- 1Institute of Biosciences and BioResources (IBBR), National Research Council of Italy (CNR), Corso Calatafimi, Palermo, Italy
- 2Department of Agronomy, Food, Natural Resources, Animals and the Environment, University of Padua, Legnaro, Italy
- 3Department of Biology, University of Padova, Padova, Italy
- 4Institute for the Animal Production System in Mediterranean Environment (ISPAAM), National Research Council (CNR), Sassari, Italy
Endophytism within Vitis represents a topic of critical relevance due to the multiple standpoints from which it can be approached and considered. From the biological and botanical perspectives, the interaction between microorganisms and perennial woody plants falls within the category of stable relationships from which the plants can benefit in multiple ways. The life cycle of the host ensures persistence in all seasons, repeated chances of contact, and consequent microbiota accumulation over time, leading to potentially high diversity compared with that of herbaceous short-lived plants. Furthermore, grapevines are agriculturally exploited, highly selected germplasms where a profound man-driven footprint has indirectly and unconsciously shaped the inner microbiota through centuries of cultivation and breeding. Moreover, since endophyte metabolism can contribute to that of the plant host and its fruits’ biochemical composition, the nature of grapevine endophytic taxa identities, ecological attitudes, potential toxicity, and clinical relevance are aspects worthy of a thorough investigation. Can endophytic taxa efficiently defend grapevines by acting against pests or confer enough fitness to the plants to endure attacks? What are the underlying mechanisms that translate into this or other advantages in the hosting plant? Can endophytes partially redirect plant metabolism, and to what extent do they act by releasing active products? Is the inner microbial colonization necessary priming for a cascade of actions? Are there defined environmental conditions that can trigger the unleashing of key microbial phenotypes? What is the environmental role in providing the ground biodiversity by which the plant can recruit microsymbionts? How much and by what practices and strategies can these symbioses be managed, applied, and directed to achieve the goal of a better sustainable viticulture? By thoroughly reviewing the available literature in the field and critically examining the data and perspectives, the above issues are discussed.
Introduction
Plant microbial endophytism represents a wealth of interactive relationships that are widespread in nature and often rely on mutual benefits due to plant growth-promoting (PGP) phenotypes. In some cases, the beneficial effect is directly related to microbial metabolism, such as nitrogen fixation, phytohormone production, phosphate solubilization, and pathogen suppression; or conversely, such benefits can be mediated by the stimulation of specific activities of the host plant, leading to increased enzymatic catalysis, and enhanced water and nutrient uptake or defense responses. Moreover, endophytic microorganisms occupy an ecological niche that overlaps that of many phytopathogens, and their exploitation as biocontrol agents (BCAs) represents a possible strategy to reduce the use of pesticides in vineyards (Compant et al., 2013). Much of the applicative potential of agriculture and biotechnology is derived from plant–microbe interactions, but some requisites are the isolation of ex planta and multiplication of useful strains. According to some authors, this condition appears proportionally frequent compared with the situation observed in bulk soil or water environments (Finkel et al., 2017). However, other authors report the opposite and note the considerable proportion of non-plate-culturable endophytes (Thomas et al., 2017). West et al. (2010) recorded a certain degree of difference between culturable endophytic communities and those obtained by culture-independent approaches, suggesting a non-negligible level of non-culturability among endophytes. This limitation has been confirmed by further studies, which testified a major issue of recalcitrance to cultivation in grapevine endophytic bacteria (Thomas et al., 2017). When amenable to growth, selected members of the inner microbiota can be exploited as inoculants to foster plant growth in agriculture, horticulture, and silviculture or used for soil or water decontamination, such as in phytoremediation applications (Santoyo et al., 2016).
The precise identification of endophytic microorganisms is necessary to determine their distribution in host plants and their beneficial effects. The traditional methods used for the identification of BCAs are based on isolation and culturing on artificial media, followed by biochemical, immunological, and molecular characterization; however, the techniques of fluorescence microscopy can be used to detect endophytes inside plant tissues (Figures 1A, B). Over the last few years, advanced molecular techniques have allowed researchers to investigate the biodiversity of grapevine endophytes, particularly endophytic species that are slow growing or cannot be grown in axenic culture. Culture-independent methods, including automated ribosomal intergenic spacer analysis (ARISA) (Pancher et al., 2012), denaturing gradient gel electrophoresis (DGGE) (West et al., 2010), and single-strand conformation polymorphism (SSCP) analysis (Rezgui et al., 2016), can provide a general overview of the grapevine endophytic microbiome composition, although metagenomic approaches based on massive DNA sequencing of prokaryotic 16SrDNA or eukaryotic ITS1-5.8S-ITS2 rDNA genes are the methods of choice to assess the composition of grapevine endophytic communities (Pinto et al., 2014; Morgan et al., 2017; Dissanayake et al., 2018).
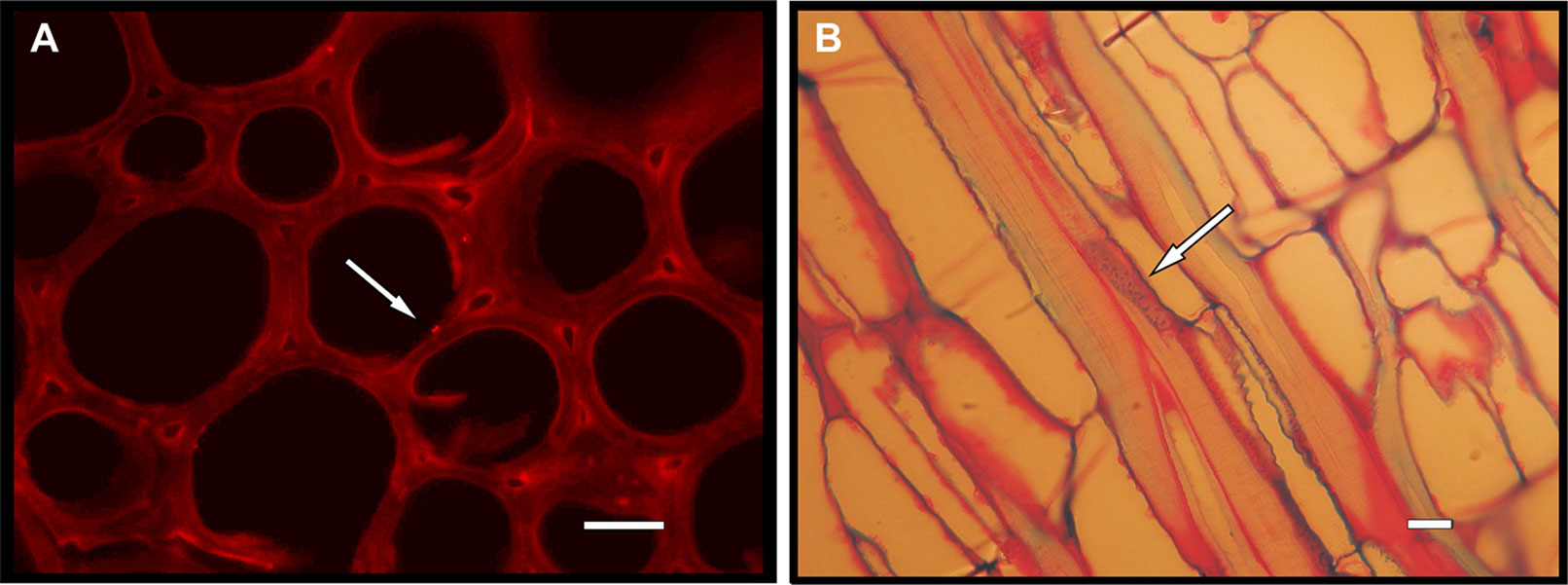
Figure 1 Endophytes inside plant tissues detected trough fluorescence microscopy techniques. (A) Epifluorescence image on transverse sections of a Centaurea horrida stem stained by propidium iodide. Arrow: endophytic bacterium cell adhering to a vessel within the plant’s vascular system. Bar = 15 μm. (B) Optical microscopy of basic fuchsin/astra blue double-stained longitudinal thin sections from a Hedysarum glomeratum root in proximity to the crown. Endophytic bacteria (indicated by the arrow) are distinguishable within a xylem vessel. Bar = 10 μm.
Biodiversity
Plant Influence on Endophytic Composition
Regarding the endophytic presence, a vast diversity of taxa has been documented in both cultivated (Vitis vinifera subsp. vinifera) and wild (V. vinifera subsp. sylvestris) grapevines. Different factors could shape the grapevine microbiome, as follows: seasonality, plant genotype, age, pedo-climatic features, surrounding wild plants, presence of pathogens, etc.
A survey of grapevine cultivar Glera plants from six different vineyards yielded 381 culturable bacterial isolates from the inner portions of surface-sterilized stems and leaves (Baldan et al., 2014). A large share of these bacteria (30%) belonged to the genus Bacillus, while the remainder included Paenibacillus, Microbacterium, Staphylococcus, Micrococcus, Stenotrophomonas, Variovorax, Curtobacterium, and Agrococcus. A certain degree of local specificity was reported as populations from different vineyards were rather different; in addition, seasonality was recorded, and differences were observed between the May and October samplings (Baldan et al., 2014).
In cultivated grapevine, the composition of bacterial communities is significantly associated with the rootstock genotype and shows different interactions; the nature of the rootstock was addressed as a possible variable explaining endophyte diversity. Marasco et al. (2018) investigated how different rootstocks could affect the recruitment of bacteria from the surrounding soil and found that bacterial community diversity and networking in the roots were profoundly influenced by the rootstock type. Interestingly, it has been observed that despite selecting different bacterial components, grapevine rootstock genotypes possess PGP traits similar to those exhibited by different bacteria that provide fundamental ecological services (Marasco et al., 2018).
The age of grapevine plants has been explored as a possible factor affecting endophytic community composition. Three-year-old and 15-year-old plants of the cv. Corvina were compared and found to be differentially invaded. Actinobacteria and Bacilli prevailed in the 3-year-old plants, while the older plants featured more Proteobacteria. Younger Vitis also have a higher diversity of taxa and a particular abundance of the Rhizobium genus, while the old plants contained higher shares of Pantoea. The phenotypes represented more in the 15-year-old plants were phosphate solubilization and 1-aminocyclopropane-1-carboxylate (ACC) deaminase activity (Andreolli et al., 2016).
Campisano et al. (2015) explored the difference between wild and cultivated grapevines in terms of endophytic communities; overall, 155 bacterial strains were isolated from 88 plants of the two types grown in the same climate. The species diversity resulted considerably higher in wild grapes than domesticated varieties with 25 genera versus six, respectively. In addition, regarding the multivariate ordination by phenotypes, the clustering was distinct to the isolates from the wild or cropped specimens, even when the bacteria belonged to the same genus.
An important question in this type of study is as follows: to what extent is a plant-associated microbiome specific to its host compared with the surrounding vegetation? The degree of overlap in bacterial communities isolated from grapevines and those found in weeds sharing the same vineyard has been analyzed (Samad et al., 2017). Assuming that the same soil offers the different coexisting plants a pool of microbes from which to choose, the authors identified the rhizosphere and inner root bacterial checklists of V. vinifera and those of four herbaceous plants, including three annual therophytes (Stellaria media, Veronica arvensis, and Lamium amplexicaule) and one perennial (Lepidium draba). The analysis was carried out using culture-independent 16S amplification and metabarcoding. The shared portion of the microbiome featured 145 operational taxonomic units (OTUs) in the rhizosphere, and only nine belonged to the truly endophytic contingent isolated from inside the roots. The bacteria associated with the weeds had a higher diversity in the rhizosphere than those related to the grapevine, but the endophytic subgroup displayed more diversity in the perennial plants (Vitis or Lepidium). The PGP traits were more represented in the isolates from the weeds. Overall, it appears that even plants sharing the same soil are characterized by significantly different microbiomes both at the rhizosphere level and inside the roots, but in the latter compartment, their differences are more pronounced.
