- 1Department of Forestry and Natural Resources, Hardwood Tree Improvement and Regeneration Center, Purdue University, West Lafayette, IN, United States
- 2John T. Harrington Forestry Research Center, New Mexico State University, Las Cruces, NM, United States
- 3Department of Botany and Plant Pathology, Purdue University, West Lafayette, IN, United States
Quercus spp. (oaks) are generally intermediate in shade tolerance, yet there is large variation within the genus in shade tolerance and plasticity in response to varying resource availability. Ecophysiological knowledge specific to semi-evergreen Quercus spp. from subtropical maritime forests is lacking relative to temperate deciduous oaks. We studied the influence of light availability and plant competition on leaf physiology and performance of semi-evergreen Quercus virginiana on a barrier island along the US southern Atlantic coast. Seedlings were underplanted in pine (Pinus taeda) plantation stands with varying overstory density (clear-cut, heavy thin, light thin, and non-thinned; creating a gradient of understory light availability) and vegetation (no competition removal or herbaceous competition removal) treatments. After 2 years, seedling survival was higher with increasing light availability (clear-cut = heavy thin > light thin > non-thinned). Seedling growth (i.e., diameter, height, and crown width) increased similarly with increasing thinning intensity, while vegetation control was mainly beneficial to seedling growth in clear-cuts. These responses were partially explained by foliar nitrogen and leaf trait measurements, which followed the same pattern. Q. virginiana seedlings demonstrated high plasticity in their ability to acclimate to varying resource availability, as indicated by light response curves, specific leaf area, stomatal density, stomatal pore index, and maximum theoretical stomatal conductance. Light compensation and saturation points illustrated seedling capacity to increase net CO2 assimilation with increased light availability. Leaves on trees in the high light environment had the highest net CO2 assimilation, stomatal density, stomatal pore index, maximum theoretical stomatal conductance, and lowest specific leaf area. Although we demonstrated the relative shade tolerance of Q. virginiana in lower light environments (i.e., heavy and light thin plots), this semi-evergreen species shows high plasticity in capacity to respond to varying resource availability, similar to other Quercus spp. from mesic and Mediterranean environments.
Introduction
Plasticity is an adaptive strategy to promote survival and fitness of long-lived forest tree species that may experience several environmental changes and associated stresses throughout their lifespan (Cavender-Bares and Ramírez-Valiente, 2017; Gil-Pelegrín et al., 2017a). Phenotypic plasticity, both physiological and morphological, affects life history traits and contributes to the large distribution ranges (Gratani et al., 2003; Niinemets, 2015) and wide ecophysiological variation (Niinemets and Valladres, 2006; Gil-Pelegrín et al., 2017b) of Quercus spp. (oaks). From deciduous to semi-evergreen or evergreen Quercus spp., plasticity has commonly been identified as a trait contributing to greater drought, cold, and shade tolerance (Valladares et al., 2002; Gimeno et al., 2009; Limousin et al., 2012; Ramírez-Valiente et al., 2017). Plasticity relates to how populations may respond to climate change as well as the development of improved management activities (Paquette et al., 2007; Gimeno et al., 2009). Plasticity is also important in understanding mechanisms that lead to regeneration and restoration success (Benito-Garzón et al., 2013; Lawson and Michler, 2014; Löf et al., 2019).
Quercus spp. are generally intermediate in shade tolerance and underplanting may provide an effective means to restore these species (Dey et al., 2012). Increased light and soil moisture resulting from overstory density reduction may benefit planted Quercus seedlings, but also pioneer species (Dey et al., 2008; Dey et al., 2012; Kern et al., 2012; Villar-Salvador, 2016). Pioneer species that acclimate rapidly and take advantage of higher light levels are particularly competitive, often suppressing oak seedling survival and growth (Paquette et al., 2006; Dey et al., 2008; Gardiner et al., 2010). Limited light, nutrients, and water resources from competition can negatively affect physiological processes, inhibiting seedling performance (Salifu et al., 2009; Grossnickle, 2012). Removal of competing vegetation, therefore, has potential to channel limited resources to planted seedlings, yet can be logistically prohibitive (Wagner and Zasada 1991; Fleming et al., 2006). Alternatively, maintaining partial overstory may introduce sufficient light to optimize growth in the target species, while restricting faster growing competition (Elliott and Swank, 1994; Paquette et al., 2006; Brown et al., 2014).
To facilitate forest restoration, there has been increased investigation in converting pine plantations to diverse hardwood forests using clear-cutting or thinning followed by planting of desired species (Parker et al., 2001; Gómez-Aparicio et al., 2009; Löf et al., 2010; Villar-Salvador, 2016; Lesko and Jacobs, 2018). Overstory removal treatments affect light, temperature, soil moisture, and soil compaction in complex feedback loops, which are dependent on species and ecosystems (Canham et al., 1990; Madrigal-González et al., 2017; Soto et al., 2017). Thus, understanding how silvicultural treatments affect plasticity and adaptive potential of target species regeneration may accelerate restoration processes by increasing availability of light, water, and nutrients.
Maritime forests of the subtropical US southern Atlantic coast, characterized by the dominant, semi-evergreen Quercus virginiana L., represent a case study where conversion of pine plantations back to diverse native hardwood forests may facilitate the restoration of associated ecosystem services (Albers and Alber, 2003; Jones et al., 2013). Within the range of Q. virginiana, there has been centuries of human land transformation, particularly on the more stable land where maritime forests develop (Bratton and Miller, 1994; Bellis, 1995; Fox et al., 2007; Jones et al., 2013). A fraction of the original estimated land area of maritime forests remains at approximately 39,000 ha, which has created interest to protect and restore maritime forests (Mathews et al., 1980; Lopazanski et al., 1988).
Many agricultural lands that were originally maritime forests on the US southern Atlantic coast were abandoned and more recently planted into pine plantations (i.e., Pinus taeda L.) for commercial investment and to minimize erosion (Fox et al., 2007; Brockerhoff et al., 2008). Pine plantations tend to perform poorly when exposed to inherent coastal stressors and abandoned monoculture pine plantations with low genetic diversity are particularly prone to disease and outbreaks of southern pine beetles (Dendroctonus frontalis Zimm.), which are an economically destructive forest pest due to exponential outbreaks (Conner et al., 2005; Fox et al., 2007; Brockerhoff et al., 2008; Watson et al., 2013; Nowak et al., 2015; Asaro et al., 2017). Clear-cuts are used to salvage residual timber value and reduce continual spread of active outbreak sites, while overstory thinning helps to minimize future outbreaks in at-risk stands (Belanger et al., 1993; Watson et al., 2013; Asaro et al., 2017). The complete or partial removal of the pine overstory provides an opportunity to restore maritime forest by regenerating Q. virginiana.
Our objective was to better understand the underlying physiological mechanisms that drive plant structure, physiology, and function of Q. virginiana, as a semi-evergreen oak. Quercus virginiana L. has a broad distribution across maritime edaphic site factors compared to other maritime oaks (Cavender-Bares and Pahlich 2009), suggesting potential for high plasticity. While the effects of varying resources such as temperature and precipitation has been studied in Q virginiana (Kurtz et al., 2013; Ramírez-Valiente et al., 2015; Cavender-Bares and Ramírez-Valiente, 2017), the species response to light and competition has yet to be explored. We experimentally evaluated the relative influence of pine overstory density and vegetation control treatments on Q. virginiana seedling performance. We hypothesized that Q. virginiana survival, growth, and leaf development would peak in the thinned treatments, when competition was controlled, reflective of the relative shade tolerance of most Quercus spp. Under this scenario, seedlings should show higher net CO2 assimilation and greater growth and development due to increased light compared to the control, while avoiding excessive light in the clear-cut.
Materials and Methods
Experimental Site
The experiment was conducted on the north end of St. Simon’s Island, Georgia at Cannon’s Point Preserve (N 31°15’29“ W 81°20’45”), which is a 246-ha wilderness tract with approximately 50 ha dominated by abandoned pine plantations (mostly P. taeda L. with some P. elliotti Englem.). Tree rings and cores indicated that the pine stands were approximately 50 years old. In 2015 and 2016, areas of natural and planted pines affected by southern pine beetles were clear-cut to salvage timber and reduce the southern pine beetle outbreak.
Soils at Cannon’s Point Preserve are a mixture of fine sandy soils dominated by Mandarin fine sand and Cainhoy fine sand, 0–5% slopes. Pottsburg sand and Rutledge fine sand are also present (NRCS, 2017). At each plot, four soil samples were composited to evaluate physical and chemical characteristics using Mehlich III extraction (Brookside Laboratories, New Brennan, Ohio). Soil characteristics were similar with slight differences creating variability across replicate blocks (Table 1).
This region receives an average annual precipitation of 114 cm and average annual temperature was 20.0°C. During the study period 2017–2018, average annual precipitation was 90 cm and temperature was 21.0°C (Sapelo Island National Estuarine Research Reserve Meterological Monitoring, 2018; U.S. Climate Data, 2018). Hurricane Irma (September 2017; 7 months into experiment) resulted in temporarily increased precipitation, saltwater inundation, salt spray, and strong winds across the region, yet no damage to our experimental site.
Experimental Design and Treatments
A randomized complete block design with a split-plot structure was used for this study. The whole plot factor consisted of four overstory densities (clear-cut, heavy thin, light thin, and non-thinned). The subplot factor was two levels of vegetation control (no vegetation removal and 2 years of vegetation removal). A total of 25 seedlings were planted within each subplot. All treatment combinations were replicated by four blocks resulting in 800 total seedlings.
