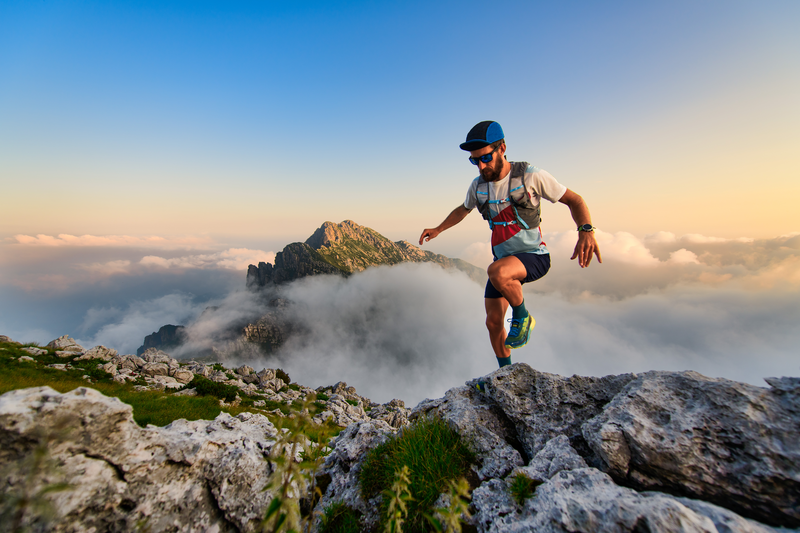
94% of researchers rate our articles as excellent or good
Learn more about the work of our research integrity team to safeguard the quality of each article we publish.
Find out more
MINI REVIEW article
Front. Plant Sci. , 20 March 2020
Sec. Plant Metabolism and Chemodiversity
Volume 11 - 2020 | https://doi.org/10.3389/fpls.2020.00305
This article is part of the Research Topic Responses of Tea Plants to Climate Change: From Molecules to Ecosystems View all 15 articles
Rising atmospheric carbon dioxide, an important driver of climate change, has multifarious effects on crop yields and quality. Despite tremendous progress in understanding the mechanisms of plant responses to elevated CO2, only a few studies have examined the CO2-enrichment effects on tea plants. Tea [Camellia sinensis (L.)], a non-deciduous woody perennial plant, operates massive physiologic, metabolic and transcriptional reprogramming to adapt to increasing CO2. Tea leaves elevate photosynthesis when grown at CO2–enriched environment which is attributed to increased maximum carboxylation rate of RuBisCO and maximum rates of RuBP regeneration. Elevated CO2-induced photosynthesis enhances the energy demand which triggers respiration. Stimulation of photosynthesis and respiration by elevated CO2 promotes biomass production. Moreover, elevated CO2 increases total carbon content, but it decreases total nitrogen content, leading to an increased ratio of carbon to nitrogen in tea leaves. Elevated CO2 alters the tea quality by differentially influencing the concentrations and biosynthetic gene expression of tea polyphenols, free amino acids, catechins, theanine, and caffeine. Signaling molecules salicylic acid and nitric oxide function in a hierarchy to mediate the elevated CO2-induced flavonoid biosynthesis in tea leaves. Despite enhanced synthesis of defense compounds, tea plant defense to some insects and pathogens is compromised under elevated CO2. Here we review the physiological and metabolic responses of tea plants to elevated CO2. In addition, the potential impacts of elevated CO2 on tea yield and defense responses are discussed. We also show research gaps and critical research areas relating to elevated CO2 and tea quality for future study.
Increasing atmospheric CO2 is the most prominent driver of global warming. At present, global atmospheric CO2 concentration is 407.65 ppm (recorded in September 20191), which was only 270 ppm during the preindustrial era. Over the last 200 years, such an unparalleled increase in atmospheric CO2 occurred due to massive human anthropogenic activities such as deforestation, fossil-based fuel combustion, rapid urbanization and industrialization (Ahuja et al., 2010). The concentration of atmospheric CO2 is still increasing, and it will possibly reach 800 ppm by the end of the 21st century (IPCC, 2014). It is inferred that with increasing CO2, the adversities of extreme climate events such as heatwave, drought, and frost will be increasing, which will variously affect tea yield, quality and ecosystem (Li et al., 2018, 2019a; Ahmed et al., 2019). Nonetheless, tea plantations play a significant role in CO2 sequestration and thus tea gardens can be useful in mitigating global warming (Pramanik and Phukan, 2020).
