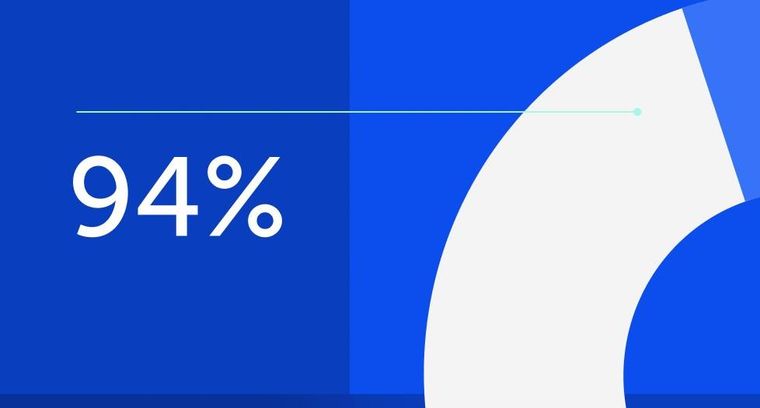
94% of researchers rate our articles as excellent or good
Learn more about the work of our research integrity team to safeguard the quality of each article we publish.
Find out more
ORIGINAL RESEARCH article
Front. Plant Sci., 16 September 2021
Sec. Crop and Product Physiology
Volume 12 - 2021 | https://doi.org/10.3389/fpls.2021.637783
This article is part of the Research TopicBiological and Genetic Basis of Agronomical and Seed Quality Traits in LegumesView all 30 articles
Nano-silicon application is an efficient novel approach to mitigate the deleterious impacts of drought stress on field crops, which is expected to increase owing to climate change, especially in arid regions. Two-season field studies investigated the influence of foliar-applied nano-silicon (0.5, 1, and 1.5 mM) on physiological and biochemical attributes and their impacts on crop water productivity (CWP) and the agronomic traits of faba beans (Vicia faba). The plants were evaluated under two irrigation regimes: well-watered (100% ETc giving 406 mm ha−1) and drought stress (65% ETc giving 264 mm ha−1). It was found that drought stress significantly decreased gas exchange (leaf net photosynthetic rate, stomatal conductance, and rate of transpiration), water relations (relative water content and membrane stability index), nutrient uptake (N, P, K+, and Ca+2), flavonoids, and phenolic content. In contrast, drought stress significantly increased oxidative stress (H2O2 and ) and enzymatic and non-enzymatic antioxidant activities compared with the well-watered treatment. These influences of drought stress were negatively reflected in seed yield-related traits and CWP. However, foliar treatment with nano-silicon, particularly with 1.5 mM, limited the devastating impact of drought stress and markedly enhanced all the aforementioned parameters. Therefore, exogenously applied nano-silicon could be used to improve the CWP and seed and biological yields of faba bean plants under conditions with low water availability in arid environments.
Faba beans (Vicia faba L.) are an important legume crop grown worldwide (Gasim et al., 2015). These plants have high crude protein content and essential amino acids (Vogelsang-O'dwyer et al., 2020) and improve soil nitrogen content through the symbiotic fixation of atmospheric nitrogen, which reduces the requirement for nitrogen fertilizer in agricultural production systems (Liu et al., 2019). Faba beans are cultivated in the Mediterranean region as a rotational crop and fix more than 80% of the nitrogen requirements of the plant (Denton et al., 2017). However, it is highly sensitive to water deficits compared with other field crops (Parvin et al., 2019).
The Mediterranean region is one of the most vulnerable areas to the deleterious impacts of climate change, with fluctuations in precipitation and water shortage being projected to increase, particularly in arid and semi-arid environments (García-Ruiz et al., 2011; Cook et al., 2016; Mansour et al., 2018; Spinoni et al., 2018; Chiwetalu et al., 2020). As a result, water scarcity causes destructive alterations in the biochemical and physiological processes of plants and, consequently, reduces their growth and productivity (Siddiqui et al., 2015; Attia et al., 2021; Desoky et al., 2021; Mansour et al., 2021). Therefore, it is crucial to mitigate the deleterious impacts of water deficiency using practical approaches to boost drought tolerance in field crops (Semida et al., 2020; Abd El-Mageed et al., 2021; El-Sanatawy et al., 2021).
Silicon (Si) considerably increases mechanical strength and membrane stability of cell. It also maintains membrane integrity, mineral nutrition, photosynthesis efficiency, and the tolerance defense system (Spinoni et al., 2018; Desoky et al., 2020). As a result, Si can be used to alleviate the negative impacts of water deficits and improve plant growth and productivity owing to its beneficial physicomechanical functions (Rady et al., 2019).
Recently, nanomaterials have become a desirable solution to many technological and environmental challenges in numerous fields (Ansari and Husain, 2012). Nano-silicon has displayed superior physicochemical properties owing to its microscopic size compared with bulk Si (Prasad et al., 2012; Rastogi et al., 2019). Furthermore, nano-silicon has a larger surface area, greater surface reactivity and solubility, and numerous well-characterized surface properties compared with bulk Si (Qados and Moftah, 2015). In particular, particle size is considered to be one of the most crucial factors impacting particle adhesion, uptake, and transportation into plant cells (Smith et al., 2008; Wang et al., 2009). In addition, nanoparticles interact with plant cells and assist in the transportation of different substances that can regulate plant metabolism and several physiological processes (Galbraith, 2007; Torney et al., 2007; Giraldo et al., 2014).
Investigations into the influence of nano metals and their mechanisms are still at the rudimentary stage. Studies related to nano-silicon application and its contribution to the attenuation of the adverse impacts of drought stress and the increasing of faba bean productivity under water-deficit conditions, particularly under field conditions, are lacking. Based on the results of previous investigations, we hypothesized that the application of nano-silicon would notably improve faba bean plant performance (growth and productivity) by improving the efficiency of enzymatic and non-enzymatic antioxidants, in turn reducing the overproduction of reactive oxygen species (ROS). Accordingly, we investigated the role of exogenously applied nano-silicon dioxide (SiO2) at different concentrations in ameliorating the drought tolerance of faba bean plants at the morphological, physiological, biochemical, and agronomic levels. This knowledge will assist in enhancing the drought tolerance of faba bean plants for their cultivation in arid environments.
A field experiment was undertaken during the 2018–2019 and 2019–2020 winter growing seasons at the experimental farm of the Faculty of Agriculture, Zagazig University, Zagazig, Egypt (30°36′57″N, 31°46′58″E). The site was characterized by low precipitation and an arid climate, with an average annual rainfall of ~60 mm. The results of the soil analysis, including bulk soil density, field capacity, wilting point, pH, texture, and soil composition, are presented in Supplementary Table 1. The monthly minimum and maximum temperatures and rainfall for the two winters and the 35-year averages (from 1986 to 2020) were obtained from a station close to the experimental site (Supplementary Table 2).
Phosphorus fertilizer was added at a rate of 31 kg P ha−1 as calcium superphosphate [Ca(H2PO4)2, 15.5% P2O5] before sowing. Nitrogen (N) fertilizer was added at a rate of 45 kg N ha−1 as ammonium-sulfate [(NH4)2SO4, 21% N] as fertigation one time at sowing. Potassium (K) fertilizer was applied at a rate of 95 kg K ha−1 as potassium sulfate (K2SO4, 48% K2O) in two equal doses every two weeks after sowing. The sowing dates of both seasons were performed according to the optimal period for growing faba beans in the region during the first week of November. The genotype used in this experiment was a recommended commercial cultivar in the region (Giza-843). Standard agronomic practices, comprising drip irrigation, sowing date, chemical fertilization, weed, disease, and pest control, were applied as recommended for the commercial production of faba beans.
