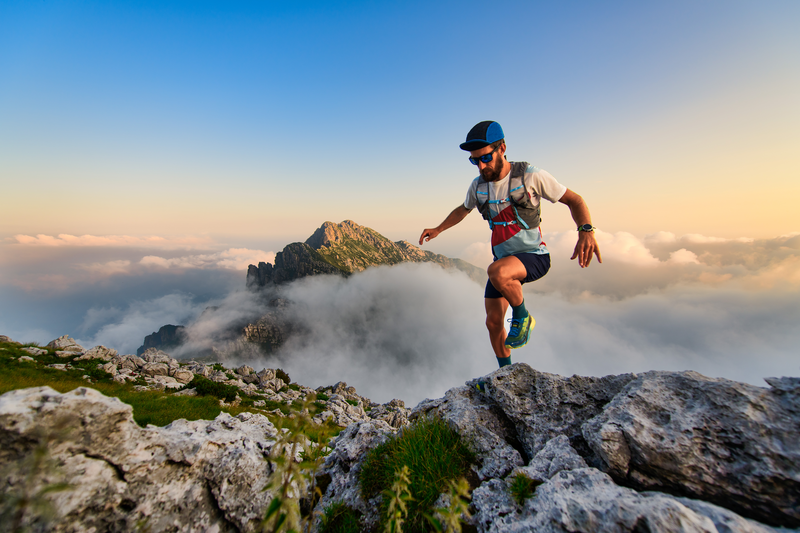
94% of researchers rate our articles as excellent or good
Learn more about the work of our research integrity team to safeguard the quality of each article we publish.
Find out more
ORIGINAL RESEARCH article
Front. Plant Sci. , 30 October 2020
Sec. Plant Nutrition
Volume 11 - 2020 | https://doi.org/10.3389/fpls.2020.568887
This article is part of the Research Topic Heavy Metal Toxicity in Plants: Recent Insights on Physiological and Molecular Aspects View all 14 articles
Hyperaccumulators are the preferred materials for phytoremediation. Sedum alfredii Hance is a cadmium (Cd) hyperaccumulator plant in China, although its detoxification mechanism remains unresolved. In our study, we cloned a gene belonging to the plant cadmium resistance (PCR) family, named SaPCR2, from the hyperaccumulating ecotype (HE) of S. alfredii. Sequence analysis indicated that SaPCR2 contained a cysteine-rich domain highly conserved in the PCR family and played an important role in Cd detoxification. Based on the relative quantitative results, SaPCR2 was highly expressed in the roots of HE S. alfredii, but not the shoots and Cd exposure did not significantly affect SaPCR2 expression. In contrast, the expression level of SaPCR2 was very low in plants of its non-hyperaccumulating ecotype (NHE). The subcellular localization of SaPCR2 in tobacco leaves and yeasts showed that SaPCR2 was localized on the plasma membrane and the expression of the SaPCR2 protein in a Zn/Cd-sensitive yeast Δzrc1 significantly increased its tolerance to Cd stress by decreasing the Cd content in cells. Heterologous expression of SaPCR2 in plants of both Arabidopsis thaliana and NHE S. alfredii significantly reduced the Cd levels in the roots, but not in the shoots. These results suggest that the overexpression of SaPCR2 in plants provides a route for Cd leak out of the root cells and protects the root cells against phytotoxicity of Cd stress. To the best of our knowledge, this is the first study of transporter-mediated root efflux of Cd in hyperaccumulator S. alfredii.
Cadmium (Cd) is one of the most toxic elements for plants, animals, and human beings. High Cd exposure to crops via the biogeochemical behavior of soil-plant systems significantly threatens human health (Godt et al., 2006; Marisela Fernandez-Valverde et al., 2010; Shahid et al., 2017). During the past few decades, heavy metals (including Cd) have become the greatest pollutants worldwide due to the rapid development of the chemical industry, metallurgy, and electroplating (Hu et al., 2016). For instance, Cd is reported to be the most severe pollutant for agricultural soils in China (Li et al., 2014; Zhao et al., 2015; Shi et al., 2019). Phytoremediation of heavy metals is an efficient and economical soil remediation technique to clear or alleviate heavy metal pollution in soils (Liu et al., 2018). Hyperaccumulators can accumulate 100–1,000-fold higher heavy metals than normal plants without toxic consequences (Rascio and Navari-Izzo, 2011) and, therefore, are of high scientific interest as a representative plant material to extract heavy metals with great ability (Pollard et al., 2014).
To grow healthily in highly contaminated soils, a sophisticated detoxification system must exist in the plants of hyperaccumulators, especially for the root systems, which suffer first from heavy metal toxicity. Therefore, high root tolerance is the precondition for hyperaccumulating plants to grow well under high Cd stress. Roots of hyperaccumulators might present superior antioxidative defenses under Cd condition (Boominathan and Doran, 2003; Chiang et al., 2006; Wu et al., 2015) or sequestrated Cd in vacuoles and cell walls (Fu et al., 2011; Parrotta et al., 2015). However, the most important strategy for the root resistance of hyperaccumulators is to efflux Cd out of the root cells and subsequently translocate to the aboveground parts of plants. In hyperaccumulator Noccaea caerulescens, the great ability of xylem loading is one of the main processes participating in Cd hyperaccumulation (Sterckeman et al., 2015). High expression of HMA4 in Arabidopsis halleri supports the enhanced metal flux from the root symplasm into the xylem vessels necessary for shoot metal hyperaccumulation (Hanikenne et al., 2008). This is different from the strategies of some regular or resistant plants to prevent heavy metals from accumulating in plants. For example, OsZIP1 can function as a metal exporter in rice when zinc (Zn), copper (Cu), and Cd are present in excess in the environment and decrease the metal accumulation in plants (Liu et al., 2019). Therefore, the investigation of transporters involved in the efflux of Cd in the roots of hyperaccumulators may facilitate our understanding of metal tolerance in roots as well as its hyperaccumulation in shoots.