Tea is the most popular beverage consumed across the seven continents (Macfarlane and Macfarlane, 2004), even though its cultivation is limited to Asia and Africa. Green tea is basically manufactured from the species Camellia sinensis (L.) Kuntze through rapid roasting of fresh leaves to avoid oxidation (Han et al., 2016). The popularity of green tea is increasing day by day, not only for its pleasant flavor but also for numerous health benefits such as anti-inflammatory, anti-cancer, anti-obesity, and anti-allergic effects on humans (Kim et al., 2009; Siamwala et al., 2013; Mancini et al., 2017). Due to the increasing demand for tea, areas belonging to tea cultivation are increasing in tea growing regions including China (Han et al., 2016, 2018). Tea is a long-living commercial beverage crop that can remain productive for a century if the gardens are well managed. The long life span of tea plants compels them to face environmental challenges years after years through physiological adaptations to changing climate (Larson, 2015; Li et al., 2017). Drivers of climate change differentially affect tea yield and quality on a spatiotemporal basis (Wijeratne et al., 2007; Han et al., 2016). Although the effects of climate change on the yield of food crops have extensively been studied, its impact on tea has received less attention. In particular, research on the effect of elevated CO2 on tea is still in its infancy (Ahmed et al., 2019). However, recently more attention has been paid to the issue and inter-governmental initiatives have been taken to address climate change effects on tea under the umbrella of the Food and Agriculture Organization of the United Nations (Han et al., 2018). Several research papers on the effect of CO2 on tea yield and quality were published in last 3 years (Hui et al., 2016; Li et al., 2016, 2017, 2018, 2019a; Roy et al., 2019; Pramanik and Phukan, 2020). However, a comprehensive review of the effect of elevated CO2 on tea plants is still missing. In this review, we intend to summarize key physiological and metabolic processes associated with the tea quality in response to elevated CO2. Besides, the potential impact of elevated CO2 on tea yield and defense has been discussed. We also try to find out research gaps and critical research areas on the effect of elevated CO2 on tea quality for future study.
Evidence from a number of studies shows that elevated CO2 improves leaf number, leaf area index, branches, shoot length, root length, and overall biomass accumulation in C3 plants (Huang et al., 2007; Kimball, 2016). Similarly, exposure of tea plants to elevated CO2 (800 μmol mol–1) even for 24 days increases plant height, shoot dry weight and root dry weight (Li et al., 2017). Recently, Li et al. (2019a) also showed that exposure of the 1-year-old tea seedlings to 770.5 μmol mol–1 CO2 concentration in open-top chambers for 60 days significantly increases biomass accumulation in terms of fresh weights of leaves (+15.04%), roots (+22%), and whole plants (+16.26%). Since the yield of tea is the sum of buds and young leaves, elevated CO2-induced promotion in shoot biomass greatly contributes to tea yield.
In C3 plants, such as tea, elevated CO2 stimulates the CO2 assimilation rate by providing sufficient substrates (i.e., CO2) required for photosynthetic reactions (Figure 1). This eventually leads to the enhanced supply of energy-rich compounds, such as adenosine triphosphate (ATP) and nicotinamide adenine dinucleotide phosphate (NADPH) under elevated CO2. Notably, stomata as a basic channel for gas exchange plays an important role in CO2 and O2 exchange between plant and atmosphere. A leaf gas exchange analysis showed that exposure of tea seedlings to elevated CO2 for 60 days increases the net photosynthetic rate (+20%) and intercellular CO2 concentrations (+15.74%); however, it decreases the stomatal conductance (−5.52%) and transpiration rate (−9.40%) in tea leaves (Li et al., 2019a). Intriguingly, the increase in net photosynthetic rate is much higher (+87.9%) in case of short duration (24 days) CO2-enrichment treatment, suggesting a potential photosynthetic acclimation following prolonged exposure to elevated CO2 in tea plants (Li et al., 2017). This raises the question of how tea plants increase the photosynthetic rate under reduced stomatal conductance. In C3 plants, the activity of ribulose-1,5-bis-phosphate (RuBP) carboxylase/oxygenase (RuBisCO) is critical for CO2 assimilation (Eisenhut et al., 2019; Thomey et al., 2019). Elevated CO2 increases maximum carboxylation rate of RuBisCO and maximum rates of RuBP regeneration in tea plants (Li et al., 2017), thus facilitating carboxylation over oxygenation of RuBP (Amthor, 1997; Li et al., 2013), which potentially contributes to increased CO2 assimilation in tea leaves (Li et al., 2017). Although exposure of tea plants to elevated CO2 (648–65 μmol mol–1) for 45 days does not significantly affect photosynthetic pigment content (Hui et al., 2016), a 3-month long CO2 enrichment (750 μmol mol–1) results in 18.4, 22.0, and 20.1% increased chlorophyll a, chlorophyll b, and carotenoid concentrations in tea shoots (Jiang et al., 2005). Through respiration, a part of the carbon is consumed by leaves, buds, shoots, and roots, and the rest is released as CO2 via stomata to the atmosphere (Huang et al., 2007; Eisenhut et al., 2019). Ultimately, respiration supplies energy in the form of ATP to plant cells. In tea plants, elevated CO2 (800 μmol mol–1) promotes total respiration rate (+28.9–53.6%), which is attributed to concurrent increases in the salicylhydroxamic acid (SHAM)-resistant respiration as well as the cyanide (CN)-resistant respiration (Li et al., 2017), suggesting that elevated CO2 triggers both electron transport pathways, i.e., the ATP-coupling SHAM-resistant cytochrome pathway and the CN-resistant alternative pathway utilized by plant mitochondria. Notably, the alternative respiration greatly contributes to redox homeostasis by minimizing the excess production of reactive oxygen species via the mitochondrial electron transport chain (Gong et al., 2020). It is believed that elevated CO2-induced photosynthesis enhances energy demand which triggers respiration as well.
