- 1College of Horticulture, China Agricultural University, Beijing, China
- 2College of Plant Science and Technology, Beijing University of Agriculture, Beijing, China
The ripening of fleshy fruits is coupled with the degradation of both chlorophyll and cell walls, as well as changes in the metabolism of phenylpropanoids, flavonoids, starch/sucrose, and carotenoids. These processes are controlled by phytohormones and other factors, including abscisic acid (ABA), ethylene, auxin, polyamines, sugar, and reactive oxygen species. The ripening of climacteric fruits is controlled by ethylene and non-climacteric fruit ripening is regulated mainly by ABA. Also, ABA and ethylene may interact in both types of fruit ripening. ABA concentrations in fleshy fruits are regulated in response to developmental and environmental cues and are controlled by the relative rates of ABA biosynthesis and catabolism, the former mainly via 9-cis-epoxycarotenoid dioxygenases (NCEDs) and β-glucosidases and the latter via ABA 8'-hydroxylases (CYP707As) and β-glycosyltransferases. In strawberry fruit ripening, ABA is perceived via at least two receptors, Pyrabactin resistance (PYR)/PYR-like (PYL) and putative abscisic acid receptor (ABAR), which are linked separately to the conserved signaling pathway ABA-FaPYR1-FaABIl-FaSnRK2 and the novel signaling pathway ABA-FaABAR-FaRIPK1-FaABI4. Downstream signaling components include important transcription factors, such as AREB (ABA responsive element binding protein)/ABF (ABRE binding factors ABA responsive factor), ethylene response factor (ERF), and V-myb Myeloblastosis viral oncogene homolog (MYB), as well as ripening-related genes. Finally, a comprehensive model of ABA linked to ethylene, sugar, polyamines, auxin and reactive oxygen species in the regulation of strawberry fruit ripening is proposed. Next, new integrated mechanisms, including two ABA signaling pathways, ABA and ethylene signaling pathways, and ABA/ethylene to other phytohormones are interesting and important research topics in ripening, especially in non-climacteric fruits.
Introduction
Angiosperm fruits can generally be divided into dry or fleshy types. Flowering plants have evolved both conserved and divergent mechanisms for development and maturation of both fleshy and dry fruits (Hershkovitz et al., 2011; Garceau et al., 2017). The molecular mechanisms of dry fruit development and maturation have been revealed through studies of the model plant Arabidopsis (Gazzarrini and Tsai, 2015), while studies of various non-ripening tomato fruit mutants have defined the mechanisms of fleshy fruit ripening (Liu et al., 2015).
Fleshy fruit ripening is mainly divided into two types, climacteric and non-climacteric, based on the presence or absence of a transient peak in respiration rate and ethylene emission. In climacteric fruits, such as tomato and banana, peaks in both respiration and ethylene level occur during ripening; in contrast, no such peaks occur in non-climacteric fruits, such as grape and strawberry, the ripening of which is controlled by abscisic acid (ABA) in an ethylene-independent manner (Kumar et al., 2014; Shen and Rose, 2014). Fruit color break, coinciding with the conversion of chloroplasts into chromoplasts, is a visible sign of ripening in both climacteric and non-climacteric fruits, and is followed by softening and changes in sugars, color, and flavor (Azoulay et al., 2008). During the past decade, studies using strawberry fruit as a model have led to major breakthroughs in understanding the regulation of non-climacteric fruit ripening by ABA and its interaction with other phytohormones. In this review, we focus on the physiological and molecular mechanisms of ABA in regulation of fleshy fruit ripening.
Central Roles of ABA in Regulation of Non-Climacteric Fruit Ripening
Classical studies of ABA effects on fleshy fruit ripening were done on grape (Vitis vinifera) berries and uncovered vital roles for ABA in veraison and ripening processes, including coloration, sugar accumulation, acid decline, and flesh softening (Coombe, 1976; Davies et al., 1997). However, a deeper understanding of ABA action in non-climacteric fruit ripening was gained from a breakthrough study in strawberry (Jia et al., 2011). The importance of ABA has recently been confirmed in many non-climacteric fruits, such as sweet cherry (Prunus avium; Tijero et al., 2016; Shen et al., 2017), watermelon (Citrullus lanatus; Wang et al., 2017b), blueberry (Semen trigonellae; Oh et al., 2018), bilberry (Vaccinium myrtillus; Karppinen et al., 2018), jujube (Ziziphus jujuba; Kou et al., 2019), litchi (Litchi chinensis; Hu et al., 2019), orange (Citrus sinensis; Romero et al., 2019), and wolfberry (Lycium ruthenicum; Li et al., 2019).
ABA Perception and Signaling in Strawberry Fruit Ripening Represent a Model of Non-climacteric Ripening
In developing strawberry fruit, ripening is markedly stimulated by exogenous ABA or DMSO (dimethyl sulfoxide, an accelerator of ABA biosynthesis), whereas fluridone (an inhibitor of ABA biosynthesis) significantly inhibits ripening; furthermore, downregulation of either FaNCED1 (9-cis-epoxycarotenoid dioxygenase, a key gene in ABA biosynthesis) or FaCHLH/ABAR (magnesium chelatase H subunit/putative ABA receptor) inhibits ripening; importantly, exogenous ABA can rescue coloration of FaNCED1-RNAi fruit but not of FaCHLH/ABAR-RNAi fruit, confirming that ABA is a key signal molecule in the promotion of strawberry ripening (Jia et al., 2011). Kadomura-Ishikawa et al. (2015) identified FaMYB10 as an important transcription factor mediating signal transduction downstream of ABA perception by the ABAR to stimulate anthocyanin biosynthesis during strawberry fruit ripening. ABA and FaMYB10 are therefore key regulators for strawberry ripening (Kim et al., 2019). In all, ABA homeostasis in cells involves the regulation of both ABA catabolism and ABA biosynthesis by feedback and feedforward loops, which are tightly linked to the repression of FveCYP707A4a expression, key to ABA degradation, and promotion of FveNCED expression, key to ABA biosynthesis, in response to the onset of strawberry fruit ripening (Liao et al., 2018). Tightly controlled, synergistic regulation of ABA from biosynthesis to signaling is required to meet the requirements of plant development and fruit ripening.
In addition, the importance of ABA in strawberry ripening was also confirmed by the silencing of genes encoding another ABA receptor, FaPYR1 (Pyrabactin Resistance1; Chai et al., 2011), and its interacting protein FaABI1 (type 2C protein phosphatase1; Jia et al., 2013a). A strawberry FERONIA/FER-like receptor kinase, FaMRLK47, negatively regulates ABA signaling and fruit ripening through its interaction with FaABI1 (Jia et al., 2017a). Importantly, the core ABA signaling pathways, Pyrabactin Resistance 1 (PYR1)/PYR1-like (PYL)/Regulatory Component of ABA Receptor (RCAR) – Type 2C Protein Phosphatase (PP2C)-SNF1-Related Protein Kinase2 (SnRK2) are conserved in plant responses to developmental and environmental cues during stress adaptation, flowering, seed germination, and fruit ripening (Li et al., 2011; Cheng et al., 2019; Gupta et al., 2020).
CHLH/ABAR has multiple functions (Liang et al., 2015). For example, in Arabidopsis, CHLH/ABAR regulates various plant developmental processes in different pathways, such as stomatal movement, seed germination, and seedling growth, through ABA-ABAR-WRKY40-ABI5/ABI4 (Shang et al., 2010). To further explore the mechanisms of FaABAR action in strawberry fruit ripening, Hou et al. (2018) used FaABAR as bait in a yeast two-hybrid assay and isolated a strawberry leucine-rich repeat (LRR) receptor-like kinase, red-initial protein kinase 1 (FaRIPK1) that interacts with FaABAR. FaRIPK1 serves as a co-receptor of FaABAR to synergistically regulate strawberry fruit ripening, namely, FaRIPK1 participates in the onset of ripening while FaABAR is responsible for completion of ripening. Importantly, FaABAR binds to ABA with a Kd (dissociation constant) of 50 μM (Zhang et al., 2017), suggesting that FaABAR is an ABA receptor in strawberry (Jia et al., 2011; Zhang et al., 2017; Hou et al., 2018). Notably, the ABA-ABAR-RIPK1-ABI4 signaling pathway that controls fruit ripening in strawberry (Hou et al., 2018) is distinct from the ABA-ABAR-WRKY40-ABI5/ABI4 pathway in Arabidopsis (Shang et al., 2010).
