- 1Genetics, Genomics and Crop Improvement Division, International Potato Center, Lima, Peru
- 2Latin American & Caribbean Regional Program, International Potato Center, Lima, Peru
- 3Crop and Systems Science Division, International Potato Center, Lima, Peru
- 4Crop Husbandry Department, Universidad Nacional Agraria La Molina, Lima, Peru
Crop wild relatives of sweetpotato [Ipomoea series Batatas (Choisy) D. F. Austin] are a group of species with potential for use in crop improvement programs seeking to breed for drought tolerance. Stress memory in this group could enhance these species’ physiological response to drought, though no studies have yet been conducted in this area. In this pot experiment, drought tolerance, determined using secondary traits, was tested in 59 sweetpotato crop wild relative accessions using potential short-term memory induction. For this purpose, accessions were subjected to two treatments, i) non-priming: full irrigation (up to field capacity, 0.32 w/w) from transplanting to harvest and ii) priming: full irrigation from transplanting to flowering onset (FO) followed by a priming process from FO to harvest. The priming process consisted of three water restriction periods of increasing length (8, 11, and 14 days) followed each by a recovery period of 14 days with full irrigation. Potential stress memory induction was calculated for each accession based on ecophysiological indicators such as senescence, foliar area, leaf-minus-air temperature, and leaf 13C discrimination. Based on total biomass production, resilience and production capacity were calculated per accession to evaluate drought tolerance. Increase in foliar area, efficient leaf thermoregulation, improvement of leaf photosynthetic performance, and delayed senescence were identified in 23.7, 28.8, 50.8, and 81.4% of the total number of accessions, respectively. It was observed that under a severe drought scenario, a resilient response included more long-lived green leaf area while a productive response was related to optimized leaf thermoregulation and gas exchange. Our preliminary results suggest that I. triloba and I. trifida have the potential to improve sweetpotato resilience in dry environments and should be included in introgression breeding programs of this crop. Furthermore, I. splendor-sylvae, I. ramosissima, I. tiliacea, and wild I. batatas were the most productive species studied but given the genetic barriers to interspecific hybridization between these species and sweetpotato, we suggest that further genetic and metabolic studies be conducted on them. Finally, this study proposes a promising method for improving drought tolerance based on potential stress-memory induction, which is applicable both for wild species and crops.
Introduction
In 2018, sweetpotato [Ipomoea batatas (L.) Lam.] was the root crop with the second highest global production after cassava [Manihot esculenta Crantz] (FAO, 2020). Sweetpotato is highly nutritious and outperforms most carbohydrate-based foods in terms of vitamins, minerals, dietary fiber, and total protein, making it a priority in crop-based strategies to enhance global food and nutrition security (Woolfe, 1992; Motsa et al., 2015). Sweetpotato is also known for its ability to grow in drought-prone soils with low external input (fertilizer and pesticide) requirements, while thanks to a short growing cycle, it is also recognized as an excellent crop for post-crisis (hurricane, flooding, refugee settlement, etc.) situations (Bradbury and Holloway, 1987; Mwanga and Ssemakula, 2011; Mekonnen et al., 2015). However, a range of biotic and abiotic stress factors such as viruses, weevils, and severe drought, are inhibiting farmers’ ability to achieve sweetpotato’s full yield potential (Valverde et al., 2007; Mwanga and Ssemakula, 2011; Agili and Nyende, 2012; Kivuva et al., 2015). Moreover, climate change’s negative effect on sweetpotato productivity is of rising concern in regions affected by increasing global temperatures, such as tropical and subtropical areas of Sub-Saharan Africa (Low et al., 2009; Schulze, 2011; Knox et al., 2012). Resilient sweetpotato varieties could play an extremely important food and nutrition security role in the developing world, one that will increase in importance as the availability of good cropping areas becomes more limited.
Series Batatas [Ipomoea series Batatas (Choisy) D. F. Austin] is a subdivision within Ipomoea, the largest genus in the morning glory (Convolvulaceae) family. This group includes the cultivated hexaploid sweetpotato [I. batatas (L.) Lam.], wild tetraploid I. batatas (L.) Lam. (Ozias-Akins and Jarret, 1994), and 15 closely related wild species (Austin, 1978; McDonald and Austin, 1990; Austin et al., 1993; Wood et al., 2015; Wood et al., 2020). The wild species include Ipomoea trifida (H.B.K.) G. Don, Ipomoea cordatotriloba Dennstedt, Ipomoea cynanchifolia Meisn., Ipomoea grandifolia (Dammer) O’Donell, Ipomoea lacunosa L., Ipomoea leucantha Jacquin, Ipomoea littoralis Blume, Ipomoea ramosissima (Poir.) Choisy, Ipomoea splendor-sylvae House, Ipomoea tabascana McDonald and Austin, Ipomoea tenuissima Choisy, Ipomoea tiliacea (Willd.) Choisy in D. C., Ipomoea triloba L., Ipomoea lactifera J.R.I. Wood and Scotland, and Ipomoea australis (O’Donell) J.R.I. Wood & P. Muñoz. Since sweetpotato crop wild relatives (SP-CWR) are well adapted to diverse, even extreme environmental conditions (Iwanaga, 1988; Guarino and Lobell, 2011), they are considered a prominent genetic resource to improve both biotic and abiotic stress tolerance in cultivated crops (Iwanaga, 1988; Komaki, 2004; Nimmakayala et al., 2011). However, Khoury et al. (2015) pointed out that the full potential offered by SP-CWR, especially in terms of drought tolerance, is far from being fully exploited.
From a physiological point of view, drought tolerance encompasses all mechanisms that enable plants to avoid or tolerate dehydration, such as maintenance of cell turgor and water uptake, and reduction of water loss, among others (Fischer and Maurer, 1978; Turner, 1986; Turner, 1997). In sweetpotato, several physiological traits have been used for screening drought tolerant genotypes such as chlorophyll concentration (Mbinda et al., 2018; Mbinda et al., 2019), canopy cover (Laurie et al., 2014), leaf temperature (Laurie et al., 2014; Rukundo et al., 2017; Low et al., 2020), and 13C discrimination (Low et al., 2020). The latter has been reported as one of the most accurate criteria for selecting drought tolerant genotypes (Tuberosa, 2012; Low et al., 2020) since it is a good indicator of stomatal conductance (Condon et al., 2004), water use efficiency (Turner, 1997), and photosynthetic performance (Jefferies and Mackerron, 1997; Dawson et al., 2002).
Previous studies in other plants such as Arabidopsis thaliana (Ling et al., 2018; Serrano et al., 2019), potato (Watkinson et al., 2006; Ramírez et al., 2015a), cassava (Cayón et al., 1997), wheat (Ahmed et al., 2016), and grasses (Walter et al., 2011) have shown that a previous exposure to stress in early development stages “prepares” the plant for a subsequent exposure to the same stress. This behavior, known as stress memory, is achieved through accumulation of transcription factor or signaling proteins and epigenetic changes, which are later translated into an enhanced physiological response (Bruce et al., 2007). In this study, we tested potential short-term memory occurrence, which involves tolerant responses within the plant life cycle to previous stress periods (Conrath et al., 2006; Bruce et al., 2007), as opposed to transgenerational memory, in which the tolerant response is inherited by the next plant generation (Han and Wagner, 2014). Wild species are considered to be possible sources of useful genes/alleles related to stress tolerance as they have evolved under natural selection to survive consecutives periods of climate extremes (Sharma et al., 2013). In the present study, we focused on the previously described physiological traits to determine potential stress memory induction in SP-CWR.
Farmers have traditionally selected drought tolerant genotypes by choosing those genotypes that maintained high yield under drought stress conditions. However, this selection strategy may penalize resilience or production stability, as shown by Blum (1996), who found that some genotypes with high tolerance presented low yield performance in the absence of stress conditions. Fernandez (1992) classified plants into four groups based on yield performance in stress and non-stress conditions: Group A (accessions expressing uniform superiority in both stress and non-stress conditions), Group B (accessions presenting good performance only under potential conditions and not under stress conditions), Group C (accessions expressing a relatively higher performance only under stress), and Group D (accessions with poor performance in both environments). Recently, Thiry et al. (2016) proposed a yield-based definition of drought tolerance in terms of resilience and productivity of crops useful to facilitate the classification of genotypes for breeding programs. These authors stated that the resilience capacity index (RCI) expresses the yield decrease of genotypes under stress within a population, compared with yield potential conditions, whereas the production capacity index (PCI) expresses the mean production of genotypes under both stressed and non-stressed conditions within a population. Moreover, a genotype could be classified in any of Fernandez’s (1992) Groups with those genotypes scoring the highest values being the most drought tolerant ones. Using combined indices such as resilience and productivity simultaneously in sweetpotato breeding programs would reduce costs and save time.
The aim of this study was i) to determine potential short-term memory induction in SP-CWR and its manifestation in ecophysiological traits like senescence, foliar area, leaf-minus-air temperature, and leaf 13C discrimination and ii) to identify the memory-induced physiological mechanisms associated with the development of drought tolerance (in terms of resilience and productivity) in SP-CWR in order to identify new sources for breeding towards improved drought tolerance in sweetpotato.
Material and Methods
Experimental Conditions and Management
A pot experiment was conducted under greenhouse conditions from July 13th to December 18th, 2018 at the experimental station of the International Potato Center (CIP) in San Ramón, Junín, Peru (11° 7’ 39.3” S, 75°21’ 23.4” W, 850 m a.s.l.). The station is located in the mountainous Amazon area of central Peru. The region is characterized by a rainy, warm, and very humid climate (SENAMHI, 2020) with an average annual maximum temperature, average annual solar radiation and annual precipitation of 30.8 ± 0.46°C, 34.2 ± 1.51 MJ m-2 day-1, and 1,294 mm, respectively (data from 2019, CIP–San Ramón weather station). Pots were distributed in two neighboring screenhouses covered with an anti-aphid mesh with an opening size of 0.26 × 0.82 mm. The screenhouses were also covered with a translucid plastic and a black mesh (hole size of 2 × 2 mm) to prevent high radiation stress and provide shelter from rain. During the study period, the average relative humidity, and maximum and minimum temperatures were 81.2 ± 0.96%, 32.7 ± 0.47°C, and 19.8 ± 0.21°C respectively. The average solar radiation and maximum vapor pressure deficit were 18.5 ± 0.94 MJ m-2 day-1 and 2.7 ± 0.13 kPa (Supplementary Table S1). These variables were measured every 5 min. Temperature, relative humidity (S-THB-M008 model), and solar radiation (S-LIB-M003 model) sensors were recorded with a HOBO U30 datalogger (Onset Computer Corporation, Bourne, MA, USA).
On July 13th eight seeds per accession were scarified individually and placed on moist filter paper in petri dishes. After germination seedlings were planted in peat pellets (Jiffy Products Ltd., Canada) for 15 days to promote root development. Subsequently, plantlets were transferred to pots (6.4 L) filled with 6.5 kg of a 2:1 mixture of sand and peat-based substrate (PRO-MIX, Premier Tech Horticulture, Canada). All pots were randomized regularly i.e. the positions of individual plants within the greenhouses were changed to avoid a significant effect of pot positions on the measured traits. In each screenhouse two HOBO U30 datalogger were placed at different positions (one near the central isle and another near the outside screen wall) to monitor temperature and humidity gradients.
Each pot received seven fertilizer applications scheduled every 2 weeks using 0.51 g N, 0.78 g P2O5, and 0.60 g, K2O (Peters Professional ICL Ltd., Israel). To control thrips and whitefly, folding traps with pheromones were installed and insecticides (Ocaren, active ingredient: profenofos and fipronil, Interoc S.A., Peru and Vertimec, active ingredient: abamectina; Farmex S.A., Peru) were applied at a dose rate of 1 mL L-1 when necessary. Since the wild species included in this study are twiners, a wire-made spiral welded to three vertical rods was placed in each pot to support the plants, in order to facilitate vertical growth and the expansion of foliar area.