Figure 1. Schematic representation of the overall effect of elevated CO2 on tea plants. Elevated CO2 increases photosynthesis and respiration, leading to enhanced primary metabolism. Stimulation of primary metabolism increases biomass accumulation as well as carbon-flow toward secondary metabolic pathways. Elevated CO2 increases concentrations of carbohydrates (starch and sugars), flavonoids, theanine, and salicylic acid, but it decreases caffeine content in tea leaves. Despite enhanced production of secondary metabolites and defense compounds, tea plant defense against aphids and necrotrophic fungi is greatly compromised under elevated CO2. The green arrows indicate promotion, while the red arrows or blunt arrow-heads indicate inhibition.
Among numerous plant metabolites, only a minor portion of metabolites are directly used for plant growth and development, commonly termed as “primary metabolites”; however, numerous other metabolites, commonly termed as “secondary metabolites,” are used for various functions, including plant defense against biotic and abiotic stresses (Zhao et al., 2013, 2019). When tea plants are grown under elevated CO2, tea leaves accumulate more soluble sugar, sucrose and starch (Li et al., 2017, 2019a). However, elevated CO2 (770.5 μmol mol–1) decreases free fatty acid content with no effect on soluble protein content in tea leaves (Li et al., 2019a). In addition to the influence on nutrient compositions, elevated CO2 affects functional components of tea leaves. For instance, a 24 days or 60 days exposure of tea plants to elevated CO2 significantly increases the concentrations of free amino acids, theanine, and tea polyphenols, but it decreases the content of caffeine in tea leaves (Li et al., 2017, 2019a). Tea polyphenols are the major antioxidant compounds in tea leaves that provide astringency to tea infusion (Chen et al., 2018; Li et al., 2019b). Nonetheless, polyphenols have numerous health benefits, such as relieving inflammation and oxidative stress (Zhao et al., 2016; Du et al., 2018; Fei et al., 2018). Under elevated CO2, tea polyphenols such as total catechins (major flavonoids) as well as (−)-epigallocatechin (EGC) and (−)-epigallocatechin-3-gallate (EGCG) concentrations become high (Li et al., 2017). A couple of comprehensive studies on the effect of elevated CO2 on tea quality show that elevated CO2 upregulates the expression of genes encoding key enzymes required for the biosynthesis of phenylpropanoids that act as precursors of different catechins. More importantly, the transcript levels of ANTHOCYANIDIN REDUCTASE (CsANR) remarkably upregulates (>500%) under elevated CO2, which encodes specific enzymes that catalyze the conversion of anthocyanidins into epicatechins (Li et al., 2017, 2019a). Interestingly, elevated CO2 also increases the concentrations of salicylic acid (SA) in tea leaves compared with the ambient CO2 (Li et al., 2017, 2019a). It has been further revealed that SA mediates elevated CO2-induced flavonoid biosynthesis in tea leaves and SA acts downstream of CO2 and enhances nitric oxide (NO) production to increase flavonoid biosynthesis (Li et al., 2019c). However, a SA-independent NO production pathway may also function under elevated CO2 (Figure 2).
Figure 2. A schematic model showing potential mechanisms of elevated CO2-induced changes in tea functional components and defense responses. Elevated CO2 enhances salicylic acid (SA) concentrations in tea leaves that trigger nitric oxide (NO) accumulation and subsequent flavonoid (FLA) biosynthesis by stimulating the activity of phenylalanine ammonia-lyase (PAL) under elevated CO2 conditions. Nonetheless, elevated CO2 may also promote FLA biosynthesis via NO in a SA-independent manner. Meanwhile, elevated CO2 inhibits caffeine biosynthesis, leading to reduced accumulation of jasmonic acid (JA) via the lipoxygenase (LOX)-dependent pathway. Decreased JA biosynthesis compromises caffeine-induced resistance to C. gloeosporioides (C. g., the anthracnose fungus) in tea plants. On the other hand, elevated CO2 increases the concentrations of soluble sugars, soluble proteins, and free fatty acids in tea leaves, which might contribute to the increased population abundance of T. aurantii (T. a.; the tea aphid) under elevated CO2. Arrows indicate promotion, while blunt arrow-heads indicate inhibition. Dotted lines are assumptions.
Theanine is a kind of non-protein amino acid that comprises about 50% of total amino acid content in tea leaves and it imparts the “umami” taste of tea (Vuong et al., 2011; Cheng et al., 2017). Theanine has numerous health benefits including improvement of memory, reduction of blood pressure, stress relief and stimulation of relaxation (Unno et al., 2017). Studies have revealed that elevated CO2 increases theanine concentration in tea leaves (Li et al., 2017, 2019a). However, concentrations of some essential amino acids are differentially modulated under elevated CO2 in tea leaves (Li et al., 2017, 2019a). There are also some discrepancies in existing literature relating to the elevated CO2 effect on theanine content, which is largely attributed to the exposure duration in different studies. For instance, Li et al. (2017) and Li et al. (2019a) exposed tea seedlings to elevated levels of CO2 (800 and 770 μmol mol–1) for 24 and 60 days, respectively. They found a significant increase in leaf theanine concentration under elevated CO2 conditions. However, Jiang et al. (2006) exposed tea seedlings to elevated CO2 (750 μmol mol–1) for 6 months and found a significant decrease in leaf theanine content. It is highly likely that long-term exposure to elevated CO2 would affect total carbon and nitrogen pools, causing an increase in the C:N ratio, which might also affect the biosynthesis of nitrogenous compounds, including theanine. Likewise, Hui et al. (2016) also found a negative effect of elevated CO2 on amino acid (including theanine) content, which eventually increased polyphenols to amino acid ratio in tea leaves.
