- 1Changins College for Viticulture and Oenology, University of Sciences and Art Western Switzerland, Nyon, Switzerland
- 2Unité de recherche Œnologie EA 4577, USC 1366 INRAE, Bordeaux, France
- 3Institut des Sciences de la Vigne et du Vin CS 50008, Villenave d'Ornon, France
- 4College of Science & Engineering, Flinders University, Bedford Park, SA, Australia
- 5Agriculture and Food (Commonwealth Scientific and Industrial Research Organisation), Glen Osmond, SA, Australia
- 6Department of Agronomy, Food, Natural Resources, Animals and Environment, University of Padova Agripolis, Legnaro, Italy
- 7Dipartimento di Scienze Agrarie, Alimentari e Ambientali, Università degli Studi di Perugia, Perugia, Italy
- 8Faculty of Land and Food Systems, Wine Research Centre, The University of British Columbia, Vancouver, BC, Canada
Temperature, water, solar radiation, and atmospheric CO2 concentration are the main abiotic factors that are changing in the course of global warming. These abiotic factors govern the synthesis and degradation of primary (sugars, amino acids, organic acids, etc.) and secondary (phenolic and volatile flavor compounds and their precursors) metabolites directly, via the regulation of their biosynthetic pathways, or indirectly, via their effects on vine physiology and phenology. Several hundred secondary metabolites have been identified in the grape berry. Their biosynthesis and degradation have been characterized and have been shown to occur during different developmental stages of the berry. The understanding of how the different abiotic factors modulate secondary metabolism and thus berry quality is of crucial importance for breeders and growers to develop plant material and viticultural practices to maintain high-quality fruit and wine production in the context of global warming. Here, we review the main secondary metabolites of the grape berry, their biosynthesis, and how their accumulation and degradation is influenced by abiotic factors. The first part of the review provides an update on structure, biosynthesis, and degradation of phenolic compounds (flavonoids and non-flavonoids) and major aroma compounds (terpenes, thiols, methoxypyrazines, and C13 norisoprenoids). The second part gives an update on the influence of abiotic factors, such as water availability, temperature, radiation, and CO2 concentration, on berry secondary metabolism. At the end of the paper, we raise some critical questions regarding intracluster berry heterogeneity and dilution effects and how the sampling strategy can impact the outcome of studies on the grapevine berry response to abiotic factors.
Grape Berry Phenolics
Phenolic compounds constitute a large group of secondary metabolites, which are produced via different branches of the phenylpropanoid pathway. They are widely distributed throughout the plant kingdom and function as pigments, antioxidants, signaling molecules, structural elements, and components of defense mechanisms (Rienth et al., 2019; Santos-Sánchez et al., 2019).
They are composed of a phenyl ring backbone with a hydroxyl group or other substitutes and are generally classed into non-flavonoids and flavonoids. Non-flavonoids consist of simple C6 backbone phenolics such as hydroxybenzoic acids, hydroxycinnamic acids, and volatile phenols, and C6-C2-C6 backbone compounds such as stilbenes. Flavonoid compounds that occur in grapes comprise flavones, flavonols, flavanones, flavan-3-ols, and anthocyanins (Figure 1).
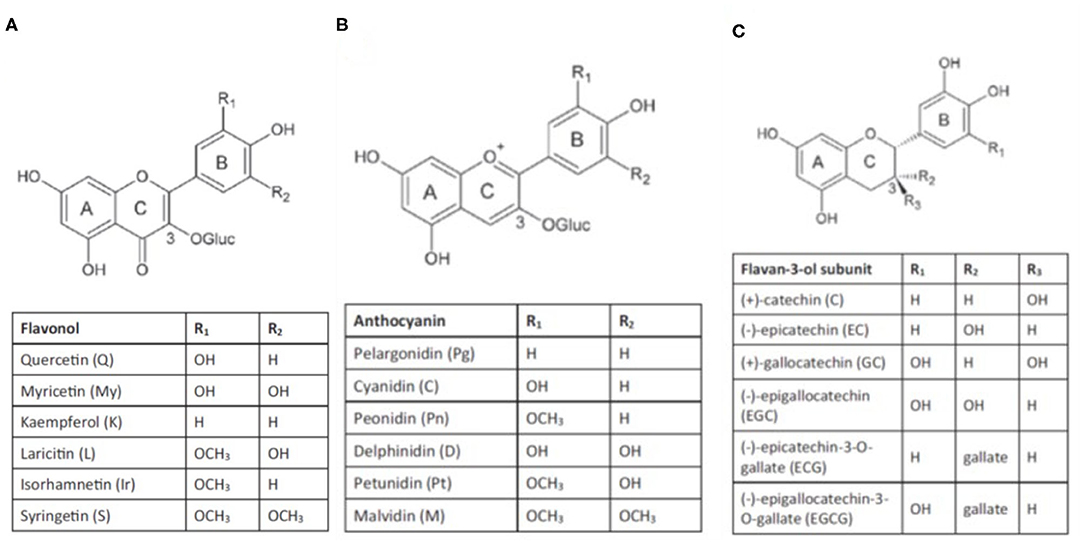
Figure 1. Structure of flavonols (A), anthocyanins (B) and flavan-3-ols (C) (from Gouot et al., 2018).
Phenolic compounds are synthesized via the general phenylpropanoid pathway (GPP) and its downstream reactions. After the generation of intermediates in the GPP, the carbon flow is directed into specific branch pathways to produce flavonoids, stilbenes, and phenolic acids (Deng and Lu, 2017). The starting point of the GPP is the aromatic amino acid phenylalanine, a product of the shikimate pathway, which constitutes a major link between primary and secondary metabolism in vascular plants (Santos-Sánchez et al., 2019).
The GPP leads to the production of p-coumaroyl-CoA from phenylalanine, via reactions catalyzed by phenylalanine ammonia lyase (PAL), cinnamate-4-hydroxylase (C4H), and 4-coumarate-CoA ligase (4CL). Further downstream p-coumaroyl-CoA and malonyl-CoA are catalyzed by chalcone synthase (CHS) and stilbene synthase (STS) in the first steps of the flavonoid and stilbenoid pathway, respectively.
Recently, two transcription factors (TFs), VviMYB4a and VviMYB4b, have been characterized as negative regulators of phenylpropanoid and hydrocinnamic acid synthesis (Cavallini et al., 2015) and can repress upstream genes of the phenylpropanoid biosynthesis pathway in berry skins (Muñoz et al., 2019).
Non-flavonoid Phenolics
The concentrations of non-flavonoid phenolic compounds present in grapes and wines are relatively low (25–60 mg L−1), with the exception of hydroxycinnamic acids (150–200 mg L−1) (Kennedy et al., 2006). They are principally located in the berry pulp and are the major phenolic compounds in berries of white cultivars, although they reach similar magnitudes in reds (Kennedy et al., 2006).
The major non-flavonoid compounds present in grapes are the hydroxycinnamic acids: p-coumaric acid, caffeic acid, ferulic acid, and their esterified forms, coutaric, caftaric, and fertaric acid (Zhang et al., 2013). Their biosynthesis occurs during the first phase of berry growth until the lag phase (herbaceous plateau) and is catalyzed by caffeic acid 3-O-metyltransferase (COMT) and caffeoyl-CoA 3-O-methyltransferase (CCoAOMT) downstream of the GPP. Although the accumulation occurs predominantly in the flesh, they are present in all berry tissues (Cadot et al., 2006; Braidot et al., 2008). In hypodermal, mesocarp, and placental cells of the pulp, hydroxycinnamates may be conjugated with anthocyanins (Cadot et al., 2006; Conde et al., 2007; Castellarin et al., 2012; Kuhn et al., 2013). Minor non-flavonoid compounds comprise hydroxybenzoic acids that are present in grapes in much lower concentrations than hydroxycinnamic acids, and include gentisic acid, salicylic acid, gallic acid, and p-hydroxybenzoic acid (Vanhoenacker et al., 2001; Pozo-Bayon et al., 2003; Ali et al., 2010). The most represented hydroxybenzoic acid is gallic acid, which is present in both free form or, in the seed, as an acyl substituent of flavan-3-ols (described below) (Adams, 2006).
Grape stilbenoids include cis- and trans-resveratrol, piceatannol, cis- and trans-piceid, astringin, pallidol and α-, β-, γ-, δ-, ε-viniferin. Although stilbenoids are present in trace quantities in wine, they have been drawing increasing attention due to their potential health benefits (Akinwumi et al., 2018). They have been shown to protect the plant against various pathogens and have been detected in significant concentrations in leaves and berries of highly stressed vines and disease-tolerant Vitis species such V. amurensis (Kiselev et al., 2017). Therefore, they represent important biomarkers and a molecular target for breeding strategies aiming to develop disease-resistant cultivars (Gindro et al., 2012; Viret et al., 2018). Recent research seeking alternatives to pesticides highlighted the potential of stilbenes extracted from wooden grapevine tissue as natural fungitoxic substances (Schnee et al., 2008; Biais et al., 2017).
Stilbenes are mostly accumulated in berries after the onset of ripening (véraison) (Gatto et al., 2008), and the regulation of their biosynthesis is strongly modulated by both biotic and abiotic factors (Vannozzi et al., 2012; Savoi et al., 2017). The concentration of stilbenes in grapes is variety-dependent; generally, red grapes have higher stilbene levels than white grapes (Bavaresco et al., 2016). Reported levels in grapes range from 0.2 to 1.8 mg kg−1 fresh weight of healthy grapes for cultivars that were classified as low stilbene producers (e.g., Nebbiolo or Aglianico) but can reach levels up to 33.4 mg kg-1 in high stilbene producers (> 2.3 mg kg−1) such as Pinot Noir (Gatto et al., 2008) or Syrah (Favre et al., 2020).
Stilbenes can be glycosylated or methylated. The stilbene with the simplest molecular structure is resveratrol, which exists as a trans or cis isomer (Kiselev et al., 2017). Resveratrol has been associated with the so called “French paradox,” where the rather low risk of cardiovascular disease within the French population despite of a high intake of saturated fat was attributed to relatively high resveratrol intake through wine consumption (Renaud and de Lorgeril, 1992). In subsequent studies, resveratrol was designated the main substance amongst other phenolic compounds accountable for such protective effects (Pastor et al., 2019). Its anticancer, anti-inflammatory, anticarcinogenic, cardioprotective, vasorelaxant, phytoestrogenic, and neuroprotective properties have been reported in numerous studies (Guerrero et al., 2009; Chalons et al., 2018; Ramírez-Garza et al., 2018; Salehi et al., 2018; Wurz, 2019).
Resveratrol is the precursor for other stilbenoids such as piceids, trans- and cis-resveratrol-3-O-β-D-glucopyranosidade, and astringin. Pterostilbene (3,5-dimethoxy-4′-hydroxystilbene), which exhibits an enhanced antifungal activity compared to the non-methylated stilbenoid forms, is formed by the addition of two methyl groups to resveratrol (Chong et al., 2009). The oxidation of resveratrol produces oligomers called viniferins. The most important viniferins are α-, β-, γ-, δ-, and ε-viniferin, consisting essentially of cyclic oligomers of resveratrol (Castellarin et al., 2012).
In the grapevine genome, forty-five stilbene synthases genes (STS), the key enzyme of resveratrol biosynthesis, have been described, of which at least 33 encode full-length proteins. Multiple tandem and segmental duplication events led to the rise of this gene family (Vannozzi et al., 2012). Many of those VviSTSs exhibit changing expression pattern during fruit development and ripening as shown by transcriptomic analysis (Massonnet et al., 2017). In red berry cultivars, the expression of VviSTSs is increased during the late stages of ripening, consistently with the expression of two R2R3 MYB TFs, VviMYB14 and VviMYB15 (Holl et al., 2013), which are known to regulate stilbene biosynthesis. Amongst the many TFs proposed to regulate this pathway (Wong et al., 2016; Vannozzi et al., 2018) the two WRKY TFs, VviWRKY24 and VviWRKY03, contribute at different levels to VviSTS regulation. This occurs by a direct activation of VviSTSs and synergistic action with MYB TFs. Recently, Jiang et al. (2019) indicated that VvWRKY8 represses VvSTS15/21 expression and thus stilbene biosynthesis through the interaction with VvMYB14.
Flavonoid Phenolics
Flavonoids make up a significant proportion of phenolic compounds in red grapes (1,000–1,800 mg L−1) and can be considered as the most important quality-determining compounds in red wines as they contribute to color, flavor, texture, and astringency. Flavonoids are C6–C3–C6 polyphenolic compounds, where the two hydroxylated benzene rings are joined by a three-carbon chain. According to the oxidation state of the C3 ring, these compounds are divided into flavonols, flavan-3-ols (which include simple flavan-3-ols and their polymeric forms proanthocyanidins, also known as tannins), and anthocyanins (Castellarin et al., 2012). Flavonoids are mainly localized in both the peripheral layers of the berry pericarp (skin) and in the seed coat (Teixeira et al., 2013).
The flavonoid pathway has been well-characterized in grapevine. Most of the genes encoding structural elements of the flavonoid pathway are present in low copy numbers except genes coding flavonoid-3′,5′-hydroxylases (F3′5′H′s). p-Coumaroyl-CoA and malonyl-CoA are the substrates of chalcone synthase (CHS), which catalyzes the first step of flavonoid synthesis. Subsequently, naringenin chalcone is transformed to naringenin flavanone by chalcone isomerase (CHI) and to dihydrokaempferol by flavonoid-3-hydrolase (F3H). The pathway is then divided into two major branches catalyzed by two flavonoid hydroxylases, flavonoid-3′-hydroxylase (F3′H) and flavonoid-3′5′-hydroxylase (F3′5′H), which generate di-hydroxylated and tri-hydroxylated flavonoids, respectively (Azuma, 2018). In the grapevine genome a proliferation of the F3′5′Hs has occurred, giving rise to 15 paralogs that are predominantly expressed in grapes (Falginella et al., 2010, 2012).
Flavonols, such as kaempferol, quercetin, myricetin, isorhamnetin, laricitrin, and syringetin are synthesized by flavonol synthases (FLSs) (Downey et al., 2004), regulated by a light-induced TF (VviMYBF1/VviMYB12) (Czemmel et al., 2009). Two studies showed that three additional bZIP TFs, VviHYH, VviHY5, and VvibZIPC22, contribute to the regulation of flavonol synthases and the accumulation of flavonol in berries (Malacarne et al., 2015; Loyola et al., 2016). The TF VviMYBF1 is part of a regulatory cascade of VviHY5/HYH that potentially involves positive feedback (Loyola et al., 2016; Czemmel et al., 2017). Flavonols are glycosylated by flavonol-3-O-glycosyltransferases (VviGT3-5-6) and flavonol-3-O-rhamnosyltransferase (VviRhaT1) and are present in the berry as galactosides, rhamnosides, rutinosides, and glucuronides (Ono et al., 2010; Czemmel et al., 2017).
Flavan-3-ols are synthesized from anthesis until the lag phase via leucoanthocyanidin reductases (LAR1-2) or an anthocyanidin reductase (ANR) (Bogs et al., 2005), which are regulated by TFs of the MYB family. In particular, VviLAR1 and VviANR are under the control of VviMYBPA1 and VviMYBPA2 (Bogs et al., 2007; Terrier et al., 2009), whereas VviLAR2 is regulated by VviMYBPAR (Koyama et al., 2014). In grapes, the main monomeric flavan-3-ols are (+)-catechin, (-)-epicatechin, (-)-epicatechin-3-O-gallate, (+)-gallocatechin and (-)-epigallocatechin (Mattivi et al., 2009), and are the building blocks for pro-anthocyanidins (condensed tannins). The mechanisms involved in tannin polymerization, galloylation, and transport into the vacuoles are not well-elucidated (Zhao et al., 2010). Three different glycosyltransferases (VviGT1-3) are ostensibly involved in the synthesis of hydroxycinnamic esters and in the galloylation of proanthocyanidins (Khater et al., 2012), and two specific transporters of proanthocyanidin have been identified (VviPAMATE1-2) (Perez-Diaz et al., 2014).
Anthocyanins are the pigments that are responsible for the color of red grapes and wines. They are synthesized in the epidermal and hypodermal cells of the berry skin and stored in the cell vacuoles from the onset of ripening (véraison). Few red cultivars, commonly called by the French word “Teinturier”, accumulate anthocyanins also in the flesh (Ageorges et al., 2006; Castellarin et al., 2011; Falginella et al., 2012).
In Vitis vinifera, anthocyanins are glycosylated at the 3′ position. They can be substituted with two (di-oxygenated: cyanidin- and peonidin-3-O-glucosides) or three (tri-oxygenated: delphinidin-, petunidin-, and malvidin-3-O-glucosides) hydroxyl (-OH) and/or methoxyl (-OCH3) groups in the side-ring (B) of the flavonoid structure. Glycosylation occurs via the activity of the enzyme UDP-glucose, flavonoid-3-O-glucosyltransferase (UFGT) (Boss et al., 1996a,b). Anthocyanin-O-methyl transferases (VviAOMT1-3) methylate cyanidin-3-O-glucoside and delphinidin-3-O-glucoside into peonidin-3-O-glucoside, petunidin-3-O-glucoside, and malvidin-3-O-glucoside (Fournier-Level et al., 2011). Most anthocyanins can be acylated at the 6′ position of the glucose mediated by an anthocyanin-3-O-glucoside-6′-O-acyltransferase (Vvi3AT) to produce 3-O-6′-acetyl-, 3-O-6′-coumaroyl- and 3-O-6′-caffeoyl-monoglucosides (Rinaldo et al., 2015). Anthocyanins composition (the relative portion of individual anthocyanins, the ratio of di-oxygenated vs. tri-oxygenated side-ring forms, the ratio of acylated vs. non-acylated derivatives, etc.) is variable among grapevine cultivars (Monagas et al., 2003; Theodorou et al., 2019) and can be modified by abiotic factors as discussed in later sections.
The two TFs VviMYBA1 and A2 are the pivotal genetic determinants of berry anthocyanin synthesis (Kobayashi et al., 2004; Walker et al., 2007). Recently it has been shown that VviMYBA6 and VviMYBA7, additional MYBA family members, regulate anthocyanin biosynthesis in vegetative organs (Matus et al., 2017) and that both also possess the capacity to alter fruit anthocyanin pigmentation and composition under extreme abiotic conditions (i.e., UV-B) during ripening (Czemmel et al., 2017). Recently, Costantini et al. (2017) identified a set of new candidate genes responsible for anthocyanin variation among cultivars.
The translocation of anthocyanin-acylglucosides into the vacuole is mediated by MATE-type transporters localized in the tonoplast (VviAnthoMATE1-3) (Gomez et al., 2009), whereas translocation of glycosylated anthocyanins occurs via a glutathione- dependent, ATP-binding cassette (ABC) protein (VviABCC1) (Francisco et al., 2013). Furthermore, glutathione S-transferases (VviGSTs) have been associated with the transport of anthocyanins (Conn et al., 2008). Notably, VviGST1 and VviGST4 have been shown to be involved in anthocyanin accumulation in the vacuole (Pérez-Díaz et al., 2016).
The synthesis of hydroxycinnamic acids, stilbenes, flavonols, flavan-3-ols, and anthocyanins is spatially and temporally separated during grape berry development and tightly regulated by a vast transcriptional gene network. In addition to the already described TFs, two MYB (VviMYB5a-b) have been shown to be general regulators of the flavonoid pathway and, in particular, modulate the expression profile of several flavonoid genes such as VviCHI, VviF3′5′H, VviLDOX, VviLAR, and VviANR during berry development (Deluc et al., 2006; Cavallini et al., 2015). Recently, several R2R3-MYBs (VviMyb4a, VviMyb4b, VviMybC2-L1, VviMybC2-L2, VviMybC2-L3, and VviMyb4-like) were characterized as repressors of both proanthocyanidin and anthocyanin biosynthesis (Huang et al., 2014; Cavallini et al., 2015; Muñoz et al., 2019). Moreover, a bHLH (VviMYC1) interacts with VviMYB5a-b, VviMYBPA1, and VviMYBA1-A2 in the transcriptional regulation of proanthocyanidin and anthocyanins biosynthesis in grapevine (Hichri et al., 2011).
Grape Berry Aroma Compounds
In this section, a brief overview of aroma compound formation in grape berries is given to lay the basis for subsequent parts on abiotic interaction with their synthesis. For more detailed information we refer the reader to the recently published review of Lin et al. (2019).
The major groups of grape and wine aroma compounds are mono- and sesquiterpenes, methoxypyrazines, furan derivatives, lipoxygenase pathway products, phenylpropanoid pathway products, norisoprenoids, and volatile sulfur compounds such as thiols. They are mainly found as non-volatile precursors in the berry and require further modifications to be perceived (Dunlevy et al., 2013; Parker et al., 2018; Lin et al., 2019).
Terpenes
Terpenes play roles as phytohormones, protein modification reagents, anti-oxidants, repellents of herbivores and attractants of predators and parasitoids of herbivores (Pichersky and Raguso, 2018). The grape terpenoids of major aromatic importance in grapes can be divided according to their chemical structure into monoterpenes (C10) and sesquiterpenes (C15). Moreover, carotenoids (C40) can be included in this list as aroma precursors (Dunlevy et al., 2013; Ilc et al., 2016).
Around 50 monoterpenes (including cis and trans forms) have been identified in grapes and wine with a significant proportion of them being linalool derivatives (Strauss et al., 1986; Black et al., 2015; Ilc et al., 2016). The main monoterpenes present in grapes are linalool, geraniol, nerol, citronellol, (E)-hotrienol, α-terpineol, and rose oxides (Figure 2) (Matarese et al., 2014), which confer flowery and fruity notes to wines (Siebert et al., 2018). Generally, in aromatic cultivars, terpene concentrations peak early during green berry development, decline until véraison, and strongly increase during ripening (De Billerbeck et al., 2003; Matarese et al., 2013; Costantini et al., 2017). Inversely, Poitou et al. (2017b) found 1,8-cineole concentration to decrease during ripening. 1,8-cineole, commonly known as eucalyptol, is the major aroma compound present in the leaves of many Eucalyptus species. In Australian wines, the presence of this compound has been attributed to the proximity of vineyards to eucalyptus trees and the incorporation of Eucalyptus material into harvested grapes (Capone et al., 2012). 1,8-cineole was also identified as a contributor to the varietal aroma reminiscent of menthol and overall green perception of unripe grapes of the cultivars from the Carmenet family, and partially linked to the proximity of the invasive plant Artemisia verlotiorum (Poitou et al., 2017b).
Grape cultivars can be grouped roughly into three classes based on their total free monoterpene concentration and their monoterpene profile in their wines: cultivars named “neutral” (e.g., Chardonnay and Chasselas) have low terpene concentrations, cultivars more “aromatic” (e.g., Gewürztraminer, Albariño, Scheurebe, Auxerrois, and Riesling) have moderate (1–4 mg L−1) terpene concentrations, and “Muscat type” cultivars (e.g., Muscat de Frontignan, Muscat of Alexandria, Muscat d'Ottonel, Muscat de Hambourg, White Muscat, etc.) have high (as much as 6 mg L−1) terpene concentrations (Darriet et al., 2012).
Sesquiterpenes contribute little to grape and wine aroma, as their concentrations are often below the olfactory threshold. The most studied sesquiterpene is (-)-rotundone, which confers a peppery character to some red and white cultivars, such as Syrah, Duras, Durif, and Viognier (Wood et al., 2008; Geffroy et al., 2014, 2018, 2019).
