- 1LEAF—Linking Landscape Environment Agriculture and Food, Instituto Superior de Agronomia, University of Lisbon, Lisbon, Portugal
- 2Department of Environmental Science, Section for Environmental Microbiology and Biotechnology, Environmental Microbial Genomics Group (EMG), Aarhus University, Roskilde, Denmark
Phaeomoniella chlamydospora is a tracheomycotic fungus that colonizes the xylem of grapevines (Vitis vinifera L.), causing wood discoloration, brown wood streaking, gummosis, and wood necrosis, which negatively affect the overall health, productivity, and life span of vines. Current control strategies to prevent or cope with P. chlamydospora infections are frequently ineffective. Moreover, it is unclear how fungicides commonly applied in vineyards against downy and powdery mildew agents affect the wood mycobiome, including wood pathogens such as P. chlamydospora. In this study, we used next-generation sequencing to assess the effects of foliar spray of grapevines with inorganic (copper oxychloride and sulfur), synthetic (penconazole and fosetyl-aluminum), and natural (Blad) fungicides currently used against the downy and powdery mildews. The subjects of our investigation were (i) the resident wood mycobiome, (ii) the early colonization by a consortium of fungal wood endophytes (ACEA1), (iii) the wood colonization success of P. chlamydospora, and (iv) the in planta interaction between P. chlamydospora and ACEA1, under greenhouse conditions, in rooted grapevine cuttings of cv. Cabernet Sauvignon. The data obtained suggest that the resident mycobiome is affected by different fungicide treatments. In addition, the early colonization success of the endophytes composing ACEA1 varied in response to fungicides, with relative abundances of some taxa being overrepresented or underrepresented when compared with the control. The wood colonization by P. chlamydospora comported significant changes in the mycobiome composition, and in addition, it was greatly affected by the foliar spray with Blad, which decreased the relative abundance of this pathogen 12-fold (4.9%) when compared with the control (60.7%) and other treatments. The presence of the pathogen also decreased considerably when co-inoculated into the plant with ACEA1, reaching relative abundances between 13.9% and 2.0%, depending on the fungicide treatment applied. This study shows that fungicides sprayed to prevent infections of powdery and downy mildews have an effect on non-target fungi that colonize the endosphere of grapevines. We suggest two potential control strategies to fight P. chlamydospora, namely, the foliar spray with Blad and the use of ACEA1. Further studies to confirm these results are required.
Introduction
Protecting grapevines (Vitis vinifera L.) from fungal pests by means of fungicides dates back to the late 1800s, with the accidental discovery that a mixture of copper sulfate and lime, sprayed on vine leaves, was not only able to deter thieves from stealing grapes but also able to prevent downy mildew infections (Morton and Staub, 2008). Since then, research on fungicides improved the use of copper and sulfur as contact fungicides and introduced additional synthetic broad-spectrum systemic fungicides (e.g., benzimidazoles, triazoles, fludioxonil, and organophosphorus compounds) during the mid-late 1900s (Morton and Staub, 2008). Overuse of fungicides in vineyards came at a cost. Several were shown to be phytotoxic to grapevines (Dias, 2012; Juang et al., 2012) and toxic to humans and the environment (Komárek et al., 2010), and some affected non-target organisms (La Torre et al., 2018), while others hampered beneficial insects (Thomson et al., 2000). In addition, the development of pathogen resistance pushed the search of ever more effective chemicals capable of dealing with increasingly resilient species (Verweij et al., 2009).
It was only in recent years, with the advent of molecular ecology and its tools, such as metagenomics and metabarcoding, that scientists have begun to understand how fungicides, along with herbicides and insecticides, impact the microbial ecology of environments with unpredictable medium- and long-term consequences (Komárek et al., 2010; Setati et al., 2012; Karlsson et al., 2014; Morrison-Whittle et al., 2017). The vineyard is an extremely diverse environment, characterized by complex interactions among plants, soil microbes, endophytes, and epiphytes, which are associated not only with grapevine health (Zarraonaindia and Gilbert, 2015; Jayawardena et al., 2018) but also with the concept of terroir, as it is a source of yeasts and bacteria especially important in winemaking (Pretorius et al., 1999; Setati et al., 2012).
Concerning plant health, grapevine trunk diseases (GTDs) are currently a major issue in viticulture, considered by some as “the new Phylloxera” due to their potential destructive power (Bruez et al., 2013). Early scientific reports on GTDs are over a century old (Surico, 2009), and the reasons underlying their recent worldwide outbreak have not yet been fully addressed. Unlike powdery and downy mildews agents (Erysiphe necator and Plasmopara viticola) and Phylloxera (Daktulosphaira vitifoliae), which were introduced from the Americas and found a susceptible host in V. vinifera (Jackson, 2014), GTD pathogens have presumably been co-evolving with grapevines for centuries, if not millennia (Mugnai et al., 1999).
Copper and sulfur fungicides were introduced approximately two decades before the first scientific reports on GTDs and the recent outbreak began some 30 years ago (Surico et al., 2004; Fontaine et al., 2016), approximately two decades after the massive introduction of synthetic broad-spectrum systemic fungicides in vineyards. To date, no study has investigated the effect of these fungicides, and that of other natural active ingredients, on the wood mycobiome and its possible implications in GTDs. To address this issue, we used metabarcoding (Illumina® next-generation sequencing, NGS) and tested four hypotheses. (1) Are fungicides commonly sprayed in vineyards, to fight powdery and downy mildews, capable of affecting the resident wood mycobiome and/or (2) an inoculated consortium of fungal wood endophytes? (3) Is Phaeomoniella chlamydospora, a wood pathogen associated with several syndromes in grapevine (Surico 2009), affected by such fungicides, when artificially inoculated in wood? (4) Is the fungal consortium inoculation potentially exploitable in the control of early infections by P. chlamydospora, independently of fungicide treatments?
Materials and Methods
Experimental Setup
Plant and Fungal Material
The grapevines used in this assay were 1-year-old canes of cv. Cabernet Sauvignon, provided by the Viveiros VitiOeste nursery (Pó, Portugal), that were cut at three-bud-long size. The phytosanitary status of the propagation material was assessed by ensuring the absence of leaf symptoms and any visible wood symptomatology. The cuttings were rooted, potted in a mixture of peat and sand (1:1 v/v), and maintained under greenhouse conditions, at the temperature of 24 ± 5°C day/18 ± 5°C night.
The pathogenic ascomycete tested in this study was P. chlamydospora (CBS 161.90), from the CBS culture collection (Westerdijk Fungal Biodiversity Institute, Netherlands). Four grapevine endophytes, isolated from asymptomatic grapevine wood (cv. Cabernet Sauvignon), were used to produce the fungal consortium named “ACEA1,” namely, Alternaria (Al.) alternata A101, Epicoccum nigrum E279, Cladosporium sp. C22, Aureobasidium (Au.) pullulans AU86. All fungi were maintained in Petri dishes with vents, on potato dextrose agar medium (Difco™), at 25°C, in the dark.
Inoculum Preparation and Delivery
Four different inocula were prepared for delivery into the plant wood, three of them consisting of a suspension of conidia and/or fungal cells and the fourth of a control. Inoculum (i) consisted of a conidia suspension of P. chlamydospora, which was prepared by flooding a 2-week-old colony. The conidia were dislodged from the mycelium with a sterile glass rod, and the suspension was filtered through a double layer of cheesecloth. The conidial concentration was determined using a hemocytometer and adjusted to 1 × 105 conidia per milliliter with sterile distilled water (SDW). Inoculum (ii) consisted of ACEA1, which was prepared by joining conidia suspensions of ascomycetes Al. alternata, E. nigrum, Cladosporium sp., and cells of yeast Au. pullulans. Cultures growth conditions and conidia/cell suspension preparation occurred similarly to inoculum (i), where the final concentration of each one of the four fungi was 1 × 105 (conidia/cells) per milliliter. Inoculum (iii) was prepared by joining conidia suspensions of P. chlamydospora and ACEA1, in order to produce a solution with a final concentration of 1 × 105 (conidia/cells) per milliliter of each one of the five fungi. Inoculum (iv) consisted of SDW.
The delivery of the inocula into the rooted plants was performed as follows. Grapevine cuttings were surface disinfected with 70% (v/v) ethanol, and a wound in the wood was produced with the aid of a cork borer (4 mm diameter, 4 mm deep), approximately 1 cm below the green shoot (Figure 1). The four inocula were delivered with a micropipette by depositing 50 µl of solution in freshly made wounds, which were immediately sealed with Parafilm®. Each inoculum was delivered in 32 plants (Figure 1).
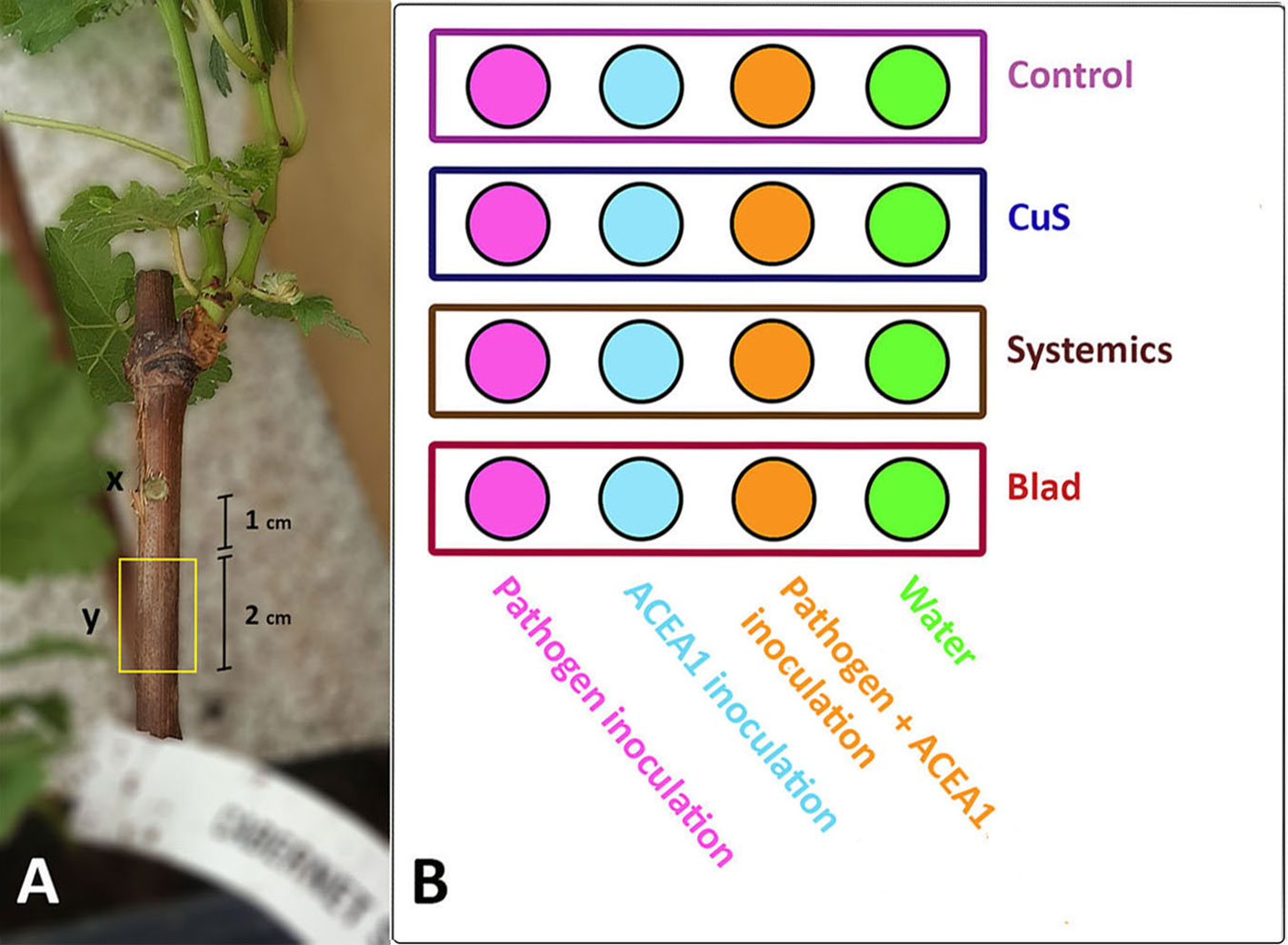
Figure 1 (A) Grapevine rooted cutting of cv. Cabernet Sauvignon. (x) Inoculation point of a conidia suspension of Phaeomoniella chlamydospora, ACEA1, P. chlamydospora and ACEA1, or sterile distilled water. (y) Two centimeters of wood, located 1 cm below the inoculation point, was sampled 3 months post-inoculation, and the wood’s mycobiome was examined through metabarcoding (n = 5 for each combination inoculum/treatment). (B) Experimental setup. Each circle represents a set of eight plants that underwent the same treatment. Same colors in the circles represent the same inoculum type, while rectangles represent different treatments. All possible combinations of inoculum and fungicide application by foliar spray are shown.
