- 1College of Horticulture and Landscape Architecture, Northeast Agricultural University, Harbin, China
- 2Chair of Crop Science and Plant Biology, Estonian University of Life Sciences, Tartu, Estonia
Senescence is the main limitation for cut foliage display in vase. Naturally occurring polyamines such as putrescine (Put) have been considered effective anti-senescence agents. However, effect of Put on cut foliage in vase in a realistic indoor environment has not yet been revealed. In the present study, effects of Put spraying on the postharvest performance of cut foliage of Nephrolepis cordifolia L. were investigated. Cut fronds sprayed with deionized water (Put0) showed visible injuries after 10 days in vase. Meanwhile, chlorophyll (Chl), soluble protein (Sp), and proline (Pro) content were decreased by 60.15, 57.93, and 73.09% respectively, photochemical activity reflected by Chl fluorescence parameters was inhibited, whereas electrolyte leakage (EL), contents of soluble sugar (Ss), malondialdehyde (MDA), and hydrogen peroxide (H2O2) were increased (+194.29, +44.83, +34.06, and +178.01%, respectively). Put spraying extended the vase life of the cut foliage and the 2.0 mM Put had a longer vase life (21 days) than 0.2 mM (15 days). Leaf spraying of 2.0 mM Put for 10 days significantly ameliorated the losses of Chl, Sp, and Pro content (−10.72, −26.29, and −42.64%, respectively), followed by 0.2 mM Put (−27.36, −36.24, and −60.55%, respectively). Put spraying also improved photochemical capability and prevented membrane impairment as well as visible injury in comparison with Put0. In addition, 2.0 mM Put had a better mitigating ability than that of 0.2 mM. Leaf spraying of 2.0 mM Put greatly reduced the decline of the effective quantum yield of photochemical energy conversion in PSII (ΦPSII), the maximal quantum yield of PSII photochemistry measured in the dark-adapted state (Fv/Fm) and electron transport rate (ETR) (−7.89, −12.91, and −10.06%, respectively), and also inhibited the increases of EL, MDA, Ss, and H2O2 (+31.87, +6.43, +16.22, and +49.40%, respectively). Overall, Put played important roles in deterring the degradation of Chl, Ss, and Pro, detoxifying the H2O2, weakening the sugar signaling, mitigating the decline of photochemical activity, and eventually postponing the leaf senescence. The present study gives new insights into effects of Put on leaf senescence and provides a strategy for preserving post-harvest cut foliage.
Introduction
In recent decades, with continuous improvement of standard of living and increasing desire for a better indoor environment, indoor greening and decoration has emerged, with a strong demand for cut flowers and foliage. The short vase life and the low ability to cope with unsuitable environment during floral arrangement and display are the most important constraints for the commercialization of cut flowers and foliage (Zamani et al., 2011; Zhao et al., 2017).
For cut flowers and foliage, senescence is the main reason for short vase life (Shabanian et al., 2018). Although cut foliage plays important roles in floriculture industry, studies on its postharvest senescence are very limited compared with cut flowers (Malakar et al., 2017; Che-Husin et al., 2018; Lin et al., 2019). Senescence caused by the detachment of leaves from plants is associated with various biochemical and physiological changes. Leaf yellowing is often the first visible symptom of senescence, mainly due to the loss of chlorophyll (Chl) (Skutnik et al., 2004). It has been reported that after leaves were detached from rabbit’s foot fern (Phlebodium aureum), Chl a and Chl b contents decreased (Teerarak and Laosinwattana, 2019). Chl breakdown often occurs due to the remobilization of nitrogen from Chl-binding proteins to other processes during leaf senescence (Hörtensteiner, 2006). Besides Chl, protein is also considered as a marker of senescence since it degrades during leaf senescence (Breeze et al., 2011). Furthermore, many studies have found that reactive oxygen species (ROS) level increases during leaf senescence (Merzlyak and Hendry, 1994; Breusegem and Dat, 2006). Plants orchestrate a myriad of antioxidants such as ascorbic acid (AsA) as a strategy to cope with the detrimental ROS. For instance, it has been observed that increases in peroxidase (POD) and superoxide dismutase (SOD) activity occur in senescing leaves (Kim et al., 2004). Moreover, senescence induces an increase in the content of polyamines such as putrescine (Put), spermidine (Spd), and spermine (Spm) (Serrano et al., 2001; Sobieszczuk-Nowicka et al., 2016). Study on the maximum quantum yield of photosystem II (PSII) photochemistry measured in the dark-adapted state (Fv/Fm) shows that it decreases in Sorghum bicolor during leaf senescence (Chen et al., 2015).
Polyamines (PAs) are biodegradable organic compounds and have no adverse environmental effects (Sharma et al., 2017). Naturally occurring PAs such as Put have been reported as effective anti-senescence agents and lower Put content was found in aged cell (Paschalidis and Roubelakis-Angelakis, 2005; Kusano et al., 2008). The addition of PAs (Put, Spd, and Spm) increases the longevity of isolated flowers of Nicotiana plumbaginifolia (Nisar et al., 2015). Conditioning of cut stems of rose in Put solution results in a higher Chl a and Chl b content (Rubinowska et al., 2012). Exogenously supplied Put reduces hydrogen peroxide (H2O2) accumulation, electrolyte leakage (EL) and malondialdehyde (MDA) content, while increasing the activities of catalase (CAT) and ascorbate peroxidase (APX), thereby prolonging the vase life of cut lisianthus flowers (Ataii et al., 2015). Leaf senescence is different from petal senescence. For example, cut foliage can still sustain photosynthesis and do not need energy to maintain its survival at the late stage of senescence process (Zhang and Becker, 2015). However, information about the effects of PAs on cut foliage is very limited. In the limited studies, scientists focused on the roles of PAs in Chl and protein loss and antioxidant system with or without light (Altman, 1982; Cho and Hong, 1988). Generally, these studies have uncoupled the dark and light responses to investigate the senescence mechanisms. However, to reveal how Put affects postharvest quality of cut foliage, the holistic effects on leaf senescence should be considered in a realistic light-dark cycle. To our best knowledge, relevant reports are very few (Sood and Nagar, 2003). Furthermore, the state of art technology supplies novel insights to reveal the mechanism of leaf senescence. For example, Chl fluorescence imaging provides a non-destructive method to detect the photochemical damage in a whole leaf scale and very suitable for studying the anti-aging effect of Put. However, this method has not yet been applied in this field.
Sword fern (N. cordifolia L.) is commonly used in the garden and its cut green foliage has a high decorative value in floral arrangements due to it providing a vertical accent to floral designs (Safeena et al., 2019). In addition, it is available throughout the year. Therefore, in the present study, we selected sword fern as plant material and aimed to investigate the potential mitigating effects of Put on the senescence of cut foliage under realistic indoor environment and to reveal the underlying physiological and biochemical mechanisms. These results could provide a theoretical basis for prolonging the vase life of cut foliage using exogenous Put.
Materials and Methods
Plant Material
Sword fern (N. cordifolia L.) was selected as plant material. A total of 50 pots of plants were bought from Harbin Flower Market, CN. The diameter of the pots was 16 cm and the average height of the plants was ca. 30 cm. Before treatments, the plants were adapted for one week in a greenhouse where the average temperature was ca. 25 °C and relative humidity ca. 65%. During this period, the light period was 16/8 h (day/night), and the average light intensity was ca. 800 µmol (photon) m-2 s-1. The plants were irrigated to the soil water capacity every day.
Treatment and Vase Life Observation
A total of 72 healthy fronds with similar size (ca. 20 cm long, in which both the lamina and the stipe were 10 cm) were cut from the plants using a sharp knife, then each frond was immediately placed upright into one individual conical flask containing 100 ml of deionized water. The conical flasks were covered with transparent plastic films to decrease water evaporation and placed on a table in the laboratory. Fronds together with conical flasks were randomly and equally divided into four groups (18 cut fronds for each group). For the control group, the cut fronds were observed and sampled immediately, then the samples were stored in -80 °C for the following measurements. The other three groups were sprayed with 0.2 mM Put (Put0.2), 2.0 mM Put (Put2.0), or deionized water (Put0), respectively. The Put concentrations were selected based on references (Yiu et al., 2009; Rubinowska et al., 2012) and a preliminary assay. During the preliminary assay, we used 0.2, 2.0, 4.0 mM Put to test whether they can extend the vase life of cut fronds and found that the frond sprayed with 4.0 mM Put had a shorter vase life than that with Put0 (data not shown). Spraying treatments (2 ml for each frond) were conducted every day in the morning (09:00 am) for consecutive 10 days till 50% of the fronds in Put0 group showed leaflet drop. Then 12 cut fronds of each group were observed and sampled immediately. The samples were also stored in -80 °C for the following measurements. The remaining six cut fronds in each group continue to be sprayed as before to observe the vase life. When 50% of the fronds in each group showed leaflet drop, the vase life was recorded. During the treatment period, the range of relative humidity was ca. 40–55%, the temperature ca. 28/24 °C (day/night), the light period 16/8 h (day/night), and the average light intensity ca. 100 µmol (photon) m-2 s-1.
Leaf Appearance
The cut foliage was photographed before and after 10 days of treatment in different groups. Six intact fronds in each treatment group were selected. The percentage of injured area on each cut frond was observed and recorded by three surveyors using a 5 and 1% step scale independently (Paoletti et al., 2009).
Chl Content
Fresh leaflet (0.3 g, n = 6) was sampled and ground with quartz sand and ethanol (95%, v/v), then the extract was filtered into a 25 ml brown volumetric flask, in which the volume was kept at 25 ml using ethanol (95%, v/v). Absorbance of the extracting solution was measured at 470, 649, 665 nm using an Ultraviolet-Visible spectrophotometer (T6 New Century, CN). Contents of Chl a, Chl b, and total Chl, were calculated according to Scrob et al. (2019).