By contrast, FaPYR1 regulates fruit ripening through the conserved core signaling pathway PYR1-PP2C-SnRK2 (Cheng et al., 2019; Gupta et al., 2020). In addition, among members of FaPYR/PYL and FaPP2C families in strawberry, only FaPYL2/4/9/11 and FaABI1/FaPP2C16 interact with each other, and the interaction of FaPYL2 with FaABI1 might also play a role in strawberry fruit ripening (Hou et al., 2020). Notably, the SnRK2.6/OST1 protein acts as a linker between ABAR and PYR/PYL/RCAR in Arabidopsis guard cells (Liang et al., 2015). Thus, the relationships between ABAR and PYR/PYL/RCAR in strawberry fruit ripening should be established in the future.
ABA is a Major Player in the Ripening of Various Non-climacteric Fruits
The relationship between ABA and fruit ripening defined in strawberry facilitates studies of ripening in other non-climacteric fruits, such as grape (Villalobos-González et al., 2016), sweet cherry (Shen et al., 2017), watermelon (Wang et al., 2017b), blueberry (Oh et al., 2018), bilberry (Karppinen et al., 2018), jujube (Kou et al., 2019), litchi (Hu et al., 2019), orange (Romero et al., 2019), and wolfberry (Li et al., 2019).
It is previously reported that ABA is a major phytohormone involved in regulating the onset of grape berry ripening and berry skin secondary metabolism (Villalobos-González et al., 2016). ABA positively regulates sweet cherry development, ripening, and quality through the interaction of PacPP2C1 with six PacSnRK2s, including PacSnRK2.2/2.3/2.6 and PacPP2C1-3 (Tijero et al., 2016; Shen et al., 2017). It is also a major player in watermelon fruit ripening (Wang et al., 2017b). While application of ABA to blueberry fruits has no remarkable effect on fruit growth, it stimulates fruit coloration and softening; however, softening is undesirable during harvest and storage (Oh et al., 2018; Chung et al., 2019). Chinese jujube is a non-climacteric fruit, the ripening of which is regulated by ABA (Kou et al., 2019; Zhang et al., 2019). In addition, ABA plays a key role in ripening-related processes including fruit coloration and softening in both bilberry (Karppinen et al., 2018) and orange fruits (Romero et al., 2019). ABA-mediated anthocyanin biosynthesis during wolfberry fruit maturation and coloration involves the transcription factor complex MYB-bHLH-WD40 (Li et al., 2019). Interestingly, recent reports suggest that the grape U-box E3 ubiquitin ligase VlPUB38 negatively regulates berry ripening in an ABA-dependent manner by degradation of abscisic-aldehyde oxidase (VlAAO), the enzyme catalyzing the last step of ABA biosynthesis (Yu et al., 2020). A synergistic interaction between CrNAC036 and CrMYB68 appears to inhibit CrNCED5-mediated ABA biosynthesis at the transcription level in citrus fruit (Zhu et al., 2020).
De-greening (chlorophyll degradation) and coloration (such as anthocyanin biosynthesis) are major physiological changes during fruit ripening. Application of ABA rapidly initiates chlorophyll breakdown in litchi fruit, and peak ABA concentration is concommitant with the subsequent onset of anthocyanin biosynthesis, demonstrating an important role for ABA during ripening (Hu et al., 2019). ABA response element-binding factors (LcABF1/2/3) play a vital role in litchi fruit ripening: LcABF1 expression increases in parallel with chlorophyll degradation, while LcABF3 expression rises at the onset of coloration; moreover, LcABF1/2 mediate expression of ABA-responsive genes related to chlorophyll degradation, while LcABF2/3 mediate expression of genes related to anthocyanin biosynthesisin an ABA-dependent manner (Hu et al., 2019). Collectively, ABA is a major player in regulation of the ripening of various non-climacteric fruits.
Non-climacteric Fruit Ripening is Regulated by ABA Through Multiple Synergistic Mechanisms
In non-climacteric strawberry fruit, ABA and auxin (IAA) are important regulators, functioning together or independently in response to developmental and environmental cues; auxin is involved in receptacle development and ABA participates in fruit ripening. By contrast, ethylene and gibberellins do not play a prominent role in ripening (Medina-Puche et al., 2016). Contents of both IAA and GA4 (gibberellic acid) are highest in small, green strawberry fruit and gradually decrease throughout fruit development; ABA content increases rapidly, coincident with coloration; methyl jasmonate concentration shows no remarkable variation over time, while salicylic acid content gradually increases; and jasmonic acid and ethylene contents are too low to quantify (Kim et al., 2019). Auxin is produced mainly in achenes, while ABA, ethylene, cytokinins and gibberellins are predominantly biosynthesized in receptacles (Gu et al., 2019). Gibberellin delays ripening to some extent, while cytokinins and ethylene appear to be involved in the later stages of ripening, mostly highlighting possible mechanisms of ABA and auxin interaction in the ripening process (Gu et al., 2019). With the onset of fruit ripening in strawberry, the roles of ABA, ethylene, and polyamines are reinforced while those of GA and IAA are weakened (Wang et al., 2017a). Available data from strawberry suggest that regulation of non-climacteric fruit ripening is rather complex.
Interaction of ABA With Ethylene
Although ethylene is well known as a key regulator in climacteric fruit ripening (Liu et al., 2015), this gaseous molecule also participates in non-climacteric fruit ripening through its interaction with ABA (Li et al., 2020; Tosetti et al., 2020). In postharvest strawberry fruit, ethylene facilitates ABA accumulation in receptacle tissue but does not affect ABA catabolism (Tosetti et al., 2020). Overexpression of FveERF (FvH4_5g04470.1), an ethylene response regulator, activates both acyltransferase (AAT) gene transcription and ester accumulation during strawberry fruit ripening (Li et al., 2020). The promoter regions of ACS1 from both climacteric “Santa Rosa” plum and its non-climacteric mutant “Sweet Miriam” have only minor differences in sequence; however, ABI5 (ABA insensitive5) expression in “Sweet Miriam” fruit is lower than that in “Santa Rosa” fruit during ripening, suggesting a vital role of ABA in ethylene production (Sadka et al., 2019). A cucumber (Cucumis sativus) MADS-box (SHATTERPROOF) protein, CsSHP, participates in fruit maturation through ABA-mediated protein complex associated to CsSEPs (SEPALLATA; Cheng et al., 2020). ABA- and ethylene-related genes are differentially modulated during grape berry ripening by a set of transcription factors, including MADS-box, MYB, NAC, AP2/ERF, bHLH, and ZIP (Wong et al., 2016). Thereby, the interaction of ABA with ethylene plays a vital role in non-climacteric fruit ripening.
Interaction of ABA With IAA
Greater accumulation of IAA and ABA in developing achenes than in the receptacle is important for strawberry fruit maturation and ripening, suggesting a complex ripening mechanism regulated by the two hormones (Araújo et al., 2012; Symons et al., 2012). In fact, auxin plays a dominant role in receptacle growth while ABA plays a vital role in ripening, and other hormones function to different degrees with ABA or ethylene (Estrada-Johnson et al., 2017). Some ripening-related genes are co-regulated by IAA and ABA during strawberry fruit development (Castillejo et al., 2004), such as FaRGlyase1 (rhamnogalacturonate lyase gene; Molina-Hidalgo et al., 2013), FaSHP (a C-type MADS-box gene; Daminato et al., 2013), FaβGal4 (β-galactosidase gene; Paniagua et al., 2016), and FaNIP1;1 (plasma membrane aquaporin protein gene; Molina-Hidalgo et al., 2015), which are positively regulated by ABA and negatively regulated by auxins during strawberry fruit ripening. Also, annexins FaAnn5 and FaAnn8 might be involved in the regulation of both ABA and IAA during strawberry fruit growth and ripening through calcium signaling (Chen et al., 2016). The receptor-like kinase and ubiquitin ligase respond to both IAA and ABA and may play an important role in crosstalk between the two hormones (Chen et al., 2016). IAA and ABA are therefore key regulators of strawberry fruit maturation and ripening.