Treatments via Foliar Spray
Four treatments were tested for each inoculum type. The chemicals used and their application rate are reported in Table 1. The spray occurred every fortnight, starting from the seventh day post-inoculation and consisting in a total of six applications per treatment. Plants were sprayed on leaves and stem, with the exception of the control, which was sprayed exclusively on leaves. The chemicals were prevented from dripping onto the soil. All possible combinations of fungal inoculum and foliar treatment were tested, for a total of 16 combinations, each one consisting of eight biological replicates (Figure 1). The assay ended 1 week after the last spray (99 days post-inoculation).
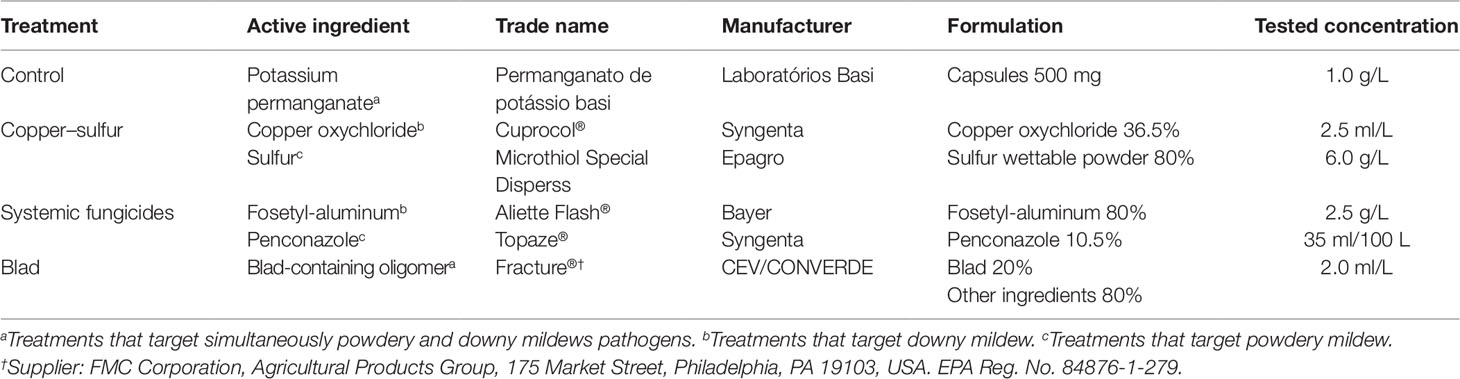
Table 1 Chemical treatments sprayed fortnightly on grapevine rooted cuttings. Six applications were performed during 3 months. Each active ingredient was sprayed separately.
Potassium permanganate (KMnO4) was chosen as control treatment due to the need of dealing with natural infections of powdery and downy mildews, which normally occur under greenhouse conditions. This chemical is effective against these pathogens due to its strong oxidative properties (La Torre et al., 2004), and we used the assumption that, when sprayed exclusively on leaves, it would not interact in a significant way with the wood mycobiome. Concerning treatments “Copper-Sulfur” and “Systemic fungicides,” the two active ingredients of each treatment were sprayed approximately 3 h apart from one another.
Assessment of the Plant Health Status
Three parameters were evaluated to assess the health status of the plants at the moment of sampling. First, a visual inspection of the leaves and green shoots to establish whether any symptomatology related to the pathogen or ACEA1 inoculation manifested (n = 8 for each combination inoculum/treatment). Second, the shoot length was recorded, to understand if inoculated fungi and/or treatments had an effect on the growth of the plants (n = 8 for each combination inoculum/treatment). Lastly, for each combination of inoculum/treatment, three vines were subject to a destructive inspection to assess the presence and degree of internal wood symptomatology (e.g., brown streaking) visible in the stem of plants and starting from the inoculation point. Three categories of symptomatology degree were established: Category 1, absence of wood symptoms; Category 2, presence of brown streaking in proximity of the inoculation point; and Category 3, presence of brown streaking that extended several centimeters from the inoculation point (Figure 2).
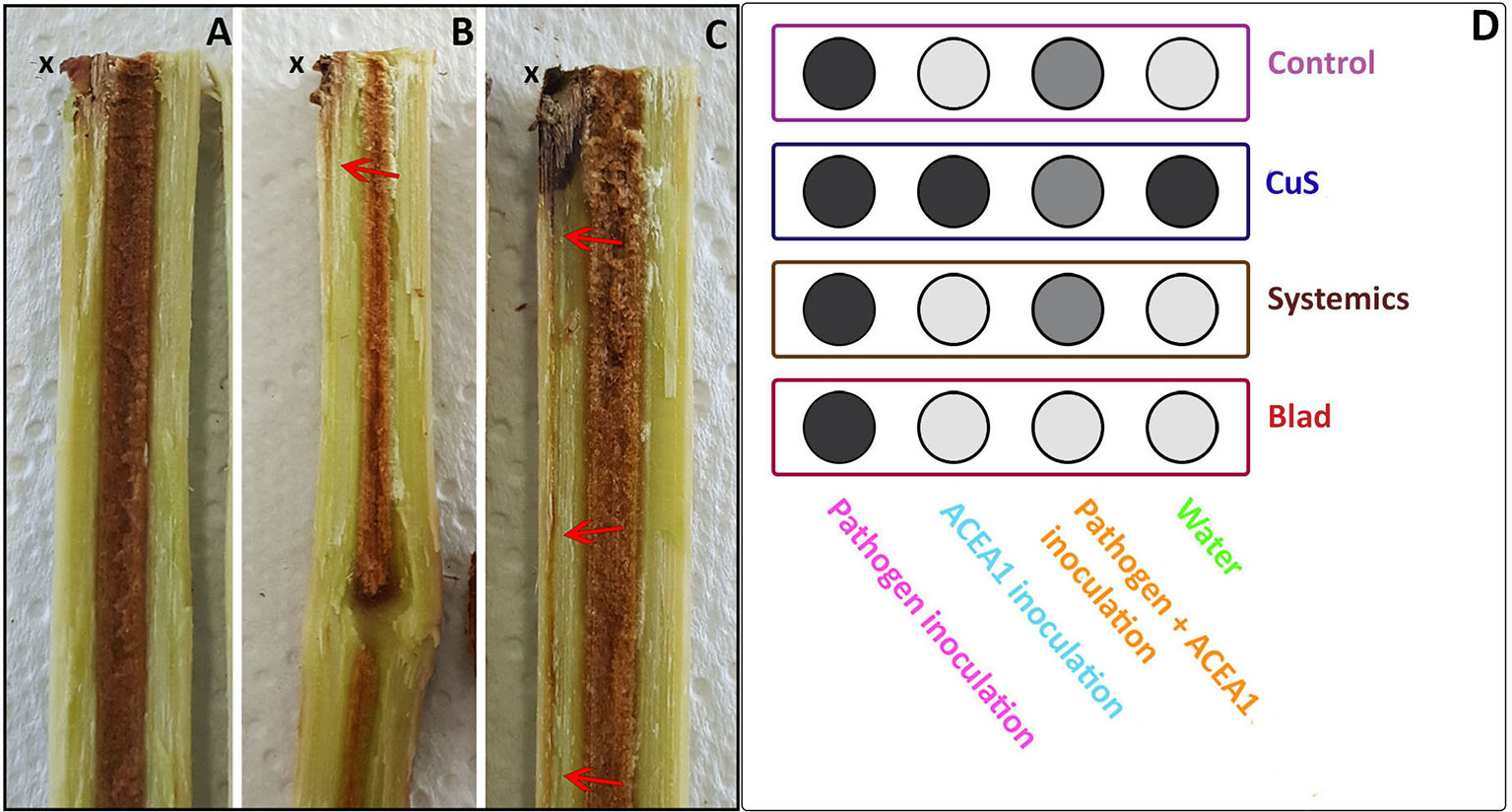
Figure 2 Grapevine rooted cuttings were examined, revealing different degrees of brown wood streaking symptomatology. Category 1, absence of symptoms (A) departing from the inoculation point (x) downwards; Category 2, presence of mild symptomatology (B); Category 3, extensive symptomatology (C). (D) Each category is presented in a different scale of gray, as an average of the observations for each treatment/inoculum (n = 3), where Category 1 is shown in light gray, Category 2 in gray, and Category 3 in dark gray.
Wood Sampling, DNA Extraction, and Amplification
Grapevines were uprooted and the stem processed under laboratory conditions (n = 5 for each combination inoculum/treatment). The bark was sterilized by dipping the stem in 70% (v/v) ethanol followed by flame sterilization and then removed with the aid of a sterile scalpel. Exactly 2 cm of wood was sampled, 1 cm below the inoculation point (Figure 1). Samples were frozen, freeze-dried, and stored at −80°C.
Wood samples were ground to dust using sterile mortars and pestles, aided by liquid nitrogen. An aliquot of ground wood (0.25 ± 0.01 g) of each sample was added to DNA extraction columns (FastDNA™ SPIN Kit for Soil, MP Biomediacals® LLC), and total DNA was extracted as described by the kit manufacturer. Three negative controls of the DNA extraction (no template) were included in this step and underwent the DNA extraction procedure.
The amplicon chosen for the metabarcoding analysis targets the internal transcribed spacer ITS2 region, and the primer set selected was ITS86F and ITS4R (Op De Beeck et al., 2014). For building libraries, we used a double-step PCR approach as reported by Feld et al. (2016). The full primer sequences including Illumina overhangs are ITS86F (5′-TCGTCGGCAGCGTCAGATGTGTATAAGAGACAG-GTGAATCATCGAATCTTTGAA-3′) and ITS4R (5′-GTCTCGTGGGCTCGGAGATGTGTATAAGAGACAG-TCCTCCGCTTATTGATATGC-3′).
Every first PCR contained 12.5 µl of Supreme NZYTaq II 2x Green Master Mix™ (NZYtech™), 0.5 µl of forward and reverse primers from a 10 µM stock, 1.5 µl of sterile water, and 5 µl of template. Each reaction was pre-incubated at 95°C for 2 min, followed by 40 cycles of 95°C for 15 s, 55°C for 15 s, and 72°C for 40 s; a further extension was performed at 72°C for 10 min. Negative controls of the PCRs were included alongside the samples. No-template DNA extracted samples also underwent the library preparation procedure.
A second PCR step for barcoding, MagBio bead fragment purification, and Qubit quantification were performed as reported in Gobbi et al. (2018). Final pooling was performed at 10 ng per sample. DNA sequencing was performed using an in-house Illumina MiSeq instrument and 2 × 250 paired-end reads with V2 Chemistry.
