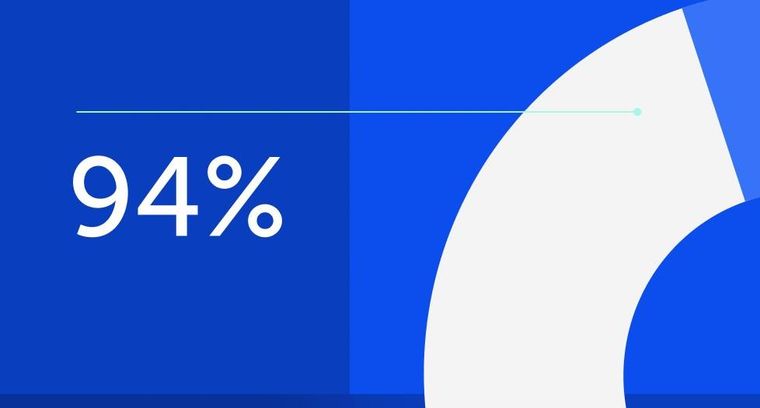
94% of researchers rate our articles as excellent or good
Learn more about the work of our research integrity team to safeguard the quality of each article we publish.
Find out more
ORIGINAL RESEARCH article
Front. Plant Sci., 19 August 2020
Sec. Plant Pathogen Interactions
Volume 11 - 2020 | https://doi.org/10.3389/fpls.2020.01250
This article is part of the Research TopicAdvances and Challenges of RNAi Based Technologies for PlantsView all 11 articles
Colorado potato beetle (CPB) is an agricultural pest of solanaceous crops, notorious for its rapid resistance development to chemical pesticides. Foliar spraying of dsRNA formulations is a promising innovative technology providing highly specific and environmentally acceptable option for CPB management. We designed dsRNA to silence CPB mesh gene (dsMESH) and performed laboratory feeding trials to assess impacts on beetle survival and development. We compared the effectiveness of in vivo and in vitro produced dsRNA in a series of laboratory experiments. We additionally performed a field trial in which the efficacy of dsRNA sprayed onto potato foliage was compared to a spinosad-based insecticide. We showed that dsMESH ingestion consistently and significantly impaired larval growth and decreased larval survival in laboratory feeding experiments. In vivo produced dsRNA performed similarly as in vitro synthesized dsRNA in laboratory settings. In the field trial, dsMESH was as effective in controlling CPB larvae as a commercial spinosad insecticide, its activity was however slower. We discuss limitations and benefits of a potential dsMESH-based CPB management strategy and list some important RNAi based CPB research topics, which will have to be addressed in future.
Colorado potato beetle (CPB), Leptinotarsa decemlineata, is a serious pest of potato and other solanaceous crops. It is well known for its ability to rapidly evolve resistance to insecticides; it has already evolved resistance to all major insecticide classes (Alyokhin et al., 2008). Extensive use of conventional insecticides can have undesirable effects on the environment, non-target organisms and human health. Compared to chemical pesticides, double-stranded RNAs (dsRNAs) have the advantage of high selectivity towards the target organism and rapid environmental degradation into non-toxic compounds (Dubelman et al., 2014; Albright et al., 2017). Therefore, this novel pest management approach has the potential to decrease the extensive use of conventional insecticides.
When delivered into cells, dsRNAs activate the RNA interference (RNAi) mechanism that mediates a sequence-specific suppression of transcription, also called gene silencing (Joga et al., 2016). In CPB, unlike some other insects, dsRNAs are not degraded by gut nucleases, are efficiently taken up by the gut epithelium cells, and can trigger local as well as systemic RNAi response (Cappelle et al., 2016). This makes CPB an excellent candidate for pest management using dsRNAs, which was first recognized in a study by Baum et al. (2007) that has also identified several RNAi targets. This was followed by studies that identified novel effective target genes in CPB (Zhu et al., 2011; Zhou et al., 2013; Wan et al., 2014; Fu et al., 2015; Lü et al., 2015; Meng et al., 2015; Zhu et al., 2015; Fu et al., 2016; Guo et al., 2016; Shi et al., 2016a; Shi et al., 2016b; Meng et al., 2018; Xu et al., 2018) and for some targets also validated in field trials (Guo et al., 2018). In western corn rootworm, Hu et al. (2016) identified another target gene—mesh (alternatively named dvssj2) which encodes a smooth septate junction protein important for structural integrity of the midgut epithelium. They showed that silencing mesh impairs midgut barrier function which results in increased larval mortality (Hu et al., 2019).
In this study, we used in vitro and in vivo synthesized dsRNA designed to silence the mesh gene in CPB. We performed laboratory-based feeding assays with CPB at different stages of larval development as well as a small-scale field trial to validate the designed dsRNA’s pesticidal potential in a commercial production system. Therefore, our study offers new data on dsMESH effectiveness in another coleopteran, CPB, which represents an important crop pest and the most probable first candidate for spray-induced gene silencing commercialization.
To quantify the expression levels of mesh, RNA was extracted from three to four individual larvae (three to four biological replicates), except for the study of mesh expression in CPB body parts where one pooled sample from 3–4 beetles for each body part was analyzed. RNA extraction was performed using TRIzol reagent (Invitrogen) and Direct-zol RNA Microprep kit (Zymo Research). DNase treatment and reverse transcription were performed as described previously (Petek et al., 2012). RNA concentration and integrity were validated using a NanoDrop ND-1000 spectrophotometer and agarose gel electrophoresis. The efficiency of DNase treatment was confirmed by qPCR with no RT samples.