Confirmedly, strong “antagonism” between IAA and ethylene and substantial “synergism” between IAA and ABA occur during grape berry development (Ziliotto et al., 2012). At berry pre-ripening, high auxin levels promote seed tissue development, and transcripts of auxin-response-factor genes accumulate in pericarp tissue; at veraison, auxin action is weakened while ABA action is enforced, suggesting a role for a higher IAA/ABA ratio during growth and a lower ratio during ripening (Gouthu and Deluc, 2015). ABA, auxin and ethylene play important roles in the regulation of non-climacteric fruit maturation and ripening via a complex network (Corso et al., 2016).
Interaction of ABA With Polyamines
Blocking ethylene biosynthesis affects the levels of both ABA and putrescine in melon fruit (Concepción Martínez-Madrid et al., 2002). During strawberry fruit ripening, polyamines (PAs), especially spermine, interact with ABA, IAA, and ethylene in a coordinated manner (Guo et al., 2018). Increased transcription of NCED3, key to ABA production, at the onset of strawberry fruit ripening facilitates rapid accumulation of ABA, which inhibits transcription of FaPAO5 (polyamine oxidase5), key to PA degradation, leading to accumulation of spermine and spermidine (Mo et al., 2020). Interestingly, the increase in spermine and spermidine contents triggers expression of SAMDC (SAM decar boxylase), SPDS (spermidine synthase), and SPMS (spermine synthase) genes, key to PA biosynthesis, further accelerating spermine and spermidine accumulation and ripening, suggesting that FaPAO5 regulates spermine and spermidine levels in response to ABA signaling during strawberry fruit ripening (Mo et al., 2020). Thus, interactions between ABA and PA play an important role in the regulation of strawberry fruit ripening.
Interaction of ABA With Sugars
Sugars play a central role in fruit ripening and quality because sugar metabolism and accumulation greatly influence taste. Notably, sucrose also serves as a signal, which facilitates strawberry fruit ripening by stimulating ABA production and accumulation (Jia et al., 2011, 2013b). Both ABA and sucrose induce grape berry ripening, with sucrose acting in both ABA-dependent and ABA-independent manners (Jia et al., 2017b). ASR (ABA-, stress-, and ripening-induced protein) regulates strawberry fruit ripening through crosstalk between ABA and sucrose (Jia et al., 2016). Application of sucrose to unripe strawberry fruit promotes ripening by ABA and its derivatives (Siebeneichler et al., 2020). ABA and sucrose accelerate strawberry fruit ripening and induce accumulation of H2O2, leading to a transient decrease in glycolysis, demonstrating that the interaction of ABA with sucrose affects ripening by inhibiting glycolysis (Luo et al., 2020a). Moreover, FaGAPC2 (cytosolic glyceraldehyde-3-phosphate dehydrogenase)/FaGAPCp1 (plastid glyceraldehyde-3-phosphate dehydrogenase), key glycolytic enzymes, negatively regulate ABA- and sucrose-mediated ripening in strawberry fruit (Luo et al., 2020b). Therefore, the interaction between ABA and sugar plays a pivotal role in strawberry fruit ripening.
ABA is also Involved in the Regulation of Climacteric Fruit Ripening
Although ethylene is an essential regulator of climacteric tomato fruit ripening (Liu et al., 2015), in which, as has been demonstrated over the past decade, ABA also plays a role. Application of ABA accelerates coloration and ethylene biosynthesis in tomato fruit as well as accumulation of phenolic compounds and emission of volatile aromatics (Wu et al., 2018). A tomato gene key to ABA biosynthesis, SINCED1, triggers the onset of tomato fruit ripening (Zhang et al., 2009), and suppression of SINCED1 expression leads to increases in lycopene and β-carotene contents and in shelf life from 15 to 29 days (Sun et al., 2012a,b). Most PYL ABA receptors interact with SlPP2Cs in an ABA-dependent manner; SlPP2C2/SlPP2C3 interact with SlSnRK2s; SlSnRK2.5 interacts with SlABF2/4; and many core components of ABA signaling respond to ABA (Chen et al., 2016). In addition, downregulating the expression of either SlUGT75C1 (ABA uridine diphosphate glucosyltransferase gene) or SlPP2C1 (type 2C protein phosphatase gene) alters tomato fruit ripening (Sun et al., 2017; Zhang et al., 2018). The tomato ABA receptor SlPYL9 positively regulates ABA signal transduction and fruit ripening in an ABA-dependent manner (Kai et al., 2019). Overexpression of LYCOPENE β-CYCLASE (LCYb) leads to an increase in β-carotene and ABA concentrations, as well as a decrease in ethylene emission, which delays softening and increases shelf life, suggesting that manipulation of β-carotene levels can improve nutritional value and shelf life (Diretto et al., 2020). Similarly, 24 PYL, 87 PP2C, and 11 SnRK2 genes have been identified in banana fruit ripening, providing new insights into understanding PYL-PP2C-SnRK2 core signaling (Hu et al., 2017).
The interaction between ABA and ethylene has been studied extensively, contributing to our understanding of the mechanism of ABA involvement in climacteric fruit ripening. ABA may promote ethylene emission and signal transduction by recruiting a set of important genes, including LeACO1 (1-amino-cyclopropane-1-carboxylic acid oxidase1), LeACS4 (1-amino-cyclopropane-1-carboxylic acid synthase4), GR (green-ripe), and LeETR6 (ethylene receptor6); while ethylene plays an essential role in inducing ABA accumulation at the initiation of ripening, and blocking or removing ethylene leads to low ABA levels (Meyer et al., 2017). Furthermore, a set of transcription factors specific to ethylene production and signaling, including MADS-RIN (MADS-ripening inhibitor), TAGL1 (tomato Agamous-like1), CNR (colorless non-ripening), and NOR (non-ripening), are also ABA responsive, suggesting a crucial function of these transcription factors in ABA-ethylene interactions (Mou et al., 2016). Transient overexpression of SlAREB1, a tomato transcription factor gene downstream of ABA signaling, promotes expression of a set of genes including NOR, SlACS2, SlACS4, and SlACO1, suggesting that SlAREB1-NOR crosstalk plays a vital role in ABA-mediated ethylene biosynthesis during tomato fruit ripening (Mou et al., 2018).
In addition to tomato, ABA is also involved in ripening of other climacteric fruits. Application of ABA to apple facilitates ethylene emission by AREB/ABF-mediated regulation of MdACS1/3 and MdACO1 expression, suggesting a role for ABA in ethylene biosynthesis (Wang et al., 2018). In banana, ABA promotes MaMADS2 expression and fruit ripening (Yakir et al., 2018). A peach ethylene response transcription factor, PpERF3, positively regulates PpNCED2/3-mediated ABA biosynthesis during fruit ripening (Wang et al., 2019a), while PpERF2 regulates fruit-ripening by inhibiting PpNCED2/3 expression. PpERF2 binds to the promoters of PpNCED2, PpNCED3, and PpPG1 (Wang et al., 2019b).
In all, the ABA-PYL-PP2C-SnRK2 core signaling pathway is also conserved in climacteric fruit. A set of crucial regulators related to the biosynthesis and signaling of both ABA and ethylene, such as NCED, AREB/ABF, ACS, ACO, and ERF, likely play important roles in the interaction of these two hormones, as elucidated by AREB/ABF-mediated ACS/ACO expression and ERF-mediated NCED expression. MADS-RIN, TAGL1, CNR, and NOR, transcription factors specific to ethylene biosynthesis and signaling, are probably also involved.