Bioinformatics
After sequencing, demultiplex was performed using an Illumina MiSeq platform, and the raw data were analyzed using QIIME 2 v. 2018.2 (Caporaso et al., 2010), using the same pipeline described in Gobbi et al. (2018); denoised reads were quality-trimmed by 15 bp on the left to remove adapters and primers by using the quality filter plugin implemented in QIIME 2 (Bokulich et al., 2013). Filtered reads were then analyzed using DADA2 with the amplicon sequence variant (ASV) methods (Callahan et al., 2017). To minimize barcoding noise, operational taxonomic unit (OTU) counts below 25 reads were filtered out across all samples. Taxonomic assignments were performed at 99% identity using QIIME feature-classifier classify-sklearn with a naïve Bayes classifier trained with UNITE (Nilsson et al., 2013) v. 7.2 for ITS. After taxonomy assignment, the dominant features assigned to high taxonomical ranks such as order, class, or family were further investigated using BLAST to refine the analyses (Bokulich et al., 2018). The raw data of this study are available in the European Nucleotide Archive (ENA accession number PRJEB32853).
Data Analysis
Shoot length data were subjected to analysis of variance (two-way ANOVA) to evaluate the effects of factors “fungicide treatment” and “inoculum type.” Significant means were compared using the Tukey post hoc test at a 5% significance level (GraphPad Prism 7.05).
The frequency table and its taxonomy table were combined, converted to biom format in QIIME (Caporaso et al., 2010), then merged with a table of metadata into an S4 object, and analyzed in R (v. 3.4.3) using the following packages: phyloseq, v. 1.22.3 (McMurdie and Holmes, 2013); vegan, v. 2.5.2 (Oksanen et al., 2007); DeSeq2 v. 1.22.1 (Love et al., 2014); ggplot2, v. 3.0.0 (Wickham, 2016); metacoder, v. 0.2.1.9005 (Foster et al., 2017); adespatial, v. 0.1.1 (Dray et al., 2018); data.table, v. 1.10.4.3 (Dowle and Srinivasan, 2017). R code is publicly available at github.com/Marieag/EMG.
The alpha diversity was measured using the Shannon diversity index and Pielou’s evenness and tested with one-way ANOVA with Tukey’s honestly significant difference (HSD) post hoc to determine differences among treatments and inoculum types.
We analyzed the beta dispersion to measure between-sample variances in abundance, computing average distances of the individual samples. The resulting ordination was plotted using non-metric multidimensional scaling (NMDS) combined with a Jaccard index matrix. To assess overall inter-group variance, we performed a PERMANOVA, using a Jaccard distance matrix with 999 permutations. The post hoc test for the PERMANOVA (performed on the ASV counts) was done using the adonis function from the vegan package in R.
To investigate any systematic changes between treatments, we calculated the differential abundance of any taxon with a relative abundance (RA) greater than 0.1% using DESeq2. Twenty-four pairwise analyses were run for every combination of fungicide treatment per inoculum type; log2 fold changes were calculated for each taxon resolved to species or genus level and tested for significance using a Wald test on the negative binomial distribution of the pairwise treatments. The P-value threshold was set to 0.05, and log fold change threshold to 0.1. In order to illustrate the results and to factor in the RA of unresolved species, we created differential heat trees using MetacodeR. A Wilcoxon rank sum test was applied to test differences between the same species in different treatments, and the resulting P-values were corrected for multiple comparisons using FDR, as implemented in MetacodeR. P-value threshold was set to 0.05.
Results
Evaluation of Plant Health Parameters
Visual inspection of grapevine cuttings, shoots length measurements, and wood examination occurred on the day of sampling. The visual inspection revealed no foliar symptomatology attributable to the wood pathogen P. chlamydospora or to the inoculated fungal consortium ACEA1.
The statistical analysis of the green shoot length measurements, performed as a two-way ANOVA, revealed no significant differences for the factor “inoculum type” (P = 0.190), meaning that shoot growth was not influenced by inoculation with the pathogen, ACEA1, or the combination of both. However, a positive effect was present when comparing the factor “fungicide treatment” (P < 0.01), revealing that Blad and/or systemic fungicides increased the shoot growth of inoculated plants (pathogen, ACEA1, and pathogen + ACEA1), when compared with one or both the two other treatments (Figure 3). No significant differences were detected from the interaction between the two factors under examination (P = 0.136).
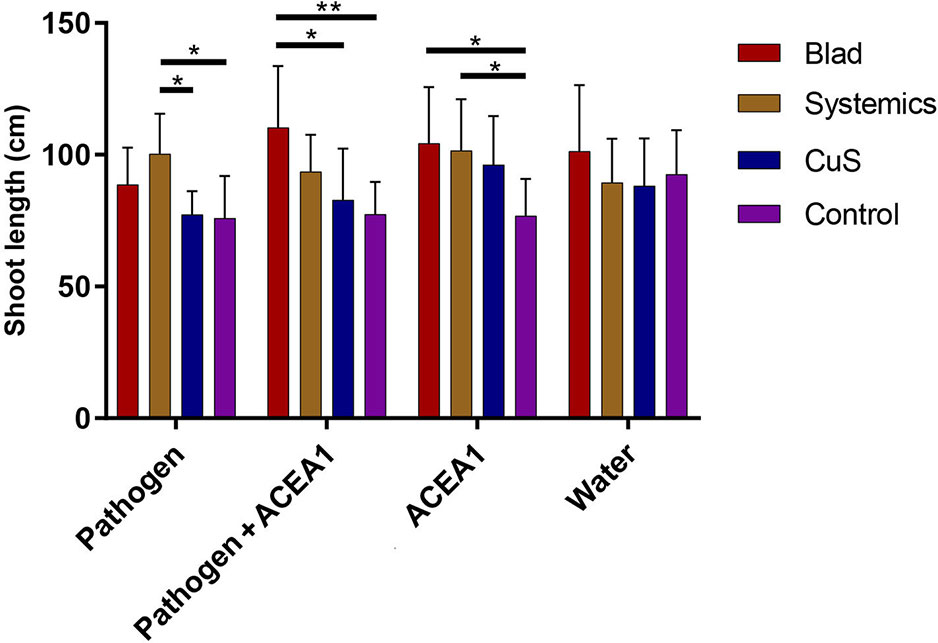
Figure 3 Barplot showing the measurements of grapevine shoot length. Error bars represent the standard deviation. Grapevines were inoculated with water, Phaeomoniella chlamydospora (Pathogen), a consortium of wood endophytes (ACEA1), and a combination of both (Pathogen + ACEA1). Grapevines were also treated with a foliar spray of potassium permanganate (Control), copper oxychloride and sulfur (CuS), and fosetyl-Al and penconazole (Systemics), Blad. n = 8 for each combination inoculum/treatment. Statistical differences, according to Tukey’s post hoc test, are shown by asterisk, where *P 0.05 and **P 0.01.
The examination of the wood revealed different degrees of symptomatology, ranging from absence of symptoms to extensive brown streaking departing from the inoculation point, as shown in Figure 2. Plants inoculated with P. chlamydospora presented extensive wood discoloration (Category 3; Figures 2C, D), under all spray treatments, while mild to absent symptoms, such as those shown in Figures 2A, B were recorded when P. chlamydospora was inoculated along with ACEA1. Plants inoculated with only water or ACEA1 did not show brown wood streaking symptoms, with the exception of the copper–sulfur treatment, suggesting that either copper oxychloride or sulfur or the combination of both may contribute to this plant response, independently from the inoculation of the pathogen.
Sequencing Dataset Description
The dataset containing the NGS data produced and analyzed in this study accounts for 78 samples. These samples are represented by 6,454,075 high-quality reads distributed in 506 unique features. The average number of reads per sample is 82,744, which allowed an adequate sequencing depth to unravel the complexity of the grapevine cuttings wood mycobiome (alpha-rarefaction curves obtained with Shannon and observed OTUs are shown in Figure S1, results reported in Table S2). The raw ASV table (read counts) is available in Table S3.
Using BLAST to refine the taxonomic classification of the taxa composing ACEA1, taxon “Pleosporales incertae sedis” was assigned to E. nigrum in 98.12% of the reads. Alternaria sp. was retrieved in 16 sequence variants, with the dominant feature corresponding to 97.88% of the total. After blasting this dominant sequence, the assignment was refined to Al. alternata, with 100% identity. The genus Cladosporium sp. appears 12 times in our database for a total of 61,288 high-quality reads. Blasting the four dominant features, which cover 94.7% of the total amount of reads, they were all assigned to Cladosporium sp.
A Qualitative Overview of the Wood Mycobiome
The richness of fungal taxa, identified examining all grapevine cuttings (n = 78), amounts to 66 species or genera of both ascomycetes and basidiomycetes. Among these 66 taxa, 30 of them are represented in an RA greater than 0.1%, while the remaining 36 taxa are considered rare taxa (RA < 0.1%; Tables 2 and S1). Among the 30 most abundant taxa, several genera of ascomycetes are known to be involved in GTDs (e.g., Neofusicoccum, Diaporthe, Cadophora, and Fusarium), while no known pathogenic basidiomycete was detected (Table 2). The most frequent (non-inoculated) taxa are Debaryomyces sp., Cryptococcus sp., Malassezia restricta, Malassezia globosa, Diaporthe sp., Acremonium alternatum, Candida sake and Candida friedrichii. Sixteen out of the 30 most abundant taxa (Table 2) were detected in less than 10% of the total number of vines, and among these 16, eight of them were present only in a single rooted cutting. This observation highlights the high variability often encountered when comparing individual plants.
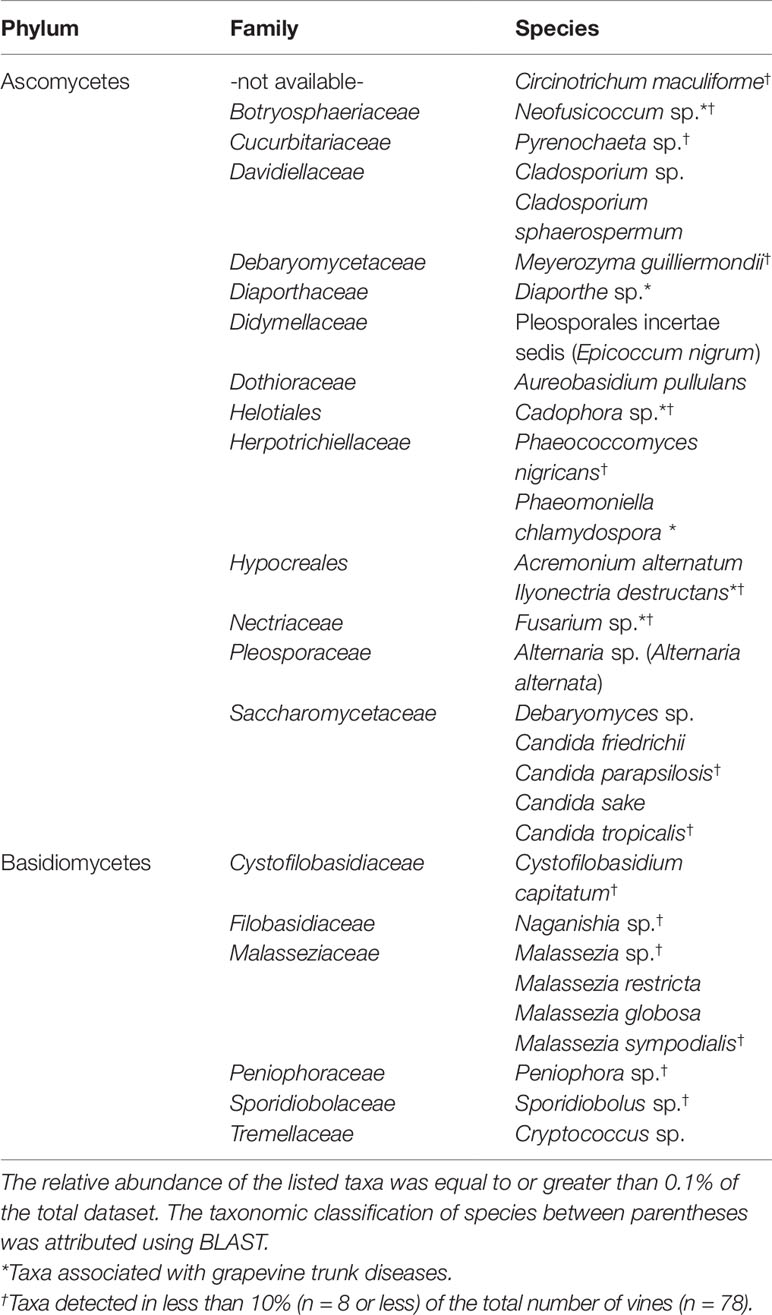
Table 2 Taxonomic classification of the most abundant taxa identified to genus or species level in the wood of grapevine rooted cuttings.