Overstory density treatments were randomly applied to a 66 × 44 m area. Within the treated area, 26 × 14 m research plots were established. All plots were fenced (2.5 m height) to exclude white-tailed deer (Odocoileus virginianus Zimm.) as herbivory is cited as a limiting site factor in maritime forest restoration (Thyroff et al., 2019). Overstory density treatments were installed by modifying the basal area of the original pine overstory. Target basal areas were clear-cut at 0 m2 ha−1, heavy thin at 4–9 m2 ha−1, light thin at 18–23 m2 ha−1, and non-thinned at 27+ m2 ha−1. Logging operations to implement overstory treatments were completed in December 2016. Target basal areas were monitored by a contracted forester and logger. Additionally, all mid-story trees, understory vegetation, and large slash were removed immediately after the harvest activity to reduce possible confounding effects. Subplots requiring vegetation control over 2 years were done so throughout both growing seasons using mechanical methods (i.e., brush saws and hand clippers).
Plant Material
One-year-old Q. virginiana bare-root seedlings were planted in February 2017. Seedlings were obtained from Superior Trees in Lee, Florida with a Louisiana seed source. From baseline morphology analysis (n = 20), mean seeding diameter was 5 mm (± 0.20), mean seedling height was 54 cm (± 2.00), and root to shoot dry mass (g) ratio was 0.89 (± 0.76). Seedlings were sorted prior to planting and randomly assigned treatments. Seedlings were hand planted with planting bars at 2-m spacing. To maintain planting density and interspecific seedling competition, a perimeter of buffer trees was planted 2 m from the research seedlings.
Plot Characteristics
For each plot, basal area and canopy closure data were collected in summer 2017. All mature tree species within each fenced plot and the buffer areas around them were identified and measured for diameter at breast height (DBH; 1.37 m) to the nearest centimeter. DBH of mature trees were used to calculate the basal area. Three hemispherical photographs were taken under homogeneous diffuse sky conditions and across the centerline, working from west to east cardinal directions, at approximately 4.7-m intervals (1/3 of the plot width). Photographs were analyzed with CIMES software (Gonsamo et al., 2011) to determine percent canopy closure.
To record soil moisture and temperature, each plot had an Em50 digital data logger with two 5TM sensors (Decagon, Pullman, Washington) located in the subplot center. Each sensor was installed at a depth of 25 cm and recorded measurements every 2 h. Four of the plots (all of block 1) had additional sensors installed; photosynthetically active radiation (PAR) sensors to monitor light and VP4 sensors to capture air temperature and relative humidity recorded every 2 h. At the peak of vegetation cover on site (September 2018), five seedlings from each treatment (160 total) were randomly selected for a 1-m2 plot vegetation survey to assess percent competing vegetation cover, mean height of competition, and top competing species within each plot.
Seedling Performance
At the time of planting (February 2017), measurements of ground line diameter and height to last live bud were recorded. At the end of the growing seasons (November 2017 and 2018), survival, diameter, height, and crown width were recorded. Survival was recorded as a binary response; “alive” included seedlings with any number of green leaves. At the end of the second growing season (November 2018), foliar nitrogen (N) was determined by randomly sampling seven seedlings per subplot, resulting in 224 total sampling units. Three leaves per seedling were collected and composited, dried at 60°C for 72 h, weighed, pulverized in vials with stainless steel balls, and analyzed with an ECS 4010 CHNSO Analyzer (Costech, Valencia, California).
Ecophysiology and Leaf Trait Measurements
Gas exchange, specific leaf area (SLA), stomatal density, stomatal pore index (SPI), and maximum theoretical stomatal conductance (gmax) were measured during the growing season (June 2018). Leaf gas-exchange was measured with a portable LI-6400XT (LI-COR Biosciences, Lincoln, Nebraska) to create light response curves. Two Q. virginiana seedlings were randomly selected per subplot, resulting in 64 total sampling units. One upper-canopy, fully expanded, recently mature leaf per tree was measured between the hours 10:00 and 14:00. Light levels used to create light response curves were: 1,600, 1,400, 1,200, 1,000, 800, 600, 400, 300, 200, 100, 50, 0 (µmol m−2 s−1). Infrared gas analyzers of the LI-6400XT (IRGAs; reference and sample) were matched at the beginning and end of each light curve measurement. Relative humidity (∼ 60%), vapor pressure deficit (<3.0 kPa), and temperature (leaf and block) were monitored for consistency. The gas exchange data point was taken after sample gas values (H2O and CO2) and net CO2 assimilation were stable, based on coefficient of variation. Q. virginiana leaves did not fully fill the 2 × 3 cm LI-6400XT leaf chamber, therefore, gas-exchange measurements were adjusted for actual leaf areas. Leaf areas were determined from a photo of the leaf in the chamber using ImageJ (National Institutes of Health, Bethesda, Maryland). Light response curves were created by plotting net CO2 assimilation (AN, µmol CO2 m−2 s−1) against PAR. The curves were fitted to a non-rectangular hyperbola (SigmaPlot V11.0, Systat Software, San Jose, California). Methodology to calculate final parameters from the model followed Chartier and Prioul (1976). Final parameters were used to calculate light compensation (µmol m−2 s−1) and light saturation (µmol m−2 s−1) points.
SLA, stomatal density, SPI, and gmax were sampled from the same four selected Q. virginiana seedlings per plot used for gas-exchange measurements. Three selected, upper-canopy, fully expanded, recently mature leaves were used for each seedling. In the non-thinned overstory some seedlings did not have many leaves, therefore in those cases only two leaves were collected. SLA was calculated by dividing leaf area by leaf mass (cm2 g−1). Collected whole leaves were scanned to measure leaf area (cm2) using ImageJ. Leaves were dried at 60°C for 48 h then weighed for leaf mass (g).
Impressions of the abaxial leaf surface were made in the middle of each leaf, midway between the midrib and the leaf margin. Leaf impressions were made on microscope slides using cyanoacrylate. Five leaf impression images (DCM 900 microscope CMOS Camera, Oplenic Optronics, Hangzhou, China) were taken of a 0.19 × 0.14 mm (0.0266 mm2) area under 40× magnification using a microscope (BH-2 microscope, Olympus, Tokyo, Japan). Stomatal counts were conducted using ImageJ and the cell counter plug-in (Kurt De Vos, University of Sheffield). For unbiased counting, all whole stomata were counted within the impression image area and stomata partially within the image were only counted on the top and right sides of the image area. Stomatal density (mm−2) was calculated by dividing the number of stomata in the image by image area. To calculate SPI and gmax, stomatal lengths and widths were calculated from the leaf impressions using ImageJ and using the Feret’s diameter measurement (Supplementary Appendix A). SPI was calculated by multiplying stomatal density by stomatal length squared, while gmax (mmol m−2 s−1) was calculated using stomatal length and width following equations from McElwain et al. (2016) methodology.
Statistical Analyses
All data was analyzed with R software version 3.5.3 (R Core Team, 2019) using: lme4 package (Bates et al., 2015) for general linear models, linear regressions, and logistic regression; nlme (Pinheiro et al., 2018) package for repeated measures models; multcomp package (Hothorn et al., 2008) for pairwise comparisons. Plot characteristics (basal area, canopy closure, and data loggers) were analyzed with general linear mixed models, with overstory as the fixed factor and block as a random factor. A logistic regression model was used to analyze survival with overstory, vegetation control, and interaction as fixed factors and block as a random factor. Diameter, height, and crown width were analyzed with repeated measures general linear mixed models with overstory, vegetation control, time, and resulting interactions as fixed factors; block and individual tree as random factors. Foliar N, light response curves including light compensation/saturation points, leaf traits (i.e., SLA, stomatal density, SPI, gmax), and vegetation survey dependent variables were analyzed separately with general linear mixed models, with overstory, vegetation control, and interaction as fixed factors and block as a random factor. Linear regression models were used to analyze light saturation points and growth parameters. Residuals from all response variables were tested to ensure normality and homogeneity of variance. Crown width did not meet assumptions and data was square root transformed. For all analyses, when significant treatment effects were detected (p ≤ 0.05), Tukey’s HSD test was used to test for pairwise comparisons (α = 0.05). All statistical output results are provided in Appendices B and C. Although the number of sampling units from each experimental unit varied across measurements (per details above), the number of experimental units was always n = 4.
Results
Plot Characteristics
Basal area (m2 ha−1), canopy closure (%), light (PAR), mean air temperature (°C), and mean soil temperature (°C) followed a progression of overstory density (Table 2). Clear-cuts had the lowest basal area and canopy closure, resulting in greatest PAR. This pattern was consistent along the light progression with thinned plots having intermediate basal area and canopy closure. Non-thinned plots had the greatest basal area and canopy closure, resulting in the lowest PAR. Mean air and soil temperature increased with increased thinning density resulting in highest temperatures in clear-cut plots. From April 2017 to May 2018, average soil moisture was consistently greater in vegetation control subplots than non-vegetation control subplots. Soil moisture peaked in late summer/early autumn and was often greatest in heavy thin plots, followed by clear-cut and light thin, and lastly non-thinned plots (Figure 1).

Table 2 Mean (± SE) target basal area from logging operation, basal area from stand inventory, canopy closure from hemispherical photos.