Plant cadmium resistance (PCR) family transporters, especially PCR1 and PCR2, are involved in the efflux of Cd and other bivalent cations from cells to the outside, mainly via the lateral transport and xylem loading in the roots. The first PCR family gene found in A. thaliana, named AtPCR1, showed a strong tolerance for Cd in the selection of Cd-tolerant genes of A. thaliana using yeast Cd-sensitive mutants (Song et al., 2004). The study of OsPCR1 in rice showed that the OsPCR1 knockdown lines decreased the weight and Cd content of grains (Song et al., 2015). AtPCR2 is mainly distributed on the epidermis of the root hair zone, as well as the vascular and epidermal cells of the elongation zone, which plays a very important role in the translocation of Zn (Song et al., 2010). In Brassica juncea, BjPCR1 was found to be localized on the plasma membrane of the root epidermal cells at the subcellular level and is mainly responsible for the absorption and root-to-shoot transportation of calcium (Ca) (Song et al., 2011). However, to the best of our knowledge, no study has been undertaken on the function of the PCR family protein in hyperaccumulating plants.
Sedum alfredii Hance, a Chinese native Zn/Cd hyperaccumulator belonging to the Crassulaceae family (Yang et al., 2004, 2006), has a high capacity for tolerating, translocating, and accumulating high amounts of Cd (Lu et al., 2008; Tian et al., 2011, 2017). The Cd concentration in the shoots of HE S. alfredii is as high as 9,000 mg/kg (Yang et al., 2004). However, the molecular mechanisms involved in root resistance and translocation of Cd remain unknown. In the present study, a high expression gene belonging to the PCR family, named SaPCR2, was cloned from this plant species and the functions of this gene were investigated.
The hyperaccumulating ecotype (HE) S. alfredii and its non-hyperaccumulating ecotype (NHE) were originally collected from a Zn/Cd mine in Quzhou (Zhejiang province, China) and tea plantation in Hangzhou (Zhejiang province, China), respectively. The plant materials used in this experiment were cultured after several generations of asexual cuttings. The same seedlings were cut and exposed to deionized water until rooting and were then added to 1/4, 1/2, and full nutrient solution gradually, which was replaced every 3 days. The formulation of the full nutrient solution contained 2.0 mM Ca(NO3)2, 0.7 mM K2SO4, 0.1 mM KH2PO4, 0.1 mM KCl, 0.5 mM MgSO4, 20 μM Fe-EDTA, 5 μM ZnSO4, 10 μM H3BO3, 5 μM ZnSO4, 0.5 μM MnSO4, 0.2 μM CuSO4, and 0.01 μM (NH4)6Mo7O24. The temperature of incubation was set at 25–28°C, with a 16 h light/8 h dark cycle. Three-week-old seedlings were used in subsequent experiments.
The results of the previous transcriptome sequence of HE S. alfredii illustrated that the sequence (Sa_Contig10958, GenBank: HE728063) was predicted to encode the gene from the PCR family (Gao et al., 2013). According to this sequence (Sa_Contig10958), we designed RACE primers to clone the full-length sequence from HE and NHE S. alfredii using the Smart RACE cDNA amplification kit (Clontech). The cDNA sequences of SaPCR2 are the same in two ecotypes (Supplementary Figure S1), so we named this gene as SaPCR2. The primer used for SaPCR2 5′-RACE was 5′-CACGGAGACTCTTGTAGATCATAC-3′ and the primer used for SaPCR2 3′-RACE was 5′-TGCCATCTACGGCCTGATTT-3′. The transmembrane domain of SaPCR2 was predicted using the TMHMM Online analysis tool1. The protein sequence alignment analysis was compared with ClustalX. The phylogenetic tree analysis was performed using MEGA5.0 with the neighbor-joining method.
Two ecological types of S. alfredii were treated by CdCl2 with different concentrations (HE: 10 and 100 μM, NHE: 10 μM) and times (0, 6, 24 h, 3, and 7 days). The roots, stems, and leaves of each plant were separated and frozen rapidly in liquid nitrogen. The total RNA was extracted using a Spin Column Plant Total RNA Purification Kit (Sangon) and then synthesized to cDNA with a HiScript II Q RT SuperMix for qPCR (+ gDNA wiper) (Vazyme). Real-time quantitative PCR (RT-qPCR) was performed using a ChamQ SYBR Color qPCR Master Mix (Without ROX) (Vazyme), with LightCycler 480 System (Roche, United States). The RT-qPCR protocol was as follows: 95°C for 3 min, 40 cycles of 95°C for 10 s, 60°C for 30 s. The melting-curve analysis was included to verify the specificity of the primer. The mean amplification efficiency was analyzed with the LinReg software (Ruijter et al., 2009). The specific primers for RT-qPCR were designed according to the SaPCR2 sequence as follows: SaPCR2 forward 5′-GCGGTGGGATGTGGTCTAC-3′ and SaPCR2 reverse 5′-CGATAATCTCGGCTATTTGGC-3′, SaACTIN1 forward 5′-TGTGCTTTCCCTCTATGCC-3′, and reverse: 5′-CGCTCAGCAGTGGTTGTG-3′ (Chao et al., 2010). The relative expression levels were calculated using 2−ΔCt method.
All the SaPCR2 sequences for reconstruction were amplified using the ClonExpress II One Step Cloning Kit (Vazyme), and the primers used are listed in Table 1. To generate yeast expression vectors, the open reading frame of SaPCR2 was cloned into the SpeI and EcoRI sites of the pDR196 (pDR196-SaPCR2 vector), which contained a uracil amino acid selection marker. To produce the green fluorescent protein (GFP)-fused SaPCR2 expression vector (35Spro-SaPCR2-GFP vector) for transient expression in tobacco, the open reading frame of SaPCR2 sequence without stop codon was cloned into the pCAMBIA 1300-eGFP vector between the KpnI and BamHI sites, which was controlled by the cauliflower mosaic virus (CaMV) 35S promoter. Taking pCAMBIA 1300 as the plant overexpression vector, we cloned the full-length SaPCR2 into the AccI and XbaI restriction sites.