The experimental design was a split-plot, with randomized irrigation regimes in the main plots and foliar treatments in sub-plots in three replicates. Each plot consisted of six rows that were 5 m long with 0.65 m between rows. The plant spacing was 0.15 m, resulting in ~205,130 plants per ha−1. A drip irrigation system was used to meet the study objectives, with drip laterals and emitters were spaced at 0.65 and 0.3 m, respectively. The operating pressure and emitter flow rate were kept at 1 bar and 4 L h−1, respectively, and maintained using a valve and pressure gauge for each irrigation sector. Irrigation water quantity was measured independently for each irrigation regime using a flow meter. Irrigation scheduling was based on potential crop evapotranspiration (ETc) replacement according to the crop coefficient approach (Allen et al., 1998). The ETc was determined by multiplying the daily reference evapotranspiration (ETo) by the Food and Agriculture Organization (FAO) crop coefficients (Kc) of faba beans (Allen et al., 1998). The ETo was determined from weather data using the FAO-56-standardized Penman–Monteith equation as stated by Allen et al. (1998). Daily meteorological data, including minimum, maximum, and dew point temperatures and wind speed, were taken from the closest weather station to calculate the ETo. The Kc figures for faba beans, as suggested by the FAO-56, were altered based on the obtained climatic values, including the wind speed and relative humidity of the experimental site. During the first and second growing seasons, the total amount of the full irrigation regime (100% ETc) was 400 and 412 mm ha−1, respectively. The drought stress regime was 35% less (260 and 268 mm ha−1) than the well-watered treatment from the seedling establishment to physiological maturity in both seasons. Irrigation was applied weekly from full emergence to flowering and then two times a week from flowering to maturity. Irrigation was discontinued 2 weeks before harvest (mid-April).
Nano-silicon dioxide (99.5% pure; 20–30 nm) (Sigma-Aldrich Chemie GmbH, Taufkirchen, Germany), with a corresponding surface area of 180–600 m2 g−1, was used in the study (Supplementary Figure 1). Foliar sprays of 0, 0.5, 1, and 1.5 mM nano-SiO2 were applied using a pressurized spray bottle with 0.1% Tween 20 as a surface spreader. Spraying with distilled water was used as a control for foliar treatments.
At the end of the growing season, the plant height, which was from the soil surface to the uppermost leaf tip, was measured for 10 replicate plants in each plot. Three middle rows from each plot were harvested from a total area of 9.8 m2 to determine the yield components (number of pods per plant and seeds per pod and 100-seed weight), seed yield, and aboveground biomass. The 100-seed weight was assessed from the weight of the three sets of 100 seeds.
The crop water productivity (kg m−3) for seed yield (CWPs) and aboveground biomass (CWPab) was estimated as the ratio of seed yield or aboveground biomass (kg ha−1) to crop evapotranspiration (ET, m3) following the formula of Pereira et al. (2012) and Fernández et al. (2020):
Crop evapotranspiration (ET, mm) was calculated according to the water balance equation (James, 1988): ET = IW + P + Cr + Dp ± Rf ± ΔS, where IW is the irrigation water amount (mm), P is the seasonal precipitation (mm), Cr is the capillary rise to the root zone (mm), Dp is the deep percolation (mm), Rf is the surface runoff (mm), and ΔS is the soil moisture change in the crop root zone (mm). The Cr in this study was zero as the groundwater table was 15 m below the ground surface. The Dp and Rf were neglected owing to the use of a drip irrigation system. Furthermore, the soil water content was determined using an oven drying method for all the experimental plots. Soil samples were collected at planting and harvest from soil depths of 0–30, 30–60, and 60–90 cm to estimate the initial and final soil moisture content during the two growing seasons. The figures were converted to a volumetric basis and multiplied by soil depth and bulk density.
All gas exchange measurements were performed using a Li-6400XT portable photosynthesis system equipped with a 6400-40 leaf chamber fluorescence head and a 6400-02 B LED light source (Li-Cor Inc., Lincoln, NE, USA) between 09:00 and 11:00 a.m. To avoid errors, CO2 leakage was corrected according to Flexas et al. (2007). All types of measurement (light response curves and CO2 response curves) were applied on the third leaves of three plants at each light intensity. Before starting the CO2 response curve measurements, the leaf was adapted for 5–20 min to ensure that photosynthesis, stomatal conductance, and fluorescence signal were stable and rubisco was fully activated. The first point was measured under a reference CO2 concentration (Ca) of 400 ppm. The A–Ci measurements were conducted at the reference CO2 concentration of 400 ppm, followed by 300, 200, 100, 50, 150, 250, 350, 600, 900, 1,200, and 1,500 ppm under the saturating light conditions of 1,300 μmol m2 s−1 (Loriaux et al., 2013). The fluorometer measuring light was turned on and set to measure light frequency = 10 kHz, intensity = 3, filter = 5, and gain = 10. The flash was set to multiphase pulse, with a target intensity = 9, ramp depth = 30%, measuring frequency = 20 kHz, and filter = 50 kHz. The three phases were 320, 350, and 200 ms long (Chen et al., 2014).
Relative water content (RWC) was assessed following the method of Osman and Rady (2014) and determined using the following equation: , where FM is fresh mass, DM is dry mass, and TM is turgid mass. The membrane stability index (MSI) was determined by adding 200 mg of fresh leaves into test tubes containing 10 cm3 of double-distilled water. One set was heated at 40°C for 30 min in a water bath, and the electrical conductivity of the solution was recorded on a conductivity bridge (C1). A replicated set was boiled at 100°C in a water bath for 10 min, with conductivity also being measured (C2). The MSI was calculated as described by Rady (2011) using the following equation: , where EC1 is the electrical conductivity at 40°C and EC2 is the electrical conductivity at 100°C.
The total soluble sugars of 0.2 g of leaf washed with 5 ml of 70% ethanol and homogenized with 5 ml of 96% ethanol were determined following the method of Irigoyen et al. (1992). Briefly, the extract was centrifuged at 1,372 × g for 10 min, and the supernatant was collected and stored at 4°C. Freshly prepared anthrone (3 ml) was added to a 0.1 ml supernatant and incubated in a hot water bath for 10 min. Absorbance was measured at 520 nm (Jenway Spectrophotometer 6705, Staffordshire, UK), and the sugar content was determined from the standard curve (Supplementary Figure 2). One gram of glucose was dissolved in distilled water, with the volume amounting to 1 L. The different volumes of the glucose solution were taken and made to amount to 100 ml with distilled water in volumetric flasks. Finally, the relationship between the readings at 520 nm and the known concentration of glucose was plotted (Supplementary Figure 2). A rapid colorimetric assay was performed to measure the proline content in 0.5 g dried leaf samples according to Bates et al. (1973). The absorbance was recorded at 520 nm, with the proline content being determined from the standard curve. The proline standard curve was developed using 1 g of pure proline, which was purchased from Sigma-Aldrich (Supplementary Figure 3).
Enzyme extraction was performed according to the method of Vitória et al. (2001). Briefly, fresh leaf samples were collected in an icebox and taken to the laboratory. Distilled water was used to wash the leaves, following which, the surfaces of the leaves were wiped out of moisture. The leaf sample (0.5 g) was homogenized in 0.1 M of an ice-cold phosphate buffer (pH 7.5) containing 0.5 mM of ethylenediaminetetraacetic acid (EDTA) using a pre-chilled mortar and pestle. The homogenate was then transferred to centrifuge tubes and centrifuged at 4°C in a Beckman Coulter refrigerated centrifuge (Beckman Coulter, Inc., California, USA) at 15,000 × g for 15 min. The supernatant was transferred to 30-ml tubes and referred to the enzyme extract.
