- Plant Science Laboratory, Cranfield University, Bedfordshire, United Kingdom
Strawberry shelf life is limited, and little is known about the postharvest regulation of senescence in different fruit tissues. Strawberry is classified as a non-climacteric fruit, yet it is known that ethylene affects strawberry ripening. Here the effects of continuous exogenous ethylene (50 µl l−1) were investigated in cold stored strawberry (5°C). The physiological and biochemical responses of ripe strawberry were evaluated across 6 days, together with hormonal profiles of the whole fruit and individual tissues (achenes and receptacle). Continuous exposure to ethylene induced as a first response an accumulation of abscisic acid (ABA) in the receptacle tissue, followed by an increase in CO2 production. Ethylene also elicited sucrose hydrolysis and malic acid catabolism, with the major effect seen after 4 days of ethylene exposure. Additionally, accumulation of phenolics (epicatechin and chlorogenic acid) were also observed in ethylene treated strawberry. Achenes did not exhibit a response to ethylene, yet catabolism of both ABA and auxins increased by two thirds during air storage. In contrast, ethylene induced ABA accumulation in the receptacle tissue without ABA catabolism being affected. This hormonal disequilibrium in response to ethylene between the two tissues was maintained during storage, and therefore might be the precursor for the following biochemical variations reported during storage.
Introduction
The high perishability of strawberry represents a bottleneck for expanding the fresh market supply chain (Terry et al., 2011). Strawberry is classified as a non-climacteric fruit and its ripening is mainly regulated by the abscisic acid (ABA)/auxins ratio (Moya-León et al., 2019). In particular, auxins have been associated with ripening inhibition, while ABA accumulation has been linked with promotion of strawberry fruit ripening (Jia et al., 2011; Symons et al., 2012; Leng et al., 2014; Chen et al., 2015; Chen et al., 2016; Giné-Bordonaba and Terry, 2016; Jia et al., 2016; Li et al., 2016b; Medina-Puche et al., 2016). Despite this, an increasing number of studies have highlighted that ethylene does affect the late stages of ripening (Gu et al., 2019). Confirmations of ethylene involvement in strawberry ripening are also supported by research using the ethylene binding inhibitor, 1-methylcyclopropene (1-MCP) (Ku et al., 1999; Jiang et al., 2001; Bower et al., 2003; Villarreal et al., 2010; Villareal et al., 2016).
Ethylene plays a key role in the development of strawberry color, in the accumulation of taste-related compounds (flavonoids, phenolics, organic acids, and sugars), and in the softening process (Villarreal et al., 2010; Merchante et al., 2013; Li et al., 2016a; Villareal et al., 2016; Moya-León et al., 2019). Moreover, it has been reported that ethylene biosynthesis and signaling genes are differentially expressed during ripening of strawberry, and that some Ethylene Response Factors (ERFs) showed an expression pattern similar to those found in a climacteric fruit (Trainotti et al., 2005; Sun et al., 2013; Sánchez-Sevilla et al., 2017). Nowadays, it is well understood that ripening involves cross-talk among different hormones rather than single hormone action (Chang et al., 2013; Chen et al., 2015). However, the complexity of ripening regulation in strawberry is also attributable to the peculiar anatomy of the fruit, which is composed of achenes (true fruit) and a swollen fleshy receptacle. The two tissues are different in terms of origin, physiological and biochemical roles, and metabolic networks (Fait et al., 2008; Csukasi et al., 2011; Symons et al., 2012; Gu et al., 2019). It has been demonstrated that endogenous ethylene production in ripening strawberry is mainly attributable to the achenes (Iannetta et al., 2006). Nevertheless, a recent study describing the transcriptional and hormonal profiles of the two tissues highlighted that ethylene related genes were upregulated in the receptacle only (Sánchez-Sevilla et al., 2017). Strawberry ripening seems therefore to depend on how the achenes and receptacle differentially respond and synergistically interplay with one another (Merchante et al., 2013).
Despite increasing knowledge about strawberry ripening, it is surprising that little work has evaluated the postharvest hormonal regulation of strawberry physiology. Previous evidence has suggested that ethylene and the control thereof can have both positive and negative effects during postharvest storage of strawberry (Terry et al., 2007a), but the interplay between exogenous ethylene and ABA during postharvest is still unknown. One of the consequences of this paucity of information is also reflected in the limitation of strawberry shelf life management strategies.
To better characterise the role of ethylene, the postharvest effects of continuous exogenous ethylene (50 µl l−1) exposure were investigated. The physiological and biochemical responses of ripe strawberry were evaluated across 6 days of cold storage, together with the hormonal profiles of whole fruit and individual tissues (achenes and receptacle).
Materials and Methods
Plant Material and Ethylene Treatment
Ripe strawberry fruit (cv. Sonata, n = 252) were purchased from a local grower (H & H Duncalfe in Cambs., UK). Plants were grown under standard commercial conditions (Spanish tunnels), and fruit harvested on 6th August before being transferred to Cranfield University within 2 h. On arrival, disease-free fruits of similar size and weight were selected, placed in plastic punnets and subjected to two different storage environments: continuous air (control), continuous ethylene supplementation (50 µl l−1). Each punnet contained n = 12 fruit and for each treatment there were n = 5 punnets (n = 60 fruit per treatment). The punnets were placed inside labeled 13 L sealed plastic boxes stored at 5°C for 6 days to simulate shelf life conditions in a domestic refrigerator, and constantly flushed to avoid the creation of modified atmosphere. Continuous exposure to ethylene was achieved by flushing ethylene in air (50 µl l−1, 250 ml min−1) using a blower manifold (Amoah et al., 2017) (custom built and supplied by Air Equipment, Beds., UK). After 2 h from the beginning of treatment the concentration of ethylene (50 µl l−1) was quantified as previously described (Terry et al., 2007a). Control strawberries were constantly flushed with cleaned air (250 ml min−1) from a cylinder of pure air to ensure complete air exchange within the boxes and to prevent the creation of a modified atmosphere.
Fruit Sampling
At each sampling time (0 d; days of storage, 1 d, 2 d, 4 d, 6 d), the 13 l boxes were opened for few seconds and 12 fruit from each treatment (n = 12) were collected. They were separated into three blocks (n = 4 fruit) and assessed for CO2 and ethylene production, weight loss and color changes. Each of these parameters was assessed on three replicates per treatments. After the physiological measurements, the fruit were longitudinally halved. Following division, fruit were immediately snap frozen and freeze dried for further analysis. One half was used as whole fruit and the other was divided into achenes and receptacle tissues (Terry et al., 2007b). The receptacle tissue and achenes were manually separated from freeze dried material, using a pair of tweezers. All the achenes from four halves of strawberry fruit per treatment (* 3 blocks) were collected at each time point. Fruit assessed on day zero, before continuous ethylene exposure, were used for baseline measurements.
Physiological Assessments
CO2 Production Measurement and Weight Loss
Real time CO2 production rate of strawberries were measured in situ (5°C) connecting the 13 l boxes to a Sable Respirometer System (Model 1.3.8 Pro, Sable Systems International, NV, USA) according to Alamar et al. (2017). The blocks (four fruits) for each treatment were weighed before each measurement. The weight was used to calculate the CO2 production per kg of fruit, and the percentage of weight loss.
Ethylene Production Rate
Real time ethylene production was continuously monitored (for 3 h) ex situ (20°C) with a laser-based photoacoustic ethylene detector ETD-300 (Sensor Sense B.V., Nijmegen, The Netherlands) as previously described (Salman et al., 2009).
Color Measurements
Objective color (lightness; L*), chroma index (color saturation; C*), hue angle (H°) was determined for each fruit (n = 12) per treatment as previously reported (Terry et al., 2007a). Measurements were obtained using a Minolta CR-400 colorimeter and DP-400 data processor (Minolta Co. Ltd., Japan).