The tobacco leaves were used to observe the subcellular localization of SaPCR2. Agrobacterium tumefaciens GV3101 monoclonal containing the 35Spro-SaPCR2-GFP vector was picked, activated, and suspended in 10 mM MgCl2 and 10 mM MES (pH = 5.6). The final OD600 of the bacteria concentration was adjusted to approximately 0.4 and it was then injected into the epidermis of the tobacco leaves using a needleless syringe, cultured for 24–36 h. The epidermis was treated with 0.8 M mannitol for at least 10 min to induce plasmolysis and the fluorescence was detected using a confocal microscopy (LSM700, Carl Zeiss, Germany). FM 4–64 fluorescence was used as a plasma membrane marker.
To express the SaPCR2 in yeast, the pDR196-SaPCR2 vector or pDR196 empty vector were transformed into the wild type BY4743 and Zn/Cd-sensitive mutant Δzrc1 (MAT1; his3; leu2; met15; ura3; YMR243c: kanMX4) using the LiAc/PEG/ssDNA method (Gietz and Schiestl, 2007). The positive strains were screened out by selected synthetic dropout (SD) solid media (absence of uracil amino acid) and verified by PCR with specific primers: forward 5′-GCGGTGGGATGTGGTCTAC-3′ and reverse 5′-CGATAATCTCGGCTATTTGGC-3′. For Cd tolerance assay, yeast cells were cultured in selected liquid SD until OD600 = 1. Then, 5 μL serial dilutions (OD600 = 1.0, 0.1, 0.01, and 0.001) were spotted on SD with 0, 10, and 60 μM CdCl2 at 30°C. Photographs were taken after 3-days incubation. For Cd accumulation testing, the yeast was cultured overnight to OD600 = 0.1 and then added to the SD liquid medium with 2.5, 5.0, and 10.0 μM CdCl2 for 24 h at 30°C. The yeast cells were collected via centrifugation and washed three times with ultra-pure water. The cells were dried for 2 days at 85°C and digested in HNO3 to determine the heavy metal concentration in the yeast strains by inductively coupled plasma mass spectrometry (Agilent, United States).
To investigate SaPCR2 in S. alfredii, the transgenic system of NHE S. alfredii was constructed. The seeds of NHE S. alfredii were sterilized in 0.1% HgCl2 for 5 min and washed with sterile water five to six times and were then spotted on 1/2 Murashige and Skoog (MS) medium (Murashige and Skoog, 1962). After approximately 8 weeks, young stems of the aseptic seedlings were cut into approximately 1–2 cm and transferred to callus-inducing medium (Table 2) with 6-benzylaminopurine (6-BA) and 1.0 mg/L 2,4-dichlorophenoxyacetic acid (2,4-D). When the stem segments appeared to be differentiated and enlarged, A. tumefaciens GV3101 (OD600 = 0.6) was used for transfection. Then the explants were co-cultured with Ag. tumefaciens containing the 35Spro-SaPCR2 vector for 2 days and then removed to the selection medium (Table 2). The hyg-resistant explants were moved to differential medium (Table 2). After emergence, the medium was changed to 1/2 MS-agar medium. Then, the well-rooted plants were moved to nutrient solution for subsequent experiments. SaPCR2 overexpressing A. thaliana was obtained using the Agrobacterium-mediated floral dip method (Clough and Bent, 1998).
The total plant genomic DNA was extracted from the fresh leaves of transgenic plants and their wild type using the CTAB method, identified by the following primers: forward 5′-ATGTATCCATCTTTGTCTG-3′ and reverse 5′- TCATCTACTCATCCCCTGC-3′. The expression level of SaPCR2 in the transgenic NHE S. alfredii was investigated by RT-qPCR as mentioned above. Two independent transgenic lines of NHE S. alfredii (SaPCR2-L1, SaPCR2-L2) and A. thaliana of T2 plants (SaPCR2-OX1, SaPCR2-OX2) were identified as SaPCR2 overexpressing lines for subsequent analysis.
The seeds of the transgenic A. thaliana were collected and spotted on 1/2 MS solid medium and then 4-days-old seedlings were transferred to nutrient agar plates with 15 or 30 μM CdCl2 for 7 days. There were 20 plants for one treatment and each treatment was repeated three times. Well-rooted transgenic NHE S. alfredii lines and their corresponding wild type plants in the same growth states were hydroponically cultured for 3 weeks and then treated with 10 μM CdCl2 for 5 days. For Cd concentration determination, all the plant samples were rinsed in 20 mM Na2-EDTA to remove excess Cd2+ attached to the surface and were then separated into roots, stems, and leaves for drying and digesting in HNO3-H2O2. The Cd concentration was analyzed using an inductively coupled plasma optical emission spectrometer (Agilent, United States).
The Cd uptake (μg/kg root FW) = Total Cd in the plants (μg)/Fresh weight of roots (kg).
The plants were exposed to 10 μM CdCl2 for 7 days. The plasma membrane integrity and lipid peroxidation of roots were investigated using the analysis of Evans blue uptake, MDA content, as well as Schiff’s reagent staining (Tian et al., 2012).