The interaction between endophytes and plants occurs in different areas, including the root and foliar surfaces. Regarding the provenance of the endophytic microbiota and its way of entry into plants, it is generally assumed that the main route is from soil to roots with endophytes ascending to the epigeal parts or alternatively through gaps along the plant overall surface, including wounds or stomata (Compant et al., 2010). Once endophytes enter into the plant, they move systemically (Nigris et al., 2018), spreading in different tissues as shown in Figure 2, where green fluorescent protein (GFP)-tagged Bacillus licheniformis can be visualized within the stem of a grapevine-inoculated plant. Indeed, endophytes can colonize other tissues and organs, including reproductive organs, thus allowing their transfer through the vascular system (Lidor et al., 2018) or apoplastic spaces (Compant et al., 2011). An analysis of different aerial portions of grapevines was carried out and revealed differential colonization by endophytes; both Pseudomonas and Bacillus were found within the flower and ovules, while only the latter was present in the berries and seeds (Compant et al., 2011). Using DGGE and fatty acid methyl ester profiling, some authors have also documented the possibility that grapevine epiphytes originally dwelling on the external surface of leaves and stems could become endophytes when windows of opportunity for such internalization arise (West et al., 2010). However, there are documented exceptions that add complexity to the picture. In grapevines, the sap-feeding leafhopper pest Scaphoideus titanus, which is recognized as the vector for phytoplasma diseases, has also been found to be capable of transferring various species of endophytic bacteria from one grapevine plant (source plant) to another (sink plant) (Lòpez-Fernàndez et al., 2017); the acquired taxa included members of the phyla Proteobacteria, Actinobacteria, and Firmicutes. While the insect spends time exclusively on leaves or stems, in sink plants, the root endophytic communities acquired a composition very close to that found within the insect vector. These data suggest that the insect-mediated exchange of endophytes among plants could be an important and still overlooked component of the mechanisms underlying these plant–microbe interactions. However, an even more extreme example of the cross-transfer of microbes to plants concerns the taxon Propionibacterium acnes, which has been shown to have been acquired by grapevine in an inter-kingdom transmission from humans (Campisano et al., 2014a). Such occurrence is reminiscent of several cases in which bacterial host switches were traced from domestic animals to humans, but here, the case is particularly remarkable as it could involve the opposite direction and a member of the plant kingdom as a recipient sink.
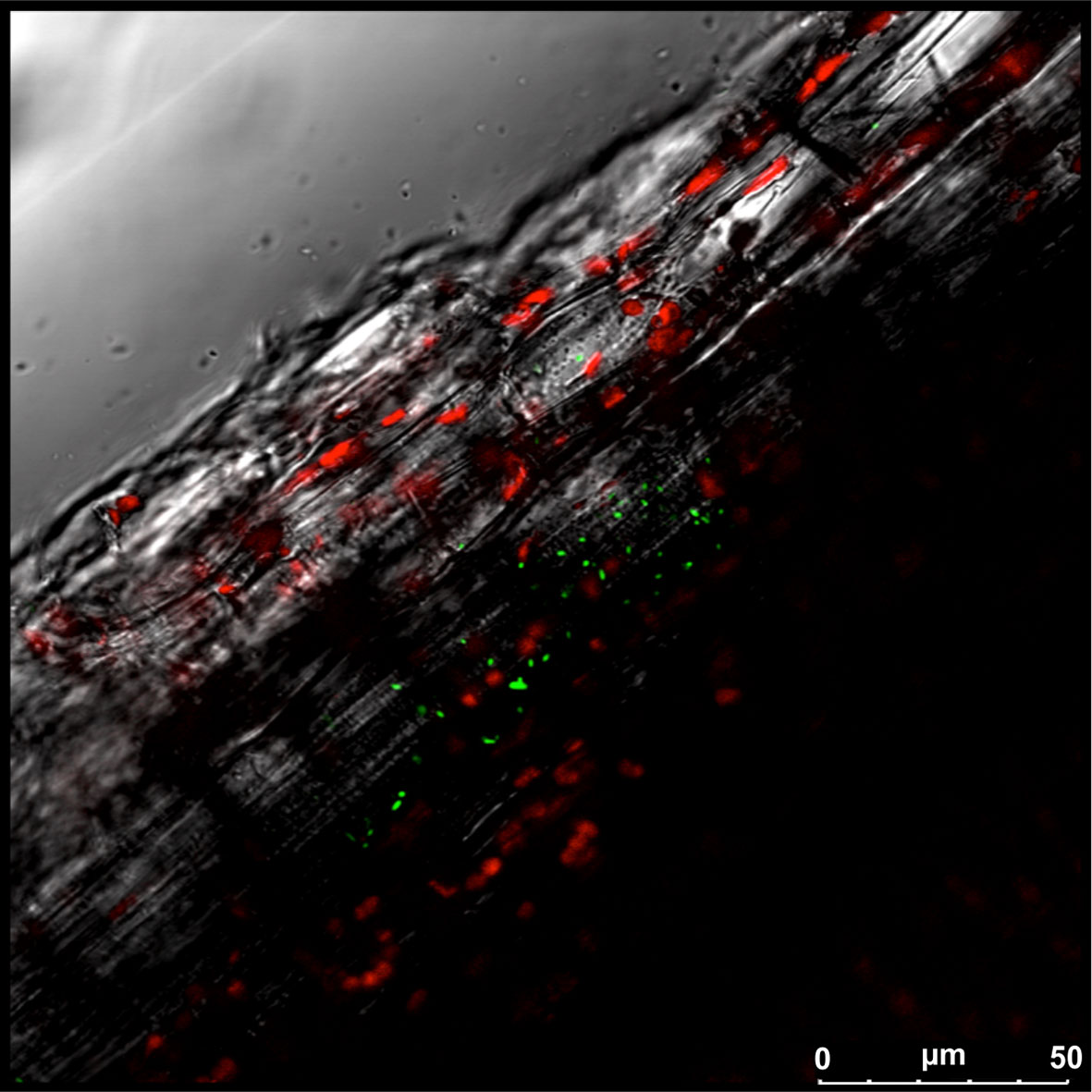
Figure 2 Bacillus licheniformis colonizing a grapevine plant. Laser scanning confocal microscopy of stem sections of Glera cuttings inoculated with Bacillus licheniformis GL174::gfp2x. Overlay of green fluorescent protein (GFP) signal (green), chlorophyll (red), and bright field.
A further factor conducive to differences in the endophytic communities of grapevine is the presence of pathogens, such as Agrobacterium vitis, which is the agent of grapevine crown gall disease (Faist et al., 2016). The authors have found the differences in the microbial community occurring at the graft union as discriminative. In particular, the microbial community from plants without crown gall disease showed a transient composition, changing from across seasons, while that from plants with crown galls had a higher species diversity and regularly featured the dominance of the same three taxa, including Pseudomonas sp. A. vitis and a member of the Enterobacteriaceae family (Faist et al., 2016). The diversity and abundance of endophytes have been investigated and compared between healthy grapevine and plants affected by flavescence dorée (FD) and bois noir phytoplasmas while considering the seasonal variation (Bulgari et al., 2011). The authors sampled during three different time points and reported that independent of the sampling period, phytoplasmas can shape endophytic bacterial communities by enriching such communities with strains able to elicit plant defense responses. Genera associated with the infection process include some that have been reported to be associated with biocontrol action, such as Burkholderia, Methylobacterium, Sphingomonas, and Pantoea. Overall, the communities found in the healthy grapevine featured a higher diversity than those in the diseased plants. Endophytes and plant pathogens can indeed coexist within the same host and result in evolutionary paths that diverge at a certain stage. A study concerning grapevine invaded or not invaded by the black fungi Aureobasidium pullulans and Epicoccum nigrum reported that their presence did not result in detectable changes in the associated bacterial populations (Grube et al., 2011).
A different approach to studying endophytism relies on genomic data to search for genes that could distinguish endophytic strains from pathogenic strains belonging to closely related lineages. This type of survey was carried out in the genomes of seven endophytes isolated from grapevine, which were compared with members of the same genera but involved in pathogenic interactions (Lòpez-Fernàndez et al., 2015). Their comparison showed that endophytes and pathogens have a high number of common virulence-related genes in their core pangenome with a high degree of conservation in each genus regardless of the endophytic or pathogenic lifestyles. Therefore, the structural organization of endophyte genomes is reflective of the conservation of properties spanning over different attitudes, including pathogenicity. Through a bioinformatics approach, it is possible to identify particular genes in endophyte genomes strictly associated with the endophytic lifestyle, which can contribute to the peculiar recognition of beneficial microorganisms (Ali et al., 2014).
Environmental Influence on Endophytic Diversity
The microbial component in viticulture and oenology has been increasingly recognized to have a major imprint on the regional terroir. A complex of environmental variables is linked to the geographical origin and its ensuing endophytic community composition. Studies have shown that the microbiome involved during the early fermentation stages, which is partially determined by endophytic plant-borne yeasts and bacteria, complies with a well-delineated biogeography reflecting the signatures of different winegrowing regions with an additional but minor influence from the grape variety and vintage year (Bokulich et al., 2014; Gilbert et al., 2014). Notwithstanding, the soil is a main reservoir for endophyte recruitment, and a strong selection process appears to be operative in favor of strains endowed with relevant PGP phenotypes, as the final community is not merely a mirror of the soil array of microbiota but rather a distinct sub-community (Novello et al., 2017). As the terroir concept implies, soil is ultimately regarded as a major environmental factor conditioning the microbial populations that are eventually associated with their host plants. This issue has been thoroughly addressed, and the factors particularly critical for the determination of grapevine inner microbiota include the soil pH, soil carbon, and C:N ratio; most taxa associated with grapevine organs originated in soil and had a marked degree of local vineyard scale specificity (Zarraonaindia et al., 2015). Among the environmental conditions, soil pH is generally recognized as a major driver shaping bacterial communities. Its effect in modelling those interacting with grapevine was addressed in plants grown in acidic or alkaline soils (Karagöz et al., 2012); in total, 27 genera were identified; overall, gram-negative taxa were dominant in both soil types, and Pseudomonas and Bacillus were recurring genera. The observed differences included that Acidovorax delafieldii, Pseudomonas mendocina, Aeromonas ichthiosmia, Hafnia alvei, Raoultella terrigena, Paenibacillus alginolyticus, Arthrobacter aurescens, Kocuria kristinae, Curtobacterium flaccumfaciens, Flavobacterium johnsoniae, and Sphingobacterium spiritivorum were isolated only from alkaline sites. In contrast, Ralstonia eutropha, Citrobacter amalonaticus, Enterobacter hormaechei, Arthrobacter globiformis, Rhizobium rubi, Paracoccus denitrificans, Pseudomonas syringae, Citrobacter freundii, Serratia odorifera, Arthrobacter oxydans, Kocuria rosea, and Brevibacterium epidermidis were isolated only from the acidic soils.
Other studies addressed pest management (organic vs. integrated) as a variable and determined its effect on the bacterial endophyte community of Merlot and Chardonnay grapevine cultivars (Campisano et al., 2014b). The results indicated that while Ralstonia, Burkholderia, and Pseudomonas were present in all samples, the relative abundance of the taxa defined the consistent differences, and the genera Mesorhizobium, Caulobacter, and Staphylococcus were found more frequently in the organically managed vineyards, while Ralstonia, Burkholderia, and Stenotrophomonas were abundant in the vineyards where integrated pest management was operative. In this respect, the differences related to the cultivar were minor compared with those ascribed to the management practices. Pancher et al. (2012) showed that the possibility of differentiating communities in relation to management or the cultivar also depends on the technique used. The fungal taxa detected in a parallel analysis of endophytic fungi in the same two grapevine cultivars included Absidia glauca, Pennicillium restrictum, Alternaria sp., Botrytis cinerea, Fusarium sp., Trichoderma reesei, Fusarium oxysporum, Neurospora crassa, Pennicillium chrysogenum, Podospora anserina, and Davidiella tassiana; the culture-dependent methods yielded overlapping results, while the molecular DNA-based approaches had sufficient power to resolve the differences between the organic and integrated pest managements.
Beneficial Effects of Grapevine Endophytes on Abiotic Stress Tolerance
Endophytes employ different mechanisms to exert beneficial effects on plant growth or biotic/abiotic stress resistance, and these mechanisms act either directly by releasing plant hormones, secondary metabolites (Table 1), biocides, or antibiotics (an example is shown in Figure 3) or indirectly by modifying the plant physiology and nutrient balance, leading to a reduced susceptibility to diseases or abiotic stresses (Lugtenberg and Kamilova, 2009; Compant et al., 2013).
