- College of Horticulture, Jilin Agricultural University, Changchun, China
In this study, we investigated the mechanism of photosynthesis and physiological function of blueberry leaves under low temperature stress (4–6°C) by exogenous hydrogen sulfide (H2S) by spraying leaves with 0.5 mmol·L–1 NaHS (H2S donor) and 200 μmol·L–1 hypotaurine (Hypotaurine, H2S scavenger). The results showed that chlorophyll and carotenoid content in blueberry leaves decreased under low temperature stress, and the photochemical activities of photosystem II (PSII) and photosystem I (PSI) were also inhibited. Low temperature stress can reduce photosynthetic carbon assimilation capacity by inhibiting stomatal conductance (Gs) of blueberry leaves, and non-stomatal factors also play a limiting role at the 5th day of low temperature stress. Low temperature stress leads to the accumulation of Pro and H2O2 in blueberry leaves and increases membrane peroxidation. Spraying leaves with NaHS, a donor of exogenous H2S, could alleviate the degradation of chlorophyll and carotenoids in blueberry leaves caused by low temperature and reduce the photoinhibition of PSII and PSI. The main reason for the enhancement of photochemical activity of PSII was that exogenous H2S promoted the electron transfer from QA to QB on PSII acceptor side under low temperature stress. In addition, it promoted the accumulation of osmotic regulator proline under low temperature stress and significantly alleviated membrane peroxidation. H2S scavengers (Hypotaurine) aggravated photoinhibition and the degree of oxidative damage under low temperature stress. Improving photosynthetic capacity as well as alleviating photosynthetic inhibition and oxidative stress with exogenous H2S is possible in blueberry seedlings under low temperature stress.
Introduction
Hydrogen sulfide H2S has dual effects on plant growth and development. A high concentration of H2S can cause cytotoxicity, while a low concentration of H2S does not cause toxicity to plants and may act as a signaling molecule (Duan et al., 2015; Li et al., 2016). Recently, many studies have found that H2S can regulate plant growth and development, such as inducing plant seed germination (Liu and Lal, 2015), improving photosynthetic capacity (Coyne and Bingham, 1978; Chen et al., 2011), regulating stomatal movement (Lisjak et al., 2011; Scuffi et al., 2014), promoting the development of lateral roots (Jia et al., 2015; Fang T. et al., 2014), regulating secondary metabolism of sugar, polyamines, organic acids and amino acids (Shi et al., 2015; Chen et al., 2016), participating in protein modification (Mustafa et al., 2009), maintaining ion balance in plants (Wang et al., 2012; Lai et al., 2014), delaying ripening and senescence of postharvest fruits during storage (Fu et al., 2014; Hu et al., 2014), and improving antioxidant capacity (Luo et al., 2015). In addition, H2S has been shown to participate in the regulation of resistance (Hua et al., 2010; Christou, 2013; Jin et al., 2018). The application of exogenous H2S could promote plant growth and seed germination (Zhang et al., 2010a), increase the survival and regeneration ability of Nicotiana tabacum cells under heat stress, alleviate cell electrolyte leakage and malondialdehyde (MDA) accumulation after heat shock (Li et al., 2012), and alleviate the inhibition of heavy metal stress on plant root growth (Chen et al., 2013). H2S also interacts with other hormones and signaling substances in plants (Hancock and Whiteman, 2016). H2S can alleviate the inhibition of salt stress on the growth of Medicago sativa seedlings and is closely related to the increase of NO content (Wang et al., 2012). Under lead stress, H2S and NO can improve the antioxidant system and mineral balance of sesame by interacting (Amooaghaie et al., 2017). H2S can be used as upstream signaling molecule of H2O2 to promote mung bean (Vigna radiata) seed germination (Li and He, 2015). H2S can be used as a signal molecule of salicylic acid (SA) to participate in Cd tolerance in Arabidopsis thaliana (Qiao et al., 2015). H2S has a complex relationship with Ca2+ in regulating abiotic stressors such as high temperature (Li et al., 2012), Cr6+ (Fang H. et al., 2014), and drought (Jin et al., 2013). Cheng et al. (2013). found that H2S could inhibit the production of reactive oxygen species and ethylene and alleviate the death of Pisum sativum root tip induced by hypoxia by simulating flooding and a hypoxic environment.
Low temperature is one of the most common adversities facing agricultural production in cold regions. Low temperature stress inhibits plant growth and physiological function, which is also related to the decrease of photosystem II (PSII) and photosystem I (PSI) activity (Shen et al., 1990), the limitation of assimilation synthesis (Strauss et al., 2010), the decrease of dark reaction-related enzymes activity, and the disturbance of active oxygen metabolism (Joanna et al., 2019). In the early spring in northern China, blueberries often suffer from low temperature damage, so improving low temperature tolerance is of great significance in the flowering and fruiting stages of blueberries. Sodium hydrosulfide (NaHS) can form H2S in solution, and hypotaurine (Hypotaurine) can scavenge H2S by directly binding with sulfides. Although a large number of studies have proved that exogenous NaHS with appropriate concentration can improve plant resistance to abiotic stresses, there are few studies on H2S improving plant resistance to low temperature, especially on photosynthetic function of blueberry under low temperature stress.Therefore, NaHS and Hypotaurine are often used as the donor and scavenger of H2S, respectively (Wang et al., 2012). In this paper, the effects of exogenous NaHS and Hypotaurine on the photosynthetic function and physiological characteristics of blueberry leaves under simulated low temperature stress were studied. The aim of the study was to explore the mechanism of exogenous H2S regulating the physiological characteristics and photosynthetic function of blueberry leaves under low temperature stress and to provide theoretical basis for improving the low temperature tolerance of blueberry seedlings in the greenhouse and during transplanting.
Materials and Methods
Materials and Treatments
This study was conducted using annual seedlings of Meiden, a lowbush blueberry cultivar with strong cold resistance, which is popular in northern China, at the College of Horticulture, Jilin Agricultural University, Jilin, China in 2018. The seedlings were seeded in pots with a top diameter of 20 cm, a bottom diameter of 16 cm, and a height of 20 cm. The pots were filled with well mixed turf soil and vermiculite (volume ratio 2:1). Plants were grown in an artificial climate chamber with a temperature of 25°C, a light intensity of 400 μmol·m–2·s–1, and a light cycle of 12 h light, 12 h dark.
Thirty seedlings with similar growth were selected for the experiment. The treatment group was sprayed with 0.5 mmol·L–1 NaHS and 200 μmol·L–1 Hypotaurine, respectively, and the control group was treated with distilled water. The leaves were sprayed uniformly on both sides until the solution on the leaves formed fine mist-like droplets. Each treatment contained 10 plants as repeats. After spraying NaHS and Hypotaurine, the droplets on the leaf surface were allowed to dry naturally. After three days, all groups were removed to the temperature controlled growth cabinet and the cabinet was maintained at 4–6°C. Light intensity and humidity were identical for all treatments. Physiological indexes were determined before the treatment (marked as day 0) and at the 2nd and 5th days after the treatment.
Parameters and Methods of Determination
Determination of Fast Chlorophyll Fluorescence Induction Curve (OJIP) and 820 nm Light Reflection Curve
The unfolded penultimate leaves of blueberry in different treatments were selected and dark adapted for 30 min by dark adaptation clips. The OJIP curves and 820 nm light reflection curves of leaves after dark adaptation were measured using a Hansatech M-PEA (Multi-Function Plant Efficiency Analyser). Five repetitions were carried out for each treatment (biological experiments). According to the formulas VO–P=(Ft–Fo)/(FP–Fo) and VO–J=(Ft–Fo)/(FJ–Fo), OJIP curves were standardized by O–P and O–J to obtain VO–P and VO–J curves. The relative variable fluorescence VJ of J point (2 ms) on VO–P curve and the relative variable fluorescence VK of K point (0.3 ms) on the VO–J curve were also defined. In the formula, Ft is the relative fluorescence intensity at each time point on the OJIP curve, while Fo, FJ and FP represent the relative fluorescence intensity at 0.01, 2, and 1,000 ms time points, respectively. The standard VO–P and VO–J curves of blueberry leaves in different treatments were compared with those of CK curves and expressed as △VO–P and △VO–J. A JIP-test analysis was conducted on the OJIP curve to obtain the maximum photochemical efficiency of PSII (Fv/Fm), the performance index of PSII based on absorption (PIABS), and the JIP-test analysis of OJIP curves following the method described by Strasser et al. (1995). The activity of the PSI reaction center is reflected by the relative decrease (△I/Io) of the 820 nm light reflection curve (MR820 nm) signal and the slope of the MR820 nm curve as it descends in the initial stage (1–2.5 ms). Io and △I represent the maximum of the reflected signal and the difference between the maximum and minimum reflected signals in the 820 nm light reflection curve, respectively (Zhang et al., 2018b).
Determination of Photosynthetic Gas Exchange Parameters and Carboxylation Efficiency (CE)
The unfolded penultimate leaves of blueberry in different treatments were selected to measure the photosynthetic gas exchange parameters by Li-6800 photosynthetic system (Licor Corporation, UK). The net photosynthetic rate (Pn), stomatal conductance (Gs), transpiration rate (Tr), and intercellular CO2 concentration (Ci) of blueberry leaves in different treatments were measured under the conditions of 400 μmol·mol–1 CO2 fixed by CO2 cylinder and 1,000 μmol·m–2·s–1 PFD set by built-in light source. The measurements were repeated five times (biological experiments). The light intensity PFD was fixed to 1,500 μmol·m–2·s–1 (saturated light intensity) using the built-in light source of the Li-6400 photosynthetic system. The CO2 concentration (Ci) was controlled by CO2 cylinders to 400, 300, 200, 150, 100, and 50 μmol·mol–1, respectively, to obtain the corresponding Pn. The initial slope of Pn–Ci response curve was considered the carboxylation efficiency (CE).
Determination of Physiological Indexes
The content of chlorophyll a (Chla), chlorophyll b (Chlb), and carotenoids (Car) was determined by visible spectrophotometry with 80% acetone extraction (Lichtenthaler, 1987). The proline (Pro) content was measured by acidic ninhydrin colorimetry with 3% sulfosalicylic acid boiling water extraction (Bates et al., 1973). The measurement of H2O2 content followed the methods described by Alexieva et al. (2001). To monitor lipid peroxidation and membrane integrity, malondialdehyde (MDA) concentration was determined with fresh leaves as described previously (Wang et al., 2010). All physiological indexes were repeated three times (biological experiments).
Statistical Analysis
Excel (2003) and SPSS (22.0) software were used for statistical analysis. All data were the means ± standard error (SE). One-way ANOVA and least significant difference (LSD) were used for the comparison of the differences between different datasets. A P value less than 0.05 was considered statistically significant.
Results and Analysis
Chlorophyll and Carotenoid Content
As shown in Figure 1, the Chl a, Chl b, Chl (a+b), and Car content in blueberry leaves decreased significantly under low temperature stress, and the extent of the reduction increased with the increased duration of low temperature stress. The Chl a, Chl b, and Chl (a+b) content in blueberry leaves treated with NaHS under low temperature stress increased to varying degrees compared with those treated with LT, but the difference was not significant (P > 0.05). The Chl a, Chl b, and Chl content after treatment with Hypotaurine was significantly lower than that of the LT treatment (P < 0.05). Car content of blueberry leaves treated with NaHS was 17.14% (P < 0.05) and 23.25% (P < 0.05) higher than the leaves treated with LT at the 2nd and 5th day of low temperature, respectively. In contrast, the Car content in blueberry treated with Hypotaurine was 8.29% (P > 0.05) and 38.59% (P < 0.05) lower than that in the LT treatment, respectively.