As an unessential element, Cd is suggested to enter/exit plant cells through the transporters of bivalent cations for nutrient elements, such as Zn/Mn/Fe (Ueno et al., 2008; Willems et al., 2010; Sasaki et al., 2012). The PCR family has been confirmed as membrane transporters not only for the transportation of Cd, but also for Zn, Fe, and Ca (Song et al., 2004, 2010, 2011). In this study, the putative PCR gene was cloned from S. alfredii according to the published transcription sequence (Gao et al., 2013). Phylogenetic analysis revealed that the putative PCR amino acid sequence was 44.68% similar to AtPCR2 belonging to A. thaliana (Figure 1A). Therefore, we named this PCR transporter SaPCR2. The SaPCR2 protein sequence contained 184 amino acids and a transmembrane domain predicted with the TMHMM Online analysis tool (Figure 1B). A multiple sequence alignment with PCR from A. thaliana was undertaken, showing that the SaPCR2 protein exhibited the same familial signature (Figure 1C). Analysis of the amino acid sequences showed that SaPCR2 contained a highly conserved CCXXXXCPC domain. This small cysteine-rich protein may play an important role in the detoxification mechanisms of Cd (Song et al., 2004). Therefore, SaPCR2 identified in the HE S. alfredii may be of great importance in Cd transportation.
Figure 1. Sequence analysis of SaPCR2. (A) Phylogenic tree of SaPCR2 proteins in Arabidopsis thaliana, Oryza sativa, and Sedum alfredii. The 0.1 scale showed substitution distance. (B) SaPCR2 protein transmembrane topology prediction. (C) Amino acid sequence alignment of SaPCR2, AtPCR1, AtPCR2, and AtPCR3. Identical residues are shown in black, residues with a similarity over 70% are shown in red, and 50% or more are shown in blue. The fully conserved CCXXXXCPC domains are framed by the red box.
To understand the expression characteristics of SaPCR2 gene, we analyzed its expression in the roots and shoots of the two S. alfredii ecotypes using RT-qPCR. High expression of SaPCR2 was observed in the roots of the HE S. alfredii, but not in the shoots (Figure 2A). The expression level of SaPCR2 in the roots was approximately 33-fold higher than that in the stems of the HE (Figure 2A). This differed from that of AtPCR1, which was exclusively expressed in the aboveground parts of A. thaliana (Song et al., 2004), yet similar to that of BjPCR1 in Brassica juncea (Song et al., 2011). To investigate the effects of Cd exposure on SaPCR2 expression, we analyzed the variations in the SaPCR2 expression levels in the HE roots after different Cd exposure for 6 h to 7 days. Cd exposure did not result in any significant variation of the SaPCR2 expression levels in the HE roots (Figure 2B). Therefore, this gene is not induced by Cd stress. In plants of NHE S. alfredii, the SaPCR2 expression was not observed in either roots or shoots regardless of Cd treatments (Figure 2A).
Figure 2. Expression of SaPCR2 and subcellular location of SaPCR2. (A) Expression level of SaPCR2 in roots, stems, and leaves of two ecotypes (HE and NHE) of Sedum alfredii. (B) Expression levels of SaPCR2 in the roots of the HE S. alfredii at different time points. The plants were treated with 0, 10, or 100 μM CdCl2 for 6, 12, 24 h, 3, or 7 days. Error bars are ± SE of n = 3. Relative expression levels were normalized to the level of constitutively expressed gene SaACTIN1. ∗Indicates significant difference between ecotypes (p < 0.05). (C) Subcellular location of SaPCR2 in epidermal cells of tobacco. Distribution of green fluorescent protein (GFP)-SaPCR2 fusion protein and red fluorescent protein (plasma membrane marker) in tobacco leaf epidermis cells. Scale bar = 10 μm.
These results are similar to the transcript levels of several reported Cd-related transporters in plants of HE S. alfredii. The high expression of these genes may result from the adaption of high Cd exposure during long-term evolution. For example, SaHMA3 plays an essential role in Cd detoxification and its expression in HE S. alfredii was also significantly higher than that in NHE plants (Zhang et al., 2016), however, no significant difference was observed after Cd treatment (Liu et al., 2017). The high expression levels of these transporters in plants of HE S. alfredii may result from the long-term adaption and evolution of this plant species exposed to the high heavy metal levels in its natural habitat soils.
To determine the subcellular location of SaPCR2, GFP fused to SaPCR2 under the control of the CaMV 35S promoter (35Spro-SaPCR2-GFP) construct was generated for the transient expression in epidermal cells of tobacco leaves. The green fluorescence signal of the SaPCR2-GFP fusion protein was observed in the plasma membrane showing co-localization with the plasma membrane marker dye FM 4-64 (Figure 2C). When exposed to a high concentration of sucrose, the green fluorescence signal was in close to the cell wall, but not organelle membrane, which was similar with BjPCR1 (Song et al., 2011).
The effect of SaPCR2 on Cd resistance was assayed in Δzrc1 yeast strains. The SaPCR2 transcript was recombined in the yeast expression vector pDR196. In the absence of Cd, there was no significant difference between the transgenic yeast strains (Figure 3A). On the SD solid medium supplemented with 60 μM CdCl2, the growth of the empty vector (EV) and SaPCR2-expressing lines in the Δzrc1 backgrounds were inhibited. However, the Δzrc1 strain transfected with EV showed higher sensitivity to Cd than the Δzrc1 strain expressing SaPCR2 (Figure 3A). To test the Cd-transporting activity of SaPCR2, we determined the Cd concentration in the SaPCR2-expressing Δzrc1 mutant strain treated with Cd. When cultured in the presence of 2.5 or 5.0 μM CdCl2, SaPCR2-expressing Δzrc1 cells accumulated lower Cd than EV-transformed control cells (p < 0.05) (Figure 3B). These results indicate that SaPCR2 enhanced yeast Cd resistance by reducing Cd concentration in cells. Therefore, the SaPCR2 may function as a transporter at plasma membrance in the roots of HE S. alfredii, which mediates Cd reduction in cells.
Figure 3. Expressing SaPCR2 enhanced cadmium (Cd) tolerance in yeast mutant Δzrc1 by reducing Cd concentration. (A) The yeast strain BY4743 and Δzrc1 transformed with pDR196-SaPCR2 vector or pDR196 empty vector (EV) were diluted (OD600 = 1.0, 0.1, 0.01, and 0.001) and spotted on SD medium with 0, 10, and 60 μM CdCl2 for 3 days at 30°C. (B) Cd concentration in yeasts. Δzrc1 strains expressed EV and pDR196-SaPCR2 were cultured in SD liquid medium with 2.5, 5, and 10 μM CdCl2 for 24 h at 30°C. Error bars are ± SE of n = 4. ∗Indicates significant differences between Δzrc1-EV and Δzrc1-SaPCR2 (p < 0.05).
