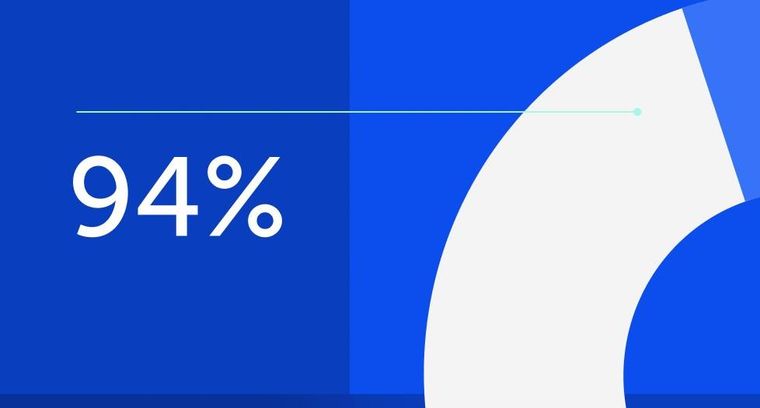
94% of researchers rate our articles as excellent or good
Learn more about the work of our research integrity team to safeguard the quality of each article we publish.
Find out more
ORIGINAL RESEARCH article
Front. Plant Sci., 16 July 2020
Sec. Plant Physiology
Volume 11 - 2020 | https://doi.org/10.3389/fpls.2020.01090
This article is part of the Research TopicStructure-Function Relationships in the XylemView all 5 articles
Increased abiotic stress along with increasing temperatures, dry periods and forest disturbances may favor biotic stressors such as simultaneous invasion of bark beetle and ophiostomatoid fungi. It is not fully understood how tree desiccation is associated with colonization of sapwood by fungi. A decrease in xylem sap surface tension (σxylem) as a result of infection has been hypothesized to cause xylem embolism by lowering the threshold for air-seeding at the pits between conduits and disruptions in tree water transport. However, this hypothesis has not yet been tested. We investigated tree water relations by measuring the stem xylem hydraulic conductivity (Kstem), σxylem, stem relative water content (RWCstem), and water potential (Ψstem), and canopy conductance (gcanopy), as well as the compound composition in xylem sap in Norway spruce (Picea abies) saplings. We conducted our measurements at the later stage of Endoconidiophora polonica infection when visible symptoms had occurred in xylem. Saplings of two clones (44 trees altogether) were allocated to treatments of inoculated, wounded control and intact control trees in a greenhouse. The saplings were destructively sampled every second week during summer 2016. σxylem, Kstem and RWCstem decreased following the inoculation, which may indicate that decreased σxylem resulted in increased embolism. gcanopy did not differ between treatments indicating that stomata responded to Ψstem rather than to embolism formation. Concentrations of quinic acid, myo-inositol, sucrose and alkylphenol increased in the xylem sap of inoculated trees. Myo-inositol concentrations also correlated negatively with σxylem and Kstem. Our study is a preliminary investigation of the role of σxylem in E. polonica infected trees based on previous hypotheses. The results suggest that E. polonica infection can lead to a simultaneous decrease in xylem sap surface tension and a decline in tree hydraulic conductivity, thus hampering tree water transport.
Trees respond to abiotic stress factors (e.g. heat, water deficit) by controlling water use and photosynthetic production with their leaf stomata, in addition to modifying growth rate and use of stored carbohydrates. Pre-existing water stress may also predispose trees to pests and pathogens (Netherer et al., 2015; Hossain et al., 2018; Matthews et al., 2018). Bark beetles are major pests of conifers in Europe (Kautz et al., 2017), and they simultaneously vector several species of ophiostomatoid fungi (Perry, 1991; Linnakoski et al., 2012; Linnakoski et al., 2016). Some of these ophiostomatoid species are likely interfering with tree water transport in the xylem. However, the mechanism by which these pathogen infections disturb tree water transport and their role in weakening tree vigor during an invasion remain unclear (Kuroda, 2005; Linnakoski et al., 2012; Giordano et al., 2013).
Xylem conduits can become air-filled, i.e. embolized, typically by air-seeding (Tyree and Sperry, 1989). Embolism reduces the ability to transport water in xylem, i.e. lowers the tree hydraulic conductance. In extreme cases, embolism may even lead to a feedforward cycle of loss in hydraulic conductance, resulting in hydraulic failure and subsequent tree mortality (McDowell et al., 2008; Brodribb and Cochard, 2009). Air-seeding occurs when the pressure difference over a water-air interface in the bordered pit membrane becomes larger than the xylem sap surface tension (σxylem) force required to restrict the air bubble (Sperry and Tyree, 1988; Delzon et al., 2010; Jansen et al., 2012). Thus, with lower σxylem a conduit is embolized already at higher Ψstem. The threshold for air-seeding is given by the Young-Laplace equation (Tyree and Zimmermann, 2002)
where ΔP is the difference in pressure between a water-filled and an air-filled conduit, σ is surface tension, α is the contact angle, and r is the radius of the pores that separate the air-water meniscus in the pit membranes between adjacent conduits (Sperry and Tyree, 1988). The theoretical framework thus suggests the first chain in the events should be the decrease in σxylem, followed by embolism formation and a decrease in hydraulic conductivity.
σxylem has typically been thought to equal the surface tension of pure water. However, the variability of σxylem in natural conditions has recently received attention (Christensen-Dalsgaard et al., 2011; Domec, 2011; Schenk et al., 2015; Losso et al., 2017; Schenk et al., 2017). A decrease in σxylem, e.g. due to surfactants, has been shown to increase xylem vulnerability to embolism in both angiosperms (Crombie et al., 1985; Sperry and Tyree, 1988; Choat et al., 2004) and gymnosperms (Cochard et al., 2009; Hölttä et al., 2012). These experiments have documented that a decrease in σxylem is proportional to Ψstem causing a given amount of embolism in accordance with Eq. (1) (Figure 1A). In addition, a decrease in σxylem is expected to lead to a lowered stem water content, as embolism formation empties the water conduits and decreases the capillary forces retaining water in extracellular spaces (Tyree and Yang, 1990) (Figure 1B). The surfactants responsible for changes in σxylem are not well known, although lipid surfactants have recently been found to be naturally present in xylem sap (Schenk et al., 2015; Schenk et al., 2017).
Figure 1 Theoretical relationship of σxylem, Kstem and Ψstem (A), and the theoretical relationship of σxylem, RWCstem and Ψstem (B) when σxylem is changed. Symbols refer to surface tensions of pure water (σxylem1) and xylem sap with lowered surface tension (σxylem2), P50 (1) is the P50 value (i.e. the Ψstem where 50% of the conductivity in water transport is lost because of embolism) when the xylem sap surface tension corresponds to σxylem1, and P50 (2) is the P50 value when the xylem sap surface tension corresponds to σxylem2. The relationship between P50 and σxylem is linear and is demonstrated also for conifers in Cochard et al. (2009).
