- 1College of Plant Protection, Hebei Agricultural University/Technological Innovation Center for Biological Control of Crop Diseases and Insect Pests of Hebei Province, Baoding, China
- 2Graduate School of Chinese Academy of Agricultural Sciences, Beijing, China
NAC transcription factors are one of the largest transcription factor families having functions in a variety of stress responses. Few NACs have been reported for interactions between wheat and the wheat rust fungus Puccinia triticina (Pt). In this study, based on analysis of RNA-seq data from wheat line TcLr19 inoculated by Pt, the NAC transcription factor TaNAC069 was cloned from wheat, and its transcriptional activity and homologous dimer formation were verified. Quantitative real-time PCR analysis showed that the expression of TaNAC069 was induced by Pt and associated signaling molecules. To further characterize the function of the TaNAC069 gene in wheat resistance to Pt, virus-induced gene silencing (VIGS) was utilized, and it revealed that Pt resistance in TaNAC069-silenced plants was significantly reduced. Potential interaction targets of TaNAC069 from wheat and Pt were screened and identified by yeast two-hybrid technology. Eukaryotic elongation factor eEF1A, CBSX3 protein, and cold acclimation protein WCOR410c were screened by yeast one-hybrid technology. The results indicate that the TaNAC069 gene plays a positive regulatory role in wheat resistance to Pt, laying a good foundation to analyze the molecular mechanisms of TaNAC069 and its functional role in wheat resistance to Pt.
Introduction
At the transcriptional level, controlling gene expression is key to regulate many plant cell responses, including normal signal transduction and cell morphological changes in response to stress (Hu et al., 2010). Many studies have shown that transcription factors regulate the expression of stress-related genes to activate or repress the expression of target genes involved in stress responses (Erpen et al., 2018). NAC transcription factors are one of the largest families of plant-specific transcription factors that respond to biotic and abiotic stresses by participating in plant stress signal transduction pathways and regulating the expression of downstream target genes (Zhang et al., 2018). A common feature of NAC transcription factor family members is that they contain a conserved NAC domain in the N-terminus, which is usually divided into five subdomains—A, B, C, D, and E—as well as a highly variable regulatory domain in the C-terminus (Shao et al., 2015). The C-terminus of NAC is rich in serine, threonine, proline, and some acidic amino acids and can activate or repress the expression of downstream target genes (Olsen et al., 2005). Additionally, the C-terminus may have transcriptional activity, containing different motifs having specific functions.
NAC transcription factors generally function by forming homodimers or heterodimers of NAC proteins. Different NAC proteins have different biological functions (Ooka et al., 2003). Many reports have demonstrated that NAC transcription factors play important roles in enhancing drought and salt resistance (Nguyen et al., 2019), improving tolerance to dehydration and high salt stress (Nakashima et al., 2007), enhancing tolerance to Erysiphe cichoracearum and Phytophthora parasitica var. nicotianae (Zhu et al., 2012), and regulating plant defense responses against fungal and bacterial pathogens (Wang et al., 2007).
Many studies have shown that NAC proteins play significant roles in different signaling pathways. NAC proteins respond to biotic and abiotic stresses through regulation of downstream gene expression or through interaction with other proteins (Wu et al., 2018). GhirNAC2 plays a positive role in improving drought tolerance in cotton by regulating abscisic acid (ABA) biosynthesis (Shang et al., 2020). The NAC transcription factor CrNAC036 cooperates with CrMYB68 transcription factors to repress ABA biosynthesis by negatively regulating the expression of the CrNCED5 (9-cis-epoxycarotenoid dioxygenase 5) gene in citrus fruit (Zhu et al., 2020). TaNACL-D1 (Triticum aestivum NAC-like transcription factor) can interact with TaFROG (T. aestivum fusarium resistance orphan gene) and positively enhances wheat resistance to Fusarium head blight disease (Perochon et al., 2019). The expression levels of the TaNAC4 and TaNAC8 genes were significantly up-regulated during incompatible interactions between wheat and Pst (Puccinia striiformis f. sp. tritici) (Xia et al., 2010).
Wheat leaf rust is a fungal disease caused by the obligate, biotrophic plant pathogen Puccinia triticina (Pt), which cannot grow without living hosts. It is one of the most widespread and destructive diseases of wheat worldwide, causing significant yield losses in major wheat-growing regions (Kolmer, 1996). Frequent variations in virulence genes of Pt yield new virulent species that can evade detection by wheat resistance genes, leading to the loss of rust-resistant wheat cultivars (Kolmer et al., 2020), which is likely to result in the outbreak of leaf rust. Thus, the excavation, screening, and identification of wheat resistance genes is crucial to understanding the molecular mechanisms underlying resistance against the wheat leaf rust pathogen. Wheat leaf rust resistance gene, Lr19, originally identified in Agropyron elongatum, on the 7DL chromosome, provides effective resistance to most pathotypes of Pt throughout the growth period of wheat (Sarma and Knott, 1966; Ordoñez and Kolmer, 2008). Recently, 453 NAC proteins in polyploid wheat were identified (Borrill et al., 2017). However, the specific functions and characteristics of NAC transcription factors are unknown, especially those involved in wheat resistance against Pt. In our previous study, based on the analysis of RNA-seq data of wheat, TcLr19, a NAC transcription factor induced by Pt (Traes_5DL_D1D0CA79E), was screened by combining differential gene expression and functional annotation (Zhang et al., 2020). In this study, we cloned the NAC transcription factor, aimed to identify its function in wheat resistance to Pt using barley stripe mosaic virus (BSMV)-induced gene silencing, and screened for interacting protein targets by yeast two-hybrid and yeast one-hybrid technologies, laying a good foundation to analyze the molecular mechanism of this NAC transcription factor underlying wheat resistance to Pt.
Materials and Methods
Plant Materials and Inoculation System
The near-isogenic lines TcLr19, Thatcher, Jinhe, and Pt race 07-10-426-1 (PHNT) were used for gene expression and functional analyses. TcLr19, which expresses a typical hypersensitive response (HR) (scale 0), and Thatcher, which is susceptible to PHNT (scale 4), were inoculated with Pt race PHNT according to Roelfs’ standard (Roelfs et al., 1984). Leaf, stem, and root samples were taken from PHNT and mock inoculated (with sterile distilled water) wheat plants at 0, 6, 12, 24, 48, 72, 96, 144, and 168 h post-inoculation (hpi) for total RNA extraction. Then, 0.1 mmol/L ABA, salicylic acid (SA), ethephon (ETH), and methyl jasmonate (MeJA) were sprayed on the surface of leaves of 7-day-old wheat seedlings at the primary leaf stage, respectively. Wheat leaf samples were harvested at 0, 24, 48, 72, 96, and 120 h post-treatment (hpt) for total RNA extraction.
Cloning of the TaNAC069 Gene and TaNAC069 Promoter and Bioinformatics Analysis
A pair of gene-specific primers, TaNAC069-F and TaNAC069-R (Supplementary Table 1), was designed based on conserved regions of the NAC sequence mapping in the RNA-seq data. A mixture of RNA isolated at different time points from wheat leaves infected by Pt was used as the template in reverse transcription polymerase chain reaction (RT-PCR) to obtain fragments of TaNAC069. The conserved domains of TaNAC069 gene were deduced using NCBI (http://www.ncbi.nlm.nih.gov/Structure/cdd/wrpsb.cgi). CLUSTALW was used to create multiple alignments. MEGA5 (http://www.megasoftware.net) was used to generate phylogenetic trees.
Searching sequences upstream of TaNAC069 genes in the wheat database (http://plants.ensembl.org/Taestivum/Info/ Index), an approximately 2.0-kb sequence of the homologous sequence of TaNAC069 on 5D was intercepted from each gene and used to design primers pTaNAC069-F and pTaNAC069-R (Supplementary Table 1). Genomic DNA of TcLr19 was used as template, and RT-PCR was performed to generate the promoter of the TaNAC069 gene. The cis-acting elements of the promoter were analyzed using the PlantCARE (http://bioinformatics.psb.ugent.be/ webtools/plantcare/html/) and PLACE (http://www. dna.affrc.go.jp/PLACE/index.html) databases (Sun et al., 2015).
Expression Analysis of TaNAC069 by qPCR
The specific primers qTaNAC069-F and qTaNAC069-R were used to investigate the expression level of TaNAC069. Wheat glyceraldehyde-3-phosphate dehydrogenase (GAPDH, GenBank: AF251217) was used as an endogenous control as previously described by Gao et al. (2015). Quantitative real-time PCR (qPCR) was performed using TransStart® Top Green qPCR SuperMix (TransGen Biotech, China) according to the manufacturer’s instructions. All qPCR experiments were performed on a LightCycler® 96 Real-Time PCR System (Roche, Switzerland), and relative gene expression was calculated using the 2–ΔΔCt method. Three independent biological replicates were performed per treatment.
Functional Analysis via Virus-Induced Gene Silencing Experiments
Target fragments for silencing (V1 and V2) that had no or low similarity with other genes and thus unlikely to cause off-target effects were selected based on predictions made using si-Fi software (http://www.snowformatics.com/si-fi.html). The plasmids (γ-V1 and γ-V2) utilized for gene silencing were constructed according to the methods described by Holzberg et al. (2002). Plasmids α, β, γ, γ-PDS, γ-V1, and γ-V2 were each linearized. The linearized plasmids were used as templates for in vitro transcription using the mMESSAGE mMACHINE® Kit High Yield Capped RNA Transcription Kit (Ambion) according to the manufacturer’s protocol. BSMV VIGS vectors harboring target gene sequences and Pt urediospores were co-inoculated into wheat cultivars TcLr19 and Thatcher according to previously described methods (Wang et al., 2020). Plants mock inoculated with sterile water and plants inoculated with a VIGS vector targeting the wheat phytoene desaturase (TaPDS) gene were used as negative and positive controls, respectively.
Detection of Hydrogen Peroxide Accumulation, Enzyme Activity, and Expression of the Marker Genes
H2O2 accumulation was detected using 3,3-diaminobenzidine (DAB; Coolaber, China) staining as described by Thordal-Christensen et al. (1997) and observed with a Nikon Ti2-LAPP Ti2 Laser Application System (Nikon Corporation, Japan). A minimum of 50 infection sites were examined for every treatment. Superoxide dismutase (SOD) and catalase (CAT) enzyme activities were detected using Total Superoxide Dismutase Assay Kit with NBT (Leagene) and Catalase Assay Kit with Ultraviolet Colorimetry (Leagene), respectively, according to the manufacturers protocol. In addition, the expression levels of marker genes, TaPR1 (GenBank: HQ848391), TaPR2 (GenBank: HQ848392), TaTLP1 (GenBank: KJ764822), TaSOD (GenBank: CB307850), and TaCAT (GenBank: X94352), were analyzed by qPCR in silenced plants. Each treatment included three independent biological replicates.
Identification of Transcriptional Activity
To confirm the presence of an activation domain of TaNAC069, a yeast assay system was used as described previously (Fujita et al., 2010). The recombinant plasmids pBD-TaNAC069, pBD-TaNAC069-N (1–417 bp), pBD-TaNAC069-C (418–1065 bp), and pGBKT7 (negative control) were each transformed into yeast strain AH109. The transformed yeast cells were individually streaked on selective dropout/-tryptophan (SD/-Trp) medium and selective dropout/-tryptophan/-histidine/-adenine with X-α-Gal (SD/-Trp/-His/-Ade/X) medium and then incubated at 30°C for 3 days. The recombinant vectors pBD-TaNAC069-N and pAD-TaNAC069 were constructed and co-transformed into yeast strain Y2HGold to verify the self-interaction of TaNAC069. Each yeast strain was incubated on SD/-Trp and SD medium in the absence of tryptophan, leucine, histidine, and adenine with X-α-Gal (SD/Trp-/Leu-/His-/Ade-/X), respectively.