The expression of mesh was assessed by quantitative PCR (qPCR). Mesh gene model from i5k genome version 0.5.3 (LDEC006484; Schoville et al., 2018) was corrected based on alternative models and mapped RNA-seq reads available in i5k’s WebApollo instance (Supplementary Figure 1 and Supplementary Data 1.1). The qPCR primers and probes were designed in Primer Express 2.3 (Applied Biosystems) using default parameters for TaqMan amplicons and were synthesized by IDT. Assay specificity was verified in silico using blastn queries against all transcripts predicted in the CPB genome (Schoville et al., 2018). The linear ranges and amplification efficiencies were determined across five 10-fold serial dilutions of cDNA. Target gene accumulation was normalized to three endogenous control genes: LdRP4 (Shi et al., 2013), 18S rRNA (Eukaryotic 18S rRNA Endogenous Control, Applied Biosystems) and LdSmt3 (Petek et al., 2014). Primer and probe sequences, qPCR chemistry and other assay metadata are available in Supplementary Table 1.
FastStart Universal Probe Master Rox mastermix (Roche) was used for TaqMan chemistry based assays and Power SYBR mastermix (Applied Biosystems) for SYBR Green chemistry based assays. Dilution of cDNA samples and pipetting of qPCR reagents onto 386-well plates was performed on a Microlab STARlet automated liquid handling system (Hamilton). Reactions were performed in 5 μl total volume on LightCycler 480 (Roche) as described previously (Petek et al., 2012). Melting curve analysis was applied for SYBR green chemistry based assays LdRP4 and LdSmt3 to control for primer dimer formation and amplification specificity in each reaction. Each sample was analyzed in two replicates of two dilutions to check for the presence of inhibitors in the sample. Cq values were calculated using instrument manufacturer software and exported as text files. Amplification quality control for each sample and relative quantification based on the standard curve method was performed in quantGenius software (Baebler et al., 2017). For every gene, the limit of quantification (LOQ) was determined from the standard curve. The normalized target copy numbers calculated by quantGenius were exported to an Excel file to calculate standard errors and Student’s t-test statistics.
To avoid sequence regions that might affect other species due to nucleotide conservation we used EMBOSS splitter (Rice et al., 2000) to generate all possible 21-mers for the CPB mesh transcript. These 21-nt sequences were queried using BLASTn against non-target organism transcriptomes including Homo sapiens, Apis mellifera, Bombus terrestris, Danaus plexippus, Drosophila melanogaster, Megachile rotundata, Nasonia vitripennis, and Tribolium castaneum. Regions of the transcripts with 20 or 21 nt BLASTn hits in non-target organisms were excluded from dsRNA design. Based on the above metrics, the longest CPB-specific region was selected as the input sequence to design a long dsRNA molecule using e-RNAi web service (Horn and Boutros, 2010) using default parameters. Such bioinformatics design however does not exclude the possibility of off-target effects. For example, due to crosstalk between siRNA and miRNA pathways, off-target silencing could be triggered by siRNAs with less sequence conservation. Also, due to limited genomics and transcriptomics sequence availability in Arthropods a comprehensive bioinformatics analysis is not possible (Christiaens et al., 2018). As non-specific dsRNA control, the dsEGFP with sequence corresponding to a fragment of enhanced green fluorescent protein (Guo et al., 2015) was used (sequences in Supplementary Data 1.2). In vitro synthesis of dsMESH and dsEGFP was performed by AgroRNA (South Korea). The quality and quantity of dsRNA was determined using agarose gel electrophoresis and NanoDrop.
To in vivo synthesize dsMESH, a 417 bp fragment of the gene was amplified by PCR from a pooled CPB midgut cDNA sample using Phusion DNA polymerase (Biolabs) and cloned into L4440gtwy (Addgene) using pCR8/GW/TOPO TA Cloning Kit (Invitrogen) to obtain MESH::L4440. The correct fragment insertion was confirmed by Sanger sequencing (Eurofins Genomics). The GFP::L4440 plasmid (Addgene), containing a full-length (857 bp) green fluorescence protein sequence insert was used to produce dsGFP. Heat-shock induced competent Escherichia coli HT115 (DE3) bacteria were transformed with MESH::L4440 and GFP::L4440, respectively. Transformation was confirmed by colony PCR using KAPA2G Robust HotStart Polymerase (Kapa Biosystems).
To produce dsRNA, cultures of transformed bacteria were grown to OD600 0.5 in 250 ml of liquid LB media. Production of dsRNAs was induced with 400 µM IPTG (Thermo Scientific). After 4 h, cells were pelleted, re-suspended in 6 ml nuclease-free water (Sigma) and lysed by boiling followed by four freezing-thawing cycles and a 15 min treatment in ultrasonic bath SONIS 4 (Iskra PIO). Bacterial lysates were centrifuged at 9,000 g for 20 min and the supernatant was concentrated to 1/10 volume using GeneVac EZ-2plus (Genevac Ltd).
To estimate the quantity of dsRNA produced, total RNA was extracted from bacterial lysates using Direct-zol RNA MiniPrep Plus kit (Zymo Research), treated with DNase I (Zymo Research) and reverse transcribed using High-Capacity cDNA Reverse Transcription Kit (Applied Biosystems). In vivo synthesized dsMESH and dsGFP quantities were estimated from 1% agarose E-Gel EX (Thermo) RNA band intensities. Identity of dsRNA was confirmed by RNase If treatment (Supplementary Data 1.3, Supplementary Figure 2).