Terpenes are produced via two independent pathways: (1) the plastidial 2C-methyl-D-erythritol-4-phosphate (MEP) pathway, which is the predominant pathway for monoterpenes (C10) and diterpenes (C20), and (2) the cytosolic mevalonate (MVA) pathway, which is the primary pathway for sesquiterpenes (Bohlmann and Keeling, 2008).
The key enzyme of the MEP pathway in grapevine has been shown to be 1-deoxy-D-xylulose 5-phosphate synthase (VviDXS) (Battilana et al., 2009, 2011; Duchene et al., 2009a). Further downstream terpene synthases (TPSs) regulate the monoterpene or sesquiterpene production (Martin et al., 2010; Matarese et al., 2013, 2014). The genome of Vitis vinifera contains 69 putative TPSs, of which 39 were functionally characterized (Martin et al., 2010) and can be divided into seven clades: TPS-a, -b, -c, -d, -e/f, -g, and -h (Chen et al., 2011). The TPS-a clade (30 genes) is mainly composed of sesquiterpene and possibly diterpene synthases, whereas the TPS-b clade (19 genes) and TPS-g clade (17 genes) is composed principally of monoterpene synthases and the TPS-c (2 genes) and TPS-e/f (1 gene) clades is composed of genes related to plant hormone metabolism and are typically present in a single copy in plant genomes. No full-length TPS-d and TPS-h are known yet in grapevine (Martin et al., 2010). Several genes, such as nudix hydroxylase, vesicle-associated proteins, ABCG transporters, glutathione S-transferases, and amino acid permeases are potential candidate genes for monoterpene biosynthesis regulation and accumulation in the berry (Costantini et al., 2017). Cramer et al. (2014) hypothesized that ethylene signaling could play a role in the biosynthesis of terpenes which has been point out by a positive correlation between aroma production and ERF TFs. The hormones jasmonic acid and methyljasmonate are putatively involved as major regulators of terpene biosynthesis in grapes (Savoi et al., 2016; D'Onofrio et al., 2018). The key genes involved in the synthesis of the sesquiterpene (-)-rotundone have been identified as VviGuaS, VviTPS24, and VviSTO2 (Takase et al., 2015; Drew et al., 2016).
Most monoterpenes and sesquiterpenes are present in berries as non-volatile terpene glycosides. So far, only three monoterpenol glycosyltransferases have been characterized in grapevine, VviGT7-14-15 (Li et al., 2017). Also, the cytochrome P450 CYP76F14 is implicated in several enzymatic transformations of monoterpenes including hydroxylation (mono- and poly- hydroxylations), cyclization, and oxidation through successive reactions including dehydration of alcohol functions in acidic media. These phenomena can lead to an increase in the diversity of monoterpenes in grapes and wines (Schwab and Wüst, 2015). As an example, cytochrome P450 catalyzes the conversion of linalool to (E)-8-carboxylinalool, which, during wine fermentation, generates a wine-lactone, a key odorant of Gewürztraminer wines (Ilc et al., 2016).
Norisoprenoids
Norisoprenoids are a diverse group of widespread compounds derived from the oxidative breakdown of carotenoids (Figure 3) – pigments that contribute to light harvesting and to the protection of the photosynthetic apparatus from photooxidation (Rodríguez-Concepción et al., 2001). Carotenoids are synthesized from isopentenyl diphosphate (IPP) and its double-bond isomer dimethylallyl diphosphate (DMAPP) via the MEP pathway with DXS as the rate-limiting enzyme.
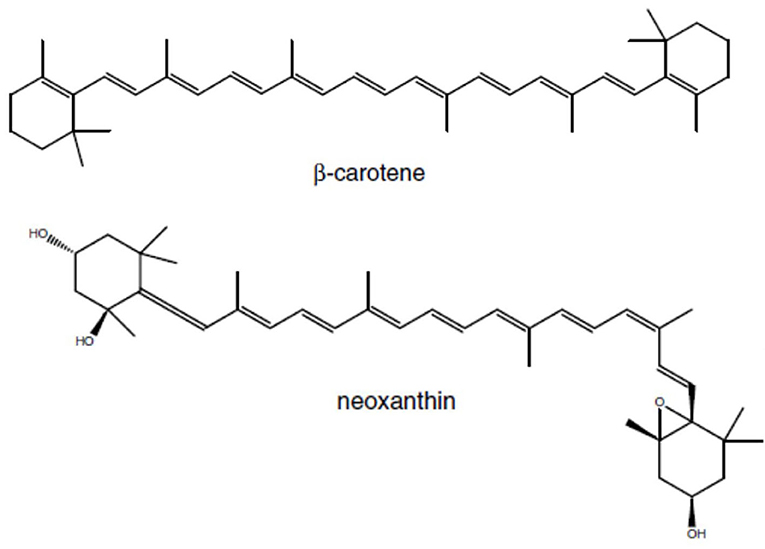
Figure 3. Examples of carotenoid precurors for C13 Norisprenoids in grapes: β-carotene is the precureur of β-ionone, and neoxanthin the precurseur of β-damascenone.
In grapevine, carotenoids are cleaved via carotenoid cleavage dioxygenases (CDD) to form norisoprenoids with C13 carbons (C13-norisoprenoids) such as β-ionone, β-damascenone, vitispirane, actinidol, 4-(2,3,6-trimethylphenyl) buta-1,3-diene (TPB), 1,1,6-trimethyl-1,2-dihydronaphthalene (TDN), and 2,2,6-trimethylcyclohexanone (TCH) (Figure 4). Odorous C10 norisoprenoids such as safranal and ß-cyclocytral have also been found in several grape cultivars (Poitou et al., 2017a).
The cleavage of carotenoids occurs after véraison, during berry ripening, where a simultaneous increase in C13-norisoprenoid content correlates with a decrease in carotenoid content (Razungles et al., 1993; Yuan and Qian, 2016) and is followed by enzymatic reduction and glycosylation (Mathieu et al., 2005; Leng et al., 2017).
At the end of maturation, and during vinification and wine aging, C13-norisoprenoid compounds are formed in acidic media, through chemical reactions from several volatile and non-volatile precursors (Darriet et al., 2012).
β-ionone (violet-like flavor) and β-damascenone (apple sauce – rose like flavor) are the most ubiquitous C13-norisporenoids, whereas significant quantities of TDN are only found in few cultivars such as Riesling and Ugni blanc, whose wines can have a TDN concentration as high as 200 μg L−1 and have a characteristic kerosene/petroleum flavor (Schüttler et al., 2015).
Nine CDD have been identified in Arabidospis, but only VviCCD1 and VviCCD4 have been shown to cleave carotenoids at the 9,10 and 9',10' double bonds (Huang et al., 2009; Ahrazem et al., 2010; Lashbrooke et al., 2013). Fifty four genes putatively involved in carotenoid metabolism in V. vinifera were identified by Young et al. (2012) and Leng et al. (2017) and seven CCDs have been annotated in grapevine (VviCCD1.1, VviCCD1.2, VviCCD4a, VviCCD4b, VviCCD4c, VviCCD7, and VviCCD8) (Young et al., 2012; Lashbrooke et al., 2013).
Fatty Acid Derivates
The unsaturated C18 fatty acids linoleic acid and linolenic acid are the precursors of other volatile organic compounds such as C6-aldehydes and alcohols like hexanal and hexanol (Kalua and Boss, 2009). Their synthesis occurs mainly before véraison in the green berry (Kalua and Boss, 2009) and they have green-grassy aromas even though, considering their detection threshold, they rarely contribute to the herbaceous character of wines. They are formed by the activity of lipoxygenases (VviLOX) (Podolyan et al., 2010), hydroperoxide lyase (VviHPL1 and VviHPL2) (C6-aldehydes and alcohols-2) (Zhu et al., 2012), enal isomerase (3Z)-(2E), and alcohol dehydrogenase (VviADH) (Kalua and Boss, 2009). The levels of these compounds in wines are mainly modulated by winemaking processes; the more odorous C6 aldehydes (hexanal, hexenals) are reduced to less odorous C6 alcohols by Saccharomyces cerevisiae during alcoholic fermentation (Ferreira et al., 1995).
Methoxypyrazines
Methoxypyrazines, nitrogen heterocycle compounds belonging to the pyrazine group, are present in both animals and plants (Maga, 1992). Among the various methoxypyrazines, some alkylated methoxypyrazines, such as 2-methoxy-3-isobutylpyrazine (IBMP), 3-sec-butyl-2-l methoxypyrazine (SBMP), and 2-methoxy-3-isopropylpyrazine (IPMP) (Figure 5) are extremely odorous, with very low odor thresholds (nanogram per liter range in water) (Sidhu et al., 2015). In cultivars of the Carmenet family, such as Sauvignon blanc, Cabernet Sauvignon, Cabernet Franc, Merlot, and Fer, the vegetable-like aromas are reminiscent of pea pods and green peppers, and depending on the concentration they can contribute to earthy nuances (Geffroy et al., 2020). In the grape cluster, the stems contain the largest proportion of IBMP (79.2%), and in the berries, most of the IBMP is located in the skin (72%), followed by seeds (23.8%) and pulp (4.2%) (Roujou-de-Boubée and Botella, 2003).
In grape berries, methoxypyrazines rapidly accumulate between fruit set and the lag phase, peak at 2 to 3 weeks before véraisonr, then decrease continuously during ripening until harvest (Ryona et al., 2008, 2010; Gregan and Jordan, 2016). The extent to which they are synthesized is still not fully elucidated, whether in situ in the berries (Roujou de Boubee et al., 2000; Koch et al., 2010) or in the leaves with subsequent transport via the phloem to the berries where they are degraded (Lei et al., 2018).
Their biosynthesis begins with dicarbonyl addition to the amino acid valine or leucine for IPMP and IBMP and is followed by a methoxylation to form the final methoxypyrazines. In grape, four O-methyltransferases (VviOMT1-4) have been characterized, with VviOMT3 playing a major role in IBMP production (Dunlevy et al., 2013; Guillaumie et al., 2013).
Thiols
Thiols are volatile sulfur compounds and crucial components of the “varietal character” of several cultivars. 4-methyl-4-sulfanylylpentan-2-one (4MSP), 4-merthyl-4-sulfanylpentan-2-ol (4MSPOH) and the 3- sulfanylhexan-1-ol (3SH) (Figure 6) are the most important thiols present as precursors in the berry of white wine cultivars such as Sauvignon Blanc, Semillon, Petite Arvine, Riesling, Chenin Blanc, Muscat blanc, Colombard, and Alvarino (Tominaga et al., 2000; Fretz et al., 2005), but were found also to contribute to perceived fruitiness in red wines made from Cabernet Sauvignon and Merlot (Bouchilloux et al., 1998). Many of the cultivars known for their high thiol content share parent-offspring or sibling relationships (Duchene et al., 2009b). Thiols are generally linked to flavors reminiscent of passionfruit, box tree, black currant, garlic, and asparagus and are also important volatile components of meat, mushrooms, and many other plants. The first discovered thiol was 4MSP, responsible for aroma of box tree (Buxus) (Darriet et al., 1995), followed by the characterization of 3 SH and its ester 3SHA (3-sulfanylhexyl acetate) (Tominaga et al., 1996), often associated with aroma of grapefruit and passionfruit (Tominaga et al., 1998).
Thiols are accumulated during ripening in their non-volatile form, bound to S-cysteine or S-glutathione. The biosynthesis of thiol precursors is linked to glutathione (GSH) metabolism but the biosynthetic pathway of these thiol precursor is not fully elucidated yet. It is related to the conjugation of GHS and α,β-unsaturated carbonyl compounds by S-glutathione transferase as a part of the plant's endogenous metabolism. From this, S-glutathione glutamic acid and glycine is removed resulting in the S-cysteine conjugate. Kobayashi et al. (2011) showed that two genes, VviGST3 and VviGST4, are involved in the production of thiol precursors in grapes.
The localization of different thiol precursors inside the berry is also unclear, and studies often report contradictory results. For example, des Gachons et al. (2005) found that in Sauvignon Blanc grapes at harvest, 4MSP-Cys and 4MSPOH-Cys were localized in the flesh (80%), while 3SH-Cys was equally distributed (50%) between the flesh and the skin. On the contrary, Roland et al. (2011) found that 3SH-Cys and 4MSPOH-Glu were mainly located in the skin (78 and 81%).
The yeast enzyme β-lyase, which is encoded by the gene IRC7 (Howell et al., 2005; Roncoroni et al., 2011; Santiago and Gardner, 2015), cleaves thiol precursors during alcoholic fermentation. Several other factors, such as active transportation of S-cysteine and S-glutathione conjugates through the plasma membrane, influence thiol release during fermentation and contribute to the varietal expression of thiol-containing cultivars, which can sometimes be considered as too intense and as off-flavors, depending on the concentration.
Berry Metabolism Mediated by Abiotic Factors
Temperature, water, light, and CO2 concentration are among the most important abiotic factors interacting with vine and fruit development in a genotypic-dependent manner (Keller, 2010). These factors are expected to be largely modulated by global climate change. The atmospheric concentration of the greenhouse gas (GHG) CO2, the main driver of global warming, has been continuously rising since the beginning of the industrial revolution (around 1,750) due to anthropogenic use of fossil fuel. Concentration increased in the twentieth century from 300 ppm in the early 1960's, when systematic direct atmospheric measurements started (Keeling, 1960), to 400ppm at present time and will, depending on future CO2 emission scenarios, reach between 600 (moderate emission scenario) and 1,000 ppm (worst case scenario) by the end of the twenty first century (IPCC, 2013). This entails a rise of temperature, which is expected to be in a range of 2–4.8°C as a function of anthropogenic CO2 emissions (IPCC, 2013, 2018). Besides its direct impact on plant physiology, higher temperatures lead to higher evapotranspiration rates and to increased water requirements during the growing season. Together with predicted alterations in precipitation patterns, more severe drought periods can be expected and have already been observed in most viticulture regions (Schultz, 2016). Regarding solar radiation of different wavelengths reaching the earth's surface, predictions of climate change models are ambiguous. Due to its high energy and impact on all living organisms, ultraviolet (UV) radiation (wavelength from 100 to 400 nm) plays a crucial role in the physiology of different plants, mammals and human beings, and terrestrial ecosystems (Ballaré et al., 2011). The UV-B radiation reaching the earth's surface is mostly impacted by the gas composition of the atmosphere. The ozone layer in the upper stratosphere absorbs most of the UV radiation and has been highly degraded by ozone-depleting substances (ODSs) such as chlorofluorocarbons (CFCs) during the last 40 years, which caused a significant increase in UV-B radiation reaching earth's surface. Since the reduction of ODS emissions following the ratification of the Montreal protocol in 1989, the depletion of the ozone layer in the stratosphere was slowed; it remains unclear how UV-B radiation will develop during different climate change scenarios. Certainly the continuous rise of CO2 is altering the physical structure of the atmosphere (Chipperfield et al., 2017). Measured concentrations of ozone show an increase of 2–4% per decade, at mid-latitudes and the tropics, in the upper stratosphere (altitude 35–45 km) since about 2000. This increase is consistent with the recovery of stratospheric ozone as a consequence of decreasing concentrations of ODSs and increases in GHGs. In fact, GHGs in the troposphere will isolate upper layers of the atmosphere and a cooler stratosphere which slows down catalytic ozone breakdown (Williamson et al., 2014; United Nations Environment Programme, Environmental Effects Assessment Panel, 2017; Bais et al., 2018, 2019). An additional factor to consider in respect to UV, is its variability due to cloud cover, which often exceeds the variability due to the ozone column depth and is expected to vary in the future due to global climate change as well and remains hard to predict (Enriquez-Alonso et al., 2016).
Altogether the alteration of the different aforementioned abiotic factors will continue to impact viticulture worldwide in different ways. This has triggered discussions among scientists regarding the sustainability of traditional viticulture regions (Fraga et al., 2012, 2013). Different authors predict that the land suitable for viticulture will decrease in main growing regions between 25 and 73% in the future (Hannah et al., 2013), which has however been questioned by other groups as there was no consideration of the capability of cultivars to adapt to changing conditions (van Leeuwen et al., 2013). Recently, more integrated modeling approaches predict that under the most optimistic climate change scenario (2°C increase) and in the most pessimistic scenario (4°C increase) 56 and 85% of current wine growing regions will become climatically unsuitable for viticulture by the end of the twenty first century, respectively (Morales-Castilla et al., 2020). In general, predicted and already observed consequences for wine quality are wines with higher alcohol content, lower acidity, and altered aroma profiles (Schultz, 2000, 2016; Mira de Orduna, 2010; Pons et al., 2017b; van Leeuwen and Destrac-Irvine, 2017) which altogether leads to a loss of typicity and terroir expression (van Leeuwen and Destrac-Irvine, 2017; Van Leeuwen et al., 2018; van Leeuwen et al., 2020).
In the subsequent sections, the most recent and pertinent literature dealing with the impact of the main abiotic factors modified by global climate change on berry metabolism is reviewed.
Temperature
Temperature is the main driver of vine phenology and its increase advances the vegetative and reproductive cycle of the grapevine and consequently shifts the berry developmental stages toward warmer months of the growing season (van Leeuwen and Destrac-Irvine, 2017). This has been widely confirmed in studies analyzing historical records of harvest dates worldwide which show overall advances of 1–2 weeks over the last several decades (Jones and Davis, 2000; Chuine et al., 2004; Duchêne and Schneider, 2005; Jones et al., 2005; Petrie and Sadras, 2008; Webb et al., 2012; Cook and Wolkovich, 2016). However, crop-yield reductions and evolving management practices may have also contributed to the advancement of ripening (Webb et al., 2013).
The combined effects of advanced phenology and increased temperatures during ripening lead to grapes with higher sugar and less organic acid concentration as well as altered composition in secondary metabolites, such as phenolic and aroma compounds (van Leeuwen and Destrac-Irvine, 2017). In the recent decades, myriad temperature studies have been conducted with a multitude of experimental approaches (Bonada and Sadras, 2015; Gouot et al., 2018). Results are often ambiguous, which makes it difficult to draw general conclusions. Published studies vary from whole plant to bunch level stress applications with variations in the duration of stress application from a couple of hours to several months. A wide range of temperature intensities have been tested from moderate increases (up to 35°C) to severe heat stress (up to 45°C) during day and/or night and at different berry developmental stages. The cultivar and the type of plant material used, as well as the experimental constraints for such studies, affect the responses of berry metabolism to temperature regimes. Control climate chamber experiments are mostly conducted with small model plants such as fruiting cuttings (Pillet et al., 2012; Carbonell-Bejerano et al., 2013; Lecourieux et al., 2017) or mutant microvines (Rienth et al., 2012, 2014a,b, 2016, 2017; Houel et al., 2015; Luchaire et al., 2017; Torregrosa et al., 2017, 2019; Pellegrino et al., 2019).
The dissociation of temperature and radiation effects is difficult to achieve in field trials as compared to climate chamber experiments where heat is applied by convection. However, some early and methodically groundbreaking field experiments succeeded to control clusters' temperature in an radiation-independent way by using different row orientation (Bergqvist et al., 2001), bunch cooling (Spayd et al., 2002) or in situ convection systems (Tarara et al., 2008). These studies concluded that some fruit components (in particular anthocyanins) are especially sensitive to temperature (Poni et al., 2018), which to some extent have been subsequently confirmed by molecular studies.
Temperature Impact on General Fruit Metabolism
Probably the earliest controlled study of the temperature effect on berry metabolism is Ravaz 1912, which inserted single clusters of a vine into a heated glass chamber. Many temperature studies focusing mainly on sugar accumulation, primary metabolisms, and berry growth dynamics have been conducted from the 1960's to the 90's (Kliewer, 1964, 1971, 1977a,b; Kliewer and Lider, 1968, 1970; Buttrose et al., 1971; Kliewer and Torres, 1972; Hale and Buttrose, 1974; Lakso and Kliewer, 1975; Matsui et al., 1986; Sepulveda and Kliewer, 1986) and are summarized in the very first review on the effect of elevated temperature on berry metabolism by Coombe (1987). In summary, moderate temperature increases during ripening hasten berry development and sugar accumulation as observed already in early studies from Buttrose et al. (1971), whichnoted an increase in total soluble solids (TSS) in Cabernet Sauvignon berries when exposed to higher temperatures (30°C). Kliewer and Torres (1972) observed a similar sugar accumulation increase, correlated with increasing temperature until around 35°C, in Cardinal, Pinot noir, and Tokay and Sangiovese even until 41.7°C (Pastore et al., 2017). During early green stages, moderate temperature increases accelerate berry growth, malic and tartaric acid accumulation (Rienth et al., 2016; Arrizabalaga et al., 2018).
When heat stress becomes severe (>35°C), a decrease or arrest of sugar accumulation as well as an inhibition or cessation of berry growth and a delay of ripening is observed (Matsui et al., 1986; Sepulveda and Kliewer, 1986; Greer and Weston, 2010; Greer and Weedon, 2013; Lecourieux et al., 2017; Gouot et al., 2019). This slackening of berry development has been attributed to a lack of carbon supply by photosynthesis, which is impeded and slows down the rate of cell division, which may limit berry size (Keller, 2010). Furthermore, a decrease in ABA biosynthesis as indicated by the repression of VviNCED2 and VviNCED4 transcripts and the upregulation of VviABI3 a B3-domain TF that is a part of the core ABA signaling network were associated with slower berry growth (Rienth et al., 2014b; Lecourieux et al., 2017). In addition, the role of auxins biosynthesis and regulation, which inhibits berry growth and sugar and anthocyanin accumulation (Davies et al., 1997; Bottcher et al., 2010) could putatively contribute to the delay of berry development and onset of ripening under severe heat stress as evidenced by the reduction of IAA-amido synthetase (VviGH3) and an increase in the expression of transcripts encoding IAA-amino acid hydrolases (Lecourieux et al., 2017).
The slackening of sugar accumulation rates under heat stress has been correlated with a down-regulation of sugar transporters (STPs) and invertase-encoding genes (GIN2) putatively involved in the import and accumulation of hexoses into vacuoles. The latter genes were found to be repressed in fruiting cuttings of Cabernet Sauvignon (Lecourieux et al., 2017), Muscat Hamburg (Carbonell-Bejerano et al., 2013) and microvines (Rienth et al., 2014b) upon exposure to high temperature. Curiously, a recent field study using an open top heating system found an altered glucose / fructose ratio due to lower glucose concentration in Riesling berries under high temperature (+10°C) (Brandt et al., 2019). Similar observations have been reported in historical studies and attributed the altered glucose/fructose ratio to over-ripeness of grapes (Kliewer, 1965, 1967; Sepulveda and Kliewer, 1986). This has, however, not been observed or closely investigated in other heat stress studies (Carbonell-Bejerano et al., 2013; Sweetman et al., 2014; Lecourieux et al., 2017).
Drawing upon the results described above, it seems that the most sensitive berry stage to biotic and abiotic stress is just around or during the véraison phase as evidenced by a couple of studies with precise single berry sampling protocols (Rienth et al., 2014b, 2016; Torregrosa et al., 2019; Ghaffari et al., 2020).
Concerning malic and tartaric acid, the two main organic acids in grape berries, it appears that moderately increased temperature during early berry development from anthesis to lag phase accelerates their accumulation (Sweetman et al., 2014; Rienth et al., 2016; Lecourieux et al., 2017). However, when heat stress becomes too severe, their synthesis is inhibited, as reported, for example, by Gouot et al. (2019), where temperatures reached up to 45°C during early green growth.