Plant Material
Fifty-nine accessions belonging to the Ipomoea series Batatas (Choisy) D. F. Austin, the closest wild relatives of sweetpotato, were selected from the SP-CWR collection of CIP’s genebank (Table 1). The plant material included four accessions of cultivated hexaploid I. batatas (L.) Lam. (hereinafter “sweetpotato”) namely “Beauregard,” “Tanzania,” and two accessions deriving from crosses between the two varieties: “B×T” (Wu et al., 2018). Also, two accessions of wild tetraploid (4x) I. batatas (L.) Lam. (Ozias-Akins and Jarret, 1994) and 53 accessions encompassing 10 species of the series Batatas were included. The wild relative species assessed in this study were: I. australis (O’Donell) J.R.I. Wood & P. Muñoz (5), I. cordatotriloba Dennstedt (1), I. cynanchifolia Meisn. (3), I. grandifolia (Dammer) O’Donell (5), I. leucantha Jacquin (2), I. ramosissima (Poir.) Choisy (8), I. splendor-sylvae House (3), I. tiliacea (Willd.) Choisy (2), I. trifida (H.B.K.) G. Don (16), and I. triloba L. (8) (Khoury et al., 2015; Wood et al., 2020). The basic chromosome number of all accessions in this study is x = 15. While most SP-CWRs of our taxon sample are diploid (2n = 2x = 30), four accessions are tetraploid (2n = 4x = 60) and the cultivated sweetpotato accessions are hexaploid (2n = 6x = 90) (G. Rossel, CIP, pers. comm.) (Table 1). The taxon sample of the wild species focused on Central and South American species and considered geographic distribution range and morphological variation between accessions of the same species.
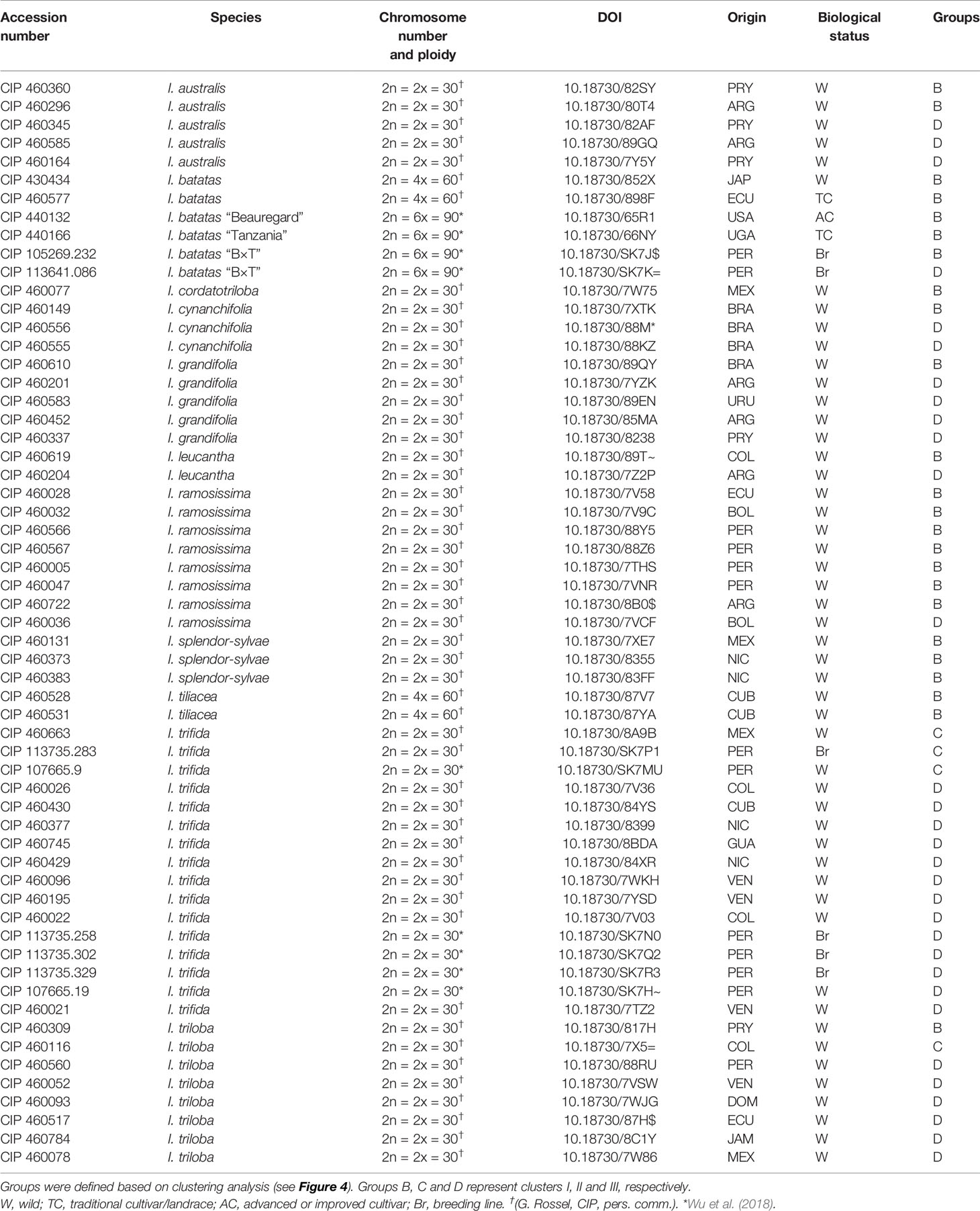
Table 1 Sweetpotato crop wild relatives [Ipomoea series Batatas (Choisy) D. F. Austin] accessions from CIP’s genebank used in this study, with chromosome number and ploidy (basic chromosome number: x=15), country of origin and biological status for each of them.
Drought Priming Process
Eight individual plants per accession were distributed at random in both greenhouses. Four individual plants per accession (i.e. four replications) were randomly assigned to the two experimental treatments. Previous stress memory studies by Liu and Charng (2012); Ling et al. (2018) and Serrano et al. (2019) were used to guide the experimental design of this study. Treatments consisted of a series of water restriction periods of increasing length (priming), versus full irrigation (non-priming). After transplantation of seedlings into pots, all pots were watered until field capacity (0.32 w/w) three times per week, following the protocol of Rolando et al. (2015), until harvest. Non-primed plants received full irrigation during the entire experimental cycle while plants undergoing the water restriction treatment were watered until flowering onset (FO) (Figure 1). Priming was initiated when 50% of samples conforming an accession started to exhibit flower buds (defined as FO occurrence) (Thiry et al., 2016). Two different starting dates were established for early-flowering (20 accessions) and late-flowering (18 accessions) or non-flowering (21 accessions) accessions (Supplementary Table S2). Three priming events, or water restriction periods, were carried out with 8, 11, and 14 days of total water restriction. Each of these water restricted periods was followed by a recovery period of 14 days in which plants were irrigated until field capacity (Figure 1). Therefore, the priming process consisted of three water restriction periods: the first one started at FO whereas the second and third priming events were initiated at 68 and 94 days after transplanting (DAT) for early as well as 94 and 122 DAT for late and non-flowering accessions, respectively.
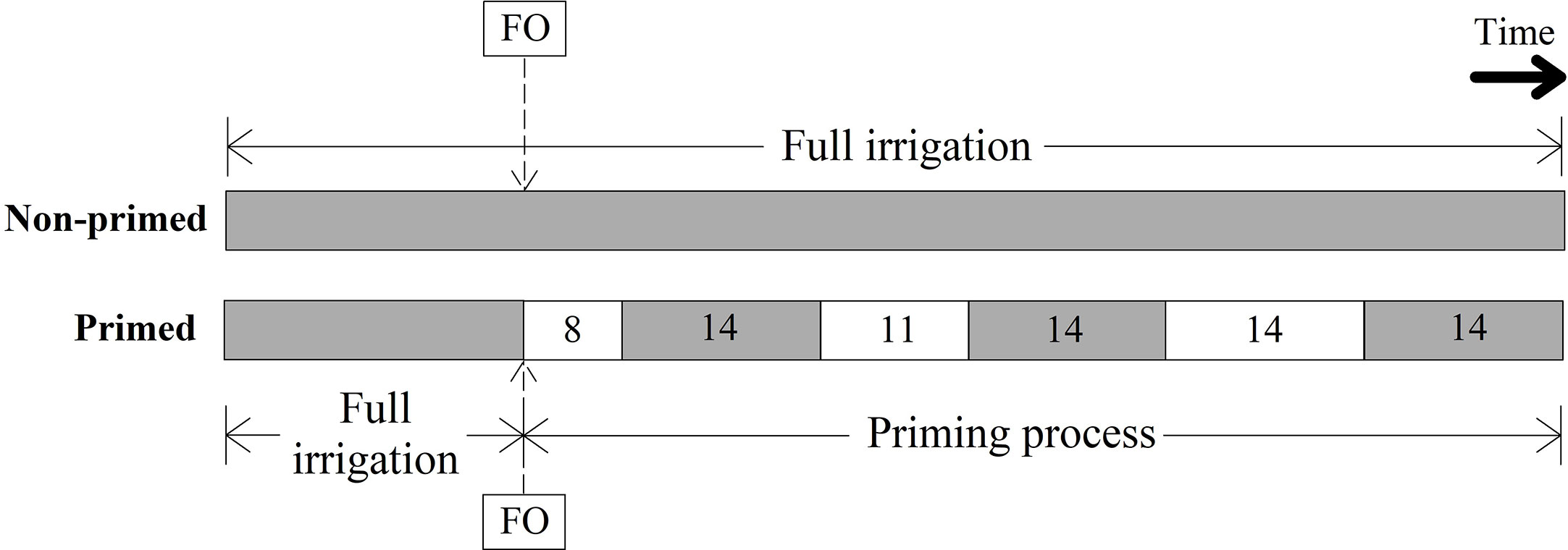
Figure 1 Timeline representation for the watering treatments per accession: non-primed plants (no water restriction) and primed plants (water restriction periods). Gray and white blocks mean substrate watering with full irrigation or no irrigation, respectively. Duration of every period during the priming process is indicated in days within the blocks. The priming process started after flowering onset (FO).
Drought stress responses were measured at the end of the first water restriction period to corroborate if 8 days was a sufficiently long period to induce a significant drought stress. For this purpose, maximum stomatal conductance at saturated light (gs_max) was measured (sensu Medrano et al., 2002) in one young and sun-exposed leaf of eight plants of 19 randomly selected accessions using a portable photosynthesis system (LI-6400TX, LI-COR, Nebraska, USA). Measurements were carried out during early morning hours from 6:00 to 10:00 h local time. The following micro-environmental parameters were recorded: photosynthetic active radiation = 1,200 µmol m-2 s-1, CO2 concentration = 400 ppm, and atmospheric humidity = 50%.
Ecophysiological Measurements to Determine Potential Short-Term Memory Occurrence
Chlorophyll concentration (ChlSPAD) of all accessions was measured using a portable chlorophyll meter (SPAD-502 Plus, Konica Minolta Inc., Osaka, Japan) on 17 occasions during the study period. An average of four readings from four young and sun-exposed leaves were taken per plant. Senescence (S) was estimated on each plant as the slope generated by fitting ChlSPAD (from maximum leaf greenness to harvest) vs. time on a linear function (Li et al., 2019). Assuming that an extended senescence delay (high S) is associated with a higher probability of fixing more carbon during the lifespan, short-term memory proxy’s (STM) effect on S (STMS) was calculated as follows:
Where Spr and Snpr are S average values for primed and non-primed plants, respectively. Regular assessments of foliar area (FA) were carried out on both primed and non-primed plants before and after each water restriction and recovery period by taking visible images (using a Nikon model D7000 camera, Nikon Corp., Japan) of each plant. Images were acquired following CIP procedures (PSE-CIP, 2013) and processed with Image Canopy software (Barreda et al., 2017) that allows the calculation of foliar area through an image segmentation technique separating healthy green vegetation from other components within a picture. To test the effect of STM on FA (STMFA) the following equation was calculated:
Where FAmax_pr and FAmax_npr are FA average maximum value of the temporal assessments corresponding to primed and non-primed plants, respectively. Leaf temperature was measured radiometrically from 14:00 to 15:00 h following the protocols of Idso et al. (1981) and Rinza et al. (2019). Measurements were taken using an infrared thermometer (DT-882 model, CEM, China) during 14 measurement events throughout the study period. Air temperature was registered with four data loggers (HOBO U23 Pro v2 Temperature/Relative Humidity, Onset Computer Corporation, Bourne, MA, USA), two of which were located inside each greenhouse at canopy level. The difference between leaf and air temperature (dT) was calculated using average leaf temperature and average air temperature registered at the same time when leaf temperature was measured. STM effect on dT (STMdT) was calculated as follows:
Where dTmin_pr and dT min_npr are the average minimum dT over time reached by primed and non-primed plants of an accession. Finally, two composed leave samples per accession and treatment were collected at the end of each recovery period. Six assessments in total were carried out from FO until harvest at 66, 93, and 121 DAT for early flowering accessions and at 94, 121, and 148 DAT for late- or non-flowering accessions. Each composed sample consisted of 20 leaves—five young leaves per plant—which were oven dried at 60°C for 48 h (BLUE M Model POM-166EY, BLUE M Electronic Company, IL, USA). Dried leaves were milled with a ball miller (MBIX-100 model, MRC, Israel) and packed in tin capsules (Ramírez et al., 2015b). Capsules were sent to the Stable Isotope Facility at the University of Davis, USA, for carbon isotope composition (δ13C) analysis using a PDZ Europa ANCA-GSL elemental analyzer coupled to PDZ Europa 20-20 isotope ratio mass spectrometer (Sercon Ltd., Cheshire, UK). Leaf 13C discrimination (Δ) was calculated as described by Farquhar et al. (1989):
Where δp is δ13C of the sample and δa is the δ13C of the atmospheric CO2, -8‰. STM effect on Δ (STMΔ) was estimated as follows:
Where Δmax_pr and Δmax_npr are the maximum Δ over time for primed and non-primed plants within an accession, respectively. The STM occurrence was defined when primed plants’ response exceeded that of non-primed plants, i.e. when the STM value was higher than zero (STM>0) in the case of S in Equation 1, and higher than one (STM>1) in the case of FA, dT and Δ in Equation 2, 3, and 5, respectively. For all accessions, the priming process started at the same physiological stage (following recommendations from Thiry et al., 2016) so that all the ecophysiological indicators (S, Amax, dTmin, and Δmax) used to determine potential STM occurrence were comparable between accessions regardless of the starting date of the priming process.