Membrane Integrity
EL was determined according to the method of Lutts et al. (1996). Fresh leaf sample (0.1 g, n = 6) was put into test tubes containing 10 ml of deionized water at 25 °C. After 24 h, EL was determined using a conductivity meter as EL1. Then samples were maintained in boiling water bath (100 °C) for 20 min. After cooling to 25 °C, a last EL reading (EL2) was obtained. The EL was defined as: EL1/EL2×100%.
Fresh leaflet sample (0.15 g, n = 6) was ground with trichloroacetic acid (10%). Then the homogenate was centrifuged at 4,000×g for 10 min. The supernatant (1 ml) was mixed with 1 ml of thiobarbituric acid (0.6%) and then maintained in boiling water bath for 15 min. After cooling, the mixture was centrifuged at 4,000×g for 10 min. The absorbance of supernatant was detected at 450, 532, 600 nm using an Ultraviolet-Visible spectrophotometer (T6 New Century, CN). The MDA content was calculated according to Li et al. (2018).
Reactive Oxygen Species (ROS)
Fresh leaflet sample (0.5 g, n = 6) was put in an ice-cold mortar and ground with 50 mM phosphate extraction buffer (PBS, 3 ml, pH 7.8). Thereafter the homogenate was centrifuged at 12,000×g for 15 min at 4 °C and the supernatant was used to measure the content of superoxide anion (O2• −). The content of O2• − was determined according to the method of Tian et al. (2003). The concentration of H2O2 was determined by the method of Patterson et al. (1984). To determine the H2O2 content, the leaflet sample (1.0 g, n = 6) was put in a mortar and ground in cold acetone (5 ml). The homogenate was centrifuged at 5,000×g for 5 min at 4 °C and the supernatant was used to determine the content of H2O2.
Antioxidant System
Preparation of SOD and CAT sample solution is the same as O2• −. SOD activity (U g-1 fresh weight, FW) was quantified according to the method of Syeed et al. (2011). One unit of SOD activity (U) was defined as the amount of enzyme that inhibited the photoreduction of 50% nitro blue tetrazolium (NBT). Activity of CAT was quantified based on the method described by Díaz-Vivancos et al. (2008). One unit of CAT activity represented the amount of enzyme catalyzing the decomposition of 1 µmol (H2O2) min-1 g-1 FW. Ascorbic acid (AsA) content was measured according to Law et al. (1983). The method is based on the reduction of Fe3+ to Fe2+ by AsA in acidic solution. Then Fe2+ forms complexes with bipyridyl, which appears pink at an absorption of 525 nm.
Cellular Macromolecules
Soluble sugar (Ss), soluble protein (Sp), and proline (Pro) levels were estimated. Anthrone-sulfuric acid method (Leng et al., 2016) was employed to measure Ss. Finally, the absorbance of solution was detected at 620 nm. The Sp content was measured based on the binding of Coomassie Brilliant Blue G-250 to protein according to the Bradford method (Bradford, 1976). Fresh leaf (0.5 g, n = 6) was homogenized using 3% sulfosalicylic acid solution (5 ml) and then maintained in boiling water bath (100 °C) for 10 min (stirred often). After cooling, Pro extract was obtained by filtering the mixture. According to the method (Teklić et al., 2010), the supernatant was used to determine the Pro content.
Chl a Fluorescence
In the early morning of sampling date, Chl a fluorescence of cut frond was measured using an IMAGING-PAM Chlorophyll Fluorometer (Heinz Walz, Effeltrich, Germany). Six intact fronds per treatment were used. The selected cut fronds were adapted in the dark for 20 min. Thereafter fluorescence induction curves were automatically recorded using the slow kinetic program in the saturation pulse analysis mode. The values of the minimal fluorescence yield in the dark-adapted state (Fo) and the maximal fluorescence yield of the dark-adapted state (Fm) were obtained with a modulated light [650 nm, 0.5 µmol (photon) m-2 s-1] and a 0.8 s saturating pulse [650 nm, 3,700 µmol (photon) m-2 s-1], respectively. After 40s, an actinic light irradiation [650 nm, 196 µmol (photon) m-2 s-1] was turned on. Then, a saturating pulse was imposed every 20 s until 5 min to determine the maximal fluorescence in the irradiation-adapted state (Fm’). Fv/Fm was calculated as: Fv/Fm=(Fm−Fo)/Fm (Kitajima and Butler, 1975). Stern-Volmer non-photochemical quenching coefficient (NPQ), coefficient of photochemical quenching of variable fluorescence based on the puddle model of PSII (qP), effective quantum yield of photochemical energy conversion in PSII (ΦPSII), quantum yield of regulated energy dissipation in PSII (ΦNPQ), quantum yield of non-regulated energy dissipation in PSII (ΦNO), and electron transport rate (ETR) were calculated by the ImagingPamGigE software (Heinz Walz, Effeltrich, Germany). Values of four circular areas (from the top to the bottom positions) on each cut frond were recorded, then averaged as a replicate for statistical analysis.
Images of the fluorescence parameters were stored in the ImagingPamGigE software and displayed by means of a false color code ranging from black (0.0) to purple (ending at 1.0) via red, yellow, green, and blue.
Statistical Analysis
All data were analyzed using SPSS (v.12, SPSS, Chicago, IL, USA). One-way analysis of variance (ANOVA) was used to identify the effect of Put solution. Post-hoc Duncan’s test was used to compare means of each parameter among different treatments. The relative effect of Put spraying on each parameter was calculated as [(Valuetreatment–Valuecontrol)/Valuecontrol].
Results
Vase Life of Cut Foliage
According to the experimental observation, when sprayed with deionized water for 10 days, 50% of the cut fronds in the deionized water group showed leaflet drop, whereas the vase life was extended by 5 days in Put0.2 group and by 11 days in Put2.0 group.
Leaf Appearance and Visible Symptoms
The foliage before treatment (Control) exhibited no visible injury (Figure 1). After 10 days of treatment, six cut fronds were starting to shed the leaflets and the other six had chlorotic blades under Put0 (15.92% of the total fronds area). Compared with Put0 treatment, the average percentages of visible yellowing area over the leaf surface under Put0.2 and Put2.0 were significantly lower, 2.40 and 1.18%, respectively (Table 1).
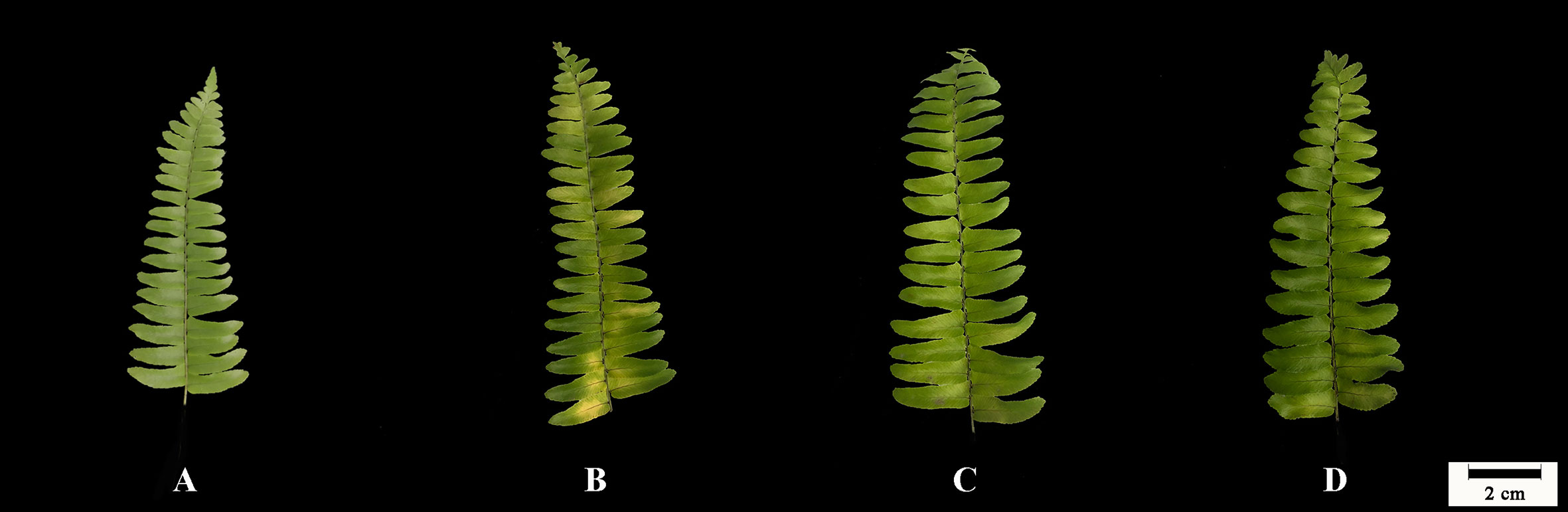
Figure 1 Leaf appearance of cut foliage of N. cordifolia before (Control, A) and after 10 days spraying with deionized water (Put0, B), 0.2 mM Put (Put0.2, C), and 2.0 mM Put (Put2.0, D).
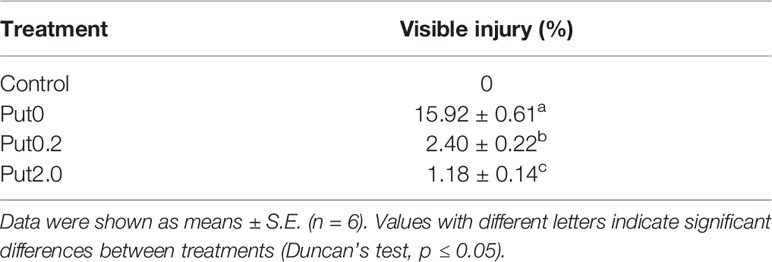
Table 1 Visible injury on the cut foliage of Nephrolepis cordifolia before (Control) and after 10 days spraying with deionized water (Put0), 0.2 mM Put (Put0.2), and 2.0 mM Put (Put2.0).