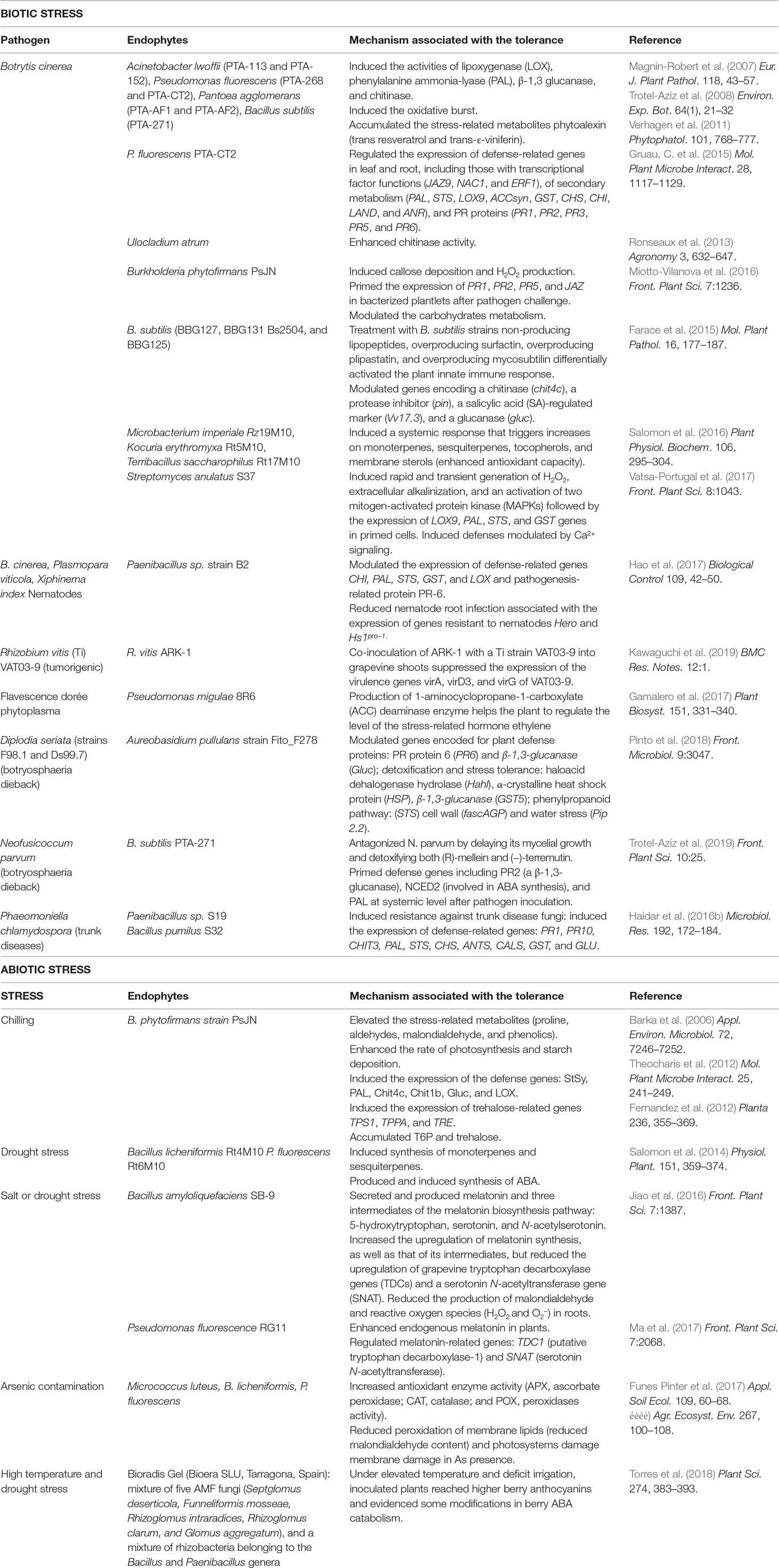
Table 1 Potential roles and mechanisms of main endophytes involved in biotic and abiotic stress tolerance.
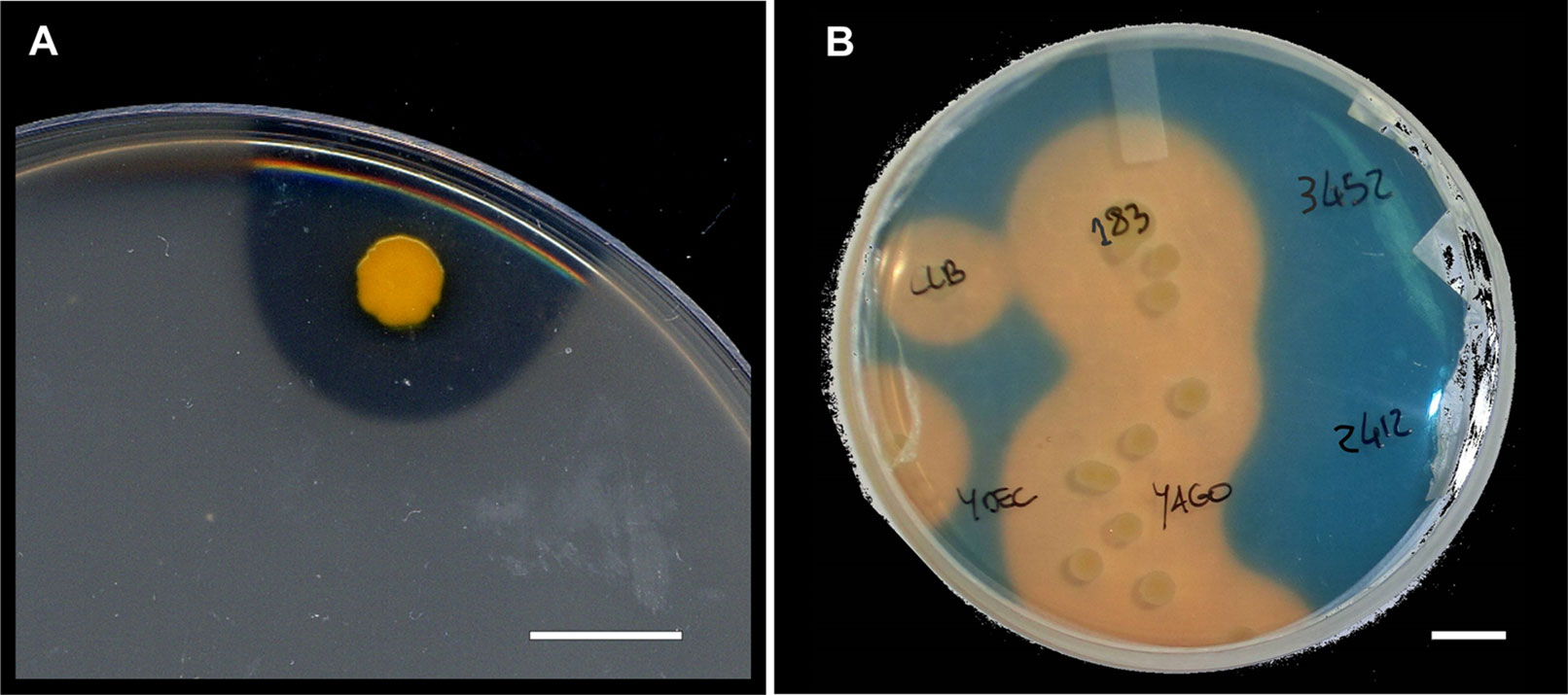
Figure 3 Isolated grapevine endophytes. (A) Grapevine endophyte showing antibiosis production towards a layer of Bacillus subtilis on a petri dish. Bar = 1 cm. (B) Grapevine endophytes, including some showing positivity (discoloring to yellow background in the Chrome Azurol-S assay) to an iron-solubilization test for siderophores. Bar = 1 cm.
Abiotic stresses, such as heat, salinity, and drought, trigger trehalose synthesis in plants, which can provide the plants stress endurance. Trehalose forms a gel during cellular dehydration, preventing excessive water loss due to its high stability against acids and heat. Inoculation with the Paraburkholderia phytofirmans strain PsJN has been observed to be conducive as a stress-preventive trait as plants bearing this endophyte began to accumulate trehalose and its intermediate trehalose-6-phosphate (T6P) at 26°C (Fernandez et al., 2012). In particular, it has been reported that P. phytofirmans can affect trehalose metabolism in grapevine plants through the stimulation of T6P synthesis or the inhibition of T6P degradation (Esmaeel et al., 2018). A deep analysis of 48 genomes of Burkholderia species revealed that 161 clusters involved in secondary metabolite synthesis were likely involved in signaling between grapevine and endophytic bacteria (Esmaeel et al., 2016). It has been demonstrated that P. phytofirmans sets grapevine metabolism to a state of alert, enabling rapid and intense resistance responses to subsequent stresses. In this case, the benefit provided by endophytes does not occur directly through the induction of stress-related genes but rather occurs by preparing the plant to activate a faster and stronger defense response upon stress (Esmaeel et al., 2016). In addition, P. phytofirmans can induce different PR genes [encoding chitinase, phenylalanine ammonia-lyase (PAL), lipoxygenase (LOX), and glucanase], protecting the plant from low temperatures (Theocharis et al., 2012). In addition, the endophyte is able to induce genes coding enzymes involved in reactive oxygen species (ROS) scavenging; a decrease in the ROS concentration is important because it converts detoxification compounds into signaling molecules.
A different major factor of stress in most plants is drought. Soil microorganisms have different strategies to cope with limited water potential, including the accumulation of compatible solutes (glycine-betaine, proline, trehalose, and exopolysaccharide production) and exopolysaccharide production (Ngumbi and Kloepper, 2016). Their phenotypes have been shown to be transferrable to the plants that they can invade as endophytes (Mayak et al., 2004; Timmusk et al., 2014). Regarding grapevine, an extensive analysis of endophytes from different rootstocks and the cv. Barbera in relation to drought has been performed (Rolli et al., 2015). In this study, eight strains from the Pseudomonas, Acinetobacter, Bacillus, Delftia, and Sphingobacterium genera were selected from over 510 total isolates and shown to be capable of colonizing different hosts, such as Arabidopsis. It has been demonstrated that different strains of the Pseudomonas and Acinetobacter genus and Bacillus subtilis could alleviate drought damage because of their root/shoot growth-promoting activities (Rolli et al., 2015; Rolli et al., 2017). These bacteria were characterized by the production of indole acetic acid (IAA), ACC deaminase activity, phosphate solubilization, and ammonia production via peptone mineralization. Using the same isolates, in pepper plants, the authors demonstrated that the PGP activity phenotypes related to drought were not constitutive but underwent activation in planta when the host was challenged with drought stress. Drought-sensitive rootstocks displayed the most enhanced effects. Three strains were further tested on grapevine under outdoor conditions and conferred higher root biomass to the plants (Rolli et al., 2015). The retardation of water loss in Vitis vinifera was also demonstrated by root isolates of Bacillus licheniformis and Pseudomonas fluorescens, whose effects, as measured from 30 days after the inoculation of in vitro-cultured plants, also extended to other measurable and possibly related parameters. These parameters included the accumulation of abscisic acid (ABA) (stimulated 76-fold over the untreated control by B. licheniformis and 45-fold by P. fluorescens) and synthesis of defense-related terpenes, such as α-pinene, terpinolene, 4-carene, limonene, eucalyptol, lilac aldehyde A, α-bergamotene, α-farnesene, nerolidol, and farnesol (Salomon et al., 2014). Regarding sensu lato, endophytic grapevine drought stress has also been shown to be highly alleviated by the presence of mycorrhizal fungi, especially in plants grafted on drought-sensitive rootstocks, such as 775P, 101-14Mgt, and 5BB (Nikolaou et al., 2003). A related stress factor in plants is soil salinity, and in this case, the recruitment of microorganisms under the endophytic condition is a critical aspect (Numan et al., 2018). The mechanisms by which internalized microbes could help alleviate salt stress appear to be linked to their production of plant hormones, including auxins, gibberellins, and ABA (Yanni et al., 2001; Jha and Subramanian, 2013).
Many endophytes have the ability to synthetize ACC deaminase, which alleviates plant stress consequences, as it contributes to lowering the ethylene level. Ethylene is an important plant hormone that is extensively studied as a mediator of plant stress response signaling. Ethylene is formed from methionine via S-adenosyl-l-methionine, which is converted into 1-aminocyclopropane-1-carboxylic acid by the enzyme ACC oxidase. The stress-induced accumulation of ethylene is usually deleterious to plant growth and health. Several endophytes, such as P. fluorescens, P. phytofirmans, and Pseudomonas migulae, possess the ability to produce the enzyme ACC deaminase, which, by cleaving the ethylene precursor, avoids ethylene accumulation, masking drought and salinity stress and, thus, improving stress tolerance and plant growth (Miliute et al., 2015; Gamalero et al., 2017).
Other molecules are released by endophytes that can intervene in ROS scavenging and, thus, help grapevine cope with different abiotic stresses, such as cold, drought, or salinity. B. licheniformis has been demonstrated to release several types of secondary metabolites, such as monoterpenes, exerting an antioxidant activity, and sesquiterpenes, showing antimicrobial properties (Salomon et al., 2014). The other secondary metabolites in the terpenoid biosynthesis pathway are produced by endophytic microorganisms that could be important for their beneficial activity in grapevine. Indeed, B. licheniformis produces carotenoids that could act as antioxidant species, which is particularly helpful under different stress conditions (Cohen et al., 2018). Notably, these compounds are precursors of ABA in plants, serving as the base of the drought resistance conferred by B. licheniformis (Salomon et al., 2016). It has also been reported that B. licheniformis and P. fluorescens are able to induce the expression of the genes coding components of ABA synthesis and signaling pathways in grapevine plants (Salomon et al., 2014). Both bacterial endophytes and endophytic fungi (Septglomus deserticola, Funneliformis mosseae, Rhizoglomus intraradices, Rhizoglomus clarum, and Glomus aggregatum) could modulate ABA metabolism in inoculated grapevine plants, giving an advantage over uninoculated plants under drought conditions (Torres et al., 2018). It has also been reported that in grapevine, B. licheniformis enhanced ascorbate peroxidase activity, while Micrococcus luteus and P. fluorescens augmented peroxidase activity, exerting strong antioxidant activity (Funes Pinter et al., 2017).