Biochemical Analysis
Sugars and Organic Acids Extraction and Quantification
Sugars and organic acids were extracted from whole fruit samples as previously reported (Terry et al., 2007a) and quantified using an Agilent 1200 series HPLC binary pump system (Agilent, Berks., UK) coupled with either an Agilent refractive index detector (RID) G1312A for sugars or a G1364C/G1315D photodiode array for organic acids. The concentration of glucose, fructose and sucrose, and citric, ascorbic and malic acids were calculated by comparison against an external calibration curve prepared with authentic standards (Sigma-Aldrich, Dorset, UK) and the results expressed as g kg−1 of dry weight.
Phenolic Compounds Extraction and Quantification
Phenolic compounds were extracted from whole fruit samples as previously described (Terry et al., 2007b). Concentrations of total anthocyanins, pelargonidin-3-glucoside, pelargonidin-3-glucoside derivative, cyanidin-3-glucoside, quercetin-3-glucoside, catechin, epicatechin, ellagic and chlorogenic acids (Sigma-Aldrich, Dorset, UK or Extrasynthese, Lyon, France) were calculated against authentic standards and expressed as mg kg−1 of dry weight.
Extraction and Quantification of Strawberry Phytohormones
Phytohormones were extracted from both whole fruit and individual tissues (achenes and receptacle) and analyzed as previously described (Ordaz-Ortiz et al., 2015). An Agilent 6540 Ultra High Definition Accurate Mass Q-TOF LC-MS System was used to quantify abscisic acid (ABA), 7'OH-abscisic acid (7'OH-ABA), indole-3-acetic acid (IAA) and indole-3-acetylaspartic acid (IAAsp). Calibration curves for the four compounds were generated by plotting the known concentration of the calibration level (ABA and 7'-OH ABA were purchased from National Research Council of Canada-Plant Biotechnology Institute, Ottawa, Canada. IAA and IAAsp were purchased from OlchemIm Ltd, Olomouc, Czech Republic) against the relative response calculated for each calibration level. Quantification analysis was carried out using an Agilent MassHunter Quantitative Analysis Software B.05.00. Endogenous phytohormones concentration was calculated as µg kg−1 of dry weight.
Statistical Analysis
Statistical analysis of results was performed using STATISTICA software for Windows (Dell Inc., 2015. Dell Statistica, version 12). Data were checked for residuals distribution, and tested with ANOVA, followed by a comparison of the means according to a least significant difference (LSD) test at p < 0.05.
Results
Physiological Assessments
The physiological parameters of respiration activity in terms of CO2 production, weight loss and color changes of strawberry fruit were assessed. Significant variations relating to continuous ethylene exposure were identified in the production of CO2 and weight loss (Figure 1), while color parameters were more affected by the storage time.
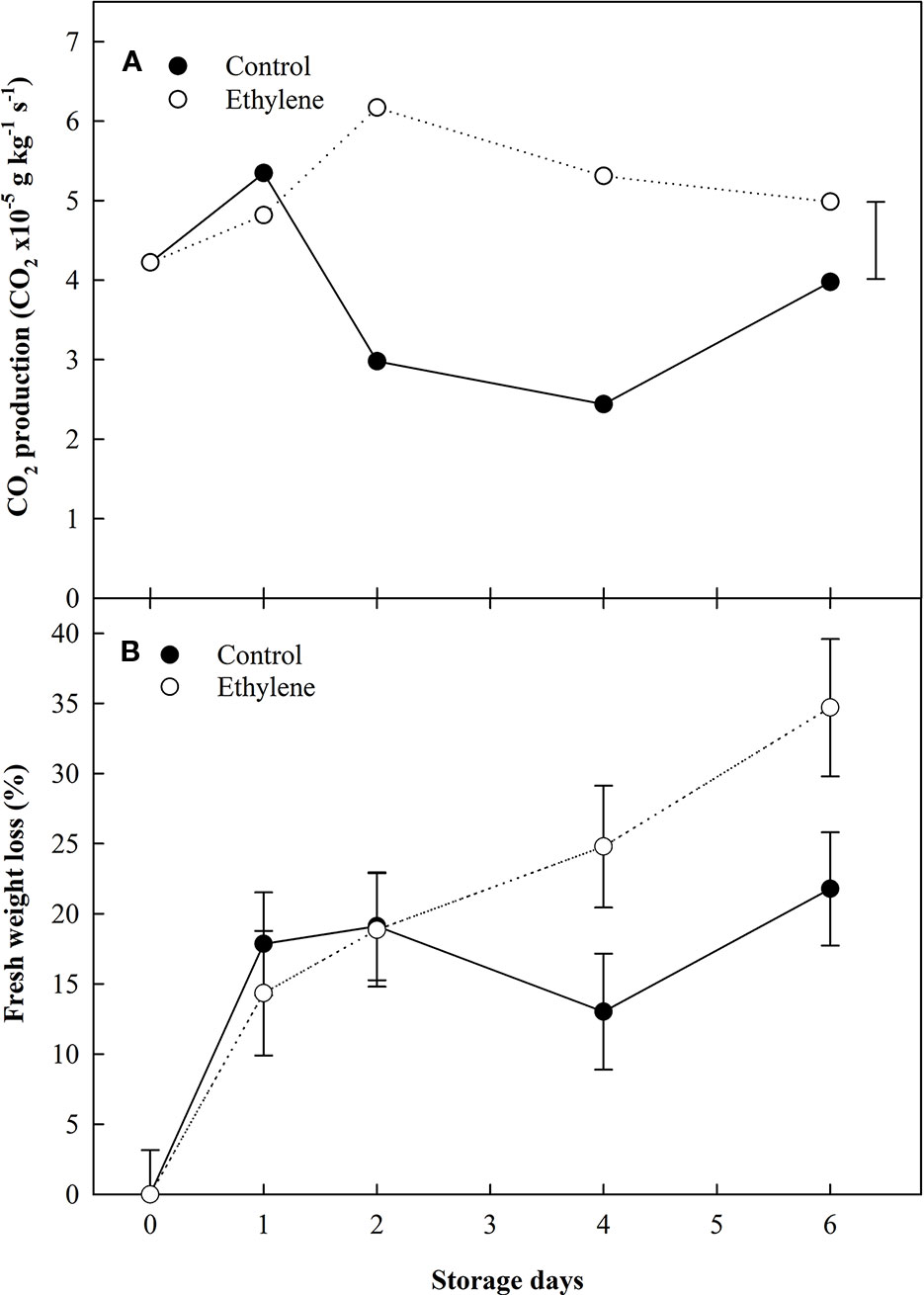
Figure 1 The effect of ethylene (continuous exposure) on CO2 production (CO2 × 10×5 g kg−1 s−1) and fresh weight variation (kg) of strawberries, cv. Sonata, stored at 5°C for 6 d. (A) CO2 production. Vertical bar represents the LSD (p <0.05). (B) Fresh weight variation. Vertical bars represent the Standard Error.
Ethylene treated fruit exhibited a more than 2-fold increase in CO2 production compared to the control fruit during the storage (Figure 1A). However, by the end storage (6 d) the CO2 production of control fruit was similar to ethylene treated fruit. The ethylene treated fruit showed a total weight loss of circa 35% at the end of storage, while the difference in weight in control fruit was around 20% (Figure 1B).
The color evolution assessment showed that the chroma index (C*) and the hue angle (H°) decreased during the storage and exhibited a more marked decline in ethylene treated fruit. However, significant differences were identified only in relation with the storage day (p < 0.0000 and p < 0.0052, respectively) (Table 1), while the lightness (L*) did not change (data not shown).
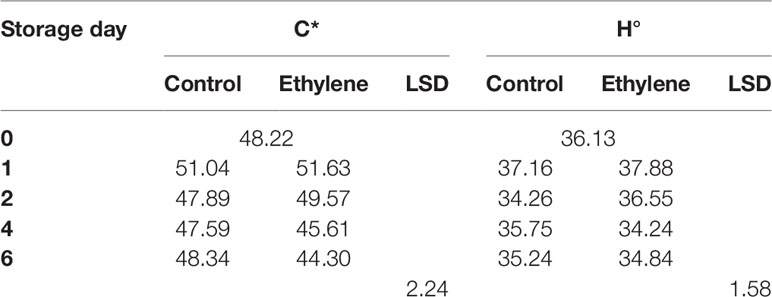
Table 1 The effect of ethylene (continuous exposure) on chroma index (C*) and the hue angle (H°) of strawberries, cv. Sonata, stored at 5°C for 6. d. LSD (p < 0.05) for the overall means of the significant variable “storage day”.