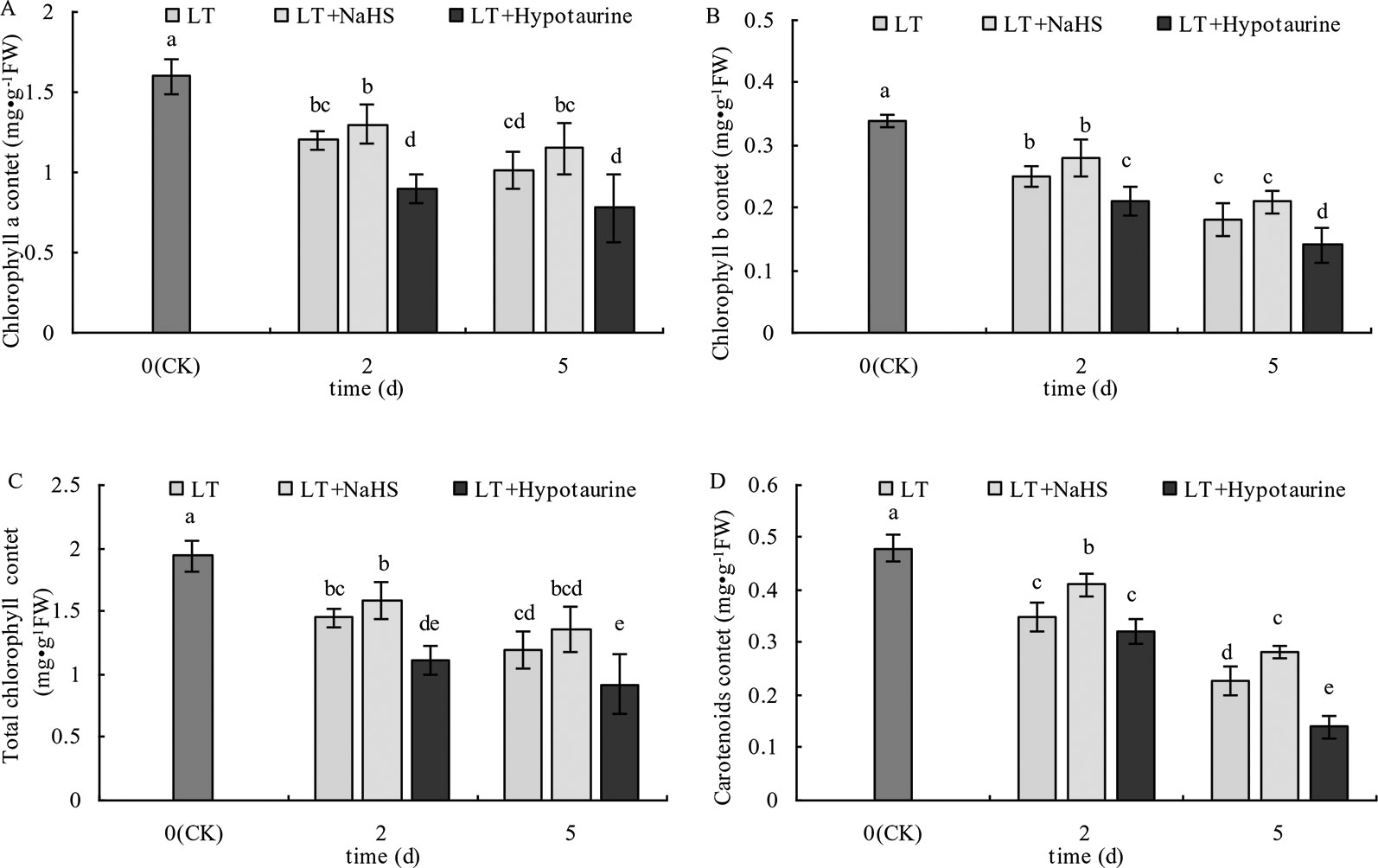
Figure 1 Effects of exogenous NaHS and Hypotaurine on chlorophyll a (A), chlorophyll b (B), total chlorophyll a (C), and carotenoid (D) contents in blueberry leaves under low temperature stress. The data in the figure are from three replicated experiments (n = 3), and represent means ± standard error (SE). Different small letters show significant differences (P < 0.05). CK: room temperature control at 25°C; LT: low temperature treatment; LT + NaHS: low temperature treatment at 4–6°C after spraying 0.5 mmol·L–1 NaHS; LT + Hypotaurine: low temperature treatment at 4-6°C after spraying leaves with 200 μmol·L–1 hypotaurine.
OJIP Curve and Photochemical Efficiency of PSII
The results in Figure 2 showed that low temperature stress significantly changed the OJIP curve of blueberry leaves. The relative fluorescence intensity Fo of point O changed little, whereas the Fp of point P decreased significantly, and the variation at the 5th day of low temperature treatment was significantly larger than that at the 2nd day. NaHS treatment significantly alleviated the decrease of Fp in blueberry leaves under low temperature stress, whereas the application of Hypotaurine increased the reduction of Fp.
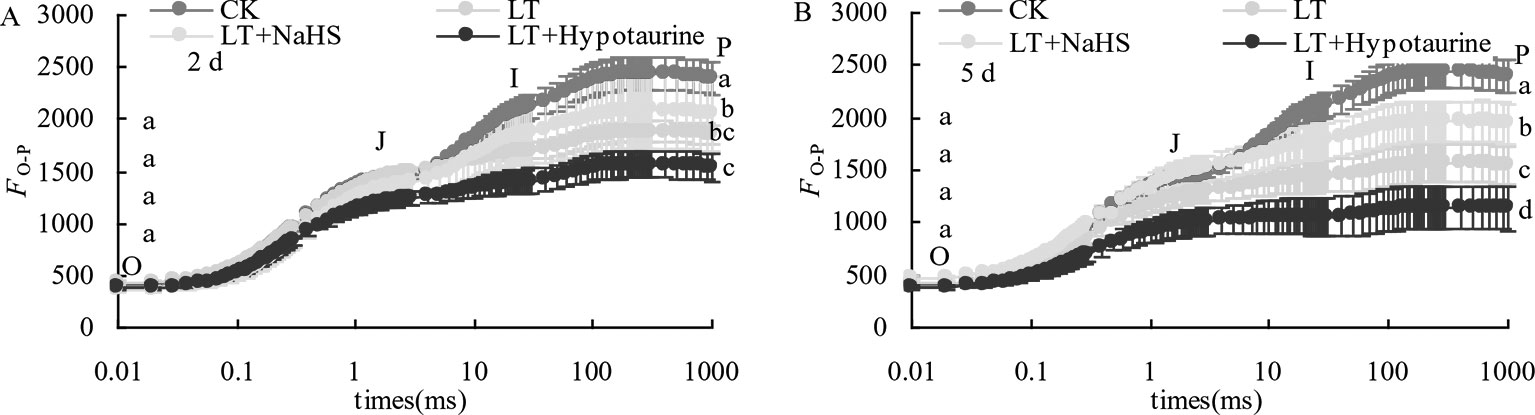
Figure 2 Effects of exogenous NaHS and Hypotaurine on OJIP curves of blueberry leaves under low temperature stress at the 2nd (A) and 5th (B) day. The data in the figure are from five replicated experiments (n = 5). Different small letters show significant differences (P < 0.05). CK: room temperature control at 25°C; LT: low temperature treatment; LT + NaHS: low temperature treatment at 4–6°C after spraying 0.5 mmol·L–1 NaHS; LT + Hypotaurine: low temperature treatment at 4-6°C after spraying leaves with 200 μmol·L–1 hypotaurine.
With the increased duration of the low temperature treatment, Fv/Fm and PIABS of blueberry leaves showed a decreasing trend. PIABS showed a greater decrease than Fv/Fm (Figure 3). Under low temperature stress, there was no significant difference of Fv/Fm between the LT+NaHS treatment and the LT treatment, but PIABS of blueberry leaves in the LT+NaHS treatment was higher than that in LT treatment by 65.35% (P < 0.05) and 36.51% (P > 0.05), respectively. Spraying with Hypotaurine resulted in an increase in the reduction of Fv/Fm and PIABS.
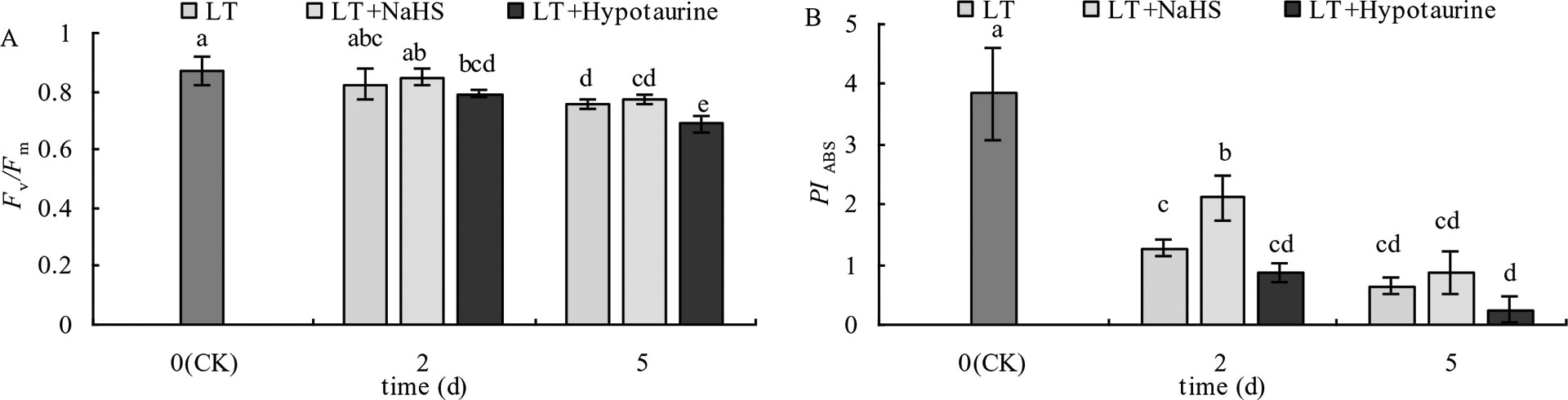
Figure 3 Effects of exogenous NaHS and Hypotaurine on Fv/Fm (A) and PIABS (B) of blueberry leaves under low temperature stress. The data in the figure are from five replicated experiments (n = 5), and represent means ± standard error (SE). Different small letters show significant differences (P < 0.05).CK: room temperature control at 25°C; LT: low temperature treatment; LT + NaHS: low temperature treatment at 4–6°C after spraying 0.5 mmol·L–1 NaHS; LT + Hypotaurine: low temperature treatment at 4–6°C after spraying leaves with 200 μmol·L–1 hypotaurine.
Standardized O–P Curve and Standardized O–J Curve
OJIP curves of blueberry leaves in different treatments were standardized by O-P (VO-P) (Figures 4A, B). The difference (△VO–P) between VO–P and CK (Figures 4C, D) showed that the relative variable fluorescence VJ at 2 ms of the VO–P curve increased significantly under low temperature stress, and the increase was greater at the 5th day than at the 2nd day. Under low temperature stress, the VJ of blueberry leaves in the NaHS treatment was lower than that in LT treatment by 23.04% (P > 0.05) and 17.55% (P > 0.05) at the 2nd and 5th day, respectively, while the Hypotaurine treatment further increased VJ (Figure 5A).