The metagenomic analysis detected P. chlamydospora in 28% of non-inoculated plants (RA > 0.1%), while the presence of wood endophytes that composed ACEA1, in non-inoculated grapevines, was revealed to be very low. Alternaria sp. and Cladosporium sp. were present in 8% of the plants, while E. nigrum (Pleosporales incertae sedis) and Au. pullulans in 3% of them (RA > 0.1%).
Alpha Diversity
The boxplots in Figure 4 show the Shannon diversity and Pielou’s evenness in grapevines treated with different fungicides and inocula. The Shannon diversity and evenness of the resident mycobiome of non-inoculated plants did not vary in relation to the fungicide treatment, according to Tukey’s HSD (P > 0.05). On the other hand, the inoculation of P. chlamydospora comported changes in both diversity and evenness (P < 0.05) of the mycobiome, when vines were sprayed with Blad (Figure 4). This active ingredient induced a reduction in Shannon diversity when compared to control-treated plants, as well as a strong trend when comparing Blad-treated plants to those treated with systemic fungicides (P = 0.058). No diversity differences were significant among other treatments (P > 0.05). The evenness of the fungal communities significantly varied when comparing Blad-treated vines with copper–sulfur-treated vines (Figure 4), and a strong trend was detected when comparing the former treatment with systemic fungicide-treated vines (P = 0.051). The application of the different fungicides did not affect the Shannon diversity and evenness of vines inoculated with either ACEA1 alone or ACEA1 and the pathogen (P > 0.05).
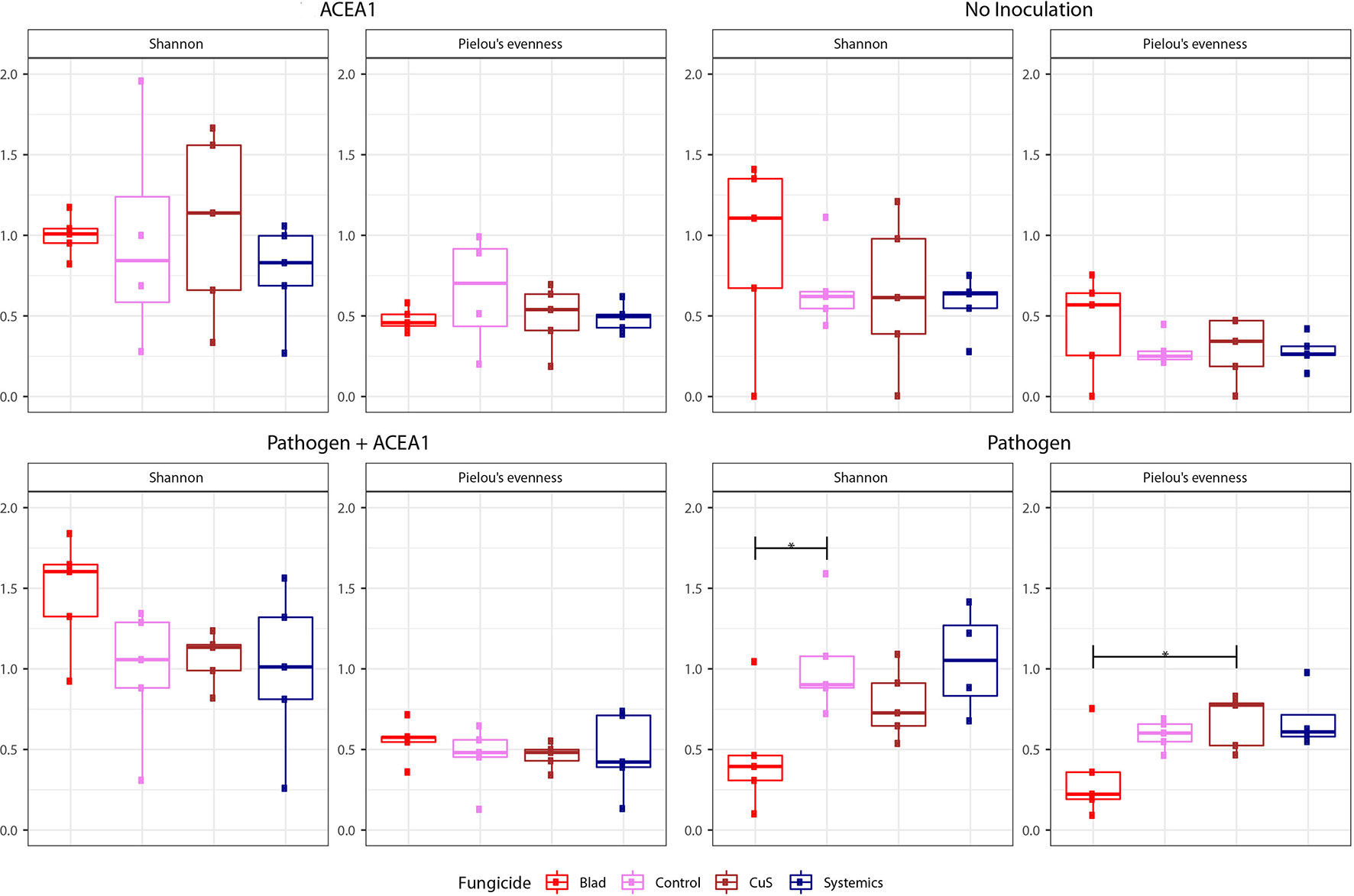
Figure 4 Box plots of diversity indexes (Shannon, Pielou’s evenness) of the fungal community present in grapevine cuttings added with water (No inoculation) or inoculated with Phaeomoniella chlamydospora (Pathogen), a consortium of fungal wood endophytes (ACEA1), or both P. chlamydospora and ACEA1. Inoculated plants were sprayed with either Blad or potassium permanganate (Control) or copper oxychloride and sulfur (CuS) or penconazole and fosetyl-aluminum (Systemics). n = 5 for each combination inoculum/treatment. The black, horizontal brackets at the top of the figures denote statistical comparisons of the two treatments at each end of the bracket, calculated using one-way ANOVA with Tukey’s honestly significant difference (HSD) post hoc. Statistical differences are shown by asterisk, where *P 0.05.
Beta Dispersion
The Jaccard index, when visualized in an NMDS plot, shows a considerable overlap for different fungicide treatments and inoculum types (Figure 5). The PERMANOVA indicates a significant difference between groups (P = 0.001), but looking at the ordination, the difference seems to lie in the clustering of the observations, rather than any distinct difference in sample composition. For example, the fungal communities in Blad-treated plants cluster more tightly than the control-treated ones, and a similar trend applies to non-inoculated vines when compared with those inoculated with both pathogen and ACEA1.
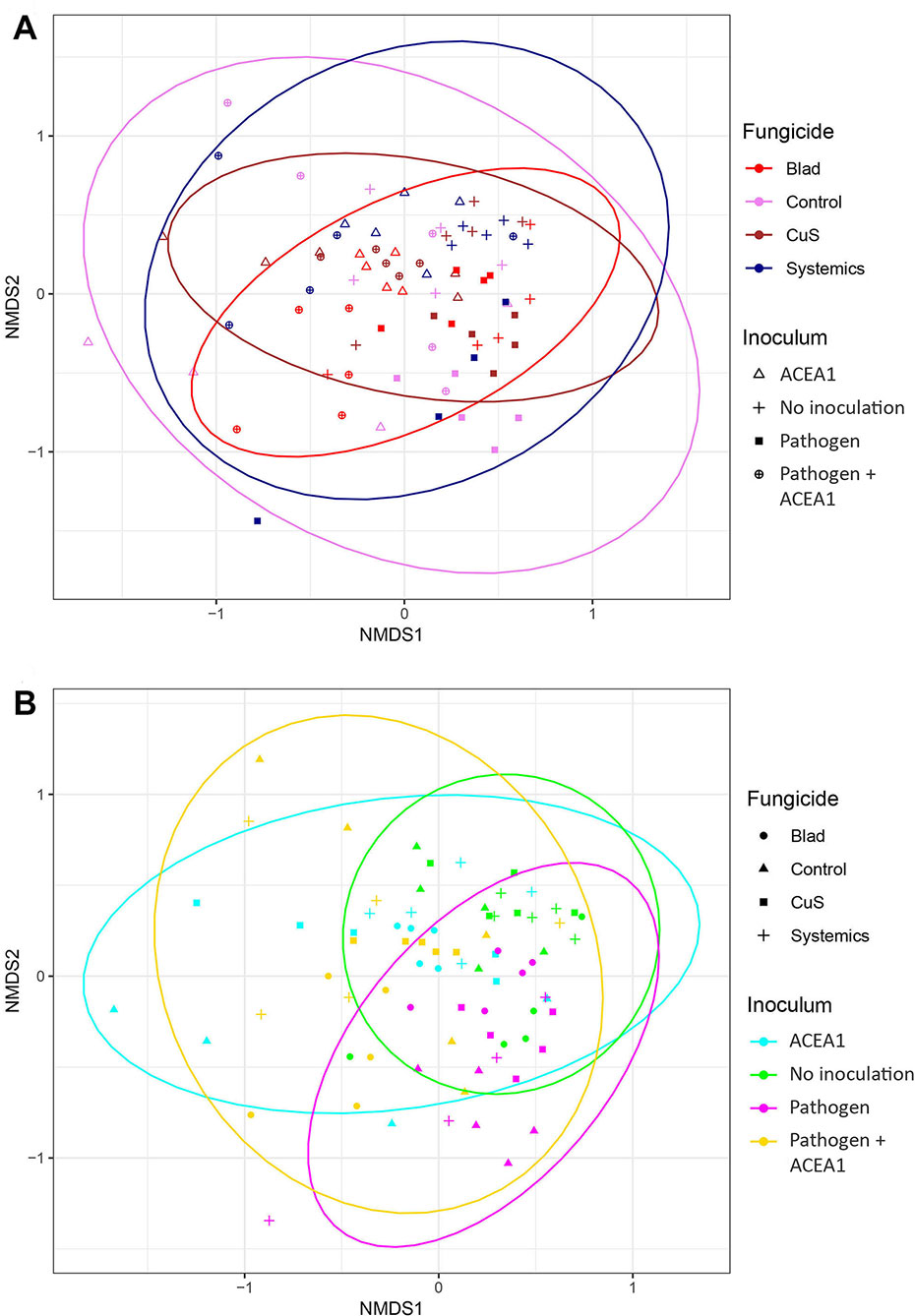
Figure 5 Non-metric multidimensional scaling (NMDS) plots based on Jaccard’s index. Fungal communities present in grapevine cuttings added with water (No inoculation), Phaeomoniella chlamydospora (Pathogen), a consortium of fungal wood endophytes (ACEA1), or both P. chlamydospora and ACEA1. Inoculated plants were sprayed with either Blad or potassium permanganate (Control) or copper oxychloride and sulfur (CuS) or penconazole and fosetyl-aluminum (Systemics). n = 5 for each combination inoculum/treatment. Ellipses illustrate the multivariate normal distribution of samples within the same fungicide (A) or inoculum (B) group.