Chl Content
After 10 days of treatment, Chl a (−50.98%), Chl b (−72.69%), and total Chl (−60.15%) content of cut leaves under Put0 decreased significantly compared with control (Figure 2). Put2.0 spraying greatly alleviated the losses of Chl a (−7.68%), Chl b (−14.86%), and total Chl (−10.72%). Cut fronds sprayed with Put0.2 also had higher Chl a (−15.89%), Chl b (−43.05%), and total Chl content (−27.36%) than those sprayed with deionized water (Put0), further supporting its anti-senescence effect.
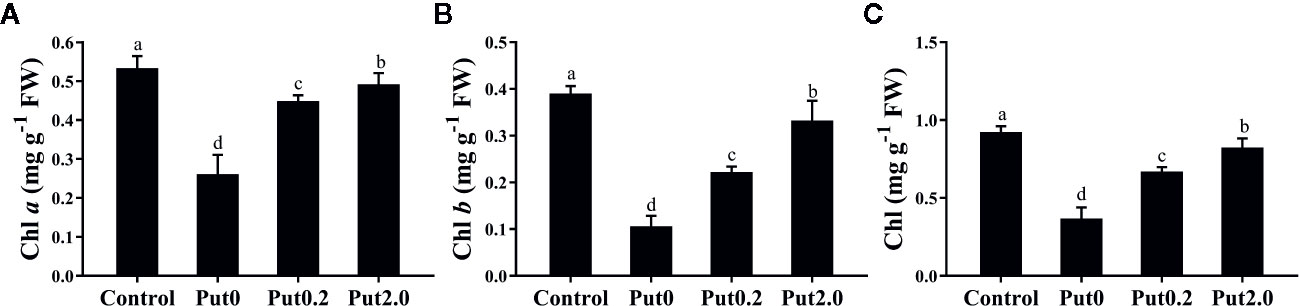
Figure 2 Mean (+ S.E., n = 6) contents of chlorophyll (Chl) a (A), Chl b (B), and total Chl (C), of cut foliage of N. cordifolia before (Control) and after 10 days spraying with deionized water (Put0), 0.2 mM Put (Put0.2), and 2.0 mM Put (Put2.0). FW is the fresh weight of the sample. Different letters show significant differences among bars (Duncan’s test, p ≤ 0.05).
Membrane Integrity
Compared to control, fronds sprayed with deionized water had significantly higher EL (+194.29%, Figure 3A). The relative increase of EL in leaves sprayed with Put0.2 and Put2.0 were lower (+69.90 and +31.87%, respectively), showing a better membrane integrity. Fronds sprayed with deionized water had a higher MDA (+34.06%) in comparison to control (Figure 3B), whereas in the leaves sprayed with Put (Put0.2 and Put2.0), MDA levels were not significantly different from control, indicating that Put could protect the plasma membrane from the ROS attack.
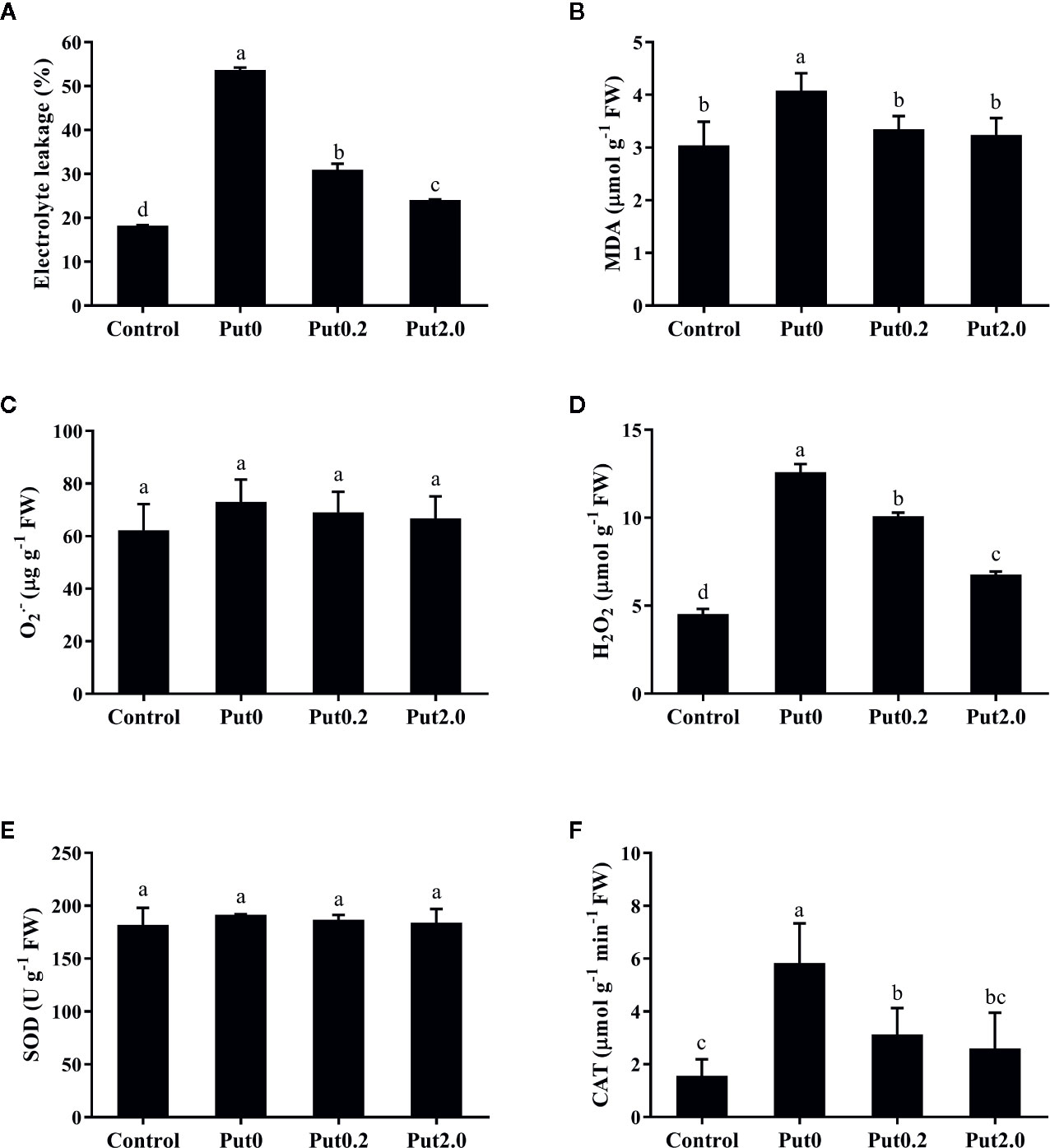
Figure 3 Electrolyte leakage (A), malondialdehyde content (MDA, B), superoxide anion content (O2• −, C), hydrogen peroxide content (H2O2, D), and activities of superoxide dismutase (SOD, E) and catalase (CAT, F) of cut foliage of N. cordifolia before (Control) and after 10 days spraying with deionized water (Put0), 0.2 mM Put (Put0.2), and 2.0 mM Put (Put2.0). FW is the fresh weight of the sample. Data were shown as means + S.E. (n = 6). Different letters show significant differences among bars (Duncan’s test, p ≤ 0.05).
Reactive Oxygen Species (ROS)
There was no significant difference of O2• − content between treatments (Figure 3C). H2O2 content significantly increased in the fronds treated with deionized water (+178.01%) compared to control. Put0.2 and Put2.0 inhibited the increase of H2O2 (+122.89 and +49.40%, respectively) (Figure 3D).
Antioxidant System
There was no significant change in SOD activity between treatments (Figure 3E). Compared to control, fronds sprayed with deionized water had significantly higher CAT activity (+273.33%, Figure 3F) and Put spraying inhibited the increase. The relative increase of CAT activity in the Put2.0-sprayed (+66.67%) fronds were the lowest, followed by the Put0.2-sprayed fronds (+100%). After 10 days of treatment, AsA contents of cut fronds under Put0, Put0.2, and Put2.0 decreased significantly compared with control (−54.71, −76.24, and −72.87%, respectively) (Figure 4D). However, the AsA content was not significantly different between Put0.2 and Put2.0.