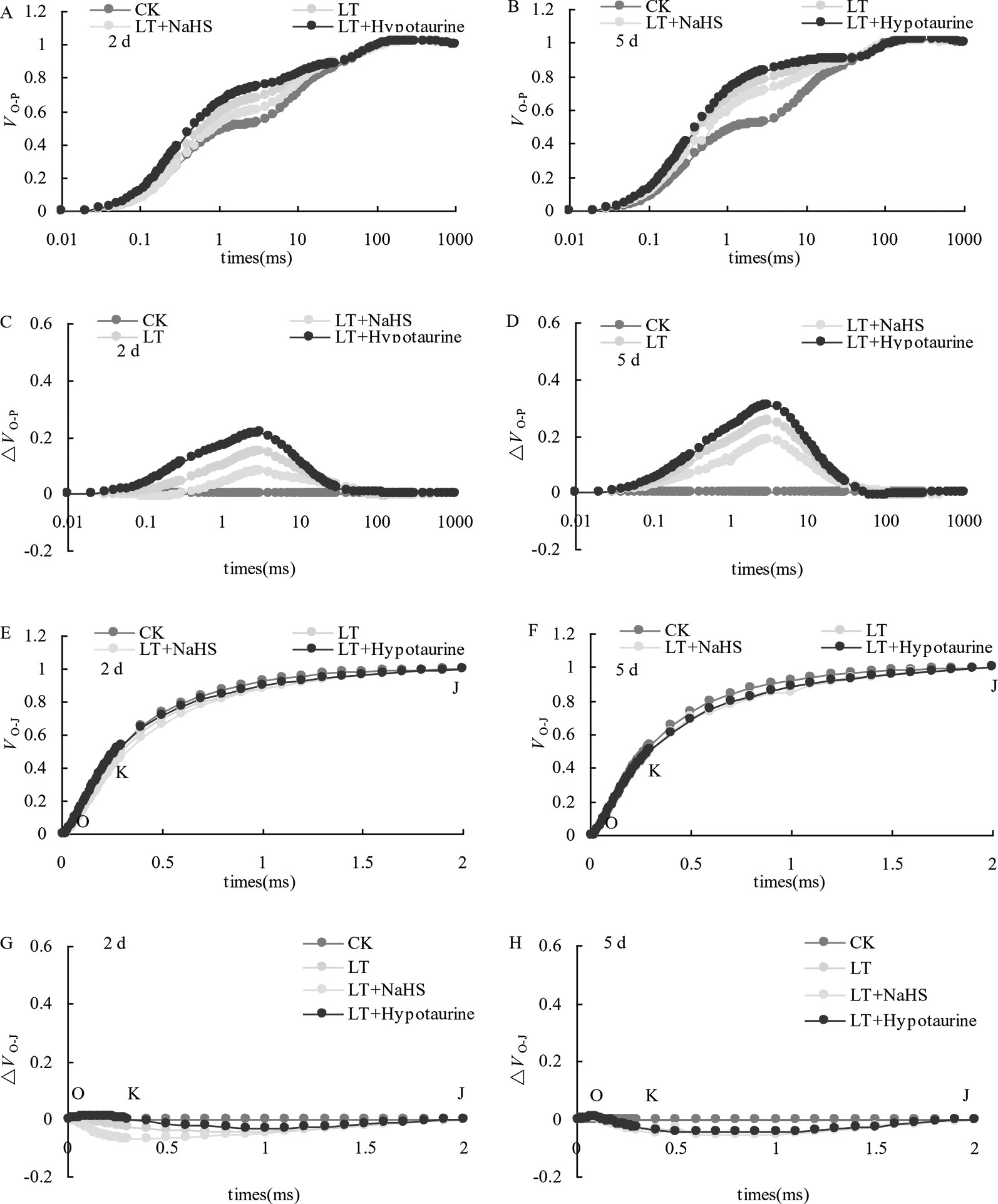
Figure 4 Effects of exogenous NaHS and Hypotaurine on VO-P and VO-J curves of blueberry leaves under low temperature stress. The data in the figure are from five replicated experiments (n = 5). CK: room temperature control at 25°C; LT: low temperature treatment; LT + NaHS: low temperature treatment at 4–6°C after spraying 0.5 mmol·L–1 NaHS; LT + Hypotaurine: low temperature treatment at 4–6°C after spraying leaves with 200 μmol·L–1 hypotaurine. Effects of exogenous NaHS and Hypotaurine on OJIP curves of blueberry leaves under low temperature stress. OJIP curves of blueberry leaves in different treatments were standardized by O-P (VO-P) (A, B). The difference (ΔVO-P) between VO-P and CK (C, D). OJIP curves were standardized by O-J (VO-J) (E, F). The difference(ΔVO-J) between the VO-J curve and the CK (G, H). The data in the figure are from five replicated experiments (n = 5). CK: room temperature control at 25°C; LT: low temperature treatment; LT + NaHS: low temperature treatment at 4–6°C after spraying 0.5 mmol•L-1 NaHS; LT + Hypotaurine: low temperature treatment at 4-6°C after spraying leaves with 200 μmol•L-1 hypotaurine.
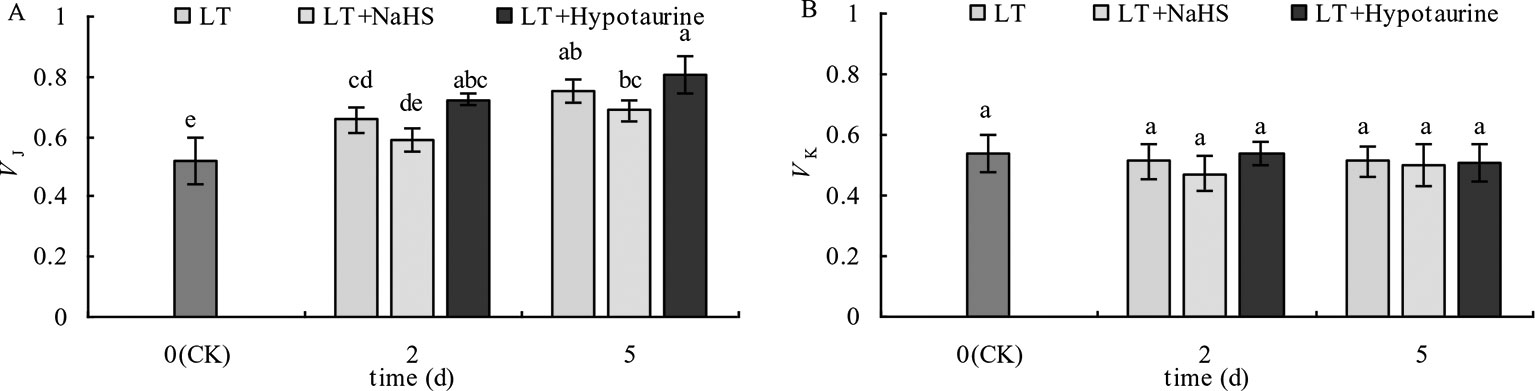
Figure 5 Effects of exogenous NaHS and Hypotaurine on VJ (A) and VK (B) of blueberry leaves under low temperature stress. The data in the figure are from five replicated experiments (n = 5), and represent means ± standard error (SE). Different small letters show significant differences (P < 0.05). CK: room temperature control at 25°C; LT: low temperature treatment; LT + NaHS: low temperature treatment at 4–6°C after spraying 0.5 mmol·L–1 NaHS; LT + Hypotaurine: low temperature treatment at 4–6°C after spraying leaves with 200 μmol·L–1 hypotaurine.
OJIP curves were standardized by O–J (VO–J) (Figures 4E, F). The difference (△VO–J) between the VO–J curve and the CK (Figures 4G, H) revealed that low temperature stress had little effect on VK at 0.3 ms, and there was no significant difference between VK and CK at the 2nd and 5th day of low temperature treatment. The effect of NaHS and Hypotaurine treatments on VK was also not significant (Figure 5B).
The Modulated Reflected Signal 820 nm (MR820 nm)
Under low temperature stress, the amplitude of the MR820 nm curve of blueberry leaves decreased (Figures 6A, B), and the slope of the MR820 nm curve at the initial stage (1–2.5 ms) decreased compared with the CK (Figures 6C, D). The decrease of the MR820 nm curve at the 5th day of low temperature treatment was greater than that at the 2nd day. Exogenous NaHS significantly alleviated the amplitude of the MR820 nm curve and minimized the decrease of the initial slope. In contrast, treatment with Hypotaurine showed the opposite effect. Quantitative analysis of △I/Io changes (Figure 7) showed that △I/Io of blueberry leaves decreased by 18.78% (P < 0.05) and 46.16% (P < 0.05) on the 2nd and 5th day of low temperature treatment, respectively. The decrease of △I/Io in the LT + NaHS treatment was significantly lower than that in the LT treatment, whereas the Hypotaurine treatment maximized the decrease of △I/Io under low temperature stress.
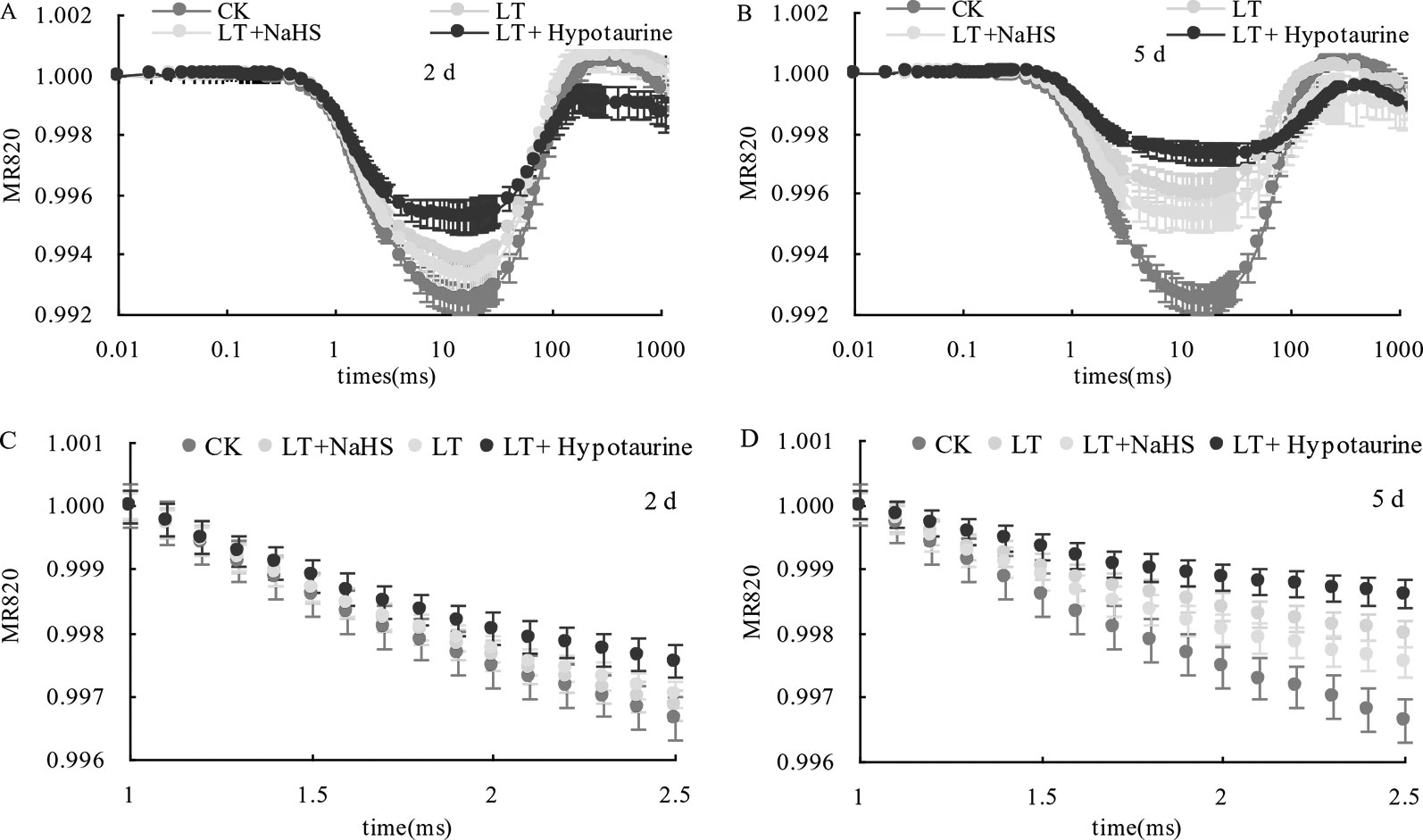
Figure 6 Effects of exogenous NaHS and Hypotaurine on the modulated reflected signal of 820 nm (MR820 nm) in blueberry leaves at the 2nd (A) and 5th (B) day of low temperature treatment and on the slope of the MR820 nm curve at the initial stage (1–2.5 ms) of decline at the 2nd (C) and 5th (D) day of treatment. The data in the figure are from five replicated experiments (n = 5). CK: room temperature control at 25°C; LT: low temperature treatment; LT + NaHS: low temperature treatment at 4–6°C after spraying 0.5 mmol·L–1 NaHS; LT + Hypotaurine: low temperature treatment at 4–6°C after spraying leaves with 200 μmol·L–1 hypotaurine.