Actually, many endophytes produce protective molecules, such as melatonin, proline, and carotenoids, which play an active defense role against abiotic stress. The action is not only local; in fact, endophytes can also produce volatile organic compounds (VOCs), which, in addition to being involved in the first interaction/recognition by the plant (Liu and Brettell, 2019), can also be precursors of important signal molecules. Interesting examples include the release of carotenoids in the host plant as precursors of hormones (ABA) or detoxifying enzymes, such as ACC deaminase, that modulate the ethylene content and consequently the damage triggered upon stress. An interesting example is melatonin accumulation, which occurs in Bacillus amyloliquefaciens-inoculated grapevines, counteracting the negative effects of salt, drought, and cold stress (Jiao et al., 2016). The same bacterial strain reduced the upregulation of tryptophan decarboxylase and serotonin N-acetyltransferase transcription (Jiao et al., 2016). Indeed, melatonin is a strong antioxidant that increases CuZn superoxide dismutase (SOD), Fe-SOD, catalase, and thylakoid‐bound ascorbate peroxidase activities (Baier et al., 2019). It has been observed that an enhancement of endogenous melatonin synthesis is triggered by various abiotic stress factors, which can also be due to endophytic bacteria, such as P. fluorescens, which is able to induce the transformation of tryptophan into melatonin in the roots of different grapevine cultivars (Ma et al., 2017). To achieve its beneficial effect, melatonin can be used in exogenous applications. However, melatonin-producing endophytes might have long-term effects on the endogenous melatonin levels in plants once they enter plant tissues. The presence of viruses in grapevine has been noted as a positive factor enhancing tolerance to water stress, shifting the role of some viruses from deleterious to mutualistic because of the long co-existence between the virus and its host (Gambino et al., 2012).
Defense Against Biotic Agents
General Aspects
Vitis vinifera can be affected by a numerous pathogens often associated with a severe reduction in yield and product quality (Armijo et al., 2016). To guarantee high-quality production, pesticides and fungicides are currently applied in vineyards, although the continuous use of chemicals may cause the emergence of resistant microorganisms, environmental pollution, and heavy consequences on human health (Pérez-Ortega et al., 2012; Damalas and Koutroubas, 2016).
Colonization by endophytic microorganisms places grapevine in a state of alert (primed) that allows a rapid and intense resistance response to subsequent stresses at a low fitness cost instead of wastefully activating defenses (Martinez-Medina et al., 2016; Mauch-Mani et al., 2017). Experimental evidence showed that endophyte perception triggers a local immune response that is significantly weaker in intensity than that occurring during non-host interactions (Bordiec et al., 2011; Trdá et al., 2014). This type of resistance can provide protection to the entire plant against a broad spectrum of fungal, oomycete, bacterial, and viral pathogens and insects (De Vleesschauwer and Höfte, 2009; Van der Ent et al., 2009; Pineda et al., 2010).
Most endophytic bacteria are well known for their capability to produce secondary metabolites that have an inhibitory effect on a wide range of phytopathogens. Some compounds are important for protection because they can inhibit the growth of other bacteria and fungi (antimicrobial compounds) (Shameer and Prasad, 2018), but they also play a significant role in the mechanisms underlying signaling, defense, and gene regulation. These metabolites comprise phytoalexins (Gruau et al., 2015) and various biocide compounds (Verhagen et al., 2011; Esmaeel et al., 2018), such as HCN (Samad et al., 2017) and antibiotics (Nigris et al., 2018; Shameer and Prasad, 2018). A common trait in the group of Pseudomonas and Bacillus is HCN production (Shameer and Prasad, 2018). HCN can act as a biocide, suppressing the growth of different pathogens, although in grapevine, it has been mainly studied due to its property of breaking summer bud dormancy (Sudawan et al., 2016). Several VOCs produced by different endophyte strains exhibit antibacterial and/or antifungal activity because they can reduce fungal growth, impair fungal spores and hyphae, and/or promote plant growth (Haidar et al., 2016b). An important strategy that helps endophyte-colonized plants cope with pathogens is the ability of endophytes to modulate plant metabolism to restrict pathogen growth and invasion (Miotto-Vilanova et al., 2016). Considering that many factors can influence the effectiveness of BCAs, their efficacy must be confirmed through appropriate experimental procedures both in vitro and in vivo possibly using a multi-organ screening approach (Haidar et al., 2016a). The organ host and pathogen genotype/strain may considerably affect the antagonist efficiency. Haidar et al. (2016a) reported that the antagonist efficiency of Pantoea agglomerans (S2 and S3) and Enterobacter sp. (S24) on Botrytis cinerea in grapevine leaves was lower than that observed in unwounded berries. Moreover, some endophytic species active against one tested pathogen could stimulate other diseases as reported in Bacillus sp. S43, which improved the symptoms caused by Neofusicoccum parvum when applied to grapevine cuttings against B. cinerea (Haidar et al., 2016a).
Protection Against Bacterial Pathogens
Grapevine diseases caused by bacteria are characterized by a biotrophic host–pathogen relationship in which the microorganism interacts with the living plant without killing it. Crown gall caused by tumorigenic Rhizobium vitis is the most important bacterial disease of grapevine worldwide (Armijo et al., 2016). The biological control of crown gall disease in grapevine was achieved by the application of antagonistic endophytic bacteria, including Enterobacter agglomerans, Rahnella aquatilis, and Pseudomonas sp. (Bell et al., 1995), and the non-pathogenic R. vitis strains F2/5 (Burr and Reid, 1994) and ARK-1 (Kawaguchi, 2013). Moreover, endophytic Pseudomonas fluorescens 1100-6, Bacillus subtilis EN63-1, and Bacillus sp. EN71-1 isolated from Malus domestica were reported as potential BCAs of crown gall (Eastwell et al., 2006). The inhibition of tumorigenic Rhizobium spp. occurs through different mechanisms, such as the production of antibacterial compounds (Chen et al., 2007), quorum sensing, caseinolytic protease activation (Kaewnum et al., 2013), and suppression of virulence gene expression (Kawaguchi, 2015; Kawaguchi et al., 2019).
Xylella fastidiosa, which is the agent of grapevine Pierce’s disease (PD), is a gram-negative, xylem-limited bacterium transmitted by leafhopper vectors (Kyrkou et al., 2018). Due to the impact of X. fastidiosa infections, several attempts to identify potential BCAs against this pathogen have been published. Virulent strains of X. fastidiosa acting as an antagonistic of the wild-type strain reduced the severity of PD symptoms in the grapevine cv. Carignan (Hopkins, 2005). In particular, the X. fastidiosa strain EB92-1 isolated from elderberry provided good control of PD in vineyard of cv. Flame Seedless and cv. Cabernet Sauvignon (Hopkins, 2005). Newman et al. (2008) demonstrated that the incidence and severity of PD were significantly reduced in grapevine co-inoculated with X. fastidiosa and Pseudomonas spp. strain G compared with those in plants inoculated with the pathogen alone. The disease reduction was dependent on endophyte interference with diffusible signal factor (DSF)-mediated signaling. Specifically, the highest suppression level was obtained using carAB mutants of Pseudomonas spp. strain G characterized by superior capabilities of DSF degradation (Newman et al., 2008). Lindow et al. (2005) confirmed the ability of endophytic species within the genera Paenibacillus, Pseudomonas, Staphylococcus, and Bacillus in reducing disease symptoms by altering X. fastidiosa DSF-mediated signaling. A promising result was obtained using the Burkholderia phytofirmans strain PsJN against X. fastidiosa in different grapevine cultivars co-inoculated or even inoculated 30 days after the pathogen (Lindow et al., 2017). In planta experiments suggested that the pathogen limitation could be due to not only molecular interference with X. fastidiosa quorum-sensing regulation and biofilm formation but also induction of the grapevine immune defense responses (Lindow et al., 2017). Deyett et al. (2017) found that endophytic P. fluorescens and Achromobacter xylosoxidans showed significant negative correlations with the X. fastidiosa titer; in particular, P. fluorescens emerged as a promising BCA of PD.
Phytoplasmas are phloem-limited plant-pathogenic bacteria transmitted by phloem-feeding insect vectors (Dickinson et al., 2013). Different “Candidatus phytoplasma” species can infect the grapevine to induce a complex of diseases commonly referred to as grapevine yellows (GYs) (Maejima et al., 2014). The contribution of endophytic Pseudomonas migulae 8R6 to inducing resistance to grapevine FD phytoplasma was demonstrated in the experimental host Catharanthus roseus by Gamalero et al. (2017). Specifically, the increased resistance was related to the bacterial ACC deaminase activity, which regulates the level of the stress hormone ethylene, leading to lower symptom expression (Gamalero et al., 2017). Bulgari et al. (2011, 2014) reported that the grapevine cv. Barbera recovered from FD showed a higher level of bacterial diversity than infected plants. In particular, some bacterial species associated with induced systemic resistance (ISR), such as Burkholderia sp., Bacillus pumilis, and Paenibacillus pasadenensis, were found only in the recovered but not in the infected grapevines (Bulgari et al., 2011). Moreover, the population dynamics of endophytic bacteria, such as Burkholderia, Methylobacterium, and Pantoea, were influenced by the presence of phytoplasma (Bulgari et al., 2014). The presence of ISR-inducing bacteria in the recovered plants could indicate the possible involvement of endophytes in recovery from GYs.
Protection Against Fungal Pathogens
Recently, several new potential BCAs were identified by culture-dependent techniques coupled with the in vitro assessment of fungal inhibition. Some endophytes isolated from Glera grapevine have been shown to produce biocontrol molecules active against phytopathogenic fungi; among them, Bacillus licheniformis GL174 was found to secrete cyclic lipopeptides (LPs) belonging to the surfactins and lichenisins families (Favaro et al., 2016; Nigris et al., 2018). Andreolli et al. (2016) isolated 11 bacterial strains within the genera Bacillus, Brevibacillus, Lysinibacillus, Nocardioides, Stenotrophomonas, Microbacterium, Pantoea, and Pseudoxanthomonas from field-grown grapevines cv. Corvina, showing in vitro antifungal activity against the necrotrophic pathogen B. cinerea, which is the agent of grapevine gray mold. The ability of endophytic Bacillus spp. to induce resistance against B. cinerea in grapevine was also reported by Farace et al. (2015), who showed that grapevine plant cells perceive three families of cyclic LPs from B. subtilis that differentially activate the plant innate immune response. Campisano et al. (2015) isolated 25 endophytic bacterial strains showing high inhibitory effects against B. cinerea from domesticated and wild grapevines in northern Italy. Among them, the most effective strains belonged to the genera Bacillus and Pantoea.
Several works proved the effectiveness of Pseudomonas spp. as BCAs against B. cinerea. P. fluorescens PTA-CT2 can elicit defense responses in grapevine against B. cinerea at both the local and systemic levels (Trotel-Aziz et al., 2008; Verhagen et al., 2011; Gruau et al., 2015). Although PTA-CT2 can colonize grapevine roots but not above-ground plant organs, a systemic defense response is activated by molecular signals transferred from the roots to distal leaves (Gruau et al., 2015). Distinct patterns of defense-related gene expression were found in grapevine roots and leaves, particularly in some genes associated with cell death and hypersensitive response (HR) (Gruau et al., 2015). Following B. cinerea infection in grapevine leaves, PTA-CT2-mediated ISR enhanced stilbene accumulation, glutathione 3-transferase gene expression, the downregulation of HR and cell death marker genes (Gruau et al., 2015). Verhagen et al. (2010) found that the resistance to B. cinerea in grapevine could be induced by Pseudomonas aeruginosa (7NSK2), P. fluorescens (strains CHA0, Q2-87 and WCS417), and Pseudomonas putida (WCS358) by the production of phytoalexins and stimulation of oxidative bursts.
Streptomyces anulatus S37 isolated from wild V. vinifera is an endophytic PGP rhizobacterium (PGPR) that confers resistance against different pathogens, including B. cinerea (Loqman et al., 2009). Vatsa-Portugal et al. (2017) showed that S. anulatus S37 perception by grapevine cells triggers early and late defense responses, such as ion fluxes, oxidative burst, extracellular alkalinization, activation of protein kinases, induction of defense gene expression, and phytoalexin accumulation. Moreover, S. anulatus S37-primed grapevine cells became refractory to infection by B. cinerea, showing a reduction in pathogen-induced cell death (Vatsa-Portugal et al., 2017).