European spruce bark beetle (Ips typographus) acts as a vector of ophiostomatoid fungi, of which Endoconidiophora polonica is the most virulent pathogen, capable of causing extensive necrotic lesions in infected tissues followed by wilting symptoms of the canopy and eventually tree death (Horntvedt et al., 1983; Krokene and Solheim, 1998; Repe et al., 2015; Linnakoski et al., 2017a; Linnakoski et al., 2017b). Bark beetles bore through bark, enabling the insect-vectored fungi to spread to the cambial zone, where the hyphae rapidly grow in the phloem and through the cell walls to adjacent living cells (Franceschi et al., 1998). The fungal hyphae grow radially in the ray parenchyma cells towards the xylem, where the hyphae spread among the tracheids via the bordered pits (Franceschi et al., 1998). Virulence of E. polonica is related to its capability to invade deeper into the xylem than other pathogenic ophiostomatoid fungi (Horntvedt et al., 1983; Krokene and Solheim, 1998). The role of reduced σxylem in xylem water transport during pathogenic infection, such as E. polonica, has previously been hypothesized but remains to be investigated (Tyree and Sperry, 1989; Christiansen and Fjone, 1993; Kuroda, 2005).
The aim here was to investigate the hypothesis of decreasing σxylem in Norway spruce (P. abies) saplings during E. polonica infection, focusing on the tree hydraulics perspective at the later stage of infection, i.e. after visible symptoms occur in the xylem of infected trees. The role of σxylem in tree water transport is not well known, especially during biotic interactions. To the authors’ knowledge, previous studies have not reported actual measurements of σxylem in infected trees. Our study may elucidate the physiological connection between E. polonica and its host tree, providing insight into the possible mechanisms involved in the decrease in tree vitality. We hypothesized that infection will result in (i) a decrease in σxylem and (ii) embolism formation with a decrease in xylem hydraulic conductivity. Thus, σxylem and stem hydraulic conductivity (Kstem) were measured along with canopy conductance (gcanopy), xylem water potential (Ψstem) and stem relative water content (RWCstem). Additionally, analyses of chemical compounds in the xylem sap were conducted to determine whether the concentration of specific compounds would correlate with σxylem.
The study was conducted in a greenhouse at the Viikki campus of the University of Helsinki, Finland (60° 13' N 25° 1' E) from June 10 to September 15, 2016. The environmental conditions in the greenhouse were set to day and night temperatures (24±2°C and 20±3°C) and relative humidity (57±10% and 67±10%). The sapling material (approximately 1.2 m in height; diameter above grafting 1.5±0.2 cm) was moved from the Haapastensyrjä nursery, southern Finland (60° 4’ N, 24° 3’ E) to Viikki campus in mid-May 2016. Two grafted clones (64 and 1510) of Norway spruce with 22 saplings in each clone were chosen as plant material for the study. Clones were used for reducing the variation caused by the tree genotypes in the studied tree responses to the E. polonica infection. The saplings were immediately replanted in 10-liter pots with a turf and soil mixture, and placed in the greenhouse. The saplings were allocated to three treatment groups: inoculated trees (10 per clone), wounded control trees (10 per clone), and intact control trees (2 per clone) for ruling out the wounding effect. The inoculated trees were inoculated with E. polonica (strain F5) (Linnakoski et al., 2017b) growing on 2% Malt Extract Agar (MEA). Inoculation was performed using a sterile 6 mm hole puncher to cut a bark disc, and an inoculum of the same size was placed onto the exposed xylem surface. The inoculation site was then covered with the bark disc and sealed with parafilm. The wounded trees were mock-inoculated following the same protocol, using sterile 2% MEA (later referred as wounded control), and the control trees were left intact. Each sapling was inoculated at approximately 40, 50, and 70 cm above the tree stem base, with the lowest site always above the grafting and all inoculations on the same side of the stem. The experiment with clone 64 began and ended a week before the experiment with clone 1510. Both clones, as well as the different treatments, were randomly distributed in the greenhouse. The saplings were irrigated weekly with approximately the same amount of water lost by transpiration during the week (~ 1 L).
Physiological and hydraulic measurements are compiled in Table 1. Tree water relations were monitored weekly from the second week onwards after inoculation by measuring the whole-tree transpiration rate and Ψstem above the inoculation point. The transpiration rate was measured gravimetrically by weighing the water loss of the saplings over a few hours, at about the time of the highest transpiration. gcanopy was calculated as the sapwood area-specific whole-tree transpiration rate divided by the vapor pressure deficit (VPD). VPD was calculated from temperature and relative humidity, which were measured by a Priva measuring box (Priva, Beijing, China), and the mean VPD over each measurement time was used for the calculation of gcanopy. Ψstem was measured simultaneously with the transpiration measurement from detached needle tips with a Scholander-type custom-made pressure chamber. The needles were covered with an aluminum bag for approximately 20 min to stop transpiration before collection of needle tips for Ψstem measurement (e.g. Turner, 1988).
Table 1 Physiological and hydraulic variables studied in Endoconidiophora polonica-inoculated and wounded control Picea abies saplings.
Saplings were always sampled in pairs (one inoculated and one wounded control at the same time) with two to three repetitions per week altogether for 13 weeks per clone. The first saplings were sampled approximately 3 weeks after the experiment started, starting with clone 64, thereafter sampling one clone every second week. The intact controls were sampled in weeks 6 and 13 following the treatment. The timing of the first samplings was determined in a trial experiment (not shown). This trial study indicated response to inoculation in embolism, detected by ultra-acoustic emission, ca. 2-3 weeks after inoculation.
The uppermost inoculation point was used for the hydraulic conductivity (Kstem) measurement and the collection of the σxylem sample. Kstem is the hydraulic conductance per given length and cross-sectional area (e.g. within a stem segment). Once a piece of a tree stem (about 10 cm in length) was cut, it was sealed in a plastic zipper bag. Within half an hour from the sampling, bark was peeled from one centimeter of the sample piece end. Silicon tape was wrapped around the peeled part of the stem to ensure water flowed only through the xylem and not through the bark. Stem segment ends were cut under water and attached to tubes with silicon tape, the stem base end to the upper tube with a water column and the stem apex end to the lower tube measuring the water flow rate. Before installing the lower tube, the first water drops coming through the xylem were collected in a plastic tube and used for the σxylem measurements, apart from the first drop, which was discarded to avoid contamination of the σxylem sample. Kstem was measured by flow of water pushed through sampled stem segments with approximately 0.1 bar pressure (Sperry et al., 1988). The pressure was created by a 1-meter column of water solution with HCl, KCl, and milli-Q water (pH ~ 2.2; osmolality 0.03 mol kg-1) through a tube with diameter between 4 and 9 mm according to the diameter of the stem piece. Water flow rate through the stem pieces was measured from the water meniscus with a caliper for 20 min by measuring the water level in the tube in upright position. Thereafter, the volumetric water flow rate through the stem piece was calculated by multiplying with the cross-sectional tube area. In addition to the Kstem measurement, the saturated Kstem (Kstem,saturated) was measured after pulling water through the stem pieces at ca. 0.8 bar pressure for 30 min with a vacuum pump (XX5522050, Millipore, MA, USA), and thereafter saturating the stem pieces in milli-Q water for 24 h prior to the measurement. Percentage loss of conductivity was thereafter calculated according to Pammenter and Van der Willigen (1998):
The σxylem measurements were carried out with a capillary rise method using the setup of Vanhanen et al. (2008). Glass capillary tubes were cleaned with sulphuric acid and distilled water before each measurement. The contact on angle of the capillary tubes was expected to be close to zero as it is known to be for clean glass (Cras et al., 1999). Dry capillary tubes were then placed in a holder of the collected samples in plastic tubes at room temperature (20° C) for 1 h. The capillary rise was then measured with a hand-held caliper. The inner radius of the tubes was calibrated using distilled water, which has a well-known surface tension at a specified temperature. σxylem of the xylem was calculated according to Bikerman (1947) and Vanhanen et al. (2008):
where σ is surface tension (N m-1), ρ is water density (kg m-3), g is the gravitational acceleration (9.81 m s-2), r is the radius of capillary tube (m), and h is the height of capillary rise inside the tube (m). In reality, the density of xylem sap is slightly higher than the density of water; however, xylem sap is such a dilute solution (Borghetti et al., 1991) that we disregarded the effect of dissolved solutes on xylem sap density. Each σxylem measurement is a mean of two measurements from the same sample.