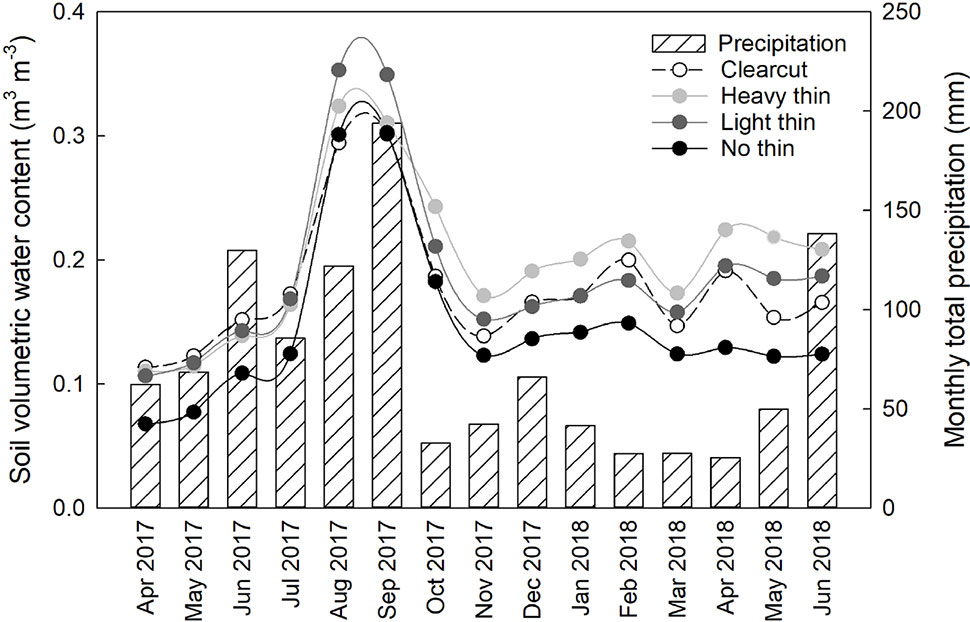
Figure 1 Mean soil volumetric water content (m3 m−3) from all plots, averaged by overstory treatment from April 2017 to June 2018. Volumetric water content was recorded every 2 h. Secondary y axis, monthly total precipitation (mm) from Sapelo Island National Estuarine Research Reserve Meteorological Monitoring station from April 2017 to June 2018.
The interaction of overstory and vegetation control was significant for percent vegetation cover (F3,54 = 6.84, p = 0.001) as the effect of vegetation control was different between the overstory treatments. Vegetation control decreased percent vegetation cover in clear-cut, heavy thin, and light thin plots, but had no effect in non-thinned plots (Figure 2). Additionally, height of competing vegetation was 58.3 cm (± 14.4) in non-vegetation control subplots compared to 18.8 cm (± 3.0) in vegetation control subplots (F1,57 = 35.56, p < 0.001). Top competing species included: Ilex vomitoria, Paspalum notatum, Rubus trivialis, Vitis rotundifolia, and Morella cerifera.
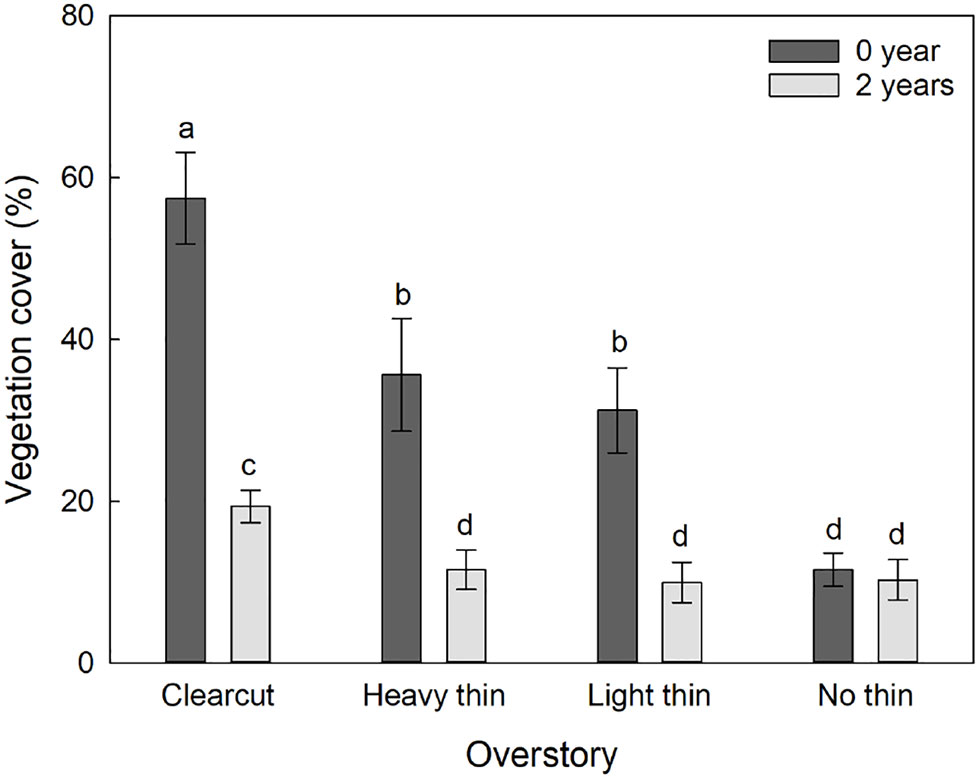
Figure 2 Mean (± SE) vegetation cover (%) of competing vegetation in a 1-m2 survey around Quercus virginiana seedlings planted in clear-cut, heavy thin, light thin, no thin plots taken at the end of the second growing season. Competing vegetation removed for 0 or 2 years. Different letters indicate significant differences among treatments (α = 0.05).
Seedling Performance
Initial height and diameter of planted seedlings were similar across all treatments with an average height of 48 cm (± 0.9) and an average diameter of 3.8 mm (± 0.1). Overall survival after two growing seasons was 75.5% (± 1.9). The treatment interaction was not significant, however the main effect of overstory was significant with increased survival with increased thinning intensity (X23,794 = 9.86, p = 0.020); clear-cut and heavy thin plots had the highest survival at 81.5 and 81.0%, light thin was intermediate at 73.0%, and non-thinned had the lowest survival at 65.5%.
The interaction of overstory, vegetation control, and time was significant for diameter (F6,595 = 13.30, p < 0.001), height (F6,1217 = 3.22, p = 0.004), and crown width (F3,587 = 13.00, p < 0.001). The effect of vegetation control differed among the overstory density treatments and the effects varied over time. Overall diameter, height, and crown width increased with increased thinning intensity. Vegetation control was most beneficial for seedlings in clear-cut plots followed by heavy thin plots and had no effect in non-thinned plots (Figure 3).
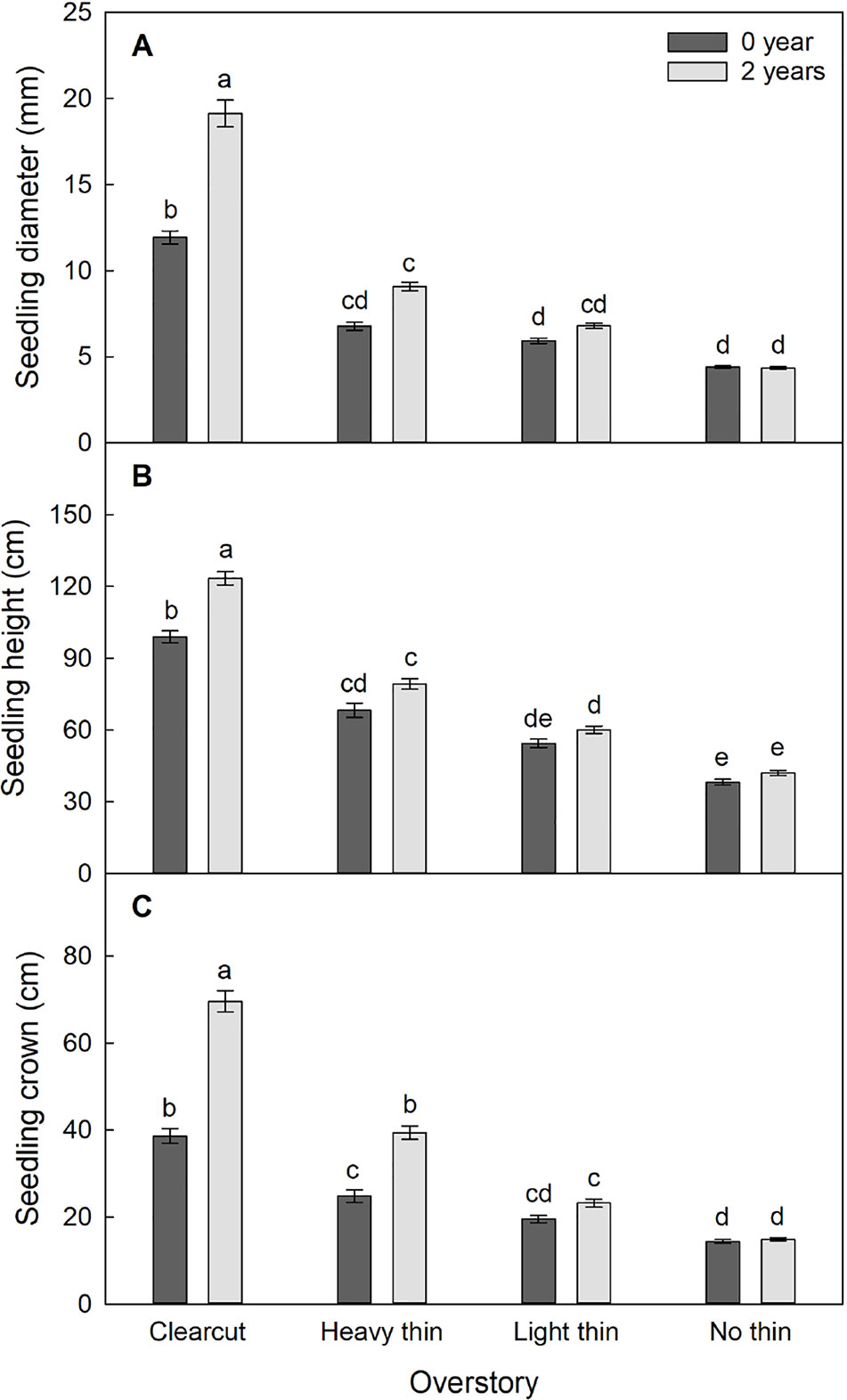
Figure 3 Mean (± SE) (A) diameter (mm), (B) height (cm), and (C) crown width (cm) growth of Quercus virginiana seedlings after the second (2018) growing season. Planted in overstory density (clear-cut, heavy thin, light thin, or no thin plots) and vegetation control treatments (0 or 2 years of subplots). Different letters indicate significant differences among treatments (α = 0.05).