Catalase (CAT) was estimated spectrophotometrically according to Britton and Mehley (1955). Briefly, the enzyme extract (100 μl) was added to 100 μl of 100 mM of H2O2, and the total volume has amounted to 1 ml with 250 mM of the phosphate buffer at pH 6.8. The reduction in optical density at 240 nm against the blank was measured every minute. The activity of peroxidase (POD) was estimated as described by Thomas et al. (1982) using guaiacol as the substrate. The reaction mixture contained 3 ml of the phosphate buffer (0.1 M, pH 7), 50 ml of enzyme extract, 30 ml of H2O2 (20 mM), and 50 ml of guaiacol (20 mM). The reaction mixture was incubated in a cuvette for 10 min at room temperature. The optical density was recorded at 436 nm, and the enzyme activity was expressed as the number of absorbance units g−1 fresh weight of leaves. The superoxide dismutase (SOD) activity was determined by recording the reduction in the absorbance of the superoxide-nitro blue tetrazolium complex by the enzyme SOD (Sairam et al., 2002). Approximately 3 ml of a reaction mixture comprising 0.2 ml of 200 mM methionine, 0.1 ml of 3 mM EDTA, 0.1 ml of 1.5 M sodium carbonate, 1.5 ml of 100 mM potassium phosphate buffer pH 7, 0.1 ml of 2.25 mM nitro blue tetrazolium, 1 ml of distilled water, and 0.05 ml of the enzyme, which were all collected in duplicates from each of the enzyme samples into test tubes. Two tubes without the enzyme extract were used as controls. The reaction was started by adding 0.1 ml riboflavin (60 μM) and placing the tubes below a light source (two 15-W fluorescent lamps) for 15 min. The reaction was stopped by turning off the light and covering the tubes with a black cloth. The tubes without the enzyme developed the maximum color. A non-irradiated complete reaction mixture that did not develop color served as a blank. The absorbance was recorded at 560 nm, and one unit of enzyme activity was taken as the quantity of enzymes that reduced the absorbance readings of samples to 50% compared with the tubes lacking enzymes.
The content of ascorbate (AsA; μmol g−1 FW) was determined as described by Kampfenkel et al. (1995). Reduced glutathione (GsH; μmol g−1 FW) was estimated according to the method of Griffith (1980). The α-tocopherol was estimated in accordance with the methods of Konings et al. (1996) and Ching and Mohamed (2001). Finally, the level of hydrogen peroxide (H2O2 μmol g−1 leaf FW) was determined as reported by Velikova et al. (2000) and superoxide () level was determined as described by Kubiś (2008).
Dried powder samples (0.1 g) were digested for 12 h in a mixture of 2 ml of perchloric acid (80%) with 10 ml of concentrated H2SO4. Each sample was diluted with distilled water to a volume of 100 ml. Total N was estimated using a micro-Kjeldahl method as described by Chapman and Pratt (1982). Total P was estimated colorimetrically using the ascorbic acid method as described by Watanabe and Olsen (1965). Flame photometry was used to analyze the K+ and Ca+2 content according to the method of Williams and Twine (1960).
The seeds were homogenized using a lab grinder and stored in airtight jars maintained at 4°C. Dried materials (10 g) were defatted by hexane 60–80 and then separately extracted successively with ethanol 70% (1:10 ratio) by soaking at room temperature for 12 h. The extract was centrifuged at 784 × g for 15 min (Jouan, MR 1822, Pays de la Loire, France). Extraction and filtration were repeated until the residue was colorless. The solvent was removed under vacuum at 40°C using a rotary evaporator (Laborota 4000-efficient, Heidolph, Germany), and extracts were freeze-dried using a lyophilizer. The obtained powder extracts were preserved in light-protected containers at −18°C.
The antioxidant activity was determined using 2, 2-diphenyl-1-picrylhydrazyl (DPPH). The free radical scavenging activity was estimated as a percentage of DPPH discoloration as described by Blois (1958). The flavonoids content in the extracts was estimated following the colorimetric method based on the formation of flavonoid-aluminum compounds (Zhishen et al., 1999). Total polyphenols were estimated following the Folin–Ciocalteu method of Singleton and Rossi (1965).
Discontinuous Sodium dodecyl sulfate-polyacrylamide gel electrophoresis (SDS-PAGE) (Laemmli, 1970) was used to provide the molecular weight of the isolated proteins through comparisons to standard molecular weight markers (Marker, 28–250 kDa; Sigma-Aldrich). The protein bands were visualized by staining with Coomassie Brilliant Blue G-250 (Sigma-Aldrich).
The R statistical software version 3.6.1 was used to analyze the data. A combined analysis of variance was performed for the split-plot design with irrigation regimes, foliar applications, and their interactions as fixed effects, while growing seasons, replications, and their interactions were considered random effects. The differences among irrigation regimes, foliar applications, and their interactions were separated by the least significant difference at p ≤ 0.05. A biplot of a principal component analysis (PCA) estimated the association between evaluated traits.
The water-deficit treatment significantly decreased plant height, 100-seed weight, number of pods per plant, number of seeds per pod, seed yield, and aboveground biomass compared with the well-watered treatment (Table 1). Nano-SiO2 substantially enhanced all agronomic traits compared with the corresponding untreated plants under both watering regimes. The greatest yield was achieved with 1.5 mM, followed by 1 mM nano-SiO2 in the well-watered treatment. Treatment with 1.5 mM nano-SiO2 also increased the seed yield by 14.2% in well-watered plants and 27.7% in drought-stressed plants compared with those in untreated plants (Figure 1).
Table 1. Impacts of nano-silicon dioxide (nano-SiO2) foliar application on Vicia faba plant height (PH, cm), number of pods plant−1 (NP/P), number of seeds pod−1 (NS/P), 100-seed weight (100-SW, g), seed yield (SY, kg ha−1), aboveground biomass (AB, Kg ha−1), crop water productivity (kg m−3) for seed yield (CWPs), and aboveground biomass (CWPab).
Figure 1. Percentage change (increase or decrease) in morphological, agronomic, physiological, and biochemical attributes upon the application of 1.5 mM nano-silicon dioxide (nano-SiO2) compared with untreated faba bean (Vicia faba) plants under well-watered and drought-stressed conditions.
The CWP for seed yield (CWPs) ranged from 1.22 to 1.65 kg m−3, while the aboveground biomass (CWPab) ranged from 2.81 to 3.72 kg m−3 (Table 1). Under drought stress conditions, faba bean plants possessed higher CWPs (on average 1.5 kg m−3) and CWPab (3.8 kg m−3) than those that underwent the well-watered treatment (1.31 and 3.12 kg m−3, respectively). Drought-stressed plants also had a higher CWP than well-watered plants due to their more efficient water consumption and water loss reduction due to osmotic regulation. The application of nano-SiO2 also significantly improved CWPs and CWPab compared with the untreated stressed controls. The highest CWPs (1.65 kg m−3) and CWPab (3.72 kg m−3) were achieved with the application of 1.5 mM nano-SiO2.
Drought stress significantly reduced the net photosynthetic rate (Pn), transpiration rate (Tr), stomatal conductance (gs), RWC, MSI, and nutrient content (N, P, K+, and Ca+2) of faba bean plants (Table 2). All nano-SiO2 treatments mitigated the damage of drought stress and significantly improved all physiological parameters. The highest values were seen in well-watered plants treated with 1.5 mM nano-SiO2, while the lowest values were recorded in the control water-stressed plants (Table 2).