Biochemical Changes Induced by Continuous Ethylene Exposure: Sugars/Organic Acids, and Phenolics Compounds
Sugars data showed significant differences related to both treatment and storage day (Figure 2). Ethylene induced sucrose hydrolysis and accumulation of reducing sugars. In particular, ethylene treated strawberries showed increased sucrose hydrolysis from day 4 of storage, and by 6d, ethylene treated fruit had halved their initial sucrose values. In contrast, sucrose concentrations in control fruit decreased by ca. 25% compared to the initial amounts (Figure 2A). Concomitantly, ethylene-treated also induced the accumulation of reducing sugars in treated fruit (glucose 1.05-fold increase, fructose 1.1-fold increase) (Figures 2B, C, respectively).
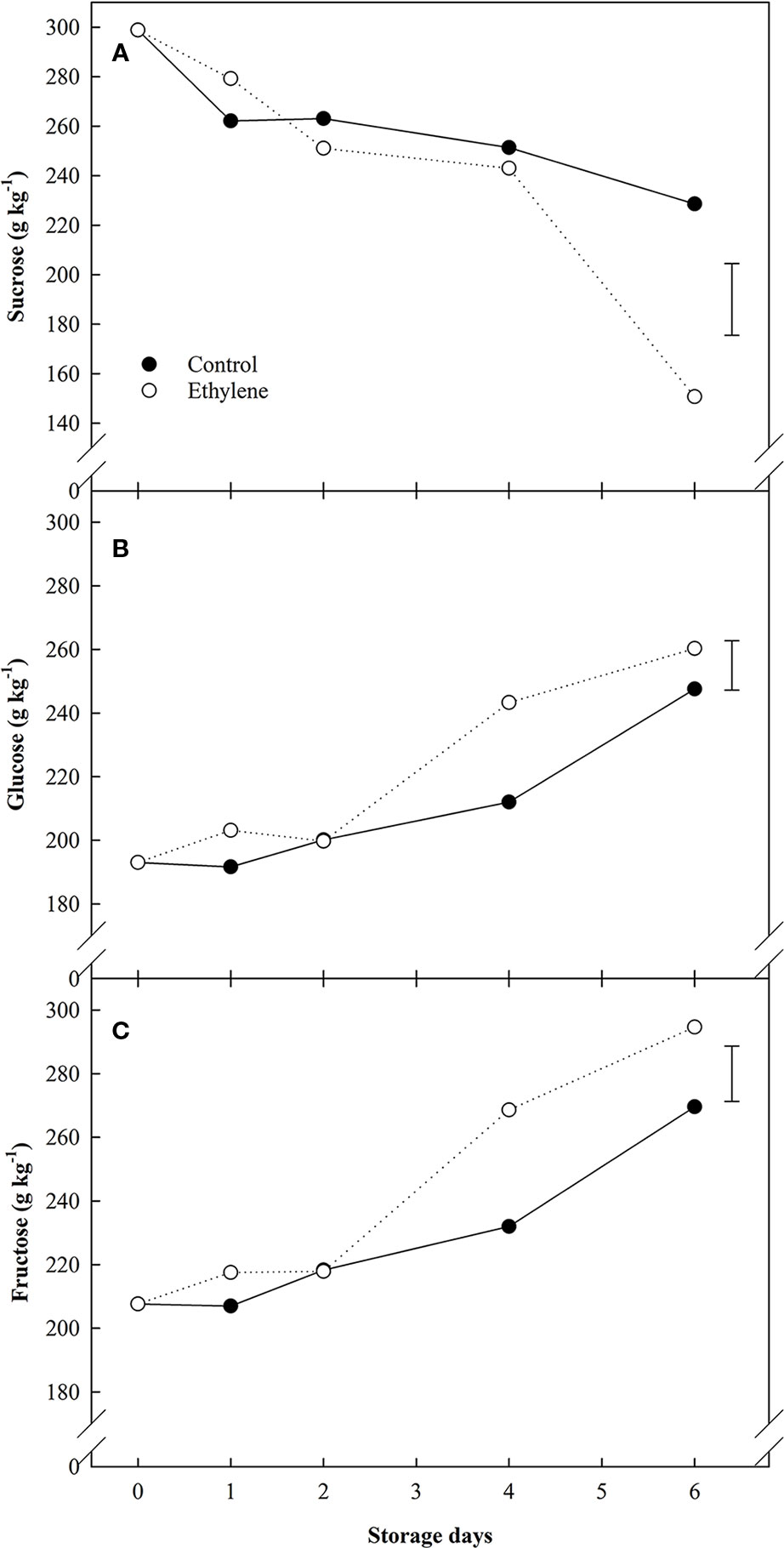
Figure 2 The effect of ethylene (continuous exposure) on sugars content (g kg−1) of strawberries, cv. Sonata, stored at 5°C for 6 d. (A) Sucrose content. (B) Glucose content. (C) Fructose content. Vertical bar represents LSD (p <0.05) for the overall means of the significant variable “treatment”.
Of the organic acids analyzed, ethylene only had a significant impact on malic acid, and this effect was similar to the response for sucrose in treated fruit (Figure 3A). Citric and ascorbic acid content were affected by the storage time only (Figure 3B). At the beginning of storage, the most abundant organic acid was citric acid (67 g kg−1), followed by the malic (38 g kg−1), oxalic (6.7 g kg−1) and ascorbic acid (4.3 g kg−1), respectively; despite these variations in initial organic acids levels, an overall decline was observed at the end of the storage.