In addition to flavonoids and theanine, caffeine accumulation also depends on environmental factors (Li et al., 2016, 2017). Studies have revealed that caffeine content declines when tea plants are grown under elevated CO2 conditions (Li et al., 2016, 2017, 2019a). Accordingly, transcript analysis of caffeine synthetic pathway genes reveals that elevated CO2 remarkably suppresses the expression of INOSINE 5′-MONOPHOSPHATE DEHYDROGENASE (CsTIDH), s-ADENOSYL-L-METHIONINE SYNTHASE (CssAMS) and TEA CAFFEINE SYNTHASE 1 (CsTCS1) in tea leaves (Li et al., 2017).
A general perception is that elevated CO2-induced stimulation of primary metabolism promotes secondary metabolism in plants, leading to enhanced synthesis of defense-related compounds (Noctor and Mhamdi, 2017). Although there is no discrepancy regarding the enhancement of SA levels under elevated CO2, changes in jasmonic acid (JA) levels were found different in two studies in tea plants. A study that used 2-year-old Longjing 43 tea seedlings showed that exposure of tea plants to elevated CO2 (800 μmol mol–1) for 14 days significantly decreases JA concentrations (Li et al., 2016); however, recently Li et al. (2019a) reported that exposure of 1-year-old Longjing Changye tea seedlings to elevated CO2 (770 μmol mol–1) for 60 days significantly increases JA concentrations (+98.6%) in tea leaves (Li et al., 2019a). Despite the increased accumulation of these metabolites under elevated CO2, plant resistance to some insects and pathogens is compromised in tea plants when grown under elevated CO2 (Li et al., 2019a). This raises another outstanding question, how tea plants balance growth and defense while improving tea quality under high levels of atmospheric CO2 concentrations.
Pathogens and insects invade plants in search of nutrients. While pathogens may reside inside plant cells to complete their life-cycle, insects mostly feed on plants. Therefore, palatably of host leaves matters to insect pests, which is largely dependent on the nutrient constituents of the plant organs (Alba et al., 2014). Studies have revealed that elevated CO2 alters nutrient constituents as well as defense compounds in leaves, leading to significant changes in insect infestation (Robinson et al., 2012; Li et al., 2019a). Thus the growth and development of herbivorous and sap-sucking insects is indirectly altered by elevated CO2 through its direct effects on plant biomass and nutrient compositions (Kazan, 2018; Li et al., 2019a). In general, elevated CO2 triggers population growth of sap-sucking insects such as aphids (e.g., Myzus persicae), whiteflies (e.g., Bemisia tabaci), and planthoppers (e.g., Nilaparvata lugens) (Jiang et al., 2016; Li et al., 2019a). However, the growth, survival rates and population density of most leaf-chewing insects are suppressed by elevated CO2 possibly because of the potential deterioration of nutrient quality of their feeds (Coll and Hughes, 2008; Kazan, 2018). In a recent study, Li et al. (2019a) showed that the population abundance of tea aphid (Toxoptera aurantii) significantly increased (+4.24–41.17%) when these aphids were fed on tea seedlings grown under elevated CO2. Although the study concludes that a reduction in caffeine content under elevated CO2 is potentially involved in reduced defense against aphids, such a low decrease (−3.38%) in caffeine content questions this claim. Therefore, it is possible that improved leaf nutrient status under elevated CO2, i.e., increased soluble sugars, soluble proteins and free amino acids might play a major role in increased tea aphid abundance due to the characteristics features of aphids, such as short life span, high body weight, increased fecundity and population growth under elevated CO2. Notably, stomatal closure and subsequent minimization of transpirational water losses can result in improved leaf water status, which promotes the infestation of pea aphid (Acyrthosiphon pisum) in Medicago truncatula (Sun et al., 2015; Kazan, 2018). As elevated CO2 also reduces the transpiration rate in tea plants by regulating stomatal conductance, the leaf water status may play a role in increased tea aphid abundance.