Table 2. Impacts of nano-silicon dioxide (nano-SiO2) foliar application on the net photosynthetic rate (Pn, μmol CO2 m−2 s−1), transpiration rate (Tr, μmol CO2 m−2 s−1), stomatal conductance (gs, μmol CO2 m−2 s−1), relative water content (RWC, %), membrane stability index (MSI, %), nitrogen (N, %), phosphorus (P, %), potassium (K, %), and calcium (Ca, %) contents of faba bean (Vicia faba) grown under well-watered and drought-stressed conditions over two growing seasons (2019 and 2020).
Drought-stressed plants increased free proline and soluble sugars and the activity of the antioxidant enzymes CAT, POD, and SOD. Furthermore, the non-enzymatic antioxidants AsA, GsH, and α-tocopherol were higher compared with non-stressed faba beans (Table 3). In contrast, the application of nano-SiO2 significantly increased the free proline and soluble sugar contents and the activities of antioxidant enzymes and non-enzymatic antioxidant compounds compared with the untreated plants under both irrigation regimes. The highest values were achieved with the application of 1.5 mM of nano-SiO2 to the stressed plants (Table 3). Increasing free proline, soluble sugars, antioxidant enzyme activities, and non-enzymatic antioxidant compound content helped the faba bean plants alleviate the negative impacts of water scarcity.
Table 3. Impacts of nano-silicon dioxide (nano-SiO2) foliar application on the proline content (Pro, μmol g−1 DW), soluble sugars (SSu, mg g−1 DW), peroxidase activity (POD, unit mg−1 protein), catalase activity (CAT, unit mg−1 protein), superoxide dismutase activity (SOD, unit mg−1 protein), ascorbic acid (AsA, μmol g−1 DW), glutathione (GsH, μmol g−1 FW), superoxide radical (, A580 g−1 FW), hydrogen peroxide (H2O2, μmol g−1 FW), and α-tocopherol (α-TOC, μmol g−1 DW) of well-watered and drought-stressed faba beans (Vicia faba) over two growing seasons (2019 and 2020).
In addition, irrigation regimes and nano-SiO2 application significantly impacted oxidative stress biomarkers (Table 3). The levels of H2O2 and were significantly increased in stressed compared with non-stressed plants. Furthermore, the application of nano-SiO2 to plants in the water-deficit treatment significantly lowered H2O2 and levels compared with those in untreated plants.
Drought stress caused a significant increase in antioxidant activity, flavonoids, and phenolics (Table 4). The application of nano-SiO2 significantly enhanced these parameters compared with those in the untreated plants under both irrigation regimes. The highest antioxidant activity and values of flavonoids and phenolics were produced by the 1.5-mM nano-SiO2 application in the water-deficit treatments, while the lowest values were recorded in the well-watered control plants (Table 4).
Table 4. Impact of nano-silicon dioxide (nano-SiO2) foliar application on antioxidant activity (%), flavonoids content (μg QE/g), and phenolic content (μg GAE/g) of faba beans (Vicia faba) under well-watered or drought-stressed conditions.
The SDS-PAGE patterns displayed nine protein bands (170, 158, 110, 90, 81, 63, 57, 40, and 30 kD) in untreated faba bean plants grown under well-watered or water-deficit conditions (Table 5). However, the nano-SiO2 concentrations of 1 and 1.5 mM created a protein fraction with a molecular weight of 129 kD only in the faba bean plants grown under drought stress. Only nine bands were observed in the rest of the treatments in both watering regimes.
Table 5. Effect of nano-silicon dioxide (nano-SiO2) on protein profile in faba bean (Vicia faba) seeds under well-watered and drought-stressed conditions.
The PCA of the agronomic, physiological, and biochemical traits showed that the first two principal components accounted for most of the variability, which was ~98.41% (83.43% by PC1 and 14.98% by PC2; Figure 2). In the PC biplot, the traits were represented by parallel vectors or those that were close to each other, indicating a strong positive association, whereas those that were situated approximately opposite (at 180°) showed a highly negative relationship. In addition, the vectors toward the sides expressed a slight relationship. The 30 investigated traits could be classified into three groups: the first group (15 traits) comprises agronomic traits, namely, Pn, Tr, gs, MSI, RWC, and nutrient contents (N, P, K+, and Ca+2), the second group (13 traits) consisted of the CWP for seed yield and aboveground biomass, antioxidant activity, flavonoids and phenolic contents, proline and soluble sugar contents, enzymatic antioxidants (POD, CAT, and SOD), and non-enzymatic antioxidant compounds (AsA, GsH, α-TOC, proline, and soluble sugars), and the third group contained H2O2 and . Furthermore, PC1 separated the treatments into two groups. The well-watered treatments were located on the positive side, while those under drought stress were located on the negative side (Figure 2). The traits in the first group were associated with well-watered plants, especially those that were treated with 1 and 1.5 mM nano-SiO2, while the biochemical attributes in the second and third groups were associated with the drought-stressed treatments.
Figure 2. Biplot of the first two principal components for the physiological, biochemical, and agronomic traits of faba beans (Vicia faba). The physiological parameters comprised of net photosynthetic rate (Pn), transpiration rate (Tr), stomatal conductance (gs), relative water content (RWC), membrane stability index (MSI), nitrogen (N), phosphorus (P), potassium (K), calcium (Ca) content, proline content (Pro), soluble sugars (SSu), peroxidase activity (POD), catalase activity (CAT), superoxide dismutase activity (SOD), ascorbic acid (AsA), glutathione (GsH), hydrogen peroxide (H2O2), superoxide radical (), and α-tocopherol (α-TOC). The biochemical parameters included antioxidant activity % (Ant%), flavonoids content (Flav), and phenolic content (Phen). The agronomic traits were plant height (PH), number of pods plant−1 (NP/P), number of seeds pod−1 (NS/P), 100-seed weight (100-SW), seed yield (SY), aboveground biomass (AB), crop water productivity for seed yield (CWPs), and aboveground biomass (CWPab). WW-Cont, WW-T1, WW-T2, and WW-T3 were foliar applications using tap water, 0.5, 1, and 1.5 mM of nano-silicon dioxide (nano-SiO2) under the well-watered treatment, respectively. DS-Cont, DS-T1, DS-T2, and DS-T3 were foliar applications using tap water, 0.5, 1, and 1.5 mM of nano-SiO2 under the drought stress treatment.
Water scarcity stress poses great challenges to sustainable faba bean production due to its sensitivity to drought. Consequently, it is vital to identify novel approaches to enhance drought tolerance, especially under conditions of abrupt climate change. In the current study, drought-stressed faba bean plants exhibited a decline in seed yield-related traits and CWP and an increase in oxidative and osmotic stress compared with those of well-watered plants. As a result, exogenously applied nano-SiO2 enhanced gas exchange, water relations, nutrient uptake, activities of antioxidant enzymes, and non-enzymatic antioxidant compounds, which substantially reduced oxidative stress and positively reflected the improvement of drought tolerance in faba bean plants with enhancements in yield-related traits and CWP.