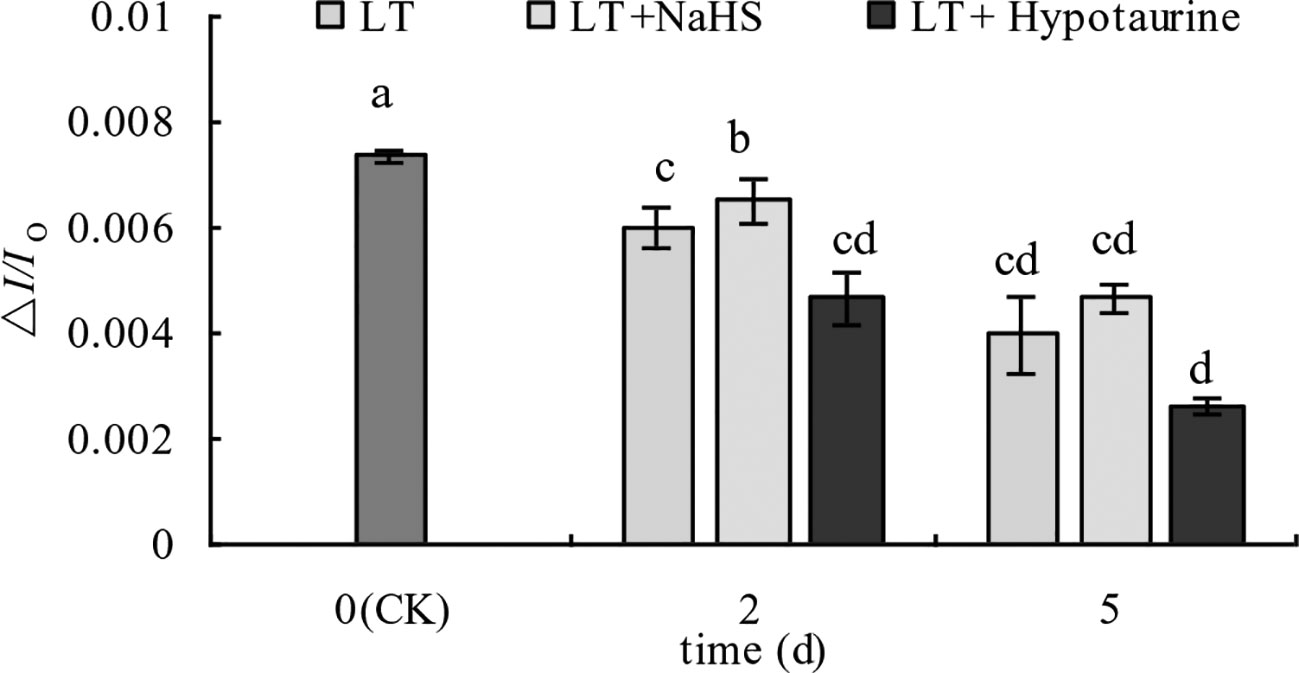
Figure 7 Effects of exogenous NaHS and Hypotaurine on △I/Io of blueberry leaves under low temperature stress. The data in the figure are from five replicated experiments (n = 5), and represent means ± standard error (SE). Different small letters show significant differences (P < 0.05). CK: room temperature control at 25°C; LT: low temperature treatment; LT + NaHS: low temperature treatment at 4–6°C after spraying 0.5 mmol·L–1 NaHS; LT + Hypotaurine: low temperature treatment at 4–6°C after spraying leaves with 200 μmol·L–1 hypotaurine.
Gas Exchange Parameters of Photosynthesis
The results in Figure 8 showed that the Pn, Gs, and Tr of blueberry leaves decreased significantly under low temperature stress; however, the decrease of Pn, Gs, and Tr was alleviated to varying degrees after spraying with exogenous NaHS. After spraying with Hypotaurine, Pn, Gs, and Tr showed a more evident decrease compared with the control. Ci in blueberry leaves did not change significantly at the 2nd day of low temperature, but increased significantly at the 5th day. Exogenous NaHS had no significant effect on Ci in blueberry leaves under low temperature stress, but Hypotaurine treatment increased Ci significantly.
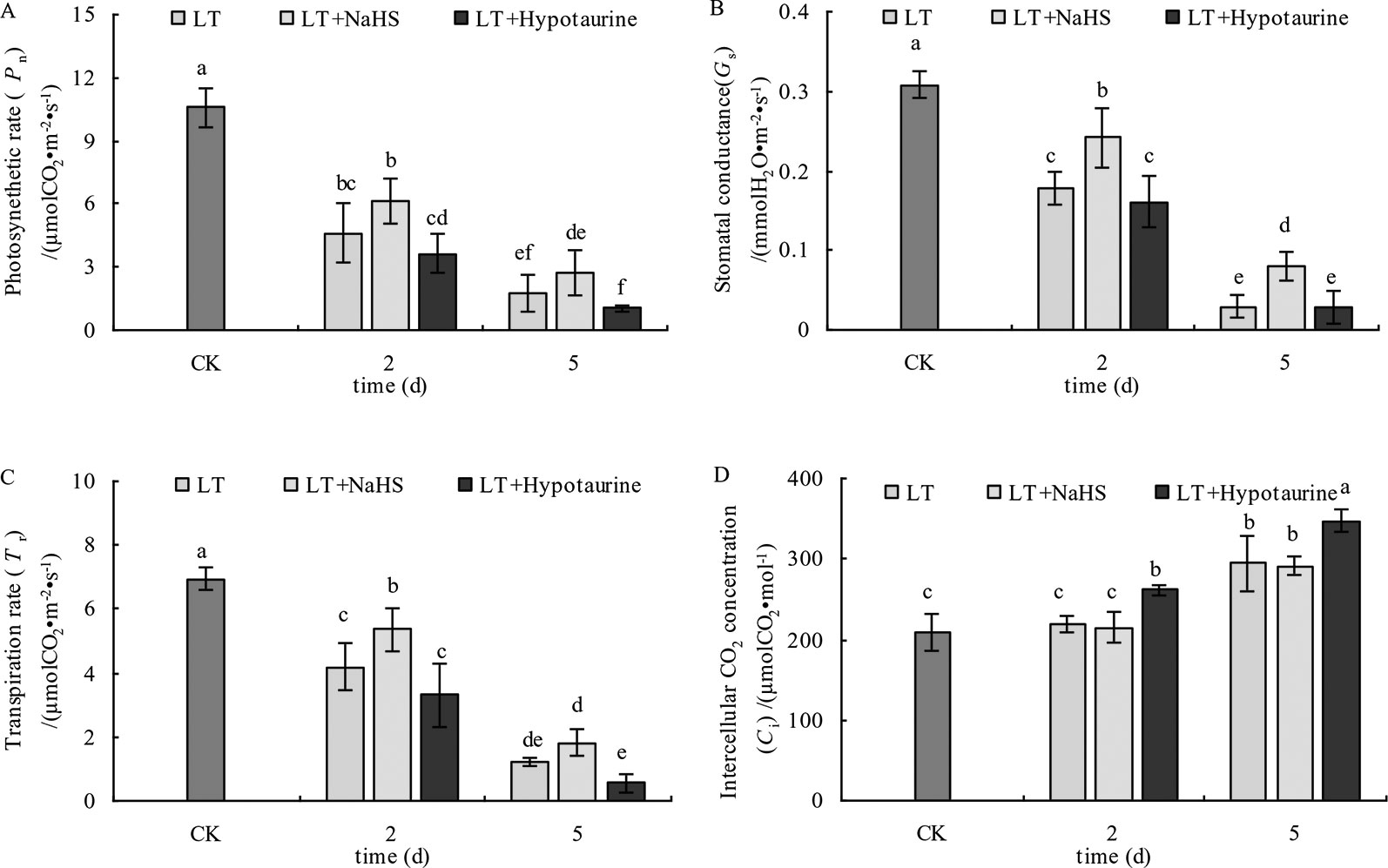
Figure 8 Effects of exogenous NaHS and Hypotaurine on net photosynthetic rate (A), stomatal conductance (B), transpiration rate (C), and intercellular CO2 concentration (D) of blueberry leaves under low temperature stress. The data in the figure are from five replicated experiments (n=5), and represent means ± standard error (SE). Different small letters show significant differences (P < 0.05).CK: room temperature control at 25°C; LT: low temperature treatment; LT + NaHS: low temperature treatment at 4–6°C after spraying 0.5 mmol·L–1 NaHS; LT + Hypotaurine: low temperature treatment at 4–6°C after spraying leaves with 200 μmol·L–1 hypotaurine.
Change in the Activity of the Dark Reaction
The determination of the initial slope of the CO2 response curve (Figures 9A, B) and CE (Figure 9C) showed that CE in blueberry leaves decreased significantly under low temperature stress. However, CE in the LT + NaHS treatment was significantly greater than that in the LT treatment on the 2nd and 5th day of cold treatment (P < 0.05), whereas the decrease of CE in LT+ Hypotaurine treatment was significantly greater than that in LT treatment.