Effect of Inoculum Type and Fungicide Treatments on Taxa Abundance
When examining the effect of fungicide treatments on the fungal communities of both non-inoculated and inoculated plants, no differences are detected according to the Wilcoxon test (P > 0.05). However, several taxa were found to have significantly different abundances between treatments according to the Wald test on the pairwise treatment comparisons, and the combined MetacodeR and DESeq analyses revealed overrepresentation and underrepresentation of numerous taxa under different treatments (Figures 6 and 7). Among the taxa identified as differently abundant, we find some of the fungi present in less than 10% of the total number of plants (Table 2). This low frequency suggests that the detected differential abundance may not lie in the effect of the treatments, but instead in the uneven distribution (presence/absence) of these taxa in individual vines. For this reason, these taxa are not going to be further examined.
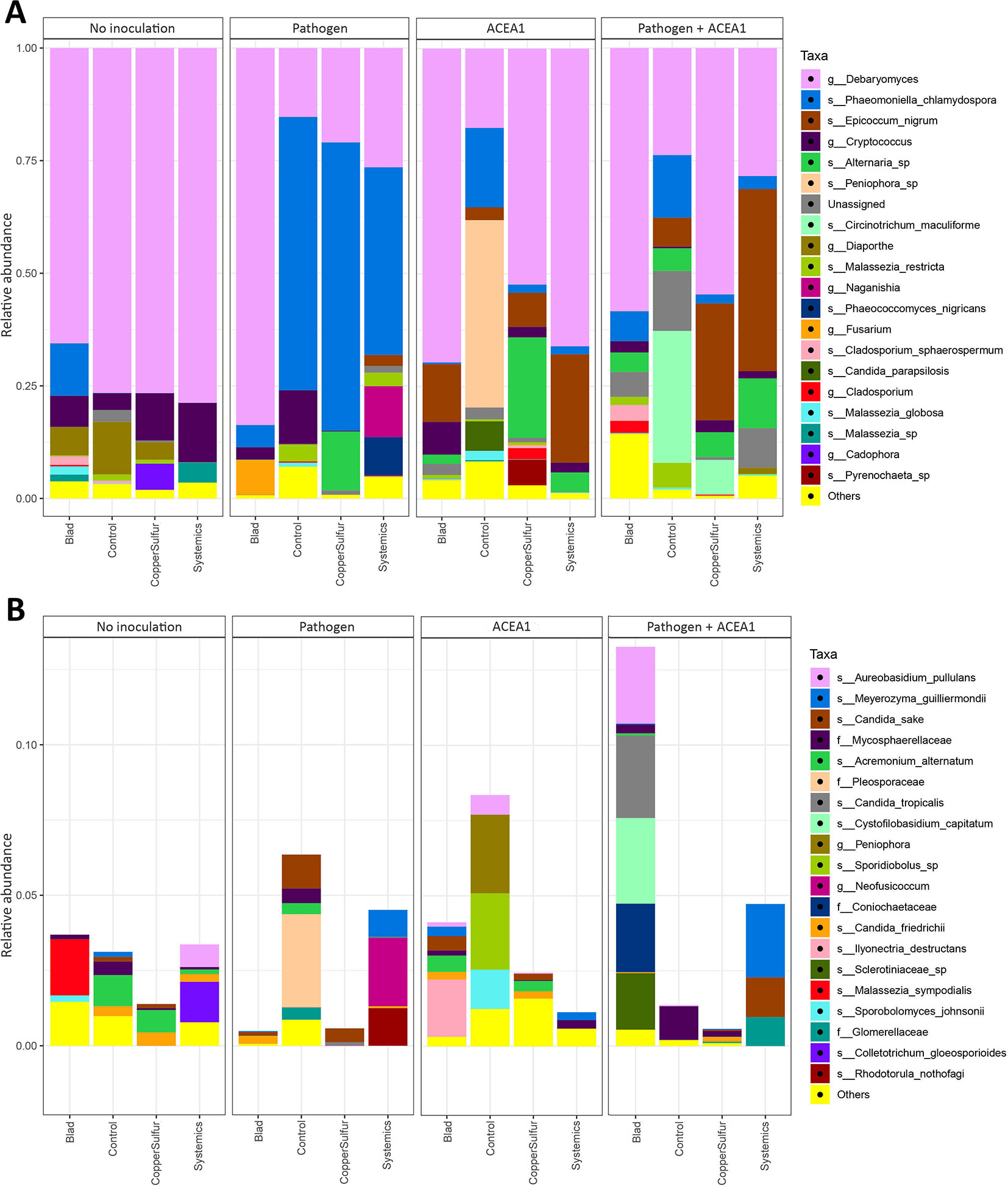
Figure 6 Barplots of the relative abundance of the 20 most abundant taxa (A) identified to species (s_), genus (g_), or family (f_) level, found in rooted grapevine cuttings non-inoculated (No inoculation) or inoculated with Phaeomoniella chlamydospora (Pathogen) or a consortium of fungal wood endophytes (ACEA1) or a combination of both (Pathogen + ACEA1). Grapevines were treated with either Blad or potassium permanganate (Control) or copper oxychloride and sulfur (CopperSulfur) or fosetyl-aluminum and penconazole (Systemics). n = 5 for each combination inoculum/treatment. “Unassigned” are taxa identified to a lower taxonomic level than family or non-identified, “Others” are taxa not included in the 20 most abundant. (B) The 20 most abundant taxa within the “Others” group of (A) are shown.
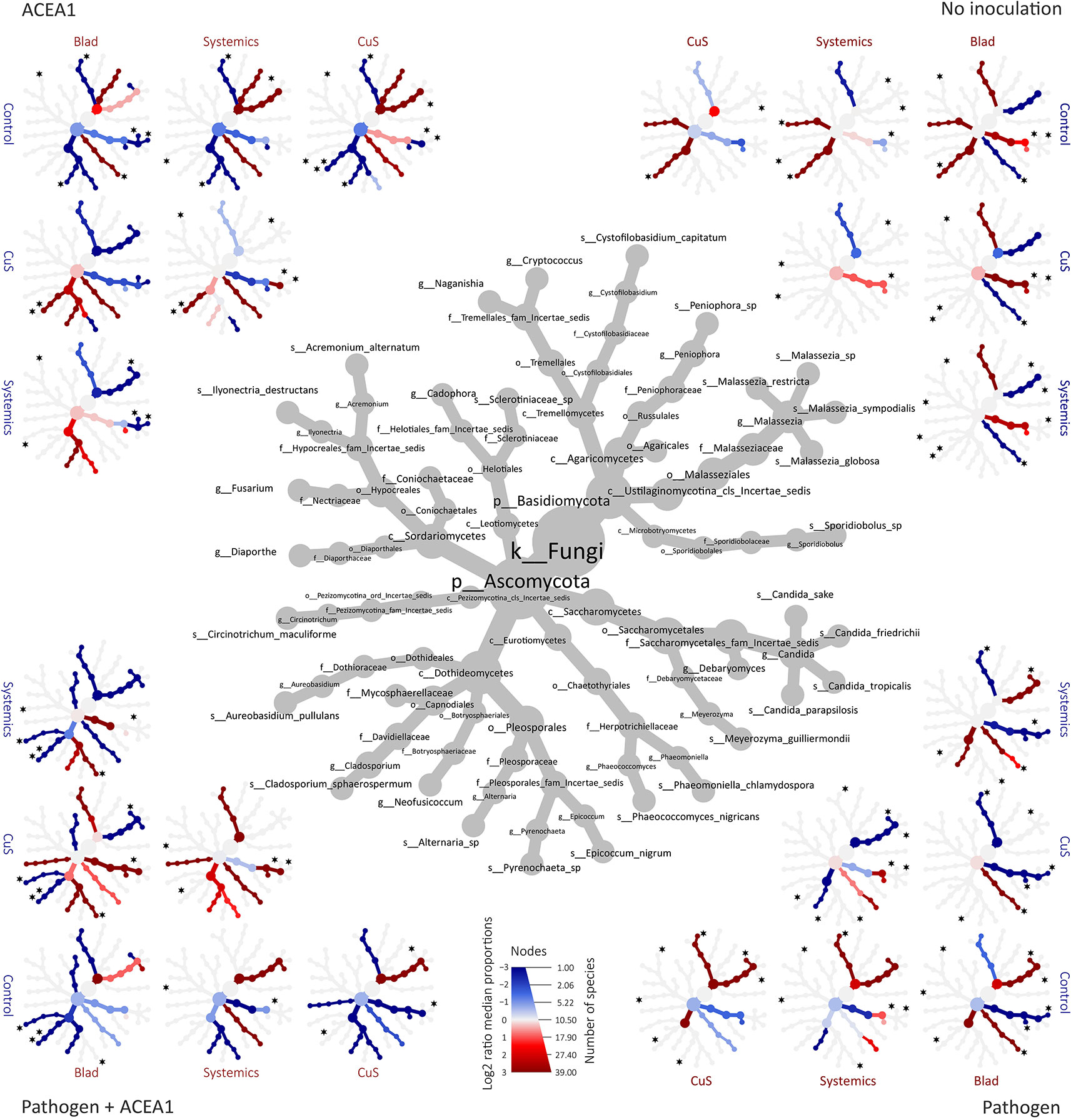
Figure 7 Differential heat tree matrixes depicting the change in taxon abundance between different fungicide treatments, represented in the dataset with a relative abundance (RA) > 0.1% (n = 5 for each combination inoculum/treatment). The size of the individual nodes in the gray cladogram depicts the number of taxa identified at that taxonomic level. The smaller cladograms show pairwise comparisons between each treatment, with the color illustrating the log2 fold change: a red node indicates a lower abundance of the taxon in the treatment group stated on the abscissa than in the treatment group stated on the ordinate. A blue node indicates the opposite. A black star next to a node represents the statistical differences according to DeSeq2 (P 0.05), for the most frequent taxa (Table 2).
In non-inoculated vines, Candida sake and C. friedrichii, present in control-treated plants, are not detected in Blad-treated vines; similarly, C. sake, Malassezia Restricta, and M. globosa are present in control-treated plants but absent in systemic fungicide-treated vines; Diaporthe sp. was detected under all treatments with the exception of systemic fungicides. None of the most frequent taxa differs significantly when comparing control- with copper–sulfur-treated grapevines (Figure 7).
In inoculated vines, we assumed that a successful colonization only occurred when inoculated fungi were detected in at least 50% of the biological replicates (per treatment/inoculation combination). The inoculation with P. chlamydospora, whether alone or in combination with ACEA1, resulted in a successful wood colonization, 1 cm below the inoculation point, in 87% of the cases. In the pathogen-only inoculation, fungicide treatments affected the RA of P. chlamydospora (Figure 7, Table 3) and that of other taxa. The foliar spray with Blad significantly decreased the RA of this fungus when compared with the control and other treatments (P < 0.05).