Drought Tolerance Indices and Statistical Analysis
At the end of the priming process, total biomass was harvested and subsequently oven dried at 60°C for 48 h to calculate the dry weight of total biomass of primed (Ypr) and non-primed (Ynpr) plants. In this study, total biomass comprises above and below ground biomass and is generally expressed on a dry matter (DM) basis. The stress susceptibility index (SSI) (Fischer and Maurer, 1978) and the geometric mean productivity index (GMP) (Fernandez, 1992) were calculated as follows:
Where Ypr and Ynpr are the average total biomass (DM) production of the primed and non-primed plants, respectively, and and are the overall average total biomass (DM) production of the primed and non-primed plants, respectively. SSI and GMP of a genotype were estimated as the resilience capacity index (RCI) and the production capacity index (PCI) following the score values method described by Thiry et al. (2016) (Supplementary Appendix A). For of both score indices (RCI and PCI), a score value of 1 means high susceptibility whereas a score of 10 represents high drought tolerance.
A repeated measures analysis of variance (rmANOVA) was run to evaluate the effects of the treatments (between subjects factor) and time (within subjects factor) on assessed ecophysiological variables (SPAD, FA and dT, except for Δ, due to its composed samples). A one-way ANOVA was used to assess differences (at p < 0.05) among accessions in total dry weight (Y). A t-student test was used to identify significant differences between treatments for every physiological evaluation of SPAD, FA, dT, Δ, and Y. A Pearson correlation analysis was performed to evaluate the relationship between STM traits and both RCI and PCI. A principal component analysis (PCA) was run to analyze the accessions ordination through the association of STM trait effects and RCI, as well as PCI. Finally, a cluster analysis following the Ward’s method was computed using the R package “FactoMineR” (Lê et al., 2008) to classify all accessions into any of the four Groups of plants described by Fernandez (1992). All above described tests were performed with RStudio software (R Core Team, 2019).
Results
Short-Term Memory Occurrence
ChlSPAD, FA, dT, and Δ values ranged between 10.3–51.4 SPAD units, 0.0–1,537.3 cm2, −12.2 – 4.1°C, and 20.4–25.3‰, respectively. Effects of the water treatment for ChlSPAD, FA, and dT were significant (p < 0.05) for 33.9, 81.4, and 81.4% of the total accessions and the effect of time was significant in all accessions for ChlSPAD, FA, and dT. The percentage of accessions with a significant (p < 0.05) interaction between watering treatments and time for ChlSPAD, FA, and dT were 93.2, 98.3, and 78.0%, respectively (Supplementary Table S3). At the end of the first water restriction period, at 53 DAT, primed plants had 78.6% less average gs_max than non-primed plants (0.02 ± 0.03 and 0.10 ± 0.04 mol H2O m-2 s-1 for primed and non-primed plants, respectively) of the 19 assessed accessions. Moreover, 16 accessions (81.3%) presented primed plants with average gs_max below 0.05 mol H2O m-2 s-1 (Supplementary Table S4). STM occurrence was detected in 23.7, 28.8, 50.8, and 81.4% of the total number of accessions for FA, dT, Δ, and S, respectively (Figure 2). For STMS, I. leucantha presented the highest value (0.48 for CIP 460204) and I. trifida had the widest range (−0.09 – 0.35) (Figure 3A) while for STMFA, I. ramosissima presented the highest value (1.5 for CIP 460032) as well as the widest range (0.76–1.5) (Figure 3B). I. australis showed both the highest STMdT value (1.26 for CIP 460296) and the widest STMdT range (0.66–1.26) (Figure 3C) whereas I. batatas had the highest STMΔ value (1.04 for CIP 430434) and I. trifida the widest STMΔ range (0.93–1.03) (Figure 3D). The raw data and RGB images of each plant are available online (Guerrero-Zurita et al., 2020).
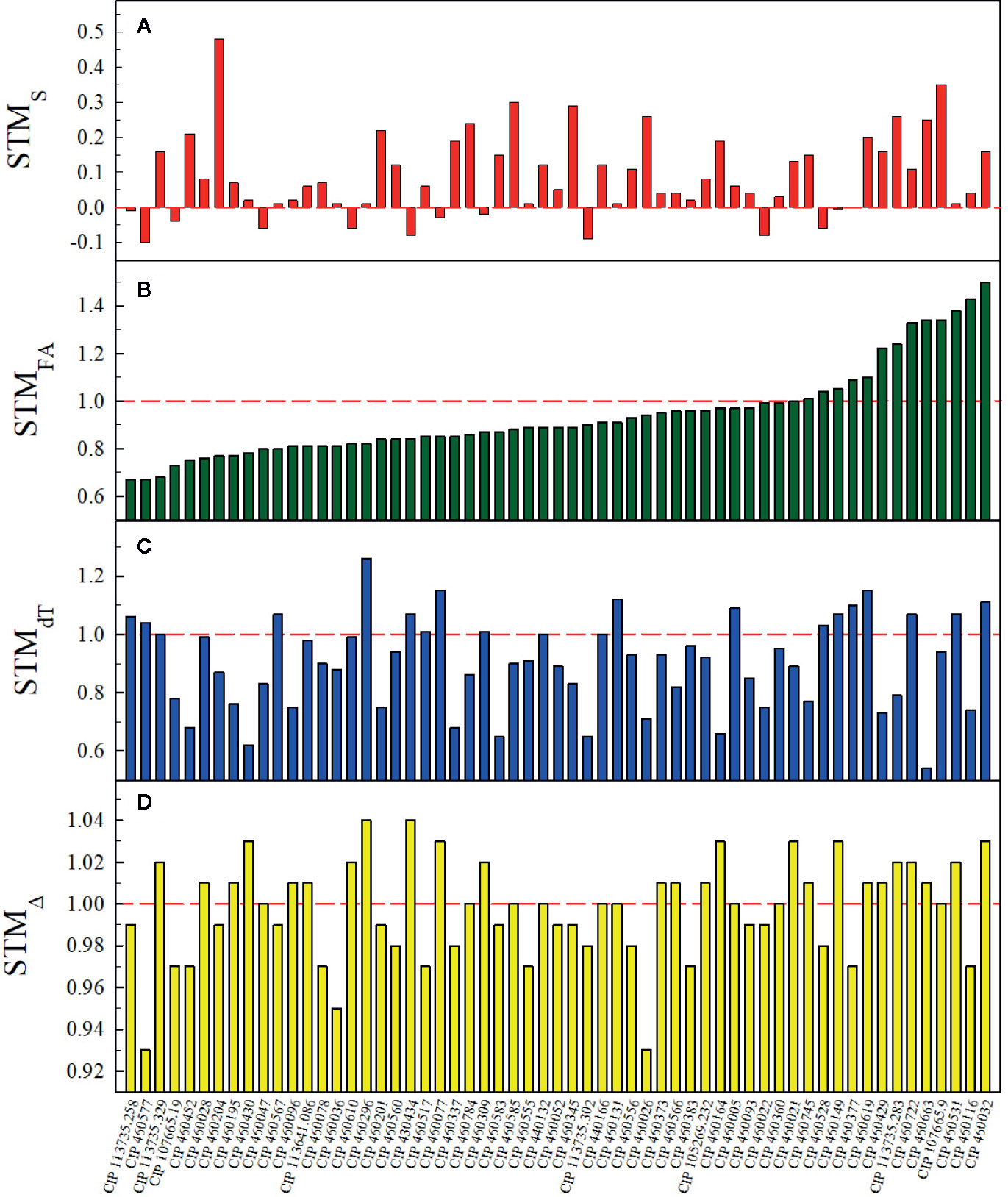
Figure 2 Short-term memory (STM) values per accession for senescence (STMS) (A), foliar area (STMFA) (B), leaf-minus-air temperature (STMdT) (C) and leaf 13C discrimination (STMΔ) (D). Red dashed line indicates STM occurrence.
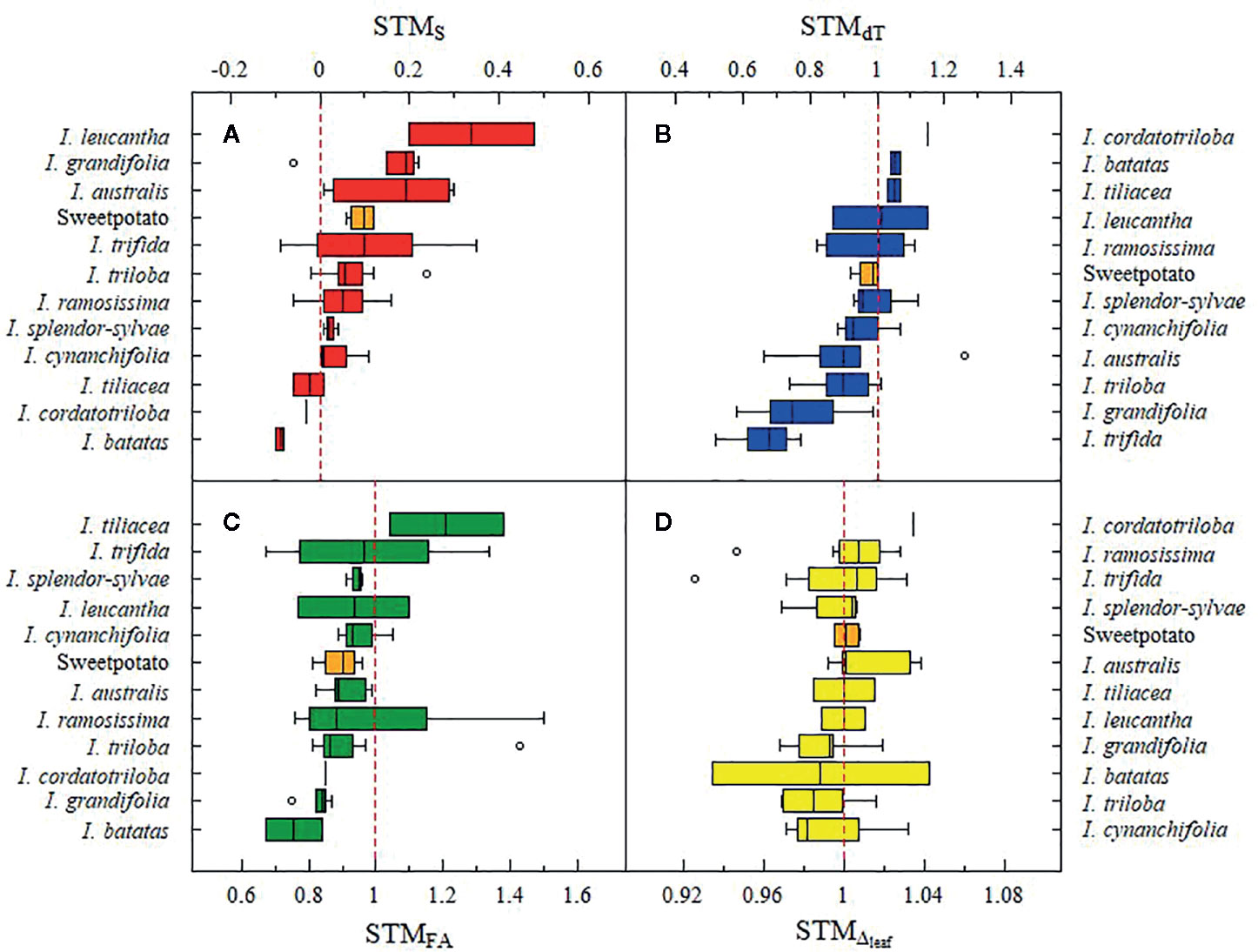
Figure 3 Boxplot of short-term memory (STM) values per crop wild relatives (CWR) species and sweetpotato cultivars (orange boxplots) for senescence (STMS) (A), foliar area (STMFA) (B), leaf-minus-air temperature (STMdT) (C) and leaf 13C discrimination (STMΔ) (D). Red dashed line indicates the STM occurrence. In each specie’s boxplot, black line represents the median value. Boxplot contains the variation between 25 and 75% and gray circles are the outlier values.