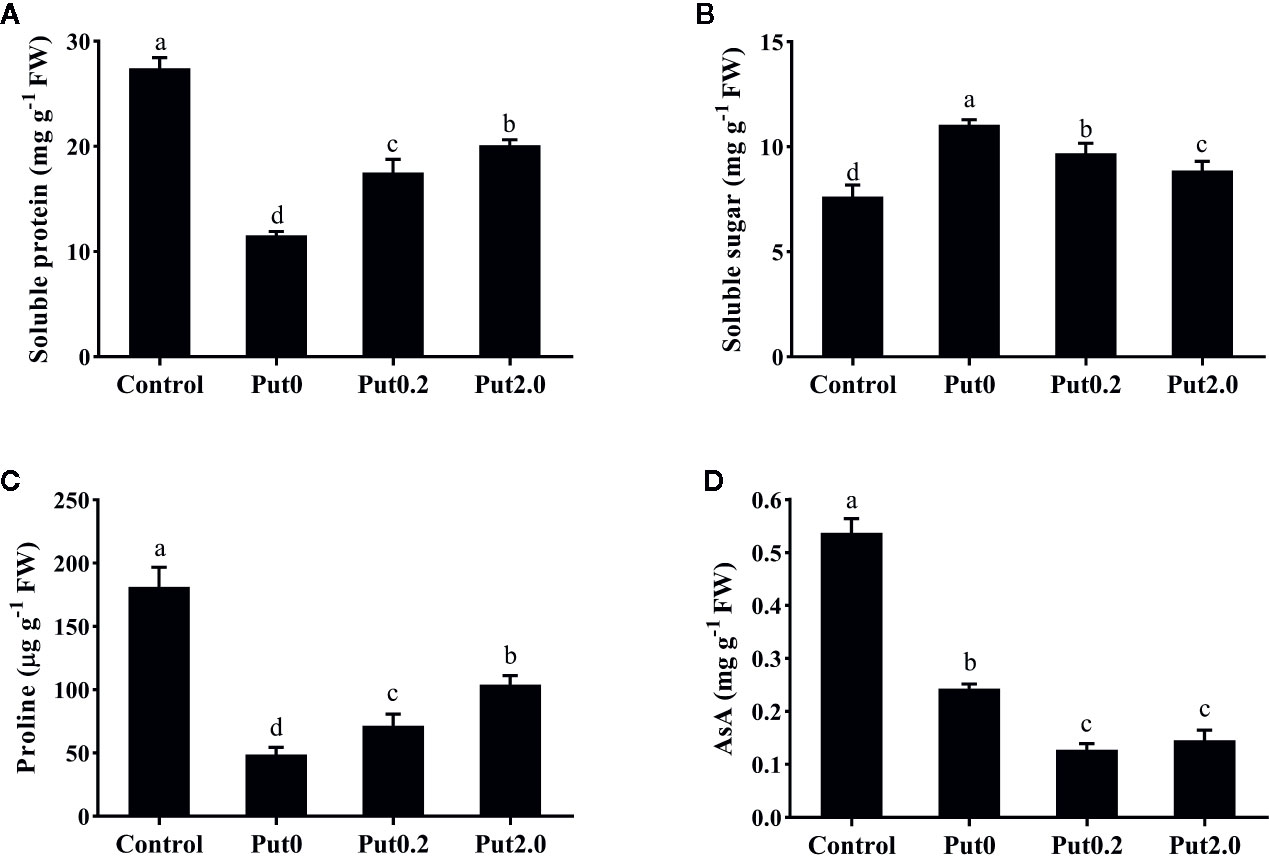
Figure 4 Soluble protein (A), soluble sugar (B), proline (C), and ascorbic acid (AsA, D) contents of cut foliage of N. cordifolia before (Control) and after 10 days spraying with deionized water (Put0), 0.2 mM Put (Put0.2), and 2.0 mM Put (Put2.0). FW is the fresh weight of the sample. Data were shown as means + S.E. (n = 6). Different letters show significant differences among bars (Duncan’s test, p ≤ 0.05).
Cellular Macromolecules
After 10 days of treatment, Sp (−57.93%) and Pro (−73.09%) content of cut fronds under Put0 decreased significantly compared with control (Figure 4). Put2.0 spraying greatly alleviated the losses of Sp (−26.29%) and Pro (−42.64%). Cut fronds sprayed with Put0.2 also had lower losses of Sp (−36.24%) and Pro content (−60.55%). Cut fronds sprayed with deionized water had higher Ss content (+44.83%, Figure 4B) than control and Put spraying inhibited the increase. The relative increase of Ss content in the Put2.0-sprayed fronds was the lowest (+16.22%), followed by the Put0.2 (+26.92%).
Chl a Fluorescence
Both Fm (−50.02%) and variable fluorescence (Fv, −59.12%) decreased in the fronds under Put0 after 10 days of spraying with deionized water in comparison to control, whereas these values were higher in the fronds sprayed with Put0.2 (−46.05 and −53.34%, respectively) and Put2.0 (−35.19 and −43.55%, respectively) relative to Put0 (Figure 5). Fv/Fm (−18.80%), ΦPSII (−31.21%), ΦNPQ (−12.98%), NPQ (−47.88%), qP (−31.09%), and ETR (−30.28%) of the cut fronds sprayed with deionized water decreased relative to control, while ΦNO increased (+54.84%) (Figure 6). The relative losses of Fv/Fm (−12.91%), NPQ (−3.13%), and ETR (−10.06%) in the fronds treated with Put2.0 were lower than those with other treatments. The relative increases of ΦNO (+11.76%) in fronds treated with Put2.0 were lower than those upon other treatments.
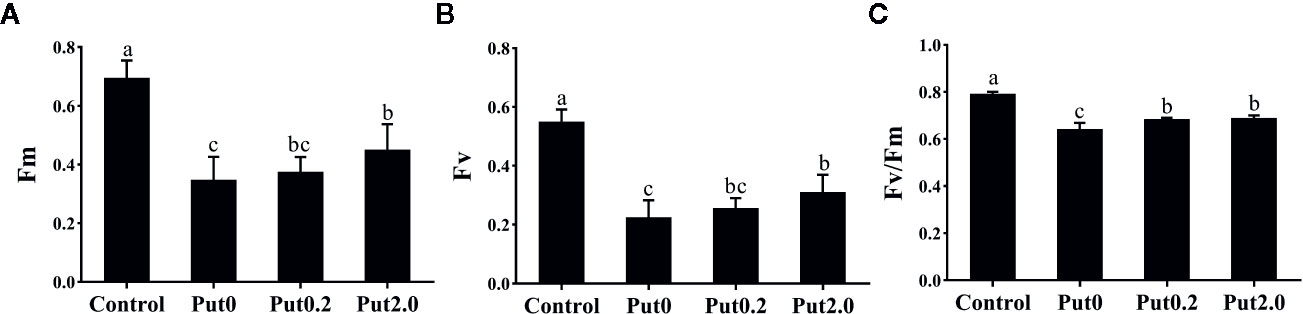
Figure 5 The maximal fluorescence yield of the dark-adapted state (Fm, A), variable fluorescence (Fv, B), the maximal quantum yield of PSII photochemistry measured in the dark-adapted state (Fv/Fm, C) of cut foliage of N. cordifolia before (Control) and after 10 days spraying with deionized water (Put0), 0.2 mM Put (Put0.2), and 2.0 mM Put (Put2.0). Data were shown as means + S.E. (n = 6). Different letters show significant differences between bars (Duncan’s test, P ≤ 0.05).
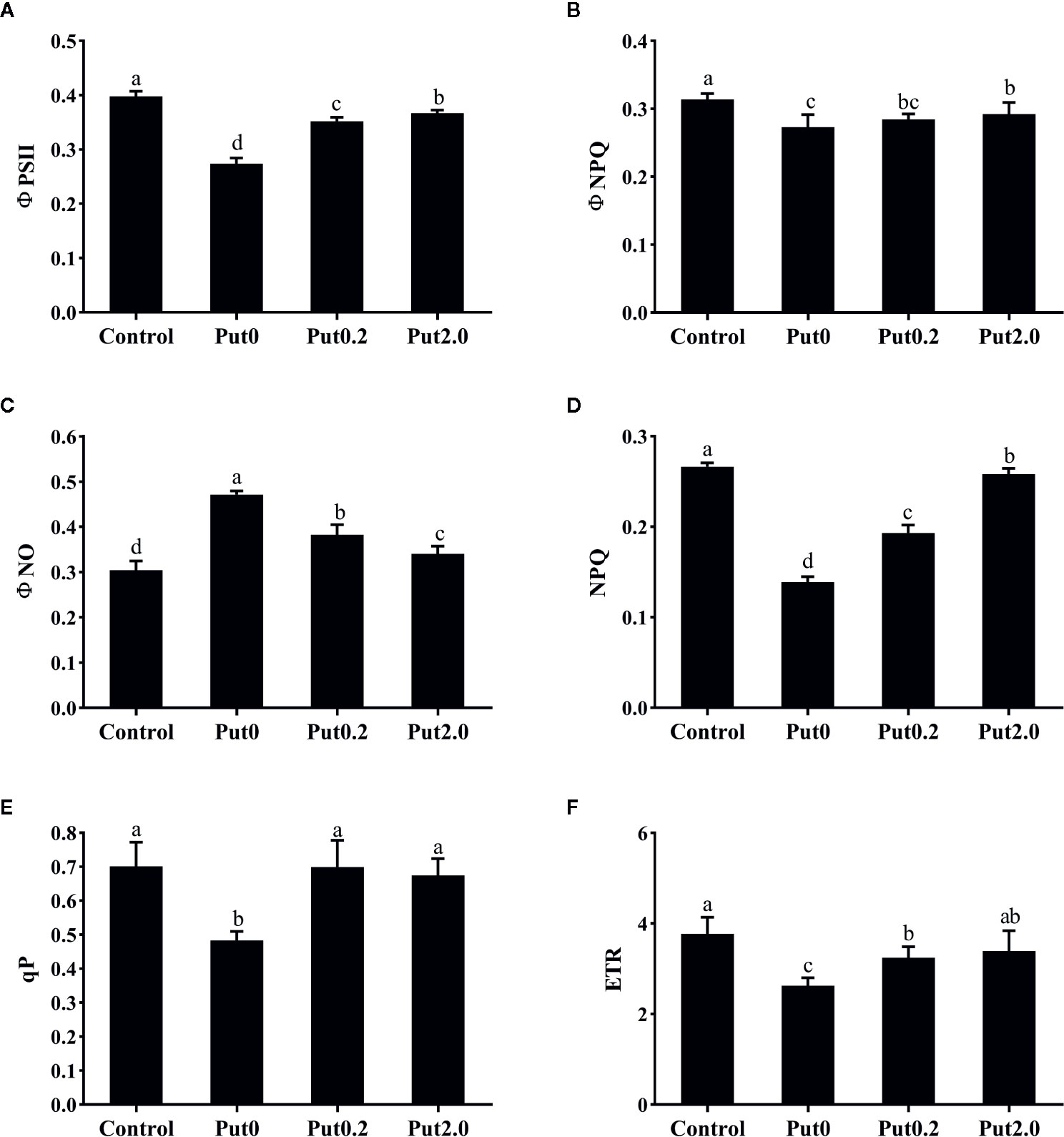
Figure 6 The effective quantum yield of photochemical energy conversion in PSII (ΦPSII, A), quantum yield of regulated energy dissipation in PSII (ΦNPQ, B), quantum yield of non-regulated energy dissipation in PSII (ΦNO, C), non-photochemical quenching coefficient (NPQ, D), coefficient of photochemical quenching of variable fluorescence based on the puddle model of PSII (qP, E), and electron transport rate (ETR, F) of cut foliage of N. cordifolia before (Control) and after 10 days spraying with deionized water (Put0), 0.2 mM Put (Put0.2), and 2.0 mM Put (Put2.0). Data were shown as means + S.E. (n = 6). Different letters show significant differences between bars (Duncan’s test, P ≤ 0.05).