CPBs were reared on potato plants cv. Désirée in conditions described previously (Petek et al., 2014). Larvae, which hatched on the same day, were reared on non-treated detached potato leaves until most larvae reached desirable treatment stage. For each feeding trial, larvae were randomly selected and assigned into treatment groups, enclosed into plastic or glass containers and reared on untreated potato foliage. DsRNA were either sprayed on detached leaves, potted whole plants, or CPB eggs, or pipetted onto freshly cut leaf disks (Table 1). To protect detached leaves from desiccation, the petioles were placed in sterile 2 ml microcentrifuge tubes filled with 0.5% agarose gel, whereas leaf disks were placed into flat bottom 24-well plates with bottom covered by 0.5% agarose gel. After consumption of leaf disk (trials three and four, Table 1), the larvae were moved back to plastic containers and daily supplied with untreated detached potato leaves.
Nuclease-free water (Sigma) was used for blank control treatment and dilution of all dsRNAs. In all, except trial four, in vitro synthesized dsEGFP (Guo et al., 2015) was used as non-specific dsRNA treatment control. In trial four, in vivo synthesized dsGFP sequence from GFP::L4440 plasmid (Addgene) was used instead. During the study, we adhered to national and institutional biosafety standards. More details on feeding trials are given in Table 1 and Supplementary Data 1.4-8.
Analysis of right-censored survival data was performed using Cox proportional hazards regression model fit and statistical tests implemented in R survival package version 2.42 (Therneau and Grambsch, 2000). Data analysis execution calls are given in Supplementary Data 1.9.
A small-scale field trial was conducted in June and July 2019 on three locations near Ljubljana, Slovenia. Two trials were performed on adjacent potato fields in Šentjakob (46°05’13.4”N 14°34’06.8”E) and one in Iška vas (45°56’28.8”N 14°30’31.0”E). The experiment was designed following EPPO guidelines (EPPO, 2008). Cultural conditions were uniform for all plots of the trial at each location and conformed to local agricultural practice. To assess the efficacy of dsMESH the only difference between treatments was the method of CPB management. Three 25 m2 plots were marked at each location. Each plot was divided into four replicate sub-plots, giving four replicates per treatment. On each sub-plot, an individual potato plant infested by at least 15 CPB larvae was randomly selected and marked, giving four plants per treatment at each location. Before treatment, foliage from potato plants and weeds surrounding the marked potato plants was removed to restrict larval movement between plants. Any unhatched CPB eggs from the marked potato plants were removed. CPB larvae were counted and larval stages and plant defoliation percentages were determined for each plant separately. The leaf damage caused by the CPB larval herbivory was estimated visually by inspecting the first ten fully developed leaves from the topmost apical plant meristem downwards on each marked potato plant.
Marked plants were sprayed with in-vitro synthesized dsMESH in concentration 10 µg/ml. We used potato plants sprayed with the manufacturer recommended 0.5% diluted spinosad formulation (insecticide Laser 240 SC, Dow AgroSciences) as a positive control treatment and unsprayed plants as a negative control. Two days post treatment (dpt), CPB larvae on marked plants were recounted and stages determined, and 7 dpt larvae were counted again, their stages determined, and leaf damage estimated. From the relative change of leaf damage assessed before treatment and at 7 dpt, the parameter “leaf damage increase” was calculated.
Statistical differences in leaf damage, leaf damage increase, and larval mortality according to Henderson-Tilton were calculated using ANOVA and Bonferroni’s Multiple Comparison Test in GraphPad Prism 5.00 (GraphPad Software). The dataset was also analyzed using a general linear model (GLM), where the effect of factors treatment (dsMESH, spinosad, and control), experiment (trials 1–3) and replicate (1–4) on previously mentioned parameters was assessed. Further, Fisher’s least significance difference (LSD) procedure at 95% confidence level was used to discriminate between the treatments within the three-trial dataset. These analyses were performed with the statistical software Statgraphics Centurion XVI (StatPoint Technologies).
To test whether an RNAi insecticide targeting mesh will work against all CPB’s developmental stages, we profiled mesh expression through the stages. Constitutive expression of mesh was detected in all developmental stages. Expression in the gut is highest in fourth instar larvae preceding pupation and in adults (Figure 1 and Supplementary Dataset 1). Constitutive expression of mesh in larval and adult stages is also evident from mapped RNA-Seq data available at i5k CPB genome browser (Supplementary Figure 1A). This expression pattern is suitable for RNAi insecticide targets as the gene is expressed in stages in which the beetles feed on plant leaves. We also qualitatively showed higher expression of mesh in samples of foregut, midgut and hindgut tissues compared to samples of legs, head and antennae (Supplementary Figure 3 and Supplementary Dataset 2).
Figure 1 Expression of mesh at different CPB developmental stages. Gene expression values are shown relative to early egg sample average. In the eggs and neonatal larval stages, entire organisms were sampled, whereas in later stages, only guts were sampled. Bars show the range of data and lines shown the mean.
To test the efficiency of dsMESH in silencing the target gene and its potential as a bioinsecticide we performed three laboratory feeding trials in which we treated CPB at different life stages (Table 1). Firstly, we fed 2nd instar larvae continuously on in vitro synthesized dsRNA-sprayed potato foliage and left them to pupate and emerge as adults (trial one, Table 1). We confirmed silencing of mesh gene by dsMESH after 4 days of treatment. Compared to dsEGFP treatment, dsMESH reduced mesh expression by 71% (p<0.001, Supplementary Dataset 3) and larval survival at that point was 48, 80, and 95% for dsMESH, dsEGFP, and water treatment, respectively (Supplementary Figure 4 and Supplementary Dataset 4).