The role of caffeine in tea plant defense against pathogenic fungus has been reported under elevated CO2. A reduction in caffeine content in tea leaves under elevated CO2 conditions increases the susceptibility of the tea plants to Colletotrichum gloeosporioides, which causes anthracnose, brown blight and dieback disease of tea in different geographical locations depending on the weather conditions (Li et al., 2016). Interestingly, exogenous application of caffeine suppresses the necrotic lesions caused by C. gloeosporioides, which is attributed to the caffeine-induced elevation of endogenous JA content under elevated CO2 conditions in tea leaves. The study also revealed that caffeine-induced increase in JA levels is attributed to JA biosynthesis through the lipoxygenase (LOX) pathway under elevated CO2 in tea leaves (Figure 2). Based on this study, it is quite clear that caffeine plays a vital role in tea plant defense against necrotrophic fungal pathogens in tea plants; however, this response is compromised under elevated CO2 due to reduced biosynthesis of caffeine and JA (Li et al., 2016). Conversely, elevated CO2 improves the resistance of Arabidopsis thaliana to the fungal plant pathogen Plectosphaerella cucumerina and the oomycete pathogen Hyaloperonospora arabidopsidis by stimulating the JA-dependent and SA-dependent defense priming, respectively (Williams et al., 2018).
Surveys of literature show that the effects of elevated CO2 on tea were mostly studied by exposing the plants to a range of artificially enriched CO2 levels (550, 650, 750, and 800) for various durations (24 days, 45 days, 60 days, and 6 months) in open-top chambers or controlled closed chambers, suggesting that studies using free air CO2 enrichment (FACE) for long-duration are needed to better understand the realistic responses of tea plants to climate change. Notably, about a 100-year life span of tea plants allows them to witness and experience gradual changes in atmospheric CO2 concentrations in a single generation, which possibly compels the tea plants to operate massive physiologic, metabolic and transcriptional reprogramming to adapt to changing climate. The two levels of CO2, 550 and 800 that are frequently used for CO2-enrichment studies, are predicted atmospheric CO2 concentrations of the year 2050 and 2100, respectively. Thus, it is quite unusual that tea plants will experience such high levels of CO2 overnight, which is possibly the main limitation of the existing research. Therefore, it will be more meaningful to explore how small changes in atmospheric CO2 levels would affect tea quality.
Climate change is a cumulative effect of multiple factors, such as changes in temperatures, radiation, precipitation, and CO2 levels. Thus it is important to study the combined effects of multiple factors along with elevated CO2 to better mimic the real-world situations. Availability or deficiency of essential macro and micro elements and their effect on tea quality under elevated CO2 conditions can also be considered from the point of nutraceutical value. In this regard, the role of elevated CO2 in the regulation of γ-aminobutyric acid (GABA), a non-proteinogenic amino acid in tea with numerous health benefits, should be considered. Moreover, safety factors such as the occurrence of toxicants, mycotoxins, and contaminants in tea under elevated CO2 remain largely unknown. In this aspect, biotic factors such as insect herbivory and pathogen infection can also be included. Notably, how the quality of so-called “bug-bitten tea,” a kind of Oolong tea that is produced under special circumstances upon infestation with tea green leafhoppers (Empoasca vitis), is influenced by elevated CO2, could be an interesting research topic. One factor, which has been ignored in studying elevated CO2 effects on tea plants, is the volatile emission. Plants emit multiple volatile compounds upon insect and pathogen attacks. The volatile compounds not only impart characteristic aroma to tea as a quality parameter but also serve as defense signals and media for plant-plant communication as well as plant-insect interactions (Zhao et al., 2019). Therefore, this issue is expected to address in future studies.
When CO2 concentration increases in the atmosphere, it not only influences plants but also other animals including insects and pests. Elevated CO2 can influence the virulence, aggressiveness, growth, development, fecundity, fitness, and survival of pests and pathogens by altering host physiology (Kazan, 2018). Therefore, it is highly likely that insects and pathogens may also evolve adaptive strategies in response to elevated CO2. However, studies revealing elevated CO2 effects on insect herbivory and pathogen infections rarely used elevated CO2-adapted insects and pathogens. Thus, it is important to consider this issue to better understand the response of tea plants to pests and pathogens. Moreover, genotype screening in response to elevated CO2 is necessary to develop tea cultivars resilient to climate change.
Plant β-carbonic anhydrases (βCAs) are important CO2 sensing and metabolizing proteins that catalyze the interconversion between CO2 and (DiMario et al., 2017; Kazan, 2018). However, the role of βCAs in tea plants under elevated CO2 remains far from being substantiated. Multiple hormones and their interaction mediates plant response to biotic and abiotic factors. Although a few studies have highlighted a role for gibberellins, abscisic acid, brassinosteroids, and SA in tea quality, the role of hormones and signaling molecules in elevated CO2-induced changes in tea quality remains largely unknown. In addition, major transcription factors, such as, MYB, WRKY, bHLH, and WD40 that regulate the biosynthesis of terpenoids and flavonoids in other plants, should be taken into account to explore the mechanisms of elevated CO2-induced regulation of tea quality.
In summary, existing literature suggests that elevated CO2 promotes tea yield and quality, but it attenuates tea plant resistance to some insects and pathogens, which poses a serious threat to future tea production systems. Tea quality is a complex perception of multiple factors, which is believed to be improved under elevated CO2 as tea plants grown under elevated CO2 accumulate high levels of catechins and theanine, and low level of caffeine in tea leaves. It appears that decreased caffeine accumulation under elevated CO2 is one of the main reasons of attenuated defense response in tea plants. However, there are some technical limitations in the studies relating to elevated CO2 effects on tea plants, which should be taken into account to design more realistic experiments to better understand the responses of tea plants to elevated CO2 in the future.