To further determine the effects of SaPCR2 under Cd stress, we overexpressed SaPCR2 in A. thaliana. Two lines transfected with SaPCR2 (OX1 and OX2) were selected for subsequent experiments (Supplementary Figure S2A). The root lengths of transgenic lines were similar to the wild type in each treatment (Supplementary Figures S2B,C). However, the overexpressed SaPCR2 significantly decreased Cd concentration in the roots compared with the wild type when plants were grown with 15 or 30 μM CdCl2 (p < 0.05) (Figure 4). The Cd accumulations in the roots of two independent overexpressing lines were 27–37% and 18–21% lower than the wild type, respectively (Figure 4A). By contrast, there was no significant difference in the accumulations of Cd in the shoots (Figure 4B). These results suggest that the overexpressed SaPCR2 decreased Cd accumulation in the roots of A. thaliana.
Figure 4. Cd concentration in SaPCR2 overexpressing Arabidopsis thaliana. Cd concentration of (A) roots and (B) shoots in wild type, OX1, and OX2. Plants were cultured on 1/2 MS solid medium with 15 and 30 μM CdCl2 for 7 days, every 20 plants for one treatment, each treatment repeated three times. Error bars are ± SD of n = 3. *Indicates significant difference between genotypes (p < 0.05).
Due to the much higher expression levels of SaPCR2 in HE plants than that of NHE, we generated SaPCR2 NHE S. alfredii overexpressing lines (L1 and L2) to test the effect of SaPCR2 in NHE plants under Cd exposure. Higher expression levels of SaPCR2 were observed in L1 and L2. Compared with the wild type, the transcript levels of the SaPCR2 in the roots of overexpressing lines were 109–114-fold higher (Supplementary Figures S3A,B). After culturing in nutrient solution with 10 μM CdCl2, the leaves of wild type (NHE S. alfredii) wilted and the biomass showed a downtrend (Supplementary Figure S3D), whereas the L1 and L2 plants showed little toxicity phenomenon, especially in the roots (Figure 5). The Cd concentrations in the roots of the wild type were significantly higher than those of the SaPCR2 overexpressing lines (p < 0.05) (Figure 6). In the stems, L2 contained approximately 26% lower concentrations than the wild type; however, there was no significant difference in the leaves (Figure 5). Therefore, the heterologous overexpressing of SaPCR2 significantly reduced Cd accumulation in the roots of the transgenic NHE S. alfredii, without translocating increased Cd to its shoots.
Figure 5. Cd-induced loss of plasma membrane integrity (A,C) and lipid peroxidation (B,D) in the root tips of SaPCR2 overexpressing NHE Sedum alfredii line (L1 and L2). The plants were cultured in nutrient solution with 10 μM CdCl2 for 7 days, each treatment was repeated three times. Error bars are ± SD of n = 3. **Indicates significant difference between genotypes (p < 0.01). Scale bar = 250 μm.
Figure 6. Cd concentration in the root, stem, and leaf of wild type and SaPCR2-overexpressed transgenic NHE Sedum alfredii lines (L1 and L2). The plants were cultured in nutrient solution with 10 μM CdCl2 for 5 days, each treatment was repeated three times. Error bars are ± SD of n = 3. *Indicates significant difference between genotypes (p < 0.05).
Currently, many studies on the physiological mechanisms of Cd tolerance in the roots of hyperaccumulators have been undertaken, which have shown three main mechanisms in the roots of HE S. alfredii that play an important role in Cd homeostasis, including (1) transportation: Cd could be transformed from root to shoot to avoid excess Cd accumulation in roots (Lu et al., 2008, 2009; Tian et al., 2017); (2) segmentation: HE S. alfredii can transfer Cd from the cytoplasm to the vacuole or cell wall (Tian et al., 2010, 2017); (3) chelation and anti-oxidation: small molecule compounds and intracellular Cd2+ are combined to reduce the ion concentration and the antioxidant system is activated to alleviate the Cd stress at the same time (Tian et al., 2012). Many transporters have been used to testify the mechanisms mentioned above. For example, ZIP, HMA, or YSL proteins are implicated in Cd transport across membranes (Gallego et al., 2012). P-type ATPases HMA2 and HMA4, localizing to the plasma membrane, play essential roles in controlling Cd translocation (Hussain et al., 2004; Wong and Cobbett, 2009; Nocito et al., 2011). CAL1 acts by chelating Cd in the cytosol and facilitating Cd secretion to extracellular spaces, thus lowering the cytosolic Cd concentration while driving long-distance Cd transport via xylem vessels (Luo et al., 2018). Therefore, in hyperaccumulating plants, the capacity to translocate Cd to the shoots is a comprehensively proved mechanism involved in the tolerance of the roots. However, further calculation showed that overexpressing A. thaliana and NHE S. alfredii lines of SaPCR2 had lower Cd uptake than the wild type (Figures 7, 8). Therefore, the Cd decrease in roots not only depends on the efficient translocation systems, but is also attributed to the limit of Cd uptake and accumulation. For plants, there are two approaches to reduce the Cd concentration in roots—rapid root-to-shoot translocation and efficient efflux out of plants, both of which essentially protect the roots from too much Cd poison. For instance, heavy metal P-type ATPase OsHMA6 in rice, which is likely a Cu efflux protein (Zou et al., 2020). Therefore, we proposed a mechanism that has not been reported in the mechanism of Cd tolerance in HE S. alfredii, “leaking Cd out of plants.”