An Integrated, Comprehensive Understanding of ABA in Fleshy Fruit Ripening
The developmental processes of fleshy fruits include cell division and expansion during fruit growth, followed by chlorophyll degradation, cell wall softening, and changes to phenylpropanoid, flavonoid, starch/sucrose, and carotenoid metabolism during ripening. These processes are controlled by phytohormones, most notably ethylene in climacteric fruit ripening and ABA in non-climacteric fruit ripening, as well as ABA-ethylene interactions in both types of fruit (Forlani et al., 2019; García-Gómez et al., 2020; Jiao et al., 2020).
De-greening through loss of chlorophyll is an obvious indicator of fruit ripening, and ethylene and ABA interact with light during chlorophyll degradation (Zhu et al., 2017). In addition, a decrease in water content occurs at the onset of ripening in both climacteric and non-climacteric fruit in an ethylene-independent manner, suggesting that oxidant-induced cell wall remodeling and consequent wall dehydration may trigger stress signaling that initiates fruit ripening (Frenkel and Hartman, 2012). Fruit ripening and senescence are associated with changes in fruit texture, color, aroma, and flavor, processes in which reactive oxygen species play an important role (Kumar et al., 2016). The interaction of auxin with ethylene is important in ripening of both climacteric and non-climacteric fruits (Paul et al., 2012). The APETALA2/ethylene response factor (AP2/ERF) transcription factor is a crucial response regulator in ethylene signaling, which not only modulates biosynthesis of ABA, ethylene, gibberellin, and cytokinin in a feedback manner but also responds to signaling associated with ABA, IAA, jasmonate, and cytokinin (Wang et al., 2015; Gu et al., 2017). The promotion of grape berry ripening by ABA, ethylene, and brassinosteroids, and ripening inhibition by IAA, jasmonic acid, GAs, cytokinins, and PAs reveal complex interactions among signals (Fortes et al., 2015). Notably, polyamines regulate strawberry fruit ripening by ABA, auxin and ethylene (Guo et al., 2018), while FaPAO5 regulates Spm/Spd levels as a signaling during strawberry fruit ripening by integrating multiple signals including Ca2+ and GAs (Mo et al., 2020).
Finally, we propose an integrative model of ABA regulation of fleshy fruit ripening (Figure 1). In response to developmental and environmental cues, ABA levels in fleshy fruits are controlled by ABA biosynthesis and catabolism; the former mainly through NCEDs and β-glucosidases and the latter through CYP707As and β-glycosyltransferases. ABA molecules in fleshy fruit are perceived via the ABA receptors PYR/PYL and ABAR, which are linked to the conserved signaling pathway ABA-FaPYR1-FaABIl-FaSnRK2 and the novel signaling pathway ABA-FaABAR-FaRIPK1-FaABI4 in strawberry, respectively. In the conserved ABA signaling pathway, ABA levels increase at the onset of fruit ripening; consequently, the accumulated ABA binds to its receptor FaPYR1 to form a FaPYR1-FaABIl complex, releasing the inhibition of protein kinase FaSnRK2 and in turn activating an ABA signaling cascade, which promotes fruit ripening. This pathway constitutes the conserved mechanism of ABA action in plants. In the novel ABA signaling pathway, ABA binding to the receptor FaABAR/FaRIPK1 complex activates the transcription factor FaABI4, which triggers ABA signal transduction, eventually promoting fruit ripening. This signaling pathway is different from the Arabidopsis ABA-ABAR-WRKY40 signaling pathway, thus constituting a novel ABA signaling pathway during strawberry fruit ripening.
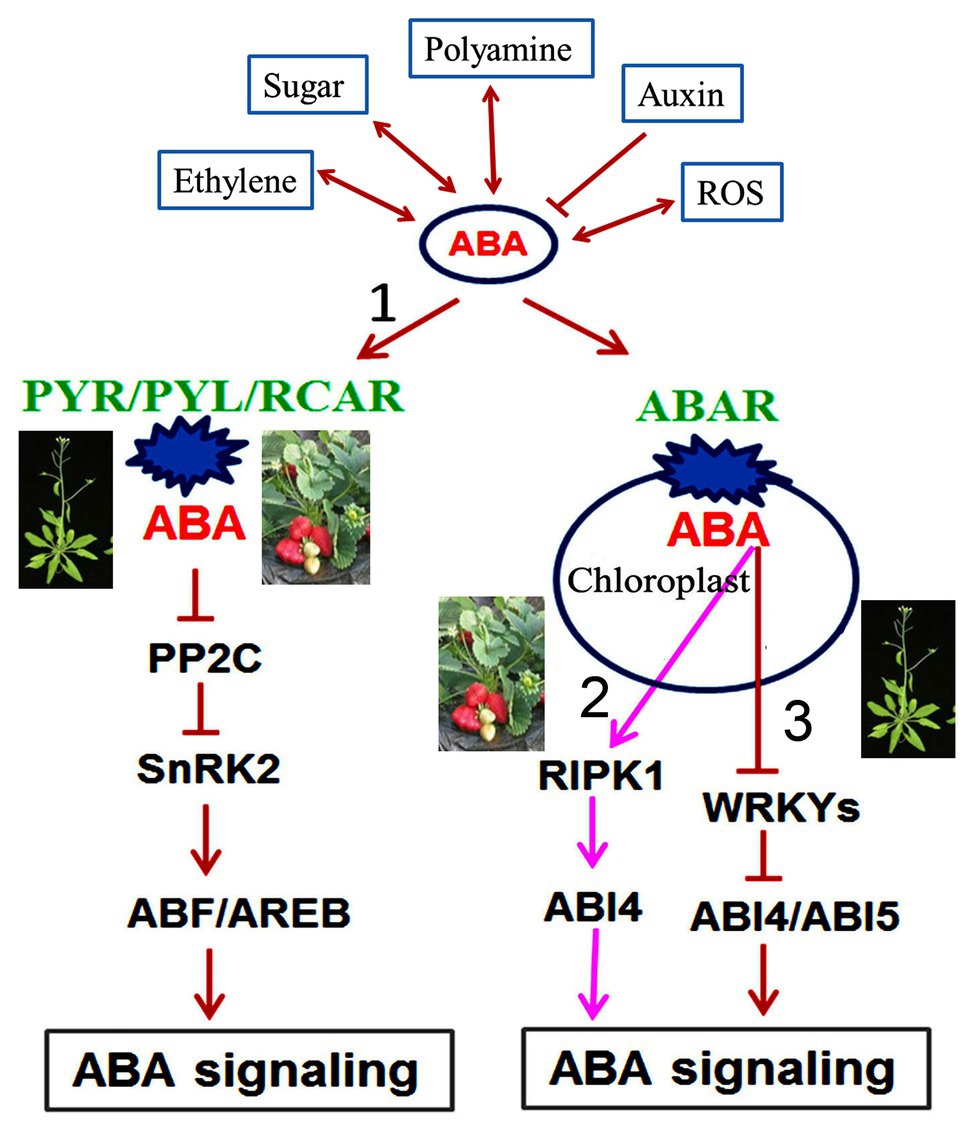
Figure 1. A comprehensive model for ABA in regulating strawberry fruit ripening. The regulation of ABA in strawberry fruit ripening is involved in conserved and novel signaling mechanisms. ABA in fleshy fruits is perceived by its receptors, PYR/PYL/RCAR and ABAR, which initiates signal transduction, including the conserved signaling pathway ABA-PYR/PYL/RCAR-PP2C-SnRK2-ABF/AREB in both Arabidopsis and strawberry (signaling pathway 1) and the novel signaling pathway ABA-FaABAR-FaRIPK1-FaABI4 in strawberry (signaling pathway 2). In strawberry ABA signaling, ABAR/FaRIPK1 serves as a receptor complex, which is distinct from the Arabidopsis ABA-ABAR-WRKY40-ABI4/ABI5 signaling pathway (signaling pathway 3). ABA interacts with several synergistically-regulated molecules such as ethylene, sugar, polyamines, auxin, and reactive oxygen species (ROS) during strawberry fruit ripening. The symbols (→, ⟞, and ↔) represent promotion, inhibition, and cooperation, respectively. ABA, abscisic acid; ABAR, putative abscisic acid receptor; PYR, Pyrabactin resistance; PYL, PYR-like; RCAR, regulatory components of ABA receptors; PP2C, Type 2C protein phosphatase; SnRK2, SNF1-related protein kinases2; ABF, ABRE binding factors ABA responsive factors; AREB, ABA responsive element binding protein; ROS, reactive oxygen species; RIPK1, red-initial protein kinase1; ABI, abscisic acid-insensitive; WRKY, tryptophan-arginine-lysine-tyrosine.