RWCstem was measured from a separate stem segment adjacent to the highest inoculation point. The fresh weight of a stem segment was weighed after removing the bark. The stem segment was then saturated in milli-Q water for 48 h, and the turgid weight was measured after extra water on the surface was gently wiped away with paper. Thereafter, dry weight was measured after samples were oven dried at 80°C for 72 h. The RWCstem was as follows:
where FW is fresh weight, DW is dry weight, and TW is turgid weight. Inoculated and wounded control trees were always measured simultaneously in pairs when Kstem, σxylem and RWCstem were measured.
Saturated stem water content was calculated as follows:
The spread and damage caused by E. polonica were assessed by using calipers to measure lengths and widths of necrotic lesions on the bark and xylem surface after removing the bark. These symptoms were investigated to determine whether the inoculation treatment was successful. The total lesion length (above and below the inoculation) and width in the bark were measured from the inner surface of the bark. A colored area in the xylem cross-section was considered a lesion, and the conical lesion area to total cross-sectional xylem area ratio was calculated based on the tangential width of the lesion, measured at the widest point, and the radial depth of the lesion. The necrotic lesions are commonly used indicators of pathogen colonization (Krokene and Solheim, 1998), although accurate estimates of pathogen spread are challenging with this and other similar commonly used methods (Linnakoski et al., 2017b).
Xylem sap for the chemical analyses was collected immediately after the surface tension sample was collected following the same protocol. The sap samples were stored in separate plastic tubes at –80°C. Samples from the same treatment and clones, collected during a week (two to three samples), were combined and analyzed as one sample. The sap was dried in a freeze-dryer and silylated with 0.5 mL of 20% TMSI-pyridine mixture [TMSI =1-(trimethylsilyl) imidazole]. The dry matter of the sap was analyzed for its chemical composition by gas chromatography-mass spectrometry (GC-MS) analysis by using a HP 6890 GC system equipped with a mass selective detector 5973 and HP-5 capillary column (30 m × 0.25 mm i.d.; 0.25 μm film thickness). Helium was used as carrier gas at a flow rate of 1.5 mL/min. Heptadecanoic acid (C:17), 1-chlorodecane, and m-erythrit were used as internal standards for resin acids, terpenes, and monosaccharides, respectively. The chromatographic conditions for resin acids were as follows: initial temperature 180°C, temperature rate 5°C/min, final temperature 300°C for 5 min, injector temperature 280°C and split ratio 1:20, MS-interface temperature 300°C, and ion source temperature 230°C. Mass spectra were obtained by electron impact (EI mode) ionization energy 70 eV. Monoterpenes and sesquiterpenes were analyzed directly from the liquid sap solution with GC-MS, using the following conditions: initial temperature 30°C, rate 10°C/min, hold time 5 min; rate 40°C/min, hold time 2 min; final temperature 230°C. For monosaccharides, the chromatographic conditions were as follows: initial temperature 110°C; rate of temperature increase 10°C/min; final temperature 320°C maintained for 14 min; injector temperature 260°C, and split ratio 1:20. The MS-interface temperature was 300°C and that for ion source was 230°C. All results were calculated by using an internal standard. Only samples from inoculated and wounded control trees were analyzed, as the sample amount was insufficient in the intact control trees with two intact control individuals per clone.
Student’s t-test and linear regression were used for statistical testing. Results were considered significant when P-value was less than or equal to 0.05. Statistical testing was carried out using R (v. 3.2.1, 2015, R Foundation for Statistical Computing, Vienna, Austria).
The inoculated trees had larger lesions than the wounded control trees (P < 0.001), accounting for 19% (clone 64) and 23% (clone 1510) of the xylem cross-sectional area at the inoculation site, with width approximately 1.6 cm in the bark of both clones, and total length 7.1 and 9.0 cm in the inner surface of the bark of clones 64 and 1510, respectively (see Figure S1 in Supplementary materials). In the wounded control trees, the lesions were only slightly larger than the inoculation wound (6 mm), covering 6% and 5% of the xylem cross-sectional area at the inoculation point, and measuring 1.0 and 0.9 cm in width and 1.4 and 1.3 cm in length in the bark of clones 64 and 1510, respectively. Additionally, declines in tree vigor and habitus were visible in inoculated trees, e.g. desiccation of shoots above the inoculation was visible in some of the inoculated trees.
The treatments began to diverge in terms of σxylem (Figures 2A, B) and Kstem (Figures 2C, D) at the first sampling in clone 1510 three weeks after inoculation, and subsequently in clone 64. The difference in Kstem was distinct in both clones towards the end of the experiment. However, the σxylem of inoculated trees returned to levels similar to the wounded control trees 13 weeks after the inoculation in clone 1510, whereas the σxylem of inoculated and wounded control trees in clone 64 differed also after 13 weeks. All σxylem observations differed from the surface tension of pure water (i.e. ~0.073 N m-1 at 20°C).
Figure 2 Responses over time following treatment, after visible symptoms of infection have occurred in xylem for σxylem of clones 64 (A) and 1510 (B); Kstem clones 64 (C) and 1510 (D); RWCstem clones 64 (E) and 1510 (F); and gcanopy calculated with the sapwood area-specific transpiration rate of clones 64 (G) and 1510 (H) in Endoconidiophora polonica–inoculated and wounded control Picea abies saplings. Inoculated trees are shown in black circles and wounded controls in gray squares. Error bars (with wide ticks in inoculated trees and narrow ticks in wounded controls) show the range between the minimum and maximum values (three observations), except in (G, H), where error bars represent standard deviations (n=3–5/treatment/clone), and no error bars are present when only 1-2 trees were measured. Both inoculated trees and wounded controls were sampled simultaneously, and the points are separated in the figure only for clarity sake. Times of irrigation (w) are shown beneath (A). Dashed lines (in A, B) indicate the surface tension of pure water at 20 °C.
The RWCstem describing the amount of wood desiccation was lower in inoculated saplings than in wounded controls in both clones throughout the experiment (P < 0.01; n = 8/treatment/clone) (Figures 2E, F). Neither gcanopy (Figures 2G, H) nor the Ψstem values differed between treatments (Supplementary Figure S2), indicating that there was no difference in stomatal conductance or water potential between the treatments.