Seedling diameter increased by 400% in clear-cut plots with vegetation control and 200% in clear-cut plots without vegetation control, while marginal growth occurred in non-thinned plots regardless of vegetation control treatment. Seedling height and crown width increased by 200% in clear-cut plots with vegetation control and 100% in clear-cut plots without vegetation control, while non-thinned plots had little growth regardless of treatment. Dieback occurred frequently in the non-thinned plots, which resulted in negative relative heights after two growing seasons.
Ecophysiology and Leaf Trait Measurements
For foliar N, only main effects were significant showing increased foliar N with increased thinning intensity (F3,217 = 24.06, p < 0.001) and increased foliar N with vegetation control (F1,217 = 34.94, p < 0.001). While the interaction was non-significant the overstory main effect was significant for the calculated light compensation (F3,57 = 8.10, p < 0.001) and light saturation points (F3,57 = 23.56, p < 0.001). With increased thinning intensity, light compensation and saturation points increased. Net CO2 assimilation was greatest in clear-cut plots, intermediate in heavy and light thin, lowest in non-thinned plots (Figure 4), and positively related to growth parameters (Supplementary Appendix D). Additionally, the vegetation control main effect was significant only for light saturation point with greater light saturation points in subplots with vegetation control compared to subplots without vegetation control (F1,57 = 5.56, p = 0.022).
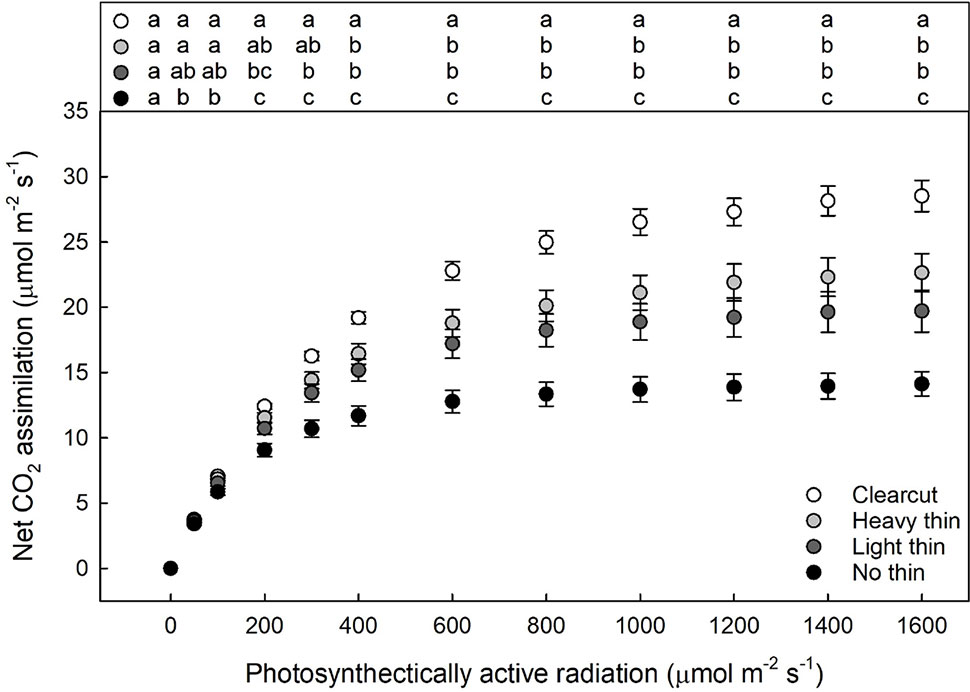
Figure 4 Mean light response curves (net photosynthesis plotted by photosynthetically active radiation) of Quercus virginiana seedlings planted either in clear-cut, heavy thin, light thin, or no thin plots taken in June 2018. Different letters indicate significant differences among treatments (α = 0.05).
For SLA, stomatal density, SPI, and gmax, only main effects were significant. With increased thinning intensity, SLA decreased (F3,57 = 12.60, p < 0.001), while stomatal density (F3,57 = 21.20, p < 0.001), SPI (F3,57 = 24.19, p < 0.001), and gmax (F3,57 = 24.48, p < 0.001) all increased (Figure 5). Vegetation control resulted in decreased SLA (F1,57 = 6.28, p = 0.015), and increased stomatal density (F1,57 = 5.37, p = 0.024), SPI (F1,57 = 6.55, p = 0.013), and gmax (F1,57 = 5.69, p = 0.020). More abaxial trichomes (i.e., leaf hairs) occurred on stomatal density impression images from clear-cut plots followed by heavy thin, light thin, and lastly no trichomes in non-thinned plots (F3,57 = 6.38, p < 0.001; Figure 6).
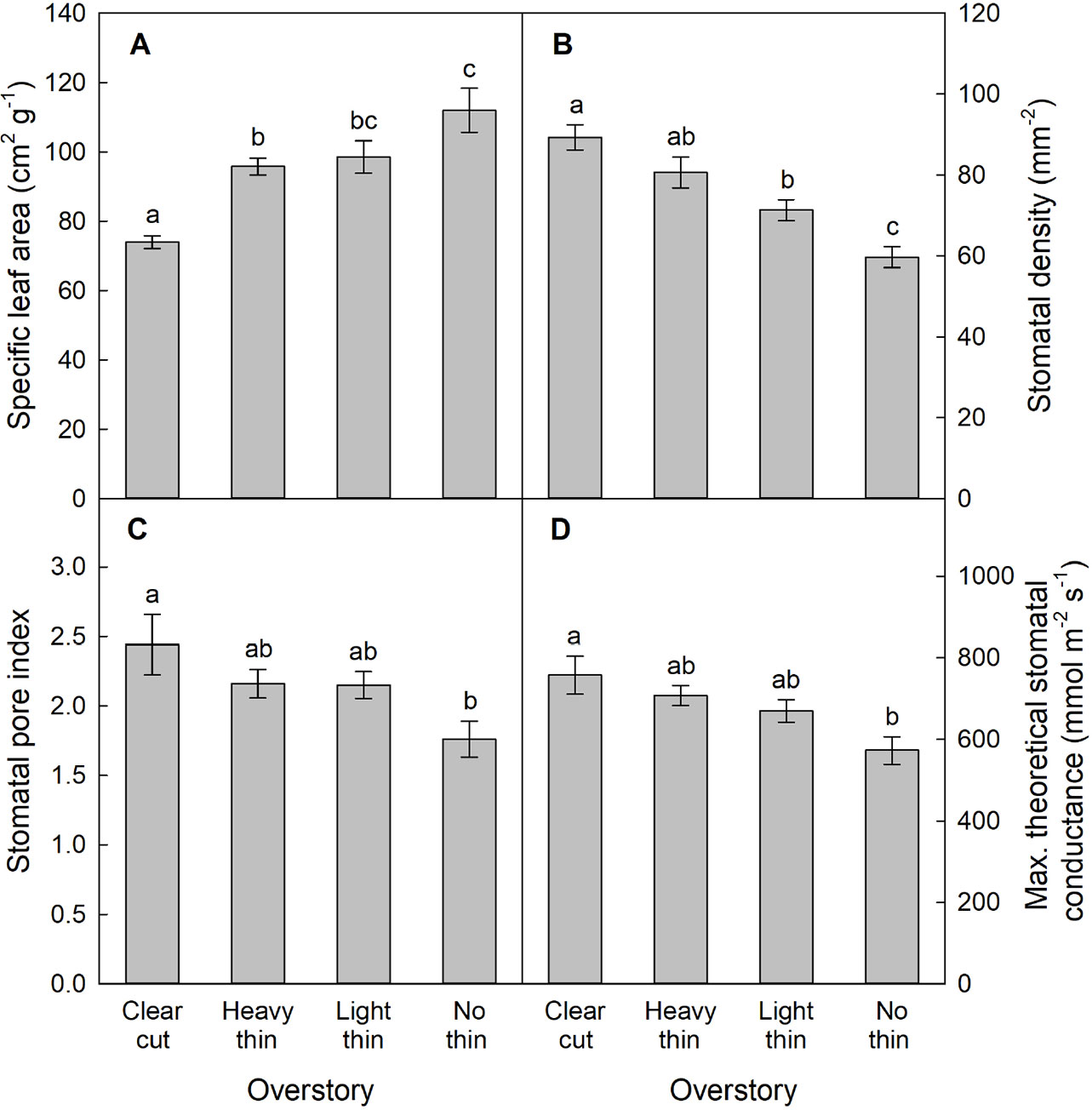
Figure 5 Mean (± SE) (A) specific leaf area (cm2 g−1), (B) stomatal density (stomata mm−2), (C) stomatal pore index, and (D) maximum theoretical stomatal conductance (gmax, mmol m−2 s−1) of Quercus virginiana seedlings planted in clear-cut, heavy thin, light thin, or no thin plots. Different letters indicate significant differences among treatments (α = 0.05).