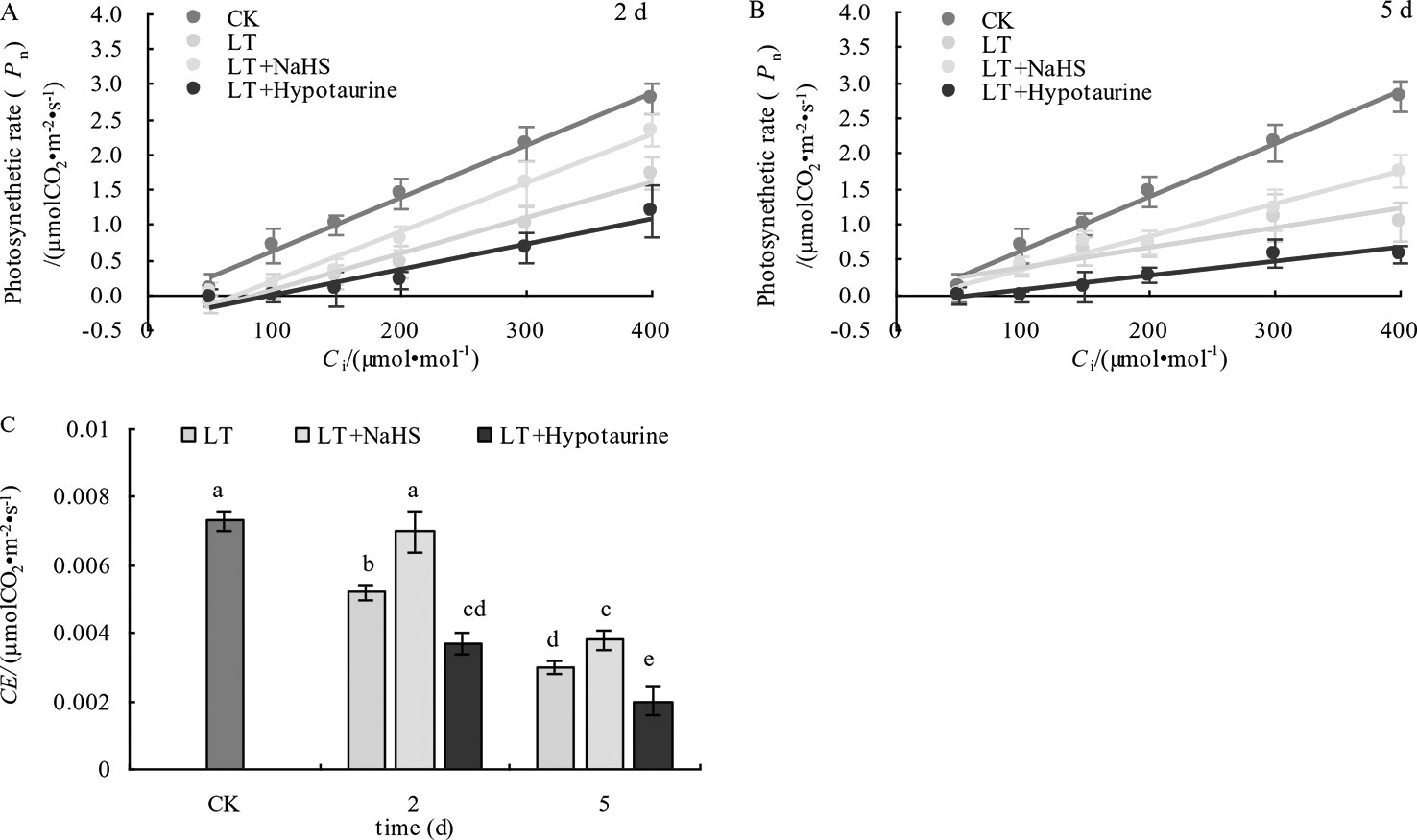
Figure 9 Effects of exogenous NaHS and HT on the initial slope (A, B) and CE (C) of the CO2 response curve of blueberry leaves under low temperature stress. The data in the figure are from five replicated experiments (n=5), and represent means ± standard error (SE). Different small letters show significant differences (P < 0.05).CK: room temperature control at 25°C; LT: low temperature treatment; LT + NaHS: low temperature treatment at 4–6°C after spraying 0.5 mmol·L–1 NaHS; LT + Hypotaurine: low temperature treatment at 4–6°C after spraying leaves with 200 μmol·L–1 hypotaurine.
Pro, H2O2, and MDA Content
With the prolongation of the low temperature treatment, the Pro, H2O2, and MDA content in blueberry leaves increased obviously (Figure 10). At the 2nd and 5th day of low temperature treatment, the Pro content of blueberry leaves treated with LT + NaHS increased by 32.69% (P < 0.05) and 19.05% (P > 0.05), respectively, compared with the plants treated with LT. The MDA content in the LT + NaHS treatment was 19.15% (P > 0.05) and 15.36% (P > 0.05) lower than that in LT treatment on the 2nd and 5th day of low temperature treatment, respectively. Therefore, spraying blueberry leaves with Hypotaurine significantly decreased Pro content and increased the accumulation of H2O2 and MDA content in blueberry leaves under low temperature stress.
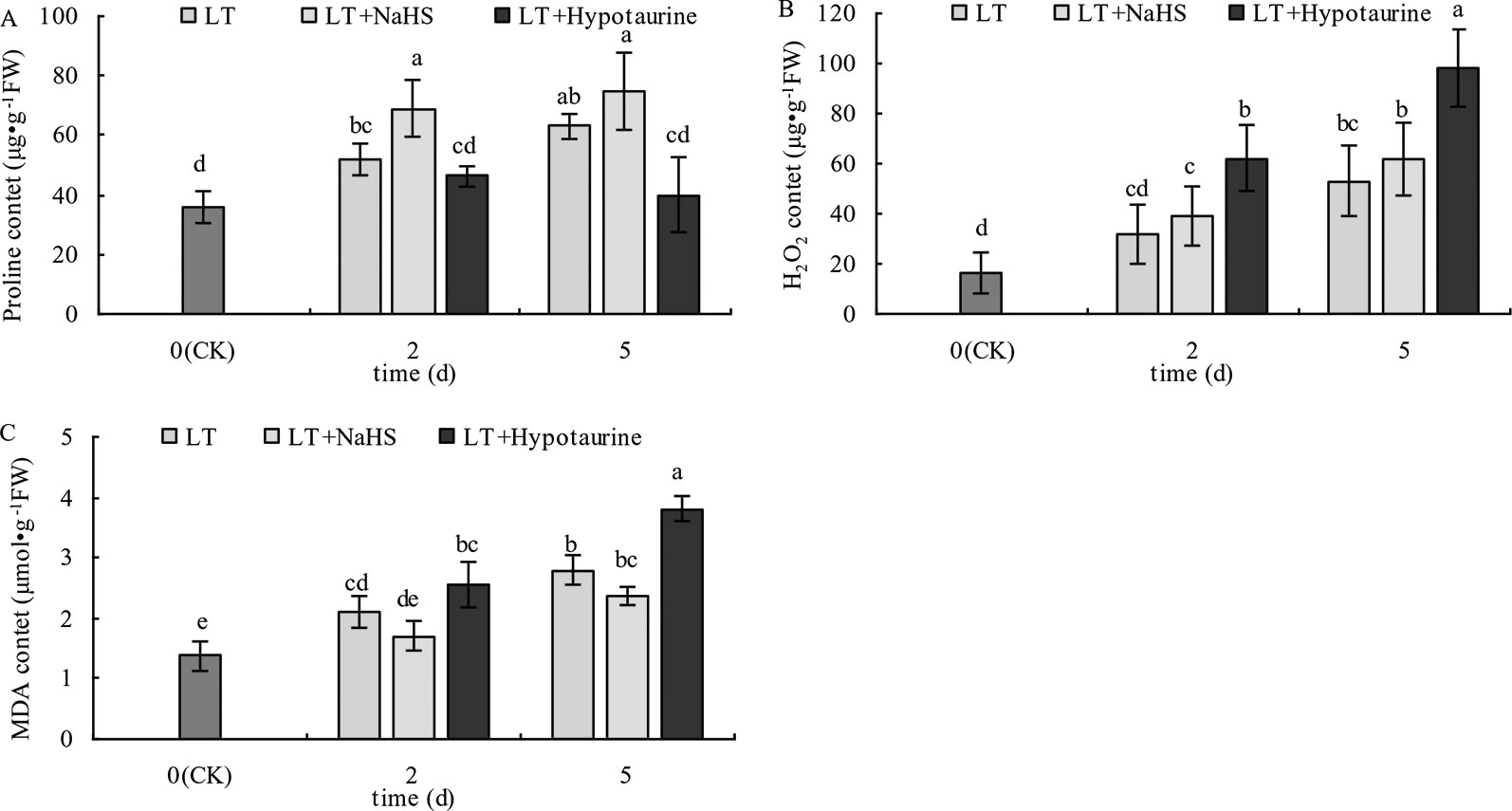
Figure 10 Effects of exogenous NaHS and Hypotaurine on proline (Pro) (A), H2O2 (B), and MDA (C) content in blueberry leaves under low temperature stress. The data in the figure are from three replicated experiments (n=3), and represent means ± standard error (SE). Different small letters show significant differences (P < 0.05). CK: room temperature control at 25°C; LT: low temperature treatment; LT + NaHS: low temperature treatment at 4–6°C after spraying 0.5 mmol·L–1 NaHS; LT + Hypotaurine: low temperature treatment at 4–6°C after spraying leaves with 200 μmol·L–1 hypotaurine.
Discussion
Chloroplasts are the main site of plant photosynthesis and one of the organelles that is most sensitive to stress. The decrease of chlorophyll content in the chloroplast inhibits the absorption and utilization of light energy by plants (Zhang et al., 2016). In our study, the Chla, Chlb, and Chla+b content in blueberry leaves were significantly decreased under low temperature stress (Figures 1A–C), which indicated that low temperature stress could lead to chlorophyll degradation or inhibit chlorophyll synthesis. The addition of exogenous NaHS could promote chlorophyll synthesis or alleviate its degradation rate (Chen et al., 2011), and exogenous NaHS could also promote chlorophyll synthesis and chloroplast development in maize under iron deficient conditions (Chen et al., 2015). Our results are consistent with these reports. The treatment with exogenous NaHS prior to low temperature stress significantly alleviated the decrease of chlorophyll content. In contrast, the application of exogenous Hypotaurine increased the reduction of chlorophyll content, indicating that exogenous H2S could prevent the degradation of chlorophyll in blueberry leaves under low temperature stress. Carotenoids are involved in the absorption and transmission of light energy by plants, as well as have strong antioxidant capacity (Zhai et al., 2016), and beneficial to the photosystem II assembly and function (Zakar et al., 2016). In the carotenoid-reduced Arabidopsis szl1 mutant, the sensitivity of PSI and PSII to low temperature increased significantly (Cazzaniga et al., 2012). Low temperature stress induced the decrease of carotenoid content in blueberry leaves (Figure 1D) and exogenous H2S alleviated the degradation of Car in blueberry leaves under low temperature stress.
Low temperature stress often leads to the decrease of PSII activity in plants. The photoinhibition of PSII decreases linearly with the decrease of temperature in the range of 4 to 25°C (Sonoike, 2011). The relative fluorescence intensity at point P of the OJIP curve decreased significantly under low temperature stress, and Fv/Fm and PIABS showed a decreasing trend, especially PIABS (Figure 3), indicating that low temperature led to the decrease of photochemical activity of PSII, and even photoinhibition. In addition, VJ increased significantly, whereas VK did not change significantly. The increase of VJ reflects the inhibition of electron transfer from QA to QB on the PSII acceptor side (Zhang et al., 2017; Zhang et al., 2018a), while the increase of VK is considered to be a specific marker of damage to oxygen-evolving complex on the PSII donor side (Zhang et al., 2018b). However, the change of VK is not only affected by injury on the PSII donor side, but also by damage on PSII acceptor side. When the injury on the acceptor side is greater than that on the donor side, VK does not increase significantly (Zhang et al., 2018c; Zhang et al., 2019b). Therefore, although the electron transfer from QA to QB on the PSII acceptor side of blueberry leaves was inhibited by low temperature, low temperature had little effect on the oxygen-evolving complex of the PSII donor side as VK did not change. The inhibition of electron transport from QA to QB in the PSII acceptor side under stress conditions was mainly related to the degradation of D1 protein, while exogenous H2S can accelerate the turnover of D1 protein in wheat leaves under drought stress to improve the drought resistance of PSII function (Li et al., 2015). Therefore, exogenous NaHS significantly alleviated the increase of VJ under low temperature stress, while exogenous Hypotaurine increased VJ, suggesting that exogenous H2S might protect the D1 protein in blueberry leaves under low temperature stress. Ultimately, this alleviates the photoinhibition of PSII.
In addition to the photoinhibition of PSII, PSI is also an important photoinhibition site under low temperature stress, especially in cold-sensitive plants. Low temperature stress makes PSI more prone to photoinhibition than PSII, and its degree of photoinhibition is often greater than PSII, and more challenging to recover (Terashima et al., 1994; Sonoike and Terashima, 1994; Zhang et al., 2010). We found that the PSI activity of blueberry leaves decreased under low temperature stress. The decrease of PSI activity was significantly alleviated by the exogenous application NaHS, while PSI activity was further decreased by Hypotaurine, an H2S scavenger, under low temperature stress. These data indicated that exogenous H2S could increase the PSI activity under low temperature stress. A previous study has reported that the photoinhibition of PSI is mainly related to the increase of reactive oxygen species in PSI (Sonoike, 1996). Thus, the accumulation of H2O2 under low temperature stress caused by Hypotaurine (Figure 10B) is an important reason for the increase of PSI photoinhibition.