Mean Kstem was significantly lower in inoculated trees than in wounded controls in both clones (P < 0.001; n = 9–11/treatment/clone) (Figure 3A), indicating more embolism formation in the inoculated saplings. Mean σxylem was also significantly lower in inoculated trees when all observations, i.e. both clones, were examined together (P < 0.05; n = 20/treatment), and in clone 64 (P < 0.05; n = 10–11/treatment) (Figure 3B). Statistical significance between the treatment groups was not tested within each individual measurement day due to the limited number of saplings and the destructive nature of the sampling. The mean σxylem was 0.066 N m-1 in the wounded control trees of both clones, and 0.060 and 0.062 N m-1 in inoculated clone 64 and 1510 trees, respectively (Figure 3B). σxylem values from clone 64 saplings ranged between 0.051 and 0.069 N m-1 (inoculated trees) and between 0.052 and 0.071 N m-1 (wounded control). However, only one observation from the wounded control trees was below 0.06 N m-1, whereas most of the observations in the inoculated trees were below 0.06 N m-1. In clone 1510 saplings, the σxylem values ranged between 0.048 and 0.069 N m-1 (inoculated trees) and between 0.061 and 0.072 N m-1 (wounded control). In intact control trees, the σxylem ranged between 0.065 and 0.066 N m-1 (clone 64) and between 0.062 and 0.068 N m-1 (clone 1510).
Figure 3 Mean Kstem (A) and σxylem (B) of clones 64 and 1510. Error bars show standard deviations. Observations include all sampled Endoconidiophora polonica inoculated and wounded control Picea abies saplings in the experiment (n = 9–11/treatment/clone). Statistically significant differences between treatments are found in Kstem (P < 0.001; n = 9–11/treatment/clone), and in σxylem when all trees are pooled together (P < 0.05; n = 20/treatment) and in clone 64 (P < 0.05; n = 10–11). Statistically significant differences between treatments are indicated by symbols: * P < 0.05; *** P < 0.001. Brackets in (A) indicate to statistically significant differences in clones 64 and 1510 separately, whereas in (B) the statistically significant difference between treatments is shown for clone 64 and when both clones are pooled together.
When excluding the data points where Kstem,saturated was smaller than Kstem, the inoculated trees demonstrated higher PLC than the wounded controls (14% vs. 6%) (see Supplementary Figure S3), albeit without statistical significance (P = 0.08; n = 9–12/treatment). However, Kstem,saturated (P < 0.001; n=13–15/treatment) and saturated stem water content (P < 0.001; n=17–18/treatment) were lower in the inoculated trees (see Supplementary Figure S4), indicating that not all conduits could be refilled with water in the inoculated samples, and therefore, the PLC calculations for the inoculated samples represent underestimates of the actual PLC, and the results based on PLC calculations should be treated with caution.
Kstem correlated positively with σxylem in the inoculated trees, whereas there was no correlation in the wounded control trees (Figure 4A). Additionally, RWCstem of the inoculated trees correlated with σxylem (Figure 4B). RWCstem remained constant in relation to Kstem in wounded controls compared with inoculated trees (Figure 4C). RWCstem for a given Ψstem was lower in inoculated trees (Figure 4D).
Figure 4 Kstem (A) (n=20/treatment) and RWCstem (B) (n=16/treatment) as a function of σxylem; RWCstem in relation to Kstem (C) (n=16/treatment) and Ψstem (D) (n=16/treatment) in all measured Endoconidiophora polonica inoculated and wounded control Picea abies saplings. Inoculated trees are shown in black (clone 64) and light brown (clone 1510) triangles, and the wounded controls in dark grey (clone 64) and light grey (clone 1510) star symbols in the figure. The linear regression equations of σxylem and Kstem (A) y=-0.288+7.665x, (P < 0.01); and σxylem and RWCstem (B) y=0.45 + 4.24x, (P < 0.05).
In xylem sap, the total concentration of compounds was larger in inoculated trees than in the wounded controls in only clone 64 (Table 2). Increased concentrations of quinic acid and alkylphenol (P < 0.05) were found in xylem sap of inoculated trees in clone 1510. Quinic acid concentration increased in xylem sap, especially in the last measurements after recovery in Kstem and σxylem in inoculated trees in clone 1510 (see Supplementary Figure S5). In addition, myo-inositol and sucrose concentrations were significantly higher in inoculated trees (P < 0.05) when both clones were examined together. Kstem of inoculated trees correlated negatively with myo-inositol concentrations in the xylem sap (see Supplementary Figure S5A). Myo-inositol of xylem sap correlated negatively also with σxylem (see Supplementary Figure S5B). In addition, the inositol isomer correlated negatively with σxylem (R2 = 0.41; P < 0.01) and Kstem (R2 = 0.58; P < 0.05) (not shown).
Table 2 Compounds analyzed from the extracted xylem sap from Endoconidiophora polonica inoculated and wounded control Picea abies saplings.
Our preliminary study with clonal Norway spruce saplings showed that E. polonica inoculation resulted in a significant decrease in σxylem, and hampered water transport in inoculated trees, thus confirming earlier studies (Horntvedt et al., 1983; Christiansen and Fjone, 1993; Kirisits and Offenthaler, 2002; Sallé et al., 2005). The observed significant decrease in σxylem in the inoculated trees has been previously hypothesized to affect tree water transport during pathogenic infections (Tyree and Sperry, 1989; Christiansen and Fjone, 1993; Kuroda, 2005; Lachenbruch and Zhao, 2019). Our results indicate that inoculated trees experienced hampered water transport locally, which was not observable in whole-tree-level water use. The measured lesions in the bark and xylem indicated that E. polonica infection caused visible reactions in the inoculated trees (Lahr and Krokene, 2013) before measurements of both Kstem and σxylem were conducted. Additionally, desiccation of shoots above the inoculation was visible in some of the inoculated trees. E. polonica inoculation have been reported to result in canopy desiccation after losing some of the conducting tissue in xylem of P. abies in other studies (Horntvedt et al., 1983; Christiansen and Fjone, 1993; Kirisits and Offenthaler, 2002), and in other tree species (Kuroda, 2005). The inoculation treatment in the present study was considered a high dosage for the saplings (Krokene et al., 1999).
E. polonica infection resulted in changes in tree Kstem, σxylem and RWCstem locally at the site of inoculation in the stem, while there was no clear difference in gcanopy and Ψstem. The decrease in local Kstem without a noticeable decrease in gcanopy may be explained by the tree hydraulic architecture. Most of the resistance to water transport is in the distal parts (i.e. needles and fine roots) and in the soil-to-root interface (Tyree and Zimmermann, 2002). Therefore, a reduction in local Kstem would have to be drastic in order to cause a noticeable effect at the whole-plant level. A noticeable effect on water transport in E. polonica-infected mature P. abies trees has been observed only after a large decrease in Kstem (Horntvedt et al., 1983; Kirisits and Offenthaler, 2002). In the present study, the stomata seemed to respond to changes in Ψstem rather than to the decrease in Kstem (see also Hölttä et al., 2012) because there was no difference in gcanopy or water potential between the treatments.
We found a decrease in RWCstem approximately 10 cm above the inoculation points. The decrease in Kstem and RWCstem both indicate substantial embolism formation in the xylem (Tyree et al., 1994; Domec et al., 2004; Rosner et al., 2019). The higher PLC in inoculated trees also indicates a higher amount of embolism, which also relates closely to a decrease in RWCstem (Rosner et al., 2019). However, the PLC results should be treated with caution since not all embolized conduits are expected to refill in conifers due to e.g. tyloses formation or aspirated pits (Flynn, 2007; Hietz et al., 2008). The saturated stem water content was also consistently smaller in the inoculated trees in our study, indicating that there are challenges in the refilling of xylem conduits and that the PLC was underestimated in the inoculated samples. Thus, if the loss of hydraulic conductivity was caused only by mechanical blockage because of the fungus or host tree defense responses, we would not expect the saturation after the initial hydraulic conductivity measurement to restore the hydraulic conductivity. RWCstem has been reported to decrease above and below the E. polonica inoculations also in the sapwood of mature P. abies trees (Horntvedt et al., 1983). Kuroda (2005) found that the sapwood of Picea jezoensis desiccated more than 20 cm above the inoculation point preceding the hyphae of E. polonica. However, the spread of fungus hyphae was not examined in the present study.