Drought Tolerance, Resilience, and Productivity Under Drought and Their Relationship With Short-Term Memory Occurrence
SSI and GMP values ranged between −0.83 – 1.74 and 7.56–78.1, respectively (Supplementary Table S5). High coefficients of determination between the original values for SSI and GMP and their RCI and PCI score indices were obtained (R2 = 0.970 for SSI vs. RCI and R2 = 0.974 for GMP vs. PCI) indicating that score indices can be used as a surrogate of their original index value. The highest RCI were found in CIP 460116 (8.0), CIP 107665.9 (7.5), and CIP 460663 (7.0) while I. triloba had the highest range of values (3.5–8.0) followed by I. trifida (2.0–7.5) and I. grandifolia (1.75–5.25). Regarding PCI, the highest values were found in CIP 440166 (9.75), CIP 460131 (6.0), and CIP 440132 (5.75) while sweetpotato cultivars presented the highest range of scores (2.75–9.75) followed by I. splendor-sylvae (4.25–6) and I. ramosissima (2.5–5.75). Moreover, I. cynanchifolia, I. australis, I. grandifolia, I. trifida, and I. triloba had higher RCI than PCI, whereas I. leucantha, I. ramosissima, I. cordatotriloba, I. tiliacea, wild I. batatas, I. splendor-sylvae, and the sweetpotato cultivars had higher PCI than RCI.
STMdT showed a significant positive and negative correlation with PCI (r = 0.52) and RCI (r = −0.30), respectively, whereas STMFA was the trait with the highest positive correlation with RCI (r = 0.38) (Table 2). The first three components of the PCA represented 72.7% of the total variance (Table 3). The first component was mainly explained by STMS and RCI with a negative effect and STMdT and PCI with a positive effect. STMFA showed a higher weight in the second principal component. The third principal component was mainly explained by STMdT with positive effect (Table 3).
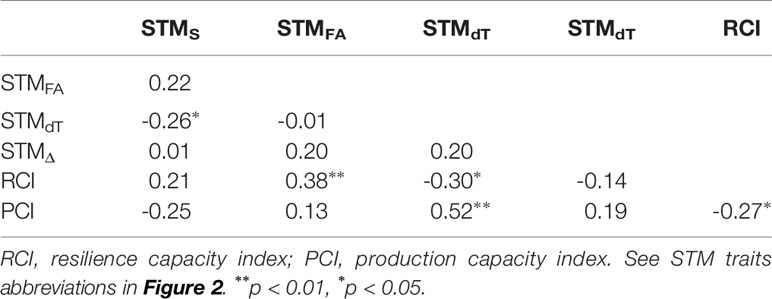
Table 2 Pearson correlation coefficients matrix among short-term memory (STM) traits and drought tolerant indices.
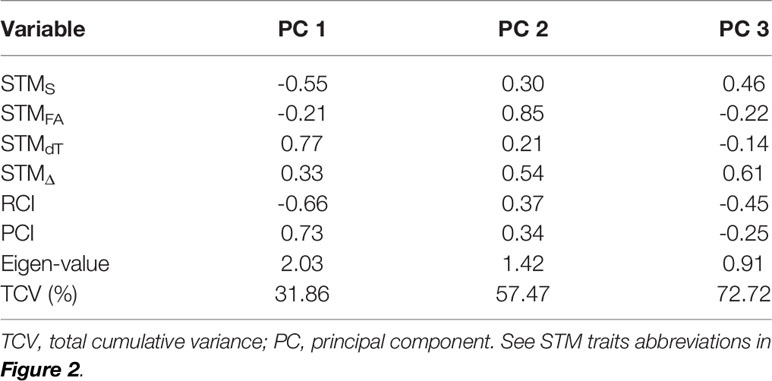
Table 3 Extracted components from Principal Component Analysis based on the ordination of short-term memory (STM) traits, drought tolerance resilience (RCI), and productivity (PCI).
Clustering analysis grouped the accessions into three clusters: I, II, and III (Figure 4). Cluster I, II, and III contained 24, 4, and 31 accessions, respectively (Table 1). When analyzing the average response per variable obtained in each group, cluster I had the highest average for STMdT, STM∆, PCI, and Ynpr (1.03 ± 0.1, 1.01 ± 0.0, 4.39 ± 1.7, and 49.1 ± 16.4 g, respectively) whereas cluster II was highest for STMS, STMFA, and RCI (0.23 ± 0.1, 1.34 ± 0.1, and 7.0 ± 1.1, respectively) (Table 4). Accessions belonging to cluster III displayed the lowest average response for all traits (Table 4). Cluster I contained the highest percentage of accessions with STM∆ occurrence (70.8%) followed by cluster II (50%) and cluster III (35.5%) (Figure 5).
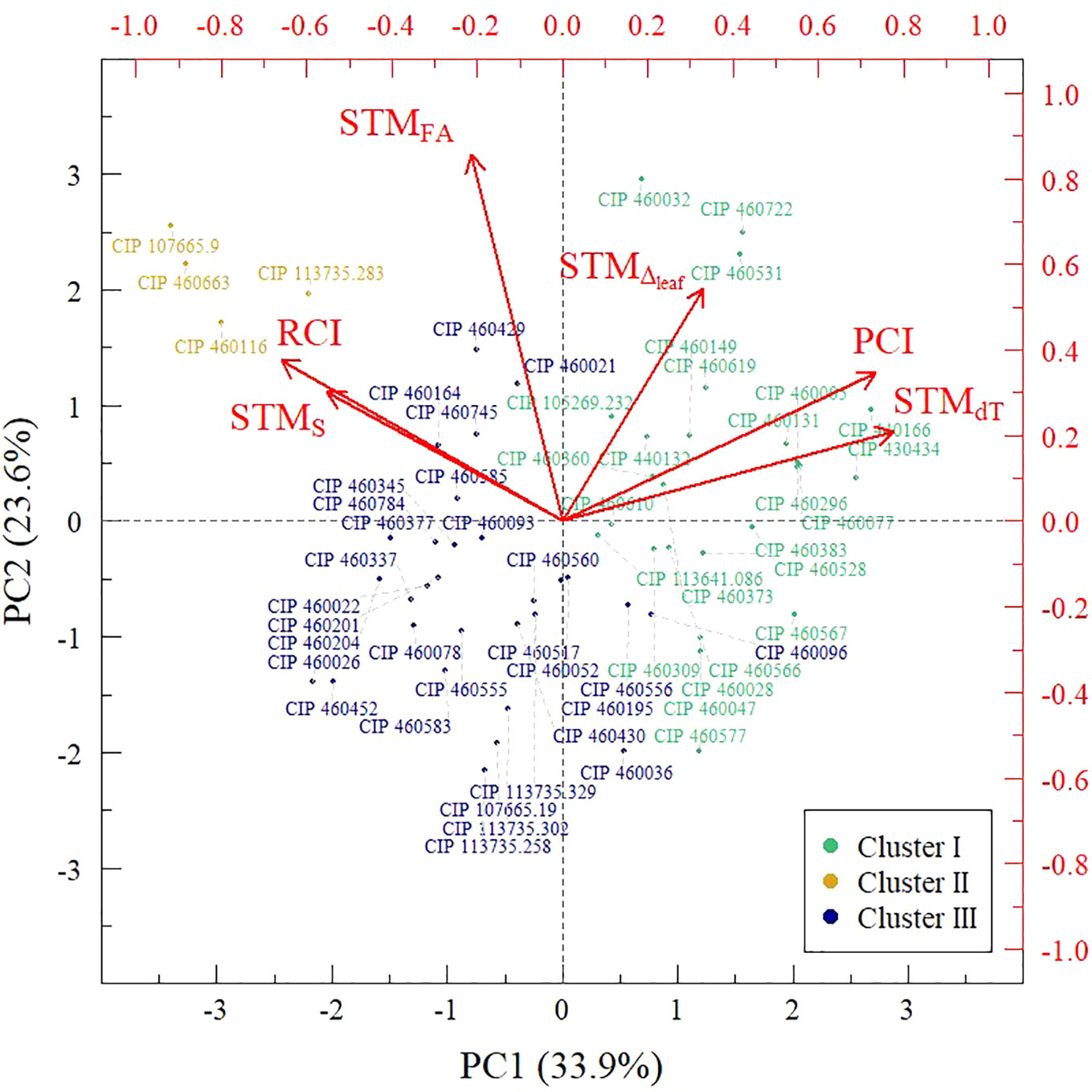
Figure 4 Clustering analysis and 2D ordination of sweetpotato cultivars and its CWR species based on Principal Component Analysis for component loadings (STM traits, PCI and RCI). Clusters I, II and III correspond to Fernandez's (1992) Groups B, C, and D, respectively.
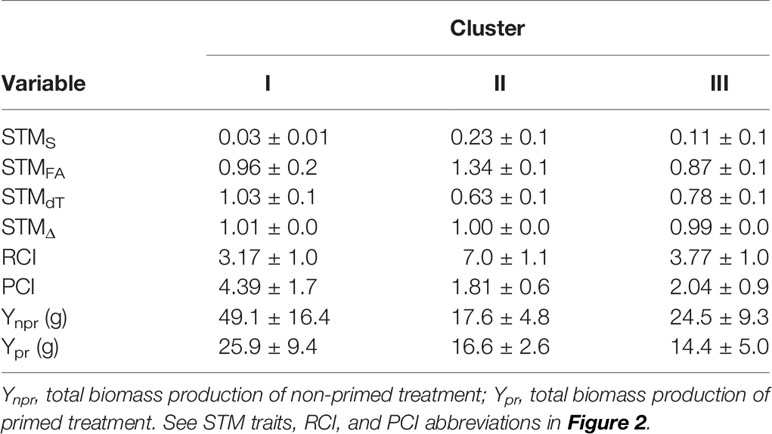
Table 4 Average value ± standard error of STM traits, drought tolerance indices and total biomass production for each cluster (I, II and III) from Principal Component Analysis.