Chl fluorescence imaging presents an instantaneous overview of the fluorescence emission pattern of the whole leaf surface (Gorbe and Calatayud, 2012). The images of Fv/Fm, ΦPSII, ΦNO, and ΦNPQ showed that the fronds were stressed under Put0 after 10 days of deionized water spraying (Figure 7). Compared with Put0 treatment, foliar application of Put exerted significant mitigating effect. Distinct effects of Put0.2 and Put2.0 spraying on Fv/Fm, ΦNO, and ΦNPQ were found. Put2.0 seems to be more efficient in mitigating the negative effects of photoinhibition or photodamage than Put0.2. In the present study, heterogeneous distributions of these four parameters over the screened leaf area were observed especially in leaves sprayed with deionized water and Put0.2.
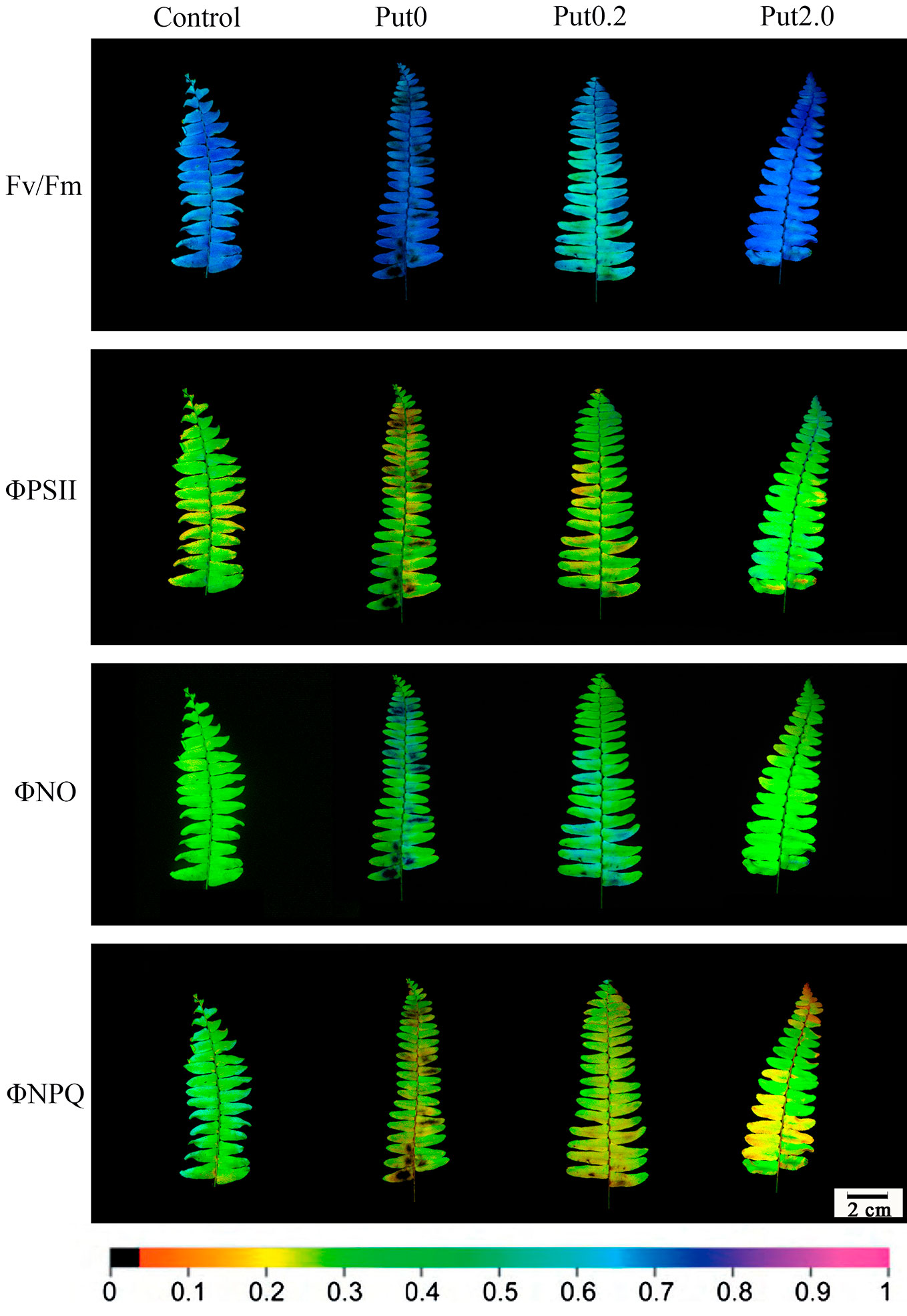
Figure 7 Detection of the effects of exogenous putrescine (Put) on cut foliage of N. cordifolia using a chlorophyll fluorescence imaging technique. Images of Fv/Fm, ΦPSII, ΦNO, and ΦNPQ, with actinic illumination of 196 µmol m-2 s-1 are shown. Each image in the same column represents the same frond. From left to right: Control, Put0, Put0.2, and Put2.0. The color scale at the bottom indicates values from 0 (black) to 1 (pink).
Discussion
Half of the replicates in the cut foliage of sword fern treated with deionized water were beginning to shed pinnae after 10 days of treatment. Meanwhile leaf yellowing appeared, which is a normal symptom of leaf senescence. It has been reported that abscission of the leaflets of sword fern started from the fourth days in vase (Banthoengsuk et al., 2011). The discrepancy between this previous study and our result is probably due to the different ways for treatment. Spraying deionized water every day could postpone the water deficit and the following pinnae abscission and Chl degradation. In our experiment, Put (0.2 and 2.0 mM) alleviated Chl (Chl a, Chl b, and total Chl) loss significantly and higher concentration had a stronger effect (Figure 2). Our results are in agreement with some previous studies, in which application of Put on leaves of Thymus vulgaris (Mohammadi et al., 2018) and flower head of broccoli (Zheng et al., 2019) increased Chl a, Chl b, and total Chl content compared to those under natural senescence. It might be due to PAs could alleviate the loss of Chl in thylakoid membranes by stabilizing photosystem complexes during storage (Beigbeder et al., 1995).
During leaf senescence ROS level increases (Breusegem and Dat, 2006). In the present study, the O2• − contents were similar between treatments, but the H2O2 was much higher under Put0 in comparison to control. Fronds sprayed with Put had lower increase of H2O2, suggesting it could play direct or indirect roles in scavenging H2O2. Both SOD and CAT are key antioxidant enzymes that have high ability of scavenging ROS under stress (Pan et al., 2006). SOD could convert O2• − into H2O2 in cells and CAT converts H2O2 into H2O and O2 (Hossain et al., 2006; Liu et al., 2015). In the current study, SOD activities of the cut foliage under spraying treatments were not significantly different in comparison to control, probably due to the similar O2• − contents between treatments. The activity of CAT was significantly elevated after 10 days in foliage sprayed with deionized water (Put0), suggesting the increase in CAT activity may be used to remove H2O2. It has been reported that an increase in PA content up-regulated the expression of genes encoding antioxidant enzymes and enhanced the activities of SOD, APX, and CAT under stress (Nayyar and Chander, 2004; Tang and Newton, 2005; Wi et al., 2006; Kuznetsov and Shevyakova, 2010). However, our results show that the SOD and CAT activities in Put-sprayed foliage were not significantly higher, even lower than those in Put0-sprayed foliage. Furthermore, the AsA content decreased with the leaf senescence, even lower in Put-sprayed foliage than that in Put0 treatment. These results indicate that Put detoxified the H2O2 directly or indirectly by triggering other pathways such as the γ-aminobutyric acid (GABA) shunt pathway (Groppa and Benavides, 2008; Palma et al., 2015; Palma et al., 2019).
Cellular membrane is very important for maintaining the osmotic balance of cells. ROS can attack the membrane and disrupt its integrity (Lim et al., 2007; Mohammadi et al., 2018). EL and MDA content are indicators of plasma membrane permeability and stability (Liu et al., 2015; Palma et al., 2016; Torabian et al., 2018). In the present study, foliage sprayed with deionized water had higher EL and MDA content while foliage sprayed with Put had slightly higher values or no significant difference compared to control, suggesting lower lipid peroxidation and higher membrane stability in Put-treated leaves (Wilhelmová et al., 2006). This result could be partly explained by the change of H2O2. In addition, this result could also be due to Put’s polycationic nature and it can directly bind to phospholipids to stabilize the membrane (Tiburcio et al., 1994; Bouchereau et al., 1999). During leaf senescence, the degradation of macromolecules and disassembly of cellular contents are often observed. In this study, Sp and Pro contents were decreased whereas Ss content was increased. This result indicates that protein degradation and Pro catabolism occurred after 10 days in vase (Zhang and Becker, 2015). However, Put spraying deterred this process. It is probably because Put is polycationic and could bind and stabilize these macromolecules (Tassoni et al., 1996). Another reason might be due to Put could enter the cell nuclear and produce thermospermine, then upregulate the prosurvival genes and activate ribosomal protein, finally produce more protein (Breeze et al., 2011; Moschou and Roubelakis-Angelakis, 2014). It has been proposed that the high level of Ss is the cause of senescence because of its repression of photosynthesis (van Doorn, 2008). Put spraying alleviated the increase of Ss content, suggesting that it could weaken the sugar signaling for anti-aging which is similar to the role of melatonin (Wang et al., 2013).