The Kstem and RWCstem correlated with σxylem in inoculated trees, but not in wounded control trees, and the RWCstem and Kstem were at distinct levels between the treatments, although the Ψstem values were similar. This is consistent with the theoretical prediction that a decrease in σxylem will decrease RWCstem and Kstem at a given Ψstem (as depicted in Figure 1), i.e. that embolism and desiccation of conduits will start already in milder water stress due to decreased σxylem. Lowering the σxylem from pure water (0.073 N m-1) to 0.055 N m-1 would lower the P50, the Ψstem where 50% of the conductivity in water transport is lost because of embolism, from -3.5 (Bartlett et al., 2016, González-Muñoz et al., 2018) to -2.6 MPa in P. abies (as depicted in Figure 1 and Cochard et al., 2009). E. polonica has previously been hypothesized to affect the σxylem and disable its function by causing embolism formation (Christiansen and Fjone, 1993; Kuroda, 2005). Recently, also other studies have reported that σxylem decreased below that of pure water. Losso et al. (2017) described seasonal fluctuations in σxylem ranging between 0.054 and 0.067 N m-1 in Norway spruce. Moreover, Christensen-Dalsgaard et al. (2011) and Schenk et al. (2017) measured a σxylem lower than that of pure water in angiosperm species. The majority of our σxylem results, in both inoculated and control trees, are similar to those of Losso et al. (2017), but in our study the range of values was slightly higher. Hence, also in our study the σxylem sap differs clearly from that of pure water, which increases the vulnerability of the xylem to embolism formation.
We were unable to identify any specific surfactant compounds that caused the lowered σxylem. We expected to find negative correlations between σxylem and specific identified surfactant compounds in xylem sap, but found only a significant negative correlation with myo-inositol. Myo-inositol has been noted to increase—not decrease—the surface tension in a solution in earlier studies (Kaushik and Bhat, 1998), and therefore, another mechanism must be responsible for the negative correlations of Kstem and σxylem with myo-inositol concentrations. Myo-inositol is known to be connected to several metabolic processes such as growth, signaling, stress responses, and osmoregulation (Loewus and Murthy, 2000; Meijer and Munnik, 2003; Valluru and Van den Ende, 2011). Thus, rising abiotic or biotic stress may increase the myo-inositol concentrations in xylem sap. Increased quinic acid concentrations, such as those found in inoculated clone 1510 saplings, have previously been observed in grapevines during a bacterial pathogen infection (Wallis and Chen, 2012). Increased sucrose concentration in xylem sap is probably not lowering the σxylem in our study, as sucrose, as well as glucose, acts contrary to surfactants (Matubayasi and Nishiyama, 2006). Malic acid is known to be a moderate surfactant (Hyvärinen et al., 2006), but its concentrations were not different between treatments, nor was there a clear relation with σxylem. The monoterpene pinene, another candidate compound and involved in local tree defense responses (Baier et al., 2002), decreases surface tension, but the effect is low because of its limited solubility (DeWitt and Makens, 1932). However, pinene or other compounds of resin were not detected in the xylem sap (e.g. Nagy et al., 2000).
It is also possible that the surface tension reduction is not caused by a single compound, but rather by the properties of the solution mixture. The surface tension of a solution may decrease with the increasing solute concentration or with changes in solute composition in a given mixture of compounds, as reported for fatty acids and model humic-like substances in mixtures with inorganic salts (Prisle et al., 2010; Kristensen et al., 2014). Surface tension of a solution is always a function of the solution composition, and thus, the contribution of single compounds is challenging to assess (e.g. Aumann et al., 2010; Kristensen et al., 2014). Therefore, the decrease in σxylem also in control trees may result from varying amounts of surfactants either in lower concentrations or in a less efficient overall mixture of surfactants in the xylem sap. The different behavior of σxylem in inoculated and wounded controls may thus result from additional compounds affecting the solution mixture and the σxylem.
The decrease in Kstem and RWCstem seemed to be permanent, while σxylem recovered, at least in clone 1510. This indicates that the embolisms were either not refilled, or other reasons, such as mechanical blockage, prevented the recovery in Kstem. Loss in Kstem may also be initiated from the formation of tylosoids, pit aspiration, embolism, and/or release of epithelial cell content in the apoplast (Yazaki et al., 2017). However, as our sampling began after the visible symptoms in xylem had occurred, we can only speculate about the initial reasons for decreased Kstem in the early stage of infection. E. polonica has been shown to proceed deeper in the sapwood through the ray parenchyma cells (Franceschi et al., 1998; Kuroda, 2005). The ray parenchyma spanning across the stem, from inner bark to stem pith (Plavcová and Jansen, 2015), provide a route for the spread of fungus hyphae (Franceschi et al., 1998). The disabling of conducting tissue can also be the result of Norway spruce defense reactions, where accumulating compounds block the invaded tissue (Ballard et al., 1982; Christiansen, 1985; Yamada, 1992). Lesions, induced by the fungal infection and host tree responses, hinder the spread of E. polonica with structural barriers and more effective resin compounds (Woodward and Pearce, 1988; Solheim, 1991; Krokene et al., 1999; Viiri et al., 2001). The induced structural barriers may, in turn, affect the water-conducting properties of the xylem tissue, apart from the embolism that recovered after water saturation (as shown above with PLC and RWC measurements).
In conclusion, a decrease in xylem sap surface tension, embolism formation, and a decrease in xylem hydraulic conductivity were observed in E. polonica-inoculated trees, as we hypothesized. However, as we started our sampling at the later stage of infection, we are unable to pinpoint the chain of events between the host and the pathogen that led to the correlation between xylem sap surface tension and a decrease in xylem hydraulic conductivity. Xylem sap surface tension may have decreased first, which was followed by embolism formation and a decrease in hydraulic conductivity. Alternatively, the host tree may first have induced cellular responses to infection or the pathogen may have first inflicted mechanical damage to e.g. the resin ducts and/or parenchyma cells, which could have released part of their content to the xylem sap and lowered the surface tension. A decrease in xylem sap surface tension would be important for the tree vulnerability to embolism whether it occurs before or after an initial decrease in hydraulic conductivity, as shown by previous studies (Sperry and Tyree, 1988; Cochard et al., 2009; Hölttä et al., 2012). Our results also indicated that part of the desiccated xylem conduits recovered after refilling with water and that not only mechanical blockage caused the decline in stem hydraulic conductivity. Further investigations of pathogen-host interactions are warranted, as the mechanisms and compounds causing the decreased xylem sap surface tension were not identified. Especially, the limited number of sampled trees prevented us from detecting the early stages of disease development. Furthermore, studies with mature trees are needed to investigate our results in natural conditions, as our study was conducted with saplings under greenhouse conditions. For example, the magnitude and efficiency of tree responses may differ between the bark beetle-vectored E. polonica infection in natural conditions and sole inoculation of E. polonica (Lahr and Krokene, 2013).
All datasets generated for this study are included in the article/Supplementary Material.