The Paraburkholderia phytofirmans strain PsJN is an endophytic PGPR known for its antifungal activity against gray mold disease (Ait Barka et al., 2000). Following root inoculation, bacterial cells diffuse through grapevine xylem vessels, forming a biofilm at the leaf surface and exerting a direct antagonistic effect on B. cinerea (Miotto-Vilanova et al., 2016). Different works revealed that the grapevine responds to P. phytofirmans PsJN inoculation by the activation of a local immune response characterized by the accumulation of phenolic compounds, salicylic acid (SA) accumulation, ion fluxes, and defense gene regulation (Compant et al., 2005; Bordiec et al., 2011; Miotto-Vilanova et al., 2016). Moreover, following infection by B. cinerea, only the bacterized grapevine plantlets trigger an oxidative burst in leaf tissues, callose deposition in the stomata, the induction of SA and jasmonic acid (JA) pathogenesis-related (PR) genes (PR1, PR2, and PR5) and changes in leaf carbohydrate metabolism (Miotto-Vilanova et al., 2016). In particular, P. phytofirmans is associated with the ability to redirect carbohydrates in favor of fructose, which is actually not useful for the fungus (Miotto-Vilanova et al., 2016).
Grapevine trunk diseases (GTDs), such as Esca, Eutypiosis, and Botryosphaeriae diebacks, are associated with a complex of fungal species causing premature decline in vines, yield loss, and poor wine quality worldwide (Gramaje et al., 2018). Pruning and fungicide treatment applied to control GTDs have limited efficacy; moreover, these approaches are expensive and not environmentally sustainable. Eutypa dieback, which is caused by the Diatrypaceous fungus Eutypa lata, is a major trunk disease in grapevines associated with the heavy loss of production (Gramaje et al., 2018). Ferreira et al. (1991) reported that an endophytic strain of B. subtilis isolated from grapevine cv. Chenin Blanc inhibited E. lata mycelium growth and spore germination in vitro and significantly reduced fungal infection on pruning wounds. Several endophytic bacterial strains were investigated due to their inhibitory effect on Phaeomoniella chlamydospora and Phaeoacremonium aleophilum, which are the agents of grapevine Esca disease colonizing the xylem tissues of vine plants (Gramaje et al., 2018). The B. subtilis strain AG1 was reported to inhibit mycelial growth of P. chlamydospora and P. aleophilum in vitro by producing antagonistic substances (antibiotics) stable at high temperatures and resistant to enzymatic degradation (Alfonzo et al., 2009). Another interesting example includes Paenibacillus sp. (S19) and Bacillus pumilus, which possess effective antagonistic activity against P. chlamydospora through the production of the volatile compound pyrazine, which inhibits mycelia growth (Haidar et al., 2016b). Similarly, two Bacillus spp. strains effective against Esca-associated fungi were isolated from field-grown grapevines cv. Corvina (Andreolli et al., 2016). More recently, the same research group identified a Pseudomonas protegens MP12 strain in a soil sample able to colonize inner grapevine tissues and is exhibiting in vitro inhibitory effects on mycelial growth of P. chlamydospora and P. aleophilum (Andreolli et al., 2019). This strain showed in vitro activity against several grapevine phytopathogens, such as B. cinerea, Alternaria alternata, Aspergillus niger, Penicillium expansum, and N. parvum, and in vivo antifungal activity against B. cinerea on grapevine leaves (Andreolli et al., 2019). Endophytic bacteria showing antagonistic activity against N. parvum, which is among the most virulent GTD-associated fungi, were isolated from grapevine wood tissues in Tunisia (Rezgui et al., 2016; Haidar et al., 2016a). Among 11 strains showing in vitro activities against N. parvum, B. subtilis B6 reduced the size of wood necrosis on young grapevines cv. Italia (Rezgui et al., 2016). Several endophytic fungi have also been proposed for the biocontrol of grapevine truncal diseases; these fungi include Pythium oligandrum as an inducer of plant resistance (Yacoub et al., 2016), Aureobasidium spp. (Gonzàlez et al., 2012), Chaetomium spp. (Spagnolo et al., 2012), Fusarium lateritium (Christen et al., 2005), Trichoderma atroviride (Pertot et al., 2016), and Epicoccum layuense (Del Frari et al., 2019)
Recently, Álvarez-Pérez et al. (2017) demonstrated the efficacy of Actinobacteria isolated from the grapevine root system as BCAs of GTDs. Field trials enabled the identification of the endophytic strain Streptomyces sp. VV/E1, which significantly reduced the infection rates of Dactylonectria sp., Ilyonectria sp., P. chlamydospora, and Phaeoacremonium minimum and is associated with a decline in young grapevines (Álvarez-Pérez et al., 2017).
Wicaksono et al. (2016) reported the potential role of endophytic bacteria from roots of Leptospermum scoparium, a New Zealand native medicinal plant, as BCA against botryosphaeriaceous species of fungi associated with GTDs. In vitro assays of 10 Burkholderia spp., Serratia sp. and Pseudomonas spp. showed that all isolates were effective against Neofusicoccum spp. by the production of antibiotic diffusible and/or volatile compounds (Wicaksono et al., 2017). Pseudomonas spp. isolates from L. scoparium, which are active against multiple botryosphaeriaceous species in vitro, showed evidence of specificity towards a particular pathogen species once inoculated in planta (Wicaksono et al., 2017).
Grapevine downy mildew caused by the oomycete Plasmopara viticola is a serious and persistent disease problem for the grapevine industry that is difficult to control through chemical and agricultural practices. Musetti et al. (2006) demonstrated the antifungal activity against P. viticola of diketopiperazines (DKPs) produced by an endophytic strain of A. alternata isolated from grapevine leaves showing anomalous downy mildew symptoms. The application of DKPs inhibited P. viticola sporulation and induced severe ultrastructural alterations on fungal mycelium (Musetti et al., 2006). Acremonium byssoides is an endophytic fungus naturally present in different grapevine varieties (Burruano et al., 2008). A. byssoides is able to actively parasitize the pathogen in grapevine leaves inoculated with P. viticola. Moreover, culture filtrates and a crude extract of an A. byssoides strain isolated from grapevine cv. Insolia completely inhibited sporangial germination of P. viticola (Burruano et al., 2008). In a recent study, B. subtilis GLB191 and B. pumilus GLB197 were identified among 239 bacterial endophytes isolated from grapevine leaves in China (Zhang et al., 2017). Their potential application as BCAs against downy mildew disease was demonstrated by leaf disk assays and under field conditions. The bacterium Paenibacillus sp. strain B2 secretes the peptide paenimyxin, which acts as a biopesticide against different grapevine pathogens. Hao et al. (2017) showed that Paenibacillus sp. strain B2 can inhibit the development of P. viticola and B. cinereain vitro and affects the activity of the ectoparasitic nematode Xiphinema indexin vitro and in planta.
Protection Against Insects
Finally, grapevine protection against piercing-sucking insects was achieved by the endophytic colonization of the entomophagous fungus Beauveria bassiana in both young potted grapevine plants in a greenhouse and mature plants in the vineyard (Rondot and Reineke, 2018). Following spray inoculation, endophytic B. bassiana was detected for at least 21 days inside the leaves of potted plants and up to 5 weeks after the final application in the field. Experimental trials demonstrated that the endophytic fungus can reduce the infestation rate and growth of the mealybug vector of leafroll and rugose wood viruses Planococcus ficus in leaves of potted grapevines and infestation of the grape leafhopper Empoasca vitis in the field (Rondot and Reineke, 2018).
Influence of Endophytes on Product Quality
Among the plant products, wine entails one of the most rewarding items in the current agricultural economy. Its market value attracts exceptional attention to the plant’s overall conditions, including its inner microbiome. Since endophyte metabolism can contribute to that of the plant host and the biochemical composition of its fruits, the nature of grapevine endophytic taxa identities, ecological attitudes, potential toxicity, and clinical relevance are all aspects worthy of a thorough investigation.
While the beneficial effect of endophytes on host plants as growth promoters and stress resistance inducers has been reported in several papers, only a few studies addressed their influence on grape and wine quality and almost exclusively focused on fungal endophytes. Recent works demonstrated that wine and its bouquet are also under the influence of endophytic colonizers of grapes. Yang et al. (2016) showed that the inoculation of eight fungal endophytes isolated from Vitis vinifera modified the physio-chemical status of field-grown grapevines in both leaves and berries during the ripening stage. In particular, fungal endophytes induced variations in the content of reducing sugar, total flavonoids, total phenols, trans-resveratrol, and activities of PAL in both tissues. Moreover, the inoculation of different strains of fungal endophytes led to different grape metabolite statuses with some strains, such as CXB-11 (Nigrospora sp.) and CXC-13 (Fusarium sp.), exerting greater promotion effects on grapevine metabolites (Yang et al., 2016). Huang et al. (2018) observed that dual cultures with different endophytic fungal strains were characterized by different metabolite compositions in grapevine flesh cells. In particular, the modification of metabolic profiles by fungal endophytes was fungal strain/genus specific. These works confirmed that endophyte–host metabolic interactions influence the introduction of specific metabolites in the host plant, supporting the possibility of using fungal endophytes to shape grape qualities and characteristics (Huang et al., 2018).
The capability of endophytic fungi to produce plant secondary metabolites or phytochemicals could be exploited for cost-effective large-scale production (Suryanarayanan et al., 2009). Over the last few years, different works reported the identification of endophytic fungal strains producing pharmaceutically valuable compounds with beneficial effects on human health, such as resveratrol. Grapevine inoculation with endophytic Acinetobacter lwoffii, Bacillus subtilis, and Pseudomonas fluorescens effective against Botrytis cinerea leads to the accumulation of host-synthesized stilbenic phytoalexins, especially trans-resveratrol (3,5,4′-tryhydroxystilbene) and its oligomer, trans-ε-viniferin, which, in turn, could contribute to the grape fruit metabolite composition (Verhagen et al., 2011). Recently, 36 endophytic fungal strains isolated in China from grapevine cv. Cabernet Sauvignon were assessed for their ability to produce resveratrol in vitro (Liu et al., 2016). The morphological and molecular analyses allowed the identification of the C2J6 strain of Aspergillus niger, showing stable high resveratrol production (Liu et al., 2016). Dwibedi and Saxena (2018) analyzed the resveratrol-producing potential of 53 endophytic fungi from different V. vinifera varieties in different regions in India. The resveratrol-producing isolates were assigned to the following seven genera: Aspergillus, Botryosphaeria, Penicillium, Fusarium, Alternaria, Arcopilus, and Lasiodiplodia. In particular, the highest resveratrol content was obtained from the culture of Arcopilus aureus isolate #12VVLPM (Dwibedi and Saxena, 2018).
In conclusion, far from being the ultimate framing of such a dynamic matter, this article describes the current picture of this entangling, paradigmatic, and multi-faceted example of a wealthy array of finely evolved plant–microbe interactions.
Author Contributions
DP, AS, FC, and MZ wrote the first draft. DP, AS, DC, EB, FS, RM, FC and MZ made substantial, direct, and intellectual contributions to this work.
Conflict of Interest
The authors declare that the research was conducted in the absence of any commercial or financial relationships that could be construed as a potential conflict of interest.