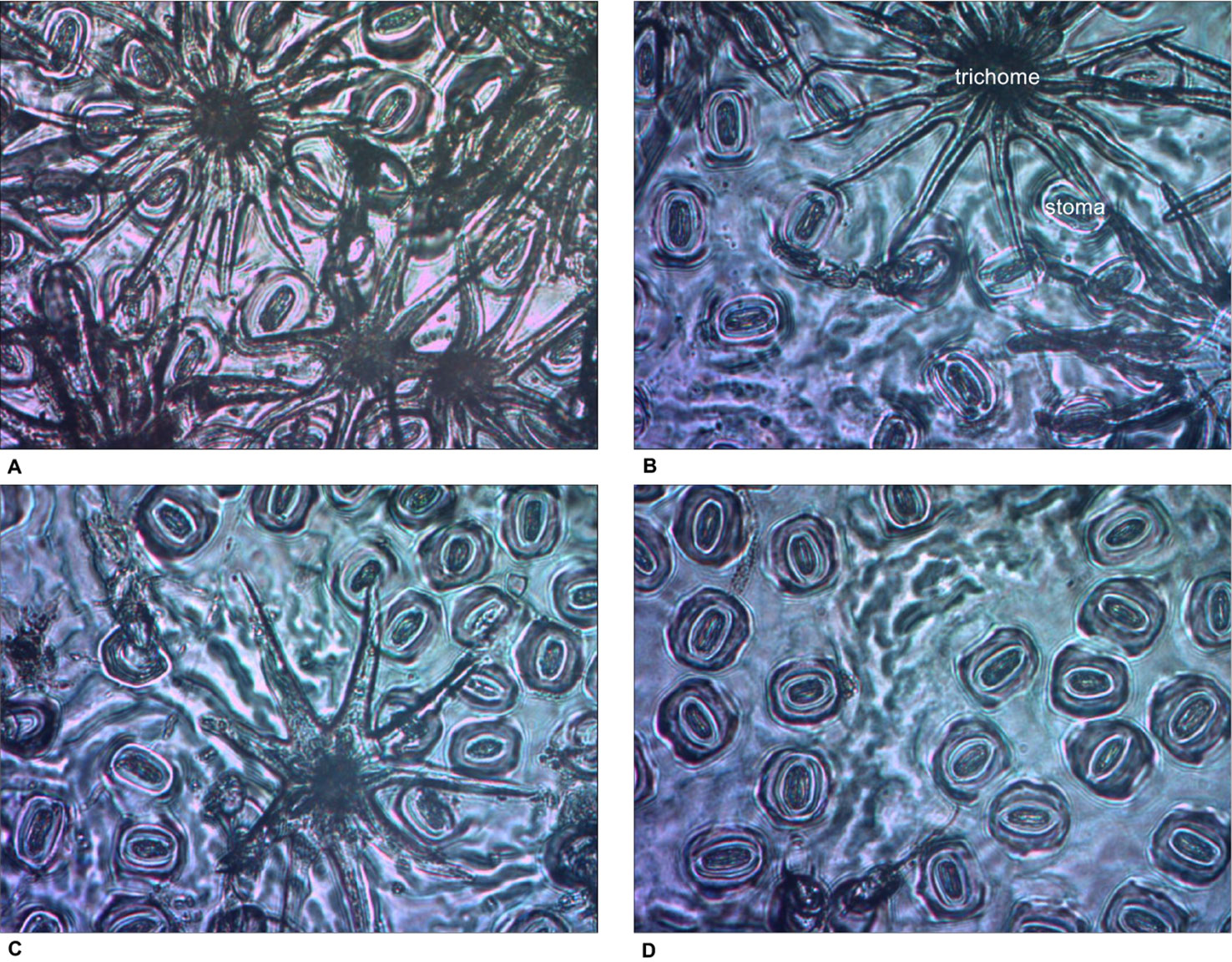
Figure 6 Impression images of abaxial trichomes Quercus virginiana seedlings planted in each of the overstory treatments [(A) clear-cut, (B) heavy thin, (C) light thin, and (D) no thin].
Discussion
Light and Competing Vegetation
Removal of stand basal area affects environmental characteristics such as light, temperature, and soil moisture, which influence seedling performance (Gil-Pelegrín et al., 2017b; Villar-Salvador, 2016). In our study, the pattern reflected in the PAR results (clear-cut had greatest PAR, followed by the thinned overstories, and lastly non-thinned) corresponded with increased seedling survival, diameter, height, crown width, and foliar N. Growth parameters were consistently greatest in clear-cut plots, intermediate in thinned plots, and lowest in non-thinned plots, rather than peaking in the thinned treatments as we hypothesized. Foliar N, which is an essential macronutrient for seedling establishment and performance (Abrams and Mostoller, 1995; Soto et al., 2017), was significantly greater in leaves of seedlings in clear-cut plots followed by the thinned overstories, indicating greater accessibility of this limiting resource to seedlings (Kobe, 2006; Uscola et al., 2015). Similar to other studies, seedlings in less dense overstory treatments were able to use available resources, and thereby increase photosynthesis and growth (Gómez-Aparicio et al., 2006; Cooper et al., 2014; Soto et al., 2017). Supporting our results, Q. virginiana has been reported as a fast growing species that preferentially allocates resources to aboveground biomass development (Cavender-Bares and Pahlich, 2009).
In a dense overstory, such as the non-thinned plots, soil moisture and nutrients (e.g., N as seen with low foliar N concentrations) are typically limited because of canopy tree dominance and competition (Cooper et al., 2014). Seedlings in non-thinned plots with dense overstories may have arrested development due to low light levels preventing their progression from seedlings to saplings (Zavala et al., 2011; Soto et al., 2019). Artificially regenerated Q. virginiana did not appear to benefit from the shade of the pine plantations as identified with Q. ilex L. (Gómez-Aparicio et al., 2009); however, this advantage may be expressed during a prolonged drought or on drier coastal sites or if studying natural regeneration. Thus, effects may also be due to the density of the pine plantations (33.9 m2 ha−1 basal area) in our study. Sites with pine overstory densities closer to the heavy thin treatment (16.9 m2 ha−1 basal area) may see the benefit of directly underplanting in a plantation.
As hypothesized, we found a consistent interaction between overstory and vegetation control treatments, with vegetation control benefiting growth parameters in clear-cut plots and not in non-thinned plots (Figure 3). Without thinning, light and soil moisture were more limiting to Q. virginiana performance than understory vegetation competition. Semi-evergreen Quercus spp. have varying photosynthetic responses to different environments, with a range of growth rates and shade tolerance (Gratani et al., 2003; Cavender-Bares and Ramírez-Valiente, 2017; Villar et al., 2017). Within the clear-cut and heavy thinning treatments, higher PAR contributed to understory vegetation becoming more abundant and thus more competitive (Ter-Mikaelian et al., 1999). In our study, vegetation control promoted seedling growth, particularly on sites with more light (i.e., clear-cut and heavy thin). The significant interaction illustrated a shift in pressure from light limited environments to resource limited environments (i.e., greater foliar N concentrations and increased soil moisture in vegetation control subplots). Overall, our results align with past studies indicating that vegetation control enhances seedling establishment and performance, particularly when released from dense overstories (Wagner and Zasada, 1991; Fleming et al., 2006). In cases where competing vegetation is expected to be high and vegetation control is not feasible, however, maintaining an overstory may reduce competition and the need for costly and sometimes controversial (i.e., herbicide) competing vegetation removal (Thiffault and Roy, 2011; Löf et al., 2014; Villar-Salvador, 2016).