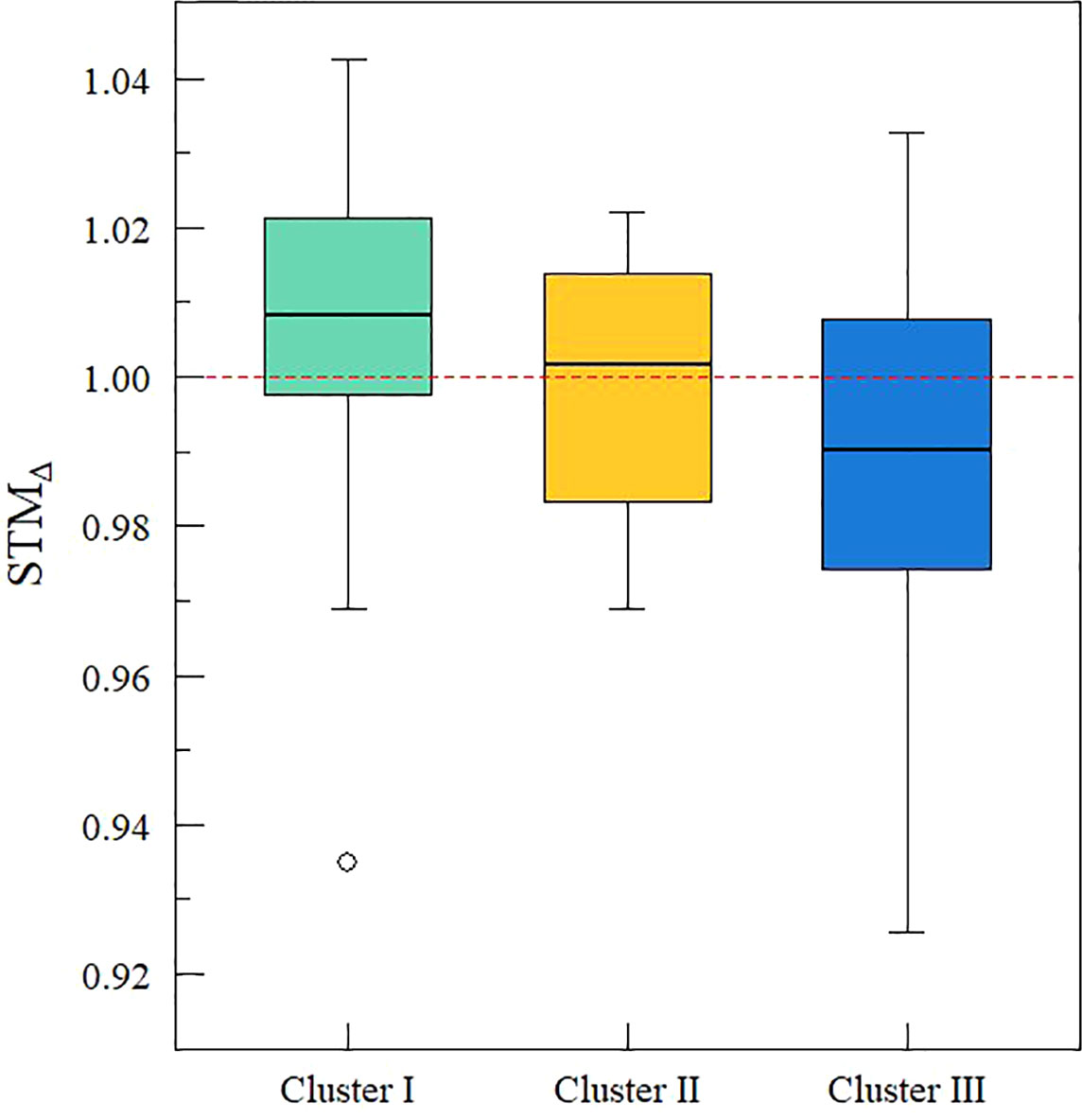
Figure 5 Boxplot of short-term memory (STM) effect on leaf 13C discrimination (STM∆) for each cluster. Red dashed line indicates the STM occurrence (STMΔ > 1). In each cluster’s boxplot, black line represents the median value. Boxplot contains the variation between 25 and 75% and gray circles are the outlier values.
Discussion
Priming Induction Was Expressed Mainly in Senescence Delay and Photosynthetic Performance Traits
The highest ChlSPAD values (51.4, 50.5, and 49.4 SPAD units for accessions CIP 440166, CIP 430434, and CIP 460528, respectively) observed in this study were higher than previously recorded in root and tuber crops such as sweetpotato (~42.0 SPAD units; Mbinda et al., 2018; Mbinda et al., 2019), cassava (47 SPAD units; Ogaddee and Girdthai, 2019), and potato (49 SPAD units; Rolando et al., 2015 and ~45 SPAD units; Ramírez et al., 2014). Also, the lower dTmin average value obtained here (−12.2 ± 0.7°C, for primed CIP 460531), was much lower than minimum dT values reported in potato (about −6°C; Stark et al., 1991) or alfalfa (approximately −10°C; Idso et al., 1981) under no-stress conditions. Given that the minimum value was achieved by primed plants in the recovery period of the third water restriction period, these results suggest an optimization in stomatal behavior as a result of the priming process. The highest Δmax average value (25.3‰ for primed CIP 460583) obtained in this study in the third water restriction period, was higher than previously reported for sweetpotato (21.7 ‰, Zhang et al., 2015 and 23.6‰, Ramírez et al., 2017) or potato (23‰, Ramírez et al., 2015b).
Studies of stress memory response in A. thaliana (Ling et al., 2018; Serrano et al., 2019) found an improvement in heat-stress tolerance after a second priming event. The ecophysiological indicators (S, FAmax, dTmin, and Δmax) assessed in our study indicated an improvement in primed plants after the first water restriction period. Delayed senescence was the most important trait increasing after water restriction events in 81.4% of the genotypes (Figure 2A). Delayed senescence is the result of a stress-response mechanism characterized by slower chlorophyll degradation over time, in comparison to unstressed genotypes (Thomas and Howarth, 2000; Rivero et al., 2007; Abdelrahman et al., 2017). In both potato (Rolando et al., 2015) and sweet potato (Smit, 1997; Bararyenya et al., 2020), delayed senescence has been attributed to outstanding yield, since it extends the period of time in which the plant can fix carbon. On the other hand, primed plants from 23.7% of the total number of accessions (Figure 2B) increased their foliar area (i.e. higher aerial biomass than non-primed plants) after the first water restriction period. These findings are corroborated by previous studies in which higher green leaf area was observed during recovery from early season drought in tolerant genotypes (Rivero et al., 2007; Puangbut et al., 2009; Lewthwaite and Triggs, 2012). Foliar area—a trait prioritized in breeding programs (Lenis et al., 2006; De Souza et al., 2017)—has been correlated to light interception and use efficiencies (Legg et al., 1979; Zhu et al., 2008; De Souza et al., 2017).
Another important trait to be considered in breeding programs that seek to improve drought tolerance is canopy temperature (Obidiegwu et al., 2015). The ability of plants to cool their leaves via stomata while at the same time saving water has been correlated with drought tolerance (Blum and Arkin, 1984; Blum, 2005; Hirayama et al., 2006; Ramírez et al., 2015b). The leaf-minus-air temperature (dT) has thus been used as an index of plant water status (Takai et al., 2010; Tuberosa, 2012; Iseki et al., 2018) or as an indirect method of indicating stomatal conductance (Jackson et al., 1977; Hatfield, 1983; Rinza et al., 2019). Previous studies have demonstrated that genotypes with the lowest dT values (here dTmin) had the highest transpiration and photosynthetic rates (Hirayama et al., 2006; Takai et al., 2010; Rukundo et al., 2017). These results are corroborated by our findings, in which primed plants from 17 accessions (Figure 2C) presented lower dT values in the second or third recovery period than those obtained by their respective non-primed plants throughout their lifespan. Our results suggest that a potential short-term memory induced cooling mechanism in primed plants enabled them to decrease leaf temperatures in response to hot and dry environments.
On the other hand, 13C discrimination integrates the photosynthetic performance throughout the period of leaf tissue synthetization (Jefferies and Mackerron, 1997; Dawson et al., 2002). According to literature, this trait is expected to decrease in drought conditions, due to a reduced discrimination against 13C by RuBisCO (Farquhar et al., 1989), and to partial recovery after re-watering (Xu et al., 2010; Ramírez et al., 2016; Silva-Díaz et al., 2020). In that respect, leaf 13C discrimination from primed plants, measured in every recovery period, reflects the effects of previous water restriction periods. Memory effects were determined by calculating the maximum leaf 13C discrimination (Δmax) reached by primed plants and comparing it with the one achieved by non-primed plants. Primed plants from 30 accessions (Figure 2D) mitigated the negative effects of the first water restriction period, and even reached a higher Δmax, at the end of the priming process, than non-primed plants grown under fully irrigated conditions suggesting a potential short-term memory occurrence. This improvement in photosynthetic performance has also been documented in potato (Ramírez et al., 2016; Silva-Díaz et al., 2020), wheat (Monneveux et al., 2005), and rice (Impa et al., 2005), but not on a level as high as observed in our study, induced by short-term memory in primed plants.
Of the four traits evaluated, the performance of senescence and photosynthesis were the most favorable following the priming process, which revealed their potential flexibility under stress memory induction. These results suggest that potential stress memory induction helps modify metabolic pathways related to chlorophyll degradation (Rivero et al., 2007; Abdelrahman et al., 2017) and photosynthesis recovery (Ramírez et al., 2016). Also, despite their corroborated relation to drought tolerance, FA and dT presented a lower percentage of STM occurrence, suggesting a more complex response enhanced by stress memory. Species such as I. leucantha, I. australis and I. grandifolia presented even higher STMS than sweetpotato cultivars (Figure 3A), leading us to recommend the consideration of these CWR species for use in breeding programs. Regarding photosynthetic performance, all SP-CWR species contained at least one accession in which STM occurrence (Figure 3D) suggests the potential of this group of species for easy recovery in environments with prolonged drought seasons.
A New Model for Drought Tolerance Improvement in Sweetpotato Based on Stress-Memory Induction and Wild Relatives
Resilience of a crop is the extent to which it is capable of surviving stress or other perturbations through physiological adaptations, with a minimum effect on yield (Holling, 1973; Trenbath, 1999). There is a differentiated capability of sweetpotato genotypes to allocate more carbon to above ground biomass than harvestable roots (e.g. Ejumula variety) under non-stress growth conditions (Coleman et al., 2006; Ramírez et al., 2017), however, the implications of this shifted resource allocation have not been explored yet. In our study, the capability to increase foliar area appears to be the main trait responsible for enhanced resilience (Table 2), an observation that is in agreement with previous studies on sorghum in which genotypes submitted to severe water restriction presented higher yields due to both increased leaf area and delayed senescence (Borrell et al., 2000). Moreover, in cassava, De Souza et al. (2017) highlight the importance of breeding for genotypes with increased leaf area to improve biomass production and drought response. Our results suggest that there is an association between delayed senescence and increased foliar area that elevated RCI response (Figure 4). In cereals, early-heading, which translates as a delayed senescence, is considered an escape strategy from adverse environmental conditions (Turner, 1979; Levitt, 1980; Dolferus, 2014) rather than representing a resilience response (Thiry et al., 2016). This study’s results suggest that delayed senescence is the cause of increased leaf area. This resilience response includes more and longer-living foliage, which provides more time and space to fix additional CO2 (Legg et al., 1979; Zhu et al., 2008; De Souza et al., 2017), and results in higher aerial biomass (Puangbut et al., 2009). However, the link between senescence delay and leaf biomass production is a relationship that should be explored more profoundly in the future.
Crop productivity has been associated with canopy temperature (e.g. dT) and the latter has been frequently used as a selection method for drought tolerance (Jackson et al., 1981; Jones, 2006; Takai et al., 2010; Zia et al., 2013). Some studies have shown that lower dT values are a consequence of higher stomatal conductance (Jackson et al., 1977; Hatfield, 1983; Rinza et al., 2019) and effective water uptake (Blum, 2009) representing the optimization of leaf thermoregulation and gas exchange. In our study, production capacity (PCI) was mainly related to STMdT (Table 2) confirming the aforementioned results of other authors. The combination of dT and leaf 13C discrimination were associated with elevated PCI values (Figure 4) suggesting that optimized leaf thermoregulation and gas exchange is a consequence of improved photosynthetic performance and recovery (Jefferies and Mackerron, 1997; Dawson et al., 2002), which ultimately leads to higher levels of productivity in SP-CWR.
Three groups of accessions performed differently in this study. Accessions from cluster I, presented higher biomass production in non-stress (Ynpr) than in water deficit conditions (Ypr) (Table 4). Accessions in this cluster correspond to Group B of Fernandez’s (1992) classification of plants based on biomass production (Table 1). Group B corresponds to Thiry et al. (2016) classification based on PCI and RCI, since accessions falling into group B also showed the highest average PCI value (4.39 ± 1.7). In cluster II, primed plants equaled the biomass production obtained from non-primed plants of the same accession (Table 4). This together with the highest average RCI value (7.0 ± 1.1), suggests that these accessions belong to Group C in Fernandez (1992) classification (Table 1). Cluster III of this study corresponds to Group D in Fernandez (1992) classification (Table 1) because the respective accessions had the lowest biomass production performance in both non-stress and stress conditions and the lowest PCI and RCI values (Table 4). Our results suggest that sweetpotato crop wild relatives possess outstanding physiological mechanisms to respond to both non-stress and water restriction scenarios. Primed SP-CWR species from cluster II (Table 1) such as I. triloba (CIP 460116) and I. trifida (CIP 107665.9, CIP 460663, and 113735.283) showed better biomass production performance under drought stress and hence could be considered for use in breeding programs (Thiry et al., 2016) to improve sweetpotato resilience, a trait much appreciated by breeders (Andrade et al., 2016). The aforementioned species coincide with previous studies (Iwanaga, 1988; Komaki, 2004; Zhang and Liu, 2005; Nimmakayala et al., 2011), which were based on biomass production under stress conditions but did not assess physiological performance. On the other hand, accessions from species such as I. splendor-sylvae, I. ramosissima, I. tiliacea, and wild I. batatas from cluster I could only be used as a potential genetic source of traits related to optimized leaf transpiration and photosynthetic performance.