We also tested the effectiveness of dsMESH on 4th instar larvae (trial two, Table 1), which is the final instar before pupation. We recorded adult emergence and inspected plant substrate for beetle carcasses at the end of the trial. Adult emergence rate was 11% in larvae exposed to dsMESH, which is significantly lower compared to more than 75% for dsEGFP and water treatments (p<0.01; Figure 2B and Supplementary Dataset 5). Additionally, in all three emerged adults from the dsMESH treated group we observed darkened deformed elytra (Supplementary Figure 5) and the beetles died within two days after emergence. In contrast, dsEGFP and water treated beetles exhibited normal phenotypes and no adult mortality. From the substrate of dsMESH treated plants, we recovered two larval and six adult carcasses (Supplementary Figure 5) whereas in substrates of dsEGFP and water treated plants we found no carcasses. Trial two thus shows that dsMESH is also effective against 4th instar larvae.
Figure 2 Effectiveness of in vitro synthesized dsMESH treatment of different Colorado potato beetle developmental stages. (A) Kaplan-Meier survival curves of 2nd instar larvae exposed to discontinuous administration of dsRNA (trial three). Survival was significantly reduced for dsMESH compared to water or dsEGFP treatment (p<0.001). (B) Proportion of beetles not emerged as adults after 4th instar larval dsRNA treatment (trial two). Adult emergence was significantly lower for dsMESH treated larvae compared to water or dsEGFP treatment (p<0.01). (C) Kaplan-Meier survival curves of larvae hatched from dsRNA‑treated eggs (trial five). Survival was significantly reduced for dsMESH compared to water or dsEGFP treatment (p<0.001). dpt – days post treatment.
In trial five, we tested the effectiveness of dsMESH spraying on CPB eggs. We sprayed freshly laid CPB egg masses (Supplementary Figure 6) and transferred 1st instar larvae to untreated potato foliage. Most larvae hatched three days after egg treatment. We observed no difference in larval emergence between dsMESH and control treatments. Massive larval die-off in dsMESH treated group occurred in 6–7 days old larvae (9–10 days post egg treatment, Figure 2C). The survival of dsMESH treated 6 days old larvae was 61%, and a day later only 23%. In comparison, for both dsEGFP and water treated groups, the survival at that time point was 100% (Figure 2C and Supplementary Dataset 8). Only 4% of dsMESH treated larvae survived until 13 dpt, whereas 97% and 95% larvae survived in dsEGFP and water treated groups, respectively (p<0.001; Figure 2C and Supplementary Data 1.9). This trial shows high insecticidal efficiency of dsMESH also when sprayed on CPB eggs.
We next compared the effect of the dsRNA administration approach. Contrary to the above-described feeding trials, where larvae were continuously fed with dsRNA-sprayed potato leaves, here we exposed each individual larva (2nd instar) to the same dose of dsRNA by discontinuous administration via treated potato leaf disks (trial three and four, Table 1). We observed similar survival trends as the ones obtained with continuous treatment regime (trial one).
In the first leaf-disk feeding trial (trial three), we observed a substantial reduction in survival 4 dpt, reaching only 18% survival in the case of dsMESH treated larvae compared to more than 90% survival in dsEGFP and water treatments (Figure 2A, Supplementary Dataset 6). In trial four, most substantial survival reduction was observed 5 dpt, where dsMESH treated larval survival rate was 54% compared to 100% survival in both dsGFP and water treatments (Figure 3, Supplementary Dataset 7). In both trials, statistical analysis indicates highly significant survival reduction for dsMESH treatment (p<0.001; Supplementary Data 1.9).
Figure 3 Efficiency of in vitro and in vivo synthesized dsMESH (trial four). (A) Larval weight throughout the trial was significantly reduced when using either in vitro or in vivo synthesized dsMESH compared to water or dsEGFP treatment. Error bars show standard error of the mean. (B) Kaplan-Meier survival curves with survival proportions plotted. Survival was significantly reduced for dsMESH compared control treatments (p<0.001). dpt – days post treatment.
Larval survival analysis and weight measurements in trial four (Figure 3; Supplementary Datasets 7 and 12) show that in vivo and in vitro synthesized dsMESH are similarly effective (Supplementary Data 1.9). In addition, by testing serial dilutions of in vitro synthesized dsMESH, we showed that ingestion of as little as 6 ng of dsMESH caused more than 90% larval mortality (Figure 3B). No significant effect of bacterially produced dsGFP treatment on larval weight or survival was observed (Figure 3).
In order to confirm the efficacy of dsMESH as potential insecticide also under environmental conditions we treated potato plants growing in three different fields with in vitro synthesized dsMESH. No formulation to increase dsRNA stability or uptake was used to make the results of the field trial more comparable to the laboratory-gained results. Mortality rates for dsMESH treatment after 7 days were significantly higher (F2, 40 = 16; P<0.0001) compared to untreated plants according to ANOVA and were 93, 84, and 95%, in the three locations, respectively. GLM analyses showed a significant effect of factor treatment on parameters leaf damage increase (F2, 41 = 34, 8; P<0.0001) and insect mortality rate (F2,40 = 13.2; P<0.0001; Figure 4). Factors experiment and replicate did not significantly affect the observed parameters. Spinosad acted more rapidly than dsMESH, causing on average 98% of larval mortality in just two days, whereas the average mortality rate of dsMESH treatment at that time point was 32% (Supplementary Dataset 13).
Figure 4 Treatment with dsMESH reduced Colorado potato beetle potato infestation in the field. (A) Potato defoliation due to CPB herbivory expressed as leaf damage increase, which was calculated by subtracting data from initial and final leaf damage assessment after seven days. (B) CPB larval mortality in the field trial. The data from all three locations were used. Bars not sharing the same lowercase letter are significantly different.