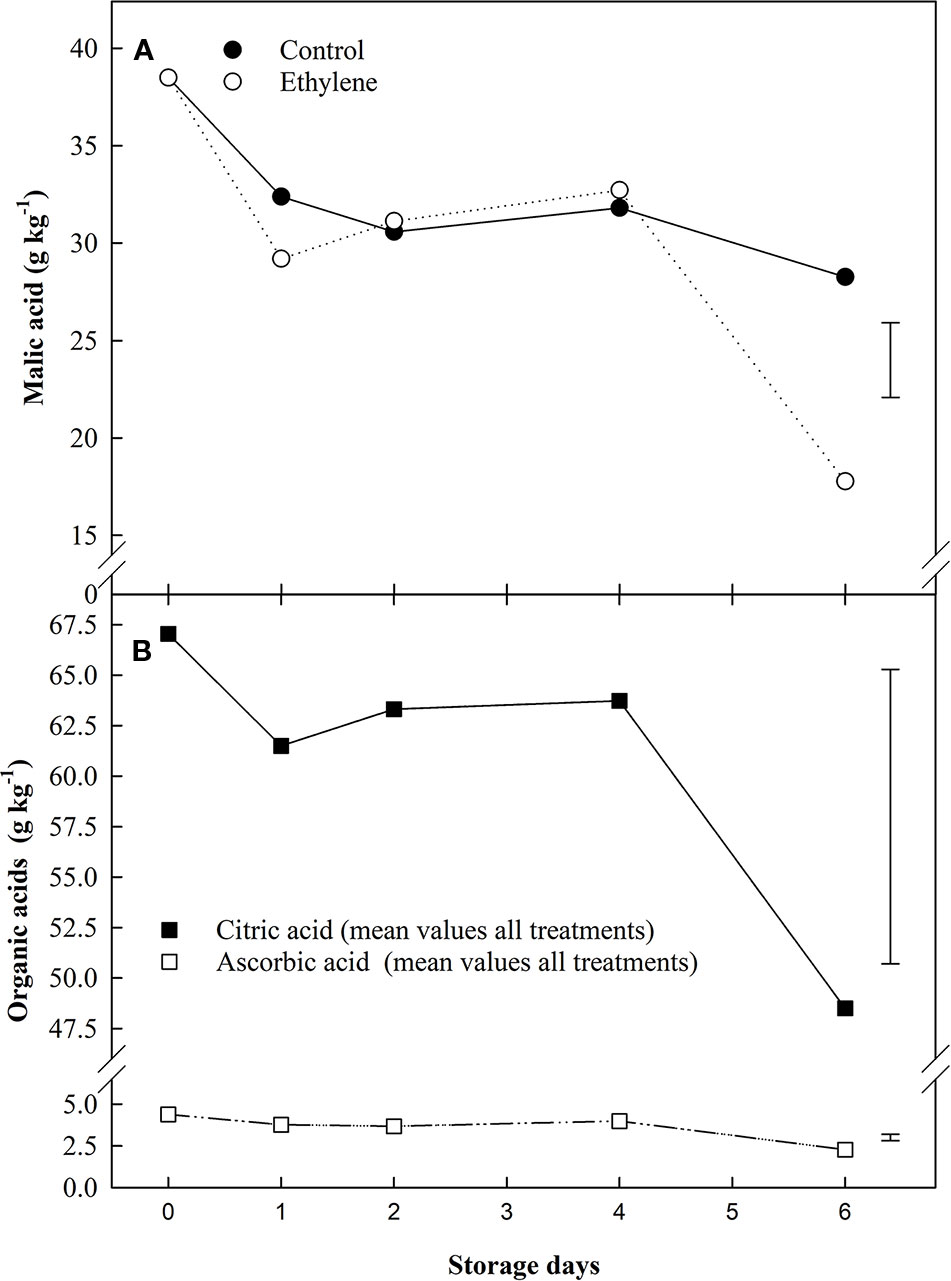
Figure 3 The effect of ethylene (continuous exposure) on organic acids content (g kg−1) of strawberries, cv. Sonata, stored at 5°C for 6 d. (A) Malic acid content (g kg−1) of strawberries, cv. Sonata, stored at 5°C for 6 d. Vertical bar represents LSD (p < 0.05) for the overall means of the significant variable “treatment”. (B) Ascorbic and citric acids. The represented values show the mean of all treatments (control and ethylene treated fruit) content. ■ citric acid. □ ascorbic acid. Vertical bar represents LSD (p < 0.05) for the overall means of the significant variable “Storage day”.
Total anthocyanins and three specific anthocyanins, cyanidin-3-glucoside, pelargonidin-3-glucoside, and pelargonidin-3-glucoside derivative, were analyzed. Significant differences were identified for total anthocyanins and pelargonidin-3-glucoside content, and were due to the storage day only (p < 0.0203 and p < 0.0047, respectively). Both compounds ca. increased 1.1-fold during storage without marked differences between ethylene and air (Supplemental Figure 1).
Besides anthocyanins, other phenolic compounds were also quantified (quercetin-3-glucoside, catechin, epicatechin, chlorogenic and ellagic acids). Among them, quercetin-3-glucose did not show any significant difference; catechin and ellagic acid showed quite steady levels during all the storage (400–450 mg kg−1 and 35.8–36.1 mg kg−1, respectively. Data not shown). In contrast, epicatechin and chlorogenic acid levels were significantly affected by ethylene supplementation (Figure 4). Ethylene induced a gradual accumulation of epicatechin in the first four days of ethylene exposure (Figure 4A), while a sudden increase was observed for chlorogenic acid from day 1 until the end of storage (50% more than untreated fruit) (Figure 4B).
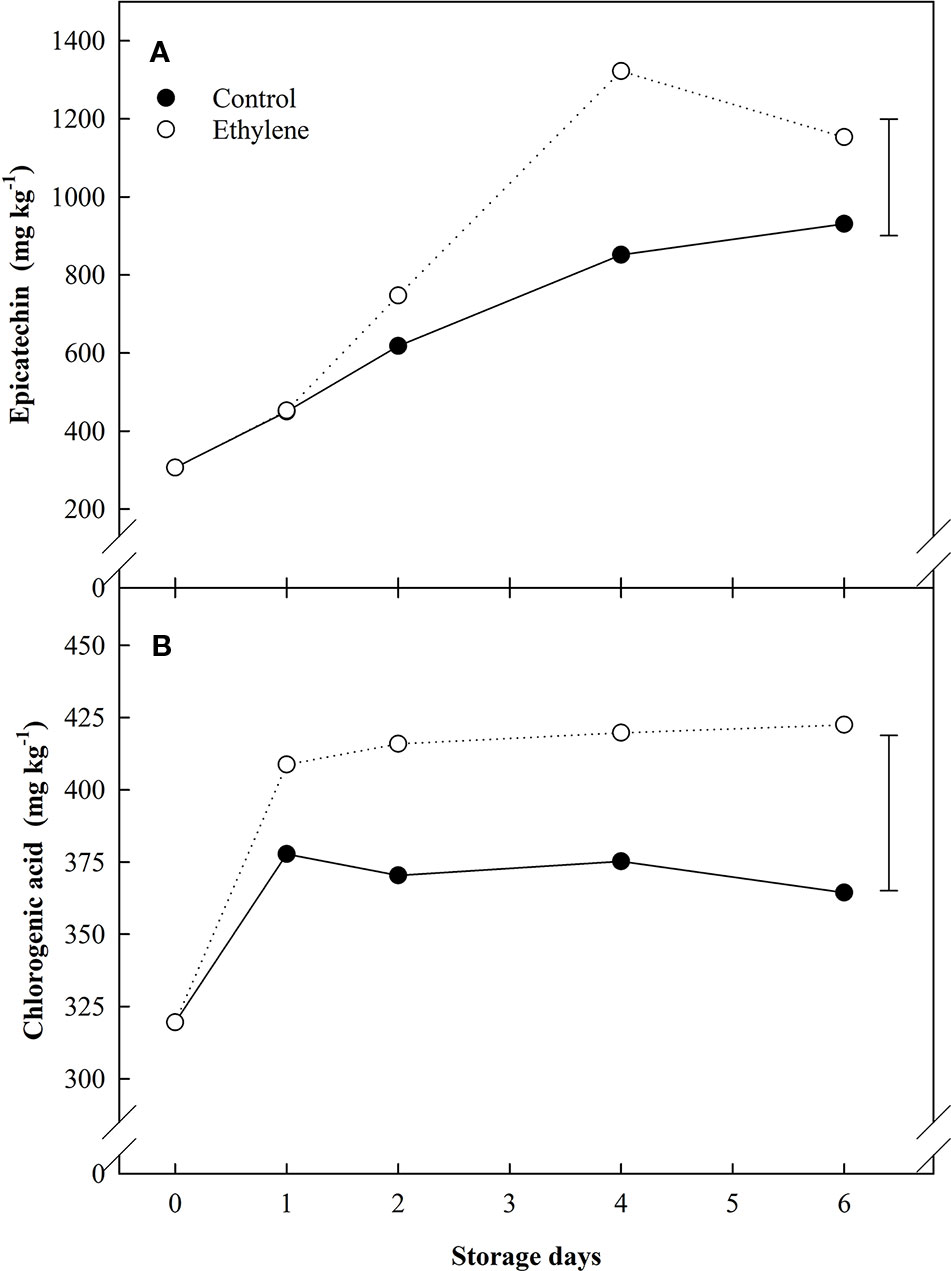
Figure 4 The effect of ethylene (continuous exposure) on phenolics content (mg kg−1) of strawberries, cv. Sonata, stored at 5°C for 6 d. (A) Epicatechin. (B) Cholorogenic acid. Vertical bar represents LSD (p < 0.05) for the overall means of the significant variable “treatment”.
Phytohormones
The effect of continuous ethylene exposure on phytohormone profile was studied. The ex-situ (20°C) monitoring of endogenous ethylene production showed a significant difference only according to the storage time, and overall the endogenous ethylene production increased during storage by 2.6-fold (Supplemental Figure 2).