It has been shown in myriad historic and recent studies that high temperatures are correlated with a decrease in malic acid concentration in the ripening berry (Ruffner et al., 1976; Ruffner and Hawker, 1977; Possner et al., 1983; Sweetman et al., 2009, 2014; Carbonell-Bejerano et al., 2013; Etienne et al., 2013; Rienth et al., 2016; Lecourieux et al., 2017; Brandt et al., 2019). This degradation is highly cultivar-dependent and can even vary among clones, as shown for example on different Tempranillo clones exposed to high temperatures (Torres et al., 2017). However, the underlying regulation of malic acid degradation remains to be elucidated. Sweetman et al. (2014) found that high temperatures accelerated the NAD dependent malic enzyme activity and decreased phosphoenolpyruvate carboxylase and pyruvate kinase activities, accompanied by the accumulation of various amino acids and γ-aminobutyric acid, suggesting an enhanced anaplerotic capacity of the TCA cycle and a need to deal with decreased cytosolic pH in heated berries. This study also found differences depending on the diurnal temperature range: that is, the loss of malic acid content in response to elevated day temperature was lessened if night temperature was also increased. It was also proposed that malate concentration is mainly depends on the thermodynamics of its tonoplastic transport (Lobit et al., 2006).
It is generally reported that tartaric acid (TA) is not metabolized by the ripening berry and thus its content is not impacted by temperature and represents an important trait for breeding programs seeking new cultivars better adapted to future climate conditions (Duchêne, 2016). Observed variation of TA in the scientific literature (Cholet et al., 2016) is a likely consequence of dilution effects or precipitation during sample processing (Rösti et al., 2018).
Temperature Impact on Phenolic Compounds
The direct and indirect effects of temperature on flavonoid composition are far from being completely unambiguous. In this section we summarize and update recent findings.
Temperature effects on flavonol and flavan-3-ol composition are not always consistent among studies (Gouot et al., 2018). However, there is unambiguous scientific evidence that shows deleterious effects of high temperature on anthocyanin levels in the grape berry. This was reported in early studies (Buttrose et al., 1971; Kliewer and Torres, 1972; Spayd et al., 2002) and more recently confirmed by physiological and molecular studies (Mori et al., 2005, 2007; Yamane et al., 2006; Azuma et al., 2012; Carbonell-Bejerano et al., 2013; Rienth et al., 2014b; Lecourieux et al., 2017; Pastore et al., 2017; Torres et al., 2017; Yan et al., 2020). Heat stress has been shown to repress major anthocyanin biosynthesis regulators such as VviMYBA1 and downstream genes such as VviUFGT, VviCHI, VviF3H2, VviDFR, and VviLDOX. However, not all of these studies showed unequivocal repression nor a correlation with lower anthocyanin accumulation. This is possibly due to the use of different plant materials (normal vines, different cultivars), different stages of berry development and intensities of treatment, and sampling strategy. Furthermore, the temperature effect on anthocyanin synthesis varies highly between genotypes. For example, when the temperature maximum during ripening exceeded 35°C, inhibition of color formation was much more pronounced in Grenache than in Carignan (Fernandes de Oliveira et al., 2015). In earlier studies, low temperature during ripening, in particular at night was associated with enhanced coloration of grapes (Kliewer and Torres, 1972), which was confirmed in a recent molecular experiment where low night temperatures enhanced anthocyanin accumulation and expression of VviCHS3, VviF3H1, VviUFGT, and VviMYBA1, in particular when applied around véraison on Corvina grapes (Gaiotti et al., 2018). In Kyoho grapes, temperature increases from 27 to 30°C during ripening induced a strong decrease of the transcript levels of anthocyanin genes, leading to less berry color (Shinomiya et al., 2015). In Merlot, an increase of day temperature of 5°C during ripening, from 20 to 25°C, resulted in a anthocyanin decrease of 37% (Yan et al., 2020). Besides the repression of anthocyanin related genes, high temperature may promote anthocyanin degradation, possibly via the increased activity of peroxidases (Mori et al., 2007). This is evidenced by the upregulation of a gene coding for a peroxidase, VviPrx31, in berries exposed to high temperature (Movahed et al., 2016), and a similar effect occurs in other plant species, such as Brunfelsia flower petals (Vaknin et al., 2005), litchi (Zhang et al., 2005), and strawberry fruits (Chisari et al., 2007).
An increased proportion of acylated and tri-hydroxylated anthocyanins under higher temperature has been observed in experiments with Merlot (Tarara et al., 2008; Yan et al., 2020), Cabernet Sauvignon (Lecourieux et al., 2017), Sangiovese fruiting cuttings (Pastore et al., 2017), and Malbec (de Rosas et al., 2017), concomitantly with the overexpression of the acyltransferase gene Vvi3AT (de Rosas et al., 2017; Yan et al., 2020). Similarly for anthocyanins, high temperature impeded flavanol accumulation strongly and increased methoxylated (isorhamnetin and syringetin) and 3′, 4′, 5′-substituted (myricetin and syringetin) flavonols in Merlot (Yan et al., 2020).
Interestingly, high temperature can cause an uncoupling of sugar accumulation and anthocyanin synthesis leading to a lower anthocyanin / sugar ratio, possibly because of a delay in the onset of anthocyanin synthesis (Sadras and Moran, 2012; Sadras et al., 2013; Yan et al., 2020) or a reduced anthocyanin accumulation during ripening (Yan et al., 2020). The magnitude of this thermal decoupling is cultivar dependent as shown for Grenache and Carignan (Fernandes de Oliveira et al., 2015) and can vary between clones of the same cultivar as shown in Tempranillo (Arrizabalaga et al., 2018).
The effect of temperature on tannins is still not well-understood. The synthesis of the tannin monomers, flavan-3-ols, was increased under elevated temperature (Cohen et al., 2012a,b), although in both latter studies, differences were no longer significant at véraison. Tannins were not affected by heat stress in Sangiovese (Pastore et al., 2017), as well as in the study of Gouot et al. (2019) who reported an absence of effect on flavan-3-ol or tannin levels, but a significantly higher percent of galloylation of flavan-3-ols, consistently with that reported by Cohen et al. (2012a) and indicated bythe overexpression of UDP glucose-gallic acid-glucosyltransferase under high temperature as reported by Rienth et al. (2016).
With regards to stilbene synthesis, heat stress inhibited the expression of members of the STS biosynthetic pathway (Rienth et al., 2014b), while grape exposure to low temperature upregulated STS transcripts indicating a higher stilbene biosynthesis at low temperature (Pastore et al., 2017).
Temperature Impact on Aroma Compounds
In plants, high temperature causes an increase in aroma compound production and emission as shown particularly for terpenes up to a certain threshold, generally around 40°C (Guenther et al., 1993; Copolovici and Niinemets, 2016). In grape berries, the temperature impact on aroma compound accumulation is ambiguous (Selmar and Kleinwächter, 2013; Lecourieux et al., 2017). From recent studies, it appears that the aroma levels are reduced when heat stress is applied both at the cluster scale (Lecourieux et al., 2017) and at the whole plant scale if stress is applied during berry ripening (Rienth et al., 2014b). In Sangiovese, Pastore et al. (2017) reported an increase in TPS expression under higher temperature before ripening and a repression of linalool synthase, delta-cadinene synthase, vetispiradiene synthase, and a germacrene enzyme activity during ripening. In Sauvignon blanc exposed to higher berry temperature through leaf removal, the concentration of thiol precursors in berries at harvest was not significantly modified (Sivilotti et al., 2017). However, Wu et al. (2019) found a lower concentration of the aldehydic glutathionylated precursor of 3SH (Glut-3SH-Al) in grapes from Cabernet Sauvignon and Sauvignon Blanc under a 1.5°C temperature increase.
Temperature studies on carotenoids, the precursors of C13 norisoprenoids (see above), are also inconsistent, most likely because of the difficulty in separating temperature and radiation effects in field trials. Higher carotenoid levels were found in Riesling and Chenin Blanc grown in warmer than cooler regions (Marais et al., 1991). In the Duoro Valley in Portugal, cooler temperature at higher altitudes possibly contributed to the lower berry carotenoids concentration in Portuguese autochthonous cultivars (Oliveira et al., 2004). Fernandes de Oliveira et al. (2015) found consistently higher carotenoid content in shaded grapes compared to grapes exposed to direct sunlight. However, leaf removal in the fruiting zone, which generally increases temperature around berries, did not alter total carotenoids in Riesling (Kwasniewski et al., 2010).
The concentration of the C13 norisoprenoid 1,1,6-trimethyl-1,2-dihydronaphtalene (TDN), which determines the kerosene-like notes of aged Riesling, is generally higher in warm climates (Marais et al., 1992; Schüttler et al., 2015). Carotenoid and C13 norisoprenoid related transcripts, such as phytoene synthase (VviPSY), which catalyzes the first step of carotenoid biosynthesis, and downstream genes coding the phytoene dehydrogenase, carotenes desaturase, carotenoid isomerase, lycopene cyclase, and carotene hydroxylase were concomitantly downregulated by vine exposure to high temperature (Rienth et al., 2014b; Lecourieux et al., 2017).
Considering the cultivars that produce MPs, wines produced in cooler regions have higher MP concentrations and display a more vegetative odor, as shown for Cabernet Sauvignon and Sauvignon Blanc (Allen et al., 1991; Falcão et al., 2007). However, it is still not clear to what extent temperature contributes to the decrease of MPs before and during ripening, and to what extent the decrease is due to the radiation (Darriet et al., 2012; Lei et al., 2018). The limited molecular data indicates a repression of IBMP synthesis already in the green berry exposed to high temperature as highlighted by the repression of the key gene VviOMT3 during heat stress experiments with fruiting cuttings (Lecourieux et al., 2017).
Recent research has characterized other aroma components contributing to overripe aromatic nuances in grapes and wines of red cultivars (i.e., nuances of jammy fruit, and prune in Merlot and Cabernet Sauvignon wines) (Pons et al., 2017a; Allamy et al., 2018). Such grapes and wines presented a greater concentration of chemical compounds belonging to the ketones, furanones and lactones family. Moreover, the analysis of wines of different vintages from a Pomerol estate contained higher concentrations of 5,6-dihydro-6-pentyl-2-(2H)-pyranone (called massoia lactone or 2-decen-5-olide) and gamma-non-alactone during vintages with higher average temperatures. This leads to the hypothesis that increased temperatures due to climate change will increase the perception of overripe fruity notes, as occurring now in hot vintages (Pons et al., 2017a).
Radiation
As described above, changes in the gas composition of the atmosphere are forecasted for the future. These changes may affect the amount and composition of solar radiation reaching the earth's surface. The exposure of grapes to sunlight is normally associated with higher berry quality due to higher levels of total soluble solids, anthocyanins, and phenolics in general, and lower levels of acids and juice pH as well as lower incidence of disease due to better microclimates (Dokoozlian and Kliewer, 1996; Bergqvist et al., 2001; Abeysinghe et al., 2019).
Impact of Radiation on Phenolic Compounds
The exposure of grapes to sunlight normally increases the level of phenolic compounds as shown, for example, in Riesling (Brandt et al., 2019), Pinot Noir (Song et al., 2015), Summer Black (Xi et al., 2016), and Cabernet Sauvignon (Blancquaert et al., 2019). In parallel, sunlight exposure enhances the expression of structural and regulatory phenylpropanoid genes (Chorti et al., 2010; Matsuyama et al., 2014; Wu et al., 2014; Friedel et al., 2015; Sun et al., 2017). Among phenolics, the most light-responsive ones are flavonoids and in particular flavonol glucosides, whose levels increase dramatically with increasing sunlight exposure, consistently with their UV radiation-screening activity and their capacity to reduce light-induced oxidative damage (Downey et al., 2004; Matus et al., 2009; Agati et al., 2013; Martínez-Lüscher et al., 2014; Reshef et al., 2017, 2018). In a recent comprehensive study that used leaf thinning and shoot removal in Cabernet Sauvignon and Petit Verdot to improve sun exposure, the flavonols kaempferol, quercetin, and myricetin significantly increased, whereas no changes of other flavonoid compounds occurred (Torres et al., 2020a). Alike, Sun et al. (2017) found higher hydroxycinnamic acids and flavonol levels in Cabernet Sauvignon when sun exposure was increasd.
Several transcriptomic studies indicate that, in the berry skin, flavonol genes – for example VviFLS1, VviGT5 and VviGT6 in Tempranillo berries – are more induced than those of other phenylpropanoids upon UV radiation (Koyama et al., 2012; Loyola et al., 2016). Reciprocally, Matus et al. (2009) found reduced expression of VviFLS4 and its putative transcriptional regulator MYB12 when berries were shaded.
It is not fully understood to what extent visible and/or UV light contribute to the stimulation of the synthesis of phenolic compounds (Keller and Torres-Martinez, 2004; Schreiner et al., 2012; Teixeira et al., 2013). Drawing upon recent studies, it seems that particularly the UV-B fraction of solar radiation is responsible for the enhanced expression of key flavonoid genes (Koyama et al., 2012; Teixeira et al., 2013; Carbonell-Bejerano et al., 2014; Martínez-Lüscher et al., 2014; Liu et al., 2015; Loyola et al., 2016). Recently, two bZIP TFs elongated hypocotyl 5 protein (HY5) orthologs, VviHY5 and VviHYH, were characterized as constituents of the UV-B response pathway in grapevine and mediated flavonol accumulation in response to high radiation exposure (Loyola et al., 2016; Matus et al., 2017).
The exposure of grape clusters to light significantly increases anthocyanin accumulation, whereas shading reduces it (Spayd et al., 2002; Downey et al., 2004; Matus et al., 2009; Song et al., 2015; Guan et al., 2016). In a comprehensive in vitro study that considered the effect of berry exposure to light and temperature treatments, Azuma et al. (2012) reported higher anthocyanin levels in grapes exposed to higher light levels. These higher levels correlated with the upregulation of most genes of the anthocyanin biosynthesis pathways. Several other studies confirm the induction of major anthocyanin genes such as the TF VviMYBAa together with VviUFGT under elevated sun exposure (Koyama et al., 2012; Shinomiya et al., 2015). Interestingly, UV-B radiation induces the expression of VviMYBA1 and significantly delays the down-regulation of VviMYBA6 and VviMYBA7 at the latter stages of berry development (Matus et al., 2017).
Low light conditions modulate the proportion of di- to tri-hydroxylated anthocyanins more toward tri-hydroxylated anthocyanins as evidenced by the downregulation of VviF3′5′Hs (Azuma et al., 2012; Koyama et al., 2012; Guan et al., 2016). Similar trends, but inconstent amongst years, have been recently reported in warm climates with Cabernet Sauvignon (Sun et al., 2017) and Petite Verdot (Torres et al., 2020a,b). It seems that low light conditions increase the concentration of non-acylated anthocyanins (Downey et al., 2006; Matus et al., 2009) but this remains to be confirmed in future studies.
Recently, a role of miRNA on the anthocyanin response to UV-B radiation has been suggested. Sunitha et al. (2019) hypothesize that the UV-B induced upregulation of miR3627/4376 facilitates anthocyanin accumulation by antagonizing a calcium effector, and showed that miR395 and miR399, which are induced by micronutrient deficiencies also known to trigger anthocyanin accumulation, respond positively to UV-B radiation. Finally, increases in the abundance of the MYB-bHLH-WD40 complex, which regulates anthocyanin production, are mediated by UV-B-induced changes in miR156/miR535. The same changes could contribute to the observed up-regulation of miR828. In turn, miR828 would regulate the AtMYB113-ortologues MYBA5, A6 and A7 (and thereby anthocyanins) via a widely conserved and previously validated auto-regulatory loop involving miR828 and phasi TAS4abc RNAs.
Impact of Radiation on Aroma Compounds
It is generally known that light exposure increases the concentration of most aroma compounds in grape berries, but that excessive sunlight as well as its total exclusion inhibits the accumulation of most aroma compounds (Bureau et al., 2000; Zhang et al., 2014; Young et al., 2016).
Because carotenoids are pigments with photoprotective function, their biosynthesis is generally enhanced under high radiation as are their degradation products. The levels of C13 norisoprenoids are as well highly correlated with extended sun exposure (Kwasniewski et al., 2010; Schüttler et al., 2015; Young et al., 2016). Sunlight exposure increased the concentration of β-ionone, but the increase was not statistically significant for UV treatment (Song et al., 2015), and TDN is typically found in high amounts in sun-exposed Riesling berries, potentially resulting in a higher petrol aroma of wines (Mendes-Pinto, 2009).
Young et al. (2016) reported that the cytosolic CCD1-encoding genes were up-regulated in exposed clusters in the earlier stages of berry development. Conversely, the chloroplastic CCD4-encoding genes were down-regulated in exposed clusters during ripening. Therefore, Young et al. (2016) suggested that the increased levels of norisoprenoids observed in exposed berries during ripening were not due to increased gene expression (of the CCD4-encoding genes) but rather due to increased substrate (carotenoid) availability.
Monoterpenes, and particularly linalool, are highly sensitive to sunlight, as shown, for example, in Sauvignon Blanc and Riesling (Sasaki et al., 2016), where the expression of VviDXS and of linalool synthases was reduced by low sun exposure and by UV-B exclusion, resulting in lower levels of linalool content. Similarly, Zhang et al. (2017) showed that, in the Muscat variety Jingxiangyu, linalool was the most sensitive compound to sunlight followed by ocimene and glycosylated geraniol. The reduction in the levels of these terpenes by sunlight exclusion correlated with the reduced expression of VviPNLinNer1, VviCSbOci, VviGT7, and VviGT14 genes. Similarly, Carbonell-Bejerano et al. (2014) reported an upregulation of genes involved in monoterpenoid biosynthesis, such as 1,8-cineole/eucalyptol synthase and two linalool synthases, in Tempranillo berries exposed to high UV-B radiation. In general, monoterpene levels were induced by exposing clusters to sunlight (Song et al., 2015; Feng et al., 2017).
Young et al. (2016) showed that increased photosynthetically active radiation (PAR) (+52%) led to higher levels of volatile terpenoids in the exposed Sauvignon Blanc berries; however, there were clear differences in the responses based on the developmental stage considered.
Similarly, Šuklje et al. (2014) found increased concentration of thiols in clusters exposed to sunlight, and it is generally known that light exposure during ripening reduces MP content in berries (Roujou de Boubee et al., 2000; Sala et al., 2004; Stummer et al., 2005; Darriet et al., 2012; Sidhu et al., 2015; Martin et al., 2016; Cassandra et al., 2019; Torres et al., 2020a). Interestingly, according to Koch et al. (2012), higher light intensity before and not during ripening has a greater impact of methoxypyrazine concentration at harvest. Dunlevy et al. (2013) showed that both the precursor of 3-isobutyl-2-hydroxypyrazine (IBHP) and the expression of VviOMT3, a gene that controls the final step of methoxypyrazine biosynthesis, were drastically reduced in exposed clusters(Koch et al., 2012).
Allamy et al. (2018) considered separately the specific impact of light exposure on berry aroma compounds during post-harvest storage. Under conditions of light exposure, significant increases in furaneol, homofuraneol and γ-nonalactone concentrations were noticed in both grape juice and wine (Allamy et al., 2018).
Water
Impact of Vine Water Status on Phenolic Compounds
Studies investigating the effect of water availability on berry physiology and quality have been recently reviewed by Scholasch and Rienth (2019), Rienth and Scholasch (2019), and Gambetta et al. (2020). Impact of water deficit on berry development depends on the intensity and duration of the stress as well as the developmental stage. Water deficit stress during the first growth phase had the highest impact on final berry volume and consequently yield. Water deficit reduces cell expansion without impacting the rate of cell division (Ojeda et al., 2001), contrary to frequent speculations. During the ripening phase, water deficit has a smaller impact on berry size than during the first growth phase, probably because of impaired hydraulic connections with the parental plant.
It is generally known that a moderate water deficit (predawn leaf water potential between−0.3 to −0.5 MPa) is beneficial for final wine quality which is in particularly true for red cultivars (Van Leeuwen et al., 2009; Zufferey et al., 2017). Because water deficit reduced berry volume, positive effects can partly be attributed to higher concentration of quality determining compounds synthesized in the skin cells (the surface of the berry). However, an enhanced accumulation of secondary metabolites independently of berry volume changes has been already highlighted by Ojeda et al. (2002) and confirmed by several molecular studies that observe an upregulation of key enzymes of the phenylpropanoid and flavonoid pathways (Castellarin et al., 2007a,b; Cramer et al., 2007; Deluc et al., 2009, 2011; Teixeira et al., 2013; Savoi et al., 2016, 2017; Zarrouk et al., 2016a,b).
The most beneficial effects are observed when water deficit occurs throughout ripening. Aside from an overall increase in the accumulation of phenylpropanoids and flavonoids (Chorti et al., 2016; Koundouras, 2018), several studies showed a modification in composition of anthocyanins toward a relative increase of tri-hydroxylated anthocyanins (3′,4′,5′-hydroxylated: delphinidin, petunidin, malvidin) (Castellarin et al., 2007a; Ollé et al., 2011; Cook et al., 2015). However, the changes in the anthocyanin profile in response to water deficit appear to be highly varietal dependent (Niculcea et al., 2014; Theodorou et al., 2019). Some reports showed an increase proanthocyanidin concentration and proanthocyanidin polymerization levels in grape berry skins (Kyraleou et al., 2016; Cáceres-Mella et al., 2017), and higher catechin levels (Zsófi et al., 2014). The observed increase of phenolic compounds when water deficit occurs prior to véraison can mainly be attributed to concentration effects (Santesteban et al., 2011; Brillante et al., 2018); however, several studies also observed an increase in anthocyanin content per berry (Ojeda et al., 2002; Castellarin et al., 2007a; Koundouras et al., 2009; Ollé et al., 2011).
Impact of Vine Water Status on Aroma Compounds
Reported effects of water availability on aroma compounds are less evident than for phenolic compounds. Most water deficit studies on grape aroma compounds show very heterogeneous results, depending on the type of aroma compounds considered, as reviewed by Alem et al. (2019). A positive relationship between increasing water deficit and the concentration of C13-norisoprenoids such as β-damascenone, β-ionone, and 1,1,6-trimethyl-1,2-dihydronaphthalene was reported. This was particularly true for red cultivars such as Cabernet Sauvignon (Bindon et al., 2007; Koundouras et al., 2009; Brillante et al., 2018), Merlot (Song et al., 2012), and Tempranillo (Talaverano et al., 2017). In of Talaverano et al. (2017), C6 compounds (hexanal, trans-2-hexenal, and 1-hexanol), phenol volatiles, ethyl esters, and lactones were also found to be increased under water deficit, as opposed to Song et al. (2012), which reported a decrease of those compounds under water deficit in Merlot.
Several studies reported increased concentrations of monoterpenes such as limonene, linalool, α -terpineol geranyl acetone, geraniol, and citronellol under light to moderate water stress (Savoi et al., 2016; Brillante et al., 2018; Wang et al., 2019; Kovalenko et al., 2021), which was associated with increased expressions of terpenoid synthases-genes in Chardonnay and Tocai Friulano (Deluc et al., 2011; Savoi et al., 2016). Some authors report higher monoterpene concentrations, even under severe water deficit (Schüttler et al., 2015). One of the few aroma compounds whose concentration in the berry is negatively correlated with moderate water deficit is the recently discovered sesquiterpene rotundone (Wood et al., 2008; Geffroy et al., 2014, 2018) associated with notes of black pepper.