GA and XL conceived and designed the manuscript, and wrote the draft manuscript. GA, XL, AL, and SC reviewed and edited the manuscript. All authors have read and approved the manuscript.
Research in the authors’ laboratories was funded by the National Key R&D Program of China (2017YFE0107500 and 2018YFD1000800) and the National Natural Science Foundation of China (31950410555, 31600561, 31872092, and 31872157).
The authors declare that the research was conducted in the absence of any commercial or financial relationships that could be construed as a potential conflict of interest.
Ahmed, S., Griffin, T. S., Kraner, D., Schaffner, M. K., Sharma, D., Hazel, M., et al. (2019). Environmental factors variably impact tea secondary metabolites in the context of climate change. Front Plant Sci 10:939. doi: 10.3389/fpls.2019.00939
Ahuja, I., De Vos, R. C., Bones, A. M., and Hall, R. D. (2010). Plant molecular stress responses face climate change. Trends Plant Sci 15, 664–674. doi: 10.1016/j.tplants.2010.08.002
Alba, C., Bowers, M. D., Blumenthal, D., and Hufbauer, R. A. (2014). Chemical and mechanical defenses vary among maternal lines and leaf ages in Verbascum thapsus L. (Scrophulariaceae) and reduce palatability to a generalist insect. PLoS One 9:e104889. doi: 10.1371/journal.pone.0104889
Amthor, J. S. (1997). Plant respiratory responses to elevated carbon dioxide partial pressure. Advances in carbon dioxide effects research 35–77. doi: 10.2134/asaspecpub61.c2
Chen, Q., Zhang, Y., Tao, M., Li, M., Wu, Y., Qi, Q., et al. (2018). Comparative metabolic responses and adaptive strategies of tea leaves (Camellia sinensis) to N2 and CO2 anaerobic treatment by a nontargeted metabolomics approach. J Agric Food Chem 66, 9565–9572. doi: 10.1021/acs.jafc.8b03067
Cheng, Y., Ahammed, G. J., Yu, J., Yao, Z., Ruan, M., Ye, Q., et al. (2017). Corrigendum: Putative WRKYs associated with regulation of fruit ripening revealed by detailed expression analysis of the WRKY gene family in pepper. Sci Rep 7, 43498. doi: 10.1038/srep43498
Coll, M., and Hughes, L. (2008). Effects of elevated CO2 on an insect omnivore: A test for nutritional effects mediated by host plants and prey. Agriculture, Ecosystems & Environment 123, 271–279. doi: 10.1016/j.agee.2007.06.003
DiMario, R. J., Clayton, H., Mukherjee, A., Ludwig, M., and Moroney, J. V. (2017). Plant carbonic anhydrases: structures, locations, evolution, and physiological roles. Molecular Plant 10, 30–46. doi: 10.1016/j.molp.2016.09.001
Du, L., Li, J., Zhang, X., Wang, L., and Zhang, W. (2018). Pomegranate peel polyphenols inhibits inflammation in LPS-induced RAW264.7 macrophages via the suppression of MAPKs activation. Journal of Functional Foods 43, 62–69.
Eisenhut, M., Roell, M. S., and Weber, A. P. M. (2019). Mechanistic understanding of photorespiration paves the way to a new green revolution. New Phytol 223, 1762–1769. doi: 10.1111/nph.15872
Fei, P., Ali, M. A., Gong, S., Sun, Q., Bi, X., Liu, S., et al. (2018). Antimicrobial activity and mechanism of action of olive oil polyphenols extract against Cronobacter sakazakii. Food Control 94, 289–294. doi: 10.1016/j.foodcont.2018.07.022
Gong, Q., Li, S., Zheng, Y., Duan, H., Xiao, F., and Zhuang, Y., et al. (2020). SUMOylation of MYB30 enhances salt tolerance by elevating alternative respiration via transcriptionally upregulating AOX1a in Arabidopsis. Plant J doi: 10.1111/tpj.14689
Han, W., Li, X., Yan, P., Zhang, L., and Jalal Ahammed, G. (2018). “Tea cultivation under changing climatic conditions,” in Global tea science Current status and future needs, eds V. S. Sharma and K. Gunasekare (Cambridge: Burleigh Dodds Science Publishing), 455–472. doi: 10.19103/as.2017.0036.19
Han, W.-Y., Huang, J.-G., Li, X., Li, Z.-X., Ahammed, G. J., Yan, P., et al. (2016). Altitudinal effects on the quality of green tea in east China: a climate change perspective. European Food Research and Technology 243, 323–330. doi: 10.1007/s00217-016-2746-5
Huang, J.-G., Bergeron, Y., Denneler, B., Berninger, F., and Tardif, J. (2007). Response of forest trees to increased atmospheric CO2. Critical Reviews in Plant Sciences 26, 265–283. doi: 10.1080/07352680701626978
Hui, X. U., Lei, L. I., Qinghui, L. I., Zhou, L., Zhu, X., and Chen, F., et al. (2016). Effects of elevated atmospheric CO2 concentration and temperature on photosynthesis system and quality components in tea plant. Journal of Nanjing Agricultural University
IPCC (2014). Climate Change 2014: Synthesis Report. Contribution of Working Groups I, II and III to the Fifth Assessment Report of the Intergovernmental Panel on Climate Change, IPCC. Geneva: Cambridge University Press.