The quantification of two phytohormones and two metabolites thereof [abscisic acid (ABA), 7'OH-abscisic acid (7'-OHABA), indole-3-acetic acid (IAA), indole-3-acetylaspartic acid (IAAsp)] was carried out, using LC-MSMS, in the whole strawberry fruit as well as in individual tissues. Continuous ethylene exposure increased ABA content in the whole fruit and in the receptacle throughout storage (Figure 5A). ABA content was 4-times higher in receptacle tissue (only) than achenes at the beginning of storage, and the ethylene-induced ABA increase in the receptacle showed a steady difference of ca. 1.2-fold from day 1 in comparison with the untreated fruit content (Figure 5B). Achenes did not exhibit a response to ethylene, yet catabolism of ABA and auxins increased by two thirds during air storage (Figure 6).
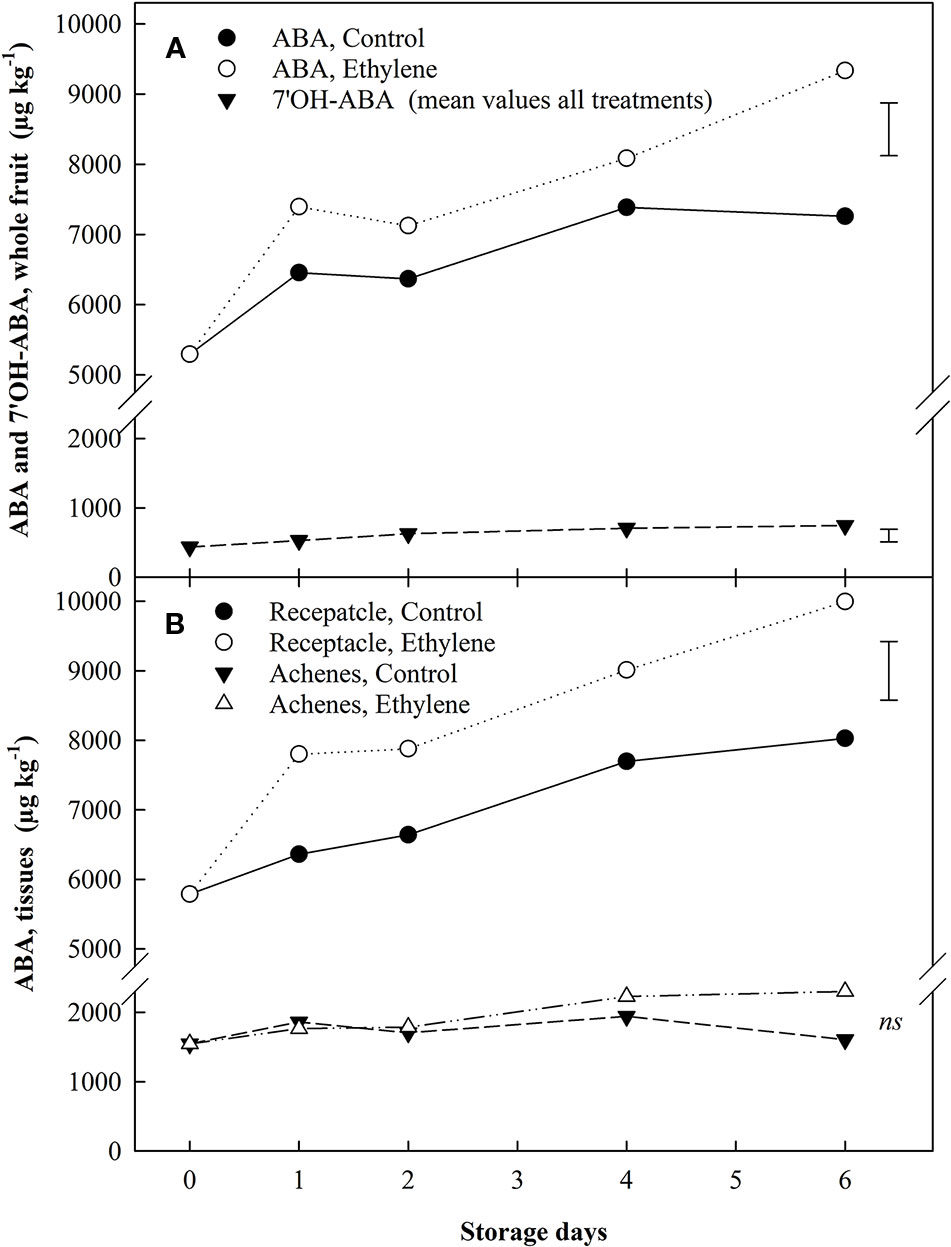
Figure 5 The effect of ethylene (continuous exposure) on ABA and the metabolite 7'OH-ABA content (µg kg−1) of strawberries, cv. Sonata, stored at 5°C for 6 d. (A), ABA and the metabolite 7'OH-ABA content in the whole fruit. For the metabolite 7'OH-ABA the represented values show the mean of all treatments (control and ethylene treated fruit) content. (B), ABA in individual tissues. Vertical bar represents LSD (p < 0.05) for the overall means of the significant variable “treatment” for ABA in both graphs and of the significant variable “Storage day” for 7'OH-ABA (whole fruit); ns, not significant.
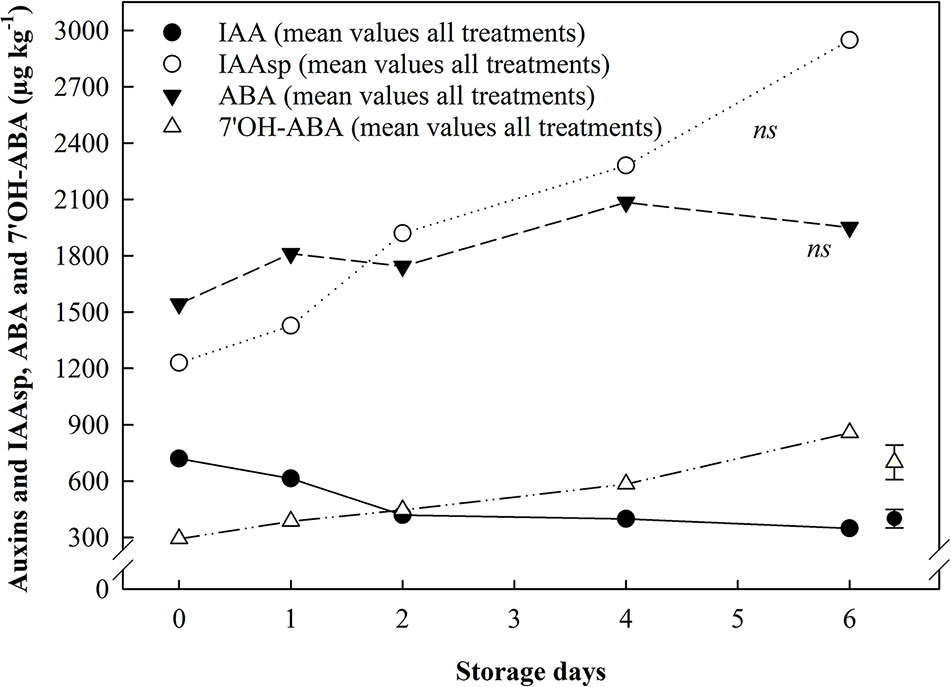
Figure 6 The effect of ethylene (continuous exposure) on auxins and ABA, and their catabolites, content (µg kg−1) in achenes of strawberries, cv. Sonata, stored at 5°C for 6 d. The represented values show the mean of all treatments (control and ethylene treated fruit) content. Vertical bar represents LSD (p <0.05) for the overall means of the significant variable “Storage day” for IAA and 7'OH-ABA; ns, not significant.
7'OH-ABA content was more affected by storage duration than by ethylene treatment. An overall increase in 7'OH-ABA was found in all the tissues analyzed. 7 OH-ABA levels in whole fruit showed a mean increase of 1.71-fold (Figure 5A), while in the receptacle tissues no significant differences were identified (data not shown). In the achenes, 7'OH-ABA exhibited ca. 3-fold increase compared to the beginning of storage (Figure 6).