Conclusions
Fruit ripening is controlled by phytohormones, with ripening of climacteric fruits controlled by ethylene and that of non-climacteric fruits regulated mainly by ABA, as well as by ABA-ethylene interplay in the two types of fruit ripening. ABA participates in the ripening of both non-climacteric and climacteric fruit. The core signaling pathway ABA-PYL-PP2C-SnRK2 is conserved, and a series of crucial regulators including NCED, AREB/ABF, ACS, ACO, and ERF may play important roles in ABA-ethylene interaction, particularly AREB/ABF-mediated ACS/ACO expression and ERF-mediated NCED expression. ABA levels in fleshy fruit are controlled through a balance between ABA biosynthesis and catabolism involving key enzymes, including NCEDs, β-glucosidases, CYP707As, and β-glycosyltransferases. ABA in fleshy fruits is perceived by at least two ABA receptors, PYR/PYL and ABAR, which are linked separately to the conserved signaling pathway ABA-FaPYR1-FaABIl-FaSnRK2 and the novel signaling pathway ABA-FaABAR-FaRIPK1-FaABI4 in strawberry fruit ripening. This model provides novel insights into the role of ABA in ripening, at least in strawberry, a model plant for non-climacteric-type fruits. Next, new integrated mechanisms, including two ABA signaling pathways, ABA and ethylene signaling pathways, and ABA/ethylene to other phytohormones and regulators, are interesting and important research topics in ripening, especially in non-climacteric fruits.
Author Contributions
YS, YH, and QB wrote the review. QB collected references. YS and YH designed the model and revised the review. All authors contributed to the article and approved the submitted version.
Funding
This study was supported by the National Natural Science Foundation of China (projects 32030100; 31672125; 32072516), the Beijing Natural Science Foundation (6171001), Scientific Research Project of Beijing Educational Committee (KZ202010020028; KM202110020006), the Construction of Beijing Science and Technology Innovation and Service Capacity in Top Subjects (CEFF_PXM2019_014207_000032), the Opening Project of Beijing Key Laboratory of New Technology in Agricultural Application (kf2020021), and Sichuan Lomon Biotechnology Co., LTD (2018001).
Conflict of Interest
The authors declare that the research was conducted in the absence of any commercial or financial relationships that could be construed as a potential conflict of interest.
Acknowledgments
We thank Guo JX very much for suggestions about the role of polyamine in fruit ripening.
References
Araújo, W. L., Tohge, T., Osorio, S., Lohse, M., Balbo, I., Krahnert, I., et al. (2012). Antisense inhibition of the 2-oxoglutarate dehydrogenase complex in tomato demonstrates its importance for plant respiration and during leaf senescence and fruit maturation. Plant Cell 24, 2328–2351. doi: 10.1105/tpc.112.099002
Azoulay, S. T., Harpaz-Saad, S., Belausov, E., Lovat, N., Krokhin, O., Spicer, V., et al. (2008). Citrus chlorophyllase dynamics at ethylene-induced fruit color-break: a study of chlorophyllase expression, posttranslational processing kinetics, and in situ intracellular localization. Plant Physiol. 148, 108–118. doi: 10.1104/pp.108.124933
Castillejo, C., de la Fuente, J. I., Iannetta, P., Botella, M. A., and Valpuesta, V. (2004). Pectin esterase gene family in strawberry fruit: study of FaPE1, a ripening-specific isoform. J. Exp. Bot. 55, 909–918. doi: 10.1093/jxb/erh102
Chai, Y. M., Jia, H. F., Li, C. L., Dong, Q. H., and Shen, Y. Y. (2011). FaPYR1 is involved in strawberry fruit ripening. J. Exp. Bot. 62, 5079–5089. doi: 10.1093/jxb/err207
Chen, P., Sun, Y. F., Kai, W. B., Liang, B., Zhang, Y. S., Zhai, X. W., et al. (2016). Interactions of ABA signaling core components (SlPYLs, SlPP2Cs, and SlSnRK2s) in tomato (Solanum lycopersicon). J. Plant Physiol. 205, 67–74. doi: 10.1016/j.jplph.2016.07.016
Cheng, S., Xian, W., Fu, Y., Marin, B., Keller, J., Wu, T., et al. (2019). Genomes of subaerial zygnematophyceae provide insights into land plant evolution. Cell 179, 1057–1067. doi: 10.1016/j.cell.2019.10.019
Cheng, Z., Zhuo, S., Liu, X., Che, G., Wang, Z., Gu, R., et al. (2020). The MADS-box gene CsSHP participates in fruit maturation and floral organ development in cucumber. Front. Plant Sci. 10:1781. doi: 10.3389/fpls.2019.01781
Chung, S. W., Yu, D. J., Oh, H. D., Ahn, J. H., Huh, J. H., and Lee, H. J. (2019). Transcriptional regulation of abscisic acid biosynthesis and signal transduction, and anthocyanin biosynthesis in ‘Bluecrop’ highbush blueberry fruit during ripening. PLoS One 14:e0220015. doi: 10.1371/journal.pone.0220015
Concepción Martínez-Madrid, M., Flores, F., and Romojaro, F. (2002). Behaviour of abscisic acid and polyamines in antisense ACC oxidase melon (Cucumis melo) during ripening. Funct. Plant Biol. 29, 865–872. doi: 10.1071/PP01164
Corso, M., Vannozzi, A., Ziliotto, F., Zouine, M., Maza, E., Nicolato, T., et al. (2016). Grapevine rootstocks differentially affect the rate of ripening and modulate auxin-related genes in cabernet sauvignon berries. Front. Plant Sci. 7:69. doi: 10.3389/fpls.2016.00069
Daminato, M., Guzzo, F., and Casadoro, G. (2013). A SHATTERPROOF-like gene controls ripening in non-climacteric strawberries, and auxin and abscisic acid antagonistically affect its expression. J. Exp. Bot. 64, 3775–3786. doi: 10.1093/jxb/ert214
Davies, C., Boss, P., and Robinson, S. (1997). Treatment of grape berries, a nonclimateric fruit with a synthetic auxin, retards ripening and alters the expression of developmentally regulated genes. Plant Physiol. 115, 1155–1161. doi: 10.1104/pp.115.3.1155
Diretto, G., Frusciante, S., Fabbri, C., Schauer, N., Busta, L., Wang, Z., et al. (2020). Manipulation of β-carotene levels in tomato fruits results in increased ABA content and extended shelf life. Plant Biotechnol. J. 18, 1185–1199. doi: 10.1111/pbi.13283
Estrada-Johnson, E., Csukasi, F., Pizarro, C. M., Vallarino, J. G., Kiryakova, Y., Vioque, A., et al. (2017). Transcriptomic analysis in strawberry fruits reveals active auxin biosynthesis and signaling in the ripe receptacle. Front. Plant Sci. 8:889. doi: 10.3389/fpls.2017.00889
Forlani, S., Masiero, S., and Mizzotti, C. (2019). Fruit ripening: the role of hormones, cell wall modifications, and their relationship with pathogens. J. Exp. Bot. 70, 2993–3006. doi: 10.1093/jxb/erz112