Some studies have reported that exogenous H2S improved the drought resistance of Arabidopsis thaliana by inducing stomatal closure (Jin et al., 2017), which was mainly due to the reduction of the stomatal diameter caused by H2S (Jin et al., 2011) or the increase in the expression of the mitogen activated protein kinase gene to prevent over opening of stomata under low temperature stress (Du et al., 2017). However, other studies have shown that exogenous H2S improved photosynthetic capacity of rice leaves by increasing stomatal aperture and density, and NaHS induced stomatal opening in Arabidopsis thaliana by inhibiting NO production (Lisjak et al., 2010). Therefore, the function of H2S in plant stomatal movement is still controversial and requires further study. The Gs of blueberry leaves decreased rapidly under low temperature stress, leading to the decrease of Tr and Pn.
After spraying leaves with exogenous NaHS, the decrease of Gs and Tr was significantly lower than that of the control. Moreover, the decrease of Pn was also alleviated to varying degrees with the application of NaHS, whereas spraying with Hypotaurine aggravated stomatal closure and further decreased the photosynthetic rate under low temperature stress (Figure 8). This indicates that exogenous H2S could improve photosynthetic capacity of blueberry leaves under low temperature stress by promoting stomatal opening. Although the Gs in the exogenous NaHS treatment was significantly higher than that in non-sprayed NaHS treatment, the variation between the Pn was not significant. These results indicated that the application of exogenous H2S also increased photosynthetic capacity under low temperature stress via non-stomatal factors. Non-stomatal factors, such as the decrease of photosynthetic enzyme activity under stress, are also important factors that limit plant photosynthesis. Under severe stress, non-stomatal factors often play a major role in limiting plant photosynthesis (Zhang et al., 2019b). At the 5th day of low temperature stress, the Ci increased significantly (Figure 8D), indicating that the reason for the decrease of photosynthetic capacity caused by long-term (5 d) low temperature stress was due to the limitation of non-stomatal factors (Arena and Vitale, 2018; Zhang et al., 2019a) Exogenous H2S can promote the transport of CO2 (Espie et al., 1989), the expression of photosynthesis-related enzymes, and the redox modification of thiol groups to improve photosynthesis Application of exogenous H2S can also promote the protein and gene expression of Ribulose-1,5-Bisphosphate Carboxylase (Rubisco) and phenol pyruvate carboxylase in maize leaves under iron deficiency conditions (Chen et al., 2011). In our study, spraying NaHS increased the CE under low temperature stress, while spraying Hypotaurine decreased CE (Figure 9). Therefore, the reason why exogenous H2S can increase photosynthetic capacity of blueberry leaves under low temperature stress is not only related to the increase of induced stomatal conductance, but also possibly related to the fact that exogenous H2S is beneficial to CO2 fixation in dark reaction under low temperature stress, which may be related to the protection of dark reaction-related enzymes.
H2S also interacts with other hormones and signaling substances in plants, such as NO (Chen et al., 2011; Wang et al., 2012), SA (Amooaghaie et al., 2017), and Ca2+ (Li et al., 2012; Qiao et al., 2015). In addition, as a very important cell signaling molecule, H2O2 content is regulated by H2S in many physiological processes during plant growth and development (Fang H. et al., 2014; Zhang et al., 2008). H2S can be used as an upstream signaling molecule of H2O2 to promote the seed germination as described for mung bean (Li et al., 2012). Li et al. found that H2S could improve salt tolerance in Arabidopsis thaliana roots, and this process required the active participation of H2O2 (Li et al., 2015). With the increased duration of low temperature stress, H2O2 content in blueberry leaves increased significantly, although exogenous NaHS treatment promoted the increase of H2O2 content in blueberry leaves to some extent, the difference was not significant, which may be related to the increase of antioxidant enzyme activity or accumulation of antioxidants by NaHS under low temperature stress. Exogenous Hypotaurine significantly increased H2O2 content under low temperature stress. A low concentration of H2O2 can be used as a signaling substance in plant response to stress, and a high concentration of H2O2 can cause oxidative damage to plant cells (Li et al., 2014). Therefore, exogenous Hypotaurine aggravates oxidative damage under low temperature stress.
H2S can alleviate the membrane peroxidation under low temperature stress by regulating the activity of antioxidant enzymes in hawthorn fruits (Cheng et al., 2016), mitigate the oxidative damage caused by Al by increasing the antioxidant capacity of wheat (Aghdam et al., 2018), and reduce the MDA content by enhancing the activity of antioxidant enzymes in alfalfa seedlings under Cd stress (Zhang et al., 2010). In addition, H2S can also induce the accumulation of ascorbic acid and glutathione in plants to improve its antioxidant capacity (Cui et al., 2014). Under low temperature stress, the increase of MDA content of blueberry leaves was significantly alleviated in NaHS treatment, while the membrane peroxidation of leaves was intensified in Hypotaurine treatment, which was consistent with the change of H2O2 content (Figure 10B).
Under stress, plant cells actively accumulate small molecules to regulate their osmotic potential and maintain their normal water content (Shan et al., 2011). Exogenous H2S could control the water potential and relative water content of spinach leaves by regulating the synthesis of soluble sugar, polyamine, and glycine betaine to enhance the adaptability of spinach seedlings to drought (Kaur and Asthir, 2015). The accumulation of Pro plays an important role in improving stress resistance of plants (Chen et al., 2016). Pro is also an inducer of osmotic stress-related genes and is a scavenger of reactive oxygen species (Hong et al., 2000; Theocharis et al., 2012), which plays an important role in improving the stability of plant cell membranes under stress (Lu and Becker, 2015). Luo et al. (2015) has found that exogenous H2S could increase the Pro content of banana under low temperature stress and significantly enhances their cold tolerance, which was mainly related to H2S increasing the activity of 1-pyrroline-5-carboxylate synthetase and decreasing the activity of proline dehydrogenase (Mansour, 2013). Li et al. (2015) also demonstrated that exogenous H2S improved the heat tolerance of maize, which was related to the Pro accumulation induced by exogenous H2S. The results of the present experiment are consistent with these previous reports. Under low temperature stress, the accumulation of Pro leaves increased, and spraying with exogenous NaHS promoted the Pro accumulation, while spraying with Hypotaurine had the opposite effect (Figure 10A). Therefore, the accumulation of Pro is an adaptive mechanism to low temperature stress, and the accumulation of Pro induced by exogenous H2S plays an active role in improving its low temperature tolerance. The mechanism of exogenous H2S donor (NaHS) alleviating photosynthesis inhibition under low temperature stress is summarized in Figure 11.
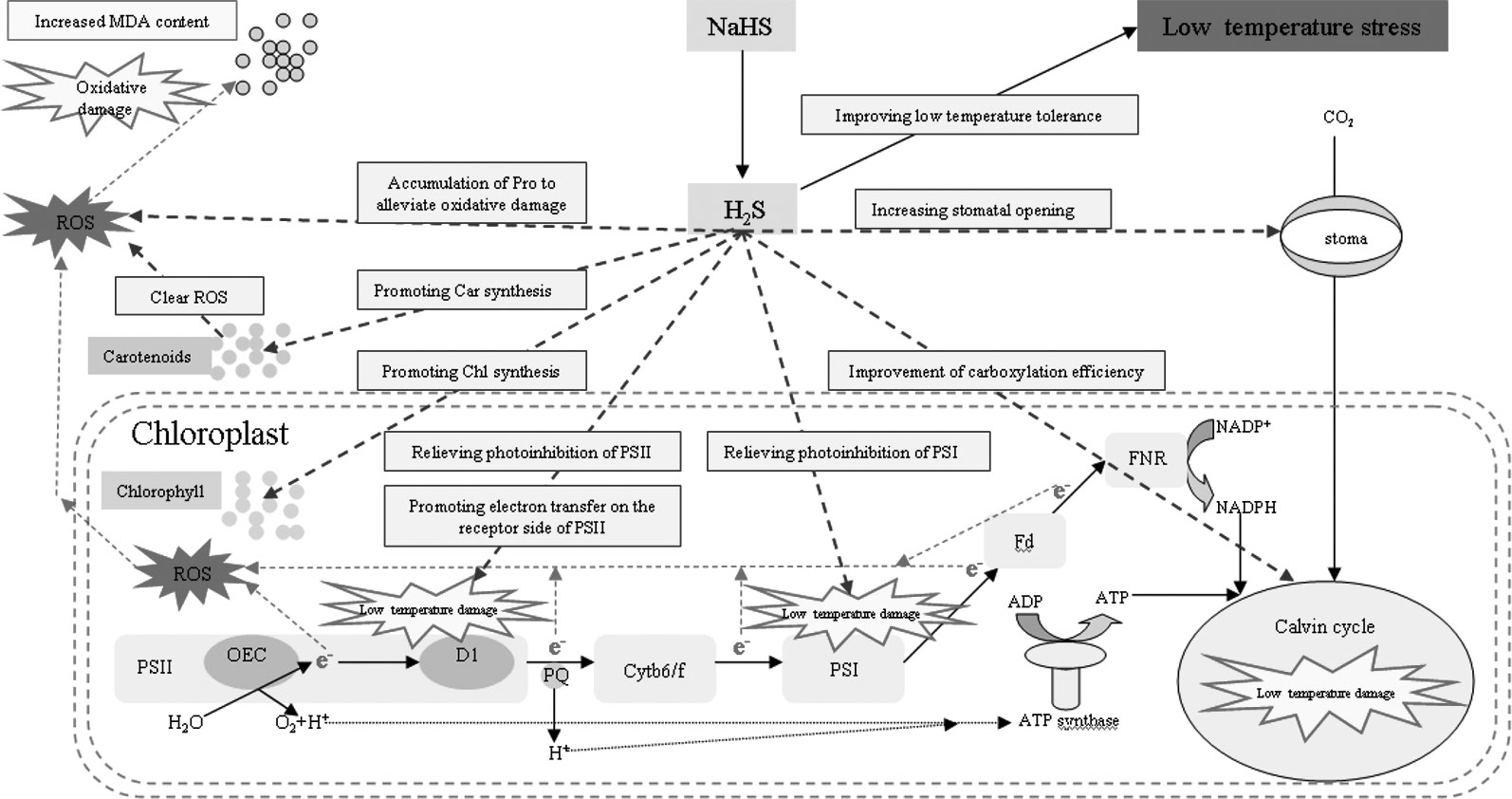
Figure 11 Mechanisms of exogenous H2S donor (NaHS) in alleviating photosynthesis inhibition under low temperature stress.
Conclusions
NaHS, an exogenous H2S donor, significantly alleviated the degradation of chlorophyll and carotenoids in blueberry leaves under low temperature stress. NaHS also increased the activities of PSII and PSI, of which the electron transfer from QA to QB on the acceptor side of PSII may be the site of primary activity of H2S. Exogenous H2S also promoted stomatal opening and photosynthetic carbon assimilation ability under low temperature stress. Promoting the accumulation of Pro plays an important role in improving the low temperature tolerance of blueberry by exogenous H2S. In contrast, spraying blueberry leaves with Hypotaurine, an H2S scavenger, aggravated the photoinhibition and oxidative damage of blueberry leaves. In conclusion, the application of exogenous H2S improved the tolerance of blueberry to low temperature stress, which was mainly related to the improvement of photosynthetic capacity and the accumulation of Pro in blueberry leaves.
Data Availability Statement
All datasets generated for this study are included in the article/supplementary material.
Author Contributions
XT, BA and XL conceived and designed experiments; All the authors performed the experiments and analyzed the data; XT and BA wrote the manuscript and prepared the figures and/or tables. XT, BA and XL reviewed drafts of the paper. XT and BA contributed equally to this work.