The enhancement of drought tolerance in our study is also supported from a physiological point of view. Ramírez et al. (2015a) defined drought tolerance enhancement based on 13C discrimination, when primed plants presented higher 13C discrimination than non-primed plants. This physiological criterium matches our RCI-PCI drought tolerant genotypes from cluster I and II, as both of them contained the highest number of accessions with STM∆ occurrence (Figure 5). Therefore, we have demonstrated, experimentally, the existence of drought tolerance and, physiologically, the drought tolerance enhancement induced by short-term memory.
Due to ploidy differences of the taxa and other barriers to hybridization, interspecific hybridization between sweetpotato and related wild species is challenging and usually requires the employment of a pre-breeding approach. However, several authors have shown that the creation of interspecific hybrids is feasible using ovule culture (Kobayashi et al., 1994), somatic cell hybridization (Liu et al., 1994; Zhang et al., 2002; Yang et al., 2009), application of phytohormones in combination with controlled pollination (Cao et al., 2009), creation of synthetic hexaploids and triploids (Nishiyama et al., 1975; Shiotani and Kawase, 1987; Freyre et al., 1991), and interploidy hybridization (Orjeda et al., 1991). Successful production of hybrids between hexaploid sweetpotato and CWR species of the Batatas complex was reported for I. trifida (Orjeda et al., 1991; Kobayashi et al., 1994), I. triloba (Kobayashi et al., 1994; Liu et al., 1994; Yang et al., 2009), I. lacunosa (Kobayashi et al., 1994; Zhang et al., 2002), I. grandifolia (Cao et al., 2009), I. littoralis (Nishiyama et al., 1975), and I. leucantha (Nishiyama et al., 1975). Moreover, modern breeding techniques (e.g. CRISPR/Cas9-mediated genome editing) offer promising options for introgression of genes from wild relatives into the hexaploid cultivated genepool which need to be further explored (Wang et al., 2019).
Conclusion
Potential short-term memory induction constitutes a promising method to enhance physiological responses in SP-CWR. Primed accessions in this study showed physiological mechanisms (delayed senescence, increased foliar area, optimized leaf transpiration, or improved photosynthetic performance) that enable plants to cope with severe drought conditions. Because potential stress memory triggered the greatest increase in foliar area in I. tiliacea and some accessions from I. ramosissima (CIP 460032, CIP 460722) and I. triloba (CIP 460116), this trait might be more relevant in breeding for dual purpose sweetpotato cultivars, used to produce food as well as livestock feed. However, with a view toward long-term memory induction, more studies are required to elucidate the underlying molecular mechanisms (epigenetic processes, gene silencing, chromatin remodeling) responsible for drought tolerance improvement in sweetpotato as shown in this study. Furthermore, we showed that SP-CWR developed drought tolerance through two basic mechanisms: i) resilience, by developing more leaves with an increased time to fix carbon and ii) productivity, by optimizing leaf thermoregulation and gas exchange. The use of resilience capacity and productivity capacity, simultaneously, allowed us to easily identify genotypes from Group C, a group of plants that is highly appreciated by breeders. This study confirms the effectiveness of a potential short-term memory induction for enhancing plants’ drought stress response, the potential applications of which include more efficient water use in irrigated crops and the production of more resilient sweetpotato planting material. It also sets a precedent in stress memory in SP-CWR and demonstrates that this group constitutes a potential and untapped source of valuable physiological traits for sweetpotato improvement programs. However, further field trials under different environmental conditions and in different years are necessary to confirm our preliminary results.
Data Availability Statement
The original contributions presented in the study are publicly available. This data can be found here: [https://doi.org/10.21223/WNZR93].
Author Contributions
BH and DR conceived and designed the study. FG-Z gathered the data, analyzed the results, and wrote the manuscript. FG-Z, BH, DR, JR, and JN performed the analysis. FG-Z, BH, DR, JN, JR, and RB edited the manuscript.
Funding
This investigation was led by North Carolina State University (NCSU), who managed the Sweetpotato CWR project in collaboration with the International Potato Center (CIP) with support from the CGIAR Research Program on Roots, Tubers, and Bananas (RTB). The study was part of the initiative “Adapting Agriculture to Climate Change: Collecting, Protecting and Preparing Crop Wild Relatives” which is supported by the Government of Norway. The initiative is managed by the Global Crop Diversity Trust with the Millennium Seed Bank of the Royal Botanic Gardens, Kew, UK, and implemented in partnership with national and international genebanks and plant breeding institutes around the world. For further information, see Dempewolf et al. (2014), and http://www.cwrdiversity.org/.
Conflict of Interest
The authors declare that the research was conducted in the absence of any commercial or financial relationships that could be construed as a potential conflict of interest.
Acknowledgments
The authors would like to thank Dr. Craig Yencho, North Carolina State University (NCSU), who managed the Sweetpotato CWR project in collaboration with the International Potato Center (CIP). We also thank Carlos Flores, César Fernández, Genoveva Rossel, and Cecilia Silva for their technical support and assistance.
Supplementary Material
The Supplementary Material for this article can be found online at: https://www.frontiersin.org/articles/10.3389/fpls.2020.567507/full#supplementary-material
References
Abdelrahman, M., El-Sayed, M., Jogaiah, S., Burritt, D. J., Tran, L.-S. P. (2017). The “STAY-GREEN” trait and phytohormone signaling networks in plants under heat stress. Plant Cell Rep. 36, 1009–1025. doi: 10.1007/s00299-017-2119-y
Agili, S., Nyende, B. (2012). Selection, yield evaluation, drought tolerance indices of orange-flesh Sweet potato (Ipomoea batatas (L.) Lam.) hybrid clone. J. Nutr. Food Sci. 02, 1–8. doi: 10.4172/2155-9600.1000138
Ahmed, M., Qadeer, U., Ahmed, Z., II, Hassan, F. (2016). Improvement of wheat (Triticum aestivum) drought tolerance by seed priming with silicon. Arch. Agron. Soil Sci. 62, 299–315. doi: 10.1080/03650340.2015.1048235
Andrade, M., II, Naico, A., Ricardo, J., Eyzaguirre, R., Makunde, G. S., Ortiz, R., et al. (2016). Genotype × environment interaction and selection for drought adaptation in sweetpotato (Ipomoea batatas [L.] Lam.) in Mozambique. Euphytica 209, 261–280. doi: 10.1007/s10681-016-1684-4
Austin, D. F., Jarret, R. L., Johnson, R. W. (1993). Ipomoea gracilis R. Brown (Convolvulaceae) and its allies. Bull. Torrey Bot. Club. 120, 49–59. doi: 10.2307/2996663
Austin, D. F. (1978). The Ipomoea batatas Complex-I. Taxonomy. Bull. Torrey Bot. Club. 105, 114–129. doi: 10.2307/2484429
Bararyenya, A., Olukolu, B. A., Tukamuhabwa, P., Grüneberg, W. J., Ekaya, W., Low, J., et al. (2020). Genome-wide association study identified candidate genes controlling continuous storage root formation and bulking in hexaploid sweetpotato. BMC Plant Biol. 20, 1–16. doi: 10.1186/s12870-019-2217-9
Barreda, C., Guerrero, J., Cruz, M. (2017). Image Canopy: Software to estimate canopy cover (Lima, Peru: International Potato Center (CIP)). doi: 10.21223/P3/50TASS
Blum, A., Arkin, G. F. (1984). Sorghum root growth and water-use as affected by water supply and growth duration. Field Crops Res. 9, 131–142. doi: 10.1016/0378-4290(84)90019-4
Blum, A. (1996). “Yield potential and drought resistance: are they mutually exclusive?,” in Increasing yield potential in wheat: breaking the barriers. Eds. Reynolds, M. P., Rajaram, S., McNab, A. (Mexico DF: CIMMYT), 90–100.
Blum, A. (2005). Drought resistance, water-use efficiency, and yield potential—are they compatible, dissonant, or mutually exclusive? Aust. J. Agr. Res. 56, 1159–1168. doi: 10.1071/AR05069
Blum, A. (2009). Effective use of water (EUW) and not water-use efficiency (WUE) is the target of crop yield improvement under drought stress. Field Crops Res. 112, 119–123. doi: 10.1016/j.fcr.2009.03.009
Borrell, A. K., Hammer, G. L., Douglas, A. C. L. (2000). Does Maintaining Green Leaf Area in Sorghum Improve Yield under Drought? I. Leaf Growth and Senescence. Crop Sci. 40, 1026–1037. doi: 10.2135/cropsci2000.4041026x
Bradbury, H., Holloway, W. D. (1987). Chemistry of tropical root crops: significance for nutrition and agriculture in the Pacific (Canberra, Australia: ACIAR Monograph).
Bruce, T. J. A., Matthes, M. C., Napier, J. A., Pickett, J. A. (2007). Stressful “memories” of plants: Evidence and possible mechanisms. Plant Sci. 173, 603–608. doi: 10.1016/j.plantsci.2007.09.002
Cao, Q., Zhang, A., Ma, D., Li, H., Li, Q., Li, P. (2009). Novel interspecific hybridization between sweetpotato (Ipomoea batatas (L.) Lam.) and its two diploid wild relatives. Euphytica 169, 345–352. doi: 10.1007/s10681-009-9967-7
Cayón, M. G., El-Sharkawy, M. A., Cadavid, L. F. (1997). Leaf gas exchange of cassava as affected by quality of planting material and water stress. Phosynthetica 34, 409–418. doi: 10.1023/A:1006868219162
Coleman, E., Maltby, J., Harper, S., O’Donnell, W., Hughes, M. M., Boreel, S., et al. (2006). Improving sweetpotato agronomy to meet new market opportunities (Sidney, Australia: Horticulture Australia).
Condon, A. G., Richards, R. A., Rebetzke, G. J., Farquhar, G. D. (2004). Breeding for high water-use efficiency. J. Exp. Bot. 55, 2447–2460. doi: 10.1093/jxb/erh277
Conrath, U., Beckers, G. J. M., Flors, V., García-Agustín, P., Jakab, G., Mauch, F., et al. (2006). Priming: Getting Ready for Battle. Mol. Plant Microbe Interact. 19, 1062–1071. doi: 10.1094/MPMI-19-1062
Dawson, T. E., Mambelli, S., Plamboeck, A. H., Templer, P. H., Tu, K. P. (2002). Stable Isotopes in Plant Ecology. Ann. Rev. Ecol. Syst. 33, 507–559. doi: 10.1146/annurev.ecolsys.33.020602.095451
De Souza, A. P., Massenburg, L. N., Jaiswal, D., Cheng, S., Shekar, R., Long, S. P. (2017). Rooting for cassava: insights into photosynthesis and associated physiology as a route to improve yield potential. New Phytol. 213, 50–65. doi: 10.1111/nph.14250
Dempewolf, H., Eastwood, R. J., Guarino, L., Khoury, C. K., Müller, J. V., Toll, J. (2014). Adapting Agriculture to Climate Change: A Global Initiative to Collect, Conserve, and Use Crop Wild Relatives. Agroecol. Sust. Food 38, 369–377. doi: 10.1080/21683565.2013.870629
Dolferus, R. (2014). To grow or not to grow: A stressful decision for plants. Plant Sci. 229, 247–261. doi: 10.1016/j.plantsci.2014.10.002
FAO (2020). FAOSTAT Production Databases. Available at: http://faostat.fao.org/ (Accessed May 14, 2020).
Farquhar, G. D., Ehleringer, J. R., Hubick, K. T. (1989). Carbon isotope discrimination and photosynthesis. Ann. Rev. Plant Physiol. 40, 503–537. doi: 10.1146/annurev.pp.40.060189.002443
Fernandez, G. C. J. (1992). “Effective selection criteria for assessing plant stress tolerance,” in Proceeding of the International Symposium on Adaptation of Vegetables and other Food Crops in Temperature and Water Stress. Ed. Kuo, C. G. (Tainan, Taiwan: AVRDC), 257–270.