Fortes, A. M., Teixeira, R. T., and Agudelo-Romero, P. (2015). Complex interplay of hormonal signals during grape berry ripening. Molecules 20, 9326–9343. doi: 10.3390/molecules20059326
Frenkel, C., and Hartman, T. G. (2012). Decrease in fruit moisture content heralds and might launch the onset of ripening processes. J. Food Sci. 77, S365–S376. doi: 10.1111/j.1750-3841.2012.02910.x
Garceau, D. C., Batson, M. K., and Pan, I. L. (2017). Variations on a theme in fruit development: the PLE lineage of MADS-box genes in tomato (TAGL1) and other species. Planta 246, 313–321. doi: 10.1007/s00425-017-2725-5
García-Gómez, B. E., Ruiz, D., Salazar, J. A., Rubio, M., Martínez-García, P. J., and Martínez-Gómez, P. (2020). Analysis of metabolites and gene expression changes relative to apricot (Prunus armeniaca L.) fruit quality during development and ripening. Front. Plant Sci. 11:1269. doi: 10.3389/fpls.2020.01269
Gazzarrini, S., and Tsai, A. Y. (2015). Hormone cross-talk during seed germination. Essays Biochem. 58, 151–164. doi: 10.1042/bse0580151
Gouthu, S., and Deluc, L. G. (2015). Timing of ripening initiation in grape berries and its relationship to seed content and pericarp auxin levels. BMC Plant Biol. 15:46. doi: 10.1186/s12870-015-0440-6
Gu, C., Guo, Z. H., Hao, P. P., Wang, G. M., Jin, Z. M., and Zhang, S. L. (2017). Multiple regulatory roles of AP2/ERF transcription factor in angiosperm. Bot. Stud. 58:6. doi: 10.1186/s40529-016-0159-1
Gu, T., Jia, S., Huang, X., Wang, L., Fu, W., Huo, G., et al. (2019). Transcriptome and hormone analyses provide insights into hormonal regulation in strawberry ripening. Planta 250, 145–162. doi: 10.1007/s00425-019-03155-w
Guo, J., Wang, S., Yu, X., Dong, R., Li, Y., Mei, X., et al. (2018). Polyamines regulate strawberry fruit ripening by abscisic acid, auxin, and ethylene. Plant Physiol. 177, 339–351. doi: 10.1104/pp.18.00245
Gupta, M. K., Lenka, S. K., Gupta, S., and Rawal, R. K. (2020). Agonist, antagonist and signaling modulators of ABA receptor for agronomic and post-harvest management. Plant Physiol. Biochem. 148, 10–25. doi: 10.1016/j.plaphy.2019.12.023
Hershkovitz, V., Friedman, H., Goldschmidt, E. E., Feygenberg, O., and Pesis, E. (2011). Effect of seed on ripening control components during avocado fruit development. J. Plant Physiol. 168, 2177–2183. doi: 10.1016/j.jplph.2011.07.010
Hou, B., Chen, X., and Shen, Y. (2020). Interactions between strawberry ABA receptor PYR/PYLs and protein phosphatase PP2Cs on basis of Transcriptome and yeast two-hybrid analyses. J. Plant Growth Regul. doi: 10.1007/s00344-020-10121-4
Hou, B. Z., Xu, C., and Shen, Y. Y. (2018). A leu-rich repeat receptor-like protein kinase, FaRIPK1, interacts with the ABA receptor, FaABAR, to regulate fruit ripening in strawberry. J. Exp. Bot. 69, 1569–1582. doi: 10.1093/jxb/erx488
Hu, B., Lai, B., Wang, D., Li, J., Chen, L., Qin, Y., et al. (2019). Three LcABFs are involved in the regulation of chlorophyll degradation and anthocyanin biosynthesis during fruit ripening in Litchi chinensis. Plant Cell Physiol. 60, 448–461. doi: 10.1093/pcp/pcy219
Hu, W., Yan, Y., Shi, H., Coombe, J., Miao, H., Tie, W., et al. (2017). The core regulatory network of the abscisic acid pathway in banana: genome-wide identification and expression analyses during development, ripening, and abiotic stress. BMC Plant Biol. 17:145. doi: 10.1186/s12870-017-1093-4
Jia, H. F., Chai, Y. M., Li, C. L., Lu, D., Luo, J. J., Qin, L., et al. (2011). Abscisic acid plays an important role in the regulation of strawberry fruit ripening. Plant Physiol. 157, 188–199. doi: 10.1104/pp.111.177311
Jia, M., Ding, N., Zhang, Q., Xing, S., Wei, L., Zhao, Y., et al. (2017b). A FERONIA-like receptor kinase regulates strawberry (Fragaria × ananassa) fruit ripening and quality formation. Front. Plant Sci. 8:1099. doi: 10.3389/fpls.2017.01099
Jia, H., Jiu, S., Zhang, C., Wang, C., Tariq, P., Liu, Z., et al. (2016). Abscisic acid and sucrose regulate tomato and strawberry fruit ripening through the abscisic acid-stress-ripening transcription factor. Plant Biotechnol. J. 14, 2045–2065. doi: 10.1111/pbi.12563
Jia, H. F., Lu, D., Sun, J. H., Li, C. L., Xing, Y., Qin, L., et al. (2013a). Type 2C protein phosphatase ABI1 is a negative regulator of strawberry fruit ripening. J. Exp. Bot. 64, 1677–1687. doi: 10.1093/jxb/ert028
Jia, H. F., Wang, Y. H., Sun, M. Z., Li, B. B., Han, Y., Zhan, Y. X., et al. (2013b). Sucrose functions as a signal involved in the regulation of strawberry fruit development and ripening. New Phytol. 198, 453–465. doi: 10.1111/nph.12176
Jia, H., Xie, Z., Wang, C., Shangguan, L., Qian, N., Cui, M., et al. (2017a). Abscisic acid, sucrose, and auxin coordinately regulate berry ripening process of the Fujiminori grape. Funct. Integr. Genomics 17, 441–457. doi: 10.1007/s10142-017-0546-z
Jiao, B., Meng, Q., and Lv, W. (2020). Roles of stay-green (SGR) homologs during chlorophyll degradation in green plants. Bot. Stud. 61:25. doi: 10.1186/s40529-020-00302-5
Kadomura-Ishikawa, Y., Miyawaki, K., Takahashi, A., Masuda, T., and Noji, S. (2015). Light and abscisic acid independently regulated FaMYB10 in Fragaria × ananassa fruit. Planta 241, 953–965. doi: 10.1007/s00425-014-2228-6
Kai, W., Wang, J., Liang, B., Fu, Y., Zheng, Y., Zhang, W., et al. (2019). PYL9 is involved in the regulation of ABA signaling during tomato fruit ripening. J. Exp. Bot. 70, 6305–6319. doi: 10.1093/jxb/erz396
Karppinen, K., Tegelberg, P., Häggman, H., and Jaakola, L. (2018). Abscisic acid regulates anthocyanin biosynthesis and gene expression associated with cell wall modification in ripening bilberry (Vaccinium myrtillus L.) fruits. Front. Plant Sci. 9:1259. doi: 10.3389/fpls.2018.01259
Kim, J., Lee, J. G., Hong, Y., and Lee, E. J. (2019). Analysis of eight phytohormone concentrations, expression levels of ABA biosynthesis genes, and ripening-related transcription factors during fruit development in strawberry. J. Plant Physiol. 239, 52–60. doi: 10.1016/j.jplph.2019.05.013
Kou, X., He, Y., Li, Y., Chen, X., Feng, Y., and Xue, Z. (2019). Effect of abscisic acid (ABA) and chitosan/nano-silica/sodium alginate composite film on the color development and quality of postharvest Chinese winter jujube (Zizyphus jujuba Mill. cv. Dongzao). Food Chem. 270, 385–394. doi: 10.1016/j.foodchem.2018.06.151