Funding
This work was supported by National key Research and Development Program of China (2017YFC0504205); Science and Technology Development Program of Jilin Province in China (2016041201XH).
Conflict of Interest
The authors declare that the research was conducted in the absence of any commercial or financial relationships that could be construed as a potential conflict of interest.
References
Aghdam, M. S., Mahmoudi, R., Razavi, F., Rabiei, V., Soleimani, A. (2018). Hydrogen sulfide treatment confers chilling tolerance in hawthorn fruitduring cold storage by triggering endogenous H2S accumulation, enhancingantioxidant enzymes activity and promoting phenols accumulation. Sci. Hort. 238, 264–271. doi: 10.1016/j.scienta.2018.04.063
Alexieva, V., Sergiev, I., Mapelli, S., Karanov, E. (2001). The effect of drought and ultraviolet radiation on growth and stress markers in pea and wheat. Plant Cell Environ. 24 (12), 1337–1344. doi: 10.1046/j.1365-3040.2001.00778.x
Amooaghaie, R., Zangenemadar, F., Enteshari, S. (2017). Role of two-sided crosstalk between NO and H2S on improvement of mineral homeostasis and antioxidative defense in Sesamum indicum under lead stress. Ecotoxicol. Environ. Saf. 139, 210–218. doi: 10.1016/j.ecoenv.2017.01.037
Arena, C., Vitale, L. (2018). Chilling-induced reduction of photosynthesis is mitigated by exposure to elevated CO2 concentrations. Photosynthetica 56, 1259–1267. doi: 10.1007/s11099-018-0843-3
Bates, L. S., Waldren, R. P., Teare, I. D. (1973). Rapid determination of free proline for water stress studies. Plant Soil 39, 205–207. doi: 10.1007/BF00018060
Cazzaniga, S., Li, Z., Niyogi, K. K., Bassi, R., Dall′Osto, L. (2012). The Arabidopsis szl1 mutant reveals a critical role of -carotene in photosystem I photoprotection. Plant Physiol. 159 (4), 1745–1758. doi: 10.1104/pp.112.201137
Chen, J., Wu, F. H., Wang, W. H., Wang, W. H., Hu, W. J., Simon, M., et al. (2011). Hydrogen sulphide enhances photosynthesis through promoting chloroplast biogenesis, photosynthetic enzyme expression, and thiol redox modification in Spinacia oleracea seedlings. J. Exp. Bot. 62 (13), 4481–4493. doi: 10.1093/jxb/err145
Chen, J., Wang, W. H., Wu, F. H., Chun, Y. Y., Ting, W. L., Xue, J. D., et al. (2013). Hydrogen sulfide alleviates aluminum toxicity in barley seedlings. Plant Soil 362 (1-2), 301–318. doi: 10.1007/s11104-012-1275-7
Chen, J., Wu, F. H., Shang, Y. T., Wang, W. H., Hu, W. J., Simon, M., et al. (2015). Hydrogen sulphide improves adaptation of Zea mays seedlings to iron deficiency. J. Exp. Bot. 66 (21), 6605–6622. doi: 10.1093/jxb/erv368
Chen, J., Shang, Y. T., Wang, W. H., Chen, X. Y., He, E. M., Zheng, H. L., et al. (2016). Hydrogen sulfide-mediated polyamines and sugar changes are involved in hydrogen sulfide-induced drought tolerance in Spinacia oleracea seedlings. Front. Plant Sci. 7, 1173. doi: 10.3389/fpls.2016.01173
Cheng, W., Zhang, L., Jiao, C. J., Su, M., Yang, T., Zhou, L. N., et al. (2013). Hydrogen sulfide alleviates hypoxia-induced root tip death in Pisum sativum. Plant Physiol. Biochem. 70 (1), 278–286. doi: 10.1016/j.plaphy.2013.05.042
Cheng, D. D., Lui, M. J., Sun, X. B., Zhao, M., Chow, W. S., Sun, G. Y., et al. (2016). Light suppresses bacterial population through the accumulation of hydrogen peroxide in tobacco leaves infected with Pseudomonas syringae pv. tabaci. Front. Plant Sci. 7, 512. doi: 10.3389/fpls.2016.00512
Christou, A. (2013). Hydrogen sulfide induces systemic tolerance to salinity and non-ionic osmotic stress in strawberry plants through modification of reactive species biosynthesis and transcriptional regulation of multiple defence pathways. J. Exp. Bot. 64 (7), 1953–1966. doi: 10.1093/jxb/ert055
Coyne, P., Bingham, G. (1978). Photosynthesis and stomatal light responses in snap beans exposed to hydrogen sulfide and ozone. Air Repair 128 (11), 1119–1123. doi: 10.1080/00022470.1978.10470715
Cui, W., Chen, H., Zhu, K., Jin, Q., Xie, Y., Cui, J., et al. (2014). Cadmium-induced hydrogen sulfide synthesis is involved in cadmium tolerance in Medicago sativa by reestablishment of reduced (Homo) glutathione and reactive oxygen species homeostases. PloS One 9 (10), e109669. doi: 10.1371/journal.pone.0109669
Du, X. Z., Jin, Z. P., Liu, D. M., Yang, G. D., Pei, Y. X. (2017). Hydrogen sulfide alleviates the cold stress through MPK4 in Arabidopsis thaliana. Plant Physiol. Biochem. 120, 112–119. doi: 10.1016/j.plaphy.2017.09.028
Duan, B. B., Ma, Y. H., Jiang, M. G., Yang, F., Ni, L., Lu, W. (2015). Improvement of photosynthesis in rice (Oryza sativa L.) as a result of an increase in stomatal aperture and density by exogenous hydrogen sulfide treatment. Plant Growth Regul. 75 (1), 33–44. doi: 10.1007/s10725-014-9929-5
Espie, G. S., Miller, A. G., Canvin, D. T. (1989). Selective and reversible inhibition of active CO2 transport by hydrogen sulfide in a Cyanobacterium. Plant Physiol. 91 (1), 387–394. doi: 10.1104/pp.91.1.387
Fang, T., Cao, Z., Li, J., Shen, W., Huang, L. (2014). Auxin-induced hydrogen sulfide generation is involved in lateral root formation in tomato. Plant Physiol. Biochem. 76 (5), 44–51. doi: 10.1016/j.plaphy.2013.12.024
Fang, H. H., Jing, T., Liu, Z. Q., Zhang, L. P., Jin, Z. P., Pei, Y. X. (2014). Hydrogen sulfide interacts with calcium signaling to enhance the chromium tolerance in Setaria italica. Cell Calcium 56 (6), 472–481. doi: 10.1016/j.ceca.2014.10.004
Fu, L. H., Hu, K. D., Hu, L. Y., Li, Y. H., Hu, L. B., Yan, H. (2014). An antifungal role of hydrogen sulfide on the postharvest pathogens Aspergillus niger and Penicillium italicum. PloS One 9, e104206. doi: 10.1371/journal.pone.0104206
Hancock, J. T., Whiteman, M. (2016). Hydrogen sulfide signaling: interactions with nitric oxide and reactive oxygen species. Ann. New York Acad. Sci. 1365 (1), 5–14. doi: 10.1111/nyas.12733
Hong, Z., Lakkineni, K., Zhang, Z., Verma, D. P. (2000). Removal of feedback inhibition of delta(1)-pyrroline-5-carboxylate synthetase results in increased proline accumulation and protection of plants from osmotic stress. Plant Physiol. 122 (4), 1129–1136. doi: 10.1104/pp.122.4.1129
Hu, K. D., Wang, Q., Hu, L. Y., Gao, S. P., Wu, J., Li, Y. H. (2014). Hydrogen sulfide prolongs postharvest storage of fresh-cut pears (Pyrus pyrifolia) by alleviation of oxidative damage and inhibition of fungal growth. PloS One 9, e85524. doi: 10.1371/journal.pone.0085524
Hua, Z., Hao, J., Jiang, C. X., Wang, S. H., Wei, Z. J., Luo, J. P., et al. (2010). Hydrogen sulfide protects soybean seedlings against drought-induced oxidative stress. Acta Physiol. Plantarum 32 (5), 849–857. doi: 10.1007/s11738-010-0469-y
Jia, H., Hu, Y., Fan, T., Li, J. (2015). Hydrogen sulfide modulates actin-dependent auxin transport via regulating ABPs results in changing of root development in Arabidopsis. Sci. Rep. 5, 8251. doi: 10.1038/srep08251
Jin, Z. P., Shen, J. J., Qiao, Z. J., Yang, G. D., Wang, R., Pei, T. X. (2011). Hydrogen sulfide improves drought resistance in Arabidopsis thaliana. Biochem. Biophys. Res. Commun. 414 (3), 481–486. doi: 10.1016/j.bbrc.2011.09.090
Jin, Z. P., Xue, S. W., Luo, Y. N., Tian, B. H., Fang, H. H., Li, H., et al. (2013). Hydrogen sulfide interacting with abscisic acid in stomatal regulation responses to drought stress in Arabidopsis. Plant Physiol. Biochem. 62 (1), 41–46. doi: 10.1016/j.plaphy.2012.10.017
Jin, Z. P., Wang, Z. Q., Ma, Q. X., Sun, L. M., Zhang, L. P., Liu, Z. Q., et al. (2017). Hydrogen sulfide mediates ion fluxes inducing stomatal closure in response to drought stress in Arabidopsis thaliana. Plant Soil 419 (1-2), 141–152. doi: 10.1007/s11104-017-3335-5
Jin, Z. P., Sun, L. M., Yang, G. D., Pei, Y. X. (2018). Hydrogen sulfide regulates energy production to delay leaf senescence induced by drought stress in Arabidopsis. Front. Plant Sci. 9, 1722. doi: 10.3389/fpls.2018.01722
Joanna, T. D., Anna, B., Jan, F., Piotr, Z., Emilia, J., Marlena, R., et al. (2019). Global analysis of gene expression in maize leaves treated with low temperature: I. Moderate chilling (14°C). Plant Sci. 177 (6), 648–658. doi: 10.1016/j.plantsci.2009.09.001
Kaur, G., Asthir, B. (2015). Proline: a key player in plant abiotic stress tolerance. Biol. Plantarum 59 (4), 609–619. doi: 10.1007/s10535-015-0549-3
Lai, D., Mao, Y., Zhou, H., Li, F., Wu, M., Zhang, J., et al. (2014). Endogenous hydrogen sulfide enhances salt tolerance by coupling the reestablishment of redox homeostasis and preventing salt-induced K+ loss in seedlings of Medicago sativa. Plant Sci. 225 (8), 117–129. doi: 10.1016/j.plantsci.2014.06.006
Li, Z. G., He, Q. Q. (2015). Hydrogen peroxide might be a downstream signal molecule of hydrogen sulfide in seed germination of mung bean (Vigna radiata). Biologia 70 (6), 753–759. doi: 10.1515/biolog-2015-0083
Li, Z. G., Gong, M., Xie, H., Tan, L., Li, J. (2012). Hydrogen sulfide donor sodium hydrosulfide-induced heat tolerance in tobacco (Nicotiana tabacum L.) suspension cultured cells and involvement of Ca2+and calmodulin. Plant Sci. 185–186 (4), 185–189. doi: 10.1016/j.plantsci.2011.10.006
Li, J. S., Jia, H. L., Wang, J., Cao, Q. H., Wen, Z. C. (2014). Hydrogen sulfide is involved in maintaining ion homeostasis via regulating plasma membrane Na+/H+ antiporter system in the hydrogen peroxide-dependent manner in salt-stress Arabidopsis thaliana root. Protoplasma 251 (4), 899–912. doi: 10.1007/s00709-013-0592-x
Li, H., Gao, M. Q., Xue, R. L., Wang, D., Zhao, H. J. (2015). Effect of hydrogen sulfide on D1 protein in wheat under drought stress. Acta Physiol. Plantarum 37 (11), 225. doi: 10.1007/s11738-015-1975-8
Li, Z. G., Min, X., Zhou, Z. H. (2016). Hydrogen sulfide: a signal molecule in plant cross-adaptation. Front. Plant Sci. 7, 1621. doi: 10.3389/fpls.2016.01621
Lichtenthaler, H. K. (1987). Chlorophylls and carotenoids: pigments of photosynthetic biomembranes. Method Enzymol. 148, 350–382. doi: 10.1016/0076-6879(87)48036-1
Lisjak, M., Srivastava, N., Teklic, T., Civale, L., Lewandowski, K., Wilson, I., et al. (2010). A novel hydrogen sulfide donor causes stomatal opening and reduces nitric oxide accumulation. Plant Physiol. Biochem. 48 (12), 931–935. doi: 10.1016/j.plaphy.2010.09.016
Lisjak, M., Teklic, T., Wilson, I. D., Wood, M., Whiteman, M., Hancock, J. (2011). Hydrogen sulfide effects on stomatal apertures. Plant Signaling Behav. 6 (10), 1444–1446. doi: 10.4161/psb.6.10.17104
Liu, R., Lal, R. (2015). Effects of low-level aqueous hydrogen sulfide and other sulfur species on lettuce (Lactuca sativa) seed germination. Commun. Soil Sci. Plant Anal. 46 (5), 576–587. doi: 10.1080/00103624.2014.998341
Lu, Z., Becker, D. F. (2015). Connecting proline metabolism and signaling pathways in plant senescence. Front. Plant Sci. 6, 522.