Figure 7. Cd uptake in wild type and SaPCR2-overexpressed transgenic Arabidopsis thaliana lines (OX1 and OX2). The plants were cultured on 1/2 MS solid medium with 15 and 30 μM CdCl2 for 7 days, every 20 plants for one treatment, each treatment repeated three times. Error bars are ± SE of n = 3. Different letters indicate significant differences between genotypes (p < 0.05).
Figure 8. Cd uptake in wild type and SaPCR2-overexpressed transgenic NHE Sedum alfredii lines (L1 and L2). The plants were cultured in nutrient solution with 10 μM CdCl2 for 5 days, each treatment repeated three times. Error bars are ± SE of n = 3. ∗Indicates significant difference between genotypes (p < 0.05).
The present study identified a gene coding SaPCR2 from HE S. alfredii expressed in roots. This transporter was localized to the plasma membrane. Heterologous overexpression of SaPCR2 reduced Cd uptake and accumulation in plants. Therefore, these findings improve the Cd detoxification mechanisms of hyperaccumulators and may contribute to the development of phytoremediation and food safety in the future.
The datasets presented in this study can be found in online repositories. The names of the repository/repositories and accession number(s) can be found in the article/Supplementary Material.
XG, JL, JiaZ, and JieZ performed the experiments. JL, LL, and SC wrote and revised the manuscript. LL designed, supervised, and obtained funding for the project. All authors gave final approval for publication.
This work was supported by projects from the National Natural Science Foundation of China (41977130 and 31672235), a project from the National Key Research and Development Program of China (2016YFD0800401).
The authors declare that the research was conducted in the absence of any commercial or financial relationships that could be construed as a potential conflict of interest.
We sincerely thank Prof. Tian Shengke for providing the seeds of NHE S. alfredii and Editage (www.editage.cn) for language editing.
The Supplementary Material for this article can be found online at: https://www.frontiersin.org/articles/10.3389/fpls.2020.568887/full#supplementary-material
HE, hyperaccumulating ecotype; NHE, non-hyperaccumulating ecotype; PCR, Plant cadmium resistance.
Boominathan, R., and Doran, P. M. (2003). Cadmium tolerance and antioxidative defenses in hairy roots of the cadmium hyperaccumulator, Thlaspi caerulescens. Biotechnol. Bioeng. 83, 158–167. doi: 10.1002/bit.10656
Chao, Y. E., Zhang, M., Feng, Y., Yang, X. E., and Islam, E. (2010). cDNA-AFLP analysis of inducible gene expression in zinc hyperaccumulator Sedum alfredii Hance under zinc induction. Environ. Exp. Bot. 68, 107–112. doi: 10.1016/j.envexpbot.2009.11.013
Chiang, H. C., Lo, J. C., and Yeh, K. C. (2006). Genes associated with heavy metal tolerance and accumulation in Zn/Cd hyperaccumulator Arabidopsis halleri: a genomic survey with cDNA microarray. Environ. Sci. Technol. 40, 6792–6798. doi: 10.1021/es061432y
Clough, S. J., and Bent, A. F. (1998). Floral dip: a simplified method for Agrobacterium-mediated transformation of Arabidopsis thaliana. Plant J. 16, 735–743. doi: 10.1046/j.1365-313x.1998.00343.x
Fu, X., Dou, C., Chen, Y., Chen, X., Shi, J., Yu, M., et al. (2011). Subcellular distribution and chemical forms of cadmium in Phytolacca americana L. J. Hazard. Mater. 186, 103–107. doi: 10.1016/j.jhazmat.2010.10.122
Gallego, S. M., Pena, L. B., Barcia, R. A., Azpilicueta, C. E., Lannone, M. F., Rosales, E. P., et al. (2012). Unravelling cadmium toxicity and tolerance in plants: Insight into regulatory mechanisms. Environ. Exp. Bot. 83, 33–46. doi: 10.1016/j.envexpbot.2012.04.006
Gao, J., Sun, L., Yang, X., and Liu, J. X. (2013). Transcriptomic analysis of cadmium stress response in the heavy metal hyperaccumulator Sedum alfredii Hance. PLoS One 8:e64643. doi: 10.1371/journal.pone.0064643
Gietz, R. D., and Schiestl, R. H. (2007). High-efficiency yeast transformation using the LiAc/SS carrier DNA/PEG method. Nat. Protoc. 2, 31–34. doi: 10.1038/nprot.2007.13
Godt, J., Scheidig, F., Grosse-Siestrup, C., Esche, V., Brandenburg, P., Reich, A., et al. (2006). The toxicity of cadmium and resulting hazards for human health. J. Occup. Med. Toxicol. 1:22. doi: 10.1186/1745-6673-1-22
Hanikenne, M., Talke, I. N., Haydon, M. J., Lanz, C., Nolte, A., Motte, P., et al. (2008). Evolution of metal hyperaccumulation required cis-regulatory changes and triplication of HMA4. Nature 453, 391–U344. doi: 10.1038/nature06877
Hu, Y., Cheng, H., and Tao, S. (2016). The challenges and solutions for cadmium-contaminated rice in China: a critical review. Environ. Int. 92-93, 515–532. doi: 10.1016/j.envint.2016.04.042
Hussain, D., Haydon, M. J., Wang, Y., Wong, E., Sherson, S. M., Young, J., et al. (2004). P-Type ATPase heavy metal transporters with roles in essential zinc homeostasis in Arabidopsis. Plant Cell 16, 1327–1339. doi: 10.1105/tpc.020487