Kumar, V., Irfan, M., Ghosh, S., Chakraborty, N., Chakraborty, S., and Datta, A. (2016). Fruit ripening mutants reveal cell metabolism and redox state during ripening. Protoplasma 253, 581–594. doi: 10.1007/s00709-015-0836-z
Kumar, R., Khurana, A., and Sharma, A. K. (2014). Role of plant hormones and their interplay in development and ripening of fleshy fruits. J. Exp. Bot. 65, 4561–4575. doi: 10.1093/jxb/eru277
Li, C. L., Jia, H. F., Chai, Y. M., and Shen, Y. Y. (2011). Abscisic acid perception and signaling transduction in strawberry: a model for non-climacteric fruit ripening. Plant Signal. Behav. 6, 1950–1953. doi: 10.4161/psb.6.12.18024
Li, Z., Wang, Z., Wang, K., Liu, Y., Hong, Y., Chen, C., et al. (2020). Co-expression network analysis uncovers key candidate genes related to the regulation of volatile esters accumulation in woodland strawberry. Planta 252:55. doi: 10.1007/s00425-020-03462-7
Li, G., Zhao, J., Qin, B., Yin, Y., An, W., Mu, Z., et al. (2019). ABA mediates development-dependent anthocyanin biosynthesis and fruit coloration in Lycium plants. BMC Plant Biol. 19:317. doi: 10.1186/s12870-019-1931-7
Liang, S., Lu, K., Wu, Z., Jiang, S. C., Yu, Y. T., Bi, C., et al. (2015). A link between magnesium-chelatase H subunit and sucrose nonfermenting 1 (SNF1)-related protein kinase SnRK2.6/OST1 in Arabidopsis guard cell signalling in response to abscisic acid. J. Exp. Bot. 66, 6355–6369. doi: 10.1093/jxb/erv341
Liao, X., Li, M., Liu, B., Yan, M., Yu, X., Zi, H., et al. (2018). Interlinked regulatory loops of ABA catabolism and biosynthesis coordinate fruit growth and ripening in woodland strawberry. Proc. Natl. Acad. Sci. U. S. A. 115, E11542–E11550. doi: 10.1073/pnas.1812575115
Liu, M., Pirrello, J., Chervin, C., Roustan, J. P., and Bouzayen, M. (2015). Ethylene control of fruit ripening: revisiting the complex network of transcriptional regulation. Plant Physiol. 169, 2380–2390. doi: 10.1104/pp.15.01361
Luo, Y., Ge, C., Ling, Y., Mo, F., Yang, M., Jiang, L., et al. (2020a). ABA and sucrose co-regulate strawberry fruit ripening and show inhibition of glycolysis. Mol. Gen. Genomics. 295, 421–438. doi: 10.1007/s00438-019-01629-w
Luo, Y., Ge, C., Yang, M., Long, Y., Li, M., Zhang, Y., et al. (2020b). Cytosolic/plastid glyceraldehyde-3-phosphate dehydrogenase is a negative regulator of strawberry fruit ripening. Genes (Basel) 11:580. doi: 10.3390/genes11050580
Medina-Puche, L., Blanco-Portales, R., Molina-Hidalgo, F. J., Cumplido-Laso, G., García-Caparrós, N., Moyano-Cañete, E., et al. (2016). Extensive transcriptomic studies on the roles played by abscisic acid and auxins in the development and ripening of strawberry fruits. Funct. Integr. Genomics 16, 671–692. doi: 10.1007/s10142-016-0510-3
Meyer, M. D., Chope, G. A., and Terry, L. A. (2017). Investigation into the role of endogenous abscisic acid during ripening of imported avocado cv. Hass. J. Sci. Food Agric. 97, 3656–3664. doi: 10.1002/jsfa.8225
Mo, A., Xu, T., Bai, Q., Shen, Y., Gao, F., and Guo, J. (2020). FaPAO5 regulates Spm/Spd levels as a signaling during strawberry fruit ripening. Plant Direct. 4:e00217. doi: 10.1002/pld3.217
Molina-Hidalgo, F. J., Franco, A. R., Villatoro, C., Medina-Puche, L., Mercado, J. A., Hidalgo, M. A., et al. (2013). The strawberry (Fragaria × ananassa) fruit-specific rhamnogalacturonate lyase 1 (FaRGLyase1) gene encodes an enzyme involved in the degradation of cell-wall middle lamellae. J. Exp. Bot. 64, 1471–1483. doi: 10.1093/jxb/ers386
Molina-Hidalgo, F. J., Medina-Puche, L., Gelis, S., Ramos, J., Sabir, F., Soveral, G., et al. (2015). Functional characterization of FaNIP1; 1 gene, a ripening-related and receptacle-specific aquaporin in strawberry fruit. Plant Sci. 238, 198–211. doi: 10.1016/j.plantsci.2015.06.013
Mou, W., Li, D., Bu, J., Jiang, Y., Khan, Z. U., Luo, Z., et al. (2016). Comprehensive analysis of ABA effects on ethylene biosynthesis and signaling during tomato fruit ripening. PLoS One 11:e0154072. doi: 10.1371/journal.pone.0154072
Mou, W., Li, D., Luo, Z., Li, L., Mao, L., and Ying, T. (2018). SlAREB1 transcriptional activation of NOR is involved in abscisic acid-modulated ethylene biosynthesis during tomato fruit ripening. Plant Sci. 276, 239–249. doi: 10.1016/j.plantsci.2018.07.015
Oh, H. D., Yu, D. J., Chung, S. W., Chea, S., and Lee, H. J. (2018). Abscisic acid stimulates anthocyanin accumulation in ‘Jersey’ highbush blueberry fruits during ripening. Food Chem. 244, 403–407. doi: 10.1016/j.foodchem.2017.10.051
Paniagua, C., Blanco-Portales, R., Barcelo-Munoz, M., Garcia-Gago, J. A., Waldron, K. W., Quesada, M. A., et al. (2016). Antisense down-regulation of the strawberry beta-galactosidase gene FabetaGal4 increases cell wall galactose levels and reduces fruit softening. J. Exp. Bot. 67, 619–631. doi: 10.1093/jxb/erv462
Paul, V., Pandey, R., and Srivastava, G. C. (2012). The fading distinctions between classical patterns of ripening in climacteric and non-climacteric fruit and the ubiquity of ethylene-An overview. J. Food Sci. Technol. 49, 1–21. doi: 10.1007/s13197-011-0293-4
Romero, P., Lafuente, M. T., and Rodrigo, M. J. (2019). A sweet orange mutant impaired in carotenoid biosynthesis and reduced ABA levels results in altered molecular responses along peel ripening. Sci. Rep. 9:9813. doi: 10.1038/s41598-019-46365-8
Sadka, A., Qin, Q., Feng, J., Farcuh, M., Shlizerman, L., Zhang, Y., et al. (2019). Ethylene response of plum ACC synthase 1 (ACS1) promoter is mediated through the binding site of abscisic acid insensitive 5 (ABI5). Plants (Basel). 8:117. doi: 10.3390/plants8050117
Shang, Y., Yan, L., Liu, Z. Q., Cao, Z., Mei, C., Xin, Q., et al. (2010). The Mg-chelatase H subunit of Arabidopsis antagonizes a group of transcription repressors to relieve ABA-responsive genes of inhibition. Plant Cell 22, 1909–1935. doi: 10.1105/tpc.110.073874
Shen, X., Guo, X., Zhao, D., Zhang, Q., Jiang, Y., Wang, Y., et al. (2017). Cloning and expression profiling of the PacSnRK2 and PacPP2C gene families during fruit development, ABA treatment, and dehydration stress in sweet cherry. Plant Physiol. Biochem. 119, 275–285. doi: 10.1016/j.plaphy.2017.08.025
Shen, Y. Y., and Rose, J. K. C. (2014). “ABA metabolism and signaling in fleshy fruits” in Abscisic acid: Metabolism, transport and signaling. ed. D. P. Zhang (Dordrecht: Springer), 271–286.