Luo, Z., Li, D., Du, R. X., Mou, W. X. (2015). Hydrogen sulfide alleviates chilling injury of banana fruit by enhanced antioxidant system and proline content. Scientia Hortic. 183 (183), 144–151. doi: 10.1016/j.scienta.2014.12.021
Mansour, M. M. F. (2013). Plasma membrane permeability as an indicator of salt tolerance in plants. Biol. Plantarum 57 (1), 1–10. doi: 10.1007/s10535-012-0144-9
Mustafa, A. K., Gadalla, M. M., Sen, N., Kim, S., Mu, W., Gazi, S. K., et al. (2009). H2S signals through protein S-sulfhydration. Sci. Signal. 2 (96), 72. doi: 10.1126/scisignal.2000464
Qiao, Z., Tao, J., Liu, Z. Q., Zhang, L. P., Jin, Z. P., Liu, D. M., et al. (2015). H2S acting as a downstream signaling molecule of SA regulates Cd tolerance in Arabidopsis. Plant Soil 393 (1-2), 137–146. doi: 10.1007/s11104-015-2475-8
Scuffi, D., Álvarez, C., Laspina, N., Gotor, C., Lamattina, L., García-Mata, C. (2014). Hydrogen sulfide generated by L-cysteine desulfhydrase acts upstream of nitric oxide to modulate abscisic acid-dependent stomatal closure. Plant Physiol. 166 (4), 2065–2076. doi: 10.1104/pp.114.245373
Shan, C. J., Zhang, S. L., Li, D. F., Zhao, Y. Z., Tian, X. L., Zhao, X. L., et al. (2011). Effects of exogenous hydrogen sulfide on the ascorbate and glutathione metabolism in wheat seedlings leaves under water stress. Acta Physiol. Plantarum 33 (6), 2533–2540. doi: 10.1007/s11738-011-0746-4
Shen, J. R., Terashima, I., Katoh, S. (1990). Cause for dark, chilling-induced inactivation of photosynthetic oxygen evolving system in cucumber leaves. Plant Physiol. 93, 1354–1357. doi: 10.1104/pp.93.4.1354
Shi, H., Ye, T., Han, N., Bian, H., Liu, X., Chan, Z. (2015). Hydrogen sulfide regulates abiotic stress tolerance and biotic stress resistance in Arabidopsis. J. Integr. Plant Biol. 57 (7), 628–640. doi: 10.1111/jipb.12302
Sonoike, K., Terashima, I. (1994). Mechanism of photosystem-I photoinhibition in leaves of Cucumis sativus L. Planta 194 (2), 287–293. doi: 10.1007/BF00196400
Sonoike, K. (1996). Degradation of psaB gene product, the reaction center subunit of photosystem I, is caused during photoinhibition of photosystem I: possible involvement of active oxygen species. Plant Sci. 115 (2), 157–164. doi: 10.1016/0168-9452(96)04341-5
Sonoike, K. (2011). Photoinhibition of photosystem I. Physiol. Plantarum 142, 56–64. doi: 10.1111/j.1399-3054.2010.01437.x
Strasser, R. J., Srivastava, A., Govindjee (1995). Polyphasic chlorophyll a fluorescence transient in plants and cyanobacteria. Photochem. Photobiol. 61 (1), 32–42. doi: 10.1111/j.1751-1097.1995.tb09240.x
Strauss, A. J., Kruger, G. H., Strasser, R. J., Van Heerden, P. D. (2010). The role of low soil temperature in the inhibition of growth and PSII function during dark chilling in soybean genotypes of contrasting tolerance. Physiol. Plantarum 131 (1), 89–105. doi: 10.1111/j.1399-3054.2007.00930.x
Terashima, I., Funayama, S., Sonoike, K. (1994). The site of photoinhibition in leaves of Cucumis sativus L.at low temperatures is photosystem I, not photosystem II. Planta 193 (2), 300–306. doi: 10.1007/BF00192544
Theocharis, A., Clément, C., Barka, E. A. (2012). Physiological and molecular changes in plants grown at low temperatures. Planta 235 (6), 1091–1105. doi: 10.1007/s00425-012-1641-y
Wang, X., Chen, S., Zhang, H., Shi, L., Cao, F., Guo, L., et al. (2010). Desiccation tolerance mechanism in resurrection fern-ally Selaginella tamariscina revealed by physiological and proteomic analysis. J. Proteome Res. 9, 6561–6577. doi: 10.1021/pr100767k
Wang, Y., Li, L., Cui, W., Xu, S., Shen, W., Wang, R. (2012). Hydrogen sulfide enhances alfalfa (Medicago sativa) tolerance against salinity during seed germination by nitric oxide pathway. Plant Soil 351, 107–119. doi: 10.1007/s11104-011-0936-2
Zakar, T., Laczko-Dobos, H., Toth, T. N., Gombos, Z. (2016). Carotenoids assist in cyanobacterial photosystem II assembly and function. Front. Plant Sci. 7, 295. doi: 10.3389/fpls.2016.00295
Zhai, S., Xia, X., He, Z. (2016). Carotenoids in staple cereals: metabolism, regulation, and genetic manipulation. Front. Plant Sci. 7, 227. doi: 10.3389/fpls.2016.01197
Zhang, H., Hu, L. Y., Hu, K. D., He, Y. D., Wang, S. H., Luo, J. P. (2008). Hydrogen sulfide promotes wheat seed germination and alleviates oxidative damage against copper stress. J. Integr. Plant Biol. 50 (12), 1518–1529. doi: 10.1111/j.1744-7909.2008.00769.x
Zhang, H., Wang, M. J., Hu, L. Y., Wang, S. H., Hu, K. D., Bao, L. J., et al. (2010a). Hydrogen sulfide promotes wheat seed germination under osmotic stress. Russian J. Plant Physiol. 57 (4), 532–539. doi: 10.1134/S1021443710040114
Zhang, Z. S., Jia, Y. J., Gao, H. Y., Zhang, L. T., Li, H. D., Meng, Q. W. (2010). Characterization of PSI recovery after chilling-induced photoinhibition in cucumber (Cucumis sativus L.) leaves. Planta 234 (5), 883–889. doi: 10.1007/s00425-011-1447-3
Zhang, H., Tan, Z. Q., Hu, L. Y., Wang, S. H., Luo, J. F., Jones, R. L. (2010b). Hydrogen sulfide alleviates aluminum toxicity in germinating wheat seedlings. J. Integr. Plant Biol. 52, 556–567. doi: 10.1111/j.1744-7909.2010.00946.x
Zhang, H. H., Zhong, H. X., Wang, J. F., Sui, X., Xu, N. (2016). Adaptive changes in chlorophyll content and photosynthetic features to low light in Physocarpus amurensis Maxim and Physocarpus opulifolius "Diabolo". Peer J. 4 (3), e2125. doi: 10.7717/peerj.2125
Zhang, H. H., Xu, N., Li, X., Jin, W. W., Tian, Q., Sun, G. Y., et al. (2017). Overexpression of 2-Cys Prx increased salt tolerance of photosystemII in tobacco. Int. J. Agric. Biol. 19 (4), 735–745. doi: 10.17957/IJAB/15.0348
Zhang, H. H., Xu, N., Wu, X. Y., Wang, J. R., Ma, S. L., Li, X., et al. (2018a). Effects of 4 kinds of sodium salt stress on plant growth, PS II and PS I function in leaves of Sorghum. J. Plant Interact. 13 (1), 506–513. doi: 10.1080/17429145.2018.1526978
Zhang, H. H., Xu, N., Teng, Z. Y., Wang, J. R., Ma, S. L., Wu, X. Y., et al. (2019a). 2-Cys Prx plays a critical role in scavenging H2O2 and protecting photosynthetic function in leaves of tobacco seedlings under drought stress. J. Plant Interact. ,14 (1), 119–128. doi: 10.1080/17429145.2018.1562111
Zhang, H. H., Feng, P., Yang, W., Sui, X., Li, X., Zhang, R. T., et al. (2018b). Effects of flooding stress on the photosynthetic apparatus of leaves of two Physocarpus cultivars. J. For. Res. 39 (4), 1049–1059. doi: 10.1007/s11676-017-0496-2
Zhang, H. H., Li, X., Zhang, S. B., Yin, Z. P., Zhu, W. X., Li, J. B., et al. (2018c). Rootstock alleviates salt stress in grafted mulberry seedlings: physiological and PSII Function responses. Front. Plant Sci. 9, 1806. doi: 10.3389/fpls.2018.01806
Zhang, H. H., Shi, G. L., Shao, J. Y., Li, X., Li, M. B., Meng, L., et al. (2019b). Photochemistry and proteomics of mulberry (Morus alba L.) seedlings under NaCl and NaHCO3 stress. Ecotoxicol. Environ. Safety 184 (30), 109624. doi: 10.1016/j.ecoenv.2019.109624
Keywords: hydrogen sulfide, low temperature, blueberry seedlings, photosynthetic, reactive oxygen species, proline
Citation: Tang X, An B, Cao D, Xu R, Wang S, Zhang Z, Liu X and Sun X (2020) Improving Photosynthetic Capacity, Alleviating Photosynthetic Inhibition and Oxidative Stress Under Low Temperature Stress With Exogenous Hydrogen Sulfide in Blueberry Seedlings. Front. Plant Sci. 11:108. doi: 10.3389/fpls.2020.00108
Received: 05 July 2019; Accepted: 24 January 2020;
Published: 28 February 2020.
Edited by:
Isabel Lara, Universitat de Lleida, SpainReviewed by:
Jose Ignacio Garcia-Plazaola, University of the Basque Country, SpainLuca Vitale, National Research Council of Italy (CNR), Italy
Copyright © 2020 Tang, An, Cao, Xu, Wang, Zhang, Liu and Sun. This is an open-access article distributed under the terms of the Creative Commons Attribution License (CC BY). The use, distribution or reproduction in other forums is permitted, provided the original author(s) and the copyright owner(s) are credited and that the original publication in this journal is cited, in accordance with accepted academic practice. No use, distribution or reproduction is permitted which does not comply with these terms.
*Correspondence: Xiaojia Liu, MjY5NjE4NTI5QHFxLmNvbQ==; Xiaogang Sun, c3VueGlhb2dhbmdAamxhdS5lZHUuY24=
†These authors have contributed equally to this work