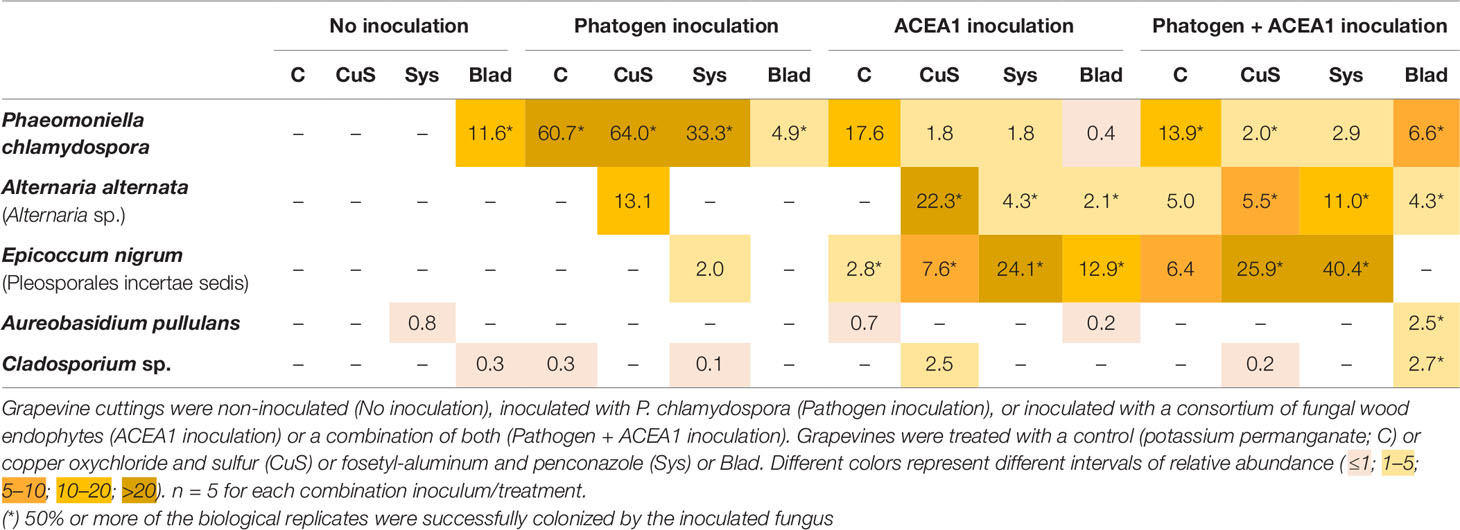
Table 3 Relative abundance of inoculated fungi in plants that were subject to different fungicide treatments.
In grapevines inoculated with ACEA1 and sprayed with potassium permanganate (control), only E. nigrum could successfully colonize the wood examined. The abundance of this ascomycete increased when plants were treated with Blad or systemic fungicides (Table 3). Al. alternata was absent (RA > 0.1%) in control-treated plants, while it was detected, in different abundances, under all other treatments (Table 3). The wood colonization by Au. pullulans was poor to absent, and it was never detected in 50% or more of the inoculated plants (Table 3). Cladosporium sp. was present exclusively in copper–sulfur-treated plants, although not detected in 50% or more of the inoculated plants (Table 3).
The simultaneous inoculation of P. chlamydospora and ACEA1 resulted in a lower abundance of the pathogen, when compared with the P. chlamydospora-only inoculum (Table 3), for all treatments except Blad. Al. alternata was detected under all fungicide treatments, except for the control-treated vines. E. nigrum exclusively colonized the wood when vines were treated with systemic fungicides or copper–sulfur. The Blad treatment facilitated the wood colonization by Au. pullulans and Cladosporium sp., which were not detected under any of the other treatments (Figure 7).
Discussion
Current knowledge on the effects of fungicides on the grapevine microbiome is scarce. Perazzolli et al. (2014) examined the effect of penconazole and a biological control agent on the grapevine phyllosphere, finding that bacterial and fungal communities are only minimally affected by both treatments. However, studies that investigated the effect of vineyard management systems (organic or integrated pest management) on endophyte communities, discovered significant differences, in both fungi (Pancher et al., 2012) and bacteria (Campisano et al., 2014). These results suggest that fungicide treatments may be one of the factors driving such microbiome changes. In this study, we investigated the changes that occur in the wood mycobiome in response to the application of fungicides, sprayed on leaves, in young plants. Due to the numerous factors that may influence our understating of this interaction, we decided to perform the experiment under semi-controlled conditions (i.e., greenhouse) and with no experimental repetitions. It follows a brief list of factors known to play a role in shaping the wood mycobiome of grapevines.
i. The age of the plants—Young grapevines (and canes) are known to host a lower richness in fungal taxa, when compared to adult vines (Dissanayake et al., 2018; Del Frari et al., 2019a).
ii. The management of the vineyard—Fungal endophytic communities found in organic vineyards can significantly differ from those found in vineyards that use an integrated pest management strategy (Pancher et al., 2012).
iii. The geographical location—In the same study by Pancher et al. (2012), significant differences in the wood mycobiome were detected when examining different vineyard locations.
iv. Grapevine cultivar—Jayawardena et al. (2018) proposed that grapevine cultivar, along with geographical location, is a determinant factor in the wood mycobiome composition. This observation is also supported by the results of a previous study by Travadon et al. (2016).
v. Seasonality—In a study by Bruez et al. (2014), grapevine wood sampled in different seasons harbored diverse community structures.
vi. The physiological status of grapevines may also influence their susceptibility to infection (e.g., biotic and abiotic stress; Gramaje et al., 2018).
Considering all these variables, it is reasonable to forecast that if any of the above factors changes, our understanding of the effect of fungicides on the wood mycobiome may vary. For this reason, the results obtained in this study should be considered as a case study, rather than a general understanding, and further investigation is required.
In order to assess the effect of fungicides on the early colonization success by wood endophytes, we selected four non-pathogenic fungi that are frequently found in grapevine wood and that are believed to hold potential in the biological control of wood pathogens (Pancher et al., 2012; Mondello et al., 2017; Jayawardena et al., 2018; Pinto et al., 2018). The choice of using a consortium instead of single fungus springs from the two following ideas:
i. To examine the effect of fungicide treatments on the early wood colonization success—The inoculation of multiple endophytes allows us to screen a higher number of fungi simultaneously.
ii. To examine the effect of simultaneously inoculating multiple endophytes and P. chlamydospora—The antagonistic interactions among endophytes and pathogen become more complex when compared to the confrontation between a single antagonist and a single pathogen. We hypothesized that the competition for space and nutrients, as well as the chemical war that occurs in the wood (e.g., competition-induced fungal metabolites; Hardoim et al., 2015), would strongly reduce the likelihood of P. chlamydospora producing a successful infection.
Visual Inspection, Shoot Length, and Brown Wood Streaking
The absence of foliar symptoms in the vines inoculated with ACEA1 confirms the current understanding that none of the selected organisms composing ACEA1, when present in the wood, are pathogenic in grapevines (Jayawardena et al., 2018). Concerning P. chlamydospora, Sparapano et al. (2001) observed that this fungus induced foliar symptom appearance, albeit only several months/years post-inoculation; therefore, the absence of foliar symptoms at the end of the experiment was expected.
The differences in length of the green shoot, only observed in response to fungicide treatments, suggest that both systemic fungicides and Blad positively affect the growth of vines (Figure 3); however, their mechanisms of action remain to be assessed.
Wood discoloration, in the form of brown wood streaking, is a result of the oxidation and polymerization of phenolic compounds, through the action of phenolases, a plant response to pathogen infections (Agrios, 2012) but also to other stresses such as mechanical injuries (Pouzoulet et al., 2013). (i) The absence of brown wood streaking in water- and ACEA1-inoculated vines and in plants treated with potassium permanganate, systemic fungicides, and Blad confirms that none of the components of ACEA1 are pathogenic. On the contrary, copper–sulfur-treated plants manifested extensive brown wood streaking. A recent report revealed that wood necrosis caused by grapevine wood pathogen Neofusicoccum parvum was promoted by the presence of high doses of copper in soil (Bruez et al., 2017). Copper is also associated with fungal pigmentation production (Griffith et al., 2007), a virulence factor (e.g., fungal melanins; Jacobson, 2000); laccase synthesis, which is involved in wood degradation (Tychanowicz et al., 2006); and induction of phytotoxic effects, with changes in plant morphology, biochemistry, and physiology (Bruez et al., 2017). Overall, these clues suggest that copper may play a relevant role in the development of brown wood streaking, by interacting with plant and endophytes. (ii) The extensive symptomatology recorded in plants inoculated with P. chlamydospora, under all fungicide treatments, indicates that the plant readily responded to infection, and it did so independently of the abundance of P. chlamydospora in the wood (e.g., Blad treatment; Table 3). Wood pathogens are known to induce the appearance of brown wood streaking, but they are not always found in symptomatic wood, especially the farther from the inoculation point (Mugnai et al., 1999). This suggests that, under some circumstances, the extent of wood streaking may not be correlated with the abundance of the pathogen. Moreover, P. chlamydospora has been previously reported in wood free from brown streaking symptoms (Rumbos and Rumbou, 2001; Abreo et al., 2011; Hofstetter et al., 2012), and its ecology requires further investigation. (iii) Moderate to absent wood symptoms were observed when co-inoculating P. chlamydospora and ACEA1, suggesting that the interactions among fungi resulted in a weaker or absent plant response to the infection. In fact, the reduction in wood streaking extent, due to the presence of fungal antagonists, has been previously reported in grapevines (Mondello et al., 2017; Del Frari et al., 2019b). It is worth remembering that the observations related to the brown wood streaking symptomatology made in this work are preliminary, and further evidence is necessary to assess the relation among wood inhabiting fungi–fungicides–wood symptomatology.
Effect of Fungicides on the Resident Mycobiome and on the Early Colonization by Endophytes
Previous studies revealed that fungicide application can affect the mycobiome of grapevines and other crops (Karlsson et al., 2014; Morrison-Whittle et al., 2017), although none of them investigated the fungal communities present in the wood. This study shows that some fungal taxa composing the resident wood mycobiome of grapevine cuttings cv. Cabernet Sauvignon are affected by fungicide application (Figure 7). This observation suggests that active ingredients interacted with fungi either directly (e.g., copper oxychloride is known to be absorbed in the xylem of grapevines, when sprayed on the bark of vines; Di Marco et al., 2011) or indirectly, for example, stimulating plant defenses (García et al., 2003). Further studies are necessary to confirm this preliminary understanding, keeping into account (i) the variability in taxa composition that may characterize individual plants and (ii) that the DNA of dead fungal cells, supposedly affected by fungicides, may persist in the wood, during short-term experiments, hiding the magnitude of the treatment effect. It is also necessary to investigate whether the changes observed in taxa abundance are temporary or long-lasting, therefore determining the overall resilience of the endophytic communities that compose the resident mycobiome.
Multitrophic interactions involving plants and fungal and bacterial endophytes are still poorly understood (Hardoim et al., 2015). Current literature shows that host and microbe genotypes, as well as abiotic factors, can influence successful colonization by endophytes (Hardoim et al., 2015). In this study, we show that also fungicide application can affect the early colonization by fungal endophytes, in particular those composing ACEA1 (Table 3). Therefore, fungicides seem to have the potential of shaping the wood mycobiome by favoring/inhibiting the early wood colonization of specific fungi. For this reason, we propose to keep in consideration fungicide treatments when describing endophytic communities in grapevines.
Effect of Fungicides on the Early Colonization by P. chlamydospora
Numerous trials have been conducted, over the last 20 years, in order to find reliable means to control infections by wood pathogens, including P. chlamydospora. Chemical control, in the form of foliar spray or endotherapy, led primarily to negative or moderate results, while more promising ones have been achieved through pruning wound protection (Mondello et al., 2017). In this study, the copper–sulfur and systemic fungicide treatments did not affect the colonization success of the pathogen, within the timings of the experiment (Figure 6). A preliminary study in support of the neutral effect of copper oxychloride on the colonization success of P. chlamydospora was presented by Di Marco et al. (2011), although no literature is available on the influence of sulfur or other synthetic fungicides. Blad was the only active ingredient capable of significantly inhibiting the wood colonization success of P. chlamydospora (Del Frari et al., 2018; Monteiro et al., 2015). This is the first report of a foliar spray capable of reducing the wood colonization success of this pathogen.