Generally, Fv/Fm, ΦPSII, and qP decrease under stresses and regulated-energy dissipation indicated by NPQ and ΦNPQ increase at first but decrease when the stress is more severe, while ΦNO increases (Gorbe and Calatayud, 2012). The decreased value of Fv/Fm often indicates photoinhibition (Thwe et al., 2014). In the present study, the fronds sprayed with deionized water (Put0) had a lower Fv/Fm compared with control, indicating the emergence of photoinhibition. The increase of ΦNO further confirmed that photodamage had occurred (Zhang et al., 2018). We found that the changes of Fv/Fm and ΦNO, indicators of photoinhibition and photodamage, respectively, were mitigated by the Put spraying. The values of ΦPSII, qP, and ETR were significantly reduced in fronds sprayed with deionized water (Put0) compared with control. The malfunction of the electron transport chain probably further induced the generation of H2O2 (Gupta et al., 2016). However, qP of the fronds sprayed with Put was not significantly different from qP in control. This result is in accord with a previous study showing that both ΦPSII and qP of cucumber plants under NaCl stress were reduced, while the reductions were alleviated to the control level by treatment with Put (Zhang et al., 2009). The mitigating effect of Put could be due to its polycationic nature, which induces the electrostatic screening, causes the stacking of thylakoid membrane and increases a spatial segregation of PSII and PSI (Barber, 1982; Ioannidis et al., 2006). In addition, Put can act as a permeant buffer to stimulate the synthesis of ATP, which can be used for PSII recovery after stress (Ioannidis et al., 2006; Ioannidis and Kotzabasis, 2007; Wu et al., 2019). The high value of NPQ indicates that plant has a high photo-protective ability and can protect itself from the damage via dissipation of excessive energy (Shu et al., 2013). In our study, we observed that NPQ in Put0 was significantly lower than that in control, suggesting the foliage was aging very serious after 10 days of treatment. Exogenous Put spraying significantly increased NPQ in the fronds compared with Put0. These results indicate that Put protects PSII against over-excitation by regulating heat dissipation probably through the aggregation of light harvest complex II (LHCII) (Pascal et al., 2005).
Chl fluorescence imaging is a non-destructive and easy operating technique that can rapidly detect spatial heterogeneities in fluorescence emission pattern and photosynthetic capacity across the leaf surface, even before visual injury appears (Omasa and Takayama, 2003; Calatayud et al., 2006; Gorbe and Calatayud, 2012). This technique is very useful for identifying early stress-induced damage. In the present study, the visible injury on frond surface was not severe and could not be detected easily by naked eye, except for fronds treated with Put0 (Figure 1), but the images of chlorophyll fluorescence parameters showed that the photochemical activity had already been inhibited and photodamage had occurred under both Put0 and Put0.2 treatments (Figure 7). In addition, there was obvious spatial heterogeneity in the fluorescence emission patterns over the frond surface under these two treatments. Mesophyll cells near the midrib in some leaflets under Put0 and Put0.2 treatments had lower Fv/Fm, ΦPSII, and ΦNPQ, but had higher ΦNO than mesophyll cells in other parts of the leaf. There is a report suggesting that leaf section next to the midrib has a more severe change of chlorophyll fluorescence parameters (Gorbe, 2009). They also find that the capacity of photoprotection of PSII, indicated by the ratio ΦNPQ/ΦNO decreases progressively as flower shoots wilt and vase life ends in cut rose flowers (Gorbe, 2009). Our results showed that the leaflets under Put0 and Put0.2 treatments lost the photoprotection ability due to the decreased ΦNPQ and increased ΦNO, while those sprayed with 2.0 mM Put still had this capability of dissipating excess energy. From the image of ΦNPQ, a significant difference among treatments was observed while the average values of this parameter among treatments were not different significantly. This result suggests that it is better to combine the qualitative and quantitative chlorophyll fluorescence analysis together to describe leaf senescence.
In conclusion, we investigated the effects of Put on senescence of cut foliage in sword fern. After fronds were detached from the plants, the Chl, Sp, and Pro contents were decreased, EL, MDA, and H2O2 contents were increased, plasma membrane was impaired, photochemical activity was inhibited, and photoinhibition and photodamage happened. Both 0.2 and 2.0 mM Put spraying extended the vase life of the cut foliage and alleviated the changes of parameters caused by senescence. In addition, 2.0 mM Put had a better mitigating ability than that of 0.2 mM. Leaf spraying of 2.0 mM Put for 10 days significantly ameliorated the losses of Chl, Sp, and Pro content (−10.72, −26.29, and −42.64%, respectively), followed by 0.2 mM Put (−27.36, −36.24, and −60.55%, respectively). Put spraying also improved photochemical capability and prevented membrane impairment as well as visible injury in comparison with cut fronds sprayed with deionized water. Leaf spraying of 2.0 mM Put greatly reduced the decline of the effective quantum yield of photochemical energy conversion in PSII (ΦPSII), the maximal quantum yield of PSII photochemistry measured in the dark-adapted state (Fv/Fm) and electron transport rate (ETR) (−7.89, −12.91, and −10.06%, respectively), and also inhibited the increases of EL, MDA, Ss, and H2O2 (+31.87, +6.43, +16.22, and +49.40%, respectively). Overall, Put played important roles in deterring the degradation of Chl, Ss, and Pro, detoxifying the H2O2, weakening the sugar signaling, mitigating the decline of photochemical activity, and postponing the leaf senescence. The present study gives new insights into effects of Put on leaf senescence and provides a strategy for preserving post-harvest cut foliage.
Data Availability Statement
All datasets presented in this study are included in the article.
Author Contributions
LZ and TW designed research. YQ, LJ, and SM conducted experiments. YQ, FX, and YC analyzed data. LZ, YQ, and TW wrote the manuscript. All authors contributed to the article and approved the submitted version.
Funding
This research was supported financially by National Natural Science Foundation of China (No. 31711530648), the open funding of State Key Laboratory of Tree Genetics and Breeding (TGB2019004), Northeast Agricultural University of China (Academic backbone Project, No.18XG07), and Achimedes Foundation of Estonia.
Conflict of Interest
The authors declare that the research was conducted in the absence of any commercial or financial relationships that could be construed as a potential conflict of interest.
Acknowledgments
The authors thank Dr. Hanna Hõrak for her kind editing of this manuscript.
References
Altman, A. (1982). Retardation of radish leaf senescence by polyamines. Physiol. Plant. 54 (2), 189–193. doi: 10.1111/j.1399-3054.1982.tb06324.x
Ataii, D., Naderi, R., Khandan-Mirkohi, A. (2015). Exogenous putrescine delays senescence of Lisianthus cut flowers. J. Ornam. Plants 5 (3), 167–174.
Banthoengsuk, S., Ketsa, S., van Doorn, W. G. (2011). 1-MCP partially alleviates dehydration-induced abscission in cut leaves of the fern Nephrolepis cordifolia. Postharvest Biol. Technolology 59 (3), 253–257. doi: 10.1016/j.postharvbio.2010.10.001
Barber, J. (1982). Influence of surface charges on thylakoid structure and function. Annu. Rev. Plant Physiol. 33, 261–295. doi: 10.1146/annurev.pp.33.060182.001401
Beigbeder, A., Vavadakis, M., Navakoudis, E., Kotzabasis, K. (1995). Influence of polyamine inhibitors on light-independent and light-dependent chlorophyll biosynthesis and on the photosynthetic rate. J. Photochem. Photobiol. 28 (3), 235–242. doi: 10.1016/1011-1344(95)07113-G
Bouchereau, A., Aziz, A., Larher, F., Martin-Tanguy, J. (1999). Polyamines and environmental challenges: recent development. Plant Sci. 140 (2), 103–125. doi: 10.1016/S0168-9452(98)00218-0
Bradford, M. M. (1976). A rapid and sensitive method for the quantitation of microgram quantities of protein utilizing the principle of protein-dye binding. Anal. Biochem. 72, 248–254. doi: 10.1016/0003-2697(76)90527-3
Breeze, E., Harrison, E., McHattie, S., Hughes, L., Hickman, R., Hill, C., et al. (2011). High-resolution temporal profiling of transcripts during Arabidopsis leaf senescence reveals a distinct chronology of processes and regulation. Plant Cell 23 (3), 873–894. doi: 10.1105/tpc.111.083345
Breusegem, F. V., Dat, J. F. (2006). Reactive oxygen species in plant cell death. Plant Physiol. 141 (2), 384–390. doi: 10.1104/pp.106.078295
Calatayud, A., Roca, D., Martínez, P. F. (2006). Spatial-temporal variations in rose leaves under water stress conditions studied by chlorophyll fluorescence imaging. Plant Physiol. Biochem. 44 (10), 564–573. doi: 10.1016/j.plaphy.2006.09.015
Che-Husin, N. M., Joyce, D. C., Irving, D. E. (2018). Gel xylem occlusions decrease hydraulic conductance of cut Acacia holosericea foliage stems. Postharvest Biol. Technol. 135, 27–37. doi: 10.1016/j.postharvbio.2017.08.015
Chen, D. Q., Wang, S. W., Xiong, B. L., Cao, B. B., Deng, X. P. (2015). Carbon/nitrogen imbalance associated with drought-induced leaf senescence in Sorghum bicolor. PloS One 10 (8), e0137026. doi: 10.1371/journal.pone.0137026
Cho, H. T., Hong, Y. N. (1988). Effects of putrescine on senescence in detached leaves of Chinese cabbage in the light. Korean J. Bot. 31 (3), 227–237.