Siebeneichler, T. J., Crizel, R. L., Camozatto, G. H., Paim, B. T., da Silva, M. R., Rombaldi, C. V., et al. (2020). The postharvest ripening of strawberry fruits induced by abscisic acid and sucrose differs from their in vivo ripening. Food Chem. 317:126407. doi: 10.1016/j.foodchem.2020.126407
Sun, Y., Ji, K., Liang, B., Du, Y., Jiang, L., Wang, J., et al. (2017). Suppressing ABA uridine diphosphate glucosyltransferase (SlUGT75C1) alters fruit ripening and the stress response in tomato. Plant J. 91, 574–589. doi: 10.1111/tpj.13588
Sun, L., Sun, Y., Zhang, M., Wang, L., Ren, J., Cui, M., et al. (2012b). Suppression of 9-cis-epoxycarotenoid dioxygenase, which encodes a key enzyme in abscisic acid biosynthesis, alters fruit texture in transgenic tomato. Plant Physiol. 158, 283–298. doi: 10.1104/pp.111.186866
Sun, L., Yuan, B., Zhang, M., Wang, L., Cui, M., Wang, Q., et al. (2012a). Fruit-specific RNAi-mediated suppression of SlNCED1 increases both lycopene and β-carotene contents in tomato fruit. J. Exp. Bot. 63, 3097–3108. doi: 10.1093/jxb/ers026
Symons, G. M., Chua, Y. J., Ross, J. J., Quittenden, L. J., Davies, N. W., and Reid, J. B. (2012). Hormonal changes during non-climacteric ripening in strawberry. J. Exp. Bot. 63, 4741–4750. doi: 10.1093/jxb/ers147
Tijero, V., Teribia, N., Muñoz, P., and Munné-Bosch, S. (2016). Implication of Abscisic acid on ripening and quality in sweet cherries: differential effects during pre- and post-harvest. Front. Plant Sci. 7:602. doi: 10.3389/fpls.2016.00602
Tosetti, R., Elmi, F., Pradas, I., Cools, K., and Terry, L. A. (2020). Continuous exposure to ethylene differentially affects senescence in receptacle and achene tissues in strawberry fruit. Front. Plant Sci. 11:174. doi: 10.3389/fpls.2020.00174
Villalobos-González, L., Peña-Neira, A., Ibáñez, F., and Pastenes, C. (2016). Long-term effects of abscisic acid (ABA) on the grape berry phenylpropanoid pathway: gene expression and metabolite content. Plant Physiol. Biochem. 105, 213–223. doi: 10.1016/j.plaphy.2016.04.012
Wang, Y., Guo, S., Tian, S., Zhang, J., Ren, Y., Sun, H., et al. (2017b). Abscisic acid pathway involved in the regulation of watermelon fruit ripening and quality trait evolution. PLoS One 12:e0179944. doi: 10.1371/journal.pone.0179944
Wang, X., Liu, S., Tian, H., Wang, S., and Chen, J. G. (2015). The small ethylene response factor ERF96 is involved in the regulation of the abscisic acid response in Arabidopsis. Front. Plant Sci. 6:1064. doi: 10.3389/fpls.2015.01064
Wang, S., Saito, T., Ohkawa, K., Ohara, H., Suktawee, S., Ikeura, H., et al. (2018). Abscisic acid is involved in aromatic ester biosynthesis related with ethylene in green apples. J. Plant Physiol. 221, 85–93. doi: 10.1016/j.jplph.2017.12.007
Wang, X., Zeng, W., Ding, Y., Wang, Y., Niu, L., Yao, J. L., et al. (2019a). PpERF3 positively regulates ABA biosynthesis by activating PpNCED2/3 transcription during fruit ripening in peach. Hortic Res. 6:19. doi: 10.1038/s41438-018-0094-2
Wang, X., Zeng, W., Ding, Y., Wang, Y., Niu, L., Yao, J. L., et al. (2019b). Peach ethylene response factor PpeERF2 represses the expression of ABA biosynthesis and cell wall degradation genes during fruit ripening. Plant Sci. 283, 116–126. doi: 10.1016/j.plantsci.2019.02.009
Wang, Q. H., Zhao, C., Zhang, M., Li, Y. Z., Shen, Y. Y., and Guo, J. X. (2017a). Transcriptome analysis around the onset of strawberry fruit ripening uncovers an important role of oxidative phosphorylation in ripening. Sci. Rep. 7:41477. doi: 10.1038/srep41477
Wong, D. C., Lopez Gutierrez, R., Dimopoulos, N., Gambetta, G. A., and Castellarin, S. D. (2016). Combined physiological, transcriptome, and cis-regulatory element analyses indicate that key aspects of ripening, metabolism, and transcriptional program in grapes (Vitis vinifera L.) are differentially modulated accordingly to fruit size. BMC Genomics 17:416. doi: 10.1186/s12864-016-2660-z
Wu, Q., Tao, X., Ai, X., Luo, Z., Mao, L., Ying, T., et al. (2018). Contribution of abscisic acid to aromatic volatiles in cherry tomato (Solanum lycopersicum L.) fruit during postharvest ripening. Plant Physiol. Biochem. 130, 205–214. doi: 10.1016/j.plaphy.2018.06.039
Yakir, E., Zhangjun, F., Sela, N., Xu, Y., Singh, V., Dagar, A., et al. (2018). MaMADS2 repression in banana fruits modifies hormone synthesis and signalling pathways prior to climacteric stage. BMC Plant Biol. 18:267. doi: 10.1186/s12870-018-1480-5
Yu, Y., Meng, X., Guo, D., Yang, S., Zhang, G., and Liang, Z. (2020). Grapevine U-box E3 ubiquitin ligase VlPUB38 negatively regulates fruit ripening by facilitating abscisic-aldehyde oxidase degradation. Plant Cell Physiol. pcaa118. doi: 10.1093/pcp/pcaa118 [Epub ahead of print]
Zhang, S. H., Hou, B. Z., Chai, L., Yang, A. Z., Yu, X. Y., and Shen, Y. Y. (2017). Sigma factor FaSigE positively regulates strawberry fruit ripening by ABA. Plant Growth Regul. 83, 417–427. doi: 10.1007/s10725-017-0308-x
Zhang, Z., Kang, C., Zhang, S., and Li, X. (2019). Transcript analyses reveal a comprehensive role of abscisic acid in modulating fruit ripening in Chinese jujube. BMC Plant Biol. 19:189. doi: 10.1186/s12870-019-1802-2
Zhang, Y., Li, Q., Jiang, L., Kai, W., Liang, B., Wang, J., et al. (2018). Suppressing type 2C protein phosphatases alters fruit ripening and the stress response in tomato. Plant Cell Physiol. 59, 142–154. doi: 10.1093/pcp/pcx169
Zhang, M., Yuan, B., and Leng, P. (2009). The role of ABA in triggering ethylene biosynthesis and ripening of tomato fruit. J. Exp. Bot. 60, 1579–1588. doi: 10.1093/jxb/erp026
Zhu, X., Chen, J., Qiu, K., and Kuai, B. (2017). Phytohormone and light regulation of chlorophyll degradation. Front. Plant Sci. 8:1911. doi: 10.3389/fpls.2017.01911
Zhu, F., Luo, T., Liu, C., Wang, Y., Zheng, L., Xiao, X., et al. (2020). A NAC transcription factor and its interaction protein hinder abscisic acid biosynthesis by synergistically repressing NCED5 in Citrus reticulata. J. Exp. Bot. 71, 3613–3625. doi: 10.1093/jxb/eraa118
Keywords: fruit ripening, non-climacteric fruit, abscisic acid, ethylene, signaling transduction, review
Citation: Bai Q, Huang Y and Shen Y (2021) The Physiological and Molecular Mechanism of Abscisic Acid in Regulation of Fleshy Fruit Ripening. Front. Plant Sci. 11:619953. doi: 10.3389/fpls.2020.619953
Edited by:
Chunying Kang, Huazhong Agricultural University, ChinaCopyright © 2021 Bai, Huang and Shen. This is an open-access article distributed under the terms of the Creative Commons Attribution License (CC BY). The use, distribution or reproduction in other forums is permitted, provided the original author(s) and the copyright owner(s) are credited and that the original publication in this journal is cited, in accordance with accepted academic practice. No use, distribution or reproduction is permitted which does not comply with these terms.
*Correspondence: Yuanyue Shen, c2ZsbW5AMTYzLmNvbQ==; Yun Huang, eXVuaHVhbmdAYnVhLmVkdS5jbg==