Use of ACEA1 in the Biological Control of P. chlamydospora
As postulated, the wood colonization success of P. chlamydospora was greatly reduced when co-inoculated with a consortium of fungal wood endophytes (ACEA1) that are considered potential antagonists (Figure 6). Some of the possible explanations are found in the interactions that occur during conidia germination and mycelial growth (e.g., release of metabolites) and by increasing the competition for space and nutrients (Hardoim et al., 2015). Fungi composing ACEA1 are frequently isolated from grapevine wood; however, their exact niche (e.g., xylem vessels, tracheids) remains to be assessed. A histological examination of the wood may unveil the exact interaction that takes place among the components of ACEA1 and P. chlamydospora. Only minor changes in the abundance of the pathogen are observed with different fungicide treatments, making ACEA1 a potential tool to be exploited in biological control. Interestingly, the Blad treatment facilitated the wood colonization by both Cladosporium sp. and Au. pullulans, while inhibiting that of E. nigrum, in the presence of P. chlamydospora, when compared with the results obtained from ACEA1-only inoculation (Table 3). This observation suggests the occurrence of complex interactions in which not only the fungicide but also the pathogen play a primary role (e.g., competition-induced metabolites), resulting in selective wood colonization. Further investigation is necessary to confirm these results also from a quantitative point of view. It is also important to understand if the effect observed by the addition of ACEA1 is long-lasting, and it may be successfully applied to plants with an established infection, as a curative strategy.
Conclusion
This study shows that some common fungicides that have been applied in viticulture for decades (copper–sulfur and systemics) affect the resident mycobiome and/or the wood colonization success of some endophytes, while leaving nearly unaffected the wood pathogen P. chlamydospora. This has unpredictable consequences, in both the medium and long terms, on the wood mycobiome ecology and its influence on grapevine well-being. It is reasonable to wonder whether the wood mycobiome composition of grapevines before the fungicide era was considerably different from that of today. As the diversity of a biological system is positively correlated with its stability (McCann, 2000), a loss in endophytic species that antagonize GTD pathogens, such as P. chlamydospora, may be a possible explanation for the recent success of these pathogenic fungi. Another explanation may lie in the fungicide-driven increased likelihood of infection by some fungi, which creates an imbalance in the stability of the plant–mycobiome biological system.
This work addressed only four endophytes, although the wood mycobiome of adult plants is known to be potentially colonized by hundreds of species, making this just the beginning of the research in this sense. In conclusion, enhancing the complexity of the wood mycobiome, for example, using multiple endophytic antagonists, may considerably increase our chances to control GTD-associated pathogens. While research on endophytic fungal consortia advances, Blad seems a promising active ingredient to confront early infections by P. chlamydospora, in young grapevines. This active ingredient is non-toxic for the environment and humans, has growth-promoting properties, and strongly antagonizes the wood colonization success of P. chlamydospora, making it a promising tool in viticulture.
Data Availability Statement
The raw data of this study are available in the European Nucleotide Archive (ENA accession number PRJEB32853).
Author Contributions
GF conceived the study, performed greenhouse experiment, performed wet-lab work, interpreted and discussed the results, and wrote the manuscript. AG performed wet-lab work, performed bio-informatics analyses, wrote the manuscript. MA performed statistical analyses and data visualization. HO, LH, and RF supervised the study and reviewed the manuscript.
Funding
This study was funded by the Horizon 2020 Programme of the European Commission within the Marie Sklodowska-Curie Innovative Training Network “MicroWine” (grant number 643063 to G. Del Frari, A. Gobbi, L. Hestbjerg Hansen and R. Boavida Ferreira) and by Portuguese national fund FCT Unit funding UID/AGR/04129/2019 (LEAF).
Conflict of Interest
The authors declare that the research was conducted in the absence of any commercial or financial relationships that could be construed as a potential conflict of interest.
Acknowledgments
The authors would like to acknowledge Elisa Filippi, João Costa and Amélia Marques for technical support, LEAF for providing facilities and chemicals, Ana Cabral for advises.
Supplementary Material
The Supplementary Material for this article can be found online at: https://www.frontiersin.org/articles/10.3389/fpls.2019.01405/full#supplementary-material
Table S1 | Fungal taxa identified to genus or species level in the wood of grapevine rooted cuttings, at relative abundances (RA) inferior to 0.1 % or 0.01% of the total.
Table S2 | Rarefaction curves results based on richness, calculated on the number of amplicon sequence variants (ASVs), and on Shannon index.
Figure S1 | Rarefaction curves of alpha-diversity indexes; each line correspond to one sample in a range from 0 to 18000 reads. Each box plot represent the distribution of the selected alpha diversity metric for each sample at each 2000 reads of sampling depth. The lower and upper whiskers of the box plot correspond to the 9th and 91st percentiles of the distribution, while the lower and upper extents of the box correspond to the 25th and 75th percentiles of the distribution (respectively). The horizontal bar through the middle of the box is the median of the distribution (i.e., the 50th percentile). Outlier points of these distributions are not shown. The lines connect all the medians from the different. A) Rarefaction curves based on richness, calculated on the number of amplicon sequence variants (ASVs). B) Rarefaction curves based on Shannon index.
References
Abreo, E., Martínez, S., Bettucci, L., Lupo, S. (2011). Phaeomoniella chlamydospora and Phaeoacremonium spp. in grapevines from Uruguay. Phytopathol. Mediterr. 50 (SUPPL.), 77–85. doi: 10.14601/Phytopathol_Mediterr-8682
Agrios, G. (2012). Plant Pathology. Academic Press. Burlington, U.S.A: Elsevier Academic Press. doi: 10.1016/C2009-0-02037-6
Bokulich, N. A., Subramanian, S., Faith, J. J., Gevers, D., Gordon, J. I., Knight, R., et al. (2013). Quality-filtering vastly improves diversity estimates from Illumina amplicon sequencing. Nat. Methods 10 (1), 57–59. doi: 10.1038/nmeth.2276
Bokulich, N. A., Kaehler, B. D., Ram Rideout, J., Dillon, M., Bolyen, E., Knight, R., et al. (2018). Optimizing taxonomic classification of marker-gene amplicon sequences with QIIME 2’s Q2-Feature-Classifier plugin. Microbiome 6 (1), 1–17. doi: 10.1186/s40168-018-0470-z
Bruez, E., Lecomte, P., Grosman, J., Doublet, B., Bertsch, C., Fontaine, F., et al. (2013). Overview of grapevine trunk diseases in France in the 2000s. Phytopathol. Mediterr. 52 (2), 262–275. doi: 10.14601/Phytopathol_Mediterr-11578
Bruez, E., Vallance, J., Gerbore, J., Lecomte, P., Da Costa, J. P., Guerin-Dubrana, L., et al. (2014). Analyses of the temporal dynamics of fungal communities colonizing the healthy wood tissues of esca leaf-symptomatic and asymptomatic vines. PLoS ONE 9 (5). doi: 10.1371/journal.pone.0095928
Bruez, E., Anatole-Monier, L., Vallance, J., Bussière, S., Denaix, L., Rey, P., et al. (2017). High concentration of copper in vineyard soil favours the development of wood necrosis caused by the Botryosphaeria dieback pathogen Neofusicoccum parvum. Phytopathol. Mediterr. doi: 10.14601/Phytopathol_Mediterr-21865
Callahan, B. J., McMurdie, P. J., Holmes, S. P. (2017). Exact sequence variants should replace operational taxonomic units in marker-gene data analysis. ISME J. 11 (12), 2639–2643. Nature Publishing Group. doi: 10.1038/ismej.2017.119
Campisano, A., Antonelli, L., Pancher, M., Yousaf, S., Pindo, M., Pertot, I. (2014). Bacterial endophytic communities in the grapevine depend on pest management. PLoS ONE 9 (11), e112763. doi: 10.1371/journal.pone.0112763
Caporaso, G., Kuczynski, J., Stombaugh, J., Bittinger, K., Bushman, F. D., Costello, E. K., et al. (2010). QIIME allows analysis of high-throughput community sequencing data. Nat. Methods 7 (5), 335–336. doi: 10.1038/nmeth0510-335
Del Frari, G., Costa, J., Oliveira, H., Boavida Ferreira, R. (2018). Endotherapy of infected grapevine cuttings for the control of Phaeomoniella chlamydospora and Phaeoacremonium minimum. Phytopathol. Mediterr. 57 (3), 439–448. doi: 10.14601/Phytopathol
Del Frari, G., Gobbi, A., Aggerbeck, M. R., Oliveira, H., Hansen, L. H., Boavida Ferreira, R. (2019a). Characterization of the wood mycobiome of Vitis vinifera in a vineyard affected by esca. spatial distribution of fungal communities and their putative relation with leaf symptoms. Front. Plant Sci. 10, 910. doi: 10.3389/fpls.2019.00910
Del Frari, G., Cabral, A., Nascimento, T., Boavida Ferreira, R., Oliveira, H. (2019b). Epicoccum layuense a potential biological control agent of esca-associated fungi in grapevine. PLoS ONE 14 (3), e0213273. doi: 10.1371/journal.pone.0213273
Di Marco, S., Osti, F., Mugnai, L. (2011). First studies on the potential of a copper formulation for the control of leaf stripe disease within esca complex in grapevine. Phytopathol. Mediterr. 50 (SUPPL.), 300–309. doi: 10.14601/Phytopathol_Mediterr-10233
Dias, M. C. (2012). Phytotoxicity: an overview of the physiological responses of plants exposed to fungicides. J. Bot. 1–4. doi: 10.1155/2012/135479
Dissanayake, A. J., Purahong, W., Wubet, T., Hyde, K. D., Zhang, W., Xu, H., et al. (2018). Direct comparison of culture-dependent and culture-independent molecular approaches reveal the diversity of fungal endophytic communities in stems of grapevine (Vitis vinifera). Fungal Divers. 90 (1), 85–107. doi: 10.1155/2012/135479
Dowle, M., Srinivasan, A. (2017). Data Table: Extension of Data Frame. Available at: https://cran.r-project.org/web/packages/data.table/index. html (accessed January 8, 2019).
Dray, S., Blanchet, G., Borcard, D., Clappe, S., Guenard, G., Jombart, T., et al. (2018). A Despatial: Multivariate Multiscale Spatial Analysis. R Package Version 0.1-1. Available at: https://cran.r-project.org/web/packages/adespatial/index.html (accessed January 8, 2019).
Feld, L., Nielsen, K., Hansen, H., Aamand, J., Albers, N. (2016). Establishment of bacterial herbicide degraders in a rapid sand filter for bioremediation of phenoxypropionate-polluted groundwater. Appl. Environ. Microbiol. 82 (3), 878–887. doi: 10.1128/AEM.02600-15.Editor
Fontaine, F., Gramaje, D., Armengol, J., Smart, R., Nagy, Z. A., Borgo, M., et al. (2016). Grapevine trunk diseases. A review. no. December: 25 pp. http://www.oiv.int/public/medias/4650/trunk-diseases-oiv-2016.pdf.