For GUS staining, the TaNAC069 promoter fragment was inserted into the pBI121 vector digested with HindIII and XbaI, using the primer pair pBI121-pTaNAC069-F/pBI121-pTaNAC069-R (Supplementary Table 1). The recombinant plasmid, pBI121-pTaNAC069, was transformed into Agrobacterium tumefaciens strain GV3101 by electroporation and then agroinfiltrated into Nicotiana benthamiana plants (grown for 45 days) (Yang et al., 2019). At 72 hpi, inoculated leaves were collected and immediately treated with GUS staining solution. Under the naked eye or microscope, the small blue dots on the white background indicate GUS expression sites.
To generate the GFP constructs, the TaNAC069 promoter fragment was recombined into the vector pCamA-GFP digested with HindIII and KpnI, using the primer pair pCamA-pTaNAC069-F/pCamA-pTaNAC069-R (Supplementary Table 1). The recombinant plasmid pCamA-pTaNAC069-GFP was used for Agrobacterium-mediated transformation.
Yeast One-Hybrid and Yeast Two-Hybrid Assays
For yeast one-hybrid assays, the recombinant yeast strain Y1HGold (pAbAi-pTaNAC069) was identified via PCR using Matchmaker Insert Check PCR Mix 1 (Clontech, Palo Alto, CA, United States) as described by Yang et al. (2019). Yeast library plasmids (10 μg) were transformed into the recombinant yeast strain Y1HGold (pAbAi-pTaNAC069). Healthy single clones were selected for colony PCR using Matchmaker Insert Check PCR Mix 2 (Clontech, Palo Alto, CA, United States) to obtain the inserted fragment of the cDNA library. The amplified products (1–2 kb) were purified by agarose gel electrophoresis and sequenced at Sangon Biotech, Shanghai, China, using the T7 promoter sequence as primer. The sequencing results were analyzed using NCBI (http://blast.ncbi.nlm.nih.gov/Blast.cgi).
For yeast two-hybrid assays, the bait vector pGBKT7-TaNAC069-N was transformed into Y2HGold yeast strain using the LiAc (Clontech, Palo Alto, CA, United States) and confirmed by toxicity and self-activation. Screening of interacting targets of TaNAC069 was done using the Matchmaker Gold Yeast Two-Hybrid (Y2H) System (Clontech, Palo Alto, CA, United States). Positive colonies were selected for colony PCR using Matchmaker Insert Check PCR Mix 2 (Clontech, Palo Alto, CA, United States) and sequenced at Sangon Biotech, Shanghai, China, using the T7 primer.
Data Availability Statement
The data presented in this study can be found in online repositories. The data have been deposited in NCBI. The SRA accession number is SRR13254555. The TSA accession number is GIXT00000000.
Results
TaNAC069 Was Obtained Based on RNA-Seq Data
A 1259-bp fragment was identified and cloned based on RNA-seq data via RT-PCR (Supplementary Figure 1); sequence analysis showed that it belongs to the NAC transcription factor family, as expected. The open reading frame (ORF) finder showed that the corresponding gene comprises a 1065-bp complete ORF encoding 354 amino acids, including the N-terminal 127 (13–139) amino acid residue NAM domain and the C-terminal highly variable transcriptional regulatory region (Figure 1A). A BLASTp search showed that the amino acid sequence was highly homologous with published sequences of wheat (T. aestivum) TaNAC69 (AAU08785.1), with homology of 98.87%; it is named TaNAC069. BLAST programs in the Chinese spring wheat database (http://plants.ensembl.org/index.html) show that TaNAC069 is highly similar to its homologs located on chromosomes 5D (TraesCS5D02G148800.1) and 5B (TraesCS5B02G142100.1). Further analysis confirmed its homology with typical NAC transcription factors, such as Arabidopsis thaliana ATAF1 (X74755) (36.6%), A. thaliana TIP (AF281062) (46.91%), Oryza sativa OsNAC3 (AB028182) (54.50%), Petunia hybrida NAM (X92204) (54.49%), NAC-like NAP (AJ222713) (56%), and Lycopersicon esculentum SENU5 (Z75524) (54.22%) (Figure 1B). TaNAC069 contains all A, B, C, D, and E conserved subdomains, which were confirmed by alignment with typical NAC transcription factors representing different NAC phylogenetic subgroups (Ooka et al., 2003). It was found to belong to the NAP subfamily via phylogenetic analysis of TaNAC069 (Figures 1B,C).
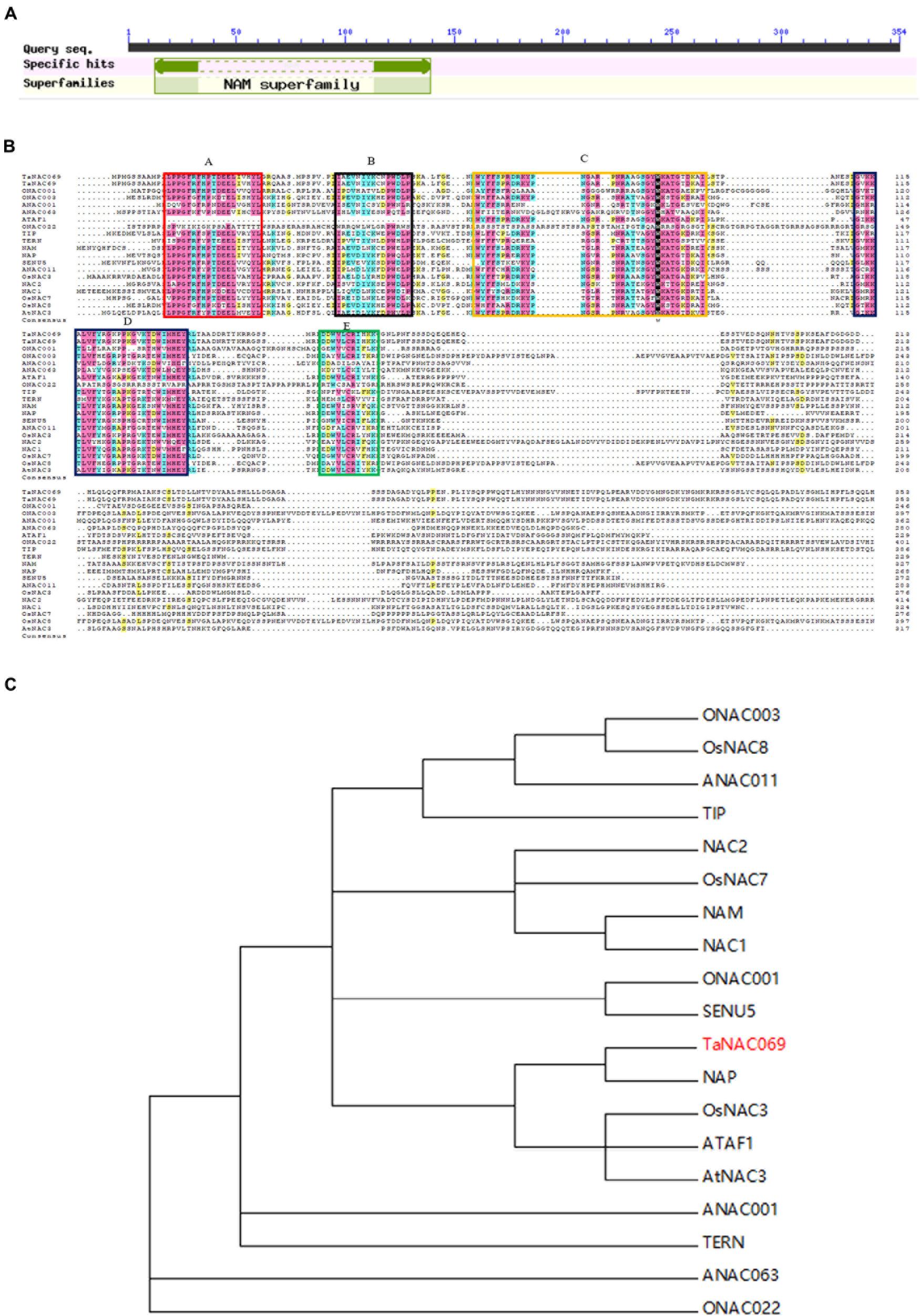
Figure 1. Analysis of TaNAC069 gene. (A) Structural domains of TaNAC069. (B) Multiple alignments of TaNAC069 and homologous genes. (C) Phylogenetic analysis of NACs. NAC subdomains (A–E) are conserved and underlined. Identical and similar residues are shaded in different colors, respectively. The unrooted phylogenetic tree of NAC domains was depicted using the CLUSTAL X program and was constructed using the neighbor-joining method. ANAC001, Arabidopsis thaliana NAC001 (AT1G01010); NAC1, A. thaliana NAC1 (AF198054); NAC2, A. thaliana NAC2 highly expressed in root meristem (AF201456); AtNAC3, A. thaliana NAC3 (AB049070); ANAC011, A. thaliana NAC011 (AT1G32510); ANAC063, A. thaliana NAC063 (AT3G55210); ATAF1, Arabidopsis transcription activation factor 1 (X74755); TIP, A. thaliana TIP interacts with turnip crinkle virus capsid protein (AF281062); ONAC001, Oryza sativa NAC001 (AK060509); ONAC003, O. sativa NAC003 (AK061716); OsNAC3, O. sativa NAC3 (AB028182); OsNAC7, O. sativa NAC7 (AB028186); OsNAC8, O. sativa NAC8 (AB028187); ONAC022, O. sativa NAC022 (AK107090); TERN, tobacco elicitor-responsive (AB021178); NAM, Petunia hybrida no apical meristem (X92204); NAP, NAC-like, activated by AP3/PI (AJ222713); SENU5, Lycopersicon esculentum SENU5 (Z75524).
TaNAC069 Expression Was Significantly Induced in TcLr19 Plants Upon Pt Infection
qPCR was used to investigate the expression of TaNAC069 in response to Pt infection. The expression level of TaNAC069 during incompatible interactions increased from 0 to 24 hpi, peaking at 48 hpi (about 23.8 times higher than the 0 hpi control), and then decreased at 72 hpi. A second expression peak observed at 96 hpi was lower than the first but 20.1 times higher than the control (Figure 2A). During compatible interactions, TaNAC069 expression was up-regulated compared with the non-inoculated control at most time points (Figure 2A). It exhibited expression peaks at 48 hpi (8.9 times higher than the control) and 168 hpi (7.3 times higher than the control) and then declined. Overall, the expression level of TaNAC069 was higher during incompatible interactions than compatible ones at most time points, indicating that TaNAC069 expression was induced by Pt infection.
