, 2573–2582. doi: 10.1242/dev.019349
Nadeem, S. M., Ahmad, M., Zahir, Z. A., Javaid, A., and Ashraf, M. (2014). The role of mycorrhizae and plant growth promoting rhizobacteria (PGPR) in improving crop productivity under stressful environments. Biotechnol. Adv. 32, 429–448. doi: 10.1016/j.biotechadv.2013.12.005
Nambeesan, S., AbuQamar, S., Laluk, K., Mattoo, A. K., Mickelbart, M. V., Ferruzzi, M. G., et al. (2012). Polyamines attenuate ethylene-mediated defense responses to abrogate resistance to Botrytis cinerea in tomato. Plant Physiol. 158, 1034–1045. doi: 10.1104/pp.111.188698
Rea, G., Metoui, O., Infantino, A., Federico, R., and Angelini, R. (2002). Copper amine oxidase expression in defense responses to wounding and Ascochyta rabiei invasion. Plant Physiol. 128, 865–875. doi: 10.1104/pp.010646
Romera, F. J., García, M. J., Lucena, C., Martínez-Medina, A., Aparicio, M. A., Ramos, J., et al. (2019). Induced systemic resistance (ISR) and Fe deficiency responses in dicot plants. Front. Plant Sci. 10:287. doi: 10.3389/fpls.2019.00287
Sagor, G. H., Takahashi, H., Niitsu, M., Takahashi, Y., Berberich, T., and Kusano, T. (2012). Exogenous thermospermine has an activity to induce a subset of the defense genes and restrict cucumber mosaic virus multiplication in Arabidopsis thaliana. Plant Cell Rep. 31, 1227–1232. doi: 10.1007/s00299-012-1243-y
Sen, S., Ghosh, D., and Mohapatra, S. (2018). Modulation of polyamine biosynthesis in Arabidopsis thaliana by a drought mitigating Pseudomonas putida strain. Plant Physiol. Biochem. 129, 180–188. doi: 10.1016/j.plaphy.2018.05.034
Shi, H., and Chan, Z. (2014). Improvement of plant abiotic stress tolerance through modulation of the polyamine pathway. J. Integr. Plant Biol. 56, 114–121. doi: 10.1111/jipb.12128
Shinohara, S., Okamoto, T., Motose, H., and Takahashi, T. (2019). Salt hypersensitivity is associated with excessive xylem development in a thermospermine-deficient mutant of Arabidopsis thaliana. Plant J. 100, 374–383. doi: 10.1111/tpj.14448
Takahashi, Y., Uehara, Y., Berberich, T., Ito, A., Saitoh, H., Miyazaki, A., et al. (2004). A subset of hypersensitive response marker genes, including HSR203J, is the downstream target of a spermine signal transduction pathway in tobacco. Plant J. 40, 586–595. doi: 10.1111/j.1365-313X.2004.02234.x
Takano, A., Kakehi, J., and Takahashi, T. (2012). Thermospermine is not a minor polyamine in the plant kingdom. Plant Cell Physiol. 53, 606–616. doi: 10.1093/pcp/pcs019
Tian, X., Song, L., Wang, Y., Jin, W., Tong, F., and Wu, F. (2018). miR394 acts as a negative regulator of Arabidopsis resistance to B. cinerea infection by targeting LCR. Front. Plant Sci. 9:903. doi: 10.3389/fpls.2018.00903
Ton, J., D’Alessandro, M., Jourdie, V., Jakab, G., Karlen, D., Held, M., et al. (2006). Priming by airborne signals boosts direct and indirect resistance in maize. Plant J. 49, 16–26. doi: 10.1111/j.1365-313X.2006.02935.x
Van der Ent, S., Van Wees, S. C., and Pieterse, C. M. (2009). Jasmonate signaling in plant interactions with resistance-inducing beneficial microbes. Phytochemistry 70, 1581–1588. doi: 10.1016/j.phytochem.2009.06.009