TH, RK, JB, YS, and TP conceived the study. TP, KR, AV, and RL were responsible for establishing the study setup. TP measured the tree water relations, measured infection lesions with KR, and prepared the chemical sampling with KR, TJ, and TL. RL and RK helped to interpret the results from the tree pathology perspective. NP and JL were responsible for the interpretation of the surface tension results. TJ and TL planned and conducted the chemical analyses of xylem sap. All authors contributed to the article and approved the submitted version.
This work was supported by the Academy of Finland grant nos. 324014, 272041, and 305763, the Finnish Cultural Foundation grant no. 00180821, and the Japan Society for the Promotion of Science (JSPS) KAKENHI (grant no. 26·04395). NLP and JJL gratefully acknowledge funding from the European Research Council (ERC) under the European Union’s Horizon 2020 research and innovation programme, Project SURFACE (grant agreement no. 717022), and the Academy of Finland (grant nos. 308238 and 314175).
The authors declare that the research was conducted in the absence of any commercial or financial relationships that could be construed as a potential conflict of interest.
The Supplementary Material for this article can be found online at: https://www.frontiersin.org/articles/10.3389/fpls.2020.01090/full#supplementary-material
Aumann, E., Hildemann, L. M., Tabazadeh, A. (2010). Measuring and modeling the composition and temperature-dependence of surface tension for organic solutions. Atmos. Environ. 44, 329–337. doi: 10.1016/j.atmosenv.2009.10.033
Baier, P., Führer, E., Kirisits, T., Rosner, S. (2002). Defence reactions of Norway spruce against bark beetles and the associated fungus Ceratocystis polonica in secondary pure and mixed species stands. For. Ecol. Manage. 159 (1-2), 73–86. doi: 10.1016/S0378-1127(01)00711-3
Ballard, R., Walsh, M., Cole, W. (1982). Blue-stain fungi in xylem of lodgepole pine: a light-microscope study on extent of hyphal distribution. Can. J. Bot. 60, 2334–2341. doi: 10.1139/b82-285
Bartlett, M. K., Klein, T., Jansen, S., Choat, B., Sack, L. (2016). The correlations and sequence of plant stomatal, hydraulic, and wilting responses to drought. Proc. Natl. Acad. Sci. 113 (46), 13098–13103. doi: 10.1073/pnas.1604088113
Bikerman, J. J. (1947). Surface Chemistry for Industrial Research (New York: Academic Press Inc., Publishers).
Borghetti, M., Edwards, W. R. N., Grace, J., Jarvis, P. G., Raschi, A. (1991). The refilling of embolized xylem in Pinus sylvestris L. Plant Cell Environ. 14, 357–369. doi: 10.1111/j.1365-3040.1991.tb00944.x
Brodribb, T. J., Cochard, H. (2009). Hydraulic failure defines the recovery and point of death in water-stressed conifers. Plant Physiol. 149, 575–584. doi: 10.1104/pp.108.129783
Choat, B., Jansen, S., Zwieniecki, M. A., Smets, E., Holbrook, N. M. (2004). Changes in pit membrane porosity due to deflection and stretching: the role of vestured pits. J. Exp. Bot. 55, 1569–1575. doi: 10.1093/jxb/erh173
Christensen-Dalsgaard, K. K., Tyree, M. T., Mussone, P. G. (2011). Surface tension phenomena in the xylem sap of three diffuse porous temperate tree species. Tree Physiol. 31, 361–368. doi: 10.1093/treephys/tpr018
Christiansen, E., Fjone, G. (1993). Pruning enhances the susceptibility of Picea abies to infection by the bark beetle-transmitted blue-stain fungus, Ophiostoma polonicum. Scand. J. For. Res. 8, 235–245. doi: 10.1080/02827589309382773
Christiansen, E. (1985). Ceratocystis polonica inoculated in Norway spruce: Blue-staining in relation to inoculum density, resinosis and tree growth. Eur. J. For. Pathol. 15, 160–167. doi: 10.1111/j.1439-0329.1985.tb00880.x
Cochard, H., Hölttä, T., Herbette, S., Delzon, S., Mencuccini, M. (2009). New insights into the mechanisms of water-stress-induced cavitation in conifers. Plant Physiol. 151, 949–954. doi: 10.1104/pp.109.138305
Cras, J. J., Rowe-Taitt, C. A., Nivens, D. A., Ligler, F. S. (1999). Comparison of chemical cleaning methods of glass in preparation for silanization. Biosens. Bioelectron. 14, 683–688. doi: 10.1016/S0956-5663(99)00043-3
Crombie, D. S., Milburn, J. A., Hipkins, M. F. (1985). Maximum sustainable xylem sap tensions in Rhododendron and other species. Planta 163, 27–33. doi: 10.1007/BF00395893
Delzon, S., Douthe, C., Sala, A., Cochard, H. (2010). Mechanism of water-stress induced cavitation in conifers: bordered pit structure and function support the hypothesis of seal capillary-seeding. Plant Cell Environ. 33, 2101–2111. doi: 10.1111/j.1365-3040.2010.02208.x
DeWitt, C. C., Makens, R. F. (1932). The surface relations of the components of pine oil and of potassium ethyl xanthate. II1. J. Am. Chem. Soc. 54, 455–464. doi: 10.1021/ja01341a007
Domec, J. C., Warren, J. M., Meinzer, F. C., Brooks, J. R., Coulombe, R. (2004). Native root xylem embolism and stomatal closure in stands of Douglas-fir and ponderosa pine: mitigation by hydraulic redistribution. Oecologia 141, 7–16. doi: 10.1007/s00442-004-1621-4
Domec, J. (2011). Let’s not forget the critical role of surface tension in xylem water relations. Tree Physiol. 31, 359–360. doi: 10.1093/treephys/tpr039
Flynn, K. A. (2007). A review of the permeability, fluid flow, and anatomy of spruce (Picea spp.). Wood Fiber Sci. 27, 278–284.
Franceschi, V., Krekling, T., Berryman, A., Christiansen, E. (1998). Specialized phloem parenchyma cells in Norway spruce (Pinaceae) bark are an important site of defense reactions. Am. J. Bot. 85, 601. doi: 10.2307/2446529
Giordano, L., Garbelotto, M., Nicolotti, G., Gonthier, P. (2013). Characterization of fungal communities associated with the bark beetle Ips typographus varies depending on detection method, location, and beetle population levels. Mycol. Prog. 12, 127–140. doi: 10.1007/s11557-012-0822-1
González-Muñoz, N., Sterck, F., Torres-Ruiz, J. M., Petit, G., Cochard, H., von Arx, G., et al. (2018). Quantifying in situ phenotypic variability in the hydraulic properties of four tree species across their distribution range in Europe. PloS One 13, e0196075. doi: 10.1371/journal.pone.0196075
Hölttä, T., Juurola, E., Lindfors, L., Porcar-Castell, A. (2012). Cavitation induced by a surfactant leads to a transient release of water stress and subsequent ‘run away’embolism in Scots pine (Pinus sylvestris) saplings. J. Exp. Bot. 63, 1057–1067. doi: 10.1093/jxb/err349
Hietz, P., Rosner, S., Sorz, J., Mayr, S. (2008). Comparison of methods to quantify loss of hydraulic conductivity in Norway spruce. Ann. For. Sci. 65, 1. doi: 10.1051/forest:2008023
Horntvedt, R., Christiansen, E., Solheim, H., Wang, S. (1983). Artificial inoculation with Ips typographus-associated blue stain fungi can kill healthy Norway spruce trees. Meddelelser fra Skogforsk. 38, 1–20.