Díaz-Vivancos, P., Clemente-Moreno, M. J., Rubio, M., Olmos, E., García, J. A., Martínez-Gómez, P., et al. (2008). Alteration in the chloroplastic metabolism leads to ROS accumulation in pea plants in response to plum pox virus. J. Exp. Bot. 59, 2147–2160. doi: 10.1093/jxb/ern082
Gorbe, E., Calatayud, A. (2012). Applications of chlorophyll fluorescence imaging technique in horticultural reaearch: a review. Sci. Hortic. 138, 24–35. doi: 10.1016/j.scienta.2012.02.002
Gorbe, E. (2009). Study of nutrient solution management in soilless rose cultivation through the analysis of physiological parameters and nutrient absorption. PhD thesis (Polytechnical University of Valencia). doi: 10.4995/Thesis/10251/6921
Groppa, M. D., Benavides, M. P. (2008). Polyamines and abiotic stress: recent advances. Amino Acids 34 (1), 35–45. doi: 10.1007/s00726-007-0501-8
Gupta, K., Sengupta, A., Chakraborty, M., Gupta, B. (2016). Hydrogen peroxide and polyamines act as double edged swords in plant abiotic stress responses. Front. Plant Sci. 7, 1343. doi: 10.3389/fpls.2016.01343
Hörtensteiner, S. (2006). Chlorophyll degradation during senescence. Annu. Rev. Plant Biol. 57, 55–77. doi: 10.1146/annurev.arplant.57.032905.105212
Hossain, Z., Mandal, A. K. A., Datta, S. K., Biswas, A. K. (2006). Decline in ascorbate peroxidase activity–A prerequisite factor for tepal senescence in gladiolus. J. Plant Physiol. 163 (2), 186–194. doi: 10.1016/j.jplph.2005.03.004
Ioannidis, N. E., Kotzabasis, K. (2007). Effects of polyamines on the functionality of photosynthetic membrane in vivo and in vitro. BBA 1767, 1372–1382. doi: 10.1016/j.bbabio.2007.10.002
Ioannidis, N. E., Sfichi, L., Kotzabasis, K. (2006). Putrescine stimulates chemiosmotic ATP synthesis. BBA-Bioenergetics 1757 (7), 821–828. doi: 10.1016/j.bbabio.2006.05.034
Kim, J. S., Yun, B. W., Choi, J. S., Kim, T. J., Kwak, S. S., Cho, K. Y. (2004). Death mechanisms caused by carotenoid biosynthesis inhibitors in green and in undeveloped plant tissues. Pestic. Biochem. Physiol. 78 (3), 127–139. doi: 10.1016/j.pestbp.2003.12.001
Kitajima, M., Butler, W. L. (1975). Quenching of chlorophyll fluorescence and primary photochemistry in chloroplasts by dibromothymoquinone. BBA-Bioenergetics 376 (1), 105–115. doi: 10.1016/0005-2728(75)90209-1
Kusano, T., Berberich, T., Tateda, C., Takahashi, Y. (2008). Polyamines: essential factors for growth and survival. Planta 228 (3), 367–381. doi: 10.1007/s00425-008-0772-7
Kuznetsov, V. V., Shevyakova, N. I. (2010). “Polyamines and plant adaptation to saline environments,” in Desert plants (Berlin, Heidelberg: Springer), 261–298. doi: 10.1007/978-3-642-02550-1_13
Law, M. Y., Charles, S. A., Halliwell, B. (1983). Glutathione and ascorbic acid in spinach (Spinacia oleracea) chloroplasts. The effect of hydrogen peroxide and of Paraquat. Biochem. J. 210, 899–903. doi: 10.1042/bj2100899
Leng, F., Sun, S., Jing, Y., Wang, F., Wei, Q., Wang, X., et al. (2016). A rapid and sensitive method for determination of trace amounts of glucose by anthrone-sulfuric acid method. Bulg. Chem. Commun. 48 (1), 109–113.
Li, X., Zhang, X. M., Wu, Y. S., Li, B. Q., Yang, Y. P. (2018). Physiological and biochemical analysis of mechanisms underlying cadmium tolerance and accumulation in turnip. Plant Divers. 40 (1), 19–27. doi: 10.1016/j.pld.2017.12.005
Lim, P. O., Kim, H. J., Gil Nam, H. (2007). Leaf senescence. Annu. Rev. Plant Biol. 58 (1), 115–136. doi: 10.1146/annurev.arplant.57.032905.105316
Lin, S., Li, H., Xian, X., Lin, X., Pang, Z., Liu, J., et al. (2019). Nano-silver pretreatment delays wilting of cut gardenia foliage by inhibiting bacterial xylem blockage. Sci. Hortic. 246, 791–796. doi: 10.1016/j.scienta.2018.11.050
Liu, X., Sui, L. H., Huang, Y. Z., Geng, C. M., Yin, B. H. (2015). Physiological and visible injury responses in different growth stages of winter wheat to ozone stress and the protection of spermidine. Atmos. Pollut. Res. 6 (4), 596–604. doi: 10.5094/APR.2015.067
Lutts, S., Kinet, J. M., Bouharmont, J. (1996). NaCl-induced senescence in leaves of rice (Oryza sativa L.) cultivars differing in salinity resistance. Ann. Bot. 78 (3), 389–398. doi: 10.1006/anbo.1996.0134
Malakar, M., Acharyya, P., Biswas, S. (2017). Effect of certain chemicals on post harvest life of some cut foliages. Int. J. Agric. Environ. Biotechnol. 10 (2), 199–207. doi: 10.5958/2230-732X.2017.00023.7
Merzlyak, M. N., Hendry, G. A. F. (1994). Free radical metabolism, pigment degradation and lipid peroxidation in leaves during senescence. P. R. Soc B.-Biol. Sci. 102, 459–471. doi: 10.1017/S0269727000014482
Mohammadi, H., Ghorbanpour, M., Brestic, M. (2018). Exogenous putrescine changes redox regulations and essential oil constituents in field-grown Thymus vulgaris L. under well-watered and drought stress conditions. Ind. Crop Prod. 122, 119–132. doi: 10.1016/j.indcrop.2018.05.064
Moschou, P. N., Roubelakis-Angelakis, K. A. (2014). Polyamines and programmed cell death. J. Exp. Bot. 65 (5), 1285–1296. doi: 10.1093/jxb/ert373
Nayyar, H., Chander, S. (2004). Protective effects of polyamines against oxidative stress induced by water and cold stress in chickpea. J. Agron. Crop Sci. 190, 355–365. doi: 10.1111/j.1439-037X.2004.00106.x
Nisar, S., Tahir, I., Ahmad, S. S. (2015). Modulation of flower senescence in Nicotiana plumbaginifolia L. by polyamines. Indian J. Plant Physiol. 20 (2), 186–190. doi: 10.1007/s40502-015-0154-7
Omasa, K., Takayama, K. (2003). Simultaneous measurement of stomatal conductance, non-photochemical quenching, and photochemical yield of photosystem II in intact leaves by thermal and chlorophyll fluorescence imaging. Plant Cell Physiol. 44 (12), 1290–1300. doi: 10.1093/pcp/pcg165
Palma, F., Carvajal, F., Ramos, J. M., Jamilena, M., Garrido, D. (2015). Effect of putrescine application on maintenance of zucchini fruit quality during cold storage: contribution of GABA shunt and other related nitrogen metabolites. Postharvest Biol. Technol. 99, 131–140. doi: 10.1016/j.postharvbio.2014.08.010
Palma, F., Carvajal, F., Jamilena, M., Garrido, D. (2016). Putrescine treatment increases the antioxidant response and carbohydrate content in zucchini fruit stored at low temperture. Postharvest Biol. Technol. 118, 68–70. doi: 10.1016/j.postharvbio.2016.03.009
Palma, F., Carvajal, F., Jiménez-Muñoz, R., Pulido, A., Jamilena, M., Garrido, D. (2019). Exogenous γ-aminobutyric acid treatment improves the cold tolerance of zucchini fruit during postharvest storage. Plant Physiol. Biochem. 136, 188–195. doi: 10.1016/j.plaphy.2019.01.023
Pan, Y., Wu, L. J., Yu, Z. L. (2006). Effect of salt and drought stress on antioxidant enzymes activities and SOD isoenzymes of liquorice (Glycyrrhiza uralensis Fisch). Plant Growth Regul. 49, 157–165. doi: 10.1007/s10725-006-9101-y
Paoletti, E., Ferrara, A. M., Calatayud, V., Cerveró, J., Giannetti, F., Sanz-Sanchez, M. J., et al. (2009). Deciduous shrubs for ozone bioindication Hibiscus syriacus as an example. Environ. Pollut. 157 (3), 865–870. doi: 10.1016/j.envpol.2008.11.009
Pascal, A. A., Liu, Z., Broess, K., van Oort, B., van Amerongen, H., Wang, C., et al. (2005). Molecular basis of photoprotection and control of photosynthetic light-harvesting. Nature 436, 134–137. doi: 10.1038/nature03795
Paschalidis, K. A., Roubelakis-Angelakis, K. A. (2005). Spatial and temporal distribution of polyamine levels and polyamine anabolism in different organs/tissues of the tobacco plant. Correlations with age, cell division/expansion, and differentiation. Plant Physiol. 138 (1), 142–152. doi: 10.1104/pp.104.055483
Patterson, B. D., Macrae, E. A., Ferguson, I. B. (1984). Estimation of hydrogen peroxide in plant extracts using titanium (IV). Anal. Biochem. 139 (2), 487–492. doi: 10.1016/0003-2697(84)90039-3
Rubinowska, K., Pogroszewska, E., Michalek, W. (2012). The effect of polyamines on physiological parameters of post-harvest quality of cut stems of Rosa ‘Red Berlin’. Acta Sci. Pol. Hortorum Cultus 11 (6), 81–93.