Jiang, S., Liu, T., Yu, F., Li, T., Parajulee, M. N., Zhang, L., et al. (2016). Feeding behavioral response of cotton aphid, Aphis gossypii, to elevated CO2: EPG test with leaf microstructure and leaf chemistry. Entomologia Experimentalis et Applicata 160, 219–228. doi: 10.1111/eea.12475
Jiang, Y., Zhang, Q., and Zhang, S. (2006). Effects of atmospheric CO2 concentration on tea quality. Journal of Tea Science 26, 299–304.
Jiang, Y., Zhang, S., and Zhang, Q. (2005). Effects of elevated atmospheric CO2 concentration on photo-physiological characteristics of tea plant. Journal of Tea Science 25, 43–48. doi: 10.13305/j.cnki.jts.2005.01.008
Kazan, K. (2018). Plant-biotic interactions under elevated CO2: a molecular perspective. Environmental and Experimental Botany 153, 249–261. doi: 10.1016/j.envexpbot.2018.06.005
Kim, T. I., Lee, Y. K., Park, S. G., Choi, I. S., Ban, J. O., Park, H. K., et al. (2009). l-Theanine, an amino acid in green tea, attenuates beta-amyloid-induced cognitive dysfunction and neurotoxicity: reduction in oxidative damage and inactivation of ERK/p38 kinase and NF-kappaB pathways. Free Radic Biol Med 47, 1601–1610. doi: 10.1016/j.freeradbiomed.2009.09.008
Kimball, B. A. (2016). Crop responses to elevated CO2 and interactions with H2O. N, and temperature. Curr Opin Plant Biol 31, 36–43. doi: 10.1016/j.pbi.2016.03.006
Larson, C. (2015). Reading the tea leaves for effects of climate change. Science 348, 953–954. doi: 10.1126/science.348.6238.953
Li, L., Wang, M., Pokharel, S. S., Li, C., Parajulee, M. N., Chen, F., et al. (2019a). Effects of elevated CO2 on foliar soluble nutrients and functional components of tea, and population dynamics of tea aphid. Toxoptera aurantii. Plant Physiol Biochem 145, 84–94. doi: 10.1016/j.plaphy.2019.10.023
Li, X., Zhang, L.-P., Zhang, L., Yan, P., Ahammed, G. J., and Han, W.-Y. (2019b). Methyl Salicylate Enhances Flavonoid Biosynthesis in Tea Leaves by Stimulating the Phenylpropanoid Pathway. Molecules 24, 362. doi: 10.3390/molecules24020362
Li, X., Zhang, L., Ahammed, G. J., Li, Y.-T., Wei, J.-P., Yan, P., et al. (2019c). Salicylic acid acts upstream of nitric oxide in elevated carbon dioxide-induced flavonoid biosynthesis in tea plant (Camellia sinensis L.). Environmental and Experimental Botany 161, 367–374. doi: 10.1016/j.envexpbot.2018.11.012
Li, X., Ahammed, G. J., Li, Z., Tang, M., Yan, P., and Han, W. (2016). Decreased Biosynthesis of Jasmonic Acid via lipoxygenase pathway compromised caffeine-induced resistance to colletotrichum gloeosporioides under elevated CO2 in tea seedlings. Phytopathology 106, 1270–1277. doi: 10.1094/PHYTO-12-15-0336-R
Li, X., Ahammed, G. J., Zhang, L., Yan, P., Zhang, L., and Han, W.-Y. (2018). “Elevated Carbon Dioxide-Induced Perturbations in Metabolism of Tea Plants,” in Stress Physiology of Tea in the Face of Climate Change, eds W.-Y. Han, X. Li, and G. J. Ahammed (Singapore: Springer Singapore), 135–155. doi: 10.1007/978-981-13-2140-5_7
Li, X., Zhang, G., Sun, B., Zhang, S., Zhang, Y., Liao, Y., et al. (2013). Stimulated leaf dark respiration in tomato in an elevated carbon dioxide atmosphere. Sci Rep 3, 3433. doi: 10.1038/srep03433
Li, X., Zhang, L., Ahammed, G. J., Li, Z.-X., Wei, J.-P., Shen, C., et al. (2017). Stimulation in primary and secondary metabolism by elevated carbon dioxide alters green tea quality in Camellia sinensis L. Scientific Reports 7, 7937. doi: 10.1038/s41598-017-08465-1
Macfarlane, A., and Macfarlane, I. (2004). The empire of tea: the remarkable history of the plant that took over the world. New York, NY: Overlook Press.