The precursors of volatile thiols (S, 4MSPOH and the 3SH) present in the berry (Tominaga et al., 2000; Fretz et al., 2005) increased under mild water deficit and decreased under severe stress (predawn leaf water potential close to −1.0 MPa) (des Gachons et al., 2005). However, since nitrogen is very important for the production of volatile thiols of grapes (Helwi et al., 2015, 2016) and its absorption can be limited by water deficit (Celette and Gary, 2013), the effects observed may be indirect and largely due to a limited nitrogen absorption. Picard et al. (2017) found that the exposure of vines to water deficit positively relates to the perception of aging bouquet typicality (truffle and underwood aroma) in premium Bordeaux wines. It appears that moderate water deficit increases the concentration of C13-norisoprenoids most likely from higher sun exposure due to reduced canopy (Koundouras et al., 2009). On the other hand, the reduced canopy caused by water deficit might favor methoxypyrazine degradation (Brillante et al. (2018) and Harris et al. (2012).
Co2 Concentration
Elevated carbon dioxide (eCO2) concentration is generally beneficial for plants because it leads to increases in the rate of photosynthetic carbon fixation by leaves. This leads primarily to increased plant growth and biomass production and translates into increases in harvestable yield of wheat, rice and soybean, all showing 12–14% yield increases under eCO2 in FACE (Free Air Carbon enrichment) experiments (Ainsworth and Long, 2005; Ainsworth and Rogers, 2007; Ainsworth, 2008). In fruit crops and vegetables, eCO2 generally increased the total antioxidant capacity as well as the concentrations of fructose, glucose, total soluble sugars, total phenolics, total flavonoids, ascorbic acid, and calcium in the edible parts (Sun et al., 2017).
Most studies on grapevine dealing with eCO2 focused on vegetative growth and photosynthesis while physiological and molecular studies on berry metabolism are relatively scarce so far. All studies report increased photosynthesis leading to a yield and biomass increase under eCO2 (Goncalves et al., 2009; Moutinho-Pereirea et al., 2009; Kizildeniz et al., 2015; Edwards et al., 2016, 2017; Wohlfahrt et al., 2018). In climate chamber studies, Martinez-Luscher et al. (2015) highlighted the dependence of berry ripening rates on the carbon fixation process which is correlated to CO2 concentration.
Only some grape attributes have been found to be affected by eCO2. In particular, sugars, acids, and berry size increased under eCO2 (Bindi et al., 2001; Kizildeniz et al., 2015). However, in a FACE study that considered Riesling and Cabernet Sauvignon vines exposed to moderate increases of atmospheric CO2, sugar concentration was not affected even though yields were increased (Wohlfahrt et al., 2018). In wines made from the latter FACE experiment must and young wines quality and compositin was not found to be negatively influenced by an eCO2 (Wohlfahrt et al., 2021). Anthocyanins and proanthocyanidins were not affected by eCO2 in most studies (Goncalves et al., 2009; Salazar-Parra et al., 2012; Kizildeniz et al., 2015).
In multistress experiments with Temperanillo fruiting cuttings where future temperature (+4°C) and CO2 (700 ppm) conditions where simulated, high CO2 in particular when combined with high temperature hastened berry ripening, sugar accumulation, malic acid respiration and reduced the aforementioned high temperature induced anthocyanin–sugar decoupling (Arrizabalaga-Arriazu et al., 2020a).
The effect of eCO2 on aroma compounds remains to be elucidated. Goncalves et al. (2009) found no impact on aroma compounds such as C6 alcohols, alcohols, esters, terpenols, carbonyl compounds, acids, volatile phenols, and C13 norisoprenoids under moderate eCO2 (500 ppm). However, the same treatment induced an increased level of ethyl 2-methylbutyrate, isoamyl acetate, ethyl hexanoate, and ethyl octanoate.
Biases Generated By Berry Heterogeneity and Volume Variation
Empirically, winegrowers and berry physiologists know that grape ripening is a very heterogenous process which can easily by visually perceived around véraison when, in red grape cultivars, berries of the same cluster change color asynchronously. For “fine scale” physiological studies that aim to reveal the effect of climate and abiotic factors on grape berry composition, the random time scale of berry sampling that is commonly adopted can cause important biases in chemical composition and gene expression data, which can potentially mask important physiological information. Poor correlation of phenotypic and transcriptomic data often observed in abiotic and biotic stress studies can be partially attributed to berry heterogeneity and sampling strategy.
Several molecular studies tried to address the complex issue of grape ripening on a single berry scale (Gouthu et al., 2014; Rienth et al., 2014b, 2016; Shahood et al., 2015, 2020; Carbonell-Bejerano et al., 2016; Rösti et al., 2018). For instance, Carbonell-Bejerano et al. (2016) performed berry density sorting by berry flotation in NaCl solutions and assessed the transcriptome of different berry ripening groups and showed that gene expression profiles clearly relate with ripening progression of different sorted berry groups. By contrast, when the same density series were sampled on two different dates from the same vineyard of Tempranillo, considerable differences were detected, which indicated that environmental differences between both sampling moments determined most of these expression differences. Latter findings highlight evidence on the convenience of a homogenization of the developmental stage and the sampling time condition for transcriptome comparisons by berry density sorting.
Previous heat stress studies using RNA from single berries sorted according to their biochemical characteristics led to the discovery of anthocyanin biosynthesis related transcripts (Rienth et al., 2014b) that have not been detected in other studies. In several studies (Carbonell-Bejerano et al., 2013; Lecourieux et al., 2017; Pastore et al., 2017), anthocyanin concentration was significantly decreased by heat stress, but the expression pattern of key anthocyanin regulator genes such as VviMybA1 and VviAOMT did not show concomitant repressions as opposed to the heat stress study of Rienth et al. (2014a,b), where berry batches where constructed according to berry biochemical characteristics. Similar discrepancies in gene expression data were observed in molecular studies conducted on berries of virus infected vines and emphasize the heterogeneity problem, where the gene expression results changed if berries were sampled at same calendar day without sorting (Vega et al., 2011) or if individual berries were grouped according to their “internal ripening clock” (Ghaffari et al., 2020). The “highest precision” is in our opinion obtained by single berry analysis of sugar and acids and a subsequent grouping of berries according to those to parameters. Obviously, such sampling strategies are labor intensive and above all time consuming. A good compromise could be berry sorting by density, which, however, will require some time for the sorting before the berries can be frozen in liquid nitrogen, during which time the transcriptome of the abscised berry may evolve leading to biases. An alternative, less time-consuming, sampling approach is to measure sugar concentration of each berry prior to N-freezing as adopted in a recent study by Cramer et al. (2020). Researchers need to weigh the advantages and drawback of different sampling strategies for each experiment and when interpreting their results.
Another potential issue is related to how the levels of compounds are expressed. This can prevent researchers from drawing clear physiological conclusions. Commonly, metabolite (sugars, acids, phenolic compounds, etc.) levels in the grape berry are expressed as concentration, i.e., amount per volume (L) or weight (g), because the metabolite concentration in the wine is more closely related to the metabolite concentration in the berry than to the total metabolite amount in the berry. The metabolite concentration depends on the amount accumulated in the cells and the berry volume which change dramatically during development and/or due to responses to abiotic stresses (e.g., limited water availability that decreases berry size). Therefore, expressing metabolites as concentrations can lead to misinterpretations of the effects of specific treatments or stresses on the metabolic responses of the berry (Famiani et al., 2015; Moscatello et al., 2019). Thus, expressing both the concentration and the amount per berry of a given metabolite allows for a better identification of direct and indirect effects of treatments/factors on such metabolite.
Conclusion
A changing climate requires a profound knowledge of how abiotic factors modulate different quality-determining compounds of the grape berry in order to implement appropriate viticultural mitigation strategies (van Leeuwen and Destrac-Irvine, 2017; Rienth et al., 2020), select varieties, clones (Wolkovich et al., 2018), and rootstocks (Ollat et al., 2016), and to identify traits, genes, or QTLs for the breeding of new cultivars better adapted to future conditions (Duchêne, 2016).
The physiological and molecular knowledge of the mechanisms involved in the biosynthesis and degradation of secondary metabolites in the grape berry has significantly increased in the past two decades and is continuously advancing due to the development and improvement of omic tools. However, the impact of environmental factors, notably light and temperature, is often ambiguous (van Leeuwen et al., 2020). This can partly be attributed to the difficulty in separating light and temperature effects and the interaction between the two. A further often neglected bottleneck in physiological studies on grape berry responses to climate and abiotic factors is the effect of heterogeneity and the aforementioned berry sampling strategy, which may impair our capability to analyses complex metabolic events. Post-transcriptional and epigenetic regulation of metabolism, which have so far rarely been addressed in physiological studies, might play important roles in the endogenous and exogenous modulation of secondary metabolism in the grape berry.
Facing global warming, viticultural practices such as cluster and shoot thinning or leaf removal, historically considered as improving quality, need to be reconsidered and adapted to changing conditions (van Leeuwen and Destrac-Irvine, 2017; Torres et al., 2020a,b). Moreover, field data on the impact of increased atmospheric CO2 concentration on berry metabolism are scarce and need to be further investigated, in particular in combination with different abiotic stresses such as increased temperature and drought. Though difficult to conduct in field conditions and so far only carried out on fruiting cuttings (Salazar-Parra et al., 2012; Kizildeniz et al., 2015; Martinez-Luscher et al., 2015; Arrizabalaga-Arriazu et al., 2020a,b) such multi-stress experiments will improve understanding of how climate change will impact vine and berry physiology, and will help develop mitigation strategies.
Data Availability Statement
The original contributions presented in the study are included in the article/supplementary material, further inquiries can be directed to the corresponding author/s.
Author Contributions
MR and SDC devised the main body and structure and content of the manuscript. PD, CS, CBu, CBo, NV, RW, and FF provided valuable ideas and triggered fruitful discussions via helpful comments and provided corrections. All others approved the final version of the manuscript.
Funding
The post-doc of NV was financed by the Swiss National Science Fondation (SNF) Project Grant IZCOZ0_189896.
Conflict of Interest
The authors declare that the research was conducted in the absence of any commercial or financial relationships that could be construed as a potential conflict of interest.
The reviewer ZD declared a past co-authorship with one of the authors PD to the handling editor.
References
Abeysinghe, M. S., Greer, D., and Rogiers, S. (2019). The effect of light intensity and temperature on berry growth and sugar accumulation in Vitis Vinifera “Shiraz” under vineyard conditions. Vitis 58, 7–16. doi: 10.5073/vitis.2019.58.7-16
Adams, D. O. (2006). Phenolics and Ripening in Grape Berries. Am. J. Enol. Vitic. 57, 249–256. Available online at: https://www.ajevonline.org/content/57/3/249
Agati, G., Brunetti, C., Di Ferdinando, M., Ferrini, F., Pollastri, S., and Tattini, M. (2013). Functional roles of flavonoids in photoprotection: new evidence, lessons from the past. Plant Physiol. Biochem. 72, 35–45. doi: 10.1016/j.plaphy.2013.03.014
Ageorges, A., Fernandez, L., Vialet, S., Merdinoglu, D., Terrier, N., and Romieu, C. (2006). Four specific isogenes of the anthocyanin metabolic pathway are systematically co-expressed with the red colour of grape berries. Plant Sci. 170, 372–383. doi: 10.1016/j.plantsci.2005.09.007
Ahrazem, O., Trapero, A., Gomez, M. D., Rubio-Moraga, A., and Gomez-Gomez, L. (2010). Genomic analysis and gene structure of the plant carotenoid dioxygenase 4 family: a deeper study in Crocus sativus and its allies. Genomics 96, 239–250. doi: 10.1016/j.ygeno.2010.07.003
Ainsworth, E. A. (2008). Rice production in a changing climate: a meta-analysis of responses to elevated carbon dioxide and elevated ozone concentration. Glob. Chang. Biol. 14, 1642–1650. doi: 10.1111/j.1365-2486.2008.01594.x
Ainsworth, E. A., and Long, S. P. (2005). What have we learned from 15 years of free-air CO2 enrichment (FACE)? A meta-analytic review of the responses of photosynthesis, canopy properties and plant production to rising CO2. New Phytol. 165, 351–371. doi: 10.1111/j.1469-8137.2004.01224.x
Ainsworth, E. A., and Rogers, A. (2007). The response of photosynthesis and stomatal conductance to rising [CO2]: mechanisms and environmental interactions. Plant Cell Environ. 30, 258–270. doi: 10.1111/j.1365-3040.2007.01641.x
Akinwumi, B. C., Bordun, K. M., and Anderson, H. D. (2018). Biological activities of stilbenoids. Int. J. Mol. Sci. 19:792. doi: 10.3390/ijms19030792
Alem, H., Rigou, P., Schneider, R., Ojeda, H., and Torregrosa, L. (2019). Impact of agronomic practices on grape aroma composition: a review. J. Sci. Food Agric. 99, 975–985. doi: 10.1002/jsfa.9327
Ali, K., Maltese, F., Choi, Y. H., and Verpoorte, R. (2010). Metabolic constituents of grapevine and grape-derived products. Phytochem. Rev. 9, 357–378. doi: 10.1007/s11101-009-9158-0
Allamy, L., Darriet, P., and Pons, A. (2018). Molecular interpretation of dried-fruit aromas in merlot and cabernet sauvignon musts and young wines: impact of over-ripening. Food Chem. 266, 245–253. doi: 10.1016/j.foodchem.2018.06.022
Allen, M. S., Lacey, M. J., Harris, R. L. N., and Brown, W. V. (1991). Contribution of methoxypyrazines to sauvignon blanc wine aroma. Am. J. Enol. Viticult. 42, 109–112.
Arrizabalaga, M., Morales, F., Oyarzun, M., Delrot, S., Gomès, E., Irigoyen, J. J., et al. (2018). Tempranillo clones differ in the response of berry sugar and anthocyanin accumulation to elevated temperature. Plant Sci. 267, 74–83. doi: 10.1016/j.plantsci.2017.11.009
Arrizabalaga-Arriazu, M., Gomès, E., Morales, F., Irigoyen, J. J., Pascual, I., and Hilbert, G. (2020a). High temperature and elevated carbon dioxide modify berry composition of different clones of grapevine (Vitis vinifera L.) cv. tempranillo. Front. Plant Sci. 11:603687. doi: 10.3389/fpls.2020.603687
Arrizabalaga-Arriazu, M., Morales, F., Irigoyen, J. J., Hilbert, G., and Pascual, I. (2020b). Growth performance and carbon partitioning of grapevine Tempranillo clones under simulated climate change scenarios: elevated CO2 and temperature. J. Plant Physiol. 252:153226. doi: 10.1016/j.jplph.2020.153226
Azuma, A. (2018). Genetic and environmental impacts on the biosynthesis of anthocyanins in grapes. Hortic. J. 87, 1–17. doi: 10.2503/hortj.OKD-IR02
Azuma, A., Yakushiji, H., Koshita, Y., and Kobayashi, S. (2012). Flavonoid biosynthesis-related genes in grape skin are differentially regulated by temperature and light conditions. Planta 236, 1067–1080. doi: 10.1007/s00425-012-1650-x
Bais, A. F., Bernhard, G., McKenzie, R. L., Aucamp, P. J., Young, P. J., Ilyas, M., et al. (2019). Ozone-climate interactions and effects on solar ultraviolet radiation. Photochem. Photobiol. Sci. 18, 602–640. doi: 10.1039/C8PP90059K
Bais, A. F., Lucas, R. M., Bornman, J. F., Williamson, C. E., Sulzberger, B., Austin, A. T., et al. (2018). Environmental effects of ozone depletion, UV radiation and interactions with climate change: UNEP environmental effects assessment panel, update 2017. Photochem. Photobiol. Sci. 17, 127–179. doi: 10.1039/C7PP90043K
Ballaré, C. L., Caldwell, M. M., Flint, S. D., Robinson, S. A., and Bornman, J. F. (2011). Effects of solar ultraviolet radiation on terrestrial ecosystems. Patterns, mechanisms, and interactions with climate change. Photochem Photobiol Sci. 10, 226–241. doi: 10.1039/c0pp90035d
Battilana, J., Costantini, L., Emanuelli, F., Sevini, F., Segala, C., Moser, S., et al. (2009). The 1-deoxy-D: -xylulose 5-phosphate synthase gene co-localizes with a major QTL affecting monoterpene content in grapevine. Theor. Appl. Genet. 118, 653–669. doi: 10.1007/s00122-008-0927-8
Battilana, J., Emanuelli, F., Gambino, G., Gribaudo, I., Gasperi, F., Boss, P. K., et al. (2011). Functional effect of grapevine 1-deoxy-D-xylulose 5-phosphate synthase substitution K284N on muscat flavour formation. J. Exp. Bot. 62, 5497–5508. doi: 10.1093/jxb/err231
Bavaresco, L., Lucini, L., Busconi, M., Flamini, R., and De Rosso, M. (2016). Wine resveratrol: from the ground up. Nutrients 8:222. doi: 10.3390/nu8040222
Bergqvist, J., Dokoozlian, N., and Ebisuda, N. (2001). Sunlight exposure and temperature effects on berry growth and composition of Cabernet Sauvignon and Grenache in the Central San Joaquin Valley of California. Am. J. Enol. Vitic. 52, 1–7. Available online at: https://www.ajevonline.org/content/52/1/1
Biais, B., Krisa, S., Cluzet, S., Da Costa, G., Waffo-Teguo, P., Mérillon, J.-M., et al. (2017). Antioxidant and cytoprotective activities of grapevine stilbenes. J. Agric. Food Chem. 65, 4952–4960. doi: 10.1021/acs.jafc.7b01254
Bindi, M., Fibbi, L., and Miglietta, F. (2001). Free air CO2 enrichment (FACE) of grapevine (Vitis vinifera L.): II. Growth and quality of grape and wine in response to elevated CO2 concentrations. Eur. J. Agron. 14, 145–155. doi: 10.1016/S1161-0301(00)00093-9
Bindon, K. A., Dry, P. R., and Loveys, B. R. (2007). Influence of plant water status on the production of C13-norisoprenoid precursors in Vitis vinifera L. Cv. cabernet sauvignon grape berries. J. Agric. Food Chem. 55, 4493–4500. doi: 10.1021/jf063331p
Black, C. A., Parker, M., Siebert, T. E., Capone, D. L., and Francis, I. L. (2015). Terpenoids and their role in wine flavour: recent advances. Aust. J. Grape Wine Res. 21, 582–600. doi: 10.1111/ajgw.12186
Blancquaert, E. H., Oberholster, A., Ricardo-da-Silva, J. M., and Deloire, A. J. (2019). Grape flavonoid evolution and composition under altered light and temperature conditions in cabernet sauvignon (Vitis vinifera L.). Front. Plant Sci. 10:1062. doi: 10.3389/fpls.2019.01062
Bogs, J., Downey, M. O., Harvey, J. S., Ashton, A. R., Tanner, G. J., and Robinson, S. P. (2005). Proanthocyanidin synthesis and expression of genes encoding leucoanthocyanidin reductase and anthocyanidin reductase in developing grape berries and grapevine leaves. Plant Physiol. 139, 652–663. doi: 10.1104/pp.105.064238
Bogs, J., Jaffe, F. W., Takos, A. M., Walker, A. R., and Robinson, S. P. (2007). The grapevine transcription factor VvMYBPA1 regulates proanthocyanidin synthesis during fruit development. Plant Physiol. 143, 1347–1361. doi: 10.1104/pp.106.093203
Bohlmann, J., and Keeling, C. I. (2008). Terpenoid biomaterials. Plant J. 54, 656–669. doi: 10.1111/j.1365-313X.2008.03449.x
Bonada, M., and Sadras, V. O. (2015). Review: critical appraisal of methods to investigate the effect of temperature on grapevine berry composition. Aust. J. Grape Wine Res. 21, 1–17. doi: 10.1111/ajgw.12102
Boss, P., Davies, C., and Robinson, S. (1996a). Expression of anthocyanin biosynthesis pathway genes in red and white grapes. Plant Mol. Biol. 32, 565–569. doi: 10.1007/BF00019111
Boss, P. K., Davies, C., and Robinson, S. P. (1996b). Analysis of the expression of anthocyanin pathway genes in developing Vitis vinifera L. cv Shiraz grape berries and the implications for pathway regulation. Plant Physiol. 111, 1059–1066. doi: 10.1104/pp.111.4.1059
Bottcher, C., Keyzers, R. A., Boss, P. K., and Davies, C. (2010). Sequestration of auxin by the indole-3-acetic acid-amido synthetase GH3-1 in grape berry (Vitis vinifera L.) and the proposed role of auxin conjugation during ripening. J. Exp. Bot. 61, 3615–3625. doi: 10.1093/jxb/erq174
Bouchilloux, P., Darriet, P., Henry, R., Lavigne-Cruège, V., and Dubourdieu, D. (1998). Identification of volatile and powerful odorous thiols in bordeaux red wine varieties. J. Agric. Food Chem. 46, 3095–3099. doi: 10.1021/jf971027d
Braidot, E., Zancani, M., Petrussa, E., Peresson, C., Bertolini, A., Patui, S., et al. (2008). Transport and accumulation of flavonoids in grapevine (Vitis vinifera L.). Plant Signal. Behav. 3, 626–632. doi: 10.4161/psb.3.9.6686
Brandt, M., Scheidweiler, M., Rauhut, D., Claus-Dieter, P., Will, F., et al. (2019). The influence of temperature and solar radiation on phenols in berry skin and maturity parameters of Vitis vinifera L. cv. riesling. OENO One 53, 261–276. doi: 10.20870/oeno-one.2019.53.2.2424
Brillante, L., Martínez-Lüscher, J., and Kurtural, S. K. (2018). Applied water and mechanical canopy management affect berry and wine phenolic and aroma composition of grapevine (Vitis vinifera L., cv. Syrah) in Central California. Sci. Hortic. 227, 261–271. doi: 10.1016/j.scienta.2017.09.048
Bureau, S. M., Razungles, A. J., and Baumes, R. L. (2000). The aroma of muscat of frontignan grapes: effect of the light environment of vine or bunch on volatiles and glycoconjugates. J. Sci. Food Agric. 80, 2012–2020. doi: 10.1002/1097-0010(200011)80:14<2012::AID-JSFA738>3.0.CO;2-X
Buttrose, M. S., HALE, C. R., and Kliewer, W. M. (1971). Effect of temperature on the composition of Cabernet Sauvignon Berries. Am. J. Enol. Viticutlure 22, 71–75.