We performed a systematic evaluation of applicability of an RNAi-based insecticide targeting the mesh gene (dsMESH) and validated its insecticidal action in CPB. The incentive to use this target gene came from its high expression in CPB gut in most developmental stages and the lethal phenotypes observed in Drosophila knockout mutants (Gramates et al., 2017) and Tribolium castaneum RNAi screens (Ulrich et al., 2015). Mesh is a transmembrane protein important for proper organization of the insect midgut septate junctions and Drosophila mesh mutants show an impaired barrier function of the midgut (Izumi et al., 2012). Silencing mesh by RNAi in Drosophila adults, however, does not impair gut integrity but increases gut bacterial load by regulating dual oxidase expression (Xiao et al., 2017).
Mesh was first identified as an effective RNAi pesticide target in western corn rootworm, Diabrotica virgifera virgifera, another coleopteran pest closely related to CPB (Hu et al., 2016). Our CPB feeding trials with in vitro synthesized dsMESH consistently showed high mortality rates in larvae with effective dose in the ng range, similarly as reported for corn rootworm by Hu et al. (2016). In our first feeding trials we used 2nd instar larvae because the first two CPB instars were described as most susceptible to RNAi (Guo et al., 2015). In addition, we showed that dsMESH treatment is effective against 4th instar larvae and CPB eggs. Surprisingly, reports of insect egg treatment by spraying or soaking in dsRNA are rare and have different outcomes. Soaking Asian corn borer (Ostrinia furnalalis) eggs in pesticidal dsRNA solutions caused reduced hatching (Wang et al., 2011). On the contrary, in the corn earworm, Helicoverpa zea, soaking eggs in dsRNA as well as larval feeding delivery had no effect, whereas injecting eggs with same dsRNA induced RNAi and reduced egg hatching rate (Wang et al., 2018). In our trial, spraying eggs with dsMESH did not affect egg hatching although we showed that mesh is expressed in eggs. The larval die-off six to seven days after emergence from dsMESH treated eggs leads to suggest that dsRNA was mostly taken up by neonatal larvae while feeding on eggshells.
The activity of dsRNA obtained in in vitro tests or laboratory feeding experiments might not reflect that on the field. Thus, we decided to validate our laboratory-based trial results in a field trial comparing dsMESH efficiency to that of spinosad. Spinosad was used as a positive control as a) it is highly effective against CPB, b) it is a bioinsecticide and can thus be used also in organic farming, c) it is an insecticide registered for control of CPB in Slovenia and d) it is an alternative to conventional chemical insecticides (e.g. thiacloprid, beta-cyfluthrin), which can be ecotoxicologically problematic, and for which we are trying to find alternatives for. The observed field mortality was slightly lower compared to laboratory trials, which is reasonable, as larval treatment on the field was not as controlled and uniform as in the laboratory. In addition, reduced dsRNA stability in the field is expected due to direct sunlight exposure and lack of formulation to improve dsRNA stability (Cagliari et al., 2019). Compared to the wide-spectrum insecticide spinosad (Kirst, 2010), dsMESH has an inherent lag phase in observed mortality, which can be attributed to its mode of action. The toxicity of dsRNA depends on target protein’s half-life (Scott et al., 2013) therefore, we expected to observe lethal effects after a few days. Despite its slower activity, the final mortality and leaf damage caused by dsMESH treatment in the field trial were not statistically different to that of spinosad.
In our experiments we used in vitro synthesized dsRNA, however, for large scale field application applying crude extract of E. coli producing dsMESH might be a good option to reduce the costs. Our laboratory feeding trials showed that in vivo produced dsMESH and the dsMESH synthesized in vitro are similarly effective. Because the dsMESH amounts in bacterial extracts was approximated from the gel, a more accurate comparison is not possible. Despite the potential advantages of applying dsRNA as a crude bacterial extract, the approach has also additional risks such the presence of synthetic DNA elements and the possibility of having a GMO status assigned (Fletcher et al., 2020).
Although plant-incorporated protectants (transgenic plants) are the most cost-effective way of using RNAi-based pesticide technology, their public acceptance might prove challenging, at least in the European Union. Other possibilities were envisioned, such as transformed insect symbionts (Whitten et al., 2016) or viruses expressing pesticidal RNA molecules (Taning et al., 2018), albeit again using genetically modified organisms. As an alternative, non-transformative strategies of dsRNAs application, i.e. spray-induced gene silencing, are being investigated and CPB is the first agricultural pest for which this technology might be commercialized (Cagliari et al., 2019).
We have shown in laboratory trials as well as in the field that spraying with insecticidal dsRNA is a highly efficient strategy for managing CPB. We are planning to test dsMESH in a larger field trial using standard agricultural spraying equipment and against a range of other insecticides. For RNAi-recalcitrant agricultural pests, future research will have to focus on formulations to improve dsRNA stability and cellular uptake. Apart from efficiency, further research is needed on biosafety implications of this new pest management strategy. This includes investigating possible impact of dsRNA on human health and the environment (Rodrigues and Petrick, 2020). For sustainable use of this technology in agriculture, integrated pest management strategies will have to be employed to delay the development of pest resistance to dsRNAs (Khajuria et al., 2018).
All datasets generated for this study are included in the article/Supplementary Material.
MP provided the initial concept and design of the study, performed gene expression analysis, laboratory-based feeding trials, contributed to execution and evaluation of the field trial and wrote the manuscript. AC and RF established the bacterial production of dsRNA. RF also helped with execution of the feeding trial four. JR designed and led the execution of the field trial. KG contributed to study design, data interpretation and manuscript drafting. All authors contributed to the article and approved the submitted version.