Ecophysiology and Leaf Trait Responses
Trees are long lived organisms that may experience several environmental changes and associated stresses and plasticity is an adaptive strategies to promote survival under environmental fluctuations (Cavender-Bares and Ramírez-Valiente, 2017; Gil-Pelegrín et al., 2017a). Our results indicate that Q. virginiana can acclimate to varying environments as maximum net CO2 assimilation occurred in clear-cuts (Figure 4), which aligns with the increased growth and foliar N in clear-cut plots. With higher light compensation and saturation points, seedlings in clear-cuts took longer to achieve positive CO2 assimilation, but were able to utilize increased PAR with higher net CO2 assimilation rates. Similar to studies with other oaks, Q. virginiana seedlings responded to the other overstory treatments in a linear progression with respect to light availability (Cavender-Bares and Pahlich, 2009; Cooper et al., 2014). Seedlings in the non-thinned plots reached positive net CO2 assimilation quickest at the lowest PAR; however, non-thinned seedlings also reached light saturation point quicker at a lower PAR. Net CO2 assimilation, therefore, was limited and lowest for seedlings in non-thinned plots. Greater net CO2 assimilation rates were supported by more stomata, not larger stomata (Supplementary Appendix A), resulting in greater gas exchange potential as seen with stomatal density, SPI, and gmax. Ability to regulate stomatal development (stomatal density and SPI) and capacity for high gmax rates leads to seedling acclimation to a wide range of environments. Additionally, seedlings in more shaded environments with decreased SPI can allow for higher water use efficiency (Yoo et al., 2009; McElwain et al., 2016; Liu et al., 2017). In other studies, Mediterranean evergreen oaks such as Quercus oleoides Schltdl. & Cham. and Quercus ilex have also shown phenotypic plasticity in growth rates, CO2 assimilation, and leaf development (Cavender-Bares and Ramírez-Valiente, 2017; Gil-Pelegrín et al., 2017b; Ramírez-Valiente et al., 2017). Niinemets and Valladares (2006) classified Q. virginiana as drought-tolerant and intermediate in shade tolerance. Not only is maximizing performance under high-light conditions beneficial, but also the capability of shade adaptation is beneficial to seedling survival (Valladares and Niinemets, 2008). Similarly, Quercus velutina Lam. is a drought-tolerant and light demanding oak for which Ashton and Berlyn (1994) showed great leaf anatomical plasticity with high net CO2 assimilation compared to other temperate deciduous oaks.
Leaf variation of Quercus spp. tends to be on the lower end of the leaf economic spectrum, aligning with early to mid-successional classification (Wright et al., 2004; Niinemets and Valladres, 2006; Gil-Pelegrín et al., 2017b). Following a conservative resource strategy, lower SLA leaves on semi-evergreen oaks can help to maintain function and extend photosynthesis (Cavender-Bares and Ramírez-Valiente, 2017). Thicker leaves are also more resistant to environmental stressors such as aridity, freezing temperatures, and solar radiation (Cavender-Bares and Ramírez-Valiente, 2017; Gil-Pelegrín et al., 2017b; Pemán et al., 2017). Whereas SLA was negatively associated with CO2 assimilation, stomatal density was positively associated with CO2 assimilation. Similarly, Ramírez-Valiente et al. (2017) found that SLA was negatively associated with net CO2 assimilation and Q. seedling growth. In clear-cut plots with low SLA (i.e., smaller, thicker leaves), seedling growth was greatest. Higher stomatal density and SPI increases gas exchange potential and gmax, which increases net CO2 assimilation (Wright et al., 2004; McElwain et al., 2016). Along with increased gas exchange potential, comes increased risk of desiccation; therefore, a trade-off is necessary to maximize performance, which was seen in the opposite associations of SLA and stomatal density with net CO2 assimilation. Trichomes are commonly found in many Quercus spp. and trichomes in the abaxial surface may be a mechanism to reduce water loss (Bickford, 2016; Gil-Pelegrín et al., 2017b) as seen in the higher light plots (Figure 6). Additionally, a smaller leaf typically has a thinner leaf boundary layer, which facilitates cooling in drier and warmer climates (e.g., clear-cuts) (Gil-Pelegrín et al., 2017b). In our experiment, Q. virginiana showed phenotypic plasticity for growth rates, gas exchange, and leaf development in response to silvicultural treatments that modified seedling environments as was observed in similar evergreen oak regeneration studies (Ramírez-Valiente et al., 2015).
Conclusions
We demonstrated that Q. virginiana is capable of acclimating to varying resource availability, with a high tolerance to full sunlight. Developmental responses of underplanted Q. virginiana did not follow the predicted trend of peaking in the heavy/light thin pine plantation overstory, but rather in clear-cuts. Nonetheless, thinning is among the most effective practices for preventing or mitigating pine beetle outbreaks by creating barriers to population growth and spread (Nowak et al., 2015; Asaro et al., 2017). Additionally, a canopy buffering-effect in thinned stands may be more beneficial for oaks on drier sites or during prolonged drought (García-Plazaola et al., 2017). Thus, rather than prescribing a single treatment (e.g., clear-cut) that optimizes Q. virginiana development, an alternative may be to prescribe several treatments that result in cost-effective restoration and reduce the need for costly competing vegetation control. Forest species with high plasticity and rapid growth, such as Q. virginiana in our study, will obtain rapid canopy closure, allowing favorable conditions for establishment of associated mid-and late-seral maritime forest species (Gómez-Aparicio et al., 2009; Bertacchi et al., 2016), helping to create resilient, diverse, and complex forests (Löf et al., 2019).
Data Availability Statement
The datasets generated for this study are available on request to the corresponding author.
Author Contributions
ET designed and installed the experiment, collected and analyzed the data, and co-wrote the paper. OB helped to design and install the experiment, contributed to data analysis and interpretation, and co-wrote the paper. MM assisted with stomatal data collection and interpretation, and co-wrote the paper. DJ supervised the research, helped to design the experiment, and co-wrote the paper.
Funding
Funding support was provided by the USDA National Institute of Food and Agriculture, McIntire Stennis projects IND011535 and NMSU1002447, Hardwood Tree Improvement and Regeneration Center, Fred M. van Eck Forest Foundation, John T. Harrington Forestry Center, and St. Simon’s Land Trust.
Conflict of Interest
The authors declare that the research was conducted in the absence of any commercial or financial relationships that could be construed as a potential conflict of interest.
Acknowledgments
St. Simon’s Land Trust provided access to research sites. In kind support from the St. Simon’s Land Trust, The Nature Conservancy, and Georgia Department of Natural Resources. Thank you to Stephanie Knox, Susan Shipman, Andrei Toca, Michael Gosney, Mercedes Uscola, Michael Szuter, Caleb Reddick, Madeline Montague, Edward Oehlman, Bailey Elkins, Noel Mano, Sofia Sifnaios, Jacob Thompson, and Tate Holbrook for valuable assistance with this project.
Supplementary Material
The Supplementary Material for this article can be found online at: https://www.frontiersin.org/articles/10.3389/fpls.2019.01526/full#supplementary-material
References
Abrams, M. D., Mostoller, S. A. (1995). Gas exchange, leaf structure and nitrogen in contrasting successional tree species growing in open and understory sites during a drought. Tree Physiol. 15, 361–370. doi: 10.1093/treephys/15.6.361
Albers, G., Alber, M. (2003). A vegetative survey of back-barrier islands near Sapelo Island, Georgia. Proc. 2003 Georg. Water Resour. Conf., 1–4.
Asaro, C., Nowak, J. T., Elledge, A. (2017). Why have southern pine beetle outbreaks declined in the southeastern U.S. with the expansion of intensive pine silviculture? A brief review of hypotheses. For. Ecol. Manage. 391, 338–348. doi: 10.1016/j.foreco.2017.01.035
Ashton, P. M. S., Berlyn, G. P. (1994). A comparison of leaf physiology and anatomy of Quercus (Section Erythrobalanus-Fagaceae) species in different light environments. Am. J. Bot. 81, 589–597. doi: 10.1002/j.1537-2197.1994.tb15489.x
Bates, D., Machler, M., Bolker, B., Walker, S. (2015). Fitting linear mixed-effects models using lme4 . J. Stat. Software 67, 1–48.
Belanger, R. P., Hedden, R. L., Lorio, P. L. J. (1993). Management strategies to reduce losses from the southern pine beetle. South. J. Appl. For. 17, 150–154. doi: 10.1093/sjaf/17.3.150
Bellis, V. J. (1995). Ecology of maritime forests of the southern Atlantic coast: a community profile. Natl. Biol. Serv. U.S. Dep. Inter., 1–95.
Benito-Garzón, M., Ha-Duong, M., Frascaria-Lacoste, N., Fernández-Manjarrés, J. (2013). Habitat restoration and climate change: dealing with climate variability, incomplete data, and management decisions with tree translocations. Restor. Ecol. 21, 530–536. doi: 10.1111/rec.12032
Bertacchi, M. I. F., Amazonas, N. T., Brancalion, P. H. S., Brondani, G. E., de Oliveira, A. C. S., de Pascoa, M. A. R., et al. (2016). Establishment of tree seedlings in the understory of restoration plantations: natural regeneration and enrichment plantings. Restor. Ecol. 24, 100–108. doi: 10.1111/rec.12290
Bickford, C. P. (2016). Ecophysiology of leaf trichomes. Funct. Plant Biol. 43, 807–814. doi: 10.1071/FP16095
Bratton, S. P., Miller, S. G. (1994). Historic field systems and the structure of maritime oak forests, Cumberland Island National Seashore, Georgia. Bull. Torrey Bot. Club 121, 1–12. doi: 10.2307/2996879
Brockerhoff, E. G., Jactel, H., Parrotta, J. A., Quine, C. P., Sayer, J. (2008). Plantation forests and biodiversity: oxymoron or opportunity? Biodivers. Conserv. 17, 925–951. doi: 10.1007/s10531-008-9380-x
Brown, C. E., Mickelbart, M. V., Jacobs, D. F. (2014). Leaf physiology and biomass allocation of backcross hybrid American chestnut (Castanea dentata) seedlings in response to light and water availability. Tree Physiol. 34, 1362–1375. doi: 10.1093/treephys/tpu094
Canham, C. D., Denslow, J. S., Platt, W. J., Runkle, J. R., Spies, T. A., White, P. S. (1990). Light regimes beneath closed canopies and tree-fall gaps in temperate and tropical forests. Can. J. For. Res. 20, 620–631. doi: 10.1139/x90-084
Cavender-Bares, J., Pahlich, A. (2009). Molecular, morphological, and ecological niche differentiation of sympatric sister oak species, Quercus virginiana and Q. geminata (Fagaceae). Am. J. Bot. 96, 1690–1702. doi: 10.3732/ajb.0800315
Cavender-Bares, J., Ramírez-Valiente, J. (2017). “Chapter 4: Physiological evidence from common garden experiments for local adaptation and adaptive plasticity to climate. American live oaks (Quercus Section Virentes): implications for conservation under global change,” in Oaks Physiological Ecology. Exploring the Functional Diversity of Genus Quercus L (Cham, Switzerland: Springer International Publishing), 107–135.