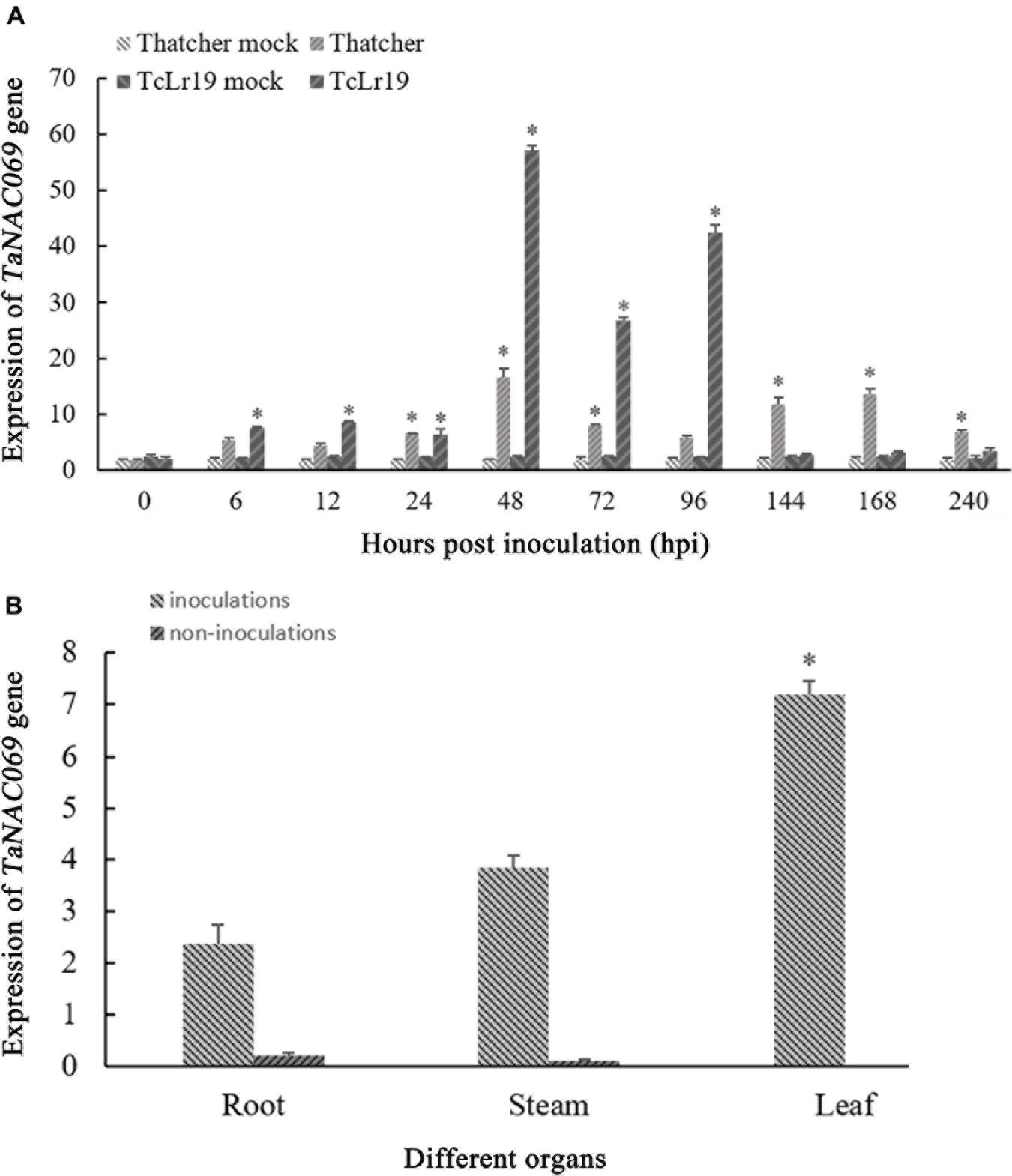
Figure 2. Analysis of TaNAC069 gene expression. (A) Expression profiles of the TaNAC069 gene in wheat infected with Pt. (B) Expression profile of the TaNAC069 gene in different organs. The y-axis indicates the amounts of gene transcripts normalized to the GAPDH gene and expressed relative to that of mock-inoculated control plants treated with 0.1% (v/v) ethanol solution. The x-axis indicates different sampling. The reference gene is GAPDH. The assays for each treatment consisted of three biological replicates. Data are means ± standard errors of three independent experiments. Differences between time-course points were assessed using SSPS. ∗p < 0.05, n = 3.
Additionally, the expression level of TaNAC069 was low in non-inoculated roots and stems and exhibited almost no expression in wheat leaves. After inoculation with Pt race PHNT, higher expression levels of TaNAC069 were observed in leaves and stems than in roots, with the expression in leaves being 3.0 times higher than in roots and 1.9 times than in stems (Figure 2B). TaNAC069 expression showed a significant variation in different plant tissues before and after inoculation with PHNT, indicating that expression is tissue specific and induced by the leaf rust pathogen.
Induction of TaNAC069 by Chemical Reagents
To better understand the signal pathways of TaNAC069, the expression profiles of TaNAC069 induced by ABA, SA, ETH, and MeJA were analyzed. The expression level of TaNAC069 increased from 0 to 6 h after ABA treatment and was markedly high at 6 hpt, being nearly 4.7 times higher than that of the control, followed by a slight decrease. During SA treatment, the TaNAC069 expression levels peaked at 48 hpt, which was nearly 11.2 times higher than those of the control, followed by a sharp decrease from 48 to 96 hpt and a subsequent increase at 96 hpt, and peaked again at 120 hpt. The expression level of TaNAC069 was significantly increased, peaking at 72 hpt, after ETH treatment, which was nearly 10.1 times higher than in the control, then followed by a decrease (Figure 3). MeJA treatment had no obvious effect on TaNAC069 expression. The above-mentioned results show that TaNAC069 expression was induced by ABA and SA, experiencing an earlier induction by ABA together with ETH; however, it was not significantly induced by MeJA.
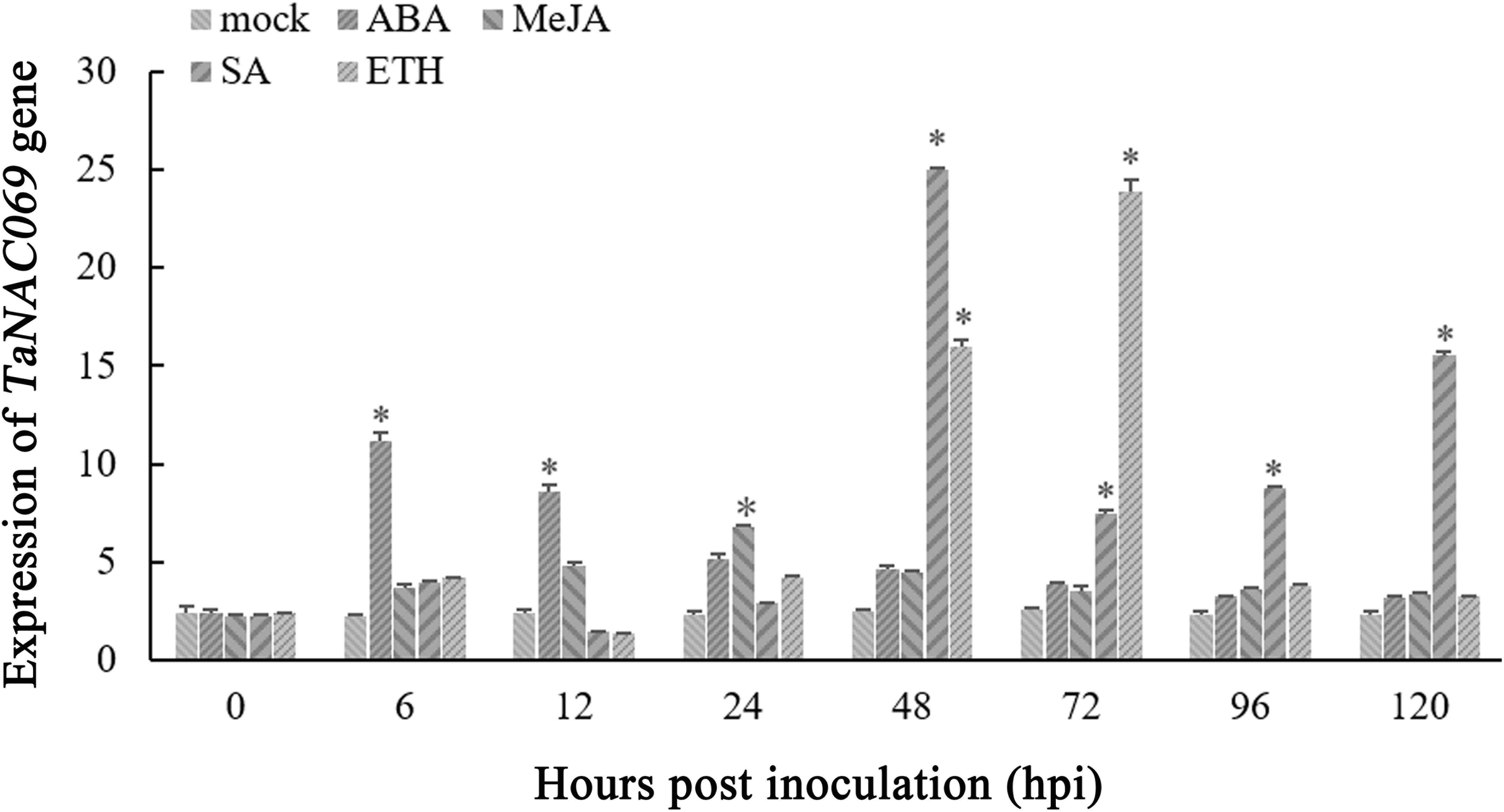
Figure 3. Expression profiles of the TaNAC069 gene in response to different chemical inducers. The y-axis indicates the amounts of gene transcripts normalized to the GAPDH gene and expressed relative to mock-inoculated control plants treated with 0.1% (v/v) ethanol solution. The x-axis indicates different sampling timepoints. The reference gene is GAPDH. The assays for each treatment consisted of three biological replicates. Data are means ± standard errors of three independent experiments. Differences between time-course sampling points were assessed using SSPS. ∗p < 0.05, n = 3.
TaNAC069 Knockdown Decreased the Resistance of Wheat Against Pt
To confirm the function of TaNAC069 in wheat resistance to Pt, the gene was knocked down using the VIGS system. Knockdown of the PDS gene in plants produces a visible photo-bleaching phenotype that can be used as a positive control to confirm the proper functioning of the VIGS system. Mock (buffer inoculated without BSMV) and BSMV:00 (empty vector)-inoculated plants displayed an immune phenotype (scale 0) and no other obvious defects at 14 days post-inoculation with Pt pathotype PHNT compared to Thatcher (scale 4), which indicated that virus inoculation did not affect the wheat–Pt incompatible interaction. BSMV:V1 and BSMV:V2 inoculated with PHNT exhibited more Pt uredinia (scale 3) compared with the mock and BSMV:00 treatments. Thus, knocking down the expression of the TaNAC069 gene reduced the resistance of wheat against PHNT (Figure 4A).