The Si nanoparticles have great availability and are easily absorbed by plants compared with bulk Si, consequently supporting greater ameliorative impacts under abiotic stresses (Suriyaprabha et al., 2012; Tripathi et al., 2016). The obtained results demonstrated that the application of nano-SiO2, particularly at 1.5 mM, considerably boosted photosynthetic effectiveness and leaf gas exchange (e.g., Pn, Tr, and gs) in plants under water-deficit stress compared to those that were untreated. Promoting photosynthetic efficiency maintenance could be attributed to optimal stomatal conductance and strong antioxidant activities, which improve plant tolerance to drought stress (Suriyaprabha et al., 2012; Haghighi and Pessarakli, 2013; Rios et al., 2017; Jia et al., 2021). Furthermore, the application of nano-SiO2 increased the water uptake from roots to leaves by enhancing the RWC and MSI in treated faba bean plants compared with those in control plants under drought stress. Previous studies have also found that maintaining RWC and MSI at a healthy status enhances osmotic adjustments and metabolic activities under conditions of drought stress (Slabbert and Krüger, 2014). In this study, nano-SiO2 application likely boosted the water content of plants by decreasing stomatal transpiration and increasing turgor pressure and potassium absorbance (Romero-Aranda et al., 2006; Tahir et al., 2012; Liu et al., 2014; Cerný et al., 2020). Furthermore, nano-SiO2 application significantly improved the absorption of the nutrients N, P, K+, and Ca+2 in faba bean plants under drought stress. In addition, it has been found that mineral nutrient uptake depends mainly on membrane activity, which plays a fundamental role in the ion movement from the soil to the plant and controls the allocation of cells (Khoshgoftarmanesh et al., 2014; Gupta et al., 2016). These enhancements in water movement and nutrient absorption enable improvements in all physiological activities of the cells.
Within cells, the accumulation of compatible solutes under stress effectively maintains the status of plant cell water. For example, proline is an excellent compatible osmolyte that improves plant antioxidant systems and affects osmotic adjustment (Zhu, 2001; Desoky et al., 2020). Soluble sugars also maintain the balance between the vacuole and the osmotic quality of cytosol under abiotic stress conditions (Sairam et al., 2002). The results indicated that the application of nano-SiO2 elevated the proline and soluble sugar contents in drought-stressed plants. Therefore, its application improved plant tolerance by elevating osmolyte content, altering osmotic potential, and maintaining higher turgor in response to drought stress (Claussen, 2005; Rizwan et al., 2015; Zhang et al., 2017; Desoky et al., 2020). Furthermore, improved enzymatic and non-enzymatic defense systems can alleviate the overproduction of ROS, such as and H2O2, produced in response to drought stress. The enzymatic defense system components SOD, CAT, and POD and the non-enzymatic defense system components GsH, AsA, and α-TOC were significantly increased following the application of nano-SiO2 compared with those in untreated plants under drought stress. Accordingly, the application of nano-SiO2 lowered the concentrations of and H2O2 and, therefore, reduced oxidative stress in drought-stressed faba bean plants. Consequently, the application of nano-SiO2 could be an effective tool to increase faba bean tolerance to oxidative stress by enhancing ROS scavenging enzymatic and non-enzymatic defense systems (Rios et al., 2017; Rady et al., 2019).
The application of nano-SiO2 enhanced flavonoids, phenolic compounds, and antioxidant activity levels, particularly in drought-stressed faba bean plants. The utilization of these protection systems helps to overcome oxidative damage, which has been suggested to occur through the synthesis of secondary metabolites, such as flavonoids and phenolic contents (Blokhina et al., 2003). These metabolites can forestall protein denaturation, DNA damage, and lipid peroxidation (Król et al., 2014; Quan et al., 2016). Although Si is not a fundamental component of plants, it has a beneficial impact in improving protection against drought stress through the initiation of protective proteins and the reduction of ROS (Suriyaprabha et al., 2013; Luyckx et al., 2017). In addition, the electrograph of the protein fraction profile showed that the application of 1 and 1.5 mM nano-SiO2 led to the generation of a new protein fraction (molecular weight: 129 kD).
The application of nano-SiO2 exhibited significant positive alterations in all the investigated physiological and biochemical attributes under water-deficit conditions. Accordingly, treated plants grew more efficiently under drought stress compared with untreated plants and coped with water deficit conditions. Nano-SiO2 application, particularly using a concentration of 1.5 mM as an optimum level, attenuated the devastating impacts of drought stress by improving photosynthetic efficiency, plant water status, nutrient absorption, nutrient uptake, non-enzymatic and enzymatic antioxidant activities, and ROS scavenging. These promotional influences were reflected in increasing seed yield and all its related traits and CWP compared with the corresponding untreated plants.
The interrelationship among the evaluated parameters (Figure 2) reflected that the agronomic traits positively associated with Pn, Tr, gs, MSI, RWC, and nutrient contents (physiological parameters). We speculate that the high values of these physiological parameters are associated with greater seed yield and contributing traits, particularly under conditions of drought stress. Otherwise, the CWPs and CWPab demonstrated a highly positive association with antioxidant activity, flavonoids, and phenolic compounds. In addition, the agronomic traits exhibited a highly negative association with H2O2 and (Desoky et al., 2020; Mansour et al., 2020, 2021). According to these results, it is interesting to detect specific physiological and biochemical parameters highly associated with yield-related traits or CWP under water-deficit conditions.
Drought stress in faba bean plants resulted in reduced gas exchange, leaf water potential, leaf turgidity, nutrient uptake, photosynthetic rate, and flavonoids and phenolic content compared with the same levels in well-watered plants. These adverse impacts were reflected in decreased yield-related traits and CWP. However, exogenously applied nano-SiO2, particularly at 1.5 mM, mitigated the negative impacts of drought stress and promoted plant growth by boosting all of the investigated physiological attributes. In addition, the application of nano-SiO2 decreased the membrane leakage of electrolytes and membrane lipid peroxidation due to reduced drought-stimulated oxidative stress by enhancing the activity of osmoprotectants and the enzymatic and non-enzymatic defense system components under drought stress. Consequently, nano-SiO2 had a positive influence on the physiological, biochemical, and agronomic traits of drought-stressed plants and alleviated the influence of drought stress on faba bean plants.
The original contributions presented in the study are included in the article/Supplementary Material, further inquiries can be directed to the corresponding author.
ED, EM, EAE, MA, TT, HE, MY, and KE-T conceived and designed the experiments. SA and RE helped in conducting the experiments and collected the literature. ED, MA, TT, HE, and KE-T analyzed the data and drafted the manuscript. EM, EAE, RE, MY, and KE-T wrote and made the final edits to the manuscript. All the authors read and approved the final version of the manuscript.
The authors declare that the research was conducted in the absence of any commercial or financial relationships that could be construed as a potential conflict of interest.
All claims expressed in this article are solely those of the authors and do not necessarily represent those of their affiliated organizations, or those of the publisher, the editors and the reviewers. Any product that may be evaluated in this article, or claim that may be made by its manufacturer, is not guaranteed or endorsed by the publisher.
KE-T thanks Abu Dhabi Award for Research Excellence-Department of Education and Knowledge (21S105) for support. KE-T also thanks the library at Murdoch University, Australia for the valuable online resources and comprehensive databases.
The Supplementary Material for this article can be found online at: https://www.frontiersin.org/articles/10.3389/fpls.2021.637783/full#supplementary-material
Abd El-Mageed, T. A., Belal, E. E., Rady, M. O., El-Mageed, A., Shimaa, A., Mansour, E., et al. (2021). Acidified biochar as a soil amendment to drought stressed (Vicia faba L.) plants: influences on growth and productivity, nutrient status, and water use efficiency. Agronomy 11:1290. doi: 10.3390/agronomy11071290
Allen, R. G., Pereira, L. S., Raes, D., and Smith, M. (1998). Crop evapotranspiration-Guidelines for computing crop water requirements-FAO Irrigation and drainage paper 56. FAO U N Rome Italy 300:D05109.