Cáceres-Mella, A., Talaverano, M. I., Villalobos-González, L., Ribalta-Pizarro, C., and Pastenes, C. (2017). Controlled water deficit during ripening affects proanthocyanidin synthesis, concentration and composition in Cabernet Sauvignon grape skins. Plant Physiol. Biochem. 117, 34–41. doi: 10.1016/j.plaphy.2017.05.015
Cadot, Y., Minana-Castello, M. T., and Chevalier, M. (2006). Anatomical, histological, and histochemical changes in grape seeds from Vitis vinifera L. cv Cabernet franc during fruit development. J. Agric. Food Chem. 54, 9206–9215. doi: 10.1021/jf061326f
Capone, D. L., Jeffery, D. W., and Sefton, M. A. (2012). Vineyard and fermentation studies to elucidate the origin of 1,8-cineole in Australian red wine. J. Agric. Food Chem. 60, 2281–2287. doi: 10.1021/jf204499h
Carbonell-Bejerano, P., Diago, M. P., Martinez-Abaigar, J., Martinez-Zapater, J. M., Tardaguila, J., and Nunez-Olivera, E. (2014). Solar ultraviolet radiation is necessary to enhance grapevine fruit ripening transcriptional and phenolic responses. BMC Plant Biol. 14:183. doi: 10.1186/1471-2229-14-183
Carbonell-Bejerano, P., Rodríguez, V., Hernáiz, S., Royo, C., Dal Santo, S., Pezzotti, M., et al. (2016). Reducing sampling bias in molecular studies of grapevine fruit ripening: transcriptomic assessment of the density sorting method. Theor. Exp. Plant Physiol. 28, 109–129. doi: 10.1007/s40626-016-0059-5
Carbonell-Bejerano, P., Santa Maria, E., Torres-Perez, R., Royo, C., Lijavetzky, D., Bravo, G., et al. (2013). Thermotolerance responses in ripening berries of Vitis vinifera L. cv Muscat Hamburg. Plant Cell Physiol. 54, 1200–1216. doi: 10.1093/pcp/pct071
Cassandra, M. P., Edward, W. H., and Thayne, M. (2019). Light and temperature independently influence methoxypyrazine content of Vitis vinifera (cv. Cabernet Sauvignon) Berries. HortSci. Horts 54, 282–288. doi: 10.21273/HORTSCI13634-18
Castellarin, S. D., Bavaresco, L., Falginella, L., Gonçalves, M. I. V. Z., and di Gaspero, G. (2012). “Phenolics in grape berry and key antioxidants,” in In The Biochemistry of the Grape Berry, eds H. Gerós, M. Chaves, and S. Delrot (Bussum: Bentham Science), 89–110. doi: 10.2174/978160805360511201010089
Castellarin, S. D., Gambetta, G. A., Wada, H., Shackel, K. A., and Matthews, M. A. (2011). Fruit ripening in Vitis vinifera: spatiotemporal relationships among turgor, sugar accumulation, and anthocyanin biosynthesis. J. Exp. Bot. 62, 4345–4354. doi: 10.1093/jxb/err150
Castellarin, S. D., Matthews, M. A., Di Gaspero, G., and Gambetta, G. A. (2007a). Water deficits accelerate ripening and induce changes in gene expression regulating flavonoid biosynthesis in grape berries. Planta 227, 101–112. doi: 10.1007/s00425-007-0598-8
Castellarin, S. D., Pfeiffer, A., Sivilotti, P., Degan, M., and Peterlunger, E. G.. (2007b). Transcriptional regulation of anthocyanin biosynthesis in ripening fruits of grapevine under seasonal water deficit. Plant Cell Environ. 30, 1381–1399. doi: 10.1111/j.1365-3040.2007.01716.x
Cavallini, E., Matus, J. T., Finezzo, L., Zenoni, S., Loyola, R., Guzzo, F., et al. (2015). The phenylpropanoid pathway is controlled at different branches by a set of R2R3-MYB C2 repressors in grapevine. Plant Physiol. 167, 1448–1470. doi: 10.1104/pp.114.256172
Celette, F., and Gary, C. (2013). Dynamics of water and nitrogen stress along the grapevine cycle as affected by cover cropping. Eur. J. Agron. 45, 142–152. doi: 10.1016/j.eja.2012.10.001
Chalons, P., Amor, S., Courtaut, F., Cantos-Villar, E., Richard, T., Auger, C., et al. (2018). Study of potential anti-inflammatory effects of red wine extract and resveratrol through a modulation of interleukin-1-beta in macrophages. Nutrients 10:1856. doi: 10.3390/nu10121856
Chen, F., Tholl, D., Bohlmann, J., and Pichersky, E. (2011). The family of terpene synthases in plants: a mid-size family of genes for specialized metabolism that is highly diversified throughout the kingdom. Plant J. 66, 212–229. doi: 10.1111/j.1365-313X.2011.04520.x
Chipperfield, M. P., Bekki, S., Dhomse, S., Harris, N. R. P., Hassler, B., Hossaini, R., et al. (2017). Detecting recovery of the stratospheric ozone layer. Nature 549:211. doi: 10.1038/nature23681
Chisari, M., Barbagallo, R. N., and Spagna, G. (2007). Characterization of polyphenol oxidase and peroxidase and influence on browning of cold stored strawberry fruit. J. Agric. Food Chem. 55, 3469–3476. doi: 10.1021/jf063402k
Cholet, C., Claverol, S., Claisse, O., Rabot, A., Osowsky, A., Dumot, V., et al. (2016). Tartaric acid pathways in Vitis vinifera L. (cv. Ugni blanc): a comparative study of two vintages with contrasted climatic conditions. BMC Plant Biol. 16:144. doi: 10.1186/s12870-016-0833-1
Chong, J., Poutaraud, A., and Hugueney, P. (2009). Metabolism and roles of stilbenes in plants. Plant Sci. 177, 143–155. doi: 10.1016/j.plantsci.2009.05.012
Chorti, E., Guidoni, S., Ferrandino, A., and Novello, V. (2010). Effect of different cluster sunlight exposure levels on ripening and anthocyanin accumulation in nebbiolo grapes. Am. J. Enol. Vitic. 61, 23–30. Available online at: https://www.ajevonline.org/content/61/1/23
Chorti, E., Kyraleou, M., Kallitharaka, S., Pavlidis, M., Koundouras, S., and Kotseridis, Y. (2016). Irrigation and leaf removal effects on polyphenolic content of grapes and wines produced from cv. “Agiorgitiko” (Vitis vinifera L.). Notulae Botanicae Horti Agrobotanici Cluj-Napoca 44, 133–139. doi: 10.15835/nbha44110254
Chuine, I., Yiou, P., Viovy, N., Seguin, B., Daux, V., and Ladurie, E. L. R. (2004). Grape ripening as a past climate indicator. Nature 432, 289–290. doi: 10.1038/432289a
Cohen, S. D., Tarara, J. M., Gambetta, G. A., Matthews, M. A., and Kennedy, J. A. (2012a). Impact of diurnal temperature variation on grape berry development, proanthocyanidin accumulation, and the expression of flavonoid pathway genes. J. Exp. Bot. 63, 2655–2665. doi: 10.1093/jxb/err449
Cohen, S. D., Tarara, J. M., and Kennedy, J. A. (2012b). Diurnal temperature range compression hastens berry development and modifies flavonoid partitioning in grapes. Am. J. Enol. Vitic, 63, 112–120. doi: 10.5344/ajev.2011.11015
Conde, C., Silva, P., Fontes, N., Dias, A. C. P., Tavares, R. M., Sousa, M. J., et al. (2007). Biochemical changes throughout grape berry development and fruit and wine quality. Food 1, 1–22.
Conn, S., Curtin, C., Bezier, A., Franco, C., and Zhang, W. (2008). Purification, molecular cloning, and characterization of glutathione S-transferases (GSTs) from pigmented Vitis vinifera L. cell suspension cultures as putative anthocyanin transport proteins. J. Exp. Bot. 59, 3621–3634. doi: 10.1093/jxb/ern217
Cook, B. I., and Wolkovich, E. M. (2016). Climate change decouples drought from early wine grape harvests in France. Nat. Clim. Chang. 6:715. doi: 10.1038/nclimate2960
Cook, M. G., Zhang, Y., Nelson, C. J., Gambetta, G., Kennedy, J. A., and Kurtural, S. K. (2015). Anthocyanin composition of merlot is ameliorated by light microclimate and irrigation in Central California. Am. J. Enol. Vitic. 66, 266–278. doi: 10.5344/ajev.2015.15006
Coombe, B. G. (1987). Influence of temperature on composition and quality of grapes. Acta Hortic. 206, 23–36. doi: 10.17660/ActaHortic.1987.206.1
Copolovici, L., and Niinemets, Ü. (2016). “Environmental impacts on plant volatile emission,” in Deciphering Chemical Language of Plant Communication, eds J. D. Blande, and R. Glinwood (Cham: Springer International Publishing), 35–59. doi: 10.1007/978-3-319-33498-1_2
Costantini, L., Kappel, C. D., Trenti, M., Battilana, J., Emanuelli, F., Sordo, M., et al. (2017). Drawing links from transcriptome to metabolites: the evolution of aroma in the ripening berry of Moscato Bianco (Vitis vinifera L.). Front. Plant Sci. 8:780. doi: 10.3389/fpls.2017.00780
Cramer, G., Ergul, A., Grimplet, J., Tillett, R., Tattersall, E., Bohlman, M., et al. (2007). Water and salinity stress in grapevines: early and late changes in transcript and metabolite profiles. Funct. Integr. Genomics 7, 111–134. doi: 10.1007/s10142-006-0039-y
Cramer, G. R., Cochetel, N., Ghan, R., Destrac-Irvine, A., and Delrot, S. (2020). A sense of place: transcriptomics identifies environmental signatures in Cabernet Sauvignon berry skins in the late stages of ripening. BMC Plant Biol. 20:41. doi: 10.1186/s12870-020-2251-7
Cramer, G. R., Ghan, R., Schlauch, K. A., Tillett, R. L., Heymann, H., Ferrarini, A., et al. (2014). Transcriptomic analysis of the late stages of grapevine (Vitis vinifera cv. Cabernet Sauvignon) berry ripening reveals significant induction of ethylene signaling and flavor pathways in the skin. BMC Plant Biol. 14:370. doi: 10.1186/s12870-014-0370-8
Czemmel, S., Holl, J., Loyola, R., Arce-Johnson, P., Alcalde, J. A., Matus, J. T., et al. (2017). Transcriptome-wide identification of novel UV-B- and light modulated flavonol pathway genes controlled by VviMYBF1. Front. Plant Sci. 8:1084. doi: 10.3389/fpls.2017.01084
Czemmel, S., Stracke, R., Weisshaar, B., Cordon, N., Harris, N., Walker, A., et al. (2009). The grapevine R2R3-MYB transcription factor VvMYBF1 regulates flavonol synthesis in developing grape berries. Plant Physiol. 151, 1513–1530. doi: 10.1104/pp.109.142059
Darriet, P., Thibon, C., and Dubourdieu, D. (2012). “Aroma and aroma precursors in grape,” in The Biochemistry of the Grape Berry, eds H. Gerós, M. Chaves, and S. Delrot (Bussum, NLD: Bentham Science Publishers Ltd.), 111–136. doi: 10.2174/978160805360511201010111
Darriet, P., Tominaga, T., Lavigne, V., Boidron, J.-N., and Dubourdieu, D. (1995). Identification of a powerful aromatic component of Vitis vinifera L. var. sauvignon wines: 4-mercapto-4-methylpentan-2-one. Flav. Frag. J. 10, 385–392. doi: 10.1002/ffj.2730100610
Davies, C., Boss, P., and Robinson, S. (1997). Treatment of grape berries, a nonclimacteric fruit with a synthetic auxin, retards ripening and alters the expression of developmentally regulated genes. Plant Physiol. 115, 1155–1161. doi: 10.1104/pp.115.3.1155
De Billerbeck, G. M., Ebang-Oke, J. P., and Ambid, C. (2003). “Oenologie 2003,” in 6th Int Symposium of Enology (Bordeaux).
de Rosas, I., Ponce, M. T., Malovini, E., Deis, L., Cavagnaro, B., and Cavagnaro, P. (2017). Loss of anthocyanins and modification of the anthocyanin profiles in grape berries of Malbec and Bonarda grown under high temperature conditions. Plant Sci. 258, 137–145. doi: 10.1016/j.plantsci.2017.01.015
Deluc, L., Barrieu, F., Marchive, C., Lauvergeat, V., Decendit, A., Richard, T., et al. (2006). Characterization of a grapevine R2R3-MYB transcription factor that regulates the phenylpropanoid pathway. Plant Physiol. 140, 499–511. doi: 10.1104/pp.105.067231
Deluc, L., Decendit, A., Papastamoulis, Y., Merillon, J., Cushman, J., and Cramer, G. (2011). Water deficit increases stilbene metabolism in Cabernet Sauvignon berries. J. Agric. Food Chem. 59, 289–297. doi: 10.1021/jf1024888
Deluc, L., Quilici, D., Decendit, A., Grimplet, J., Wheatley, M., Schlauch, K., et al. (2009). Water deficit alters differentially metabolic pathways affecting important flavor and quality traits in grape berries of Cabernet Sauvignon and Chardonnay. BMC Genomics 10:212. doi: 10.1186/1471-2164-10-212
Deng, Y., and Lu, S. (2017). Biosynthesis and regulation of phenylpropanoids in plants. CRC Crit. Rev. Plant Sci. 36, 257–290. doi: 10.1080/07352689.2017.1402852
des Gachons, C. P., Leeuwen, C. V., Tominaga, T., Soyer, J.-P., Gaudillère, J.-P., and Dubourdieu, D. (2005). Influence of water and nitrogen deficit on fruit ripening and aroma potential of Vitis vinifera L cv Sauvignon blanc in field conditions. J. Agric. Food Chem. 85, 73–85. doi: 10.1002/jsfa.1919
Dokoozlian, N., and Kliewer, W. M. (1996). Influence of light on grape berry growth and composition varies during fruit development J. Amer. Soc. Hort. Sci. 121, 869–874. doi: 10.21273/JASHS.121.5.869
D'Onofrio, C., Matarese, F., and Cuzzola, A. (2018). Effect of methyl jasmonate on the aroma of Sangiovese grapes and wines. Food Chem. 242, 352–361. doi: 10.1016/j.foodchem.2017.09.084
Downey, M. O., Dokoozlian, N. K., and Krstic, M. P. (2006). Cultural practice and environmental impacts on the flavonoid composition of grapes and wine: a review of recent research. Am. J. Enol. Vitic. 57, 257–268. Available online at: https://www.ajevonline.org/content/57/3/257
Downey, M. O., Harvey, J. S., and Robinson, S. P. (2004). The effect of bunch shading on berry development and flavonoid accumulation in Shiraz grapes. Aust. J. Grape Wine Res. 10, 55–73. doi: 10.1111/j.1755-0238.2004.tb00008.x
Drew, D. P., Andersen, T. B., Sweetman, C., Moller, B. L., Ford, C., and Simonsen, H. T. (2016). Two key polymorphisms in a newly discovered allele of the Vitis vinifera TPS24 gene are responsible for the production of the rotundone precursor alpha-guaiene. J. Exp. Bot. 67, 799–808. doi: 10.1093/jxb/erv491
Duchêne, E. (2016). How can grapevine genetics contribute to the adaptation to climate change? OENO One 50, 113–119. doi: 10.20870/oeno-one.2016.50.3.98
Duchêne, E., and Schneider, C. (2005). Grapevine and climatic changes: a glance at the situation in Alsace. Agron. Sustain. Dev. 25, 93–99. doi: 10.1051/agro:2004057
Duchene, E., Butterlin, G., Claudel, P., Dumas, V., Jaegli, N., and Merdinoglu, D. (2009a). A grapevine (Vitis vinifera L.) deoxy-D-xylulose synthase gene colocates with a major quantitative trait loci for terpenol content. Theor. Appl. Genet. 118, 541–552. doi: 10.1007/s00122-008-0919-8
Duchene, E., Legras, J. L., Karst, F., Merdinoglu, D., Claudel, P., Jaegli, N., et al. (2009b). Variation of linalool and geraniol content within two pairs of aromatic and non-aromatic grapevine clones. Aust. J. Grape Wine Res. 15, 120–130. doi: 10.1111/j.1755-0238.2008.00039.x
Dunlevy, J. D., Dennis, E. G., Soole, K. L., Perkins, M. V., Davies, C., and Boss, P. K. (2013). A methyltransferase essential for the methoxypyrazine-derived flavour of wine. Plant J. 75, 606–617. doi: 10.1111/tpj.12224
Edwards, E. J., Unwin, D., Kilmister, R., and Treeby, M. (2017). Multi-seasonal effects of warming and elevated CO2; on the physiology, growth and production of mature, field grown, Shiraz grapevines. OENO One 51, 127–132. doi: 10.20870/oeno-one.2017.51.2.1586
Edwards, E. J., Unwin, D. J., Sommer, K. J., Downey, M. O., and Mollah, M. (2016). The response of commercially managed, field grown, grapevines (Vitis vinifera L.) to a simulated future climate consisting of elevated CO2 in combination with elevated air temperature. Acta Hortic. 1115, 103–110. doi: 10.17660/ActaHortic.2016.1115.16
Enriquez-Alonso, A., Sanchez-Lorenzo, A., Calbó, J., González, J.-A., and Norris, J. R. (2016). Cloud cover climatologies in the Mediterranean obtained from satellites, surface observations, reanalyses, and CMIP5 simulations: validation and future scenarios. Clim. Dyn. 47, 249–269. doi: 10.1007/s00382-015-2834-4
Etienne, A., Genard, M., Lobit, P., Mbeguie-A-Mbeguie, D., and Bugaud, C. (2013). What controls fleshy fruit acidity? A review of malate and citrate accumulation in fruit cells. J. Exp. Bot. 64, 1451–1469. doi: 10.1093/jxb/ert035
Falcão, L. D., de Revel, G., Perello, M. C., Moutsiou, A., Zanus, M. C., and Bordignon-Luiz, M. T. (2007). A Survey of seasonal temperatures and vineyard altitude influences on 2-methoxy-3-isobutylpyrazine, C13-norisoprenoids, and the sensory Profile of Brazilian Cabernet Sauvignon wines. J. Agric. Food Chem. 55, 3605–3612. doi: 10.1021/jf070185u
Falginella, L., Di Castellarin, S., Testolin, R., Gambetta, G., Morgante, M., and Di Gaspero, G. (2010). Expansion and subfunctionalization of flavonoid 3′,5′-hydroxylases in the grapevine lineage. BMC Genomics 11:562. doi: 10.1186/1471-2164-11-562
Falginella, L., Di Gaspero, G., and Castellarin, S. D. (2012). Expression of flavonoid genes in the red grape berry of 'Alicante Bouschet' varies with the histological distribution of anthocyanins and their chemical composition. Planta 236, 1037–1051. doi: 10.1007/s00425-012-1658-2
Famiani, F., Battistelli, A., Moscatello, S., Cruz-Castillo, J. G., and Walker, R. P. (2015). The organic acids that are accumulated in the flesh of fruits: occurrence, metabolism and factors affecting their contents - a review. Rev. Chapingo Serie Hortic. 21, 97–129. doi: 10.5154/r.rchsh.2015.01.004
Favre, G., Piccardo, D., Sergio, G.-A., Pérez-Navarro, J., García-Romero, E., Mena-Morales, A., et al. (2020). Stilbenes in grapes and wines of Tannat, Marselan and Syrah from Uruguay. OENO One 54, 27–36. doi: 10.20870/oeno-one.2020.54.1.2576
Feng, H., Skinkis, P. A., and Qian, M. C. (2017). Pinot noir wine volatile and anthocyanin composition under different levels of vine fruit zone leaf removal. Food Chem. 214, 736–744. doi: 10.1016/j.foodchem.2016.07.110
Fernandes de Oliveira, A., Mercenaro, L., Del Caro, A., Pretti, L., and Nieddu, G. (2015). Distinctive anthocyanin accumulation responses to temperature and natural UV radiation of two field-grown (Vitis vinifera L.) cultivars. Molecules 20, 2061–2080. doi: 10.3390/molecules20022061
Ferreira, B., Hory, C., Bard, M. H., Taisant, C., Olsson, A., and Le Fur, Y. (1995). Effects of skin contact and settling on the level of the C18:2, C18:3 fatty acids and C6 compounds in burgundy chardonnay musts and wines. Food Qual. Prefer. 6, 35–41. doi: 10.1016/0950-3293(94)P4210-W
Fournier-Level, A., Hugueney, P., Verriès, C., This, P., and Ageorges, A. (2011). Genetic mechanisms underlying the methylation level of anthocyanins in grape (Vitis vinifera L.). BMC Plant Biol. 11:179. doi: 10.1186/1471-2229-11-179
Fraga, H., Malheiro, A. C., Moutinho-Pereira, J., and Santos, J. A. (2012). An overview of climate change impacts on European viticulture. Food Energy Secur. 1, 94–110. doi: 10.1002/fes3.14
Fraga, H., Malheiro, A. C., Moutinho-Pereira, J., and Santos, J. A. (2013). Future scenarios for viticultural zoning in Europe: ensemble projections and uncertainties. Int. J. Biometeorol. 57, 909–925. doi: 10.1007/s00484-012-0617-8
Francisco, R. M., Regalado, A., Ageorges, A., Burla, B. J., Bassin, B., Eisenach, C., et al. (2013). ABCC1, an ATP binding cassette protein from grape berry, transports anthocyanidin 3-O-Glucosides. Plant Cell 25, 1840–1854. doi: 10.1105/tpc.112.102152
Fretz, C., Kanel, S., Luisier, J. L., and Amado, R. (2005). Analysis of volatile components of petite arvine wine. Eur. Food Res. Technol. 221, 504–510. doi: 10.1007/s00217-005-1163-y
Friedel, M., Stoll, M., Patz, C. D., Will, F., and Dietrich, H. (2015). Impact of light exposure on fruit composition of white “Riesling” grape berries (Vitis vinifera L.). Vitis 54, 107–116. doi: 10.5073/vitis.2015.54.107-116
Gaiotti, F., Pastore, C., Filippetti, I., Lovat, L., Belfiore, N., and Tomasi, D. (2018). Low night temperature at veraison enhances the accumulation of anthocyanins in Corvina grapes (Vitis Vinifera L.). Sci. Rep. 8:8719. doi: 10.1038/s41598-018-26921-4
Gambetta, G. A., Herrera, J. C., Dayer, S., Feng, Q., Hochberg, U., and Castellarin, S. D. (2020). The physiology of drought stress in grapevine: towards an integrative definition of drought tolerance. J. Exp. Bot. 71, 4658–4676. doi: 10.1093/jxb/eraa245
Gatto, P., Vrhovsek, U., Muth, J., Segala, C., Romualdi, C., Fontana, P., et al. (2008). Ripening and genotype control stilbene accumulation in healthy grapes. J. Agric. Food Chem. 56, 11773–11785. doi: 10.1021/jf8017707
Geffroy, O., Armario, M., Fontaine, A., Fourure, M., Pasquier, G., Semadeni, T., et al. (2020). 3-Isobutyl-2-methoxypyrazine is neutrally perceived by consumers at usual concentrations in French Sauvignon and Fer wines from the Gaillac area. OENO One 54, 1133–1142. doi: 10.20870/oeno-one.2020.54.4.4492
Geffroy, O., Descôtes, J., Cécile, L.-G. C., Debord, C. J. P., et al. (2019). A 2-year multisite study of viticultural and environmental factors affecting rotundone concentration in Duras red wine. OENO One 53. doi: 10.20870/oeno-one.2019.53.3.2341
Geffroy, O., Descôtes, J., Serrano, E., Li Calzi, M., Dagan, L., and Schneider, R. (2018). Can a certain concentration of rotundone be undesirable in Duras red wine? A study to estimate a consumer rejection threshold for the pepper aroma compound. Aust. J. Grape Wine Res. 24, 88–95. doi: 10.1111/ajgw.12299
Geffroy, O., Dufourcq, T., Carcenac, D., Siebert, T., Herderich, M., and Serrano, E. (2014). Effect of ripeness and viticultural techniques on the rotundone concentration in red wine made from Vitis vinifera L. cv. Duras. 20, 401–408. doi: 10.1111/ajgw.12084
Ghaffari, S., Reynard, J. S., and Rienth, M. (2020). Single berry sampling reveals new insights into transcriptomic reprogramming of grapevine (V. vinifera) berries caused by leafroll virus infections Sci. Rep. 10:12905. doi: 10.1038/s41598-020-69779-1
Gindro, K., Alonso-Villaverde, V., Viret, O., Spring, J.-L., Marti, G., Wolfender, J.-L., et al. (2012). “Stilbenes: biomarkers of grapevine resistance to disease of high relevance for agronomy, oenology and human health,” in Plant Defence: Biological Control, eds J. M. Mérillon, and K. G. Ramawat (Dordrecht: Springer Netherlands), 25–54. doi: 10.1007/978-94-007-1933-0_2
Gomez, C., Terrier, N., Torregrosa, L., Vialet, S., Fournier-Level, A., Verriés, C., et al. (2009). Grapevine MATE-type proteins act as vacuolar H+−dependent acylated anthocyanin transporters. Plant Physiol. 150, 402–415. doi: 10.1104/pp.109.135624
Goncalves, B., Falco, V., Moutinho-Pereira, J., Bacelar, E., Peixoto, F., and Correia, C. (2009). Effects of elevated CO2 on grapevine (Vitis vinifera L.): volatile composition, phenolic content, and in vitro antioxidant activity of red wine. J. Agric. Food Chem. 57, 265–273. doi: 10.1021/jf8020199
Gouot, J. C., Smith, J. P., Holzapfel, B. P., and Barril, C. (2019). Impact of short temperature exposure of Vitis vinifera L. cv. Shiraz grapevine bunches on berry development, primary metabolism and tannin accumulation. Environ. Exp. Bot. 168:103866. doi: 10.1016/j.envexpbot.2019.103866
Gouot, J. C., Smith, J. P., Holzapfel, B. P., Walker, A. R., and Barril, C. (2018). Grape berry flavonoids: a review of their biochemical responses to high and extreme high temperatures. J. Exp. Bot. 70, 397–423. doi: 10.1093/jxb/ery392
Gouthu, S., O,'Neil, S. T., Di, Y., Ansarolia, M., Megraw, M., and Deluc, L. G. (2014). A comparative study of ripening among berries of the grape cluster reveals an altered transcriptional programme and enhanced ripening rate in delayed berries. J. Exp. Bot. 65, 5889–902. doi: 10.1093/jxb/eru329
Greer, D. H., and Weedon, M. M. (2013). The impact of high temperatures on Vitis vinifera cv. Semillon grapevine performance and berry ripening. Front. Plant Sci. 4:491. doi: 10.3389/fpls.2013.00491
Greer, D. H., and Weston, C. (2010). Heat stress affects flowering, berry growth, sugar accumulation and photosynthesis of Vitis vinifera cv. Semillon grapevines grown in a controlled environment. Funct. Plant Biol. 37, 206–214. doi: 10.1071/FP09209
Gregan, S. M., and Jordan, B. (2016). Methoxypyrazine accumulation and O-methyltransferase gene expression in Sauvignon blanc grapes: the role of leaf removal, light exposure, and berry development. J. Agric. Food Chem. 64, 2200–2208. doi: 10.1021/acs.jafc.5b05806
Guan, L., Dai, Z., Wu, B.-H., Wu, J., Merlin, I., Hilbert, G., et al. (2016). Anthocyanin biosynthesis is differentially regulated by light in the skin and flesh of white-fleshed and teinturier grape berries. Planta 243, 23–41. doi: 10.1007/s00425-015-2391-4
Guenther, A. B., Zimmerman, P. R., Harley, P. C., Monson, R. K., and Fall, R. (1993). Isoprene and monoterpene emission rate variability: model evaluations and sensitivity analyses. J. Geophys. Res. Atmos. 98, 12609–12617. doi: 10.1029/93JD00527
Guerrero, R. F., Garcia-Parrilla, M. C., Puertas, B., and Cantos-Villar, E. (2009). Wine, resveratrol and health: a review. Nat. Prod. Commun. 4, 635–658. doi: 10.1177/1934578X0900400503
Guillaumie, S., Ilg, A., R?ty, S., Brette, M., Trossat-Magnin, C., Decroocq, S., et al. (2013). Genetic analysis of the biosynthesis of 2-methoxy-3-isobutylpyrazine, a major grape-derived aroma compound impacting wine quality. Plant Physiol. 162, 604–615. doi: 10.1104/pp.113.218313
Hale, C. S., and Buttrose, M. S. (1974). Effect of temperature on ontogeny of berries of Vitis vinifera L. cv. Cabernet Sauvignon. J. Am. Soc. Hortic. Sci. 99, 390–394.