Li, Z., Ma, Z., van der Kuijp, T. J., Yuan, Z., and Huang, L. (2014). A review of soil heavy metal pollution from mines in China: pollution and health risk assessment. Sci. Total Environ. 468, 843–853. doi: 10.1016/j.scitotenv.2013.08.090
Liu, H., Zhao, H., Wu, L., Liu, A., Zhao, F.-J., and Xu, W. (2017). Heavy metal ATPase 3 (HMA3) confers cadmium hypertolerance on the cadmium/zinc hyperaccumulator Sedum plumbizincicola. New Phytol. 215, 687–698. doi: 10.1111/nph.14622
Liu, L., Li, W., Song, W., and Guo, M. (2018). Remediation techniques for heavy metal-contaminated soils: principles and applicability. Sci. Total Environ. 633, 206–219. doi: 10.1016/j.scitotenv.2018.03.161
Liu, X. S., Feng, S. J., Zhang, B. Q., Wang, M. Q., Cao, H. W., Rono, J. K., et al. (2019). OsZIP1 functions as a metal efflux transporter limiting excess zinc, copper and cadmium accumulation in rice. BMC Plant Biol. 19:283. doi: 10.1186/s12870-019-1899-3
Lu, L. L., Tian, S. K., Yang, X. E., Li, T. Q., and He, Z. L. (2009). Cadmium uptake and xylem loading are active processes in the hyperaccumulator Sedum alfredii. J. Plant Physiol. 166, 579–587. doi: 10.1016/j.jplph.2008.09.001
Lu, L. L., Tian, S. K., Yang, X. E., Wang, X. C., Brown, P., Li, T. Q., et al. (2008). Enhanced root-to-shoot translocation of cadmium in the hyperaccumulating ecotype of Sedum alfredii. J. Exp. Bot. 59, 3203–3213. doi: 10.1093/jxb/ern174
Luo, J. S., Huang, J., Zeng, D. L., Peng, J. S., Zhang, G. B., Ma, H. L., et al. (2018). A defensin-like protein drives cadmium efflux and allocation in rice. Nat. Commun. 9:645. doi: 10.1038/s41467-018-03088-0
Marisela Fernandez-Valverde, S., Ordonez-Regil, E., Cabanas-Moreno, G., and Solorza-Feria, O. (2010). Electrochemical behavior of Ni-Mo electrocatalyst for water electrolysis. J. Mex. Chem. Soc. 54, 169–174.
Murashige, T., and Skoog, F. (1962). A Revised medium for rapid growth and bio assays with tobacco tissue cultures. Physiol. Plant. 15, 473–497. doi: 10.1111/j.1399-3054.1962.tb08052.x
Nocito, F. F., Lancilli, C., Dendena, B., Lucchini, G., and Sacchi, G. A. (2011). Cadmium retention in rice roots is influenced by cadmium availability, chelation and translocation. Plant Cell Environ. 34, 994–1008. doi: 10.1111/j.1365-3040.2011.02299.x
Parrotta, L., Guerriero, G., Sergeant, K., Cal, G., and Hausman, J. F. (2015). Target or barrier? The cell wall of early- and later-diverging plants vs cadmium toxicity: differences in the response mechanisms. Front. Plant Sci. 6:133. doi: 10.3389/fpls.2015.00133
Pollard, A. J., Reeves, R. D., and Baker, A. J. M. (2014). Facultative hyperaccumulation of heavy metals and metalloids. Plant Sci. 217, 8–17. doi: 10.1016/j.plantsci.2013.11.011
Rascio, N., and Navari-Izzo, F. (2011). Heavy metal hyperaccumulating plants: how and why do they do it? And what makes them so interesting? Plant Sci. 180, 169–181. doi: 10.1016/j.plantsci.2010.08.016
Ruijter, J. M., Ramakers, C., Hoogaars, W. M., Karlen, Y., Bakker, O., van den Hoff, M. J., et al. (2009). Amplification efficiency: linking baseline and bias in the analysis of quantitative PCR data. Nucleic Acids Res. 37:e45. doi: 10.1093/nar/gkp045
Sasaki, A., Yamaji, N., Yokosho, K., and Ma, J. F. (2012). Nramp5 Is a major transporter responsible for manganese and cadmium uptake in rice. Plant Cell 24, 2155–2167. doi: 10.1105/tpc.112.096925
Shahid, M., Dumat, C., Khalid, S., Niazi, N. K., and Antunes, P. M. C. (2017). “Cadmium bioavailability, uptake, toxicity and detoxification in soil-plant system,” in Rev. Environ. Contam. Toxicol, ed. P. DeVoogt (New York, NY: Springer), 73–137. doi: 10.1007/398_2016_8
Shi, T., Ma, J., Wu, F., Ju, T., Gong, Y., Zhang, Y., et al. (2019). Mass balance-based inventory of heavy metals inputs to and outputs from agricultural soils in Zhejiang Province. China. Sci. Total Environ. 649, 1269–1280. doi: 10.1016/j.scitotenv.2018.08.414
Song, W. Y., Choi, K. S., Alexis, D. A., Martinoia, E., and Lee, Y. (2011). Brassica juncea plant cadmium resistance 1 protein (BjPCR1) facilitates the radial transport of calcium in the root. Proc. Natl. Acad. Sci. U.S.A. 108, 19808–19813. doi: 10.1073/pnas.1104905108
Song, W. Y., Choi, K. S., Kim, D. Y., Geisler, M., Park, J., Vincenzetti, V., et al. (2010). Arabidopsis PCR2 is a zinc exporter involved in both zinc extrusion and long-distance zinc transport. Plant Cell 22, 2237–2252. doi: 10.1105/tpc.109.070185
Song, W. Y., Lee, H. S., Jin, S. R., Ko, D., Martinoia, E., Lee, Y., et al. (2015). Rice PCR1 influences grain weight and Zn accumulation in grains. Plant Cell Environ. 38, 2327–2339. doi: 10.1111/pce.12553
Song, W. Y., Martinoia, E., Lee, J., Kim, D., Kim, D. Y., Vogt, E., et al. (2004). A novel family of cys-rich membrane proteins mediates cadmium resistance in Arabidopsis. Plant Physiol. 135, 1027–1039. doi: 10.1104/pp.103.037739
Sterckeman, T., Goderniaux, M., Sirguey, C., Cornu, J. Y., and Nguyen, C. (2015). Do roots or shoots control cadmium accumulation in the hyperaccumulator Noccaea caerulescens? Plant Soil 392, 87–99. doi: 10.1007/s11104-015-2449-x