Ansari, S. A., and Husain, Q. (2012). Potential applications of enzymes immobilized on/in nano materials: a review. Biotechnol. Adv. 30, 512–523. doi: 10.1016/j.biotechadv.2011.09.005
Attia, A., El-Hendawy, S., Al-Suhaibani, N., Tahir, M. U., Mubushar, M., Dos Santos Vianna, M., et al. (2021). Sensitivity of the DSSAT model in simulating maize yield and soil carbon dynamics in arid Mediterranean climate: effect of soil, genotype and crop management. Field Crops Res. 260:107981. doi: 10.1016/j.fcr.2020.107981
Bates, L. S., Waldren, R. P., and Teare, I. (1973). Rapid determination of free proline for water-stress studies. Plant Soil 39, 205–207. doi: 10.1007/BF00018060
Blois, M. S. (1958). Antioxidant determinations by the use of a stable free radical. Nature 181, 1199–1200. doi: 10.1038/1811199a0
Blokhina, O., Virolainen, E., and Fagerstedt, K. V. (2003). Antioxidants, oxidative damage and oxygen deprivation stress: a review. Ann. Bot. 91, 179–194. doi: 10.1093/aob/mcf118
Britton, C., and Mehley, A. (1955). Assay of catalase and peroxidase. Meth. Enzymol. 2, 764–775. doi: 10.1016/S0076-6879(55)02300-8
Cerný, J., Haninec, P., and Pokorný, R. (2020). Leaf area index estimated by direct, semi-direct, and indirect methods in European beech and sycamore maple stands. J. For. Res. 31, 827–836. doi: 10.1007/s11676-018-0809-0
Chapman, H., and Pratt, F. (1982). Determination of Minerals by Titration Method: Methods of Analysis for Soils, Plants and Water, 2nd Edn. Oakland, CA: Agriculture Division, California University, 169–170.
Chen, T.-W., Henke, M., De Visser, P. H., Buck-Sorlin, G., Wiechers, D., Kahlen, K., et al. (2014). What is the most prominent factor limiting photosynthesis in different layers of a greenhouse cucumber canopy? Ann. Bot. 114, 677–688. doi: 10.1093/aob/mcu100
Ching, L. S., and Mohamed, S. (2001). Alpha-tocopherol content in 62 edible tropical plants. J. Agric. Food Chem. 49, 3101–3105. doi: 10.1021/jf000891u
Chiwetalu, U. J., Mbajiorgu, C. C., and Ogbuagu, N. J. (2020). Remedial ability of maize (Zea mays) on lead contamination under potted condition and non-potted field soil condition. J. Bioresour. Bioprod. 5, 51–59. doi: 10.1016/j.jobab.2020.03.006
Claussen, W. (2005). Proline as a measure of stress in tomato plants. Plant Sci. 168, 241–248. doi: 10.1016/j.plantsci.2004.07.039
Cook, B. I., Anchukaitis, K. J., Touchan, R., Meko, D. M., and Cook, E. R. (2016). Spatiotemporal drought variability in the Mediterranean over the last 900 years. J. Geophys. Res. Atmos. 121, 2060–2074. doi: 10.1002/2015JD023929
Denton, M. D., Phillips, L. A., Peoples, M. B., Pearce, D. J., Swan, A. D., Mele, P. M., et al. (2017). Legume inoculant application methods: effects on nodulation patterns, nitrogen fixation, crop growth and yield in narrow-leaf lupin and faba bean. Plant Soil. 419, 25–39. doi: 10.1007/s11104-017-3317-7
Desoky, E.-S. M., Mansour, E., Yasin, M. A., El Sobky, E.-S. E., and Rady, M. M. (2020). Improvement of drought tolerance in five different cultivars of Vicia faba with foliar application of ascorbic acid or silicon. Span. J. Agric. Res. 18:16. doi: 10.5424/sjar/2020182-16122
Desoky, E.-S. M., Merwad, A.-R., Abo El-Maati, M. F., Mansour, E., Arnaout, S. M., Awad, M. F., et al. (2021). Physiological and biochemical mechanisms of exogenously applied selenium for alleviating destructive impacts induced by salinity stress in bread wheat. Agronomy 11:926. doi: 10.3390/agronomy11050926
El-Sanatawy, A. M., El-Kholy, A. S., Ali, M., Awad, M. F., and Mansour, E. (2021). Maize seedling establishment, grain yield and crop water productivity response to seed priming and irrigation management in a Mediterranean arid environment. Agronomy 11:756. doi: 10.3390/agronomy11040756
Fernández, J., Alcon, F., Diaz-Espejo, A., Hernandez-Santana, V., and Cuevas, M. (2020). Water use indicators and economic analysis for on-farm irrigation decision: a case study of a super high density olive tree orchard. Agric. Water Manag. 237:106074. doi: 10.1016/j.agwat.2020.106074
Flexas, J., Díaz-Espejo, A., Berry, J., Cifre, J., Galmés, J., Kaldenhoff, R., et al. (2007). Analysis of leakage in IRGA's leaf chambers of open gas exchange systems: quantification and its effects in photosynthesis parameterization. J. Exp. Bot. 58, 1533–1543. doi: 10.1093/jxb/erm027
Galbraith, D. W. (2007). Silica breaks through in plants. Nat. Nanotechnol. 2, 272–273. doi: 10.1038/nnano.2007.118
García-Ruiz, J. M., López-Moreno, J. I., Vicente-Serrano, S. M., Lasanta–Martínez, T., and Beguería, S. (2011). Mediterranean water resources in a global change scenario. Earth Sci. Rev. 105, 121–139. doi: 10.1016/j.earscirev.2011.01.006
Gasim, S., Hamad, S. A., Abdelmula, A., and Mohamed Ahmed, I. A. (2015). Yield and quality attributes of faba bean inbred lines grown under marginal environmental conditions of Sudan. Food Sci. Nutr. 3, 539–547. doi: 10.1002/fsn3.245
Giraldo, J. P., Landry, M. P., Faltermeier, S. M., Mcnicholas, T. P., Iverson, N. M., Boghossian, A. A., et al. (2014). Plant nanobionics approach to augment photosynthesis and biochemical sensing. Nat. Mater. 13, 400–408. doi: 10.1038/nmat3890
Griffith, O. W. (1980). Determination of glutathione and glutathione disulfide using glutathione reductase and 2-vinylpyridine. Anal. Biochem. 106, 207–212. doi: 10.1016/0003-2697(80)90139-6
Gupta, N., Ram, H., and Kumar, B. (2016). Mechanism of Zinc absorption in plants: uptake, transport, translocation and accumulation. Rev. Environ. Sci. Biotechnol. 15, 89–109. doi: 10.1007/s11157-016-9390-1
Haghighi, M., and Pessarakli, M. (2013). Influence of silicon and nano-silicon on salinity tolerance of cherry tomatoes (Solanum lycopersicum L.) at early growth stage. Sci. Hortic. 161, 111–117. doi: 10.1016/j.scienta.2013.06.034
Irigoyen, J., Einerich, D., and Sánchez-Díaz, M. (1992). Water stress induced changes in concentrations of proline and total soluble sugars in nodulated alfalfa (Medicago sativa) plants. Physiol. Plant. 84, 55–60. doi: 10.1111/j.1399-3054.1992.tb08764.x
James, L. G. (1988). Principles of Farm Irrigation Systems Design. New York, NY: John Wiley and Sons Inc.