References
Ait Barka, E., Belarbi, A., Hachet, C., Nowak, J., Audran, J. C. (2000). Enhancement of in vitro growth and resistance to grey mould of Vitis vinifera co-cultured with plant-promoting rhizobacteria. FEMS Microbiol. Lett. 186, 91–95. doi: 10.1111/j.1574-6968.2000.tb09087.x
Alfonzo, A., Conigliaro, G., Torta, L., Burruano, S., Monschetti, G. (2009). Antagonism of Bacillus subtilis strain AG1 against vine wood fungal pathogens. Phytopathol. Mediterr. 48, 155–158. doi: 10.14601/Phytopathol_Mediterr-2886
Ali, S., Duan, J., Charles, T. C., Glick, B. R. (2014). A bioinformatics approach to the determination of genes involved in endophytic behavior in Burkholderia spp. J. Theor. Biol. 343, 193–198. doi: 10.1016/j.jtbi.2013.10.007
Álvarez-Pérez, J. M., González-García, S., Cobos, R., Olego, M. A., Ibañez, A., Díez-Galán, A., et al. (2017). Use of endophytic and rhizospheric actinobacteria from grapevine plants to reduce nursery fungal infections that lead to young grapevine decline. Appl. Environ. Microbiol. 83 (24), 28986378. doi: 10.1128/AEM.01564-17
Andreolli, M., Lampis, S., Zapparoli, G., Angelini, E., Vallini, G. (2016). Diversity of bacterial endophytes in 3 and 15 year-old grapevines of Vitis vinifera cv. Corvina and their potential for plant growth promotion and phytopathogen control. Microbiol. Res. 183, 42–52. doi: 10.1016/j.micres.2015.11.009
Andreolli, M., Zapparoli, G., Angelini, E., Lucchetta, G., Lampis, S., Vallini, G. (2019). Pseudomonas protegens MP12: a plant growth-promoting endophytic bacterium with broad-spectrum antifungal activity against grapevine phytopathogens. Microbiol. Res. 219, 123–131. doi: 10.1016/j.micres.2018.11.003
Armijo, G., Schlechter, R., Agurto, M., Muñoz, D., Nuñez, C., Arce-Johnson, P. (2016). Grapevine pathogenic microorganisms: understanding infection strategies and host response scenarios. Front. Plant Sci. 7, 382. doi: 10.3389/fpls.2016.00382
Baldan, E., Nigris, S., Populin, F., Zottini, M., Squartini, A., Baldan, B. (2014). Identification of culturable bacterial endophyte community isolated from tissues of Vitis vinifera “Glera”. Plant Biosyst. 148, 508–516. doi: 10.1080/11263504.2014.916364
Baier, M., Bittner, A., Prescher, A., van Buer, J. (2019). Preparing plants for improved cold tolerance by priming. Plant Cell Env. 42 (3), 782–800. doi: 10.1111/pce.13394
Barka, E. A., Nowak, J., Clément, C. (2006). Enhancement of chilling resistance of inoculated grapevine plantlets with a plant growth-promoting rhizobacterium, Burkholderia phytofirmans strain PsJN. Appl. Environ. Microbiol. 72, 7246–7252. doi: 10.1128/AEM.01047-06
Bell, C. R., Dickie, G. A., Harvey, W. L. G., Chan, J. W. Y. F. (1995). Endophytic bacteria in grapevine. Can. J. Microbiol. 41, 46–53. doi: 10.1139/m95-006
Bokulich, N. A., Thorngate, J. H., Richardson, P. M., Mills, D. A. (2014). Microbial biogeography of wine grapes is conditioned by cultivar, vintage, and climate. Proc. Natl. Acad. Sci. U. S. A 111, E139–E148. doi: 10.1073/pnas.1317377110
Bordiec, S., Paquis, S., Lacroix, H., Dhondt, S., Ait Barka, E., Kauffmann, S., et al. (2011). Comparative analysis of defence responses induced by the endophytic plant growth-promoting rhizobacterium Burkholderia phytofirmans strain PsJN and the non-host bacterium Pseudomonas syringae pv. pisi in grapevine cell suspensions. J. Exp. Bot. 62 (2), 595–603. doi: 10.1093/jxb/erq291
Bulgari, D., Casati, P., Crepaldi, P., Daffonchio, D., Quaglino, F., Brusetti, L., et al. (2011). Restructuring of endophytic bacterial communities in grapevine yellows-diseased and recovered Vitis vinifera L. plants. Appl. Environ. Microbiol. 77, 5018–5022. doi: 10.1128/AEM.00051-11
Bulgari, D., Casati, P., Quaglino, F., Bianco, P. A. (2014). Endophytic bacterial community of grapevine leaves influenced by sampling date and phytoplasma infection process. BMC Microbiol. 14, 198. doi: 10.1186/1471-2180-14-198
Burr, T. J., Reid, C. L. (1994). Biological control of grape crown gall with non tumorigenic Agrobacterium vitis F2/5. Amer. J. Enol. Vitic. 45, 213–219.
Burruano, S., Alfonzo, A., Lo Piccolo, S., Conigliaro, G., Mondello, V., Torta, L., et al. (2008). Interaction between Acremonium byssoides and Plasmopara viticola in Vitis vinifera. Phytopathol. Mediterr. 47, 122–131. doi: 10.14601/Phytopathol_Mediterr-2615
Campisano, A., Ometto, L., Compant, S., Pancher, M., Antonielli, L., Yousaf, S., et al. (2014a). Interkingdom transfer of the acne-causing agent, Propionibacterium acnes, from human to grapevine. Mol. Biol. Evol. 31, 1059–1065. doi: 10.1093/molbev/msu075
Campisano, A., Antonielli, L., Pancher, M., Yousaf, S., Pindo, M., Pertot, I. (2014b). Bacterial endophytic communities in the grapevine depend on pest management. PLoS One 9, e112763. doi: 10.1371/journal.pone.0112763
Campisano, A., Pancher, M., Puopolo, G., Puddu, A., Lopez-Fernandez, S., Biagini, B., et al. (2015). Diversity in endophyte populations reveals functional and taxonomic diversity between wild and domesticated grapevines. Am. J. Enol. Vitic. 66, 12–21. doi: 10.5344/ajev.2014.14046
Chen, F., Guo, Y. B., Wang, J. H., Li, J. Y., Wang, H. M. (2007). Biological control of grape crown gall by Rahnella aquatilis HX2. Plant Dis. 91, 957–963. doi: 10.1094/PDIS-91-8-0957
Christen, D., Tharin, M., Perrin-Cherioux, S., Abou-Mansour, E., Tabacchi, R., Défago, G. (2005). Transformation of Eutypa dieback and esca disease pathogen toxins by antagonistic fungal strains reveals a second detoxification pathway not present in Vitis vinifera. J. Agric. Food Chem. 53 (18), 7043–7051. doi: 10.1021/jf050863h
Cohen, A. C., Dichiara, E., Jofré, V., Antoniolli, A., Bottini, R., Piccoli, P. (2018). Carotenoid profile produced by Bacillus licheniformis Rt4M10 isolated from grapevines grown in high altitude and their antioxidant activity. Int. J. Food Sci. Tech. 53 (12), 2697–2705. doi: 10.1111/ijfs.13879
Compant, S., Reiter, B., Sessitsch, A., Nowak, J., Clément, C., Ait Barka, E. (2005). Endophytic colonization of Vitis vinifera L. by plant growth-promoting bacterium Burkholderia sp. strain PsJN. Appl. Environ. Microbiol. 71, 1685–1693. doi: 10.1128/AEM.71.4.1685–1693.2005
Compant, S., Clement, C., Sessitsch, A. (2010). Plant growth-promoting bacteria in the rhizo- and endosphere of plants: their role, colonization, mechanisms involved and prospects for utilization. Soil Biol. Biochem. 42, 669–678. doi: 10.1016/j.soilbio.2009.11.024
Compant, S., Mitter, B., Colli-Mull, J. G., Gangl, H., Sessitsch, A. (2011). Endophytes of grapevine flowers, berries, and seeds: identification of cultivable bacteria, comparison with other plant parts, and visualization of niches of colonization. Microb. Ecol. 62, 188–197. doi: 10.1007/s00248-011-9883-y
Compant, S., Brader, G., Muzammil, S., Sessitsch, A., Lebrihi, A., Mathieu, F. (2013). Use of beneficial bacteria and their secondary metabolites to control grapevine pathogen diseases. BioControl 58, 435–455. doi: 10.1007/s10526-012-9479-6
Damalas, C. A., Koutroubas, S. D. (2016). Farmers’ exposure to pesticides: toxicity types and ways of prevention. Toxics 4 (1), 10. doi: 10.3390/toxics4010001
Del Frari, G., Cabral, A., Nascimento, T., Boavida Ferreira, R., Oliveira, H. (2019). Epicoccum layuense a potential biological control agent of esca-associated fungi in grapevine. PLoS One 14 (3), e0213273. doi: 10.1371/journal.pone.0213273
De Vleesschauwer, D., Höfte, M. (2009). Rhizobacteria-induced systemic resistance. Adv. Bot. Res. 51, 223–281. doi: 10.1016/S0065-2296(09)51006-3
Deyett, E., Roper, M. C., Ruegger, P., Yang, J. I., Borneman, J., Rolshausen, P. E. (2017). Microbial landscape of the grapevine endosphere in the context of Pierce’s disease. Phytobiomes 1 (3), 138–149. doi: 10.1094/PBIOMES-08-17-0033-R
Dickinson, M., Tuffen, M., Hodgetts, J. (2013). The phytoplasmas: an introduction. Methods Mol. Biol. 938, 1–14. doi: 10.1007/978-1-62703-089-2_1
Dissanayake, A. J., Purahong, W., Wubet, T., Hyde, K. D., Zhang, W., Xu, H., et al. (2018). Direct comparison of culture-dependent and culture-independent molecular approaches reveal the diversity of fungal endophytic communities in stems of grapevine (Vitis vinifera). Fungal Divers. 90, 85–107. doi: 10.1007/s13225-018-0399-3 doi:
Dwibedi, V., Saxena, S. (2018). Arcopilus aureus, a resveratrol-producing endophyte from Vitis vinifera. Appl. Biochem. Biotechnol. 186, 476–495. doi: 10.1007/s12010-018-2755-x
Eastwell, K., Sholberg, P., Sayler, R. (2006). Characterizing potential bacterial biocontrol agents for suppression of Rhizobium vitis, causal agent of crown gall disease in grapevines. Crop Protect. 25, 1191–1200. doi: 10.1016/j.cropro.2006.03.004
Esmaeel, Q., Pupin, M., Kieu, N. P., Chataigné, G., Béchet, M., Deravel, J., et al. (2016). Burkholderia genome mining for nonribosomal peptide synthetases reveals a great potential for novel siderophores and lipopeptides synthesis. MicrobiologyOpen 5 (3), 512–526. doi: 10.1002/mbo3.347
Esmaeel, Q., Miotto, L., Rondeau, M., Leclère, V., Clément, C., Jacquard, C., et al. (2018). Paraburkholderia phytofirmans PsJN–plants interaction: from perception to the induced mechanisms. Front. Microbiol. 9, 2093. doi: 10.3389/fmicb.2018.02093
Faist, H., Keller, A., Hentschel, U., Deeken, R. (2016). Grapevine (Vitis vinifera) crown galls host distinct microbiota. Appl. Environ. Microbiol. 82, 5542–5552. doi: 10.1128/AEM.01131-16
Farace, G., Fernandez, O., Jacquens, L., Coutte, F., Krier, F., Jacques, P., et al. (2015). Cyclic lipopeptides from Bacillus subtilis activate distinct patterns of defence responses in grapevine. Mol. Plant Pathol. 16, 177–187. doi: 10.1111/mpp.12170
Favaro, G., Bogialli, S., Di Gangi, I. M., Nigris, S., Baldan, E., Squartini, A., et al. (2016). Characterization of lipopeptides produced by Bacillus licheniformis using liquid chromatography with accurate tandem mass spectrometry. Rapid Commun. Mass. Spectrom. 30, 2237–2252. doi: 10.1002/rcm.7705
Fernandez, O., Vandesteene, L., Feil, R., Baillieul, F., Lunn, J. E., Clement, C. (2012). Trehalose metabolism is activated upon chilling in grapevine and might participate in Burkholderia phytofirmans induced chilling tolerance. Planta 236, 355–369. doi: 10.1007/s00425-012-1611-4
Ferreira, J. H. S., Matthee, F. N., Thomas, A. C. (1991). Biological control of Eutypa lata on grapevine by an antagonistic strain of Bacillus subtilis. Phytopathology 81, 283–287. doi: 10.1094/Phyto-81-283
Finkel, O. M., Castrillo, G., Herrera Paredes, S., Salas González, I., Dangl, J. L. (2017). Understanding and exploiting plant beneficial microbes. Curr. Opin. Plant Biol. 38, 155–163. doi: 10.1016/j.pbi.2017.04.018
Funes Pinter, I., Salomon, M. V., Berli, F., Bottini, R., Piccoli, P. (2017). Characterization of the As(III) tolerance conferred by plant growth promoting rhizobacteria to in vitro-grown grapevine. Appl. Soil Ecol. 109, 60–68. doi: 10.1016/j.apsoil.2016.10.003
Gamalero, E., Marzachì, C., Galetto, L., Veratti, F., Massa, N., Bona, E., et al. (2017). An 1-aminocyclopropane-1-carboxylate (ACC) deaminase-expressing endophyte increases plant resistance to flavescence dorée phytoplasma infection. Plant Biosyst. 151 (2), 331–340. doi: 10.1080/11263504.2016.1174172
Gambino, G., Cuozzo, D., Fasoli, M., Pagliarani, C., Vitali, M., Boccacci, P., et al. (2012). Co-evolution between Grapevine rupestris stem pitting-associated virus and Vitis vinifera L. leads to decreased defence responses and increased transcription of genes related to photosynthesis. J. Exp. Bot. 63, 5919–5933. doi: 10.1093/jxb/ers244
Gilbert, J. A., van der Lelie, D., Zarraonaindia, I. (2014). Microbial terroir for wine grapes. Proc. Natl. Acad. Sci. U. S. A 111 (1), 5–6. doi: 10.1073/pnas.1320471110
Gonzàlez, V., Tello, M. L., Andrés, P. (2012). Biocontrol of grapevine trunk pathogens with vinespecific antagonistic endophytic fungi. Phytopathol.Mediterr. 51(2), 448.