Tian, S. K., Lu, L. L., Labavitch, J., Yang, X. E., He, Z., Hu, H., et al. (2011). Cellular sequestration of cadmium in the hyperaccumulator plant species Sedum alfredii. Plant Physiol. 157, 1914–1925. doi: 10.1104/pp.111.183947
Tian, S. K., Lu, L. L., Yang, X. E., Huang, H. G., Wang, K., and Brown, P. H. (2012). Root adaptations to cadmium-induced oxidative stress contribute to Cd tolerance in the hyperaccumulator Sedum alfredii. Biol. Plant. 56, 344–350. doi: 10.1007/s10535-012-0096-0
Tian, S. K., Lu, L. L., Yang, X. E., Webb, S. M., Du, Y., and Brown, P. H. (2010). Spatial imaging and speciation of lead in the accumulatorpPlant Sedum alfredii by microscopically focused synchrotron X-ray investigation. Environ. Sci. Technol. 44, 5920–5926. doi: 10.1021/es903921t
Tian, S. K., Xie, R. H., Wang, H. X., Hu, Y., Hou, D. D., Liao, X. C., et al. (2017). Uptake, sequestration and tolerance of cadmium at cellular levels in the hyperaccumulator plant species Sedum alfredii. J. Exp. Bot. 68, 2387–2398. doi: 10.1093/jxb/erx112
Ueno, D., Iwashita, T., Zhao, F. J., and Ma, J. F. (2008). Characterization of Cd translocation and identification of the Cd form in xylem sap of the Cd-hyperaccumulator Arabidopsis halleri. Plant Cell Physiol. 49, 540–548. doi: 10.1093/pcp/pcn026
Willems, G., Frerot, H., Gennen, J., Salis, P., Saumitou-Laprade, P., and Verbruggen, N. (2010). Quantitative trait loci analysis of mineral element concentrations in an Arabidopsis halleri x Arabidopsis lyrata petraea F-2 progeny grown on cadmium-contaminated soil. New Phytol. 187, 368–379. doi: 10.1111/j.1469-8137.2010.03294.x
Wong, C. K. E., and Cobbett, C. S. (2009). HMA P-type ATPases are the major mechanism for root-to-shoot Cd translocation in Arabidopsis thaliana. New Phytol. 181, 71–78. doi: 10.1111/j.1469-8137.2008.02638.x
Wu, Z., Zhao, X., Sun, X., Tan, Q., Tang, Y., Nie, Z., et al. (2015). Antioxidant enzyme systems and the ascorbate-glutathione cycle as contributing factors to cadmium accumulation and tolerance in two oilseed rape cultivars (Brassica napus L.) under moderate cadmium stress. Chemosphere 138, 526–536. doi: 10.1016/j.chemosphere.2015.06.080
Yang, X. E., Li, T. Q., Long, X. X., Xiong, Y. H., He, Z. L., and Stoffella, P. J. (2006). Dynamics of zinc uptake and accumulation in the hyperaccumulating and non-hyperaccumulating ecotypes of Sedum alfredii Hance. Plant Soil 284, 109–119. doi: 10.1007/s11104-006-0033-0
Yang, X. E., Long, X. X., Ye, H. B., He, Z. L., Calvert, D. V., and Stoffella, P. J. (2004). Cadmium tolerance and hyperaccumulation in a new Zn-hyperaccumulating plant species (Sedum alfredii Hance). Plant Soil 259, 181–189. doi: 10.1023/B:PLSO.0000020956.24027.f2
Zhang, J., Zhang, M., Shohag, M. J. I., Tian, S. K., Song, H., Feng, Y., et al. (2016). Enhanced expression of SaHMA3 plays critical roles in Cd hyperaccumulation and hypertolerance in Cd hyperaccumulator Sedum alfredii Hance. Planta 243, 577–589. doi: 10.1007/s00425-015-2429-7
Zhao, F. J., Ma, Y. B., Zhu, Y. G., Tang, Z., and McGrath, S. P. (2015). Soil contamination in China: current status and mitigation strategies. Environ. Sci. Technol. 49, 750–759. doi: 10.1021/es5047099
Keywords: cadmium, plant cadmium resistance family, hyperaccumulator, Sedum alfredii, efflux
Citation: Lin J, Gao X, Zhao J, Zhang J, Chen S and Lu L (2020) Plant Cadmium Resistance 2 (SaPCR2) Facilitates Cadmium Efflux in the Roots of Hyperaccumulator Sedum alfredii Hance. Front. Plant Sci. 11:568887. doi: 10.3389/fpls.2020.568887
Received: 04 June 2020; Accepted: 09 October 2020;
Published: 30 October 2020.
Edited by:
Rafaqat Ali Gill, Chinese Academy of Agricultural Sciences, ChinaReviewed by:
Qingyu Wu, Chinese Academy of Agricultural Sciences (CAAS), ChinaCopyright © 2020 Lin, Gao, Zhao, Zhang, Chen and Lu. This is an open-access article distributed under the terms of the Creative Commons Attribution License (CC BY). The use, distribution or reproduction in other forums is permitted, provided the original author(s) and the copyright owner(s) are credited and that the original publication in this journal is cited, in accordance with accepted academic practice. No use, distribution or reproduction is permitted which does not comply with these terms.
*Correspondence: Lingli Lu, bGluZ2xpbHVsdUBnbWFpbC5jb20=; bHVsaW5nbGlAemp1LmVkdS5jbg==
†These authors have contributed equally to this work
Disclaimer: All claims expressed in this article are solely those of the authors and do not necessarily represent those of their affiliated organizations, or those of the publisher, the editors and the reviewers. Any product that may be evaluated in this article or claim that may be made by its manufacturer is not guaranteed or endorsed by the publisher.
Research integrity at Frontiers
Learn more about the work of our research integrity team to safeguard the quality of each article we publish.