Hannah, L., Roehrdanz, P. R., Ikegami, M., Shepard, A. V., Shaw, M. R., Tabor, G., et al. (2013). Climate change, wine, and conservation. Proc. Natl. Acad. Sci. U.S.A. 110, 6907–6912. doi: 10.1073/pnas.1210127110
Harris, S. A., Ryona, I., and Sacks, G. L. (2012). Behavior of 3-Isobutyl-2-hydroxypyrazine (IBHP), a key intermediate in 3-isobutyl-2-methoxypyrazine (IBMP) metabolism, in ripening wine grapes. J. Agric. Food Chem. 60, 11901–11908. doi: 10.1021/jf302990m
Helwi, P., Guillaumie, S., Thibon, C., Keime, C., Habran, A., Hilbert, G., et al. (2016). Vine nitrogen status and volatile thiols and their precursors from plot to transcriptome level. BMC Plant Biol. 16:173. doi: 10.1186/s12870-016-0836-y
Helwi, P., Thibon, C., Habran, A., Hilbert, G., Guillaumie, S., Delrot, S., et al. (2015). Effect of vine nitrogen status, grapevine variety and rootstock on the levels of berry S-glutathionylated and S-cysteinylated precursors of 3-sulfanylhexan-1-ol. OENO One 49, 253–265. doi: 10.20870/oeno-one.2015.49.4.40
Hichri, I., Barrieu, F., Bogs, J., Kappel, C., Delrot, S., and Lauvergeat, V. (2011). Recent advances in the transcriptional regulation of the flavonoid biosynthetic pathway. J. Exp. Bot. 62, 2465–2483. doi: 10.1093/jxb/erq442
Holl, J., Vannozzi, A., Czemmel, S., D'Onofrio, C., Walker, A. R., Rausch, T., et al. (2013). The R2R3-MYB transcription factors MYB14 and MYB15 regulate stilbene biosynthesis in Vitis vinifera. Plant Cell 25, 4135–4149. doi: 10.1105/tpc.113.117127
Houel, C., Chatbanyong, R., Doligez, A., Rienth, M., Foria, S., Luchaire, N., et al. (2015). Identification of stable QTLs for vegetative and reproductive traits in the microvine (Vitis vinifera L.) using the 18 K infinium chip. BMC Plant Biol. 15:205. doi: 10.1186/s12870-015-0588-0
Howell, K. S., Klein, M., Swiegers, J. H., Hayasaka, Y., Elsey, G. M., Fleet, G. H., et al. (2005). Genetic determinants of volatile-thiol release by Saccharomyces cerevisiae during wine fermentation. Appl. Environ. Microbiol. 71, 5420–5426. doi: 10.1128/AEM.71.9.5420-5426.2005
Huang, F. C., Molnar, P., and Schwab, W. (2009). Cloning and functional characterization of carotenoid cleavage dioxygenase 4 genes. J. Exp. Bot. 60, 3011–3022. doi: 10.1093/jxb/erp137
Huang, Y. F., Vialet, S., Guiraud, J. L., Torregrosa, L., Bertrand, Y., Cheynier, V., et al. (2014). A negative MYB regulator of proanthocyanidin accumulation, identified through expression quantitative locus mapping in the grape berry. New Phytol. 201, 795–809. doi: 10.1111/nph.12557
Ilc, T., Werck-Reichhart, D., and Navrot, N. (2016). Meta-analysis of the core aroma components of grape and wine aroma. Front. Plant Sci. 7:1472. doi: 10.3389/fpls.2016.01472
IPCC (2013). Climate Change 2013: The Physical Science Basis. Working Group I Contribution to the IPPC Fifth Assessment Report. Geneva: IPCC.
IPCC (2018). “Summary for policymakers,” in Global Warming of 1.5°C. An IPCC Special Report on the Impacts of Global Warming of 1.5°C Above Pre-industrial Levels and Related Global Greenhouse Gas Emission Pathways, in the Context of Strengthening the Global Response to the Threat of Climate Change, Sustainable Development, and Efforts to Eradicate Poverty (Geneva).
Jiang, J., Xi, H., Dai, Z., Lecourieux, F., Yuan, L., Liu, X., et al. (2019). VvWRKY8 represses stilbene synthase genes through direct interaction with VvMYB14 to control resveratrol biosynthesis in grapevine. J. Exp. Bot. 70, 715–729. doi: 10.1093/jxb/ery401
Jones, G. V., and Davis, R. E. (2000). Climate influences on grapevine phenology, grape composition, and wine production and quality for Bordeaux, France. Am. J. Enol. Vitic. 51, 249–261. Available online at: https://www.ajevonline.org/content/51/3/249
Jones, G. V., Duchêne, E., Tomasi, D., Yuste, J., Braslavska, O., Schultz, H., et al. (2005). “Changes in European winegrape phenology and relationships with climate,” in Paper presented at the XIV International GESCO Viticulture Congress (Geisenheim).
Kalua, C. M., and Boss, P. K. (2009). Evolution of volatile compounds during the development of cabernet sauvignon grapes (Vitis vinifera L.). J. Agric. Food Chem. 57, 3818–3830. doi: 10.1021/jf803471n
Keeling, C. D. (1960). The concentration and isotopic abundances of carbon dioxide in the atmosphere. Tellus 12, 200–203. doi: 10.3402/tellusa.v12i2.9366
Keller, M. (2010). Managing grapevines to optimise fruit development in a challenging environment: a climate change primer for viticulturists. Aust. J. Grape Wine Res. 16, 56–69. doi: 10.1111/j.1755-0238.2009.00077.x
Keller, M., and Torres-Martinez, N. (2004). Does UV radiation affect winegrape composition? Acta Hortic. 640, 313–319. doi: 10.17660/ActaHortic.2004.640.36
Kennedy, J. A., Saucier, C., and Glories, Y. (2006). Grape and wine phenolics: history and perspective. Am. J. Enol. Vitic. 57, 239–248. Available online at: https://www.ajevonline.org/content/57/3/239
Khater, F., Fournand, D., Vialet, S., Meudec, E., Cheynier, V., and Terrier, N. (2012). Identification and functional characterization of cDNAs coding for hydroxybenzoate/hydroxycinnamate glucosyltransferases co-expressed with genes related to proanthocyanidin biosynthesis. J. Exp. Bot. 63, 1201–1214. doi: 10.1093/jxb/err340
Kiselev, K. V., Aleynova, O. A., Grigorchuk, V. P., and Dubrovina, A. S. (2017). Stilbene accumulation and expression of stilbene biosynthesis pathway genes in wild grapevine Vitis amurensis Rupr. Planta 245, 151–159. doi: 10.1007/s00425-016-2598-z
Kizildeniz, T., Mekni, I., Santesteban, H., Pascual, I., Morales, F., and Irigoyen, J. J. (2015). Effects of climate change including elevated CO2 concentration, temperature and water deficit on growth, water status, and yield quality of grapevine (Vitis vinifera L.) cultivars. Agric. Water Manage. 159, 155–164. doi: 10.1016/j.agwat.2015.06.015
Kliewer, W. M. (1964). Influence of environment on metabolism of organic acids and carbohydrates in Vitis vinifera. I. Temperature. Plant Physiol. 6 39, 869–880. doi: 10.1104/pp.39.6.869
Kliewer, W. M. (1965). Changes in concentration of glucose, fructose, and total soluble solids in flowers and berries of Vitis vlnifera. Am. J. Enol. Vitic. 16, 101–110.
Kliewer, W. M. (1967). The glucose-fructose ratio of vitis vinifera grapes. Am. J. Enol. Vitic. 18, 33–41.
Kliewer, W. M. (1971). Effect of day temperature and light intensity on concentration of malic and tartaric acid in Vitis vinifera L. Grapes. J. Amer. Soc. Hort. Sci. 96, 372–377.
Kliewer, W. M. (1977a). Effect of high temperatures during the bloom-set period on fruit-set, ovule fertility, and berry growth of several grape cultivars. Am. J. Enol. Vitic. 28, 215–222.
Kliewer, W. M. (1977b). Influence of temperature, solar radiation and nitrogen on coloration and composition of Emperor grapes. Am. J. Enol. Vitic. 28, 96–103.
Kliewer, W. M., and Lider, L. A. (1968). Influence of cluster exposure to the sun on the composition of thompson seedless fruit. Am. J. Enol. Vitic. 19, 175–184.
Kliewer, W. M., and Lider, L. A. (1970). Effects of day temperature and light intensity on growth and composition of Vitis vinifera L. fruits. J. Amer. Soc. Hort. Sci. 95, 766–796.
Kliewer, W. M., and Torres, R. E. (1972). Effect of controlled day and night temperatures on grape coloration. Am. J. Enol. Vitic. 23, 71–77.
Kobayashi, H., Takase, H., Suzuki, Y., Tanzawa, F., Takata, R., Fujita, K., et al. (2011). Environmental stress enhances biosynthesis of flavor precursors, S-3-(hexan-1-ol)-glutathione and S-3-(hexan-1-ol)-L-cysteine, in grapevine through glutathione S-transferase activation. J. Exp. Bot. 62, 1325–1336. doi: 10.1093/jxb/erq376
Kobayashi, S., Goto-Yamamoto, N., and Hirochika, H. (2004). Retrotransposon-induced mutations in grape skin color. Science 304:982. doi: 10.1126/science.1095011
Koch, A., Doyle, C. L., Matthews, M. A., Williams, L. E., and Ebeler, S. E. (2010). 2-Methoxy-3-isobutylpyrazine in grape berries and its dependence on genotype. Phytochemistry 71, 2190–2198. doi: 10.1016/j.phytochem.2010.09.006
Koch, A., Ebeler, S. E., Williams, L. E., and Matthews, M. A. (2012). Fruit ripening in Vitis vinifera: light intensity before and not during ripening determines the concentration of 2-methoxy-3-isobutylpyrazine in Cabernet Sauvignon berries. Physiol. Plant 145, 275–285. doi: 10.1111/j.1399-3054.2012.01572.x
Koundouras, S. (2018). Environmental and viticultural effects on grape composition and wine sensory properties. Elements 14, 173–178. doi: 10.2138/gselements.14.3.173
Koundouras, S., Hatzidimitriou, E., Karamolegkou, M., Dimopoulou, E., Kallithraka, S., Tsialtas, J. T., et al. (2009). Irrigation and rootstock effects on the phenolic concentration and aroma potential of Vitis vinifera L. cv. Cabernet Sauvignon grapes. J. Agric. Food Chem. 57, 7805–7813. doi: 10.1021/jf901063a
Kovalenko, Y., Tindjau, R., Madilao, L. L., and Castellarin, S. D. (2021). Regulated deficit irrigation strategies affect the terpene accumulation in Gewürztraminer (Vitis vinifera L.) grapes grown in the Okanagan valley. Food Chem. 341:128172. doi: 10.1016/j.foodchem.2020.128172
Koyama, K., Ikeda, H., Poudel, P. R., and Goto-Yamamoto, N. (2012). Light quality affects flavonoid biosynthesis in young berries of Cabernet Sauvignon grape. Phytochemistry 78, 54–64. doi: 10.1016/j.phytochem.2012.02.026
Koyama, K., Numata, M., Nakajima, I., Goto-Yamamoto, N., Matsumura, H., and Tanaka, N. (2014). Functional characterization of a new grapevine MYB transcription factor and regulation of proanthocyanidin biosynthesis in grapes. J. Exp. Bot. 65, 4433–4449. doi: 10.1093/jxb/eru213
Kuhn, N., Guan, L., Dai, Z. W., Wu, B.-H., Lauvergeat, V., Gomès, E., et al. (2013). Berry ripening: recently heard through the grapevine. J. Exp. Bot. 65, 4543–4559. doi: 10.1093/jxb/ert395
Kwasniewski, M. T., Vanden Heuvel, J. E., Pan, B. S., and Sacks, G. L. (2010). Timing of cluster light environment manipulation during grape development affects C13 norisoprenoid and carotenoid concentrations in riesling. J. Agric. Food Chem. 58, 6841–6849. doi: 10.1021/jf904555p
Kyraleou, M., Kotseridis, Y., Koundouras, S., Chira, K., Teissedre, P.-L., and Kallithraka, S. (2016). Effect of irrigation regime on perceived astringency and proanthocyanidin composition of skins and seeds of Vitis vinifera L. cv. Syrah grapes under semiarid conditions. Food Chem. 203, 292–300. doi: 10.1016/j.foodchem.2016.02.052
Lakso, A. N., and Kliewer, W. M. (1975). The influence of temperature on malic acid metabolism in grape berries. Plant Physiol. 56, 370–372. doi: 10.1104/pp.56.3.370
Lashbrooke, J. G., Young, P. R., Dockrall, S. J., Vasanth, K., and Vivier, M. A. (2013). Functional characterisation of three members of the Vitis vinifera L. carotenoid cleavage dioxygenase gene family. BMC Plant Biol. 13:156. doi: 10.1186/1471-2229-13-156
Lecourieux, F., Kappel, C., Pieri, P., Charon, J., Pillet, J., Hilbert, G., et al. (2017). Dissecting the biochemical and transcriptomic effects of a locally applied heat treatment on developing cabernet sauvignon grape berries. Front. Plant Sci. 8:53. doi: 10.3389/fpls.2017.00053
Lei, Y., Xie, S., Guan, X., Song, C., Zhang, Z., and Meng, J. (2018). Methoxypyrazines biosynthesis and metabolism in grape: a review. Food Chem. 245, 1141–1147. doi: 10.1016/j.foodchem.2017.11.056
Leng, X., Wang, P., Wang, C., Zhu, X., Li, X., Li, H., et al. (2017). Genome-wide identification and characterization of genes involved in carotenoid metabolic in three stages of grapevine fruit development. Sci. Rep. 7:4216. doi: 10.1038/s41598-017-04004-0
Li, X.-Y., Wen, Y.-Q., Meng, N., Qian, X., and Pan, Q.-H. (2017). Monoterpenyl glycosyltransferases differentially contribute to production of monoterpenyl glycosides in two aromatic vitis vinifera varieties. Front. Plant Sci. 8:1226. doi: 10.3389/fpls.2017.01226
Lin, J., Massonnet, M., and Cantu, D. (2019). The genetic basis of grape and wine aroma. Hortic. Res. 6:81. doi: 10.1038/s41438-019-0163-1
Liu, L., Gregan, S., Winefield, C., and Jordan, B. (2015). From UVR8 to flavonol synthase: UV-B-induced gene expression in Sauvignon blanc grape berry. Plant Cell Environ. 38, 905–919. doi: 10.1111/pce.12349
Lobit, P., Michel Genard, P. S., and Habib, R. (2006). Modelling malic acid accumulation in fruits: relationships with organic acids, potassium, and temperature. J. Exp. Bot. 57, 1471–1483. doi: 10.1093/jxb/erj128
Loyola, R., Herrera, D., Mas, A., Wong, D. C., Holl, J., Cavallini, E., et al. (2016). The photomorphogenic factors UV-B RECEPTOR 1, ELONGATED HYPOCOTYL 5, and HY5 HOMOLOGUE are part of the UV-B signalling pathway in grapevine and mediate flavonol accumulation in response to the environment. J. Exp. Bot. 67, 5429–5445. doi: 10.1093/jxb/erw307
Luchaire, N., Rienth, M., Romieu, C., Nehe, A., Chatbanyong, R., Houel, C., et al. (2017). Microvine: a new model to study grapevine growth and developmental patterns and their responses to elevated temperature. Am. J. Enol. Vitic. 68, 283–292. doi: 10.5344/ajev.2017.16066
Malacarne, G., Costantini, L., Coller, E., Battilana, J., Velasco, R., Vrhovsek, U., et al. (2015). Regulation of flavonol content and composition in (Syrah × Pinot Noir) mature grapes: integration of transcriptional profiling and metabolic quantitative trait locus analyses. J. Exp. Bot. 66, 4441–4453. doi: 10.1093/jxb/erv243
Marais, J., Van Wyk, C. J., and Rapp, A. (1991). Carotenoid levels in maturing grapes as affected by climatic regions, sunlight and shade. S. Afr.J. Enol. Vitic. 12, 64–69. doi: 10.21548/12-2-2209
Marais, J., van Wyk, C. J., and Rapp, A. (1992). Effect of storage time, temperature and region on the levels of 1,l,6-Trimethyl-1,2-dihydronaphthalene and other volatiles, and on quality of weisser riesling wines. S. Afr.J. Enol. Vitic. 13, 33–44. doi: 10.21548/13-1-2197
Martin, D., Grose, C., Fedrizzi, B., Stuart, L., Albright, A., and McLachlan, A. (2016). Grape cluster microclimate influences the aroma composition of Sauvignon blanc wine. Food Chem. 210, 640–647. doi: 10.1016/j.foodchem.2016.05.010
Martin, D. M., Aubourg, S., Schouwey, M. B., Daviet, L., Schalk, M., Toub, O., et al. (2010). Functional annotation, genome organization and phylogeny of the grapevine (Vitis vinifera) terpene synthase gene family based on genome assembly, FLcDNA cloning, and enzyme assays. BMC Plant Biol. 10:226. doi: 10.1186/1471-2229-10-226
Martinez-Luscher, J., Morales, F., Sanchez-Diaz, M., Delrot, S., Aguirreolea, J., Gomes, E., et al. (2015). Climate change conditions (elevated CO2 and temperature) and UV-B radiation affect grapevine (Vitis vinifera cv. Tempranillo) leaf carbon assimilation, altering fruit ripening rates. Plant Sci. 236, 168–176. doi: 10.1016/j.plantsci.2015.04.001
Martínez-Lüscher, J., Torres, N., Hilbert, G., Richard, T., Sánchez-Díaz, M., Delrot, S., et al. (2014). Ultraviolet-B radiation modifies the quantitative and qualitative profile of flavonoids and amino acids in grape berries. Phytochemistry 102, 106–114. doi: 10.1016/j.phytochem.2014.03.014
Massonnet, M., Fasoli, M., Tornielli, G. B., Altieri, M., Sandri, M., Zuccolotto, P., et al. (2017). Ripening transcriptomic program in red and white grapevine varieties correlates with berry skin anthocyanin accumulation. Plant Physiol. 174, 2376–2396. doi: 10.1104/pp.17.00311
Matarese, F., Cuzzola, A., Scalabrelli, G., and D'Onofrio, C. (2014). Expression of terpene synthase genes associated with the formation of volatiles in different organs of Vitis vinifera. Phytochemistry 105, 12–24. doi: 10.1016/j.phytochem.2014.06.007
Matarese, F., Scalabrelli, G., and D'Onofrio, C. (2013). Analysis of the expression of terpene synthase genes in relation to aroma content in two aromatic Vitis vinifera varieties. Funct. Plant Biol. 40, 552–565. doi: 10.1071/FP12326
Mathieu, S., Terrier, N., Procureur, J., Bigey, F., and Guenata, Z. (2005). A carotenoid cleavage dioxygenase from Vitis vinifera L.: functional characterization and expression during grape berry development in relation to C13-norisoprenoid accumulation. J. Exp. Bot. 56, 2721–2731. doi: 10.1093/jxb/eri265
Matsui, S., Ryugo, K., and Kliewer, W. M. (1986). Growth inhibition of thompson seedless and napa gamay berries by heat stress and its partial reversibility by applications of growth regulators. Am. J. Enol. Vitic. 37, 67–71.