This work was financially supported by the Slovenian Research Agency (research core funding No Z4-706, J4-1772, P4-0165 and P4-0072). Presentation of results at international meetings was supported by the COST Action CA15223.
The authors declare that the research was conducted in the absence of any commercial or financial relationships that could be construed as a potential conflict of interest.
The authors thank Špela Prijatelj Novak, Tjaša Lukan, Lidija Matičič and Eva Praprotnik for technical support and prof. Guy Smagghe for supplying CPB eggs from his lab colony. The E. coli HT115 (DE3) strain was provided by the CGC, which is funded by NIH Office of Research Infrastructure Programs (P40 OD010440).
The Supplementary Material for this article can be found online at: https://www.frontiersin.org/articles/10.3389/fpls.2020.01250/full#supplementary-material
Albright, V. C., Wong, C. R., Hellmich, R. L., Coats, J. R. (2017). Dissipation of double-stranded RNA in aquatic microcosms. Environ. Toxicol. Chem. 36, 1249–1253. doi: 10.1002/etc.3648
Alyokhin, A., Baker, M., Mota-Sanchez, D., Dively, G., Grafius, E. (2008). Colorado Potato Beetle Resistance to Insecticides. Am. J. Potato Res. 85, 395–413. doi: 10.1007/s12230-008-9052-0
Baebler, Š, Svalina, M., Petek, M., Stare, K., Rotter, A., Pompe-Novak, M., et al. (2017). quantGenius: implementation of a decision support system for qPCR-based gene quantification. BMC Bioinf. 18, 276. doi: 10.1186/s12859-017-1688-7
Baum, J., Bogaert, T., Clinton, W., Heck, G. R., Feldmann, P., Ilagan, O., et al. (2007). Control of coleopteran insect pests through RNA interference. Nat. Biotechnol. 25, 1322–1326. doi: 10.1038/nbt1359
Cagliari, D., Dias, N. P., Galdeano, D. M., dos Santos, E. A., Smagghe, G., Zotti, M. J. (2019). Management of Pest Insects and Plant Diseases by Non-Transformative RNAi. Front. Plant Sci. 10, 1319. doi: 10.3389/fpls.2019.01319
Cappelle, K., de Oliveira, C. F. R., Van Eynde, B., Christiaens, O., Smagghe, G. (2016). The involvement of clathrin-mediated endocytosis and two Sid-1-like transmembrane proteins in double-stranded RNA uptake in the Colorado potato beetle midgut. Insect Mol. Biol. 25, 315–323. doi: 10.1111/imb.12222
Christiaens, O., Dzhambazova, T., Kostov, K., Arpaia, S., Joga, M. R., Urru, I., et al. (2018). Literature review of baseline information on RNAi to support the environmental risk assessment of RNAi-based GM plants. EFSA Support Publ. 15, 1424E. doi: 10.2903/sp.efsa.2018.EN-1424
Dubelman, S., Fischer, J., Zapata, F., Huizinga, K., Jiang, C., Uffman, J., et al. (2014). Environmental Fate of Double-Stranded RNA in Agricultural Soils. PloS One 9, e93155. doi: 10.1371/journal.pone.0093155
EPPO (2008). “Efficacy evaluation of insecticides,” in Leptinotarsa decemlineata. (European and Mediterranean Plant Protection Organization) PP1/012(4).
Fletcher, S. J., Reeves, P. T., Hoang, B. T., Mitter, N. (2020). A Perspective on RNAi-Based Biopesticides. Front. Plant Sci. 11, 51. doi: 10.3389/fpls.2020.00051
Fu, K.-Y., Lü, F.-G., Guo, W.-C., Li, G.‐Q. (2015). Characterization and functional study of a putative juvenile hormone diol kinase in the Colorado potato beetle Leptinotarsa decemlineata (Say). Arch. Insect Biochem. Physiol. 90, 154–167. doi: 10.1002/arch.21251
Fu, K.-Y., Li, Q., Zhou, L.-T., Meng, Q.‐W., Lü, F.-G., Guo, W.-C., et al. (2016). Knockdown of juvenile hormone acid methyl transferase severely affects the performance of Leptinotarsa decemlineata (Say) larvae and adults. Pest Manag. Sci. 72, 1231–1241. doi: 10.1002/ps.4103
Gramates, L. S., Marygold, S. J., dos Santos, G., Urbano, J.-M., Antonazzo, G., Matthews, B. B., et al. (2017). FlyBase at 25: looking to the future. Nucleic Acids Res. 45, D663–D671. doi: 10.1093/nar/gkw1016
Guo, Z., Kang, S., Zhu, X., Xia, J., Wu, Q., Wang, S., et al. (2015). The novel ABC transporter ABCH1 is a potential target for RNAi-based insect pest control and resistance management. Sci. Rep. 5, 13728. doi: 10.1038/srep13728
Guo, W.-C., Liu, X.-P., Fu, K.-Y., Shi, J.‐F., Lü, F.‐G., Li, G.‐Q. (2016). Nuclear receptor ecdysone-induced protein 75 is required for larval-pupal metamorphosis in the Colorado potato beetle Leptinotarsa decemlineata (Say). Insect Mol. Biol. 25, 44–57. doi: 10.1111/imb.12197
Guo, W., Bai, C., Wang, Z., Wang, P., Fan, Q., Mi, X., et al. (2018). Double-Stranded RNAs High-Efficiently Protect Transgenic Potato from Leptinotarsa decemlineata by Disrupting Juvenile Hormone Biosynthesis. J. Agric. Food Chem. 66, 11990–11999. doi: 10.1021/acs.jafc.8b0391
Horn, T., Boutros, M. (2010). E-RNAi: A web application for the multi-species design of RNAi reagents-2010 update. Nucleic Acids Res. 38, 332–339. doi: 10.1093/nar/gkq317