Fischer, R. A., Maurer, R. (1978). Drought resistance in spring wheat cultivars. I. Grain yield responses. Aust. J. Agric. Res. 29, 897. doi: 10.1071/AR9780897
Freyre, R., Iwanaga, M., Orjeda, G. (1991). Use of Ipomoea trifida (HBK.) G. Don germ plasm for sweet-potato improvement. 2. Fertility of synthetic hexaploids and triploids with 2 n gametes of I. trifida, and their interspecific crossability with sweet potato. Genome 34, 209–214. doi: 10.1139/g91-033
Guarino, L., Lobell, D. B. (2011). A walk on the wild side. Nat. Clim. Change 1, 374–375. doi: 10.1038/nclimate1272
Guerrero-Zurita, F., Ramirez, D., Rinza, J., Ninanya, J., Heider, B. (2020). “Replication data for: Potential Short-term Memory Induction as a Promising Method for Increasing Drought Tolerance Sweetpotato Crop Wild Relatives Ipomoea series Batatas (Choisy) Ed. Austin, D. F. Int. Potato Cent. 1. doi: 10.21223/WNZR93
Han, S. K., Wagner, D. (2014). Role of chromatin in water stress responses in plants. J. Exp. Bot. 65, 2785–2799. doi: 10.1093/jxb/ert403
Hatfield, J. L. (1983). The utilization of thermal infrared radiation measurements from grain sorghum crops as a method of assessing their irrigation requirements. Irrig. Sci. 3, 259–268. doi: 10.1007/BF00272841
Hirayama, M., Wada, Y., Nemoto, H. (2006). Estimation of drought tolerance based on leaf temperature in upland rice breeding. Breed. Sci. 56, 47–54. doi: 10.1270/jsbbs.56.47
Holling, C. S. (1973). Resilience and Stability of Ecological Systems. Ann. Rev. Ecol. Syst. 4, 1–23. doi: 10.1146/annurev.es.04.110173.000245
Idso, S. B., Jackson, R. D., Pinter, P. J., Reginato, R. J., Hatfield, J. L. (1981). Normalizing the stress-degree-day parameter for environmental variability. Agr. Meteorol. 24, 45–55. doi: 10.1016/0002-1571(81)90032-7
Impa, S. M., Nadaradjan, S., Boominathan, P., Shashidhar, G., Bindumadhava, H., Sheshshayee, M. S. (2005). Carbon Isotope Discrimination Accurately Reflects Variability in WUE Measured at a Whole Plant Level in Rice. Crop Sci. 45, 2517–2522. doi: 10.2135/cropsci2005.0119
Iseki, K., Takahashi, Y., Muto, C., Naito, K., Tomooka, N. (2018). Diversity of Drought Tolerance in the Genus Vigna. Front. Plant Sci. 9, 729. doi: 10.3389/fpls.2018.00729
Iwanaga, M. (1988). “Use of wild germplasm for sweetpotato breeding,” in Exploration, Maintaince and Utilization of Sweetpotato Genetic Resources (Lima: International Potato Center (CIP), 199–210.
Jackson, R. D., Reginato, R. J., Idso, S. B. (1977). Wheat canopy temperature: A practical tool for evaluating water requirements. Water Resour. Res. 13, 651–656. doi: 10.1029/WR013i003p00651
Jackson, R. D., Idso, S. B., Reginato, R. J., Pinter, P. J. (1981). Canopy temperature as a crop water stress indicator. Water Resour. Res. 17, 1133–1138. doi: 10.1029/WR017i004p01133
Jefferies, R. A., Mackerron, D. K. L. (1997). Carbon isotope discrimination in irrigated and droughted potato (Solanum tuberosum L.). Plant Cell Env. 20, 124–130. doi: 10.1046/j.1365-3040.1997.d01-5.x
Jones, H. G. (2006). Monitoring plant and soil water status: established and novel methods revisited and their relevance to studies of drought tolerance. J. Exp. Bot. 58, 119–130. doi: 10.1093/jxb/erl118
Khoury, C. K., Heider, B., Castañeda-Álvarez, N. P., Achicanoy, H. A., Sosa, C. C., Miller, R. E., et al. (2015). Distributions, ex situ conservation priorities, and genetic resource potential of crop wild relatives of sweetpotato [Ipomoea batatas (L.) Lam., I. series Batatas]. Front. Plant Sci. 6, 251. doi: 10.3389/fpls.2015.00251
Kivuva, B. M., Githiri, S. M., Yencho, G. C., Sibiya, J. (2015). Screening sweetpotato genotypes for tolerance to drought stress. Field Crops Res. 171, 11–22. doi: 10.1016/j.fcr.2014.10.018
Knox, J., Hess, T., Daccache, A., Wheeler, T. (2012). Climate change impacts on crop productivity in Africa and South Asia. Environ. Res. Lett. 7, 34032. doi: 10.1088/1748-9326/7/3/034032
Kobayashi, R. S., Bouwkamp, J. C., Sinden, S. L. (1994). Interspecific hybrids from cross incompatible relatives of sweetpotato. Euphytica 80, 159–164. doi: 10.1007/BF00039646
Komaki, K. (2004). “Breeding value of wild species closely related to sweetpotato,” in Proceedings of international workshop on production, utilization and development of sweetpotato (Korea: AIST), 164–172.
Laurie, R. N., Laurie, S. M., Du Plooy, C. P., Finnie, J. F., Staden, J. V. (2014). Yield of Drought-tressed Sweet Potato in Relation to Canopy Cover, Stem Length and Stomatal Conductance. J. Agric. Sci. 7, 201–214. doi: 10.5539/jas.v7n1p201
Lê, S., Josse, J., Husson, F. (2008). FactoMineR: an R package for multivariate analysis. J. Stat. Software 25 (1), 1–18. doi: 10.18637/jss.v025.i01
Legg, B. J., Day, W., Lawlor, D. W., Parkinson, K. J. (1979). The effects of drought on barley growth: models and measurements showing the relative importance of leaf area and photosynthetic rate. J. Agr. Sci. 92, 703–716. doi: 10.1017/S0021859600053958
Lenis, J., II, Calle, F., Jaramillo, G., Perez, J. C., Ceballos, H., Cock, J. H. (2006). Leaf retention and cassava productivity. Field Crops Res. 95, 126–134. doi: 10.1016/j.fcr.2005.02.007
Lewthwaite, S. L., Triggs, C. M. (2012). Sweetpotato cultivar response to prolonged drought. Agr. New Zeal. 42, 1–10.
Li, X., Ramírez, D. A., Qin, J., Dormatey, R., Bi, Z., Sun, C., et al. (2019). Water restriction scenarios and their effects on traits in potato with different degrees of drought tolerance. Sci. Hortic. 256, 108525. doi: 10.1016/j.scienta.2019.05.052
Ling, Y., Serrano, N., Gao, G., Atia, M., Mokhtar, M., Woo, Y. H., et al. (2018). Thermopriming triggers splicing memory in Arabidopsis. J. Exp. Bot. 69, 2659–2675. doi: 10.1093/jxb/ery062
Liu, H., Charng, Y. (2012). Acquired thermotolerance independent of heat shock factor A1 (HsfA1), the master regulator of the heat stress response. Plant Signal. Behav. 7, 547–550. doi: 10.4161/psb.19803
Liu, Q. C., Wang, J. X., Li, W. J., Zhou, H. Y. (1994). Protoplast fusion and regeneration of interspecific somatic hybrid plants between sweet potato (Ipomoea batatas (L.) Lam.) and its related species. J. Agric. Biotechnol. 2, 85–90.
Low, J., Lynam, J., Lemaga, B., Crissman, C., Barker, I., Thiele, G., et al. (2009). “Sweetpotato in Sub-Saharan Africa,” in The Sweetpotato. Eds. Loebenstein, G., Thottappilly, G. (Dordrecht: Springer Netherlands), 359–390. doi: 10.1007/978-1-4020-9475-0_16
Low, J. W., Ortiz, R., Vandamme, E., Andrade, M., Biazin, B., Grüneberg, W. J. (2020). Nutrient-Dense Orange-Fleshed Sweetpotato: Advances in Drought-Tolerance Breeding and Understanding of Management Practices for Sustainable Next-Generation Cropping Systems in Sub-Saharan Africa. Front. Sustain. Food Syst. 4, 50. doi: 10.3389/fsufs.2020.00050
Mbinda, W., Ombori, O., Dixelius, C., Oduor, R. (2018). Xerophyta viscosa Aldose Reductase, XvAld1, Enhances Drought Tolerance in Transgenic Sweetpotato. Mol. Biotechnol. 60, 203–214. doi: 10.1007/s12033-018-0063-x
Mbinda, W., Dixelius, C., Oduor, R. (2019). Induced Expression of Xerophyta viscosa XvSap1 Gene Enhances Drought Tolerance in Transgenic Sweet Potato. Front. Plant Sci. 10, 1119. doi: 10.3389/fpls.2019.01119
McDonald, J. A., Austin, D. F. (1990). Changes and Additions in Ipomoea Section Batatas (Convolvulaceae). Brittonia 42, 116. doi: 10.2307/2807625
Medrano, H., Escalona, J. M., Bota, J., Gulías, J., Flexas, J. (2002). Regulation of photosynthesis of C3 plants in response to progressive drought: stomatal conductance as a reference parameter. Ann. Bot. 89, 895–905. doi: 10.1093/aob/mcf079
Mekonnen, B., Tulu, S., Nego, J. (2015). Orange Fleshed Sweet Potato (Ipomoea batatas (L.) Lam.) Varieties Evaluated with Respect to Growth Parameters at Jimma in Southwestern Ethiopia. J. Agron. 14, 164–169. doi: 10.3923/ja.2015.164.169
Monneveux, P., Reynolds, M. P., Trethowan, R., González-Santoyo, H., Peña, R. J., Zapata, F. (2005). Relationship between grain yield and carbon isotope discrimination in bread wheat under four water regimes. Eur. J. Agron. 22, 231–242. doi: 10.1016/j.eja.2004.03.001
Motsa, N. M., Modi, A. T., Mabhaudhi, T., Mabhaudhi, T. (2015). Sweet potato (Ipomoea batatas (L.) Lam.) as a drought tolerant and food security crop. South Afr. J. Sci. 111, 1–8. doi: 10.17159/sajs.2015/20140252
Mwanga, R. O. M., Ssemakula, G. (2011). Orange-fleshed sweetpotatoes for food, health and wealth in Uganda. Int. J. Agr. Sustain. 9, 42–49. doi: 10.3763/ijas.2010.0546
Nimmakayala, P., Vajja, G., Reddy, U. K. (2011). “Ipomoea,” in Wild Crop Relatives: Genomic and Breeding Resources. Ed. Kole, C. (Berlin, Heidelberg: Springer), 123–132. doi: 10.1007/978-3-642-21102-7_7
Nishiyama, I., Miyazaki, T., Sakamoto, S. (1975). Evolutionary autoploidy in the sweet potato (Ipomoea batatas (L.) Lam.) and its progenitors. Euphytica 24, 197–208. doi: 10.1007/BF00147186
Obidiegwu, J. E., Bryan, G. J., Jones, H. G., Prashar, A. (2015). Coping with drought: stress and adaptive responses in potato and perspectives for improvement. Front. Plant Sci. 6, 542. doi: 10.3389/fpls.2015.00542
Ogaddee, P., Girdthai, T. (2019). Physiological and ethylene accumulation responses of cassava under drought stress. IOP Conf. Ser. Earth Environ. Sci. 346, 12092. doi: 10.1088/1755-1315/346/1/012092
Orjeda, G., Freyre, R., Iwanaga, M. (1991). Use of Ipomoea trifida germ plasm for sweet potato improvement. 3. Development of 4x interspecific hybrids between Ipomoea batatas (L.) Lam. (2n=6x=90) and I. trifida (H.B.K) G. Don. (2n=2x=30) as storage-root initiators for wild species. Theoret. Appl. Genet. 83, 159–163. doi: 10.1007/BF00226245
Ozias-Akins, P., Jarret, R. L. (1994). Nuclear DNA Content and Ploidy Levels in the Genus Ipomoea. J. Am. Soc Hortic. Sci. 119, 110–115. doi: 10.21273/JASHS.119.1.110
Production Systems and Environment (PSE), International Potato Center (CIP) (2013). Protocol for Designing and Conducting Potato Field Experiments for Modeling Purposes. (Lima, Peru: CIP), 1–16. doi: 10.4160/9789290604303
Puangbut, D., Jogloy, S., Vorasoot, N., Akkasaeng, C., Kesmalac, T., Patanothai, A. (2009). Variability in yield responses of peanut (Arachis hypogaea L.) genotypes under early season drought. Asian J. Plant Sci. 8, 254–264. doi: 10.3923/ajps.2009.254.264