Foster, Z. S. L., Sharpton, T. J., Grünwald, N. J. (2017). Metacoder: an R package for visualization and manipulation of community taxonomic diversity data. PLOS Comput. Biol. 13 (2), e1005404. doi: 10.1371/journal.pcbi.1005404
García, P. C., Rivero, R. M., Ruiz, J. M., Romero, L. (2003). The role of fungicides in the physiology of higher plants: implications for defense responses. Bot. Rev. 69 (2), 162–172. doi: 10.1663/0006-8101(2003)069[0162:TROFIT]2.0.CO;2
Gobbi, A., Santini, R., Filippi, E., Ellegaard-Jensen, L., Jacobsen, C. S., Hansen, L. H. (2018). Quantitative and qualitative evaluation of the impact of the G2 enhancer, bead sizes and lysing tubes on the bacterial community composition during DNA extraction from recalcitrant soil core samples based on community sequencing and qPCR. PLoS One 14, e0200979. doi: 10.1371/journal.pone.0200979
Gramaje, D., Urbez-Torres, J. R., Sosnowski, M. R. (2018). Managing grapevine trunk diseases with respect to etiology and epidemiology: current strategies and future prospects. Plant Dis. 102 (1), 12–39. doi: 10.1094/PDIS-04-17-0512-FE
Griffith, G. W., Easton, G. L., Detheridge, A., Roderick, K., Edwards, A., Worgan, H. J., et al. (2007). Copper deficiency in potato dextrose agar causes reduced pigmentation in cultures of various fungi. FEMS Microbiol. Lett. 276 (2), 165–171. doi: 10.1111/j.1574-6968.2007.00923.x
Hardoim, P. R., van Overbeek, L.S., Berg, G., Pirttilä, A.M., Compant, S., Campisano, A., et al. (2015). The hidden world within plants: ecological and evolutionary considerations for defining functioning of microbial endophytes. Microbiol. Mol. Biol. Rev. doi: 10.1128/MMBR.00050-14
Hofstetter, V., Buyck, B., Croll, D., Viret, O., Couloux, A., Gindro, K. (2012). What if esca disease of grapevine were not a fungal disease? Fungal Divers. 54, 51–67. doi: 10.1007/s13225-012-0171-z
Jackson, R. S. (2014). “Vineyard practice,” in Wine Science (London, U.K.: Academic Press), 143–306. doi: 10.1016/B978-0-12-381468-5.00004-X
Jacobson, E. S. (2000). Pathogenic roles for fungal melanins. Clin. Microbiol. Rev. 13 (4), 708–717. doi: 10.1128/CMR.13.4.708-717.2000
Jayawardena, R. S., Purahong, W., Zhang, W., Wubet, T., Li, X. H., Liu, M., et al. (2018). Biodiversity of fungi on Vitis vinifera L. revealed by traditional and high-resolution culture-independent approaches. Fungal Divers. 90, 1–84. doi: 10.1007/s13225-018-0398-4
Juang, K.-W., Lee, Y.-I., Lai, H.-Y., Wang, C.-H., Chen, B.-C. (2012). Copper accumulation, translocation, and toxic effects in grapevine cuttings. Environ. Sci. Pollut. R. 19 (4), 1315–1322. doi: 10.1007/s11356-011-0657-3
Karlsson, I., Friberg, H., Steinberg, C., Persson, P. (2014). Fungicide effects on fungal community composition in the wheat phyllosphere. PLoS ONE 9 (11). doi: 10.1371/journal.pone.0111786
Komárek., M., Čadková, E., Chrastný, V., Bordas, F., Bollinger, J. C. (2010). Contamination of vineyard soils with fungicides: a review of environmental and toxicological aspects. Environ. Int. 36 (1), 138–151. doi: 10.1016/j.envint.2009.10.005
La Torre, A., Spera, G., Lolleti, D. (2004). Activity of natural products against courgette powdery mildew. Commun. Agric. Appl. Biol. Sci. 69 (4), 671—678.
La Torre, A., Iovino, V., Caradonia, F. (2018). Copper in plant protection: current situation and prospects. Phytopathol. Mediterr. 57 (2), 201–236. doi: 10.14601/Phytopathol_Mediterr-23407
Love, I. M., Huber, W., Anders, S. (2014). Moderated estimation of fold change and dispersion for RNA-seq data with DESeq2. Genome Biol. 15 (500), 1–21. doi: 10.1186/s13059-014-0550-8
McCann, K. S. (2000). The diversity–stability debate. Nature 405 (6783), 228–233. doi: 10.1038/35012234
McMurdie, P. J., Holmes, S. (2013). Phyloseq: an R package for reproducible interactive analysis and graphics of microbiome census data. PLoS ONE 8 (4), e61217. doi: 10.1371/journal.pone.0061217
Mondello, V., Songy, A., Battiston, E., Pinto, C., Coppin, C., Trotel-Aziz, P., et al. (2017). Grapevine trunk diseases: a review of fifteen years of trials for their control with chemicals and biocontrol agents. Plant Dis. 102 (7), 1189–1217. doi: 10.1094/PDIS-08-17-1181-FE
Monteiro, S., Carreira, A., Freitas, R., Pinheiro, A. M., Boavida Ferreira, R. (2015). A nontoxic polypeptide oligomer with a fungicide potency under agricultural conditions which is equal or greater than that of their chemical counterparts. PLoS ONE 10 (4), 1–23. doi: 10.1371/journal.pone.0122095
Morrison-Whittle, P., Lee, S. A., Goddard, M. R. (2017). Fungal communities are differentially affected by conventional and biodynamic agricultural management approaches in vineyard ecosystems. Agric. Ecosyst. Environ. 246, 306–313. doi: 10.1016/j.agee.2017.05.022
Morton, V., Staub, T. (2008). A short history of fungicides. APSnet Feature Articles. doi: 10.1094/APSnetFeature-2008-0308
Mugnai, L., Graniti, A., Surico, G. (1999). Esca (black measles) and brown wood-streaking: two old and elusive diseases of grapevines. Plant Dis. 83 (5), 404–418. doi: 10.1094/PDIS.1999.83.5.404
Nilsson, R. H., Taylor, A. F. S., Bates, S. T., Thomas, D., Bengtsson-Palme, J., Callaghan, T. M., et al. (2013). Towards a unified paradigm for sequence-based identification of fungi. Mol. Ecol. 22 (21), 5271–5277. doi: 10.1111/mec.12481
Oksanen, J., Kindt, R., Legendre, P., O’Hara, B., Stevens, M. H. H., Oksanen, M. J., et al. (2007). The Vegan package. Community Ecol. Package 10, 631–637.
Op De Beeck, M., Lievens, B., Busschaert, P., Declerck, S., Vangronsveld, J., Colpaert, J. V. (2014). Comparison and validation of some ITS primer pairs useful for fungal metabarcoding studies. PLoS ONE 9 (6). doi: 10.1371/journal.pone.0097629
Pancher, M., Ceol, M., Corneo, P. E., Oliveira Longa, C. M., Yousaf, S., Pertot, I., et al. (2012). Fungal endophytic communities in grapevines (Vitis vinifera L.) respond to crop management. Appl. Environ. Microbiol. 78 (12), 4308–4317. doi: 10.1128/AEM.07655-11
Perazzolli, M., Antonielli, L., Storari, M., Puopolo, G., Pancher, M., Giovannini, O., et al. (2014). Resilience of the natural phyllosphere microbiota of the grapevine the chemical and biological pesticides. Appl. Environ. Microbiol. 80 (12), 3585–3596. doi: 10.1128/AEM.00415-14
Pinto, C., Custódio, V., Nunes, M., Songy, A., Rabenoelina, F., Courteaux, B., et al. (2018). Understand the potential role of Aureobasidium pullulans, a resident microorganism from grapevine, to prevent the infection caused by Diplodia seriata. Front. Microbiol. 9, 1–15. doi: 10.3389/fmicb.2018.03047
Pouzoulet, J., Jacques, A., Besson, X., Dayde, J., Mailhac, N. (2013). Histopathological study of response of Vitis vinifera cv. Cabernet Sauvignon to bark and wood injury with and without inoculation by Phaeomoniella chlamydospora. Phytopathol. Mediterr. 52 (2), 313–323. doi: 10.14601/Phytopathol_Mediterr-12581
Pretorius, I. S., van der Westhuizen, T. J., Augustyn, O. P. H. (1999). Yeast biodiversity in vineyards and wineries and its importance to the South African wine industry. S. Afr. J. Enol. Vitic. 20 (2), 61–75. doi: 10.21548/20-2-2234
Rumbos, I., Rumbou, A. (2001). Fungi associated with esca and young grapevine decline in Greece. Phytopathol. Mediterr. 40 (3), 330–335. doi: 10.1002/pssa.2210290216
Setati, M. E., Jacobson, D., Andong, U. C., Bauer, F. (2012). The vineyard yeast microbiome, a mixed model microbial map. PLoS ONE 7 (12). doi: 10.1371/journal.pone.0052609
Sparapano, L., Bruno, G., Graniti, A. (2001). Three-Year observation of grapevines cross-inoculated with esca-associated fungi. Phytopathol. Mediterr. 40 (3), 376–386. doi: 10.14601/Phytopathol_Mediterr-1643
Surico, G. (2009). Towards a redefinition of the diseases within the esca complex of grapevine. Phytopathol. Mediterr. 48 (1), 5–10. doi: 10.14601/Phytopathol_Mediterr-2870
Surico, G., Bandinelli, R., Braccini, P., Di Marco, S., Marchi, G., Mugnai, L., et al. (2004). On the factors that may have influenced the esca epidemic in Tuscany in the eighties. Phytopathol. Mediterr. 43 (1), 136–143. doi: 10.14601/Phytopathol_Mediterr-1734
Thomson, L. J., Glenn, D. C., Hoffmann, A. A. (2000). Effects of sulfur on Trichogramma egg parasitoids in vineyards: measuring toxic effects and establishing release windows. Aust. J. Exp. Agric. 40 (8), 1165–1171. doi: 10.1071/EA00074
Travadon, R., Lecomte, P., Diarra, B., Lawrence, D. P., Renault, D., Ojeda, H., et al. (2016). Grapevine pruning systems and cultivars influence the diversity of wood-colonizing fungi. Fungal Ecol.24, 82–93. doi: 10.1016/j.funeco.2016.09.003
Tychanowicz, G. K., De Souza, D. F., Souza, C. G. M., Kadowaki, M. K., Peralta, R. M. (2006). Copper improves the production of laccase by the white-rot fungus Pleurotus pulmonarius in solid state fermentation. Braz. Arch. Biol. Technol. 49 (5), 699–704. doi: 10.1590/S1516-89132006000600002
Verweij, P. E., Snelders, E., Kema, G. H. J., Mellado, E., Melchers, W. J. G. (2009). Azole resistance in Aspergillus fumigatus: A side-effect of environmental fungicide use? Lancet Infect. Dis. 9 (12), 789–795. doi: 10.1016/S1473-3099(09)70265-8
Wickham, H. (2016). Ggplot2. Use R! Cham: Springer International Publishing. doi: 10.1007/978-3-319-24277-4
Keywords: fungicides, Phaeomoniella chlamydospora, grapevine trunk diseases, mycobiome, microbial ecology
Citation: Del Frari G, Gobbi A, Aggerbeck MR, Oliveira H, Hansen LH and Ferreira RB (2019) Fungicides and the Grapevine Wood Mycobiome: A Case Study on Tracheomycotic Ascomycete Phaeomoniella chlamydospora Reveals Potential for Two Novel Control Strategies. Front. Plant Sci. 10:1405. doi: 10.3389/fpls.2019.01405
Received: 20 April 2019; Accepted: 10 October 2019;
Published: 31 October 2019.
Edited by:
Michele Perazzolli, University of Trento, ItalyReviewed by:
Josep Armengol, Polytechnic University of Valencia, SpainStéphane Compant, Austrian Institute of Technology (AIT), Austria
Raffaele Testolin, University of Udine, Italy
Copyright © 2019 Del Frari, Gobbi, Aggerbeck, Oliveira, Hansen and Ferreira. This is an open-access article distributed under the terms of the Creative Commons Attribution License (CC BY). The use, distribution or reproduction in other forums is permitted, provided the original author(s) and the copyright owner(s) are credited and that the original publication in this journal is cited, in accordance with accepted academic practice. No use, distribution or reproduction is permitted which does not comply with these terms.
*Correspondence: Giovanni Del Frari, gdelfrari@isa.ulisboa.pt
†Present address: Environmental Microbial Genomics Group (EMG), Section for Microbial Ecology and Biotechnology (MEB), Department of Plant and Environmental Science (PLEN), University of Copenhagen, Copenhagen, Denmark