Matsuyama, S., Tanzawa, F., Kobayashi, H., Suzuki, S., Takata, R., and Saito, H. (2014). Leaf removal accelerated accumulation of delphinidin-based anthocyanins in “Muscat Bailey A” [Vitis × labruscana (Bailey) and Vitis vinifera (Muscat Hamburg)] grape skin. J. Jap. Soc. Hortic. Sci. 83, 17–22. doi: 10.2503/jjshs1.CH-062
Mattivi, F., Vrhovsek, U., Masuero, D., and Trainotti, D. (2009). Differences in the amount and structure of extractable skin and seed tannins amongst red grape varieties. Aust. J. Grape Wine Res. 15, 27–35. doi: 10.1111/j.1755-0238.2008.00027.x
Matus, J. T., Cavallini, E., Loyola, R., Holl, J., Finezzo, L., Dal Santo, S., et al. (2017). A group of grapevine MYBA transcription factors located in chromosome 14 control anthocyanin synthesis in vegetative organs with different specificities compared with the berry color locus. Plant J. 91, 220–236. doi: 10.1111/tpj.13558
Matus, J. T., Loyola, R., Vega, A., Peña-Neira, A., Bordeu, E., Arce-Johnson, P., et al. (2009). Post-veraison sunlight exposure induces MYB-mediated transcriptional regulation of anthocyanin and flavonol synthesis in berry skins of Vitis vinifera. J. Exp. Bot, 60, 853–867. doi: 10.1093/jxb/ern336
Mendes-Pinto, M. M. (2009). Carotenoid breakdown products the—norisoprenoids—in wine aroma. Arch. Biochem. Biophys. 483, 236–245. doi: 10.1016/j.abb.2009.01.008
Mira de Orduna, R. (2010). Climate change associated effects on grape and wine quality and production. Food Res. Int. 43, 1844–1855. doi: 10.1016/j.foodres.2010.05.001
Monagas, M., Núñez, V., Bartolomé, B., and Gómez-Cordovés, C. (2003). Anthocyanin-derived pigments in Graciano, Tempranillo, and Cabernet Sauvignon wines produced in Spain. Am. J. Enol. Vitic. 54, 163–169. Available online at: https://www.ajevonline.org/content/54/3/163
Morales-Castilla, I., García de Cortázar-Atauri, I., Cook, B. I., Lacombe, T., Parker, A., van Leeuwen, C., et al. (2020). Diversity buffers winegrowing regions from climate change losses. Proc. Natl. Acad. Sci. U.S.A. 117, 2864–2869. doi: 10.1073/pnas.1906731117
Mori, K., Goto-Yamamoto, N., Kitayama, M., and Hashizume, K. (2007). Loss of anthocyanins in red-wine grape under high temperature. J. Exp. Bot. 58, 1935–1945. doi: 10.1093/jxb/erm055
Mori, K., Sugaya, S., and Gemma, H. (2005). Decreased anthocyanin biosynthesis in grape berries grown under elevated night temperature condition. Sci. Hortic. 105, 319–330. doi: 10.1016/j.scienta.2005.01.032
Moscatello, S., Frioni, T., Blasi, F., Proietti, S., Pollini, L., Verducci, G., et al. (2019). Changes in absolute contents of compounds affecting the taste and nutritional properties of the flesh of three plum species throughout development. Foods 8:486. doi: 10.3390/foods8100486
Moutinho-Pereirea, J., Goncalves, B., Bacelar, E., Boaventura Cunha, J., Coutinho, J. C., and Correira, M. C. (2009). Effects of elevated CO2 on grapevine (Vitis vinifera L.): physiological and yield attributes. Vitis 48, 159–165. doi: 10.5073/vitis.2009.48.159-165
Movahed, N., Pastore, C., Cellini, A., Allegro, G., Valentini, G., Zenoni, S., et al. (2016). The grapevine VviPrx31 peroxidase as a candidate gene involved in anthocyanin degradation in ripening berries under high temperature. J. Plant Res. 129, 513–526. doi: 10.1007/s10265-016-0786-3
Muñoz, C., Fanzone, M., and Lijavetzky, D. (2019). Transcriptional regulation of the anthocyanin biosynthesis pathway in developing grapevine berries in cultivar “Malbec” by putative R2R3 MYB negative regulators. Sci. Hortic. 257:108663. doi: 10.1016/j.scienta.2019.108663
Niculcea, M., López, J., Sánchez-Díaz, M., and Carmen Antolín, M. (2014). Involvement of berry hormonal content in the response to pre- and post-veraison water deficit in different grapevine (Vitis vinifera L.) cultivars. Aust. J. Grape Wine Res. 20, 281–291. doi: 10.1111/ajgw.12064
Ojeda, H., Andary, C., Kraeva, E., Carbonneau, A., and Deloire, A. (2002). Influence of pre- and postveraison water deficit on synthesis and concentration of skin phenolic compounds during berry growth of Vitis vinifera cv. Shiraz. Am. J. Enol. Viticult. 53, 261–267. Available online at: https://www.ajevonline.org/content/53/4/261.1
Ojeda, H., Deloire, A., and Carbonneau, A. (2001). Influence of water deficits on grape berry growth. Vitis 40, 141–145. doi: 10.5073/vitis.2001.40.141-145
Oliveira, C., Ferreira, A. C., Costa, P., Guerra, J., and Guedes de Pinho, P. (2004). Effect of some viticultural parameters on the grape carotenoid profile. J. Agric. Food Chem. 52, 4178–4184. doi: 10.1021/jf0498766
Ollat, N., Peccoux, A., Papura, D., Esmenjaud, D., Marguerit, E., Tandonnet, J., et al. (2016). “Rootstocks as a component of adaptation to environment,” in Grapevine in a Changing Environment, eds M. M. C. H. Gerós, H. M. Gil, and S. Delrot (John Wiley & Sons) 68–108. doi: 10.1002/9781118735985.ch4
Ollé, D., Guiraud, J. L., Souquet, J. M., Terrier, N., Ageorges, A., Cheynier, V., et al. (2011). Effect of pre- and post-veraison water deficit on proanthocyanidin and anthocyanin accumulation during Shiraz berry development. Aust J Grape Wine Res. 17, 90–100. doi: 10.1111/j.1755-0238.2010.00121.x
Ono, E., Homma, Y., Horikawa, M., Kunikane-Doi, S., Imai, H., Takahashi, S., et al. (2010). Functional differentiation of the glycosyltransferases that contribute to the chemical diversity of bioactive flavonol glycosides in grapevines (Vitis vinifera). Plant Cell 22, 2856–2871. doi: 10.1105/tpc.110.074625
Parker, M., Capone, D. L., Francis, I. L., and Herderich, M. J. (2018). Aroma precursors in grapes and wine: flavor release during wine production and consumption. J. Agric. Food Chem. 66, 2281–2286. doi: 10.1021/acs.jafc.6b05255
Pastor, R. F., Restani, P., Di Lorenzo, C., Orgiu, F., Teissedre, P. L., Stockley, C., et al. (2019). Resveratrol, human health and winemaking perspectives. Crit. Rev. Food Sci. Nutr. 59, 1237–1255. doi: 10.1080/10408398.2017.1400517
Pastore, C., Dal Santo, S., Zenoni, S., Movahed, N., Allegro, G., Valentini, G., et al. (2017). Whole plant temperature manipulation affects flavonoid metabolism and the transcriptome of grapevine berries. Front. Plant Sci. 8:929. doi: 10.3389/fpls.2017.00929
Pellegrino, A., Romieu, C., Rienth, M., and Torregrosa, L. (2019). “The microvine: a versatile plant model to boost grapevine studies in physiology and genetics,” in Advances in Grape and Wine Biotechnology (IntechOpen), 1–23. doi: 10.5772/intechopen.86166
Pérez-Díaz, R., Madrid-Espinoza, J., Salinas-Cornejo, J., González-Villanueva, E., and Ruiz-Lara, S. (2016). Differential roles for VviGST1, VviGST3, and VviGST4 in proanthocyanidin and anthocyanin transport in Vitis vinífera. Front. Plant Sci. 7:1166. doi: 10.3389/fpls.2016.01166
Perez-Diaz, R., Ryngajllo, M., Perez-Diaz, J., Pena-Cortes, H., Casaretto, J. A., Gonzalez-Villanueva, E., et al. (2014). VvMATE1 and VvMATE2 encode putative proanthocyanidin transporters expressed during berry development in Vitis vinifera L. Plant Cell Rep., 33, 1147–1159. doi: 10.1007/s00299-014-1604-9
Petrie, P. R., and Sadras, V. O. (2008). Advancement of grapevine maturity in Australia between 1993 and 2006: putative causes, magnitude of trends and viticultural consequences. Aust. J. Grape Wine Res. 14, 33–45. doi: 10.1111/j.1755-0238.2008.00005.x
Picard, M., van Leeuwen, C., Guyon, F., Gaillard, L., de Revel, G., and Marchand, S. (2017). Vine water deficit impacts aging bouquet in fine red bordeaux wine. Front. Chem. 5:56. doi: 10.3389/fchem.2017.00056
Pichersky, E., and Raguso, R. A. (2018). Why do plants produce so many terpenoid compounds? New Phytol. 220, 692–702. doi: 10.1111/nph.14178
Pillet, J., Egert, A., Pieri, P., Lecourieux, F., Kappel, C., Charon, J., et al. (2012). VvGOLS1 and VvHsfA2 are involved in the heat stress responses in grapevine berries. Plant Cell Physiol. 53, 1776–1792. doi: 10.1093/pcp/pcs121
Podolyan, A., White, J., Jordan, B., and Winefield, C. (2010). Identification of the lipoxygenase gene family from Vitis vinifera and biochemical characterisation of two 13-lipoxygenases expressed in grape berries of Sauvignon Blanc. Func. Plant Biol. 37, 767–784. doi: 10.1071/FP09271
Poitou, X., Achaintre, V.-C., Redon, P., Thibon, C., and Darriet, P. (2017a). Caractérisation de marqueurs volatils associés aux nuances végétales des vins rouges. Etude du lien avec la maturité et le pressurage. Partie 1/3 : Approche sensorielle et analytique de la famille aromatique du “végétal” dans les vins. Rev. Œnol. 164, 49–52.
Poitou, X., Thibon, C., and Darriet, P. (2017b). 1,8-cineole in french red wines: evidence for a contribution related to Its various origins. J. Agric. Food Chem. 65, 383–393. doi: 10.1021/acs.jafc.6b03042
Poni, S., Gatti, M., Palliotti, A., Dai, Z., Duchêne, E., Truong, T.-T., et al. (2018). Grapevine quality: a multiple choice issue. Sci. Hortic. 234, 445–462. doi: 10.1016/j.scienta.2017.12.035
Pons, A., Allamy, L., Lavigne, V., Dubourdieu, D., and Darriet, P. (2017a). Study of the contribution of massoia lactone to the aroma of Merlot and Cabernet Sauvignon musts and wines. Food Chem. 232, 229–236. doi: 10.1016/j.foodchem.2017.03.151
Pons, A., Allamy, L., Schüttler, A., Rauhut, D. C. T., et al. (2017b). What is the expected impact of climate change on wine aroma compounds and their precursors in grape? OENO One 51, 141–146. doi: 10.20870/oeno-one.2016.0.0.1868
Possner, D., Ruffner, H. P., and Rast, D. M. (1983). Regulation of malic acid metabolism in berries of Vitis vinifera. Acta Hort. 139, 117–122. doi: 10.17660/ActaHortic.1983.139.16
Pozo-Bayon, M. A., Hernandez, M. T., Martin-Alvarez, P. J., and Polo, M. C. (2003). Study of low molecular weight phenolic compounds during the aging of sparkling wines manufactured with red and white grape varieties. J. Agric. Food Chem. 51, 2089–2095. doi: 10.1021/jf021017z
Ramírez-Garza, S. L., Laveriano-Santos, E. P., Marhuenda-Muñoz, M., Storniolo, C. E., Tresserra-Rimbau, A., Vallverdú-Queralt, A., et al. (2018). Health effects of resveratrol: results from human intervention trials. Nutrients 10:1892. doi: 10.3390/nu10121892
Razungles, A., Guuenata, Z., Pinatel, S., Baumes, R. L., and Bayonove, C. L. (1993). Etude quantitative de compose's terpeniques, norisoprepnoiques et de leurs precurseurs dans diverses varietes de raisin. Sci. Aliments 13, 59–72.
Renaud, S., and de Lorgeril, M. (1992). Wine, alcohol, platelets, and the French paradox for coronary heart disease. Lancet 339, 1523–1526. doi: 10.1016/0140-6736(92)91277-F
Reshef, N., Agam, N., and Fait, A. (2018). Grape berry acclimation to excessive solar irradiance leads to repartitioning between major flavonoid groups. J. Agric. Food Chem. 66, 3624–3636. doi: 10.1021/acs.jafc.7b04881
Reshef, N., Walbaum, N., Agam, N., and Fait, A. (2017). Sunlight modulates fruit metabolic profile and shapes the spatial pattern of compound accumulation within the grape cluster. Front. Plant Sci. 8:70. doi: 10.3389/fpls.2017.00070
Rienth, M., Crovadore, M., Ghaffari, S., and Lefort, F. (2019). Oregano essential oil vapour prevents Plasmopora viticola infection in grapevine (Vitis vinifera) by triggering autoimmune metabolic pathway. PLoS ONE 14:e0222854. doi: 10.1371/journal.pone.0222854
Rienth, M., Dauzat, M., Pellegrino, A., Lopez, G., Torregrosa, L., and Romieu, C. (2012). First observations of the microvine development under 100% LED (light emitting diodes) illumination. Vitis 51, 167–173. doi: 10.5073/vitis.2012.51.167-173
Rienth, M., Grimplet, J., Chatbanyong, R., Torregrosa, L., Romieu, C., and Ageorges, A. (2017). Transcriptional response to temperature of ripening microvine (DRCF) depends on daytime. Acta Hortic. 238–321. doi: 10.17660/ActaHortic.2017.1157.45
Rienth, M., Lamy, F., Schoenenberger, P., Noll, D., Lorenzini, F., Viret, O., et al. (2020). A vine physiology-based terroir study in the AOC-Lavaux region in Switzerland: This article is published in cooperation with the XIIIth International Terroir Congress November 17-18 2020, Adelaide, Australia. OENO One 54, 863–880. doi: 10.20870/oeno-one.2020.54.4.3756
Rienth, M., and Scholasch, T. (2019). State of the art of tools and methods to asses vine water status. OENO One 53, 619–637. doi: 10.20870/oeno-one.2019.53.4.2403
Rienth, M., Torregrosa, L., Kelly, M. T., Luchaire, N., Pellegrino, A., Grimplet, J., et al. (2014a). Is transcriptomic regulation of berry development more important at night than during the day? PLoS ONE 9:e88844. doi: 10.1371/journal.pone.0088844
Rienth, M., Torregrosa, L., Luchaire, N., Chatbanyong, R., Lecourieux, D., Kelly, M., et al. (2014b). Day and night heat stress trigger different transcriptomic responses in green and ripening grapevine (Vitis vinifera) fruit. BMC Plant Biol. 14:108. doi: 10.1186/1471-2229-14-108
Rienth, M., Torregrosa, L., Sarah, G., Ardisson, M., Brillouet, J.-M., and Romieu, C. (2016). Temperature desynchronizes sugar and organic acid metabolism in ripening grapevine fruits and remodels their transcriptome. BMC Plant Biol. 16:164. doi: 10.1186/s12870-016-0850-0
Rinaldo, A. R., Cavallini, E., Jia, Y., Moss, S. M. A., McDavid, D. A. J., Hooper, L. C., et al. (2015). a grapevine anthocyanin acyltransferase, transcriptionally regulated by vvmyba, can produce most acylated anthocyanins present in grape skins. Plant Physiol. 169, 1897–1916. doi: 10.1104/pp.15.01255
Rodríguez-Concepción, M., Ahumada, I., Diez-Juez, E., Sauret-Gueto, S., Lois, L. M., Gallego, F., et al. (2001). 1-Deoxy-D-xylulose 5-phosphate reductoisomerase and plastid isoprenoid biosynthesis during tomato fruit ripening. Plant J. 27, 213–222. doi: 10.1046/j.1365-313x.2001.01089.x
Roland, A., Schneider, R., Charrier, F., Cavelier, F., Rossignol, M., and Razungles, A. (2011). Distribution of varietal thiol precursors in the skin and the pulp of Melon B. and Sauvignon Blanc grapes. Food Chem. 125, 139–144. doi: 10.1016/j.foodchem.2010.08.050
Roncoroni, M., Santiago, M., Hooks, D. O., Moroney, S., Harsch, M. J., Lee, S. A., et al. (2011). The yeast IRC7 gene encodes a β-lyase responsible for production of the varietal thiol 4-mercapto-4-methylpentan-2-one in wine. Food Microbiol. 28, 926–935. doi: 10.1016/j.fm.2011.01.002
Rösti, J., Schumann, M., Cleroux, M., Lorenzini, F., Zufferey, V., and Rienth, M. (2018). Effect of drying on tartaric acid and malic acid in Shiraz and Merlot berries. Aust. J. Grape Wine Res. 24, 421–429. doi: 10.1111/ajgw.12344
Roujou de Boubee, D., Van Leeuwen, C., and Dubourdieu, D. (2000). Organoleptic impact of 2-methoxy-3-isobutylpyrazine on red bordeaux and loire wines. Effect of environmental conditions on concentrations in grapes during ripening. J. Agric. Food Chem. 48, 4830–4834. doi: 10.1021/jf000181o
Roujou-de-Boubée, D., and Botella, T. (2003). Research on 2-methoxy-3-isobutylpyrazine in grapes and wines, 1–32.
Ruffner, H. P., and Hawker, J. S. (1977). Control of glycolysis in ripening berries of Vitis vinifera. Phytochemistry 16, 1171–1175. doi: 10.1016/S0031-9422(00)94354-1
Ruffner, H. P., Hawker, J. S., and Hale, C. R. (1976). Temperature and enzymic control of malate metabolism in berries of Vitis vinifera. Phytochemistry 15, 1877–1880. doi: 10.1016/S0031-9422(00)88835-4
Ryona, I., Leclerc, S., and Sacks, G. L. (2010). Correlation of 3-isobutyl-2-methoxypyrazine to 3-isobutyl-2-hydroxypyrazine during maturation of bell pepper (Capsicum annuum) and wine grapes (Vitis vinifera). J. Agric. Food Chem. 58, 9723–9730. doi: 10.1021/jf102072w
Ryona, I., Pan, B. S., Intrigliolo, D. S., Lakso, A. N., and Sacks, G. L. (2008). Effects of cluster light exposure on 3-isobutyl-2-methoxypyrazine accumulation and degradation patterns in red wine grapes (Vitis vinifera L. Cv. Cabernet Franc). J. Agric. Food Chem. 56, 10838–10846. doi: 10.1021/jf801877y
Sadras, V. O., and Moran, M. A. (2012). Elevated temperature decouples anthocyanins and sugars in berries of Shiraz and Cabernet Franc. Aust. J. Grape Wine Res. 18, 115–122. doi: 10.1111/j.1755-0238.2012.00180.x
Sadras, V. O., Moran, M. A., and Bonada, M. (2013). Effects of elevated temperature in grapevine. I berry sensory traits. Aust. J. Grape Wine Res. 19, 95–106. doi: 10.1111/ajgw.12007
Sala, C., Busto, O., Guasch, J., and Zamora, F. (2004). Influence of vine training and sunlight exposure on the 3-alkyl-2-methoxypyrazines content in musts and wines from the Vitis vinifera variety cabernet sauvignon. J. Agric. Food Chem. 52, 3492–3497. doi: 10.1021/jf049927z
Salazar-Parra, C., Aguirreolea, J., Sanchez-Diaz, M., Irigoyen, J. J., and Morales, F. (2012). Climate change (elevated CO(2), elevated temperature and moderate drought) triggers the antioxidant enzymes' response of grapevine cv. Tempranillo, avoiding oxidative damage. Physiol Plant 144, 99–110. doi: 10.1111/j.1399-3054.2011.01524.x
Salehi, B., Mishra, A. P., Nigam, M., Sener, B., Kilic, M., Sharifi-Rad, M., et al. (2018). Resveratrol: a double-edged sword in health benefits. Biomedicines 6:91. doi: 10.3390/biomedicines6030091
Santesteban, L. G., Miranda, C., and Royo, J. B. (2011). Regulated deficit irrigation effects on growth, yield, grape quality and individual anthocyanin composition in Vitis vinifera L. cv. “Tempranillo.” Agric. Water Manage. 98, 1171–1179. doi: 10.1016/j.agwat.2011.02.011
Santiago, M., and Gardner, R. C. (2015). Yeast genes required for conversion of grape precursors to varietal thiols in wine. FEMS Yeast Res. 15:fov034. doi: 10.1093/femsyr/fov034
Santos-Sánchez, F., Salas-Coronado, R., Hernández-Carlos, B., and Villanueva-Cañongo, C. (2019). “Shikimic acid pathway in biosynthesis of phenolic compounds,” in Plant Physiological Aspects of Phenolic Compounds, ed M. Soto-Hernández (Intechopen) 1–15. doi: 10.5772/intechopen.83815
Sasaki, K., Takase, H., Matsuyama, S., Kobayashi, H., Matsuo, H., Ikoma, G., et al. (2016). Effect of light exposure on linalool biosynthesis and accumulation in grape berries. Biosci. Biotechnol. Biochem. 80, 2376–2382. doi: 10.1080/09168451.2016.1217148
Savoi, S., Wong, D. C. J., Arapitsas, P., Miculan, M., Bucchetti, B., Peterlunger, E., et al. (2016). Transcriptome and metabolite profiling reveals that prolonged drought modulates the phenylpropanoid and terpenoid pathway in white grapes (Vitis vinifera L.). BMC Plant Biol. 16:67. doi: 10.1186/s12870-016-0760-1
Savoi, S., Wong, D. C. J., Degu, A., Herrera, J. C., Bucchetti, B., Peterlunger, E., et al. (2017). Multi-omics and integrated network analyses reveal new insights into the systems relationships between metabolites, structural genes, and transcriptional regulators in developing grape berries (Vitis vinifera L.) exposed to water deficit. Front. Plant Sci. 8:1124. doi: 10.3389/fpls.2017.01124
Schnee, S., Viret, O., and Gindro, K. (2008). Role of stilbenes in the resistance of grapevine to powdery mildew. Physiol. Mol. Plant Pathol. 72, 128–133. doi: 10.1016/j.pmpp.2008.07.002
Scholasch, T., and Rienth, M. (2019). Review of water deficit mediated changes in vine and berry physiology; Consequences for the optimization of irrigation strategies. OENO One 53, 619–637. doi: 10.20870/oeno-one.2019.53.3.2407
Schreiner, M., Mewis, I., Huyskens-Keil, S., Jansen, M. A. K., Zrenner, R., Winkler, J. B., et al. (2012). UV-B-induced secondary plant metabolites - potential benefits for plant and human health. CRC. Crit. Rev. Plant Sci. 31, 229–240. doi: 10.1080/07352689.2012.664979
Schultz, H. R. (2000). Climate change and viticulture: a European perspective on climatology, carbon dioxide and UV-B effects. Aust. J. Grape Wine Res. 6, 2–12. doi: 10.1111/j.1755-0238.2000.tb00156.x
Schultz, H. R. (2016). Global climate change, sustainability, and some challenges for grape and wine production. J. Wine Econ. 11, 181–200. doi: 10.1017/jwe.2015.31
Schüttler, A., Guthier, C., Stoll, M., Darriet, P., and Rauhut, D. (2015). “Impact of grape cluster defoliation on TDN potential in cool climate Riesling wines,” in Paper presented at the 38th World Congress of Vine and Wine (Mainz). doi: 10.1051/bioconf/20150501006
Schwab, W., and Wüst, M. (2015). Understanding the constitutive and induced biosynthesis of mono- and sesquiterpenes in grapes (Vitis vinifera): a key to unlocking the biochemical secrets of unique grape aroma profiles. J. Agric. Food Chem. 63, 10591–10603. doi: 10.1021/acs.jafc.5b04398
Selmar, D., and Kleinwächter, M. (2013). Stress enhances the synthesis of secondary plant products: the impact of stress-related over-reduction on the accumulation of natural products. Plant Cell Physiol. 54, 817–826. doi: 10.1093/pcp/pct054
Sepulveda, G., and Kliewer, W. M. (1986). Effect of high temperature on grapevines (Vitis vinifera L.). II. Distribution of soluble sugars. Am. J. Enol. Viticult. 37, 20–25.