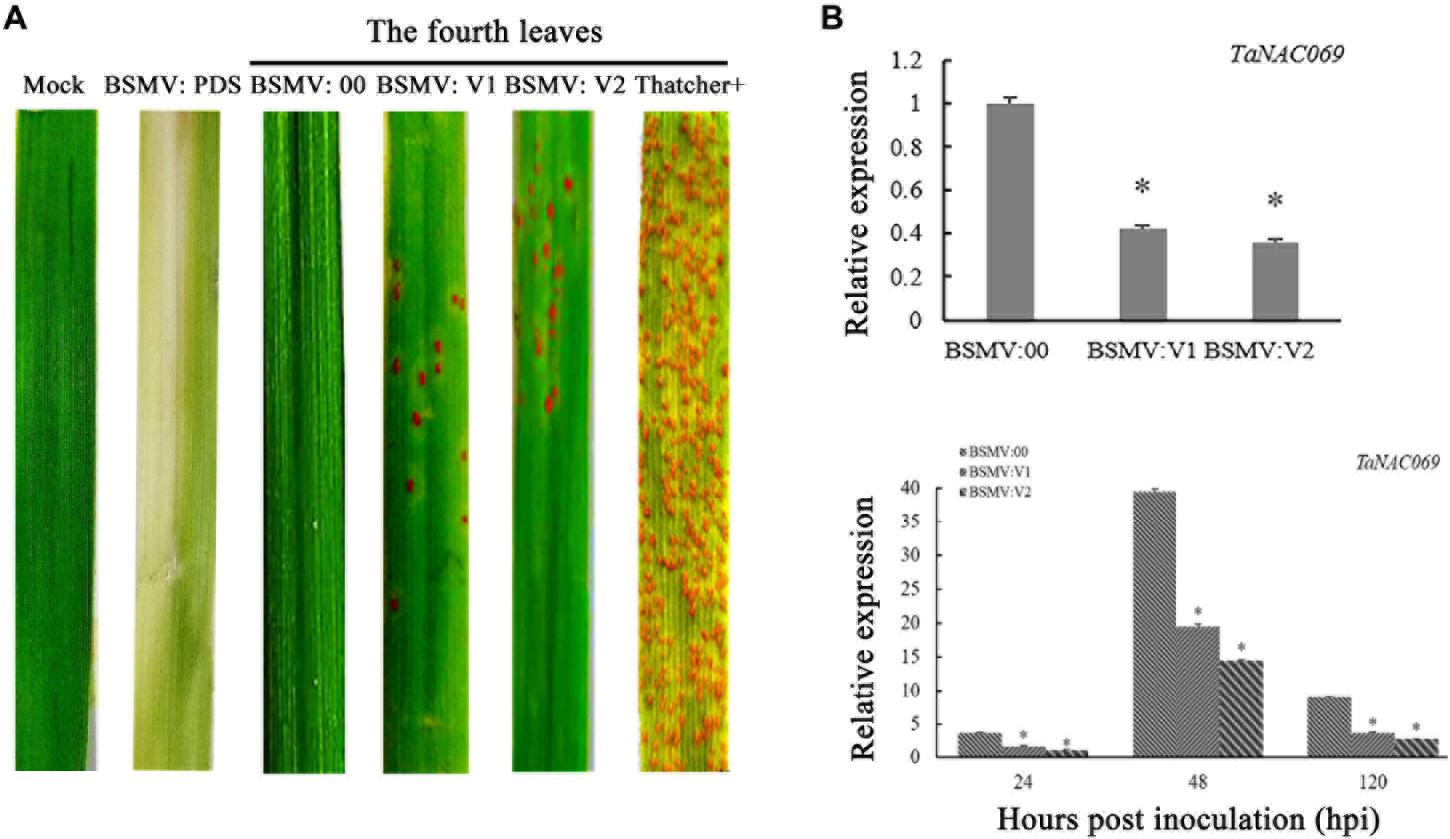
Figure 4. Functional analysis of the TaNAC069 gene in response to leaf rust infection using virus-induced gene silencing. (A) Disease symptoms of TaNAC069 gene-knockdown wheat leaves 14 days after inoculation with Pt pathotype PHNT. (B) Relative expression of wheat TaNAC069 in gene-knockdown wheat leaves after inoculation with PHNT. Mock, TcLr19 wheat leaves without barley stripe mosaic virus (BSMV) and PHNT inoculation; BSMV:00, TcLr19 after BSMV and PHNT inoculation; Tc+, Thatcher after PHNT inoculation. The reference gene is GAPDH. The assays for each treatment and phenotype combination consisted of at least three biological replicates (BSMV:00 was used as the control). Data are means ± standard errors of three independent experiments. Differences between time-course sampling points were assessed using SSPS. ∗p < 0.05, n = 3.
qPCR was used to determine the expression level of TaNAC069 in each treatment. Without inoculation, the expression level of TaNAC069 in BSMV:V1 and BSMV:V2 was reduced significantly, and the silencing efficiency was more than 50% compared to BSMV:00 (Figure 4B). After inoculation with PHNT, compared to BSMV:00, the expression of TaNAC069 was significantly reduced to 56%, 51%, and 60% in BSMV:V1 at 24, 48, and 120 h, respectively, and for the BSMV:V2 treatment expression, it was reduced to 69%, 64%, and 70% at 24, 48, and 120 h, respectively (Figure 4B).
To determine whether TaNAC069 activates or represses the expression of downstream defense-related genes, several pathogenesis-related (PR) genes and reactive oxygen species (ROS)-related genes were selected and analyzed via qPCR. The expression of TaPR1, TaPR2, TaTLP1, and ROS synthesis-related genes (TaNOX) was significantly lower in the TaNAC069-knockdown plants compared with the control, and the expression of ROS clearance-related genes (TaCAT and TaSOD) was significantly higher (Figure 5).
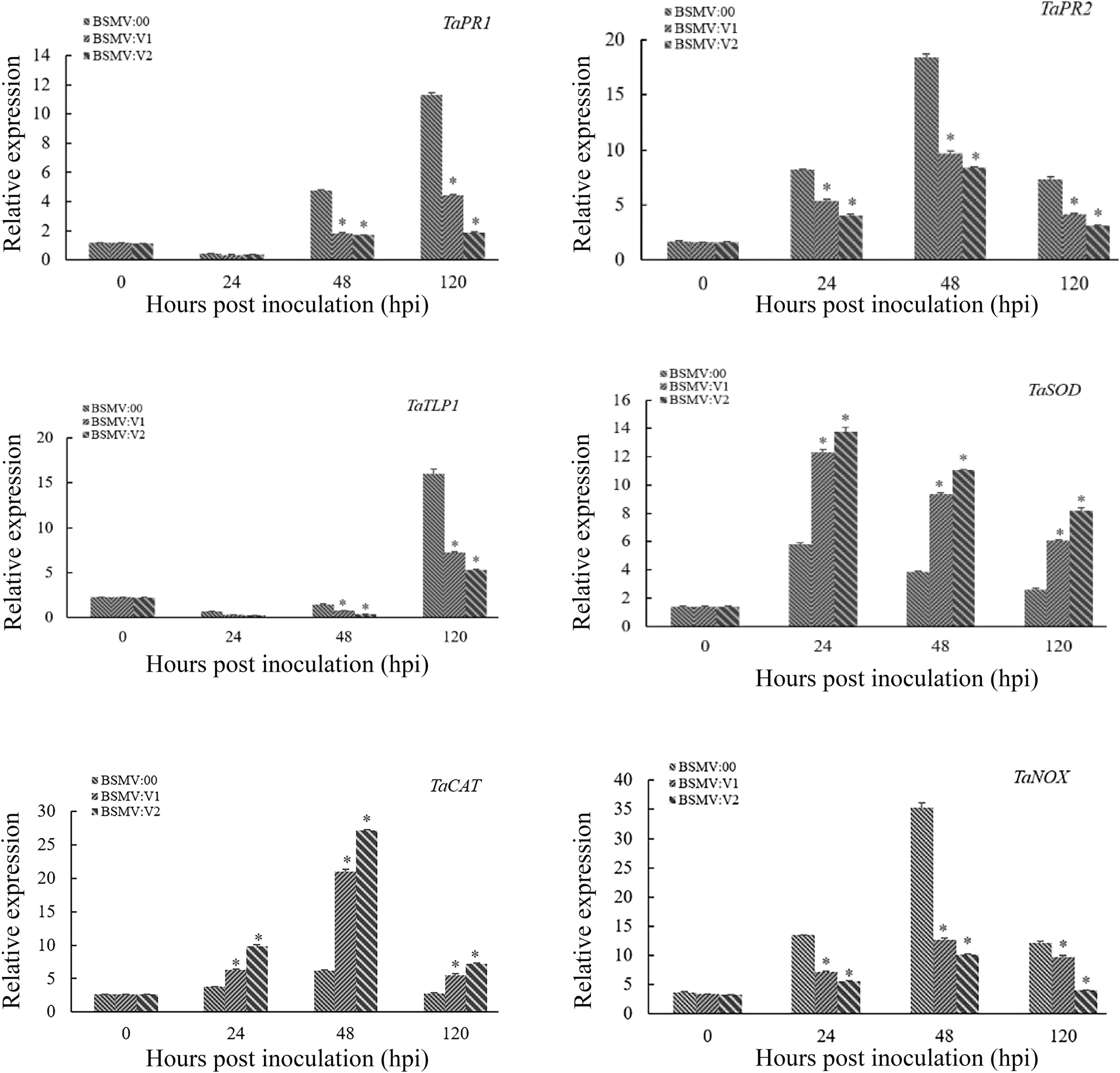
Figure 5. Relative transcript levels of TaPR1, TaPR2, TaTLP1, TaSOD, TaCAT, and TaNOX in TaNAC069-knockdown plants inoculated with PHNT. The y-axis indicates the amounts of gene transcripts normalized to the GAPDH gene and expressed relative to that of mock-inoculated control plants treated with 0.1% (v/v) ethanol solution. The x-axis indicates different sampling timepoints. The reference gene is GAPDH. The assays for each gene consisted of at least three biological replicates. Data are means ± standard errors of three independent experiments. Differences between time-course sampling points were assessed using SSPS. ∗p < 0.05, n = 3.
H2O2 accumulation by DAB staining and related enzyme activity (CAT and SOD) were measured during the wheat–Pt interaction. As shown in Figure 6, H2O2 accumulation was significantly reduced and the activities of SOD and CAT enzymes were significantly increased in TaNAC069-knockdown plants compared with the control. These results indicate that the TaNAC069 gene plays a positive regulatory role in wheat resistance to Pt.