R Core Team (2019). A language and environment for statistical computing (Vienna, Austria: R Foundation for Statistical Computing). Available at: https://www.R-project.org/
Ramírez, D. A., Yactayo, W., Gutiérrez, R., Mares, V., De Mendiburu, F., Posadas, A., et al. (2014). Chlorophyll concentration in leaves is an indicator of potato tuber yield in water-shortage conditions. Sci. Hortic. 168, 202–209. doi: 10.1016/j.scienta.2014.01.036
Ramírez, D. A., Rolando, J. L., Yactayo, W., Monneveux, P., Mares, V., Quiroz, R. (2015a). Improving potato drought tolerance through the induction of long-term water stress memory. Plant Sci. 238, 26–32. doi: 10.1016/j.plantsci.2015.05.016
Ramírez, D. A., Rolando, J. L., Yactayo, W., Monneveux, P., Quiroz, R. (2015b). Is Discrimination of 13C in potato leaflets and tubers an appropriate trait to describe genotype responses to restrictive and well-watered conditions? J. Agron. Crop Sci. 201, 410–418. doi: 10.1111/jac.12119
Ramírez, D. A., Yactayo, W., Rens, L. R., Rolando, J. L., Palacios, S., De Mendiburu, F., et al. (2016). Defining biological thresholds associated to plant water status for monitoring water restriction effects: Stomatal conductance and photosynthesis recovery as key indicators in potato. Agr. Water Manage. 177, 369–378. doi: 10.1016/j.agwat.2016.08.028
Ramírez, D. A., Gavilán, C., Barreda, C., Condori, B., Rossel, G., Mwanga, R. O. M., et al. (2017). Characterizing the diversity of sweetpotato through growth parameters and leaf traits: Precocity and light use efficiency as important ordination factors. S. Afr. J. Bot. 113, 192–199. doi: 10.1016/j.sajb.2017.08.009
Rinza, J., Ramírez, D. A., García, J., de Mendiburu, F., Yactayo, W., Barreda, C., et al. (2019). Infrared Radiometry as a Tool for Early Water Deficit Detection: Insights into Its Use for Establishing Irrigation Calendars for Potatoes Under Humid Conditions. Potato Res. 62, 109–122. doi: 10.1007/s11540-018-9400-5
Rivero, R. M., Kojima, M., Gepstein, A., Sakakibara, H., Mittler, R., Gepstein, S., et al. (2007). Delayed leaf senescence induces extreme drought tolerance in a flowering plant. Proc. Natl. Acad. Sci. 104, 19631–19636. doi: 10.1073/pnas.0709453104
Rolando, J. L., Ramírez, D. A., Yactayo, W., Monneveux, P., Quiroz, R. (2015). Leaf greenness as a drought tolerance related trait in potato (Solanum tuberosum L.). Environ. Exp. Bot. 110, 27–35. doi: 10.1016/j.envexpbot.2014.09.006
Rukundo, P., Shimelis, H., Laing, M., Gahakwa, D. (2017). Combining Ability, Maternal Effects, and Heritability of Drought Tolerance, Yield and Yield Components in Sweetpotato. Front. Plant Sci. 7, 1981. doi: 10.3389/fpls.2016.01981
Schulze, R. (2011). Approaches towards practical adaptive management options for selected water-related sectors in South Africa in a context of climate change. Water SA 37, 621–646. doi: 10.4314/wsa.v37i5.1
SENAMHI (2020). Mapa Climático del Perú. Available at: https://senamhi.gob.pe/?&p=mapa-climatico-del-peru (Accessed January 22, 2020).
Serrano, N., Ling, Y., Bahieldin, A., Mahfouz, M. M. (2019). Thermopriming reprograms metabolic homeostasis to confer heat tolerance. Sci. Rep. 9, 1–14. doi: 10.1038/s41598-018-36484-z
Sharma, S., Upadhyaya, H. D., Varshney, R. K., Gowda, C. L. L. (2013). Pre-breeding for diversification of primary gene pool and genetic enhancement of grain legumes. Front. Plant Sci. 4, 309. doi: 10.3389/fpls.2013.00309
Shiotani, I., Kawase, T. (1987). Synthetic hexaploids derived from wild species related to sweet potato. Jpn. J. Breed. 37, 367–376. doi: 10.1270/jsbbs1951.37.367
Silva-Díaz, C., Ramírez, D. A., Rodríguez-Delfín, A., de Mendiburu, F., Rinza, J., Ninanya, J., et al. (2020). Unraveling Ecophysiological Mechanisms in Potatoes under Different Irrigation Methods: A Preliminary Field Evaluation. Agron 10, 827. doi: 10.3390/agronomy10060827
Smit, N. E. J. M. (1997). The effect of the indigenous cultural practices of in-ground storage and piecemeal harvesting of sweetpotato on yield and quality losses caused by sweetpotato weevil in Uganda. Agr. Ecosyst. Environ. 64, 191–200. doi: 10.1016/S0167-8809(97)00022-4
Stark, J. C., Pavek, J. J., McCann, I. R. (1991). Using Canopy Temperature Measurements to Evaluate Drought Tolerance of Potato Genotypes. J. Am. Soc Hortic. Sci. 116, 412–415. doi: 10.21273/JASHS.116.3.412
Takai, T., Yano, M., Yamamoto, T. (2010). Canopy temperature on clear and cloudy days can be used to estimate varietal differences in stomatal conductance in rice. Field Crops Res. 115, 165–170. doi: 10.1016/j.fcr.2009.10.019
Thiry, A. A., Chavez Dulanto, P. N., Reynolds, M. P., Davies, W. J. (2016). How can we improve crop genotypes to increase stress resilience and productivity in a future climate? A new crop screening method based on productivity and resistance to abiotic stress. J. Exp. Bot. 67, 5593–5603. doi: 10.1093/jxb/erw330
Thomas, H., Howarth, C. J. (2000). Five ways to stay green. J. Exp. Bot. 51, 329–337. doi: 10.1093/jexbot/51.suppl_1.329
Trenbath, B. R. (1999). Multispecies cropping systems in India: Predictions of their productivity, stability, resilience and ecological sustainability. Agrofor. Syst. 45, 81–107. doi: 10.1023/A:1006285319817
Tuberosa, R. (2012). Phenotyping for drought tolerance of crops in the genomics era. Front. Physiol. 3, 347. doi: 10.3389/fphys.2012.00347
Turner, N. (1979). “Drought resistance and adaptation to water deficits in crop plants,” in Stress physiology in crop plants. Eds. Mussell, H., Staples, R. C. (New York: John Wiley and Sons), 343–372.
Turner, N. (1986). Adaptation to Water Deficits: a Changing Perspective. Funct. Plant Biol. 13, 175. doi: 10.1071/PP9860175
Turner, N. (1997). “Further Progress in Crop Water Relations,” in Advances in Agronomy. (Western Australia, Australia: Elsevier), 293–338. doi: 10.1016/S0065-2113(08)60258-8
Valverde, R. A., Clark, C. A., Valkonen, J. P. T. (2007). Viruses and virus disease complexes of sweet potato. Plant Viruses 1, 116–126.
Walter, J., Nagy, L., Hein, R., Rascher, U., Beierkuhnlein, C., Willner, E., et al. (2011). Do plants remember drought? Hints towards a drought-memory in grasses. Environ. Exp. Bot. 71, 34–40. doi: 10.1016/j.envexpbot.2010.10.020
Wang, H., Wu, Y., Zhang, Y., Yang, J., Fan, W., Zhang, H., et al. (2019). CRISPR/Cas9-Based Mutagenesis of Starch Biosynthetic Genes in Sweet Potato (Ipomoea Batatas) for the Improvement of Starch Quality. Int. J. Mol. Sci. 20, 4702. doi: 10.3390/ijms20194702
Watkinson, J. I., Hendricks, L., Sioson, A. A., Vasquez-Robinet, C., Stromberg, V., Heath, L. S., et al. (2006). Accessions of Solanum tuberosum ssp. andigena show differences in photosynthetic recovery after drought stress as reflected in gene expression profiles. Plant Sci. 171, 745–758. doi: 10.1016/j.plantsci.2006.07.010
Wood, J. R. I., Carine, M. A., Harris, D., Wilkin, P., Williams, B., Scotland, R. W. (2015). Ipomoea (Convolvulaceae) in Bolivia. Kew Bull. 70, 1–124. doi: 10.1007/s12225-015-9592-7
Wood, J. R. I., Muñoz-Rodríguez, P., Williams, B. R. M., Scotland, R. W. (2020). A foundation monograph of Ipomoea (Convolvulaceae) in the New World. PhytoKeys 143, 1–823. doi: 10.3897/phytokeys.143.32821
Woolfe, J. A. (1992). Sweet potato: an untapped food resource (Cambridge, England: Cambridge University Press).
Wu, S., Lau, K. H., Cao, Q., Hamilton, J. P., Sun, H., Zhou, C., et al. (2018). Genome sequences of two diploid wild relatives of cultivated sweetpotato reveal targets for genetic improvement. Nat. Commun. 9, 1–12. doi: 10.1038/s41467-018-06983-8
Xu, Z., Zhou, G., Shimizu, H. (2010). Plant responses to drought and rewatering. Plant Signal. Behav. 5, 649–654. doi: 10.4161/psb.5.6.11398
Yang, Y. F., Guan, S. K., Zhai, H., He, S. Z., Liu, Q. C. (2009). Development and evaluation of a storage root-bearing sweetpotato somatic hybrid between Ipomoea batatas (L.) Lam. and I. triloba L. Plant Cell Tiss Organ Cult. 99, 83–89. doi: 10.1007/s11240-009-9578-y
Zhang, S. S., Liu, L. F. (2005). “Utilization of the wild relatives in sweetpotato breeding,” in Sweetpotato Breeding and Industrialization in China. Ed. Liu, Q. C. (Beijing: China Agricultural University Press), 29–32.
Zhang, B. Y., Liu, Q. C., Zhai, H., Zhou, H. Y. (2002). Production of fertile interspecific somatic hybrid plants between sweetpotato and it wild relative, Ipomoea lacunosa. Acta Hortic. 583, 81–85. doi: 10.17660/ActaHortic.2002.583.8
Zhang, H., Zhu, L., Zhang, C., Ning, Y., Zhang, Y. (2015). Effect of water stress on dry mass accumulation and allocation in sweet potato based on stable isotope analysis. Can. J. Plant Sci. 95, 263–271. doi: 10.4141/cjps-2014-170
Zhu, X. G., Long, S. P., Ort, D. R. (2008). What is the maximum efficiency with which photosynthesis can convert solar energy into biomass? Curr. Opin. Biotech. 19, 153–159. doi: 10.1016/j.copbio.2008.02.004
Keywords: senescence delay, foliar area, leaf temperature, 13C discrimination, drought stress memory, Batatas complex
Citation: Guerrero-Zurita F, Ramírez DA, Rinza J, Ninanya J, Blas R and Heider B (2020) Potential Short-Term Memory Induction as a Promising Method for Increasing Drought Tolerance in Sweetpotato Crop Wild Relatives [Ipomoea series Batatas (Choisy) D. F. Austin]. Front. Plant Sci. 11:567507. doi: 10.3389/fpls.2020.567507
Received: 29 May 2020; Accepted: 12 August 2020;
Published: 03 September 2020.
Edited by:
Pietro Gramazio, University of Tsukuba, JapanReviewed by:
Robert Jarret, United States Department of Agriculture, United StatesQingchang Liu, China Agricultural University, China
Conxita Royo, Institute of Agrifood Research and Technology (IRTA), Spain
Copyright © 2020 Guerrero-Zurita, Ramírez, Rinza, Ninanya, Blas and Heider. This is an open-access article distributed under the terms of the Creative Commons Attribution License (CC BY). The use, distribution or reproduction in other forums is permitted, provided the original author(s) and the copyright owner(s) are credited and that the original publication in this journal is cited, in accordance with accepted academic practice. No use, distribution or reproduction is permitted which does not comply with these terms.
*Correspondence: Bettina Heider, Qi5IZWlkZXJAY2dpYXIub3Jn