van Loon, L. C., Rep, M., and Pieterse, C. M. (2006). Significance of inducible defense-related proteins in infected plants. Annu. Rev. Phytopathol. 44, 135–162. doi: 10.1146/annurev.phyto.44.070505.143425
Vuosku, J., Muilu-Mäkelä, R., Avia, K., Suokas, M., Kestilä, J., Läärä, E., et al. (2019). Thermospermine synthase (ACL5) and diamine oxidase (DAO) expression is needed for zygotic embryogenesis and vascular development in scots pine. Front. Plant Sci. 10:1600. doi: 10.3389/fpls.2019.01600
Walters, D. R. (2003). Polyamines and plant disease. Phytochemistry 64, 97–107. doi: 10.1016/S0031-9422(03)00329-7
Wang, W., Paschalidis, K., Feng, J. C., Song, J., and Liu, J. H. (2009). Polyamine catabolism in plants: a universal process with diverse functions. Front. Plant Sci. 10:561. doi: 10.3389/fpls.2019.00561
Wang, H., Sui, X., Guo, J., Wang, Z., Cheng, J., Ma, S., et al. (2014). Antisense suppression of cucumber (Cucumis sativus L.) sucrose synthase 3 (CsSUS3) reduces hypoxic stress tolerance. Plant Cell Environ. 37, 795–810. doi: 10.1111/pce.12200
Yang, S., Wen, C., Liu, B., Cai, Y., Xue, S., Bartholomew, E. S., et al. (2019). A CsTu-TS1 regulatory module promotes fruit tubercule formation in cucumber. Plant Biotechnol. J. 17, 289–301. doi: 10.1111/pbi.12977
Yoda, H., Fujimura, K., Takahashi, H., Munemura, I., Uchimiya, H., and Sano, H. (2009). Polyamines as a common source of hydrogen peroxide in host and nonhost hypersensitive response during pathogen infection. Plant Mol. Biol. 70, 103–112. doi: 10.1007/s11103-009-9459-0
Zhang, W., Zhang, H., Qi, F., and Jian, G. (2016). Generation of transcriptome profiling and gene functional analysis in Gossypium hirsutum upon Verticillium dahliae infection. Biochem. Biophys. Res. Commun. 473, 879–885. doi: 10.1016/j.bbrc.2016.03.143
Zhou, C., Ma, Z., Zhu, L., Xiao, X., Xie, Y., Zhu, J., et al. (2016). Rhizobacterial strain Bacillus megaterium BOFC15 induces cellular polyamine changes that improve plant growth and drought resistance. Int. J. Mol. Sci. 17:976. doi: 10.3390/ijms17060976
Keywords: thermospermine oxidation, defense response, Botrytis cinerea, rhizobacteria, polyamine
Citation: Zhu L, Qian N, Sun Y, Lu X, Duan H and Qian L (2021) Pseudomonas fluorescens DN16 Enhances Cucumber Defense Responses Against the Necrotrophic Pathogen Botrytis cinerea by Regulating Thermospermine Catabolism. Front. Plant Sci. 12:645338. doi: 10.3389/fpls.2021.645338
Edited by:
Carlos Lucena, University of Cordoba, SpainReviewed by:
Kang Qiao, Shandong Agricultural University, ChinaJie Wang, Tobacco Research Institute (CAAS), China
Copyright © 2021 Zhu, Qian, Sun, Lu, Duan and Qian. This is an open-access article distributed under the terms of the Creative Commons Attribution License (CC BY). The use, distribution or reproduction in other forums is permitted, provided the original author(s) and the copyright owner(s) are credited and that the original publication in this journal is cited, in accordance with accepted academic practice. No use, distribution or reproduction is permitted which does not comply with these terms.
*Correspondence: Lisheng Qian, cWlhbmxzQGFoc3R1LmVkdS5jbg==; Haiming Duan, ZHVhbmhtQGFoc3R1LmVkdS5jbg==