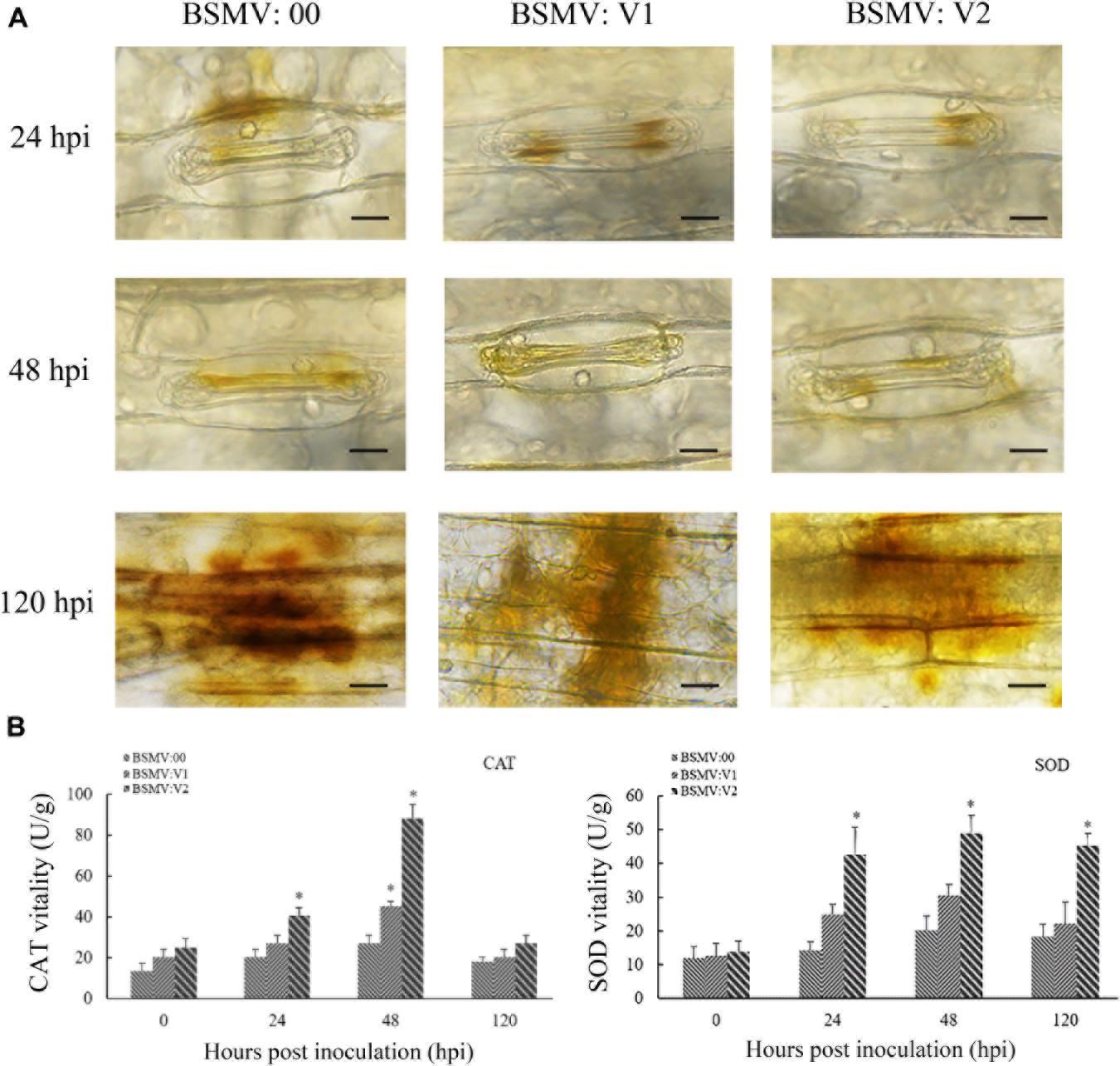
Figure 6. Determination of leaf H2O2 concentration by 3,3-diaminobenzidine (DAB) staining. (A) H2O2 accumulation in TaNAC069-knockdown wheat plants was detected by staining with DAB and viewed under differential interference contrast optics. Scale bar, 50 μm. (B) Superoxide dismutase (SOD) and catalase (CAT) activity in TaNAC069-knockdown wheat plants. The y-axis indicates the amount of SOD and CAT activities (each treatment and phenotype combination consisted of at least three biological replicates). The x-axis indicates different sampling timepoints. Data are means ± standard errors of three independent experiments. Differences between time-course sampling points were assessed using SSPS. ∗p < 0.05, n = 3 (BSMV:00 used as the control).
Identification of TaNAC069 and pTaNAC069 Activity
In order to examine the transcriptional activity and self-interaction of TaNAC069, the yeast-transformed system was used. All transformed yeast strains grew well on SD/-Trp medium, indicating that these vectors were successfully transformed into yeast cells. Yeast cells containing pBD-TaNAC069 or TaNAC069-C grew well and turned blue on SD/-Trp/-His/-Ade/X-α-Gal, whereas cells harboring pGBKT7 or pBD-TaNAC069-N did not (Figure 7A). Yeast cells containing pBD-TaNAC069-N and pAD-TaNAC069 in combination and the positive controls grew well and turned blue on SD/-Trp/-Leu/-His/-Ade/X. In contrast, replacement of either the pBD-TaNAC069-N or the pAD-TaNAC069 with vectors expressing only the AD or BD yeast proteins resulted in no production of blue coloration (Figure 7B). These results suggest that TaNAC069 exhibits transactivation activity, that the C-terminus can be attributed to transcriptional activation, and that TaNAC069 forms NAC protein homomeric dimers which possess unique biological functions.
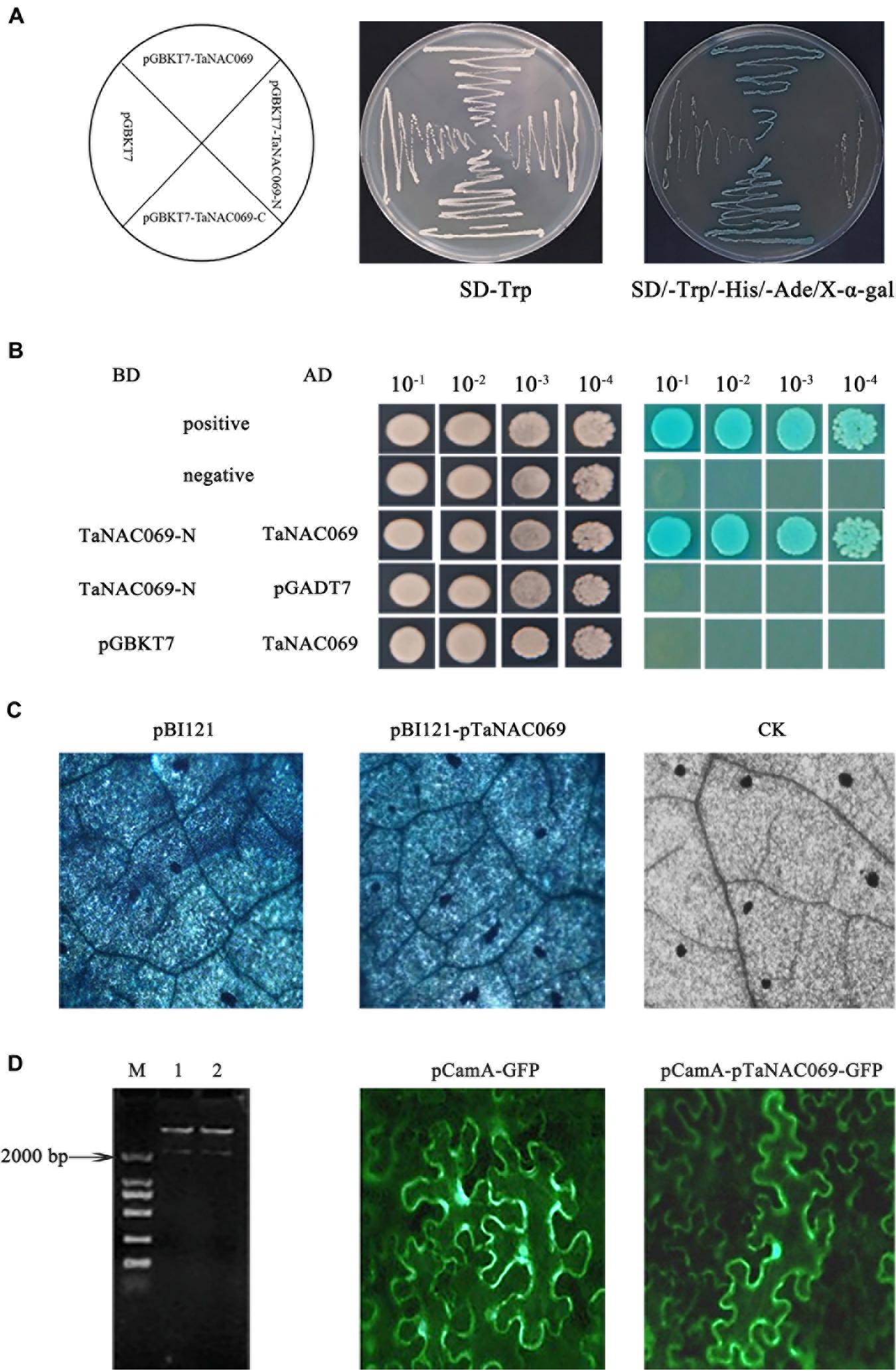
Figure 7. Identified activity of TaNAC069 and pTaNAC069. (A) Transcription activation activity of the TaNAC069. (B) Homocomplex formation of the TaNAC069 in yeast. (C) GUS histochemical staining directed by the pTaNAC069 sequences in tobacco. pBI121, GUS histochemical staining results of tobacco leaves transformed by vector pBI121; pBI121-pTaNAC069, GUS histochemical staining results of tobacco leaves transformed by pBI121-pTaNAC069; CK, GUS histochemical staining results of tobacco. Scale bars are 50 μM. (D) GFP expressed by the pTaNAC069 sequences in tobacco. M, Marker 2000; 1–2, restrictive digestion of the pCamA-pTaNAC069-GFP plasmid; pCamA-GFP, results of tobacco leaves transformed by vector pCamA; pCamA-pTaNAC069-GFP, results of tobacco leaves transformed by pCamA-pTaNAC069-GFP. Scale bars are 50 μM.
To further understand how TaNAC069 is regulated, the promoter with a length of 2,103 bp (upstream of the translational initiation codon ATG) was cloned by RT-PCR (Supplementary Figure 2). The sequence shared 98% identity compared with the wheat genome; the sequence was given the label pTaNAC069. Analysis of the pTaNAC069 sequence using PlantCARE software and PLACE database revealed that two classical promoter cis-acting elements, TATA-box (-1,977, -385, and -201 bp upstream from the transcription start site) and CAAT-box (-1435, -1136, and -878 bp upstream from the transcription start site), were present. cis-Acting motifs of the pTaNAC069 sequence were categorized into five major groups as follows: conserved motifs, abiotic stress, pathogens, phytohormones, and tissue-specific expression-responsive cis-regulatory motifs (Table 1 and Supplementary Figure 3) according to Tiwari et al. (2019). The CaMV35S promoter in the pBI121 vector was replaced by pTaNAC069 using the seamless cloning method to form the recombinant plant expression vector pBI121-pTaNAC069 (Supplementary Figure 4) to verify the promoter activity of pTaNAC069. As shown in Figure 7C, the results of GUS staining revealed a strong blue signal in N. benthamiana leaves injected with pBI121 and pBI121-pTaNAC069 that was not likewise observed for the control (normal leaves). Additionally, the promoter region of pCamA-GFP was replaced by pTaNAC069. As shown in Figure 7, GFP fluorescence was uniformly distributed throughout the cells of leaves injected with pCamA-GFP and pCamA-pTaNAC069-GFP, respectively, indicating the functional promoter activity of pTaNAC069.