Hu, X., Richtman, N. M., Zhao, J.-Z., Duncan, K. E., Niu, X., Procyk, L. A., et al. (2016). Discovery of midgut genes for the RNA interference control of corn rootworm. Sci. Rep. 6, 30542. doi: 10.1038/srep30542
Hu, X., Steimel, J. P., Kapka-Kitzman, D. M., Davis-Vogel, C., Richtman, N. M., Mathis, J. P., et al. (2019). Molecular characterization of the insecticidal activity of double-stranded RNA targeting the smooth septate junction of western corn rootworm (Diabrotica virgifera virgifera). PloS One 14, e0210491. doi: 10.1371/journal.pone.0210491
Izumi, Y., Yanagihashi, Y., Furuse, M. (2012). A novel protein complex, Mesh-Ssk, is required for septate junction formation in the Drosophila midgut. J. Cell Sci. 125, 4923–4933. doi: 10.1242/jcs.112243
Joga, M. R., Zotti, M. J., Smagghe, G., Christiaens, O. (2016). RNAi Efficiency, Systemic Properties, and Novel Delivery Methods for Pest Insect Control: What We Know So Far. Front. Physiol. 7, 553. doi: 10.3389/fphys.2016.00553
Khajuria, C., Ivashuta, S., Wiggins, E., Flagel, L., Moar, W., Pleau, M., et al. (2018). Development and characterization of the first dsRNA-resistant insect population from western corn rootworm, Diabrotica virgifera virgifera LeConte. PloS One 13, e0197059. doi: 10.1371/journal.pone.0197059
Kirst, H. A. (2010). The spinosyn family of insecticides: realizing the potential of natural products research. J. Antibiot. (Tokyo) 63, 101–111. doi: 10.1038/ja.2010.5
Lü, F.-G., Fu, K.-Y., Guo, W.-C., Li, G.-Q. (2015). Characterization of two juvenile hormone epoxide hydrolases by RNA interference in the Colorado potato beetle. Gene 570, 264–271. doi: 10.1016/j.gene.2015.06.032
Meng, Q.-W., Liu, X.-P., Lü, F.-G., Fu, K.-Y. (2015). Involvement of a putative allatostatin in regulation of juvenile hormone titer and the larval development in Leptinotarsa decemlineata (Say). Gene 554, 105–113. doi: 10.1016/j.gene.2014.10.033
Meng, Q. W., Xu, Q. Y., Deng, P., Guo, W.-C., Li, G.‐Q. (2018). Transcriptional response of Methoprene-tolerant (Met) gene to three insect growth disruptors in Leptinotarsa decemlineata (Say). J. Asia Pac. Entomol. 21, 466–473. doi: 10.1016/j.aspen.2018.02.011
Petek, M., Turnšek, N., Gašparič, M. B., Pompe Novak, M., Gruden, K., Slapar, N., et al. (2012). A complex of genes involved in adaptation of Leptinotarsa decemlineata larvae to induced potato defense. Arch. Insect Biochem. Physiol. 79, 153–181. doi: 10.1002/arch.21017
Petek, M., Rotter, A., Kogovšek, P., Baebler, Š., Mithöfer, A., Gruden, K. (2014). Potato virus Y infection hinders potato defence response and renders plants more vulnerable to Colorado potato beetle attack. Mol. Ecol. 23, 5378–5391. doi: 10.1111/mec.12932
Rice, P., Longden, I., Bleasby, A. (2000). EMBOSS: The European Molecular Biology Open Software Suite. Trends Genet. 16, 276–277. doi: 10.1016/S0168-9525(00)02024-2
Rodrigues, T. B., Petrick, J. S. (2020). Safety Considerations for Humans and Other Vertebrates Regarding Agricultural Uses of Externally Applied RNA Molecules. Front. Plant Sci. 11, 407. doi: 10.3389/fpls.2020.00407
Schoville, S. D., Chen, Y. H., Andersson, M. N., Benoit, J. B., Bhandari, A., Bowsher, J. H., et al. (2018). A model species for agricultural pest genomics: the genome of the Colorado potato beetle, Leptinotarsa decemlineata (Coleoptera: Chrysomelidae). Sci. Rep. 8, 1931. doi: 10.1038/s41598-018-20154-1
Scott, J. G., Michel, K., Bartholomay, L. C., Siegfried, B. D., Hunter, W. B., Smagghe, G., et al. (2013). Towards the elements of successful insect RNAi. J. Insect Physiol. 59, 1212–1221. doi: 10.1016/j.jinsphys.2013.08.014
Shi, X.-Q., Guo, W.-C., Wan, P.-J., Zhou, L.-T., Ren, X.-L., Ahmat, T., et al. (2013). Validation of reference genes for expression analysis by quantitative real-time PCR in Leptinotarsa decemlineata (Say). BMC Res. Notes 6, 93. doi: 10.1186/1756-0500-6-93
Shi, J.-F., Fu, J., Mu, L.-L., Guo, W.-C., Li, G.‐Q. (2016a). Two Leptinotarsa uridine diphosphate N-acetylglucosamine pyrophosphorylases are specialized for chitin synthesis in larval epidermal cuticle and midgut peritrophic matrix. Insect Biochem. Mol. Biol. 68, 1–12. doi: 10.1016/j.ibmb.2015.11.005
Shi, J.-F., Mu, L.-L., Chen, X., Guo, W.-C., Li, G.‐Q. (2016b). RNA interference of chitin synthase genes inhibits chitin biosynthesis and affects larval performance in Leptinotarsa decemlineata (Say). Int. J. Biol. Sci. 12, 1319–1331. doi: 10.7150/ijbs.14464
Taning, C. N. T., Christiaens, O., Li, X., Swevers, L., Casteels, H., Maes, M., et al. (2018). Engineered Flock House Virus for Targeted Gene Suppression Through RNAi in Fruit Flies (Drosophila melanogaster) in Vitro and in Vivo. Front. Physiol. 9, 805. doi: 10.3389/fphys.2018.00805
Therneau, T. M., Grambsch, P. M. (2000). Modeling Survival Data: Extending the Cox Model (New York: Springer New York).