Chartier, P., Prioul, J. L. (1976). The effects of irradiance, carbon dioxide and oxygen on the net photosynthetic rate of the leaf: a mechanistic model. Photosynthetica 10, 20–24.
Conner, W. H., Mixon, W. D., Wood, G. W. (2005). Maritime forest habitat dynamics on Bulls Island, Cape Romain National Wildlife Refuge, SC, following Hurricane Hugo. For. Ecol. Manage. 212, 127–134. doi: 10.1016/j.foreco.2005.03.008
Cooper, A., Shapira, O., Zaidan, S., Moshe, Y., Zangy, E., Osem, Y. (2014). Oak restoration in water-limited pine plantations: interactive effects of overstory light interception and water availability on understory oak performance. Eur. J. For. Res. 133, 661–670. doi: 10.1007/s10342-014-0794-6
Dey, D. C., Jacobs, D., McNabb, K., Miller, G., Baldwin, V., Foster, G. (2008). Artificial regeneration of major oak (Quercus) species in the Eastern United States–a review of the literature. For. Sci. 54, 77–106. doi: 10.1093/forestscience/54.1.77
Dey, D. C., Gardiner, E. S., Schweitzer, C. J., Kabrick, J. M., Jacobs, D. F. (2012). Underplanting to sustain future stocking of oak (Quercus) in temperate deciduous forests. New For. 43, 955–978. doi: 10.1007/s11056-012-9330-z
Elliott, K. J., Swank, W. T. (1994). Impacts of drought on tree mortality and growth in a mixed hardwood forest. J. Veg. Sci. 5, 229–236. doi: 10.2307/3236155
Fleming, R. L., Powers, R. F., Foster, N. W., Kranabetter, J. M., Scott, D. A., Ponder, F., et al. (2006). Effects of organic matter removal, soil compaction, and vegetation control on 5-year seedling performance: a regional comparison of Long-Term Soil Productivity sites. Can. J. For. Res. 36, 529–550. doi: 10.1139/x05-271
Fox, T. R., Jokela, E. J., Allen, H. L. (2007). The development of pine plantation silviculture in the southern United States. J. For. 105, 337–347. doi: 10.1093/jof/105.7.337
García-Plazaola, J., Hernández, A., Fernández-Marín, B., Esteban, R., Peguero-Pina, J., Verhoeven, A., et al. (2017). “Chapter 11: Photoprotective mechanisms in the Genus Quercus in response to winter cold and summer drought,” in Oaks Physiological Ecology. Exploring the Functional Diversity of Genus Quercus L (Cham, Switzerland:Springer International Publishing), 361–391.
Gardiner, E. S., Dey, D. C., Stanturf, J. A., Lockhart, B. R. (2010). Approaches to restoration of oak forests on farmed lowlands of the Mississippi River and its tributaries. Rev. Columbia For. 13, 223–236.
Gil-Pelegrín, E., Peguero-Pin, J. J., Sancho-Knapik, D. (2017a). Oaks physiological ecology. Exploring the functional diversity of genus Quercus L. Cham, Switzerland:Springer International Publishing. doi: 10.1007/978-3-319-69099-5
Gil-Pelegrín, E., Saz, M., Cuadrat, J., Peguero-Pina, J., Sancho-Knapik, D. (2017b). “Chapter 5: Oaks under Mediterranean-type climates: functional response to summer aridity,” in Oaks Physiological Ecology. Exploring the Functional Diversity of Genus Quercus L (Cham, Switzerland:Springer International Publishing), 137–193.
Gimeno, T. E., Pas, B., Lemos-Filho, J. P., Valladares, F. (2009). Plasticity and stress tolerance override local adaptation in the responses of Mediterranean holm oak seedlings to drought and cold. Tree Physiol. 29, 87–98. doi: 10.1093/treephys/tpn007
Gómez-Aparicio, L., Valladares, F., Zamora, R. (2006). Differential light responses of Mediterranean tree saplings: linking ecophysiology with regeneration niche in four co-occurring species. Tree Physiol. 26, 947–958. doi: 10.1093/treephys/26.7.947
Gómez-Aparicio, L., Zavala, M. A., Bonet, F. J., Zamora, R. (2009). Are pine plantations valid tools for restoring Mediterranean forests? An assessment along abiotic and biotic gradients. Ecol. Appl. 19, 2124–2141. doi: 10.1890/08-1656.1
Gonsamo, A., Walter, J.-M., Pellikka, P. (2011). CIMES: a package of programs for determining canopy geometry and solar radiation regimes through hemispherical photographs. Comput. Electron. Agric. 79, 207–215. doi: 10.1016/j.compag.2011.10.001
Gratani, L., Meneghini, M., Pesoli, P., Crescente, M. F. (2003). Structural and functional plasticity of Quercus ilex seedlings of different provenances in Italy. Trees 17, 515–521. doi: 10.1007/s00468-003-0269-8
Grossnickle, S. C. (2012). Why seedlings survive: influence of plant attributes. New For. 43, 711–738. doi: 10.1007/s11056-012-9336-6
Hothorn, T., Bretz, F., Westfall, P. (2008). Simultaneous inference in general parametric models. Biom. J. 50, 346–363. doi: 10.1002/bimj.200810425
Jones, G., Snider, A., Luo, S. (2013). Changes in the extent of North Carolina barrier island maritime forests 1988-2011: an assessment of past efforts at protection. J. For. 111, 186–193. doi: 10.5849/jof.12-022
Kern, C. C., Reich, P. B., Montgomery, R. A., Strong, T. F. (2012). Do deer and shrubs override canopy gap size effects on growth and survival of yellow birch, northern red oak, eastern white pine, and eastern hemlock seedlings? For. Ecol. Manage. 267, 134–143. doi: 10.1016/j.foreco.2011.12.002
Kobe, R. K. (2006). Sapling growth as a function of light and landscape-level variation in soil water and foliar nitrogen in northern Michigan. Oecologia 147, 119–133. doi: 10.1007/s00442-005-0252-8
Kurtz, C. M., Savage, J. A., Huang, I.-Y., Cavender-Bares, J. (2013). Consequences of salinity and freezing stress for two populations of Quercus virginiana Mill. (Fagaceae) grown in a common garden. J. Torrey Bot. Soc 140, 145–156. doi: 10.3159/TORREY-D-12-00060.1
Löf, M., Bergquist, J., Brunet, J., Karlsson, M., Welander, N. T. (2010). Conversion of Norway spruce stands to broadleaved woodland – regeneration systems, fencing and performance of planted seedlings. Ecol. Bull. 53, 165–173.
Löf, M., Bolte, A., Jacobs, D. F., Jensen, A. M. (2014). Nurse trees as a forest restoration tool for mixed plantations: effects on competing vegetation and performance in target tree species. Restor. Ecol. 22, 758–765. doi: 10.1111/rec.12136
Löf, M., Madsen, P., Metslaid, M., Witzell, J., Jacobs, D. F. (2019). Restoring forests: regeneration and ecosystem function for the future. New For. 50, 139–152. doi: 10.1007/s11056-019-09713-0
Lawson, S. S., Michler, C. H. (2014). Afforestation, restoration and regeneration - Not all trees are created equal. J. For. Res. 25, 3–20. doi: 10.1007/s11676-014-0426-5
Lesko, J., Jacobs, D. F. (2018).Conversion of conifer plantations to native hardwoods: influences of overstory and fertilization on artificial regeneration. New For. 49, 829–849. doi: 10.1007/s11056-018-9683-z
Limousin, J. M., Rambal, S., Ourcival, J. M., Rodríguez-Calcerrada, J., Pérez-Ramos, I. M., Rodríguez-Cortina, R., et al. (2012). Morphological and phenological shoot plasticity in a Mediterranean evergreen oak facing long-term increased drought. Oecologia 169, 565–577. doi: 10.1007/s00442-011-2221-8
Liu, C., He, N., Zhang, J., Li, Y., Wang, Q., Sack, L., et al. (2017). Variation of stomatal traits from cold temperate to tropical forests and association with water use efficiency. Funct. Ecol. 1–9. doi: 10.1111/1365-2435.12973
Lopazanski, M. J., Evans, J. P., Shaw, R. E. (1988). An assessment of maritime forest resources on the North Carolina coast. N. C. Dep. Nat. Resour. Community Dev. 1–104.
Madrigal-González, J., Ruiz-Benita, P., Ratcliffe, S., Rigling, A., Wirth, C., Zimmermann, N., et al. (2017). “Chapter 15: competition drives oak species distribution and functioning in Europe: implications under global change,” in Oaks Physiological Ecology. Exploring the Functional Diversity of Genus Quercus L (Cham, Switzerland:Springer International Publishing), 513–538.