Shahood, R., Rienth, M., Torregrossa, L., and Romieu, C. (2015). “Evolution of grapevine (Vitis vinifera L.) berry heterogeneity during ripening,” in Paper Presented at the 19. Journées Internationales de Viticulture GiESCO Gruissan (France).
Shahood, R., Torregrosa, L., Savoi, S., and Romieu, C. (2020). First quantitative assessment of growth, sugar accumulation and malate breakdown in a single ripening berry. OENO One 54, 1077–1092. doi: 10.20870/oeno-one.2020.54.4.3787
Shinomiya, R., Fujishima, H., Muramoto, K., and Shiraishi, M. (2015). Impact of temperature and sunlight on the skin coloration of the “Kyoho” table grape. Sci. Hortic. 193, 77–83. doi: 10.1016/j.scienta.2015.06.042
Sidhu, D., Lund, J., Kotseridis, Y., and Saucier, C. (2015). Methoxypyrazine analysis and influence of viticultural and enological procedures on their levels in grapes, musts, and wines. Crit. Rev. Food Sci. Nutr. 55, 485–502. doi: 10.1080/10408398.2012.658587
Siebert, T. E., Barter, S. R., de Barros Lopes, M. A., Herderich, M. J., and Francis, I. L. (2018). Investigation of 'stone fruit' aroma in Chardonnay, Viognier and botrytis Semillon wines. Food Chem. 256, 286–296. doi: 10.1016/j.foodchem.2018.02.115
Sivilotti, P., Falchi, R., Herrera, J. C., Skvarc, B., Butinar, L., Sternad Lemut, M., et al. (2017). Combined effects of early season leaf removal and climatic conditions on aroma precursors in Sauvignon Blanc grapes. J. Agric. Food Chem. 65, 8426–8434. doi: 10.1021/acs.jafc.7b03508
Song, J., Shellie, K. C., Wang, H., and Qian, M. C. (2012). Influence of deficit irrigation and kaolin particle film on grape composition and volatile compounds in merlot grape (Vitis vinifera L.). Food Chem. 134, 841–850. doi: 10.1016/j.foodchem.2012.02.193
Song, J., Smart, R., Wang, H., Dambergs, B., Sparrow, A., and Qian, M. C. (2015). Effect of grape bunch sunlight exposure and UV radiation on phenolics and volatile composition of Vitis vinifera L. cv. Pinot noir wine. Food Chem. 173, 424–431. doi: 10.1016/j.foodchem.2014.09.150
Spayd, S. E., Tarara, J. M., Mee, D. L., and Ferguson, J. C. (2002). Separation of sunlight and temperature effects on the composition of Vitis vinifera cv. Merlot Berries. Am. J. Enologu Viticult. 53, 171–182. Available online at: https://www.ajevonline.org/content/53/3/171
Strauss, C. R., Wilson, B., Gooley, P. R., and Williams, P. J. (1986). Role of monoterpenes in grape and wine flavor. ACS Symp. Series 317, 222–242. doi: 10.1021/bk-1986-0317.ch018
Stummer, B., Francis, I., Zanker, T., Lattey, K., and Scott, E. (2005). Effects of powdery mildew on the sensory properties and composition of Chardonnay juice and wine when grape sugar ripeness is standardised. Aust. J. Grape Wine Res. 11, 66–76. doi: 10.1111/j.1755-0238.2005.tb00280.x
Šuklje, K., Antalick, G., Coetzee, Z., Schmidtke, L. M., Baša Cesnik, H., Brandt, J., et al. (2014). Effect of leaf removal and ultraviolet radiation on the composition and sensory perception of Vitis vinifera L. cv. Sauvignon Blanc wine. Aust. J. Grape Wine Res. 20, 223–233. doi: 10.1111/ajgw.12083
Sun, R. Z., Cheng, G., Li, Q., He, Y. N., Wang, Y., Lan, Y. B., et al. (2017). Light-induced variation in phenolic compounds in cabernet sauvignon grapes (Vitis vinifera L.) involves extensive transcriptome reprogramming of biosynthetic enzymes, transcription factors, and phytohormonal regulators. Front. Plant Sci. 8:547. doi: 10.3389/fpls.2017.00547
Sunitha, S., Loyola, R., Alcalde, J. A., Arce-Johnson, P., Matus, J. T., and Rock, C. D. (2019). The role of UV-B light on small RNA activity during grapevine berry development. G3 9, 769–787. doi: 10.1534/g3.118.200805
Sweetman, C., Deluc, L. G., Cramer, G. R., Ford, C. M., and Soole, K. L. (2009). Regulation of malate metabolism in grape berry and other developing fruits. Phytochemistry 70, 1329–1344. doi: 10.1016/j.phytochem.2009.08.006
Sweetman, C., Sadras, V. O., Hancock, R. D., Soole, K. L., and Ford, C. M. (2014). Metabolic effects of elevated temperature on organic acid degradation in ripening Vitis vinifera fruit. J. Exp. Bot. 65, 5975–5988. doi: 10.1093/jxb/eru343
Takase, H., Sasaki, K., Shinmori, H., Shinohara, A., Mochizuki, C., Kobayashi, H., et al. (2015). Cytochrome P450 CYP71BE5 in grapevine (Vitis vinifera) catalyzes the formation of the spicy aroma compound (–)-rotundone. J. Exp. Bot. 67, 787–798. doi: 10.1093/jxb/erv496
Talaverano, I., Valdes, E., Moreno, D., Gamero, E., Mancha, L., and Vilanova, M. (2017). The combined effect of water status and crop level on tempranillo wine volatiles. J. Sci. Food Agric. 97, 1533–1542. doi: 10.1002/jsfa.7898
Tarara, J. M., Lee, J., Spayd, S. E., and Scagel, C. F. (2008). Berry temperature and solar radiation alter acylation, proportion, and concentration of anthocyanin in merlot grapes. Am. J. Enol. Vitic. 59, 235–247. Available online at: https://www.ajevonline.org/content/59/3/235
Teixeira, A., Eiras-Dias, J., Castellarin, S., and Geros, H. (2013). Berry phenolics of grapevine under challenging environments. Int. J. Mol. Sci. 14, 18711–18739. doi: 10.3390/ijms140918711
Terrier, N., Torregrosa, L., Ageorges, A., Vialet, S., Verries, C., Cheynier, V., et al. (2009). Ectopic expression of VvMybPA2 promotes proanthocyanidin biosynthesis in Vitis vinifera L. and suggests additional targets in the pathway. Plant Physiol. 149, 1028–1041. doi: 10.1104/pp.108.131862
Theodorou, N., Nikolaou, N., Zioziou, E., Kyraleou, M., Stamatina, K., Kotseridis, Y., et al. (2019). Anthocyanin content and composition in four red winegrape cultivars (Vitis vinifera L.) under variable irrigation. OENO One 53, 39–51. doi: 10.20870/oeno-one.2019.53.1.2366
Tominaga, T., Baltenweck-Guyot, R., Gachons, C. P. D., and Dubourdieu, D. (2000). Contribution of volatile thiols to the aromas of white wines made from several Vitis vinifera grape varieties. 51, 178–181. Available online at: https://www.ajevonline.org/content/51/2/178
Tominaga, T., Darriet, P., and Dubourdieu, D. (1996). Identification of 3-mercaptohexyl acetate in Sauvignon wine, a powerful aromatic compound exhibiting box-tree odor. Vitis 35, 207–210.
Tominaga, T., Furrer, A., Henry, R., and Dubourdieu, D. (1998). Identification of new volatile thiols in the aroma of Vitis vinifera L. var. Sauvignon blanc wines. Flav. Frag. J. 13, 159–162. doi: 10.1002/(SICI)1099-1026(199805/06)13:3<159::AID-FFJ709>3.0.CO;2-7
Torregrosa, L., Bigard, A., Doligez, A., Lecourieux, D., Rienth, M., Luchaire, N., et al. (2017). Developmental, molecular and genetic studies on grapevine response to temperature open breeding strategies for adaptation to warming. OENO One 51, 155–165. doi: 10.20870/oeno-one.2017.51.2.1587
Torregrosa, L., Rienth, M., Romieu, C., and Pellegrino, A. (2019). The microvine, a model for studies in grapevine physiology and genetics. OENO One 3, 373–391. doi: 10.20870/oeno-one.2019.53.3.2409
Torres, N., Hilbert, G., Luquin, J., Goicoechea, N., and Antolín, M. C. (2017). Flavonoid and amino acid profiling on Vitis vinifera L. cv tempranillo subjected to deficit irrigation under elevated temperatures. J. Food Compos. Anal. 62, 51–62. doi: 10.1016/j.jfca.2017.05.001
Torres, N., Martínez-Lüscher, J., Porte, E., and Kurtural, S. K. (2020a). Optimal ranges and thresholds of grape berry solar radiation for flavonoid biosynthesis in warm climates. Front. Plant Sci. 11:931. doi: 10.3389/fpls.2020.00931
Torres, N., Martínez-Lüscher, J., Porte, E., Yu, R., and Kaan Kurtural, S. (2020b). Impacts of leaf removal and shoot thinning on cumulative daily light intensity and thermal time and their cascading effects of grapevine (Vitis vinifera L.) berry and wine chemistry in warm climates. Food Chem. 343:128447. doi: 10.1016/j.foodchem,.2020.128447
United Nations Environment Programme Environmental Effects Assessment Panel. (2017). Environmental effects of ozone depletion and its interactions with climate change: progress report, 2016. Photochem. Photobiol. Sci. 16, 107–145. doi: 10.1039/C7PP90001E
Vaknin, H., Bar-Akiva, A., Ovadia, R., Nissim-Levi, A., Forer, I., Weiss, D., et al. (2005). Active anthocyanin degradation in Brunfelsia calycina (yesterday–today–tomorrow) flowers. Planta 222, 19–26. doi: 10.1007/s00425-005-1509-5
van Leeuwen, C., Barbe, J.-C., Darriet, P., Geffroy, O., Gomès, E., Guillaumie, S., et al. (2020). Recent advancements in understanding the terroir effect on aromas in grapes and wines: this article is published in cooperation with the XIIIth international terroir congress November 17-18 2020, Adelaide, Australia. OENO One 54, 985–1006. doi: 10.20870/oeno-one.2020.54.4.3983
van Leeuwen, C., and Destrac-Irvine, A. (2017). Modified grape composition under climate change conditions requires adaptations in the vineyard. OENO One 51, 147–154. doi: 10.20870/oeno-one.2017.51.2.1647
Van Leeuwen, C., Roby, J. P., and de Resseguier, L. (2018). Soil-related terroir factors: a review. OENO One 52, 173–188. doi: 10.20870/oeno-one.2018.52.2.2208
van Leeuwen, C., Schultz, H. R., Garcia de Cortazar-Atauri, I., Duchene, E., Ollat, N., Pieri, P., et al. (2013). Why climate change will not dramatically decrease viticultural suitability in main wine-producing areas by 2050. Proc. Natl. Acad. Sci. 110, E3051–E3052. doi: 10.1073/pnas.1307927110
Van Leeuwen, C., Tregoat, O., Chone, X., Bois, B., Pernet, D., and Gaudillere, J.-P. (2009). Vine water status is a key factor in grape ripening and vintage quality for red Bordeaux wine. How can it be assessed for vineyard management purposes? J. Int. Sci. Vigne Vin. 43, 121–134. doi: 10.20870/oeno-one.2009.43.3.798
Vanhoenacker, G., De Villiers, A., Lazou, K., De Keukeleire, D., and Sandra, P. (2001). Comparison of high-performance liquid chromatography — Mass spectroscopy and capillary electrophoresis— mass spectroscopy for the analysis of phenolic compounds in diethyl ether extracts of red wines. Chromatographia 54, 309–315. doi: 10.1007/BF02492675
Vannozzi, A., Dry, I. B., Fasoli, M., Zenoni, S., and Lucchin, M. (2012). Genome-wide analysis of the grapevine stilbene synthase multigenic family: genomic organization and expression profiles upon biotic and abiotic stresses. BMC Plant Biol. 12:130. doi: 10.1186/1471-2229-12-130
Vannozzi, A., Wong, D. C. J., Holl, J., Hmmam, I., Matus, J. T., Bogs, J., et al. (2018). Combinatorial regulation of stilbene synthase genes by WRKY and MYB transcription factors in grapevine (Vitis vinifera L.). Plant Cell Physiol. 59, 1043–1059. doi: 10.1093/pcp/pcy045
Vega, A., Gutierrez, R. A., Pena-Neira, A., Cramer, G. R., and Arce-Johnson, P. (2011). Compatible GLRaV-3 viral infections affect berry ripening decreasing sugar accumulation and anthocyanin biosynthesis in Vitis vinifera. Plant Mol. Biol. 77, 261–274. doi: 10.1007/s11103-011-9807-8
Viret, O., Spring, J.-L., and Gindro, K. (2018). Stilbenes: biomarkers of grapevine resistance to fungal diseases. OENO One 52, 235–241. doi: 10.20870/oeno-one.2018.52.3.2033
Walker, A., Lee, E., Bogs, J., McDavid, D., Thomas, M., and Robinson, S. (2007). White grapes arose through the mutation of two similar and adjacent regulatory genes. Plant J. 49, 772–785. doi: 10.1111/j.1365-313X.2006.02997.x
Wang, J., Abbey, T., Kozak, B., Madilao, L. L., Tindjau, R., Del Nin, J., et al. (2019). Evolution over the growing season of volatile organic compounds in Viognier (Vitis vinifera L.) grapes under three irrigation regimes. Food Res. Int. 125:108512. doi: 10.1016/j.foodres.2019.108512
Webb, L. B., Watterson, I., Bhend, J., Whetton, P. H., and Barlow, E. W. R. (2013). Global climate analogues for winegrowing regions in future periods: projections of temperature and precipitation. Aust. J. Grape Wine Res. 19, 331–341. doi: 10.1111/ajgw.12045
Webb, L. B., Whetton, P. H., Bhend, J., Darbyshire, R., Briggs, P. R., and Barlow, E. W. R. (2012). Earlier wine-grape ripening driven by climatic warming and drying and management practices. Nat. Clim. Chang. 2:259. doi: 10.1038/nclimate1417
Williamson, C. E., Zepp, R. G., Lucas, R. M., Madronich, S., Austin, A. T., Ballaré, C. L., et al. (2014). Solar ultraviolet radiation in a changing climate. Nat. Clim. Chang. 4:434. doi: 10.1038/nclimate2225
Wohlfahrt, Y., Patz, C. D., Schmidt, D., Rauhut, D., Honermeier, B., and Stoll, M. (2021). Responses on must and wine composition of Vitis vinifera L. cvs. Riesling and Cabernet Sauvignon under a free air CO(2) enrichment (FACE). Foods 10:145. doi: 10.3390/foods10010145
Wohlfahrt, Y., Smith, J. P., Tittmann, S., Honermeier, B., and Stoll, M. (2018). Primary productivity and physiological responses of Vitis vinifera L. cvs. under free air carbon dioxide enrichment (FACE). Eur. J. Agron. 101, 149–162. doi: 10.1016/j.eja.2018.09.005
Wolkovich, E. M., García de Cortázar-Atauri, I., Morales-Castilla, I., Nicholas, K. A., and Lacombe, T. (2018). From pinot to xinomavro in the world's future wine-growing regions. Nat. Clim. Chang. 8, 29–37. doi: 10.1038/s41558-017-0016-6
Wong, D. C. J., Schlechter, R., Vannozzi, A., Höll, J., Hmmam, I., Bogs, J., et al. (2016). A systems-oriented analysis of the grapevine R2R3-MYB transcription factor family uncovers new insights into the regulation of stilbene accumulation. DNA Res. 23, 451–466. doi: 10.1093/dnares/dsw028
Wood, C., Siebert, T. E., Parker, M., Capone, D. L., Elsey, G. M., Pollnitz, A. P., et al. (2008). From wine to pepper: rotundone, an obscure sesquiterpene, is a potent spicy aroma compound. J. Agric. Food Chem. 56, 3738–3744. doi: 10.1021/jf800183k
Wu, B.-H., Cao, Y.-G., Guan, L., Xin, H.-P., Li, J.-H., and Li, S.-H. (2014). Genome-wide transcriptional profiles of the berry skin of two red grape cultivars (Vitis vinifera) in which anthocyanin synthesis is sunlight-dependent or -independent. PLoS ONE 9:e105959. doi: 10.1371/journal.pone.0105959
Wu, J., Drappier, J., Hilbert, G., Guillaumie, H., Dai, Z., Geny, L., et al. (2019). The effects of a moderate grape temperature increase on berry secondary metabolites. OENO One 53, 321–333. doi: 10.20870/oeno-one.2019.53.2.2434
Wurz, D. A. (2019). Wine and health: a review of its benefits to human health. BIO Web Conf. 12:04001. doi: 10.1051/bioconf/20191204001
Xi, X., Zha, Q., Jiang, A., and Tian, Y. (2016). Impact of cluster thinning on transcriptional regulation of anthocyanin biosynthesis-related genes in “summer black” grapes. Plant Physiol. Biochem. 104, 180–187. doi: 10.1016/j.plaphy.2016.03.015
Yamane, T., Jeong, S. T., Goto-Yamamoto, N., Koshita, Y., and Kobayashi, S. (2006). Effects of temperature on anthocyanin biosynthesis in grape berry skins. Am. J. Enol. Vitic. 57, 54–59. Available online at: https://www.ajevonline.org/content/57/1/54
Yan, Y., Song, C., Falginella, L., and Castellarin, S. D. (2020). Day temperature has a stronger effect than night temperature on anthocyanin and flavonol accumulation in “merlot” (Vitis vinifera L.) grapes during ripening. Front. Plant Sci. 11:1095. doi: 10.3389/fpls.2020.01095
Young, P., Lashbrooke, J., Alexandersson, E., Jacobson, D., Moser, C., Velasco, R., et al. (2012). The genes and enzymes of the carotenoid metabolic pathway in Vitis vinifera L. BMC Genomics 13:243. doi: 10.1186/1471-2164-13-243
Young, P. R., Eyeghe-Bickong, H. A., du Plessis, K., Alexandersson, E., Jacobson, D. A., Coetzee, Z., et al. (2016). Grapevine plasticity in response to an altered microclimate: Sauvignon Blanc modulates specific metabolites in response to increased berry exposure. Plant Physiol. 170, 1235–1254. doi: 10.1104/pp.15.01775
Yuan, F., and Qian, M. C. (2016). Development of C13-norisoprenoids, carotenoids and other volatile compounds in Vitis vinifera L. Cv. Pinot noir grapes. Food Chem. 192, 633–641. doi: 10.1016/j.foodchem.2015.07.050
Zarrouk, O., Brunetti, C., Egipto, R., Pinheiro, C., Genebra, T., Gori, A., et al. (2016a). Grape ripening is regulated by deficit irrigation/elevated temperatures according to cluster position in the canopy. Front. Plant Sci. 7:1640. doi: 10.3389/fpls.2016.01640
Zarrouk, O., Costa, J. M., Francisco, R., Lopes, C. M., and Chaves, M. M. (2016b). “Drought and water management in mediterranean vineyards,” in Grapevine in a Changing Environment, eds H. Gerós, M. M. Chaves, H. G. Gil, and S. Delrot. 38–67. doi: 10.1002/9781118735985.ch3
Zhang, E., Chai, F., Zhang, H., Li, S., Liang, Z., and Fan, P. (2017). Effects of sunlight exclusion on the profiles of monoterpene biosynthesis and accumulation in grape exocarp and mesocarp. Food Chem. 237, 379–389. doi: 10.1016/j.foodchem.2017.05.127
Zhang, H., Fan, P., Liu, C., Wu, B., Li, S., and Liang, Z. (2014). Sunlight exclusion from muscat grape alters volatile profiles during berry development. Food Chem. 164, 242–250. doi: 10.1016/j.foodchem.2014.05.012
Zhang, L., Wang, Z., and Zhang, W. (2013). Determination of caftaric acid, p-coutaric acid and fertaric acid in grape juice, peel and seeds by ultra-high performance liquid chromatography-tandem quadrupole mass spectrometry. Se Pu 31, 122–126. doi: 10.3724/SP.J.1123.2012.09047
Zhang, Z., Pang, X., Xuewu, D., Ji, Z., and Jiang, Y. (2005). Role of peroxidase in anthocyanin degradation in litchi fruit pericarp. Food Chem. 90, 47–52. doi: 10.1016/j.foodchem.2004.03.023
Zhao, J., Pang, Y., and Dixon, R. A. (2010). The mysteries of proanthocyanidin transport and polymerization. Plant Physiol. 153, 437–443. doi: 10.1104/pp.110.155432
Zhu, B. Q., Xu, X. Q., Wu, Y. W., Duan, C. Q., and Pan, Q. H. (2012). Isolation and characterization of two hydroperoxide lyase genes from grape berries : HPL isogenes in Vitis vinifera grapes. Mol. Biol. Rep. 39, 7443–7455. doi: 10.1007/s11033-012-1577-0
Zsófi, Z., Villangó, S., Pálfi, Z., Tóth, E., and Bálo, B. (2014). Texture characteristics of the grape berry skin and seed (Vitis vinifera L. cv. Kékfrankos) under postveraison water deficit. Sci. Hortic. 172, 176–182. doi: 10.1016/j.scienta.2014.04.008
Keywords: grapevine berry, climate change, abiotic stress, secondary metabolism, phenolic compounds, aroma compounds, Vitis vinifera
Citation: Rienth M, Vigneron N, Darriet P, Sweetman C, Burbidge C, Bonghi C, Walker RP, Famiani F and Castellarin SD (2021) Grape Berry Secondary Metabolites and Their Modulation by Abiotic Factors in a Climate Change Scenario–A Review. Front. Plant Sci. 12:643258. doi: 10.3389/fpls.2021.643258
Received: 17 December 2020; Accepted: 02 February 2021;
Published: 22 March 2021.
Edited by:
Flavia Guzzo, University of Verona, ItalyReviewed by:
Zhanwu Dai, Chinese Academy of Sciences, ChinaJun Wang, China Agricultural University, China
Inmaculada Pascual, University of Navarra, Spain
Copyright © 2021 Rienth, Vigneron, Darriet, Sweetman, Burbidge, Bonghi, Walker, Famiani and Castellarin. This is an open-access article distributed under the terms of the Creative Commons Attribution License (CC BY). The use, distribution or reproduction in other forums is permitted, provided the original author(s) and the copyright owner(s) are credited and that the original publication in this journal is cited, in accordance with accepted academic practice. No use, distribution or reproduction is permitted which does not comply with these terms.
*Correspondence: Markus Rienth, bWFya3VzLnJpZW50aCYjeDAwMDQwO2NoYW5naW5zLmNo