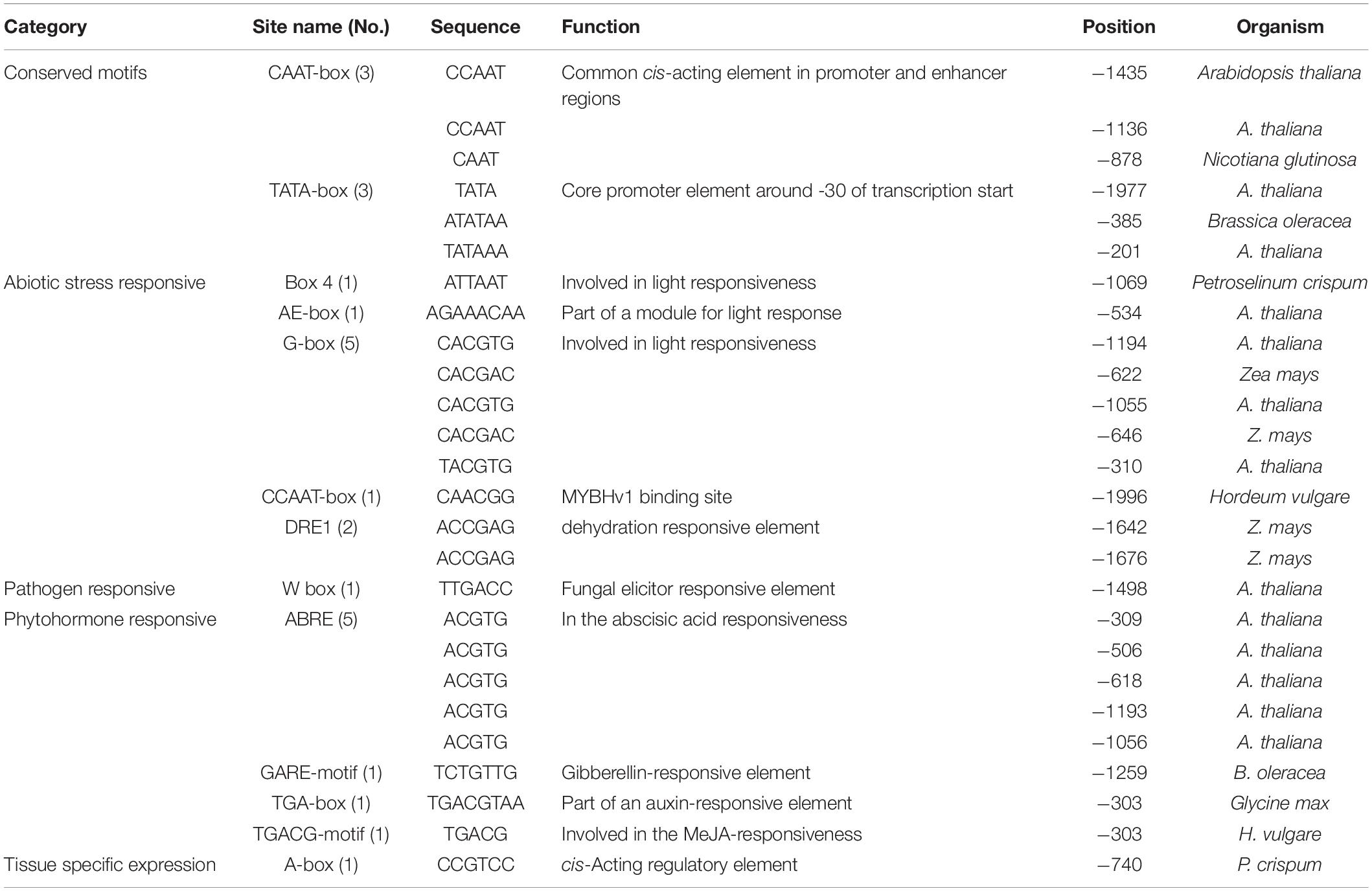
Table 1. Cis-acting elements identified on the -2103 bp (upstream to ATG) putative promoter region of TaNAC069 using PlantCARE and PLACE database.
Upstream Transcription Factors and Interacting Targets of TaNAC069 Were Determined
In order to determine the potential interacting targets of TaNAC069, the bait vector pAbAi-pTaNAC069 was successfully constructed and transformed into Y1Hgold (Supplementary Figures 5A,B). As shown in Supplementary Figure 5C, the minimum concentrations of AbA needed to suppress the basal expression of pTaNAC069 and p53 bait strains were 200 and 100 ng/ml, respectively. In total, 410 positive colonies were identified and analyzed by yeast one-hybrid assays. A total of 45 positive colonies exhibiting PCR products with bright bands and over 1000-bp-long fragments were sequenced by Sangon Biotech, Shanghai, China (Supplementary Figure 5D). Moreover, 11 interaction targets binding to the TaNAC069 promoter region were selected and analyzed, including glutathione peroxidase (AJE63414.1), eukaryotic elongation factor eEF1A (AQU14666.1), CBS domain-containing protein CBSX3 (XP_020173473.1), cold acclimation protein WCOR410c (AAB18202.1), peptidyl-prolyl cis–trans isomerase (4E1Q_A), chlorophyll A–B binding protein (IPR023329), lipid transfer protein precursor (AAK20395.1), GDT1-like protein 5, and unnamed protein product (VAI25758.1) (XP_020177476.1) (Table 2).
The bait vector pGBKT7-TaNAC069-N was successfully constructed and confirmed to have no toxicity or self-activation in the yeast strain Y2HGold (Supplementary Figure 6). In total, 450 positive colonies were identified and analyzed via the bait vector pGBKT7-TaNAC069-N and yeast library mating (Supplementary Figure 6C). A total of 60 positive colonies, exhibiting PCR products with bright bands and over 750–2000-bp-long fragments, were sequenced. The sequencing results were selected and analyzed via BLAST. A total of 55 target protein sequences were identified in wheat (Table 3), including seven papain family cysteine protease (XP_020166016.1), three hypersensitive induced reaction protein 1 (AFD54041.1), two elongation factors (EMS67172.1, AQU14666.1), three drought-induced 19 protein (Di19) (AIU99984.1), seven protein EARLY RESPONSIVE TO DEHYDRATION 15-like (ERD15) (XP_020186168.1), one salicylic acid-binding protein 2-like (XP_020164120.1), one CAT (AIZ77475.1), one E3 ubiquitin-protein ligase UPL6 (XP_020165537.1), one probable indole-3-acetic acid-amido synthetase (XP_020153870.1), and 1,3-1,4-β-D-glucanase-like (XP_020192465.1) in addition to others. Five target protein sequences were identified in Pt (Table 4), including hypothetical protein PTTG_06575, hypothetical protein PTTG_01234, NADH dehydrogenase, asparaginyl-tRNA synthetase, and 5-methyltetra hydropteroyl triglutamate-homocysteine.
Confirmation of TaNAC069 Interaction With TaCP5 by Y2H Assay
Zhang et al. (2019) reported that TaCP3, a cysteine protease family gene and a type of papain protease, plays a role in drought stress, so the papain family cysteine protease was selected for further investigation. BLASTp analysis showed that the papain family cysteine protease, labeled as screened by TaNAC069, shared 100% homology with published sequences (TraesCS5B02G208100.1) and was given the label TaCP5. To test if TaNAC069 can interact with TaCP5, pGADT7-TaCP5 plasmids were co-transformed into the yeast strain Y2HGold and cultured on the selection medium SD/-Trp/-Leu/-His/-Ade and X-α-gal together with construct pBD-TaNAC069-N. The results showed that the yeast transformant co-expressing the pBD-TaNAC069-N and pGADT7-TaCP5 plasmids produced blue coloration (Figure 8A, rows 5) similar to the positive control (Figure 8A, rows 1). In contrast, replacement of either the pBD-TaNAC069-N prey or the pGADT7-TaCP5 bait with vectors expressing either the AD or BD yeast proteins produced no blue coloration (rows 2–4).
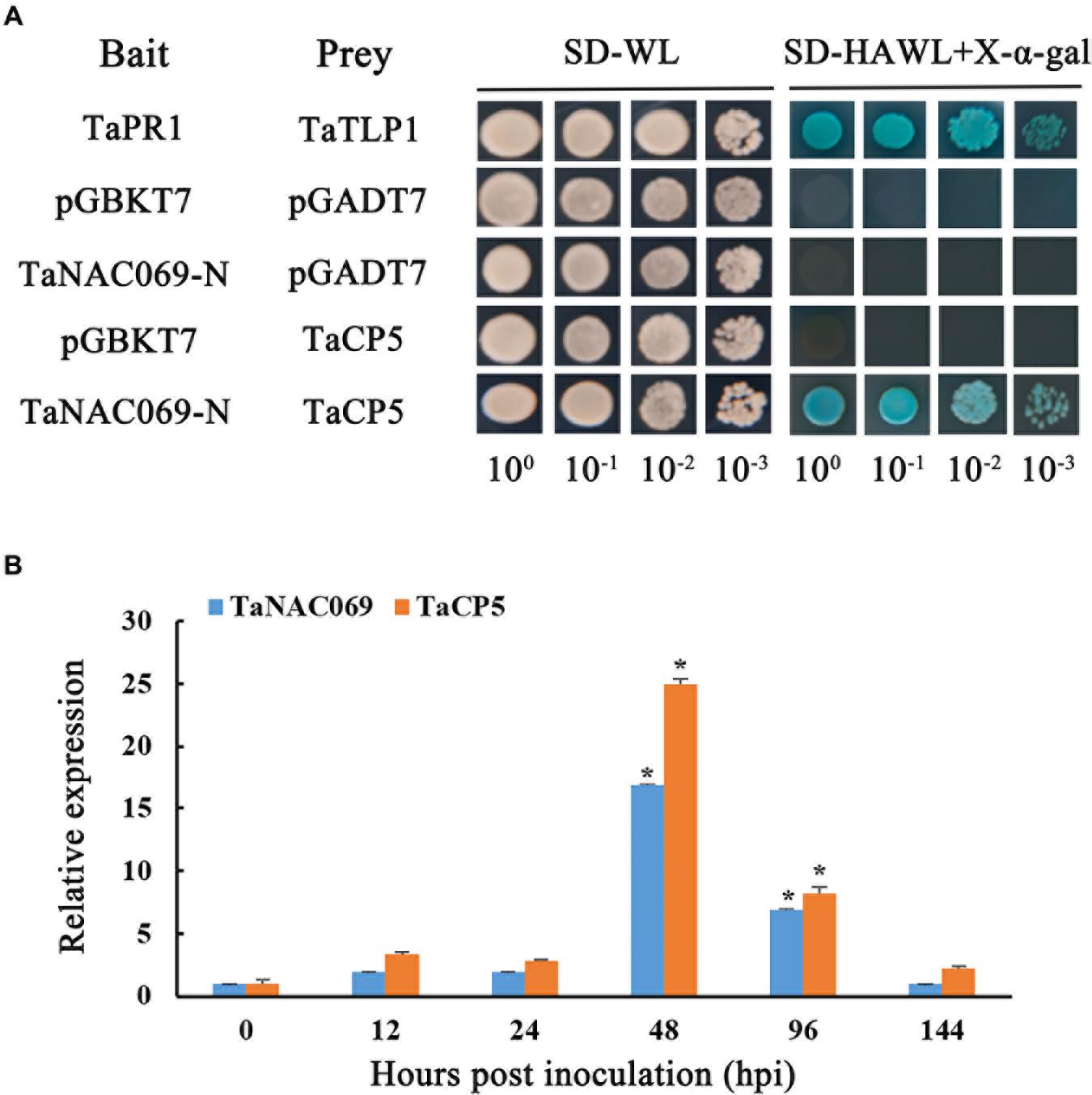
Figure 8. Interaction between TaNAC069 and TaCP5. (A) Yeast two-hybrid analysis of the interaction between TaNAC069 and TaCP5 in yeast. Yeasts expressing the indicated combinations of bait and prey proteins were spotted onto synthetic dropout medium lacking leucine and tryptophan (SD-WL) and SD medium lacking leucine, tryptophan, histidine, and adenine supplemented with X-α-Gal and Aureobasidin A (SD-HAWL + X-α-Gal). Only yeast strains co-expressing TaNAC069 and TaCP5 grew on SD-HAWL plates and developed blue coloration, indicative of an interaction between both proteins. TaPR1 and TaTLP1 are the positive controls; TaNAC069-N and pGADT7, TaCP5 and pGBKT7, and pGADT7 and pGBKT7 were used as negative controls. (B) Gene expression profiles of TaNAC069 and TaCP5 in wheat infected with Pt at different timepoints post-inoculation. The y-axis indicates the amounts of gene transcripts normalized to the GAPDH gene and expressed relative to plants at 0 hpi. The x-axis indicates different sampling timepoints. The reference gene is GAPDH. The assays for each treatment included three biological replicates. Data are means ± standard errors of three independent experiments. Differences between time-course sampling points were assessed using SSPS. *p < 0.05, n = 3.
To further explore their potential molecular functions in regulation relationship between wheat and Pt, the co-expression patterns of TaCP5 were tested in the same manner as TaNAC069. We found that the expression level of the TaNAC069 gene in TcLr19 plants at different sampling time points after inoculation with Pt were similar to TaCP5 (Figure 8B), suggesting that the expression of both TaNAC069 and TaCP5 is induced by leaf rust infection and shares similar expression profiles, supporting the hypothesis that TaNAC069 and TaCP5 are likely to interact with each other.