Mathews, T. D., Stapor, F. W., Richter, C. R., Miglarese, J. V., McKenzi, M. D., Barlcay, L. A., et al. (1980). Ecological characterization of the Sea Island coastal region of South Carolina and Georgia. Volume I: physical features of the characterization area. Department of the Interior, Fish and Wildlife Service, Marine Resource Division.
McElwain, J. C., Yiotis, C., Lawson, T. (2016). Using modern plant trait relationships between observed and theoretical maximum stomatal conductance and vein density to examine patterns of plant macroevolution. New Phytol. 209, 94–103. doi: 10.1111/nph.13579
Natural Resources Conservation Service (2017). https://websoilsurvey.nrcs.usda.gov. United States Soil Survey, accessed on 2017-01-11.
Niinemets, Ü., Valladares, F. (2006). Tolerance to shade, drought, and waterlogging of temperate northern hemisphere trees and shrubs. Ecol. Monogr. 76, 521–547. doi: 10.1890/0012-9615(2006)076[0521:TTSDAW]2.0.CO;2
Niinemets, Ü. (2015). Is there a species spectrum within the world-wide leaf economics spectrum? Major variations in leaf functional traits in the Mediterranean sclerophyll Quercus ilex. New Phytol. 205, 79–96. doi: 10.1111/nph.13001
Nowak, J. T., Meeker, J. R., Coyle, D. R., Steiner, C. A., Brownie, C. (2015). Southern pine beetle infestations in relation to forest stand conditions, previous thinning, and prescribed burning: evaluation of the southern pine beetle prevention program. J. For. 113, 454–462. doi: 10.5849/jof.15-002
Paquette, A., Bouchard, A., Cogliastro, A. (2006). Successful under-planting of red oak and black cherry in early-successional deciduous shelterwoods of North America. Ann. For. Sci. 63, 823–831. doi: 10.1051/forest:2006065
Paquette, A., Bouchard, A., Cogliastro, A. (2007). Morphological plasticity in seedlings of three deciduous species under shelterwood under-planting management does not correspond to shade tolerance ranks. For. Ecol. Manage. 241, 278–287. doi: 10.1016/j.foreco.2007.01.004
Parker, W. C., Elliott, K. A., Dey, D. C., Boysen, E., Newmaster, S. G. (2001). Managing succession in conifer plantations: converting young red pine (Pinus resinosa Ait.) plantations to native forest types by thinning and underplanting. For. Chron. 77, 721–734. doi: 10.5558/tfc77721-4
Pemán, J., Chirino, E., Espelta, J., Jacobs, D., Martín-Gómez, P., Navarro-Cerrillo, R., et al. (2017). “Chapter 14: Physiological keys for natural and artificial regeneration of oaks,” in Oaks Physiological Ecology. Exploring the Functional Diversity of Genus Quercus L (Cham, Switzerland:Springer International Publishing), 453–511.
Pinheiro, J., Bates, D., DebRoy, S., Karkar, D. R. Core Team (2018). nlme: linear and nonlinear mixed effects models.
Ramírez-Valiente, J. A., Koehler, K., Cavender-Bares, J. (2015). Climatic origins predict variation in photoprotective leaf pigments in response to drought and low temperatures in live oaks (Quercus series Virentes). Tree Physiol. 35, 521–534. doi: 10.1093/treephys/tpv032
Ramírez-Valiente, J. A., Center, A., Sparks, J. P., Sparks, K. L., Etterson, J. R., Longwell, T., et al. (2017). Population-level differentiation in growth rates and leaf traits in seedlings of the neotropical live oak Quercus oleoides grown under natural and manipulated precipitation regimes. Front. Plant Sci. 8, 1–14. doi: 10.3389/fpls.2017.00585
Salifu, K. F., Jacobs, D. F., Birge, Z. K. D. (2009). Nursery nitrogen loading improves field performance of bareroot oak seedlings planted on abandoned mine lands. Restor. Ecol. 17, 339–349. doi: 10.1111/j.1526-100X.2008.00373.x
Sapelo Island National Estuarine Research Reserve Meteorological Monitoring (2018). http://cdmo.baruch.sc.edu/. accessed on 2018-12-31.
Soto, D. P., Jacobs, D. F., Salas, C., Donoso, P. J., Fuentes, C., Puettmann, K. J. (2017). Light and nitrogen interact to influence regeneration in old-growth Nothofagus-dominated forests in south-central Chile. For. Ecol. Manage. 384, 303–313. doi: 10.1016/j.foreco.2016.11.016
Soto, D. P., Puettmann, K. J., Fuentes, C., Jacobs, D. F. (2019). Regeneration niches in Nothofagus-dominated old-growth forests after partial disturbance: insights to overcome arrested succession. For. Ecol. Manage. 445, 26–36. doi: 10.1016/j.foreco.2019.05.004
Ter-Mikaelian, M. T., Wagner, R. G., Bell, F. W., Shropshire, C. (1999). Comparison of photosynthetically active radiation and cover estimation for measuring the effects of interspecific competition on jack pine seedlings. Can. J. For. Res. 29, 883–889. doi: 10.1139/x99-088
Thiffault, N., Roy, V. (2011). Living without herbicides in Québec (Canada): historical context, current strategy, research and challenges in forest vegetation management. Eur. J. For. Res. 130, 117–133. doi: 10.1007/s10342-010-0373-4
Thyroff, E. C., Burney, O. T., Jacobs, D. F. (2019). Herbivory and competing vegetation interact as site limiting factors in maritime forest restoration. Forests 10, 1–13. doi: 10.3390/f10110950
U.S. Climate Data (2018). https://www.usclimatedata.com/. accessed on 2018-12-31.
Uscola, M., Villar-Salvador, P., Gross, P., Maillard, P. (2015). Fast growth involves high dependence on stored resources in seedlings of Mediterranean evergreen trees. Ann. Bot. 115, 1001–1013. doi: 10.1093/aob/mcv019
Valladares, F., Niinemets, Ü. (2008). Shade tolerance, a key plant feature of complex nature and consequences. Annu. Rev. Ecol. Evol. Syst. 39, 237–257. doi: 10.1146/annurev.ecolsys.39.110707.173506
Valladares, F., Chico, J. M., Aranda, I., Balaguer, L., Dizengremel, P., Manrique, E., et al. (2002). The greater seedling high-light tolerance of Quercus robur over Fagus sylvatica is linked to a greater physiological plasticity. Trees 16, 395–403. doi: 10.1007/s00468-002-0184-4
Villar, R., Ruiz-Benito, P., de la Riva, E., Poorter, H., Cornelissen, J., Quero, J. (2017). “Chapter 12: Growth and growth-related traits for a range of Quercus species grown as seedlings under controlled conditions and for adult plants from the field,” in Oaks Physiological Ecology. Exploring the Functional Diversity of Genus Quercus L, (Cham, 1040 Switzerland: Springer International Publishing) vol. pp., 393–417.
Villar-Salvador, P. (2016). Restoration of Spanish pine plantations: a main challenge for the 21st century. Reforesta, 1, 53–66. doi: 10.21750/refor.1.04.4
Wagner, R. G., Zasada, J. C. (1991). Integrating plant autecology and silvicultural activities to prevent forest vegetation management problems. For. Chron. 67, 506–513. doi: 10.5558/tfc67506-5
Watson, A. C., Sullivan, J., Amacher, G. S., Asaro, C. (2013). Cost sharing for pre-commercial thinning in southern pine plantations: willingness to participate in Virginia’s pine bark beetle prevention program. For. Policy Econ. 34, 65–72. doi: 10.1016/j.forpol.2013.05.004
Wright, I. J., Reich, P. B., Westoby, M., Ackerly, D. D., Baruch, Z., Bongers, F., et al. (2004). The worldwide leaf economics spectrum. Nature 428, 821–827. doi: 10.1038/nature02403
Yoo, C. Y., Pence, H. E., Hasegawa, P. M., Mickelbart, M. V. (2009). Regulation of transpiration to improve crop water use. CRC Crit. Rev. Plant Sci. 28, 410–431. doi: 10.1080/07352680903173175
Keywords: Quercus virginiana, canopy openness, ecophysiology, gas exchange, leaf traits, light acclimation, plant competition, forest regeneration
Citation: Thyroff EC, Burney OT, Mickelbart MV and Jacobs DF (2019) Unraveling Shade Tolerance and Plasticity of Semi-Evergreen Oaks: Insights From Maritime Forest Live Oak Restoration. Front. Plant Sci. 10:1526. doi: 10.3389/fpls.2019.01526
Received: 07 July 2019; Accepted: 01 November 2019;
Published: 20 November 2019.
Edited by:
Giovanna Battipaglia, University of Campania Luigi Vanvitelli, ItalyReviewed by:
Enrique Andivia, Complutense University of Madrid, SpainLina Fusaro, Sapienza University of Rome, Italy
Copyright © 2019 Thyroff, Burney, Mickelbart and Jacobs. This is an open-access article distributed under the terms of the Creative Commons Attribution License (CC BY). The use, distribution or reproduction in other forums is permitted, provided the original author(s) and the copyright owner(s) are credited and that the original publication in this journal is cited, in accordance with accepted academic practice. No use, distribution or reproduction is permitted which does not comply with these terms.
*Correspondence: Douglass F. Jacobs, djacobs@purdue.edu