Jia, X., Mao, K., Wang, P., Wang, Y., Jia, X., Huo, L., et al. (2021). Overexpression of MdATG8i improves water use efficiency in transgenic apple by modulating photosynthesis, osmotic balance, and autophagic activity under moderate water deficit. Hortic. Res. 8, 1–15. doi: 10.1038/s41438-021-00521-2
Kampfenkel, K., Vanmontagu, M., and Inze, D. (1995). Extraction and determination of ascorbate and dehydroascorbate from plant tissue. Anal. Biochem. 225, 165–167. doi: 10.1006/abio.1995.1127
Khoshgoftarmanesh, A. H., Khodarahmi, S., and Haghighi, M. (2014). Effect of silicon nutrition on lipid peroxidation and antioxidant response of cucumber plants exposed to salinity stress. Arch. Agron. Soil Sci. 60, 639–653. doi: 10.1080/03650340.2013.822487
Konings, E. J., Roomans, H. H., and Beljaars, P. R. (1996). Liquid chromatographic determination of tocopherols and tocotrienols in margarine, infant foods, and vegetables. J. AOAC Int. 79, 902–906. doi: 10.1093/jaoac/79.4.902
Król, A., Amarowicz, R., and Weidner, S. (2014). Changes in the composition of phenolic compounds and antioxidant properties of grapevine roots and leaves (Vitis vinifera L.) under continuous of long-term drought stress. Acta Physiol. Plant. 36, 1491–1499. doi: 10.1007/s11738-014-1526-8
Kubiś, J. (2008). Exogenous spermidine differentially alters activities of some scavenging system enzymes, H2O2 and superoxide radical levels in water-stressed cucumber leaves. J. Plant Physiol. 165, 397–406. doi: 10.1016/j.jplph.2007.02.005
Laemmli, U. K. (1970). Cleavage of structural proteins during the assembly of the head of bacteriophage T4. Nature 227, 680–685. doi: 10.1038/227680a0
Liu, L., Knight, J. D., Lemke, R. L., and Farrell, R. E. (2019). A side-by-side comparison of biological nitrogen fixation and yield of four legume crops. Plant Soil. 442, 169–182. doi: 10.1007/s11104-019-04167-x
Liu, P., Yin, L., Deng, X., Wang, S., Tanaka, K., and Zhang, S. (2014). Aquaporin-mediated increase in root hydraulic conductance is involved in silicon-induced improved root water uptake under osmotic stress in Sorghum bicolor L. J. Exp. Bot. 65, 4747–4756. doi: 10.1093/jxb/eru220
Loriaux, S., Avenson, T., Welles, J., Mcdermitt, D., Eckles, R., Riensche, B., et al. (2013). Closing in on maximum yield of chlorophyll fluorescence using a single multiphase flash of sub-saturating intensity. Plant Cell Environ. 36, 1755–1770. doi: 10.1111/pce.12115
Luyckx, M., Hausman, J.-F., Lutts, S., and Guerriero, G. (2017). Silicon and plants: current knowledge and technological perspectives. Front. Plant Sci. 8:411. doi: 10.3389/fpls.2017.00411
Mansour, E., Desoky, E. M., Ali, M. M. A., Abdul-Hamid, M. I., Ullah, H., Attia, A., et al. (2021). Identifying drought-tolerant genotypes of faba bean and their agro-physiological responses to different water regimes in an arid Mediterranean environment. Agric. Water Manag. 247:106754. doi: 10.1016/j.agwat.2021.106754
Mansour, E., Moustafa, E. S., Desoky, E.-S. M., Ali, M., Yasin, M. A., Attia, A., et al. (2020). Multidimensional evaluation for detecting salt tolerance of bread wheat genotypes under actual saline field growing conditions. Plants 9:1324. doi: 10.3390/plants9101324
Mansour, E., Moustafa, E. S., El-Naggar, N. Z., Abdelsalam, A., and Igartua, E. (2018). Grain yield stability of high-yielding barley genotypes under Egyptian conditions for enhancing resilience to climate change. Crop Pasture Sci. 69, 681–690. doi: 10.1071/CP18144
Osman, A. S., and Rady, M. M. (2014). Effect of humic acid as an additive to growing media to enhance the production of eggplant and tomato transplants. J. Hortic. Sci. Biotechnol. 89, 237–244. doi: 10.1080/14620316.2014.11513074
Parvin, S., Uddin, S., Tausz-Posch, S., Fitzgerald, G., Armstrong, R., and Tausz, M. (2019). Elevated CO2 improves yield and N2 fixation but not grain N concentration of faba bean (Vicia faba L.) subjected to terminal drought. Environ. Exp. Bot. 165, 161–173. doi: 10.1016/j.envexpbot.2019.06.003
Pereira, L. S., Cordery, I., and Iacovides, I. (2012). Improved indicators of water use performance and productivity for sustainable water conservation and saving. Agric. Water Manag. 108, 39–51. doi: 10.1016/j.agwat.2011.08.022
Prasad, T., Sudhakar, P., Sreenivasulu, Y., Latha, P., Munaswamy, V., Reddy, K. R., et al. (2012). Effect of nanoscale zinc oxide particles on the germination, growth and yield of peanut. J. Plant Nutr. 35, 905–927. doi: 10.1080/01904167.2012.663443
Qados, A. M. A., and Moftah, A. E. (2015). Influence of silicon and nano-silicon on germination, growth, and yield of faba bean (Vicia faba L.) under salt stress conditions. J. Exp. Agric. Int. 5, 509–524. doi: 10.9734/AJEA/2015/14109
Quan, N. T., Anh, L. H., Khang, D. T., Tuyen, P. T., Toan, N. P., Minh, T. N., et al. (2016). Involvement of secondary metabolites in response to drought stress of rice (Oryza sativa L.). Agriculture 6:23. doi: 10.3390/agriculture6020023
Rady, M. M. (2011). Effect of 24-epibrassinolide on growth, yield, antioxidant system and cadmium content of bean (Phaseolus vulgaris L.) plants under salinity and cadmium stress. Sci. Hortic. 129, 232–237. doi: 10.1016/j.scienta.2011.03.035
Rady, M. M., Elrys, A. S., El-Maati, M. F. A., and Desoky, E.-S. M. (2019). Interplaying roles of silicon and proline effectively improve salt and cadmium stress tolerance in Phaseolus vulgaris plant. Plant Physiol. Bioch. 139, 558–568. doi: 10.1016/j.plaphy.2019.04.025
Rastogi, A., Tripathi, D. K., Yadav, S., Chauhan, D. K., Živčák, M., Ghorbanpour, M., et al. (2019). Application of silicon nanoparticles in agriculture. 3 Biotech 9, 1–11. doi: 10.1007/s13205-019-1626-7
Rios, J. J., Martínez-Ballesta, M. C., Ruiz, J. M., Blasco, B., and Carvajal, M. (2017). Silicon-mediated improvement in plant salinity tolerance: the role of aquaporins. Front. Plant Sci. 8:948. doi: 10.3389/fpls.2017.00948
Rizwan, M., Ali, S., Ibrahim, M., Farid, M., Adrees, M., Bharwana, S. A., et al. (2015). Mechanisms of silicon-mediated alleviation of drought and salt stress in plants: a review. Environ. Sci. Pollut. Res. 22, 15416–15431. doi: 10.1007/s11356-015-5305-x
Romero-Aranda, M. R., Jurado, O., and Cuartero, J. (2006). Silicon alleviates the deleterious salt effect on tomato plant growth by improving plant water status. J. Plant Physiol. 163, 847–855. doi: 10.1016/j.jplph.2005.05.010
Sairam, R. K., Rao, K. V., and Srivastava, G. (2002). Differential response of wheat genotypes to long term salinity stress in relation to oxidative stress, antioxidant activity and osmolyte concentration. Plant Sci. 163, 1037–1046. doi: 10.1016/S0168-9452(02)00278-9
Semida, W. M., Abdelkhalik, A., Rady, M. O., Marey, R. A., and Abd El-Mageed, T. A. (2020). Exogenously applied proline enhances growth and productivity of drought stressed onion by improving photosynthetic efficiency, water use efficiency and up-regulating osmoprotectants. Sci. Hortic. 272:109580. doi: 10.1016/j.scienta.2020.109580
Siddiqui, M. H., Al-Khaishany, M. Y., Al-Qutami, M. A., Al-Whaibi, M. H., Grover, A., Ali, H. M., et al. (2015). Response of different genotypes of faba bean plant to drought stress. Int. J. Mol. Sci. 16, 10214–10227. doi: 10.3390/ijms160510214
Singleton, V. L., and Rossi, J. A. (1965). Colorimetry of total phenolics with phosphomolybdic-phosphotungstic acid reagents. Am. J. Enol. Vitic. 16, 144–158.