Mancini, E., Beglinger, C., Drewe, J., Zanchi, D., Lang, U. E., and Borgwardt, S. (2017). Green tea effects on cognition, mood and human brain function: A systematic review. Phytomedicine 34, 26–37. doi: 10.1016/j.phymed.2017.07.008
Noctor, G., and Mhamdi, A. (2017). Climate change, CO2, and defense: the metabolic, redox, and signaling perspectives. Trends in Plant Science 22, 857–870. doi: 10.1016/j.tplants.2017.07.007
Pramanik, P., and Phukan, M. (2020). Assimilating atmospheric carbon dioxide in tea gardens of northeast India. Journal of Environmental Management 256, 109912. doi: 10.1016/j.jenvman.2019.109912
Robinson, E. A., Ryan, G. D., and Newman, J. A. (2012). A meta-analytical review of the effects of elevated CO2 on plant–arthropod interactions highlights the importance of interacting environmental and biological variables. New Phytologist 194, 321–336. doi: 10.1111/j.1469-8137.2012.04074.x
Roy, S., Barooah, A. K., Ahmed, K. Z., Baruah, R. D., Prasad, A. K., and Mukhopadhyay, A. (2019). Impact of climate change on tea pest status in northeast India and effective plans for mitigation. Acta Ecologica Sinica doi: 10.1016/j.chnaes.2019.08.003
Siamwala, J. H., Dias, P. M., Majumder, S., Joshi, M. K., Sinkar, V. P., Banerjee, G., et al. (2013). L-theanine promotes nitric oxide production in endothelial cells through eNOS phosphorylation. J Nutr Biochem 24, 595–605. doi: 10.1016/j.jnutbio.2012.02.016
Sun, Y., Guo, H., Yuan, L., Wei, J., Zhang, W., and Ge, F. (2015). Plant stomatal closure improves aphid feeding under elevated CO2. Glob Chang Biol 21, 2739–2748. doi: 10.1111/gcb.12858
Thomey, M. L., Slattery, R. A., Kohler, I. H., Bernacchi, C. J., and Ort, D. R. (2019). Yield response of field-grown soybean exposed to heat waves under current and elevated [CO2]. Glob Chang Biol 25, 4352–4368. doi: 10.1111/gcb.14796
Unno, K., Noda, S., Kawasaki, Y., Yamada, H., Morita, A., Iguchi, K., et al. (2017). Reduced Stress and Improved Sleep Quality Caused by Green Tea Are Associated with a Reduced Caffeine Content. Nutrients 9, 777. doi: 10.1002/jsfa.4373
Vuong, Q. V., Bowyer, M. C., and Roach, P. D. (2011). L-Theanine: properties, synthesis and isolation from tea. J Sci Food Agric 91, 1931–1939.
Wijeratne, M. A., Anandacoomaraswamy, A., Amarathunga, M. K. S. L. D., Ratnasiri, J., Basnayake, B. R. S. B., and Kalra, N. (2007). Assessment of impact of climate change on productivity of tea (Camellia sinensis L.) plantations in Sri Lanka. Journal of the National Science Foundation of Sri Lanka 35
Williams, A., Petriacq, P., Schwarzenbacher, R. E., Beerling, D. J., and Ton, J. (2018). Mechanisms of glacial-to-future atmospheric CO2 effects on plant immunity. New Phytol 218, 752–761. doi: 10.1111/nph.15018
Zhao, N., Wang, G., Norris, A., Chen, X., and Chen, F. (2013). Studying Plant Secondary Metabolism in the Age of Genomics. Critical Reviews in Plant Sciences 32, 369–382. doi: 10.1080/07352689.2013.789648
Zhao, S., Li, J., Wang, L., and Wu, X. (2016). Pomegranate peel polyphenols inhibit lipid accumulation and enhance cholesterol efflux in raw264.7 macrophages. Food Funct 7, 3201–3210. doi: 10.1039/c6fo00347h
Keywords: climate change, tea quality, elevated CO2, secondary metabolism, catechin, theanine, caffeine, plant defense
Citation: Ahammed GJ, Li X, Liu A and Chen S (2020) Physiological and Defense Responses of Tea Plants to Elevated CO2: A Review. Front. Plant Sci. 11:305. doi: 10.3389/fpls.2020.00305
Received: 18 December 2019; Accepted: 03 March 2020;
Published: 20 March 2020.
Edited by:
Marco Landi, University of Pisa, ItalyReviewed by:
Xiumin Fu, South China Botanical Garden, Chinese Academy of Sciences, ChinaCopyright © 2020 Ahammed, Li, Liu and Chen. This is an open-access article distributed under the terms of the Creative Commons Attribution License (CC BY). The use, distribution or reproduction in other forums is permitted, provided the original author(s) and the copyright owner(s) are credited and that the original publication in this journal is cited, in accordance with accepted academic practice. No use, distribution or reproduction is permitted which does not comply with these terms.
*Correspondence: Xin Li, bGl4aW5AdHJpY2Fhcy5jb20=; Golam Jalal Ahammed, YWhhbW1lZEBoYXVzdC5lZHUuY24=
†These authors have contributed equally to this work
Disclaimer: All claims expressed in this article are solely those of the authors and do not necessarily represent those of their affiliated organizations, or those of the publisher, the editors and the reviewers. Any product that may be evaluated in this article or claim that may be made by its manufacturer is not guaranteed or endorsed by the publisher.
Research integrity at Frontiers
Learn more about the work of our research integrity team to safeguard the quality of each article we publish.