Auxins (IAA and IAAsp) content were detected only in the achenes, since the concentration of these phytohormones in the whole fruit and in the receptacle was below the quantification limit. IAA levels showed significant variations relating to storage days only. An overall 2-fold decrease in IAA content was reported across storage, although the major changes happened during the first two days. IAAsp exhibited an increasing trend (ca. 2.4-fold) in both control and ethylene treated fruit but a significant variation was not identified (Figure 6).
Discussion
Previous studies investigating the effect of high level of ethylene (100 µl l−1) did not report a clear mechanism of response in cold stored strawberry (Siriphanich, 1980; Terry et al., 2007a). As consequence, it has been suggested that high concentration of ethylene would not impact on strawberry shelf life, while very low concentration (<1 µl l−1) have been reported to promote decay and softening (Wills and Kim, 1995). To better understand whether and how high level of ethylene can affect the postharvest quality of strawberry, the current work describes the responses induced by continuous ethylene exposure (50 µl l−1) in cold stored ripe strawberry.
Strawberry is classified as a non-climacteric fruit even though an increasing number of studies highlight that the receptacle exhibits some ripening features similar to a climacteric fruit. Although strawberry does not show the typical ethylene climacteric rise in respiration, it has been reported that ethylene biosynthesis and signaling pathways are differentially affected during strawberry ripening, and these changes are mainly localized in the receptacle (Trainotti et al., 2005; Iannetta et al., 2006; Merchante et al., 2013; Sun et al., 2013; Sánchez-Sevilla et al., 2017). Besides this transcriptional activation, the role of ethylene in modulating sugars/organic acid ratio, promoting anthocyanins accumulation and softening during strawberry ripening has been reported (Villarreal et al., 2010; Merchante et al., 2013; Villareal et al., 2016; Gu et al., 2019; Moya-León et al., 2019). Ethylene has been linked with increased CO2 production and water loss in ripening strawberry fruit (Tian et al., 2000); this being in agreement with results herein. Opazo and colleagues (2013) reported differential effects of ethylene and 1-MCP on the expression of the softening-related gene xyloglucan endotransglycosylase/hydrolase 1 (XTH1), due to the presence of ethylene responsive elements in the promoter region. The finding was confirmed by Villareal and colleagues (2016) who described the response to ethylene of several strawberry cell-wall metabolic genes. It has also been extensively reported that ethylene treatment elicited anthocyanins accumulation in ripening strawberry (Ku et al., 1999; Bower et al., 2003; Villarreal et al., 2010; Merchante et al., 2013; Sun et al., 2013; Lopes et al., 2015). In addition, Sun and colleagues (2013) demonstrated that the expression of FaSAMS1(S-adenosyl-L-methionine synthase 1) and FaCTR1 (constitutive triple response 1) genes played a significant role in the softening and red-color development of strawberry fruit. The results herein did not show any effect of ethylene exposure on anthocyanins content; this difference may be explained since the fruit used in the current study were already at red ripe stage. In addition, the fruit were kept in cold storage, rather than 20°C, as indicated in previous works.
Lopes and colleagues (2015) reported that ethephon treatment increased sugars content in strawberry fruit both when applied in the field, and during postharvest. The results herein confirmed the propensity of ethylene to elicit an accumulation of reducing sugars, together with a concomitant decline in sucrose content during postharvest, as previously described (Li et al., 2019). In blueberry, ethylene can positively influence sucrose metabolism and invertase enzymes activity (Wang et al., 2020), and Jia and colleagues (2016) reported the key role of acid invertase during strawberry ripening. This may explain the activation of sucrose hydrolysis described here. Nevertheless, the taste and quality of strawberry fruit are linked to the sugars/organic acids ratio rather than sugars content alone (Giné-Bordonaba and Terry, 2009). Although both citric and malic acids are important compounds influencing strawberry acidity, malic acid is the main acid responsible for changes in pH regulation (Fait et al., 2008). The data of this work showed that malic acid catabolism was positively affected by continuous ethylene exposure, and developed concomitantly with the sucrose decline. These similar variations of malic acid and sucrose, and the role of malic acid in pH regulation seemed to suggest an ethylene-induced upregulation of different members of the invertase family activated by the changes in pH, and/or an upregulation of invertase members not localized in the cytoplasm (e.g. cell wall invertase). All these changes in water loss, respiration, and variations of fruit pH and reducing sugars can be linked to the progression of senescence (Alkan and Fortes, 2015). Taken together, the data supports the role of ethylene in promoting senescence in strawberry fruit.
It is well documented that the ABA/auxins ratio plays a major role in regulating strawberry fruit ripening (Iannetta et al., 2006; Jia et al., 2011; Symons et al., 2012; Chen et al., 2015; Chen et al., 2016; Medina-Puche et al., 2016; Moya-León et al., 2019); despite this, there is a lack of information about the role of ABA during storage (Terry et al., 2007a). Results of this study highlighted that continuous exposure to exogenous ethylene did not affect ABA and auxins metabolism in achenes. In contrast, ethylene induced a marked increase in ABA content in the receptacle as a first response (within 24 h), which was sustained through storage (50% higher ABA content than untreated fruit at the end of the storage). In the same tissue, auxins and IAAsp levels were too low to be detectable, and the ABA metabolite 7'OH-ABA did not show any response to ethylene exposure. A study on hormonal content in different strawberry fruit tissues during ripening reported higher ABA amounts in the achenes than in the receptacle (Symons et al., 2012). However, the more recent work of Gu and colleagues (2019) highlighted that ABA content in the receptacle markedly increased with the progression of the ripening and reached a maximum level only at the red stage. The presented data confirmed that in ripe (red) strawberry, ABA concentration 4-times higher in the receptacle tissue compared to achenes through storage. Furthermore, continuous ethylene exposure induced a constant upregulation of the ABA receptacle content (1.24-fold) compared to the control. These findings seemed to suggest a synergistic action of ethylene and ABA: ethylene-induced disequilibrium in the receptacle ABA homeostasis might be a trigger for, and participate in, the regulation of the senescence-related changes observed during storage.
Li and colleagues (2014) described that following UV-C stress, ABA can induce ethylene biosynthesis in unripe detached strawberry, and that ABA levels were inversely correlated to ethylene content. In contrast, the presented data showed a positive correlation between ethylene exposure and ABA content. Moreover, the increasing trend exhibited by ABA throughout storage indicated that the selected ethylene concentration (50 µl l−1) was able to keep a positive regulation on ABA accumulation in the receptacle tissue.
ABA is well-known to be also involved in water stress, and following deficit irrigation an increase in phenolic compounds was found in strawberry fruit (Terry et al., 2007b; Weber et al., 2016). Accordingly, samples exposed to ethylene showed higher weight loss, higher ABA content, and higher phenolics accumulation than untreated fruit. This positive effect of ABA on the flavonoid/phenylpropanoid pathway has been explained with the ABA-induced upregulation of FaSCL8 (SCARECROW-LIKE 8) and FaMYB10 expression (Medina-Puche et al., 2014; Pillet et al., 2015). These two genes have been proposed as key elements for the flavonoid/phenylpropanoid pathway regulation during the ripening of strawberry fruit. The results of the present study showed that epicatechin and chlorogenic acid content were positively affected by ethylene supplementation. Moreover, the sharp increase found in chlorogenic acid at day 1 of storage, and during storage resembled the trend found in ABA content following ethylene exposure. This seemed to support the idea that there is interplay between ethylene and ABA, and that both phytohormones regulate strawberry ripening/senescence during postharvest (Supplemental Figure 3).
In conclusion, in this study it is proposed that strawberry postharvest senescent decline is induced by ethylene through ABA action in the receptacle tissue, and that a better understanding of the role of ethylene and ABA and their interplay in mediating senescence would allow better design of shelf life management strategies.
Data Availability Statement
Data underlying this paper can be accessed at https://doi.org/10.17862/cranfield.rd.11876544.v1.
Author Contributions
LT conceived and designed the experiments. FE, IP, and KC performed the experiments. RT performed data analysis and wrote the manuscript with the help of LT. All authors contributed to the discussion, revised and approved the final manuscript.