Slabbert, M., and Krüger, G. (2014). Antioxidant enzyme activity, proline accumulation, leaf area and cell membrane stability in water stressed Amaranthus leaves. S. Afr. J. Bot. 95, 123–128. doi: 10.1016/j.sajb.2014.08.008
Smith, A. M., Duan, H., Mohs, A. M., and Nie, S. (2008). Bioconjugated quantum dots for in vivo molecular and cellular imaging. Adv. Drug Deliv. Rev. 60, 1226–1240. doi: 10.1016/j.addr.2008.03.015
Spinoni, J., Vogt, J. V., Naumann, G., Barbosa, P., and Dosio, A. (2018). Will drought events become more frequent and severe in Europe? Int. J. Climatol. 38, 1718–1736. doi: 10.1002/joc.5291
Suriyaprabha, R., Karunakaran, G., Kavitha, K., Yuvakkumar, R., Rajendran, V., and Kannan, N. (2013). Application of silica nanoparticles in maize to enhance fungal resistance. IET Nanobiotechnol. 8, 133–137. doi: 10.1049/iet-nbt.2013.0004
Suriyaprabha, R., Karunakaran, G., Yuvakkumar, R., Prabu, P., Rajendran, V., and Kannan, N. (2012). Growth and physiological responses of maize (Zea mays L.) to porous silica nanoparticles in soil. J. Nanopart. Res. 14:1294. doi: 10.1007/s11051-012-1294-6
Tahir, M. A., Aziz, T., Farooq, M., and Sarwar, G. (2012). Silicon-induced changes in growth, ionic composition, water relations, chlorophyll contents and membrane permeability in two salt-stressed wheat genotypes. Arch. Agron. Soil Sci. 58, 247–256. doi: 10.1080/03650340.2010.518959
Thomas, R., Jen, J., and Morr, C. (1982). Changes in soluble and bound peroxidase—IAA oxidase during tomato fruit development. J. Food Sci. 47, 158–161. doi: 10.1111/j.1365-2621.1982.tb11048.x
Torney, F., Trewyn, B. G., Lin, V. S.-Y., and Wang, K. (2007). Mesoporous silica nanoparticles deliver DNA and chemicals into plants. Nat. Nanotechnol. 2, 295–300. doi: 10.1038/nnano.2007.108
Tripathi, D. K., Singh, S., Singh, V. P., Prasad, S. M., Chauhan, D. K., and Dubey, N. K. (2016). Silicon nanoparticles more efficiently alleviate arsenate toxicity than silicon in maize cultiver and hybrid differing in arsenate tolerance. Front. Environ. Sci. 4:46. doi: 10.3389/fenvs.2016.00046
Velikova, V., Yordanov, I., and Edreva, A. (2000). Oxidative stress and some antioxidant systems in acid rain-treated bean plants: protective role of exogenous polyamines. Plant Sci. 151, 59–66. doi: 10.1016/S0168-9452(99)00197-1
Vitória, A. P., Lea, P. J., and Azevedo, R. A. (2001). Antioxidant enzymes responses to cadmium in radish tissues. Phytochemistry 57, 701–710. doi: 10.1016/S0031-9422(01)00130-3
Vogelsang-O'dwyer, M., Petersen, I. L., Joehnke, M. S., Sørensen, J. C., Bez, J., Detzel, A., et al. (2020). Comparison of faba bean protein ingredients produced using dry fractionation and isoelectric precipitation: techno-functional, nutritional and environmental performance. Foods 9:322. doi: 10.3390/foods9030322
Wang, L., Wang, Z., Xu, Y., Joo, S. H., Kim, S. K., Xue, Z., et al. (2009). OsGSR1 is involved in crosstalk between gibberellins and brassinosteroids in rice. Plant J. 57, 498–510. doi: 10.1111/j.1365-313X.2008.03707.x
Watanabe, F., and Olsen, S. (1965). Test of an ascorbic acid method for determining phosphorus in water and NaHCO3 extracts from soil. Soil Sci. Soc. Am. J. 29, 677–678. doi: 10.2136/sssaj1965.03615995002900060025x
Williams, V., and Twine, S. (1960). “Flame photometric method for sodium, potassium and calcium,” in Modern Methods of Plant Analysis, Vol. 5, eds K. Peach and M. V. Tracey, (Berlin: Springer-Verlag), 3–5.
Zhang, W., Xie, Z., Wang, L., Li, M., Lang, D., and Zhang, X. (2017). Silicon alleviates salt and drought stress of Glycyrrhiza uralensis seedling by altering antioxidant metabolism and osmotic adjustment. J. Plant Res. 130, 611–624. doi: 10.1007/s10265-017-0927-3
Zhishen, J., Mengcheng, T., and Jianming, W. (1999). The determination of flavonoid contents in mulberry and their scavenging effects on superoxide radicals. Food chem. 64, 555–559. doi: 10.1016/S0308-8146(98)00102-2
Keywords: antioxidants, crop water productivity, irrigation regimes, Mediterranean region, nano-SiO2, principal components analysis, yield contributing traits
Citation: Desoky E-SM, Mansour E, El-Sobky E-SEA, Abdul-Hamid MI, Taha TF, Elakkad HA, Arnaout SMAI, Eid RSM, El-Tarabily KA and Yasin MAT (2021) Physio-Biochemical and Agronomic Responses of Faba Beans to Exogenously Applied Nano-Silicon Under Drought Stress Conditions. Front. Plant Sci. 12:637783. doi: 10.3389/fpls.2021.637783
Received: 01 February 2021; Accepted: 11 August 2021;
Published: 16 September 2021.
Edited by:
Jose C. Jimenez-Lopez, Estación Experimental de Zaidín, Consejo Superior de Investigaciones Científicas (CSIC), SpainReviewed by:
Veronica De Micco, University of Naples Federico II, ItalyCopyright © 2021 Desoky, Mansour, El-Sobky, Abdul-Hamid, Taha, Elakkad, Arnaout, Eid, El-Tarabily and Yasin. This is an open-access article distributed under the terms of the Creative Commons Attribution License (CC BY). The use, distribution or reproduction in other forums is permitted, provided the original author(s) and the copyright owner(s) are credited and that the original publication in this journal is cited, in accordance with accepted academic practice. No use, distribution or reproduction is permitted which does not comply with these terms.
*Correspondence: Khaled A. El-Tarabily, a3RhcmFiaWx5QHVhZXUuYWMuYWU=
Disclaimer: All claims expressed in this article are solely those of the authors and do not necessarily represent those of their affiliated organizations, or those of the publisher, the editors and the reviewers. Any product that may be evaluated in this article or claim that may be made by its manufacturer is not guaranteed or endorsed by the publisher.
Research integrity at Frontiers
Learn more about the work of our research integrity team to safeguard the quality of each article we publish.