Safeena, S. A., Thangam, M., Singh, N. P. (2019). Conservation and evaluation of different cut foliage species comprising pteridophytes (ferns and fern allies) of west coast regions of India. J. Indian Soc Coastal Agric. Res. 37 (1), 7–13.
Scrob, T., Hosu, A., Cimpoiu, C. (2019). The Influence of in Vitro Gastrointestinal Digestion of Brassica oleracea Florets on the Antioxidant Activity and Chlorophyll, Carotenoid and Phenolic Content. Antioxidants 8 (7), 212. doi: 10.3390/antiox8070212
Serrano, M., Amorós, A., Pretel, M. T., Martı́nez-Madrid, M. C., Romojaro, F. (2001). Preservative solutions containing boric acid delay senescence of carnation flowers. Postharvest Biol. Technol. 23 (2), 133–142. doi: 10.1016/S0925-5214(01)00108-9
Shabanian, S., Esfahani, M. N., Karamian, R., Tran, L. S. P. (2018). Physiological and biochemical modifications by postharvest treatment with sodium nitroprusside extend vase life of cut flowers of two gerbera cultivars. Postharvest Biol. Technol. 137, 1–8. doi: 10.1016/j.postharvbio.2017.11.009
Sharma, S., Pareek, S., Sagar, N. A., Valero, D., Serrano, M. (2017). Modulatory effects of exogenously applied polyamines on postharvest physiology, antioxidant system and shelf life of fruits: a review. Int. J. Mol. Sci. 18 (8), 1789. doi: 10.3390/ijms18081789
Shu, S., Yuan, L. Y., Guo, S. R., Sun, J., Yuan, Y. H. (2013). Effects of exogenous spermine on chlorophyll fluorescence, antioxidant system and ultrastructure of chloroplasts in Cucumis sativus L. under salt stress. Plant Physiol. Biochem. 63, 209–216. doi: 10.1016/j.plaphy.2012.11.028
Skutnik, E., Rabiza-Świder, J., Wachowicz, M., Łukaszewska, A. J. (2004). Senescence of cut leaves of Zantedeschia aethiopica and Z. elliottiana. part I. chlorophyll degradation. Acta Sci. Pol. Hortorum Cultus 3 (2), 57–65.
Sobieszczuk-Nowicka, E., Kubala, S., Zmienko, A., Małecka, A., Legocka, J. (2016). From accumulation to degradation: reprogramming polyamine metabolism facilitates dark-induced senescence in barley leaf cells. Front. Plant Sci. 6, 1198. doi: 10.3389/fpls.2015.01198
Sood, S., Nagar, P. K. (2003). The effect of polyamines on leaf senescence in two diverse rose species. Plant Growth Regul. 39 (2), 155–160. doi: 10.1023/A:1022514712295
Syeed, S., Anjum, N. A., Nazar, R., Iqbal, N., Masood, A., Khan, N. A. (2011). Salicylic acid-mediated changes in photosynthesis, nutrients content and antioxidant metabolism in two mustard (Brassica juncea L.) cultivars differing in salt tolerance. Acta Physiol. Plant 33 (3), 877–886. doi: 10.1007/s11738-010-0614-7
Tang, W., Newton, R. J. (2005). Polyamines reduce salt-induced oxidative damage by increasing the activities of antioxidant enzymes and decreasing lipid peroxidation in Virginia pine. Plant Growth Regul. 46, 31–43. doi: 10.1007/s10725-005-6395-0
Tassoni, A., Antognoni, F., Bagni, N. (1996). Polyamine binding to plasma membrane vesicles isolated from zucchini hypocotyls. Plant Physiol. 110 (3), 817–824. doi: 10.1104/pp.110.3.817
Teerarak, M., Laosinwattana, C. (2019). Essential oil from ginger as a novel agent in delaying senescence of cut fronds of the fern (Davallia solida (G. Forst.) Sw.). Postharvest Biol. Technol. 156, 110927. doi: 10.1016/j.postharvbio.2019.06.001
Teklić, T., Špoljarević, M., Stanisavljević, A., Lisjak, M., Vinković, T., Paradiković, N., et al. (2010). Assessment of the influence of different sample processing and cold storage duration on plant free proline content analyses. Phytochem. Anal. 21, 561–565. doi: 10.1002/pca.1233
Thwe, A. A., Vercambre, G., Gautier, H., Gay, F., Phattaralerphong, J., Kasemsap, P. (2014). Response of photosynthesis and chlorophyll fluorescence to acute ozone stress in tomato (Solanum lycopersicum Mill.). Photosynthetica 52 (1), 105–116. doi: 10.1007/s11099-014-0012-2
Tian, M., Gu, Q., Zhu, M. Y. (2003). The involvement of hydrogen peroxide and antioxidant enzymes in the process of shoot organogenesis of strawberry callus. Plant Sci. 165, 701–707. doi: 10.1016/S0168-9452(03)00224-3
Tiburcio, A. F., Besford, R. T., Capell, T., Borrell, A., Testillano, P. S., Risueno, M. C. (1994). Mechanisms of polyamine action during senescence responses induced by osmotic stress. J. Exp. Bot. 45 (12), 1789–1800. doi: 10.1093/jxb/45.12.1789
Torabian, S., Shakiba, M. R., Dabbagh, M. N. A., Toorchi, M. (2018). Leaf gas exchange and grain yield of common bean exposed to spermidine under water stress. Photosynthetica 56, 1387–1397. doi: 10.1007/s11099-018-0834-4
van Doorn, W. G. (2008). Is the onset of senescence in leaf cells of intact plants due to low or high sugar levels? J. Exp. Bot. 59 (8), 1963–1972. doi: 10.1093/jxb/ern076
Wang, P., Sun, X., Chang, C., Feng, F., Liang, D., Cheng, L., et al. (2013). Delay in leaf senescence of Malus hupehensis by long-term melatonin application is associated with its regulation of metabolic status and protein degradation. J. Pineal Res. 55 (4), 424–434. doi: 10.1111/jpi.12091
Wi, S. J., Kim, W. T., Park, K. Y. (2006). Overexpression of carnation S-adenosylmethionine decarboxylase gene generates a broad-spectrum tolerance to abiotic stresses in transgenic tobacco plants. Plant Cell Rep. 25, 1111–1121. doi: 10.1007/s00299-006-0160-3
Wilhelmová, N., Domingues, P. M. D. N., Srbová, M., Fuksová, H., Wilhelm, J. (2006). Changes in non-polar aldehydes in bean cotyledons during aging. Biol. Plantarum 50 (4), 559–564. doi: 10.1007/s10535-006-0088-z
Wu, X., Shu, S., Wang, Y., Yuan, R., Guo, S. (2019). Exogenous putrescine alleviates photoinhibition caused by salt stress through cooperation with cyclic electron flow in cucumber. Photosynth. Res. 141 (3), 303–314. doi: 10.1007/s11120-019-00631-y
Yiu, J. C., Juang, L. D., Fang, D. Y. T., Liu, C. W., Wu, S. J. (2009). Exogenous putrescine reduces flooding-induced oxidative damage by increasing the antioxidant properties of Welsh onion. Sci. Horti. 120 (3), 306–314. doi: 10.1016/j.scienta.2008.11.020
Zamani, S., Kazemi, M., Aran, M. (2011). Postharvest life of cut rose flowers as affected by salicylic acid and glutamine. World Appl. Sci. J. 12, 1621–1624.
Zhang, L., Becker, D. (2015). Connecting proline metabolism and signaling pathways in plant senescence. Front. Plant Sci. 6, 552. doi: 10.3389/fpls.2015.00552
Zhang, R. H., Li, J., Guo, S. R., Tezuka, T. (2009). Effects of exogenous putrescine on gas-exchange characteristics and chlorophyll fluorescence of NaCl-stressed cucumber seedlings. Photosynth. Res. 100 (3), 155–162. doi: 10.1007/s11120-009-9441-30
Zhang, L., Jia, L. L., Sui, J. X., Wen, M. X., Chen, Y. J. (2018). Ameliorating effects of three kinds of antioxidants to ozone- polluted painted nettle (Coleus blumei Benth.). Photosynthetica 56 (2), 623–632. doi: 10.1007/s11099-017-0693-4
Zhao, D. Q., Wang, R., Meng, J. S., Li, Z. Y., Wu, Y. Q., Tao, J. (2017). Ameliorative effects of melatonin on dark-induced leaf senescence in gardenia (Gardenia jasminoides Ellis): leaf morphology, anatomy, physiology and transcriptome. Sci. Rep. 7, 10423. doi: 10.1038/s41598-017-10799-9
Keywords: antioxidant enzyme, biochemical, chlorophyll fluorescence imaging, polyamines, sword fern
Citation: Qu Y, Jiang L, Wuyun T, Mu S, Xie F, Chen Y and Zhang L (2020) Effects of Exogenous Putrescine on Delaying Senescence of Cut Foliage of Nephrolepis cordifolia. Front. Plant Sci. 11:566824. doi: 10.3389/fpls.2020.566824
Received: 01 June 2020; Accepted: 24 August 2020;
Published: 10 September 2020.
Edited by:
Antonio Ferrante, University of Milan, ItalyReviewed by:
Giuseppina P. P. Lima, São Paulo State University, BrazilWeibiao Liao, Gansu Agricultural University, China
Gustavo A. Martinez, Consejo Nacional de Investigaciones Científicas y Técnicas (CONICET), Argentina
Copyright © 2020 Qu, Jiang, Wuyun, Mu, Xie, Chen and Zhang. This is an open-access article distributed under the terms of the Creative Commons Attribution License (CC BY). The use, distribution or reproduction in other forums is permitted, provided the original author(s) and the copyright owner(s) are credited and that the original publication in this journal is cited, in accordance with accepted academic practice. No use, distribution or reproduction is permitted which does not comply with these terms.
*Correspondence: Lu Zhang, caszhanglu@hotmail.com; Tana Wuyun, wuyuntana@emu.ee