Funding
Engineering and Physical Sciences Research Council (EPSRC) and Johnson Matthey Plc (case number: 09000987).
Conflict of Interest
The authors declare that the research was conducted in the absence of any commercial or financial relationships that could be construed as a potential conflict of interest.
The reviewer AL declared a past co-authorship with one of the authors LT to the handling Editor.
Acknowledgments
The authors would like to thank both the Engineering and Physical Sciences Research Council (EPSRC; 09000987) and Johnson Matthey Plc for financial and Case studentship support. IP would like to thank the Institute of Agricultural and Fisheries Research and Training (IFAPA) and the European Social Fund (Programa Operativo del Fondo Social Europeo de Andalucía 2007-2013) for the postdoctoral fellowship.
Supplementary Material
The Supplementary Material for this article can be found online at: https://www.frontiersin.org/articles/10.3389/fpls.2020.00174/full#supplementary-material
Supplemental Figure 1 | The effect of ethylene (continuous exposure) on total anthocyanins and pelargonidin-3-glucoside content (mg kg-1) of strawberries, cv. Sonata, stored at 5˚ C for 6 d. The represented values show the mean (control and ethylene treated fruit) content. Vertical bar represents LSD (p < 0.05) for the overall means of the significant variable “Storage day”.
Supplemental Figure 2 | The effect of ethylene (continuous exposure) on endogenous ethylene production (x10-11 g kg-1 s-1) of strawberries, cv. Sonata, stored at 5˚ C for 6 d. Vertical bar represents LSD (p < 0.05) for the overall means of the significant variable “Storage day”.
Supplemental Figure 3 | Graphical abstract of ethylene (continuous exposure) effect on senescence in receptacle and achene tissues in strawberry fruit.
References
Alamar, M. C., Colling, E., Cools, K., Terry, L. A. (2017). Impact of controlled atmosphere scheduling on strawberry and imported avocado fruit. Postharvest Biol. Technol. 134, 6–86. doi: 10.1016/j.postharvbio.2017.08.003
Alkan, N., Fortes, A. M. (2015). Insights into molecular and metabolic events associated with fruit response to post-harvest fungal pathogens. Front. Plant Sci. 6, 1–14. doi: 10.3389/fpls.2015.00889
Amoah, R. S., Landahl, S., Terry, L. A. (2017). Design and construction of a flexible laboratory-scale mixing apparatus for continuous ethylene supplementation of fresh produce. Biosyst. Eng. 157, 86–91. doi: 10.1016/j.biosystemseng.2017.02.010
Bower, J. H., Biasi, W. V., Mitcham, E. J. (2003). Effects of ethylene and 1-MCP on the quality and storage life of strawberries. Postharvest Biol. Technol. 28, 417–423. doi: 10.1002/jsfa.3868
Chang, K. N., Zhong, S., Weirauch, M. T., Hon, G., Pelizzola, M., Li, H., et al. (2013). Temporal transcriptional response to ethylene gas drives growth hormone cross-regulation in Arabidopsis. Elife 2013, 1–20. doi: 10.7554/eLife.00675.001
Chen, J., Mao, L., Lu, W., Ying, T., Luo, Z. (2015). Transcriptome profiling of postharvest strawberry fruit in response to exogenous auxin and abscisic acid. Planta 243, 183–197. doi: 10.1007/s00425-015-2402-5
Chen, J., Mao, L., Mi, H., Lu, W., Ying, T., Luo, Z. (2016). Involvement of abscisic acid in postharvest water-deficit stress associated with the accumulation of anthocyanins in strawberry fruit. Postharvest Biol. Technol. 111, 99–105. doi: 10.1016/j.postharvbio.2015.08.003
Csukasi, F., Osorio, S., Gutierrez, J. R., Kitamura, J., Giavalisco, P., Nakajima, M., et al. (2011). Gibberellin biosynthesis and signalling during development of the strawberry receptacle. New Phytol. 191, 376–390. doi: 10.1111/j.1469-8137.2011.03700.x
Fait, A., Hanhineva, K., Beleggia, R., Dai, N., Rogachev, I., Nikiforova, V. J., et al. (2008). Reconfiguration of the achene and receptacle metabolic networks during strawberry fruit development. Plant Physiol. 148, 730–750. doi: 10.1104/pp.108.120691
Giné-Bordonaba, J., Terry, L. A. (2009). Development of a glucose biosensor for rapid assessment of strawberry quality: relationship between biosensor response and fruit composition. J. Agric. Food Chem. 57, 8220–8226. doi: 10.1021/jf901596w
Giné-Bordonaba, J., Terry, L. A. (2016). Effect of deficit irrigation and methyl jasmonate application on the composition of strawberry (Fragaria × ananassa) fruit and leaves. Sci. Hortic. 199, 63–70. doi: 10.1016/j.scienta.2015.12.026
Gu, T., Jia, S., Huang, X., Wang, L., Fu, W., Hou, G., et al. (2019). Transcriptome and hormone analyses provide insights into hormonal regulation in strawberry ripening. Planta ,250, 145–162. doi: 10.1007/s00425-019-03155-w
Iannetta, P. P. M., Laarhoven, L. J., Medina-Escobar, N., James, E. K., McManus, M. T., Davies, H. V., et al. (2006). Ethylene and carbon dioxide production by developing strawberries show a correlative pattern that is indicative of ripening climacteric fruit. Physiol. Plant 127, 247–259. doi: 10.1111/j.1399-3054.2006.00656.x
Jia, H.-F., Chai, Y.-M., Li, C.-L., Lu, D., Luo, J.-J., Qin, L., et al. (2011). Abscisic acid plays an important role in the regulation of strawberry fruit ripening. Plant Physiol. 157, 188–199. doi: 10.1104/pp.111.177311
Jia, H., Jiu, S., Zhang, C., Wang, C., Tariq, P., Liu, Z., et al. (2016). Abscisic acid and sucrose regulate tomato and strawberry fruit ripening through the abscisic acid-stress ripening transcription factor. Plant Biotechnol. J. 14, 2045–2065. doi: 10.1111/pbi.12563
Jiang, Y., Joyce, D. C., Terry, L. A. (2001). 1-Methylcyclopropene treatment affects strawberry fruit decay. Postharvest Biol. Technol. 23 (3), 227–232. doi: 10.1016/S0925-5214(01)00123-5
Ku, V. V. V., Wills, R. B. H., Ben-Yehoshua, S. (1999). 1-Methylcyclopropene can differentially affect the postharvest life of strawberries exposed to ethylene. HortScience 34, 119–120. doi: 10.21273/HORTSCI.34.1.119
Leng, P., Yuan, B., Guo, Y., Chen, P. (2014). The role of abscisic acid in fruit ripening and responses to abiotic stress. J. Exp. Bot. 65, 4577–4588. doi: 10.1093/jxb/eru204
Li, D., Luo, Z., Mou, W., Wang, Y., Ying, T., Mao, L. (2014). ABA and UV-C effects on quality, antioxidant capacity and anthocyanin content of strawberry fruit (Fragaria × ananassa Duch.). Postharvest Biol. Technol. 90, 56–62. doi: 10.1016/j.postharvbio.2013.12.006
Li, L., Lichter, A., Chalupowicz, D., Gamrasni, D., Goldberg, T., Nerya, O., et al. (2016a). Effects of the ethylene-action inhibitor 1-methylcyclopropene on postharvest quality of non-climacteric fruit crops. Postharvest Biol. Technol. 111, 322–329. doi: 10.1016/j.postharvbio.2015.09.031
Li, L., Li, D., Luo, Z., Huang, X., Li, X. (2016b). Proteomic response and quality maintenance in postharvest fruit of strawberry (Fragaria × ananassa) to exogenous cytokinin. Sci. Rep. 6, 27094. doi: 10.1038/srep27094