Hossain, M., Veneklaas, E. J., Hardy, G. E. S. J., Poot, P. (2018). Tree host–pathogen interactions as influenced by drought timing: linking physiological performance, biochemical defence and disease severity. Tree Physiol. 39, 6–18. doi: 10.1093/treephys/tpy113
Hyvärinen, A. P., Lihavainen, H., Gaman, A., Vairila, L., Ojala, H., Kulmala, M., et al. (2006). Surface tensions and densities of oxalic, malonic, succinic, maleic, malic, and cis-pinonic acids. J. Chem. Eng. Data 51, 255–260. doi: 10.1021/je050366x
Jansen, S., Lamy, J. B., Burlett, R., Cochard, H., Gasson, P., Delzon, S. (2012). Plasmodesmatal pores in the torus of bordered pit membranes affect cavitation resistance of conifer xylem. Plant Cell Environ. 35, 1109–1120. doi: 10.1111/j.1365-3040.2011.02476.x
Kaushik, J. K., Bhat, R. (1998). Thermal stability of proteins in aqueous polyol solutions: role of the surface tension of water in the stabilizing effect of polyols. J. Phys. Chem. B 102, 7058–7066. doi: 10.1021/jp981119l
Kautz, M., Meddens, A. J., Hall, R. J., Arneth, A. (2017). Biotic disturbances in Northern Hemisphere forests–a synthesis of recent data, uncertainties and implications for forest monitoring and modelling. Global Ecol. Biogeogr. 26, 533–552. doi: 10.1111/geb.12558
Kirisits, T., Offenthaler, I. (2002). Xylem sap flow of Norway spruce after inoculation with the blue-stain fungus Ceratocystis polonica. Plant Pathol. 51, 359–364. doi: 10.1046/j.1365-3059.2002.00722.x
Kristensen, T. B., Prisle, N. L., Bilde, M. (2014). Cloud droplet activation of mixed model HULIS and NaCl particles: Experimental results and κ-Köhler theory. Atmos. Res. 137, 167–175. doi: 10.1016/j.atmosres.2013.09.017
Krokene, P., Solheim, H. (1998). Pathogenicity of four blue-stain fungi associated with aggressive and nonaggressive bark beetles. Phytopathology 88, 39–44. doi: 10.1094/PHYTO.1998.88.1.39
Krokene, P., Christiansen, E., Solheim, H., Franceschi, V. R., Berryman, A. A. (1999). Induced resistance to pathogenic fungi in Norway spruce. Plant Physiol. 121, 565–570. doi: 10.1104/pp.121.2.565
Kuroda, K. (2005). Xylem dysfunction in Yezo spruce (Picea jezoensis) after inoculation with the blue-stain fungus Ceratocystis polonica. For. Pathol. 35, 346–358. doi: 10.1111/j.1439-0329.2005.00424.x
Lachenbruch, B., Zhao, J. P. (2019). Effects of Phloem on Canopy Dieback, Tested with Manipulations and a Canker Pathogen in the Corylus avellana/Anisogramma anomala Host/Pathogen System. Tree Physiol. 39, 1086–1098. doi: 10.1093/treephys/tpz027
Lahr, E. C., Krokene, P. (2013). Conifer stored resources and resistance to a fungus associated with the spruce bark beetle Ips typographus. PloS One 8, e72405. doi: 10.1371/journal.pone.0072405
Linnakoski, R., de Beer, Z. W., Niemelä, P., Wingfield, M. J. (2012). Associations of conifer-infesting bark beetles and fungi in Fennoscandia. Insects 3, 200–227. doi: 10.3390/insects3010200
Linnakoski, R., Mahilainen, S., Harrington, A., Vanhanen, H., Eriksson, M., Mehtätalo, L., et al. (2016). Seasonal succession of fungi associated with Ips typographus beetles and their phoretic mites in an outbreak region of Finland. PloS One 11, e0155622. doi: 10.1371/journal.pone.0155622
Linnakoski, R., Sugano, J., Junttila, S., Pulkkinen, P., Asiegbu, F. O., Forbes, K. M. (2017a). Effects of water availability on a forestry pathosystem: fungal strain-specific variation in disease severity. Sci. Rep. 7, 13501. doi: 10.1038/s41598-017-13512-y
Linnakoski, R., Forbes, K. M., Wingfield, M. J., Pulkkinen, P., Asiegbu, F. O. (2017b). Testing projected climate change conditions on the Endoconidiophora polonica/Norway spruce pathosystem shows fungal strain specific effects. Front. Plant Sci. 8, 883. doi: 10.3389/fpls.2017.00883
Loewus, F. A., Murthy, P. P. (2000). Myo-Inositol metabolism in plants. Plant Sci. 150, 1–19. doi: 10.1016/S0168-9452(99)00150-8
Losso, A., Beikircher, B., Damon, B., Kikuta, S., Schmid, P., Mayr, S. (2017). Xylem Sap Surface Tension May Be Crucial for Hydraulic Safety. Plant Physiol. 175, 1135–1143. doi: 10.1104/pp.17.01053
Matthews, B., Netherer, S., Katzensteiner, K., Pennerstorfer, J., Blackwell, E., Henschke, P., et al. (2018). Transpiration deficits increase host susceptibility to bark beetle attack: Experimental observations and practical outcomes for Ips typographus hazard assessment. Agric. For. Meteorol. 263, 69–89. doi: 10.1016/j.agrformet.2018.08.004
Matubayasi, N., Nishiyama, A. (2006). Thermodynamic quantities of surface formation of aqueous electrolyte solutions VI. Comparison with typical nonelectrolytes, sucrose and glucose. J. Colloid Interface Sci. 298, 910–913. doi: 10.1016/j.jcis.2006.01.008
McDowell, N., Pockman, W. T., Allen, C. D., Breshears, D. D., Cobb, N., Kolb, T., et al. (2008). Mechanisms of plant survival and mortality during drought: why do some plants survive while others succumb to drought? New Phytol. 178, 719–739. doi: 10.1111/j.1469-8137.2008.02436.x
Meijer, H. J., Munnik, T. (2003). Phospholipid-based signaling in plants. Annu. Rev. Plant Biol. 54, 265–306. doi: 10.1146/annurev.arplant.54.031902.134748
Nagy, N. E., Franceschi, V. R., Solheim, H., Krekling, T., Christiansen, E. (2000). Wound-induced traumatic resin duct development in stems of Norway spruce (Pinaceae): anatomy and cytochemical traits. Am. J. Bot. 87 (3), 302–313. doi: 10.2307/2656626
Netherer, S., Matthews, B., Katzensteiner, K., Blackwell, E., Henschke, P., Hietz, P., et al. (2015). Do water-limiting conditions predispose Norway spruce to bark beetle attack? New Phytol. 205, 1128–1141. doi: 10.1111/nph.13166
Pammenter, N. V., Van der Willigen, C. (1998). A mathematical and statistical analysis of the curves illustrating vulnerability of xylem to cavitation. Tree Physiol. 18 (8-9), 589–593. doi: 10.1093/treephys/18.8-9.589
Perry, T. J. (1991). A synopsis of the taxonomic revisions in the genus Ceratocystis including a review of blue-staining species associated with Dendroctonus bark beetles. Gen. Tech. Rep. SO-86 Vol. 16 (New Orleans, LA: US Dept of Agriculture, Forest Service, Southern Forest Experiment Station), 86.