Discussion
Many studies have shown that NAC transcription factors have a variety of biological functions and play important roles in plant resistance to disease. Xue et al. (2006) isolated 14 TaNAC69 clones which were grouped into three highly homologous TaNAC69 gene categories (TaNAC69-1, TaNAC69-2, TaNAC69-3). TaNAC69 genes are likely to have some homologous genes due to the allopolyploid nature of hexaploid wheat. By analyzing Affymetrix expression data, TaNAC69 was found to be up-regulated in rust-infected wheat leaves and involved in regulating wheat adaptation to drought stress (Xue et al., 2011). The expression of TaNAC4 isolated from wheat (T. aestivum L.) leaves was significantly induced by P. striiformis f. sp. tritici (Pst) (Xia et al., 2010), and the expression of BnNAC56 isolated from oilseed rape (Brassica napus) was rapidly and significantly induced by Sclerotinia sclerotiorum (Chen et al., 2017). In this study, TaNAC069, which is highly homologous to TaNAC69, was cloned and induced by Pt infection. To assess the role of TaNAC069 in wheat–Pt interactions, the TaNAC069 gene was knocked down using a BSMV–VIGS system, which has been successfully used to identify gene function in plants (Yang et al., 2018). The TaNAC069-silenced wheat plants exhibited reduced resistance against Pt, suggesting that TaNAC069 plays a positive role in wheat resistance against Pt.
NAC transcription factors could activate defense-related genes and enhance plant disease resistance against various stress conditions. For example, MaNAC5 interacts with MaWRKY1/2 to regulate the expression of specific PR genes in banana (Musa acuminata) against the fungus Colletotrichum musae (Shan et al., 2016). OsNAC4, a transcription factor in rice, positively regulates the HR (Kaneda et al., 2009). H2O2 accumulation is observed in a variety of defense responses, including those induced by wounding, insect feeding, and pathogen infection (Orozco-Cardenas and Ryan, 1999). H2O2 is a reactive oxygen species, which is relatively stable and participates in the HR. Balazadeh et al. (2010) reported that some NACs are involved in H2O2-mediated signaling in Arabidopsis. TaNAC2 is likely to participate in resistance responses through the generation of H2O2 during wheat–Pst compatible interactions (Zhang et al., 2018). In the present study, we found that the expression of PR genes, ROS-related genes, and H2O2 accumulation were significantly induced in TaNAC069-knockdown plants compared with controls. Based on these results, we believe that TaNAC069 enhances wheat resistance to the leaf rust pathogen through transcriptional regulation of specific downstream disease-related resistance genes.
Yeast one-hybrid assay is widely recognized as a useful technique for detecting physical interactions between DNA and DNA-binding proteins. In this study, several potential transcription factors were successfully screened by yeast one-hybrid assay, including WCOR410C, CBSX3, and eEF1A. WCOR410C is a member of the dehydrin (dehydration) multi-protein family and encodes a peripheral protein that is responsive to numerous abiotic and ABA stresses (Kiani et al., 2020). Dehydration-responsive element-binding proteins are a large family of transcription factors that induce the expression of a large number of functional genes responsible for stress endurance in plants (Agarwal et al., 2006). The dehydration-responsive element (DRE), a cis-acting element of the promoter regions, was identified (Shinozaki and Yamaguchi-Shinozaki, 2007). ABRE (ABA-responsive element) and DRE1 are present in the TaNAC069 gene promoter region. CBSX, a protein having only one pair of CBS domains without any other protein domains, binds to specific signal molecules to regulate the activity of various downstream partner proteins. In rice, OsCBSX3 was identified and up-regulated significantly by the inoculation of Magnaporthe oryzae and exogenous application of SA and MeJA (Mou et al., 2015). The TGACG motif of pTaNAC069 is involved in MeJA responsiveness, and TaNAC069 was induced by SA. Further experiments, such as Y2H and the electrophoretic mobility shift assay, are needed to determine whether WCOR410C is involved in the transcriptional regulation of pTaNAC069, and CBSX3 may regulate the expression of the TaNAC069 gene by binding to MeJA and SA.
Several potential target proteins were screened by yeast two-hybrid assay, including the salicylic acid-binding protein 2-like, papain family cysteine protease, Di19, and eEF1A proteins. SABP2 activates host SA-mediated defense responses to biotic stress (Park et al., 2007). Sweet potato papain-like cysteine protease, SPCP2, is a functional senescence-associated gene (Chen et al., 2010). The expression of Brassica oleracea BoCP4 (papain-like cysteine proteases) and A. thaliana RD19 (a dehydration-responsive cysteine protease) was dehydration responsive and repressed by water and sucrose (Coupe et al., 2003). In our study, TaNAC069 was found to interact with TaCP5 as confirmed by Y2H. Further experiments such as Y2H, bimolecular fluorescence complementation, and co-immunoprecipitation are needed to determine whether these potential target proteins are involved in interactions with TaNAC069.
Taken together, TaNAC069 enhances wheat resistance to Pt by activating PR genes or inhibiting ROS clearance-related genes. Our findings will broaden our understanding of the potentially versatile functions of NACs and provide valuable insight into the molecular mechanisms underlying plant immunity. Further experiments using stable genetic transformation of the TaNAC069 gene for reliable functional characterization are being carried out, and more than 10 T0 lines were obtained to further confirm the function of TaNAC069.
Data Availability Statement
The datasets presented in this study can be found in online repositories. The names of the repository/repositories and accession number(s) can be found below: The data presented in this study can be found in online repositories. The data has deposited in NCBI. The SRA accession number is SRR13254555. The TSA accession number is GIXT00000000.
Author Contributions
HW conceived the research plans. HW and DL supervised the experiments. YZ performed most of the experiments. HG and ZC provided technical assistance to YZ. HW and YZ designed the experiments and analyzed the data. HW conceived the project. YZ wrote the manuscript with contributions of all the authors. HW and DL supervised and complemented the writing. All authors contributed to the article and approved the submitted version.
Funding
This study was funded by Hebei Natural Science Foundation of China (No. C2020204028).
Conflict of Interest
The authors declare that the research was conducted in the absence of any commercial or financial relationships that could be construed as a potential conflict of interest.
Supplementary Material
The Supplementary Material for this article can be found online at: https://www.frontiersin.org/articles/10.3389/fpls.2021.604797/full#supplementary-material
Supplementary Figure 1 | PCR results of the TaNAC069 gene. M, Marker 2000; 1, TaNAC069.
Supplementary Figure 2 | Cloning of the TaNAC069 promoter. M, Marker 2000; 1, TaNAC069 promoter.
Supplementary Figure 3 | Cis-acting element prediction of the TaNAC069 putative promoter. The nucleotides were numbered relative to the transcription start site (+1) by the black bold font. The partial sequence of the TaNAC069 gene is indicated by the black bold font. Other important putative cis-acting elements were identified using PlantCARE and the PLACE database. These cis-acting elements are underlined and presented with different-colored background.
Supplementary Figure 4 | PCR products of the pBI121-pTaNAC069 plasmid. M, Marker 2000; 1–3, PCR products of the pBI121-pTaNAC069 plasmid.
Supplementary Figure 5 | Screening of a yeast one-hybrid library and obtaining upstream transcription factors. (A) Restriction digestion of the bait vector pAbAi-pTaNAC069. M, Marker 5000; 1, pAbAi-pTaNAC069 plasmid; 2, digestion with HindIII and KpnI. (B) Identification of bait–yeast strains by yeast colony PCR. M1, Marker 2000; M2, Marker 5000; 1–2, positive control p53; 3–4, PCR products of the bait vector pAbAi-pTaNAC069 transformed into the yeast genome. (C) The results of positive yeast clones in SD/-Leu/AbA (0–200 ng/ml) solid medium. (D) The partial PCR identifications of positive yeast clones by yeast one-hybrid assays. M3, Marker 2000.
Supplementary Figure 6 | Screening of a yeast two-hybrid library and obtaining interacting targets of TaNAC069. (A) Self-activation detection of the bait. (B) Toxicity of the bait. (C) The partial PCR identifications of positive yeast clones by yeast two-hybrid assays. M, Marker 2000.
Supplementary Figure 7 | Statistics of uredinias. Statistics of uredinia on 2-cm inoculated leaf segments at 14 dpi; six segments were taken from each sample. Data are means ± standard errors of three independent experiments. Different samples were assessed using SSPS. ∗p < 0.05; n = 3.
Supplementary Table 1 | Primers used in this study.