Li, D., Zhang, X., Xu, Y., Li, L., Aghdam, M. S., Luo, Z. (2019). Effect of exogenous sucrose on anthocyanin synthesis in postharvest strawberry fruit. Food Chem. 289, 112–120. doi: 10.1016/j.foodchem.2019.03.042
Lopes, P. Z., Fornazzari, I. M., Almeida, A. T., Galvão, C. W., Etto, R. M., Inaba, J. (2015). Effect of ethylene treatment on phytochemical and ethylene-related gene expression during ripening in strawberry fruit Fragaria x ananassa cv. Camino Real. Genet. Mol. Res. 14, 16113–16125. doi: 10.4238/2015.December.7.23
Medina-Puche, L., Cumplido-Laso, G., Amil-Ruiz, F., Hoffmann, T., Ring, L., Rodríguez-Franco, A., et al. (2014). MYB10 plays a major role in the regulation of flavonoid/phenylpropanoid metabolism during ripening of Fragaria × ananassa fruits. J. Exp. Bot. 65, 401–417. doi: 10.1093/jxb/ert377
Medina-Puche, L., Blanco-Portales, R., Molina-Hidalgo, F. J., Cumplido-Laso, G., García-Caparrós, N., Moyano-Cañete, E., et al. (2016). Extensive transcriptomic studies on the roles played by abscisic acid and auxins in the development and ripening of strawberry fruits. Funct. Integr. Genomics 16, 671–692. doi: 10.1007/s10142-016-0510-3
Merchante, C., Vallarino, J. G., Osorio, S., Aragüez, I., Villarreal, N., Ariza, M. T., et al. (2013). Ethylene is involved in strawberry fruit ripening in an organ-specific manner. J. Exp. Bot. 64, 4421–4439. doi: 10.1093/jxb/ert257
Moya-León, M. A., Mattus-Araya, E., Herrera, R. (2019). Molecular events occurring during softening of strawberry fruit. Front. Plant Sci. 10, 615. doi: 10.3389/fpls.2019.00615
Opazo, M. C., Lizana, R., Pimentel, P., Herrera, R., Moya-León, M. A. (2013). Changes in the mRNA abundance of FcXTH1 and FcXTH2 promoted by hormonal treatments of Fragaria chiloensis fruit. Postharvest Biol. Technol. 77, 28–34. doi: 10.1016/j.postharvbio.2012.11.007
Ordaz-Ortiz, J. J., Foukaraki, S., Terry, L. A. (2015). Assessing temporal flux of plant hormones in stored processing potatoes using high definition accurate mass spectrometry. Hortic. Res. 2, 15002. doi: 10.1038/hortres.2015.2
Pillet, J., Yu, H. W., Chambers, A. H., Whitaker, V. M., Folta, K. M. (2015). Identification of candidate flavonoid pathway genes using transcriptome correlation network analysis in ripe strawberry (Fragaria × ananassa) fruits. J. Exp. Bot. 66, 4455–4467. doi: 10.1093/jxb/erv205
Sánchez-Sevilla, J. F., Vallarino, J. G., Osorio, S., Bonbarely, A., Posé, D., Merchante., C., et al. (2017). Gene expression atlas of fruit ripening and transcriptome assembly from RNA-seq data in octoploid strawberry (Fragaria × ananassa). Sci. Rep. 7, 13737. doi: 10.1038/s41598-017-14239-6
Salman, A., Filgueiras, H., Cristescu, S., Lopez-Lauri, F., Harren, F., Sallanon, H. (2009). Inhibition of wound-induced ethylene does not prevent red discoloration in fresh-cut endive (Cichoriumintybus L.). Eur. Food Res. Technol. 228, 651–657. doi: 10.1007/s00217-008-0974-z
Siriphanich, J. (1980). Postharvest deterioration of strawberries as influenced by ethylene and some other volatiles. MS Thesis, University of California, 65.
Sun, J.-H., Luo, J.-J., Tian, L., Li, C.-L., Xing, Y., Shen, Y.-Y. (2013). New evidence for the role of ethylene in strawberry fruit ripening. J. Plant Growth Regul. 32, 461–470. doi: 10.1007/s00344-012-9312-6
Symons, G. M., Chua, Y.-J., Quittenden, L. J., Davies, N. W., Reid, J. B. (2012). Hormonal changes during non-climacteric ripening in strawberry. J. Exp. Bot. 63, 4741–4750. doi: 10.1093/jxb/ers147
Terry, L. A., Ilkenhans, T., Poulston, S., Rowsell, L., Smith, A. W. J. (2007a). Development of new palladium-promoted ethylene scavenger. Postharvest Biol. Technol. 45, 214–220. doi: 10.1016/j.postharvbio.2006.11.020
Terry, L. A., Chope, G. A., Giné-Bordonaba, J. (2007b). Effect of water deficit irrigation and inoculation with Botrytis cinerea on strawberry (Fragaria × ananassa) fruit quality. J. Agric. Food Chem. 55, 10812–10819. doi: 10.1021/jf072101n
Terry, L., Mena, C., Williams, A., Jenney, N., Whitehead, P. (2011). Fruit and vegetable resource maps: Mapping fruit and vegetable waste through the wholesale supply chain. Wrap 1–94.
Tian, M. S., Prakash, S., Elgar, H. J., Young, H., Burmeister, D. M., Ross, G. S. (2000). Responses of strawberry fruit to 1-methylcyclopropene (1-MCP) and ethylene. Plant Growth Regul. 32 (1), 83–90. doi: 10.1023/A:1006409719333
Trainotti, L., Pavanello, A., Casadoro, G. (2005). Different ethylene receptors show an increased expression during the ripening of strawberries: does such an increment imply a role for ethylene in the ripening of these non-climacteric fruits? J. Exp. Bot. 56, 2037–2046. doi: 10.1093/jxb/eri202
Villareal, N. M., Marina, M., Nardi, C. F., Civello, P. M., Martínez, G. A. (2016). Novel insights of ethylene role in strawberry cell wall metabolism. Plant Sci. 252, 1–11. doi: 10.1016/j.plantsci.2016.06.018
Villarreal, N. M., Bustamante, C. A., Civello, P. M., Martínez, G. A. (2010). Effect of ethylene and 1-MCP treatments on strawberry fruit ripening. J. Sci. Food Agric. 90, 683–689. doi: 10.1002/jsfa.3868
Wang, S., Zhou, Q., Zhou, X., Zhang, F., Ji, S. (2020). Ethylene plays an important role in the softening and sucrose metabolism of blueberries postharvest. Food Chem. 310, 125965. doi: 10.1016/j.foodchem.2019.125965
Weber, N., Zupanc, V., Jakopic, J., Veberic, R., Mikulic-Petkovsek, M., Stampar, F. (2016). Influence of deficit irrigation on strawberry (Fragaria × ananassa Duch.) fruit quality. J. Sci. Food Agric. 97, 849–857 doi: 10.1002/jsfa.7806
Keywords: abscisic acid homeostasis, invertase, non-climacteric fruit, senescence precursor, ethylene
Citation: Tosetti R, Elmi F, Pradas I, Cools K and Terry LA (2020) Continuous Exposure to Ethylene Differentially Affects Senescence in Receptacle and Achene Tissues in Strawberry Fruit. Front. Plant Sci. 11:174. doi: 10.3389/fpls.2020.00174
Received: 28 November 2019; Accepted: 05 February 2020;
Published: 12 March 2020.
Edited by:
Brian Farneti, Fondazione Edmund Mach, ItalyReviewed by:
Lida Fuentes-Viveros, Centro Regional de Estudios en Alimentos Saludables-CREAS, ChileAmnon Lichter, Agricultural Research Organization (ARO), Israel
Copyright © 2020 Tosetti, Elmi, Pradas, Cools and Terry. This is an open-access article distributed under the terms of the Creative Commons Attribution License (CC BY). The use, distribution or reproduction in other forums is permitted, provided the original author(s) and the copyright owner(s) are credited and that the original publication in this journal is cited, in accordance with accepted academic practice. No use, distribution or reproduction is permitted which does not comply with these terms.
*Correspondence: Leon A. Terry, bC5hLnRlcnJ5QGNyYW5maWVsZC5hYy51aw==