Plavcová, L., Jansen, S. (2015). “The role of xylem parenchyma in the storage and utilization of nonstructural carbohydrates,” in Functional and ecological xylem anatomy (Cham: Springer), 209–234.
Prisle, N. L., Raatikainen, T., Laaksonen, A., Bilde, M. (2010). Surfactants in cloud droplet activation: mixed organic-inorganic particles. Atmos. Chem. Phys. 10, 5663–5683. doi: 10.5194/acp-10-5663-2010
Repe, A., Bojović, S., Jurc, M. (2015). Pathogenicity of ophiostomatoid fungi on Picea abies in Slovenia. For. Pathol. 45, 290–297. doi: 10.1111/efp.12170
Rosner, S., Heinze, B., Savi, T., Dalla-Salda, G. (2019). Prediction of hydraulic conductivity loss from relative water loss: new insights into water storage of tree stems and branches. Physiol. Plant 165, 843–854. doi: 10.1111/ppl.12790
Sallé, A., Monclus, R., Yart, A., Garcia, J., Romary, P., Lieutier, F. (2005). Fungal flora associated with Ips typographus: frequency, virulence, and ability to stimulate the host defence reaction in relation to insect population levels. Can. J. For. Res. 35 (2), 365–373. doi: 10.1139/x04-186
Schenk, H. J., Steppe, K., Jansen, S. (2015). Nanobubbles: a new paradigm for air-seeding in xylem. Trends Plant Sci. 20, 199–205. doi: 10.1016/j.tplants.2015.01.008
Schenk, H. J., Espino, S., Romo, D. M., Nima, N., Do, A. Y., Michaud, J. M., et al. (2017). Xylem surfactants introduce a new element to the cohesion-tension theory. Plant Physiol. 173, 1177–1196. doi: 10.1104/pp.16.01039
Solheim, H. (1991). Oxygen deficiency and spruce resin inhibition of growth of blue stain fungi associated with Ips typographus. Mycol. Res. 95, 1387–1392. doi: 10.1016/S0953-7562(09)80390-0
Sperry, J. S., Tyree, M. T. (1988). Mechanism of water stress-induced xylem embolism. Plant Physiol. 88, 581–587. doi: 10.1104/pp.88.3.581
Sperry, J. S., Donnelly, J. R., Tyree, M. T. (1988). A method for measuring hydraulic conductivity and embolism in xylem. Plant Cell Environ. 11, 35–40. doi: 10.1111/j.1365-3040.1988.tb01774.x
Turner, N. C. (1988). Measurement of plant water status by the pressure chamber technique. Irrigation Sci. 9 (4), 289–308. doi: 10.1007/BF00296704
Tyree, M. T., Sperry, J. S. (1989). Vulnerability of xylem to cavitation and embolism. Annu. Rev. Plant Biol. 40, 19–36. doi: 10.1146/annurev.pp.40.060189.000315
Tyree, M. T., Yang, S. (1990). Water-storage capacity of Thuja, Tsuga and Acer stems measured by dehydration isotherms. Planta 182, 420–426. doi: 10.1007/BF02411394
Tyree, M. T., Zimmermann, M. H. (2002). “Hydraulic architecture of whole plants and plant performance,” in Xylem structure and the ascent of sap. Eds. Tyree, M. T., Zimmermann, M. H. (Berlin, Germany: Springer Berlin Heidelberg), 175–214.
Tyree, M. T., Davis, S. D., Cochard, H. (1994). Biophysical perspectives of xylem evolution: is there a tradeoff of hydraulic efficiency for vulnerability to dysfunction? IAWA J. 15, 335–360. doi: 10.1163/22941932-90001369
Valluru, R., Van den Ende, W. (2011). Myo-inositol and beyond–emerging networks under stress. Plant Sci. 181, 387–400. doi: 10.1016/j.plantsci.2011.07.009
Vanhanen, J., Hyvärinen, A. P., Anttila, T., Raatikainen, T., Viisanen, Y., Lihavainen, H. (2008). Ternary solution of sodium chloride, succinic acid and water; surface tension and its influence on cloud droplet activation. Atmos. Chem. Phys. 8, 4595–4604. doi: 10.5194/acp-8-4595-2008
Viiri, H., Annila, E., Kitunen, V., Niemelä, P. (2001). Induced responses in stilbenes and terpenes in fertilized Norway spruce after inoculation with blue-stain fungus, Ceratocystis polonica. Trees 15, 112–122. doi: 10.1007/s004680000082
Wallis, C. M., Chen, J. (2012). Grapevine phenolic compounds in xylem sap and tissues are significantly altered during infection by Xylella fastidiosa. Phytopathology 102, 816–826. doi: 10.1094/PHYTO-04-12-0074-R
Woodward, S., Pearce, R. B. (1988). Wound-associated responses in Sitka spruce root bark challenged with Phaeolus schweinitzii. Physiol. Mol. Plant Pathol. 33, 151–162. doi: 10.1016/0885-5765(88)90050-1
Yamada, T. (1992). “Biochemistry of gymnosperm xylem responses to fungal invasion,” in Defense Mechanisms of Woody Plants Against Fungi. Eds. Blanchette, R. A., Biggs, A. R. (Berlin: Springer-Verlag), 147–164.
Keywords: embolism, Endoconidiophora polonica, hydraulic conductivity, Picea abies (Norway spruce), plant-pathogen interactions, surface tension, xylem transport, xylem sap composition
Citation: Paljakka T, Rissanen K, Vanhatalo A, Salmon Y, Jyske T, Prisle NL, Linnakoski R, Lin JJ, Laakso T, Kasanen R, Bäck J and Hölttä T (2020) Is Decreased Xylem Sap Surface Tension Associated With Embolism and Loss of Xylem Hydraulic Conductivity in Pathogen-Infected Norway Spruce Saplings? Front. Plant Sci. 11:1090. doi: 10.3389/fpls.2020.01090
Received: 02 October 2019; Accepted: 02 July 2020;
Published: 16 July 2020.
Edited by:
Sean Michael Gleason, United States Department of Agriculture, United StatesReviewed by:
Paal Krokene, Norwegian Institute of Bioeconomy Research (NIBIO), NorwayCopyright © 2020 Paljakka, Rissanen, Vanhatalo, Salmon, Jyske, Prisle, Linnakoski, Lin, Laakso, Kasanen, Bäck and Hölttä. This is an open-access article distributed under the terms of the Creative Commons Attribution License (CC BY). The use, distribution or reproduction in other forums is permitted, provided the original author(s) and the copyright owner(s) are credited and that the original publication in this journal is cited, in accordance with accepted academic practice. No use, distribution or reproduction is permitted which does not comply with these terms.
*Correspondence: Teemu Paljakka, dGVlbXUucGFsamFra2FAaGVsc2lua2kuZmk=
†Present address: Kaisa Rissanen, Center for forest research, Département des sciences biologiques, Université du Québec à Montréal, Montréal, Canada
Disclaimer: All claims expressed in this article are solely those of the authors and do not necessarily represent those of their affiliated organizations, or those of the publisher, the editors and the reviewers. Any product that may be evaluated in this article or claim that may be made by its manufacturer is not guaranteed or endorsed by the publisher.
Research integrity at Frontiers
Learn more about the work of our research integrity team to safeguard the quality of each article we publish.