References
Agarwal, P. K., Agarwal, P., Reddy, M. K., and Sopory, S. K. (2006). Role of dreb transcription factors in abiotic and biotic stress tolerance in plants. Plant Cell Rep. 25, 1263–1274. doi: 10.1007/s00299-006-0204-8
Balazadeh, S., Wu, A., and Mueller-Roeber, B. (2010). Salt-triggered expression of the ANAC092-dependent senescence regulon in Arabidopsis thaliana. Plant Signal. Behav. 5, 733–735. doi: 10.4161/psb.5.6.11694
Borrill, P., Harrington, S. A., and Uauy, C. (2017). Genome-wide sequence and expression analysis of the NAC transcription factor family in polyploid wheat. G3 7, 3019–3029. doi: 10.1534/g3.117.043679
Chen, H. J., Su, C. T., Lin, C. H., Huang, G. J., and Lin, Y. H. (2010). Expression of sweet potato cysteine protease SPCP2 altered developmental characteristics and stress responses in transgenic Arabidopsis plants. J. Plant Physiol. 167, 838–847. doi: 10.1016/j.jplph.2010.01.005
Chen, Q., Niu, F., Yan, J., Chen, B., Wu, F., Guo, X., et al. (2017). Oilseed rape NAC56 transcription factor modulates reactive oxygen species accumulation and hypersensitive response-like cell death. Physiol. Plant 160, 209–221. doi: 10.1111/ppl.12545
Coupe, S. A., Sinclair, B. K., Watson, L. M., Heyes, J. A., and Eason, J. R. (2003). Identification of dehydration-responsive cysteine proteases during post-harvest senescence of broccoli florets. J. Exp. Bot. 54, 1045–1056. doi: 10.1093/jxb/erg105
Erpen, L., Devi, H. S., Grosser, J. W., and Dutt, M. (2018). Potential use of the DREB/ERF, MYB, NAC and WRKY transcription factors to improve abiotic and biotic stress in transgenic plants. Plant Cell Tissue Organ Cult. 132, 1–25. doi: 10.1007/s11240-017-1320-6
Fujita, M., Fujita, Y., Maruyama, K., Seki, M., Hiratsu, K., Ohme-Takagi, M., et al. (2010). A dehydration-induced NAC protein, RD26, is involved in a novel ABA-dependent stress-signaling pathway. Plant J. 39, 863–876. doi: 10.1111/j.1365-313X.2004.02171.x
Gao, L., Wang, S., Li, X. Y., Wei, X. J., Zhang, Y. J., Wang, H. Y., et al. (2015). Expression and functional analysis of a pathogenesis-related protein 1 gene, TcLr19PR1, involved in wheat resistance agasinst leaf rust fungus. Plant Mol. Biol. Rep. 33, 797–805. doi: 10.1007/s11105-014-0790-5
Holzberg, S., Brosio, P., Gross, C., and Pogue, G. P. (2002). Barley stripe mosaic virus-induced gene silencing in a monocot plant. Plant J. 30, 315–327. doi: 10.1046/j.1365-313X.2002.01291.x
Hu, R., Qi, G., Kong, Y., Kong, D., Gao, Q., and Zhou, G. (2010). Comprehensive analysis of NAC domain transcription factor gene family in Populus trichocarpa. BMC Plant Biol. 10:145. doi: 10.1186/1471-2229-10-145
Kaneda, T., Taga, Y., Takai, R., Iwano, M., and Che, F. S. (2009). The transcription factor OsNAC4 is a key positive regulator of plant hypersensitive cell death. EMBO J. 28, 926–936. doi: 10.1038/emboj.2009.39
Kiani, I., Naqvi, S. M. S., Sultana, T., and Khan, F. (2020). Characterization of two novel drought responsive genes in wheat. J. Plant Biochem. Biotechnol. 29, 78–85. doi: 10.1007/s13562-019-00514-2
Kolmer, J. A. (1996). Genetics of resistance to wheat leaf rust. Annu. Rev. Phytopathol. 34, 435–455. doi: 10.1146/annurev.phyto.34.1.435
Kolmer, J. A., Herman, A., Ordoñez, M. E., German, S., Morgounov, A., Pretorius, Z., et al. (2020). Endemic and panglobal genetic groups, and divergence of host-associated forms in worldwide collections of the wheat leaf rust fungus Puccinia triticina as determined by genotyping by sequencing. Heredity 124, 397–409. doi: 10.1038/s41437-019-0288-x
Mou, S. L., Shi, L. P., Lin, W., Liu, Y. Y., Shen, L., Guan, D. Y., et al. (2015). Over-expression of rice CBS domain containing protein, OsCBSX3, confers rice resistance to Magnaporthe oryzae inoculation. Int. J. Mol. Sci. 16, 15903–15917. doi: 10.3390/ijms160715903
Nakashima, K., Tran, L. S., Van Nguyen, D., Fujita, M., Maruyama, K., Todaka, D., et al. (2007). Functional analysis of a NAC-type transcription factor OsNAC6 involved in abiotic and biotic stress-responsive gene expression in rice. Plant J. 51, 617–630. doi: 10.1111/j.1365-313X.2007.03168.x
Nguyen, K. H., Mostofa, M. G., Watanabe, Y., Tran, C. D., Rahman, M. M., and Tran, L. S. P. (2019). Overexpression of GmNAC085 enhances drought tolerance in Arabidopsis by regulating glutathione biosynthesis, redox balance and glutathione dependent detoxification of reactive oxygen species and methylglyoxal. Environ. Exp. Bot. 161, 242–254. doi: 10.1016/j.envexpbot.2018.12.021
Olsen, A. N., Ernst, H. A., Leggio, L. L., and Skriver, K. (2005). NAC transcription factors: structurally distinct, functionally diverse. Trends Plant Sci. 10, 79–87. doi: 10.1016/j.tplants.2004.12.010
Ooka, H., Satoh, K., Doi, K., Nagata, T., Otomo, Y., Murakami, K., et al. (2003). Comprehensive analysis of NAC family genes in Oryza sativa and Arabidopsis thaliana. DNA Res. 10, 239–247. doi: 10.1093/dnares/10.6.239
Ordoñez, M. E., and Kolmer, J. A. (2008). Virulence phenotypes of a worldwide wollection of Puccinia triticina from durum wheat. Phytopathology 97, 344–351. doi: 10.1094/PHYTO-97-3-0344
Orozco-Cardenas, M., and Ryan, C. A. (1999). Hydrogen peroxide is generated systemically in plant leaves by wounding and systemin via the octadecanoid pathway. Proc. Natl. Acad. Sci. USA 96, 6553–6557. doi: 10.1073/pnas.96.11.6553
Park, S. W., Kaimoyo, E., Kumar, D., Mosher, S., and Klessig, D. F. (2007). Methyl salicylate is a critical mobile signal for plant systemic acquired resistance. Science 318, 113–116. doi: 10.1126/science.1147113
Perochon, A., Kahla, A., Vranić, M., Jia, J. G., Malla, K. B., and Craze, M. (2019). A wheat NAC interacts with an orphan protein and enhances resistance to fusarium head blight disease. Plant Biotechnol. J. 17, 1892–1904. doi: 10.1111/pbi.13105
Roelfs, A. P., Casper, D. H., and Long, D. L. (1984). Races of Puccinia graminis in the United States and Mexico during 1983. Plant Dis. 68, 902–905. doi: 10.1094/PD-69-902
Sarma, D., and Knott, D. R. (1966). The transfer of leaf rust resistance from agropyron to triticum by irradiation. Can. J. Genet. Cytol. 8, 137–143. doi: 10.1139/g66-018
Shan, W., Chen, J. Y., Kuang, J. F., and Lu, W. J. (2016). Banana fruit NAC transcription factor MaNAC5 cooperates with MaWRKYs to enhance the expression of pathogenesis-related genes against Colletotrichum musae. Mol. Plant Pathol. 17, 330–338. doi: 10.1111/mpp.12281
Shang, X., Yu, Y., Zhu, L., Liu, H., Chai, Q., and Guo, W. (2020). A cotton NAC transcription factor GhirNAC2 plays positive roles in drought tolerance via regulating ABA biosynthesis. Plant Sci. 296:110498. doi: 10.1016/j.plantsci.2020.110498
Shao, H., Wang, H., and Tian, X. (2015). NAC transcription factors in plant multiple abiotic stress responses: progress and prospects. Front. Plant Sci. 6:902. doi: 10.3389/fpls.2015.00902
Shinozaki, K., and Yamaguchi-Shinozaki, K. (2007). Gene networks involved in drought stress response and tolerance. J. Exp. Bot. 58, 221–227. doi: 10.1093/jxb/erl164
Sun, R. Z., Pan, Q. H., Duan, C. Q., and Wang, J. (2015). Light response and potential interacting proteins of a grape flavonoid 3’-hydroxylase gene promoter. Plant Physiol. Biochem. 97, 70–81. doi: 10.1016/j.plaphy.2015.09.016
Thordal-Christensen, H., Zhang, Z., Wei, Y., and Collinge, D. B. (1997). Subcellular localization of H2O2 in plants. H2O2 accumulation in papillae and hypersensitive response during the barley-powdery mildew interaction. Plant J. 11, 1187–1194. doi: 10.1046/j.1365-313X.1997.11061187.x
Tiwari, V., Patel, M. K., and Chaturvedi, A. K. (2019). Cloning and functional characterization of the Na+/H+ antiporter (NHX1) gene promoter from an extreme halophyte Salicornia brachiata. Gene 683, 233–242. doi: 10.1016/j.gene.2018.10.039
Wang, C. F., Huang, L. L., Buchenauer, H., Han, Q. M., Zhang, H. C., and Kang, Z. S. (2007). Histochemical studies on the accumulation of reactive oxygen species (O2- and H2O2) in the incompatible and compatible interaction of wheat-Puccinia striiformis f. sp.tritici. Physiol. Mol. Plant Pathol. 71, 230–239. doi: 10.1016/j.pmpp.2008.02.006
Wang, F., Yuan, S. T., Wu, W. Y., Yang, Y. Q., Cui, Z. C., Wang, H. Y., et al. (2020). TaTLP1 interacts with TaPR1 to contribute to wheat defense responses to leaf rust fungus. PLoS Genet. 16:e1008713. doi: 10.1371/journal.pgen.1008713
Wu, D., Sun, Y., Wang, H., Shi, H., Su, M., Shan, H., et al. (2018). The SlNAC8 gene of the halophyte Suaeda liaotungensis enhances drought and salt stress tolerance in transgenic Arabidopsis thaliana. Gene 662, 10–20. doi: 10.1016/j.gene.2018.04.012
Xia, N., Zhang, G., Liu, X. Y., Deng, L., Cai, G. L., Zhang, Y., et al. (2010). Characterization of a novel wheat NAC transcription factor gene involved in defense response against stripe rust pathogen infection and abiotic stresses. Mol. Biol. Rep. 37, 3703–3712. doi: 10.1007/s11033-010-0023-4
Xue, G. P., Bower, N. I, Mcintyre, C. L., Riding, G. A., Kazan, K., et al. (2006). TaNAC69 from the NAC superfamily of transcription factors is up-regulated by abiotic stresses in wheat and recognises two consensus DNA-binding sequences. Funct. Plant Biol. 33, 43–57. doi: 10.1071/FP05161
Xue, G. P., Way, H. M., Richardson, T., Drenth, J., Joyce, P. A., and McIntyre, C. L. (2011). Overexpression of TaNAC69 leads to enhanced transcript levels of stress up-regulated genes and dehydration tolerance in bread wheat. Mol. Plant 4, 697–712. doi: 10.1093/mp/ssr013
Yang, H., Zhou, Y., Zhang, Y. N., Wang, J., and Shi, H. Z. (2019). Identification of transcription factors of nitrate reductase gene promoters and NRE2 cis-element through yeast one-hybrid screening in Nicotiana tabacum. BMC Plant Biol. 19:145. doi: 10.1186/s12870-019-1724-z
Yang, J., Zhang, T. Y., Liao, Q. S., He, L., and Li, J. (2018). Chinese wheat mosaic virus-induced gene silencing in monocots and dicots at low temperature. Front. Plant Sci. 9:1627. doi: 10.3389/fpls.2018.01627
Zhang, B. H., Gao, X., Yang, M. M., Wang, X. G., He, Q. M., Yao, X. L., et al. (2019). Cloning of TaCP3 gene and its expression in abiotic stress. J. Triticeae Crops 39, 513–519.
Zhang, X. M., Zhang, Q., Pei, C. L., Li, X., Huang, X. L., Chang, C. Y., et al. (2018). TaNAC2 is a negative regulator in the wheat-stripe rust fungus interaction at the early stage. Physiol. Mol. Plant Pathol. 102, 144–153. doi: 10.1016/j.pmpp.2018.02.002
Zhang, Y. J., Yang, Y. Q., Yuan, X. Y., Wang, H. Y., and Liu, D. Q. (2020). Screening and analysis of wheat resistance -related genes to leaf rust based on transcriptome sequencing. J. Hebei Agric. Univer. 43, 11–16.
Zhu, F., Luo, T., Liu, C. Y., Wang, Y., Zheng, L., and Xiao, X. (2020). A NAC transcription factor and its interaction protein hinder abscisic acid biosynthesis by synergistically repressing NCED5 in Citrus reticulata. J. Exp. Bot. 71, 3613–3625. doi: 10.1093/jxb/eraa118
Keywords: RNA-seq, Puccinia triticina, NAC transcription factor, clone, VIGS
Citation: Zhang Y, Geng H, Cui Z, Wang H and Liu D (2021) Functional Analysis of Wheat NAC Transcription Factor, TaNAC069, in Regulating Resistance of Wheat to Leaf Rust Fungus. Front. Plant Sci. 12:604797. doi: 10.3389/fpls.2021.604797
Received: 10 September 2020; Accepted: 11 February 2021;
Published: 15 March 2021.
Edited by:
Guihua Bai, United States Department of Agriculture (USDA), United StatesReviewed by:
Zhiyong Liu, Institute of Genetics and Developmental Biology, Chinese Academy of Sciences, ChinaGraham Robert David McGrann, Science and Advice for Scottish Agriculture (SASA), United Kingdom
Copyright © 2021 Zhang, Geng, Cui, Wang and Liu. This is an open-access article distributed under the terms of the Creative Commons Attribution License (CC BY). The use, distribution or reproduction in other forums is permitted, provided the original author(s) and the copyright owner(s) are credited and that the original publication in this journal is cited, in accordance with accepted academic practice. No use, distribution or reproduction is permitted which does not comply with these terms.
*Correspondence: Haiyan Wang, bmR3YW5naGFpeWFuQDE2My5jb20=; Daqun Liu, MTYxMDA0MTg5MkBxcS5jb20=