Gramaje, D., Úrbez-Torres, J. R., Sosnowski, M. R. (2018). Managing grapevine trunk diseases with respect to etiology and epidemiology: current strategies and future prospects. Plant Dis. 102, 12–39. doi: 10.1094/PDIS-04-17-0512-FE
Gruau, C., Trotel-Aziz, P., Villaume, S., Rabenoelina, F., Clément, C., Baillieul, F., et al. (2015). Pseudomonas fluorescens PTA-CT2 triggers local and systemic immune response against Botrytis cinerea in grapevine. Mol. Plant Microbe Interact. 28, 1117–1129. doi: 10.1094/MPMI-04-15-0092-R
Grube, M., Schmid, F., Berg, G. (2011). Black fungi and associated bacterial communities in the phyllosphere of grapevine. Fungal Biol. 115 (10), 978–986. doi: 10.1016/j.funbio.2011.04.004
Haidar, R., Deschamps, A., Roudet, J., Calvo-Garrido, C., Bruez, E., Rey, P., et al. (2016a). Multi-organ screening of efficient bacterial control agents against two major pathogens of grapevine. Biol. Control 92, 55–65. doi: 10.1016/j.biocontrol.2015.09.003
Haidar, R., Roudet, J., Bonnard, O., Dufour, M. C., Corio-Costet, M. F., Fert, M., et al. (2016b). Screening and modes of action of antagonistic bacteria to control the fungal pathogen Phaeomoniella chlamydospora involved in grapevine trunk diseases. Microbiol. Res. 192, 172–184. doi: 10.1016/j.micres.2016.07.003
Hao, Z., Van Tuinen, D., Wipf, D., Fayolle, L., Chataignier, O., Li, X., et al. (2017). Biocontrol of grapevine aerial and root pathogens by Paenibacillus sp. strain B2 and paenimyxin in vitro and in planta. Biol. Control 109, 42–50. doi: 10.1016/j.biocontrol.2017.03.004
Hopkins, D. L. (2005). Biological control of Pierce’s disease in the vineyard with strains of Xylella fastidiosa benign to grapevine. Plan Dis. 89, 1348–1352. doi: 10.1094/PD-89-1348
Huang, L. H., Yuan, M. Q., Ao, X. J., Ren, A. Y., Zhang, H. B., Yang, M. Z. (2018). Endophytic fungi specifically introduce novel metabolites into grape flesh cells in vitro. PLoS One 13 (5), e0196996. doi: 10.1371/journal.pone.0196996
Jha, Y., Subramanian, R. (2013). Paddy plants inoculated with PGPR show better growth physiology and nutrient content under saline condition. Chil. J. Agric. Res. 73, 213–219. doi: 10.4067/S0718-58392013000300002
Jiao, J., Ma, Y., Chen, S., Liu, C., Song, Y., Qin, Y., et al. (2016). Melatonin-producing endophytic bacteria from grapevine roots promote the abiotic stress-induced production of endogenous melatonin in their hosts. Front. Plant Sci. 7, 1387. doi: 10.3389/fpls.2016.01387
Kaewnum, S., Zheng, D., Reid, C. L., Johnson, K. L., Gee, J. C., Burr, T. J. (2013). A host-specific biological control of grape crown gall by Agrobacterium vitis strain F2/5; its regulation and population dynamics. Phytopathol. 103, 427–435. doi: 10.1094/PHYTO-07-12-0153-R
Karagöz, K., Ateş, F., Karagöz, H., Kotan, R., Çakmakçı, R. (2012). Characterization of plant growth-promoting traits of bacteria isolated from the rhizosphere of grapevine grown in alkaline and acidic soils. Eur. J. Soil. Biol. 50, 144–150. doi: 10.1016/j.ejsobi.2012.01.007
Kawaguchi, A. (2013). Biological control of crown gall on grapevine and root colonization by nonpathogenic Rhizobium vitis strain ARK-1. Microbes Environ. 28, 306–311. doi: 10.1264/jsme2.ME13014
Kawaguchi, A. (2015). Biological control agent Agrobacterium vitis strain ARK-1 suppresses expression of the virD2 and virE2 genes in tumorigenic A. vitis. Euro. J. Plant Pathol. 143, 789–799. doi: 10.1007/s10658-015-0730-8
Kawaguchi, A., Nita, M., Ishii, T., Watanabe, M., Noutoshi, Y. (2019). Biological control agent Rhizobium (= Agrobacterium) vitis strain ARK-1 suppresses expression of the essential and non-essential virgenes of tumorigenic R. vitis. BMC Res. Notes. 12, 1. doi: 10.1186/s13104-018-4038-6
Kyrkou, I., Pusa, T., Ellegaard-Jensen, L., Sagot, M.-F., Hansen, L. H. (2018). Pierce’s disease of grapevines: a review of control strategies and an outline of an epidemiological model. Front. Microbiol. 9, 2141. doi: 10.3389/fmicb.2018.02141
Lidor, O., Dror, O., Hamershlak, D., Shoshana, N., Belausov, E., Zahavi, T., et al. (2018). Introduction of a putative biocontrol agent into a range of phytoplasma- and liberibacter-susceptible crop plants. Pest Manag. Sci. 74 (4), 811–819. doi: 10.1002/ps.4775
Lindow, S. E., Newman, K. L., Purcell, A., Almeida, R. (2005). Management of Pierce’s disease of grape by interfering with cell–cell communication in Xylella fastidiosa. in Proceedings of Pierce’s Disease Research Symposium, 20–22.
Lindow, S., Antonova, E., Baccari, C. (2017). Biological control of Pierce’s disease of grape with an endophytic bacterium. Interim Progress Report for CDFA Agreement Number 16-0514-SA.
Liu, Y., Nan, L., Liu, J., Yan, H., Zhang, D., Han, X. (2016). Isolation and identification of resveratrol-producing endophytes from wine grape Cabernet Sauvignon. Springerplus 5 (1), 1029. doi: 10.1186/s40064-016-2571-0
Liu, H., Brettell, L. E. (2019). Plant defense by VOC-induced microbial priming. Trends Plant Sci. 24 (3), 187–189. doi: 10.1016/j.tplants.2019.01.008
Lòpez-Fernàndez, S., Sonego, P., Moretto, M., Pancher, M., Engelen, K., Pertot, I., et al. (2015). Whole-genome comparative analysis of virulence genes unveils similarities and differences between endophytes and other symbiotic bacteria. Front. Microbiol. 6, 419. doi: 10.3389/fmicb.2015.00419
Lòpez-Fernàndez, S., Mazzoni, V., Pedrazzoli, F., Pertot, I., Campisano, A. (2017). A phloem-feeding insect transfers bacterial endophytic communities between grapevine plants. Front. Microbiol. 8, 834. doi: 10.3389/fmicb.2017.00834
Loqman, S., Ait Barka, E., Clément, C., Ouhdouch, Y. (2009). Antagonistic actinomycetes from Moroccan soil to control the grapevine gray mold. World J. Microbiol. Biotechnol. 25, 81–91. doi: 10.1007/s11274-008-9864-6
Lugtenberg, B., Kamilova, F. (2009). Plant-growth-promoting rhizobacteria. Annu. Rev. Microbiol. 63, 541–556. doi: 10.1146/annurev.micro.62.081307.162918
Ma, Y., Jiao, J., Fan, X., Sun, H., Zhang, Y., Jiang, J., et al. (2017). Endophytic bacterium Pseudomonas fluorescens RG11 may transform tryptophan to melatonin and promote endogenous melatonin levels in the roots of four grape cultivars. Front. Plant Sci. 7, 2068. doi: 10.3389/fpls.2016.02068
Maejima, K., Oshima, K., Namba, S. (2014). Exploring the phytoplasmas, plant pathogenic bacteria. J.Gen. Plant Pathol. 80, 210–221. doi: 10.1007/s10327-014-0512-8
Magnin-Robert, M., Trotel-Aziz, P., Quantinet, D., Biagianti, S., Aziz, A. (2007). Biological control of Botrytis cinerea by selected grapevine-associated bacteria and stimulation of chitinase and ß-1, 3 glucanase activities under field conditions. Eur. J. Plant Path. 118, 43–57. doi: 10.1007/s10658-007-9111-2
Marasco, R., Rolli, E., Fusi, M., Michoud, G., Daffonchio, D. (2018). Grapevine rootstocks shape underground bacterial microbiome and networking but not potential functionality. Microbiome 6 (1), 3. doi: 10.1186/s40168-017-0391-2
Martinez-Medina, A., Flors, V., Heil, M., Mauch-Mani, B., Pieterse, C. M. J., Pozo, M. J., et al. (2016). Recognizing plant defense priming. Trends Plant Sci. 21 (10), 818–822. doi: 10.1016/j.tplants.2016.07.009
Mauch-Mani, B., Baccelli, I., Luna, E., Flors, V. (2017). Defense priming: an adaptive part of induced resistance. Annu. Rev. Plant Biol. 68 (1), 485–512. doi: 10.1146/annurev-arplant-042916-041132
Mayak, S., Tirosh, T., Glick, B. R. (2004). Plant growth-promoting bacteria that confer resistance to water stress in tomatoes and peppers. Plant Sci. 166, 525–530. doi: 10.1016/j.plantsci.2003.10.025
Miliute, I., Buzaite, O., Baniulis, D., Stanys, V. (2015). Bacterial endophytes in agricultural crops and their role in stress tolerance: a review. Zemdirbyste-Agric. 102 (4), 465–478. doi: 10.13080/z-a.2015.102.060
Miotto-Vilanova, L., Jacquard, C., Courteaux, B., Wortham, L., Michel, J., Clément, C., et al. (2016). Burkholderia phytofirmans PsJN confers grapevine resistance against Botrytis cinerea via a direct antimicrobial effect combined with a better resource mobilization. Front. Plant Sci. 7, 1236. doi: 10.3389/fpls.2016.01236
Morgan, H. H., du Toit, M., Setati, M. E. (2017). The grapevine and wine microbiome: insights from high-throughput amplicon sequencing. Front. Microbiol. 8, 820. doi: 10.3389/fmicb.2017.00820
Musetti, R., Vecchione, A., Stringher, L., Borselli, S., Zulini, L., Marzani, C., et al. (2006). Inhibition of sporulation and ultrastructural alterations of grapevine downy mildew by the endophytic fungus Alternaria alternata. Phytopathol. 96, 689–698. doi: 10.1094/PHYTO-96-0689
Newman, K. L., Chatterjee, S., Ho, K. A., Lindow, S. E. (2008). Virulence of plant pathogenic bacteria attenuated by degradation of fatty acid cell-to-cell signaling factors. Mol. Plant Microbe Interact. 21, 326–334. doi: 10.1094/MPMI-21-3-0326
Ngumbi, E., Kloepper, J. (2016). Bacterial-mediated drought tolerance: current and future prospects. Appl. Soil Ecol. 105, 109–125. doi: 10.1016/j.apsoil.2016.04.009
Nigris, S., Baldan, E., Tondello, A., Zanella, F., Vitulo, N., Favaro, G., et al. (2018). Biocontrol traits of Bacillus licheniformis GL174, a culturable endophyte of Vitis vinifera cv. Glera. BMC Microbiol. 18, 133. doi: 10.1186/s12866-018-1306-5
Nikolaou, N., Angelopoulos, K., Karagiannidis, N. (2003). Effects of drought stress on mycorrhizal and non-mycorrhizal Cabernet Sauvignon grapevine, grafted onto various rootstocks. Expl. Agric. 39, 241–252. doi: 10.1017/S001447970300125X
Novello, G., Gamalero, E., Bona, E., Boatti, L., Mignone, F., Massa, N., et al. (2017). The rhizosphere bacterial microbiota of Vitis vinifera cv. Pinot Noir in an integrated pest management vineyard. Front. Microbiol. 8, 1528. doi: 10.3389/fmicb.2017.01528
Numan, M., Bashira, S., Khana, Y., Mumtaza, R., Shinwaric, Z. K., Khanb, A. L., et al. (2018). Plant growth promoting bacteria as an alternative strategy for salt tolerance in plants: a review. Microbiol. Res. 209, 21–32. doi: 10.1016/j.micres.2018.02.003
Pancher, M., Ceol, M., Corneo, P. E., Longa, C. M., Yousaf, S., Pertot, I., et al. (2012). Fungal endophytic communities in grapevines (Vitis vinifera L.) respond to crop management. Appl. Environ. Microbiol. 78 (12), 4308–4317. doi: 10.1128/AEM.07655-11
Pérez-Ortega, P., Gilbert-López, B., García-Reyes, J. F., Ramos-Martos, N., Molina-Díaz, A. (2012). Generic sample treatment method for simultaneous determination of multiclass pesticides and mycotoxins in wines by liquid chromatography–mass spectrometry. J. Chromatogr. 1249, 32–40. doi: 10.1016/j.chroma.2012.06.020