Ulrich, J., Dao, V. A., Majumdar, U., Schmitt-Engel, C., Schwirz, J., Schultheis, D., et al. (2015). Large scale RNAi screen in Tribolium reveals novel target genes for pest control and the proteasome as prime target. BMC Genomics 16, 674. doi: 10.1186/s12864-015-1880-y
Wan, P., Fu, K., Lü, F., Guo, W.-C., Li, G.‐Q. (2014). A putative Δ1-pyrroline-5-carboxylate synthetase involved in the biosynthesis of proline and arginine in Leptinotarsa decemlineata. J. Insect Physiol. 71, 105–113. doi: 10.1016/j.jinsphys.2014.10.009
Wang, Y., Zhang, H., Li, H., Miao, X. (2011). Second-Generation Sequencing Supply an Effective Way to Screen RNAi Targets in Large Scale for Potential Application in Pest Insect Control. PloS One 6, e18644. doi: 10.1371/journal.pone.0018644
Wang, J., Gu, L., Knipple, D. C. (2018). Evaluation of some potential target genes and methods for RNAi-mediated pest control of the corn earworm Helicoverpa zea. Pestic Biochem. Physiol. 149, 67–72. doi: 10.1016/j.pestbp.2018.05.012
Whitten, M. M. A., Facey, P. D., Del Sol, R., Fernández-Martínez, L. T., Evans, M. C., Mitchell, J. J., et al. (2016). Symbiont-mediated RNA interference in insects. Proc. R. Soc. B. Biol. Sci. 283, 20160042. doi: 10.1098/rspb.2016.0042
Xiao, X., Yang, L., Pang, X., Zhang, R., Zhu, Y., Wang, P., et al. (2017). A Mesh–Duox pathway regulates homeostasis in the insect gut. Nat. Microbiol. 2, 17020. doi: 10.1038/nmicrobiol.2017.20
Xu, Q.-Y., Meng, Q.-W., Deng, P., Guo, W.-C., Li, G.‐Q. (2018). Leptinotarsa hormone receptor 4 (HR4) tunes ecdysteroidogenesis and mediates 20-hydroxyecdysone signaling during larval-pupal metamorphosis. Insect Biochem. Mol. Biol. 94, 50–60. doi: 10.1016/j.ibmb.2017.09.012
Zhou, L.-T., Jia, S., Wan, P.-J., Kong, Y. Y., Guo, W.-C., Ahmat, T., et al. (2013). RNA interference of a putative S-adenosyl-L-homocysteine hydrolase gene affects larval performance in Leptinotarsa decemlineata (Say). J. Insect Physiol. 59, 1049–1056. doi: 10.1016/j.jinsphys.2013.08.002
Zhu, F., Xu, J., Palli, R., Ferguson, J., Palli, S. R. (2011). Ingested RNA interference for managing the populations of the Colorado potato beetle, Leptinotarsa decemlineata. Pest Manag. Sci. 67, 175–182. doi: 10.1002/ps.2048
Keywords: RNA interference (RNAi) feeding, dsRNA, gene silencing, RNAi pest control, survival analysis, Leptinotarsa decemlineata (Say), field trial, E coli HT115 (DE3)
Citation: Petek M, Coll A, Ferenc R, Razinger J and Gruden K (2020) Validating the Potential of Double-Stranded RNA Targeting Colorado Potato Beetle Mesh Gene in Laboratory and Field Trials. Front. Plant Sci. 11:1250. doi: 10.3389/fpls.2020.01250
Received: 11 February 2020; Accepted: 29 July 2020;
Published: 19 August 2020.
Edited by:
Jeremy Bruton Sweet, Sweet Environmental Consultants, United KingdomReviewed by:
Jia-He Wu, Chinese Academy of Sciences, ChinaCopyright © 2020 Petek, Coll, Ferenc, Razinger and Gruden. This is an open-access article distributed under the terms of the Creative Commons Attribution License (CC BY). The use, distribution or reproduction in other forums is permitted, provided the original author(s) and the copyright owner(s) are credited and that the original publication in this journal is cited, in accordance with accepted academic practice. No use, distribution or reproduction is permitted which does not comply with these terms.
*Correspondence: Marko Petek, bWFya28ucGV0ZWtAbmliLnNp
Disclaimer: All claims expressed in this article are solely those of the authors and do not necessarily represent those of their affiliated organizations, or those of the publisher, the editors and the reviewers. Any product that may be evaluated in this article or claim that may be made by its manufacturer is not guaranteed or endorsed by the publisher.
Research integrity at Frontiers
Learn more about the work of our research integrity team to safeguard the quality of each article we publish.