Pertot, I., Prodorutti, D., Colombini, A., Pasini, L. (2016). Trichoderma atroviride SC1 prevents Phaeomoniella chlamydospora and Phaeoacremonium aleophilum infection of grapevine plants during the grafting process in nurseries. BioControl 61 (3), 257–267. doi: 10.1007/s10526-016-9723-6
Pineda, A., Zheng, S.-J., van Loon, J. J. A., Pieterse, C. M. J., Dicke, M. (2010). Helping plants to deal with insects: the role of beneficial soil-borne microbes. Trends Plant Sci. 15, 507–514. doi: 10.1016/j.tplants.2010.05.007
Pinter, M. I. F., Salomon, M. V., Berli, F., Gil, R., Bottini, R., Piccoli, P. (2018). Plant growth promoting rhizobacteria alleviate stress by AsIII in grapevine. Agric. Ecosyst. Environ. 267, 100–108. doi: 10.1016/j.agee.2018.08.015
Pinto, C., Pinho, D., Sousa, S., Pinheiro, M., Egas, C., Gomes, A. C. (2014). Unravelling the diversity of grapevine microbiome. PLoS One 9, e85622. doi: 10.1371/journal.pone.0085622
Pinto, C., dos Santos Custódio, V., Nunes, M., Songy, A., Rabenoelina, F., Courteaux, B., et al. (2018). Understand the potential role of Aureobasidium pullulans, a resident microorganism from grapevine, to prevent the infection caused by Diplodia seriata. Front. Microbiol. 9, 3047. doi: 10.3389/fmicb.2018.03047
Rezgui, A., Ghnaya-Chakroun, A., Vallance, J., Bruez, E., Hajlaoui, M. R., Sadfi-Zouaoui, N., et al. (2016). Endophytic bacteria with antagonistictraits inhabit the wood tissues of grapevines from Tunisian vineyards. Biol. Control 99, 28–37. doi: 10.1016/j.biocontrol.2016.04.005
Rolli, E., Marasco, R., Vigani, G., Ettoumi, B., Mapelli, F., Deangelis, M. L., et al. (2015). Improved plant resistance to drought is promoted by the root-associated microbiome as a water stress-dependent trait. Environ. Microbiol. 17 (2), 316–331. doi: 10.1111/1462-2920.12439
Rolli, E., Marasco, R., Saderi, S., Corretto, E., Mapelli, F., Cherif, A., et al. (2017). Root-associated bacteria promote grapevine growth: from the laboratory to the field. Plant Soil 410 (1-2), 369–382. doi: 10.1007/s11104-016-3019-6
Rondot, Y., Reineke, A. (2018). Endophytic Beauveria bassiana in grapevine Vitis vinifera (L.) reduces infestation with piercing-sucking insects. Biol. Control 116, 82–89. doi: 10.1016/j.biocontrol.2016.10.006
Ronseaux, S., Clément, C., Ait Barka, E. (2013). Interaction of Ulocladium atrum, a potential biological control agent, with Botrytis cinerea and grapevine plantlets. Agronomy 3, 632–647. doi: 10.3390/agronomy3040632
Salomon, M. V., Bottini, R., de Souza Filho, G. A., Cohen, A. C., Moreno, D., Gil, M., et al. (2014). Bacteria isolated from roots and rhizosphere of Vitis vinifera retard water losses, induce abscisic acid accumulation and synthesis of defense-related terpenes in in vitro cultured grapevine. Physiol. Plant. 151 (4), 359–374. doi: 10.1111/ppl.12117
Salomon, M. V., Purpora, R., Bottini, R., Piccoli, P. (2016). Rhizosphere associated bacteria trigger accumulation of terpenes in leaves of Vitis vinifera L. cv. Malbec that protect cells against reactive oxygen species. Plant Physiol. Biochem. 106, 295–304. doi: 10.1016/j.plaphy.2016.05.007
Samad, A., Trognitz, F., Compant, S., Antonielli, L., Sessitsch, A. (2017). Shared and host-specific microbiome diversity and functioning of grapevine and accompanying weed plants. Environ. Microbiol. 19, 1407–1424. doi: 10.1111/1462-2920.13618
Santoyo, G., Moreno-Hagelsieb, G., Orozco-Mosqueda, M., del, C., Glick, B. R. (2016). Plant growth-promoting bacterial endophytes. Microbiol. Res. 183, 92–99. doi: 10.1016/j.micres.2015.11.008
Shameer, S., Prasad, T. N. V. K. V. (2018). Plant growth promoting rhizobacteria for sustainable agricultural practices with special reference to biotic and abiotic stresses. Plant Growth Regul. 84 (3), 603–615. doi: 10.1007/s10725-017-0365-1
Spagnolo, A., Magnin-Robert, M., Larignon, P., Clément, C., Fontaine, F. (2012). Preliminary screening for the possible biological control activity of a Chaetomium sp. isolate on grapevine trunk disease agents. Phytopathol. Mediterr. 51, 449–450.
Sudawan, B., Chang, C. S., Chao, H. F., Ku, M. S., Yen, Y. F. (2016). Hydrogen cyanamide breaks grapevine bud dormancy in the summer through transient activation of gene expression and accumulation of reactive oxygen and nitrogen species. BMC Plant Biol. 16 (1), 202. doi: 10.1186/s12870-016-0889-y
Suryanarayanan, T. S., Thirunavukkarasu, N., Govindarajulu, M. B., Sasse, F., Jansen, R., Murali, T. S. (2009). Fungal endophytes and bioprospecting. Fungal Biol. Rev. 23 (1-2), 9–19. doi: 10.1016/j.fbr.2009.07.001
Theocharis, A., Bordiec, S., Fernandez, O., Paquis, S., Dhondt-Cordelier, S., Baillieul, F., et al. (2012). Burkholderia phytofirmans PsJN primes Vitis vinifera L. and confers a better tolerance to low nonfreezing temperatures. Mol. Plant Microbe Interact. 25, 241–249. doi: 10.1094/MPMI-05-11-0124
Thomas, P., Sekhar, A. C., Shaik, S. P. (2017). High taxonomic diversity of cultivation-recalcitrant endophytic bacteria in grapevine field shoots, their in vitro introduction, and unsuspected persistence. Planta 246 (5), 879–898. doi: 10.1007/s00425-017-2733-5
Timmusk, S., El-Daim, I. A., Copolovici, L., Tanilas, T., Kannaste, A., Behers, L., et al. (2014). Drought-tolerance of wheat improved by rhizosphere bacteria from harsh environments: enhanced biomass production and reduced emissions of stress volatiles. PLoS One 9, e96086. doi: 10.1371/journal.pone.0096086
Torres, N., Goicoechea, N., Zamarreño, A. M., Carmen Antolín, M. (2018). Mycorrhizal symbiosis affects ABA metabolism during berry ripening in Vitis vinifera L. cv. Tempranillo grown under climate change scenarios. Plant Sci. 274, 383–393. doi: 10.1016/j.plantsci.2018.06.009
Trdá, L., Fernandez, O., Boutrot, F., Héloir, M. C., Kelloniemi, J., Daire, X., et al. (2014). The grapevine flagellin receptor VvFLS2 differentially recognizes flagellin-derived epitopes from the endophytic growth-promoting bacterium Burkholderia phytofirmans and plant pathogenic bacteria. New Phytol. 201 (4), 1371–1384. doi: 10.1111/nph.12592
Trotel-Aziz, P., Couderchet, M., Biagianti, S., Aziz, A. (2008). Characterization of new bacterial biocontrol agents Acinetobacter, Bacillus, Pantoea and Pseudomonas spp. mediating grapevine resistance against Botrytis cinerea. Environ. Exp. Bot. 64, 21–32. doi: 10.1016/j.envexpbot.2007.12.009
Trotel-Aziz, P., Abou-Mansour, E., Courteaux, B., Rabenoelina, F., Clément, C., Fontaine, F., et al. (2019). Bacillus subtilis PTA-271 counteracts Botryosphaeria dieback in grapevine, triggering immune responses and detoxification of fungal phytotoxins. Front. Plant Sci. 10, 25. doi: 10.3389/fpls.2019.00025
Van der Ent, S., Van Hulten, M., Pozo, M. J., Czechowski, T., Udvardi, M. K., Pieterse, C. M. J., et al. (2009). Priming of plant innate immunity by rhizobacteria and b-aminobutyric acid: differences and similarities in regulation. New Phytol. 183, 419–431. doi: 10.1111/j.1469-8137.2009.02851.x
Vatsa-Portugal, P., Aziz, A., Rondeau, M., Villaume, S., Morjani, H., Clément, C., et al. (2017). How Streptomyces anulatus primes grapevine defenses to cope with gray mold: a study of the early responses of cell suspensions. Front. Plant Sci. 8, 1043. doi: 10.3389/fpls.2017.01043
Verhagen, B. W. M., Trotel-Aziz, P., Couderchet, M., Höfte, M., Aziz, A. (2010). Pseudomonas spp.-induced systemic resistance to Botrytis cinerea is associated with induction and priming of defence responses in grapevine. J. Exp. Bot. 61, 249–260. doi: 10.1093/jxb/erp295
Verhagen, B., Trotel-Aziz, P., Jeandet, P., Baillieul, F., Aziz, A. (2011). Improved resistance against Botrytis cinerea by grapevine-associated bacteria that induce a prime oxidative burst and phytoalexin production. Phytopathol. 101, 768–777. doi: 10.1094/PHYTO-09-10-0242
West, E. R., Cother, E. J., Steel, C. C., Ash, G. J. (2010). The characterization and diversity of bacterial endophytes of grapevine. Can. J. Microbiol. 56, 209–216. doi: 10.1139/w10-004
Wicaksono, W. A., Jones, E. E., Monk, J., Ridgway, H. J. (2016). The bacterial signature of Leptospermum scoparium (Mānuka) reveals core and accessory communities with bioactive properties. PLoS One 11, e0163717. doi: 10.1371/journal.pone.0163717
Wicaksono, W. A., Jones, E. E., Monk, J., Ridgway, H. J. (2017). Using bacterial endophytes from a New Zealand native medicinal plant for control of grapevine trunk diseases. Biol. Control 114, 65–72. doi: 10.1016/j.biocontrol.2017.08.003
Yang, M.-Z., Ma, M.-D., Yuan, M.-Q., Huang, Z.-Y., Yang, W.-X., Zhang, H.-B., et al. (2016). Fungal endophytes as a metabolic fine-tuning regulator for wine grape. PLoS One 11 (9), e0163186. doi: 10.1371/journal.pone.0163186
Yacoub, A., Gerbore, J., Magnin, N., Chambon, P., Dufour, M. C., Corio-Costet, M. F., et al. (2016). Ability of Pythium oligandrum strains to protect Vitis vinifera L., by inducing plant resistance against Phaeomoniella chlamydospora, a pathogen involved in Esca, a grapevine trunk disease. Biol. Control 92, 7–16. doi: 10.1016/j.biocontrol.2015.08.005
Yanni, Y. G., Rizk, R. Y., El-Fattah, F. K. A., Squartini, A., Corich, V., Giacomini, A., et al. (2001). The beneficial plant growth-promoting association of Rhizobium leguminosarum bv. trifolii with rice roots. Aust. J. Plant Physiol. 28, 845–870. doi: 10.1071/PP01069
Zarraonaindia, I., Owens, S. M., Weisenhorn, P., West, K., Hampton-Marcell, J., Lax, S., et al. (2015). The soil microbiome influences grapevine-associated microbiota. MBio 6 (2), e02527–e02e14. doi: 10.1128/mBio.02527-14
Keywords: Vitis vinifera, endophytes, plant growth-promoting bacteria, stress-tolerance, biocontrol
Citation: Pacifico D, Squartini A, Crucitti D, Barizza E, Lo Schiavo F, Muresu R, Carimi F and Zottini M (2019) The Role of the Endophytic Microbiome in the Grapevine Response to Environmental Triggers. Front. Plant Sci. 10:1256. doi: 10.3389/fpls.2019.01256
Received: 08 June 2019; Accepted: 09 September 2019;
Published: 09 October 2019.
Edited by:
Dario Cantu, University of California, United StatesReviewed by:
Walter Chitarra, Council for Agricultural and Economics Research, ItalyAndrés Gárriz, CONICET Institute of Biotechnological Research, (IIB-INTECH), Argentina
Copyright © 2019 Pacifico, Squartini, Crucitti, Barizza, Lo Schiavo, Muresu, Carimi and Zottini. This is an open-access article distributed under the terms of the Creative Commons Attribution License (CC BY). The use, distribution or reproduction in other forums is permitted, provided the original author(s) and the copyright owner(s) are credited and that the original publication in this journal is cited, in accordance with accepted academic practice. No use, distribution or reproduction is permitted which does not comply with these terms.
*Correspondence: Francesco Carimi, francesco.carimi@ibbr.cnr.it