- 1Department of Agriculture, Food and Environment, University of Pisa, Pisa, Italy
- 2Institute of Integrative Biology, ETH Zürich, Zürich, Switzerland
- 3Department of Plant and Microbial Biology, University of Zürich, Zürich, Switzerland
- 4International Clinical Research Centre, St. Anne’s University Hospital, Brno, Czechia
- 5Central European Institute of Technology, Brno University of Technology, Brno, Czechia
- 6Department of Agraria, University Mediterranea of Reggio Calabria, Reggio Calabria, Italy
- 7Institute of Research on Terrestrial Ecosystems, National Research Council, Pisa, Italy
Tomato landraces, originated by adaptive responses to local habitats, are considered a valuable resource for many traits of agronomic interest, including fruit nutritional quality. Primary and secondary metabolites are essential determinants of fruit organoleptic quality, and some of them, such as carotenoids and phenolics, have been associated with beneficial proprieties for human health. Landraces’ fruit taste and flavour are often preferred by consumers compared to the commercial varieties’ ones. In an autumn-winter greenhouse hydroponic experiment, the response of three Southern-Italy tomato landraces (Ciettaicale, Linosa and Corleone) and one commercial cultivar (UC-82B) to different concentrations of sodium chloride (0 mM, 60 mM or 120 mM NaCl) were evaluated. At harvest, no losses in marketable yield were noticed in any of the tested genotypes. However, under salt stress, fresh fruit yield as well as fruit calcium concentration were higher affected in the commercial cultivar than in the landraces. Furthermore, UC-82B showed a trend of decreasing lycopene and total antioxidant capacity with increasing salt concentration, whereas no changes in these parameters were observed in the landraces under 60 mM NaCl. Landraces under 120 mM NaCl accumulated more fructose and glucose in the fruits, while salt did not affect hexoses levels in UC-82B. Ultra-performance liquid chromatography–tandem mass spectrometry analysis revealed differential accumulation of glycoalkaloids, phenolic acids, flavonoids and their derivatives in the fruits of all genotypes under stress. Overall, the investigated Italian landraces showed a different behaviour compared to the commercial variety UC-82B under moderate salinity stress, showing a tolerable compromise between yield and quality attributes. Our results point to the feasible use of tomato landraces as a target to select interesting genetic traits to improve fruit quality under stress conditions.
Introduction
Tomato is the most consumed berry fruit worldwide as well as one of the most important constituents of the Mediterranean diet representing a key source of minerals, vitamins and antioxidants (Canene-Adams et al., 2005). Fruit quality is affected by environmental conditions, such as seasonal changes, the occurrence of biotic/abiotic stress and agronomic practices (water management and fertilizer supply), as well as genetic factors (Poiroux-Gonord et al., 2010), but their mechanism of action is not completely clear. To enhance health-related compounds, different agronomic strategies have been applied, namely grafting (Sánchez-Rodríguez et al., 2012; Casals et al., 2018) or controlled water management techniques (Barbagallo et al., 2008).
Salinity induces changes in physiology and metabolism that affect the final crop yield (Pompeiano et al., 2016). Tomato is generally considered a moderately salt-tolerant crop, often cultivated in areas polluted by salinization of aquifers and consequent use of saline water for irrigation (Santa-Cruz et al., 2002). Salinity can positively modulate tomato fruit metabolism and improves the sensorial/nutritional value of the production (D’Amico et al., 2003). Salinity can increase the total soluble content (°Brix) and the titratable acidity, two important parameters influencing the quality of tomato fruits. Moreover, a high salt concentration in irrigation water generally stimulates the defence system of the plant, thereby leading to accumulation of secondary metabolites in different tissues. One common feature of plant secondary compound classes, such as carotenoids, polyphenols and terpenoids, is reactive oxygen species (ROS) scavenging activity (Ndhlala et al., 2010). Due to their strong antioxidant activity, these bioactive metabolites have been recognized as beneficial players against human cardiovascular and chronic degenerative diseases (Borguini and da Silva Torres, 2009) and tumours (Barone et al., 2018). High salinity can accelerate lycopene biosynthesis in hydroponically-grown tomato plants (Wu and Kubota, 2008). Several water management techniques applying controlled and moderate drought/salt stress in the pre-harvesting period of tomato fruits have been implemented to maintain a sufficient yield and also to produce fruits with improved nutritional level (Kubota et al., 2012).
The diurnal and seasonal changes in light intensity, vapour pressure and temperature can also explain the differences observed between seasonal experiments (spring-summer vs autumn-winter) and cultivation systems (open-field vs greenhouse) (Incerti et al., 2009; Asensio et al., 2019). Yield and quality of tomato fruits from off-season greenhouse cultivation are often reduced compared to open-field production (Hu et al., 2006). This effect depends on the association of the different climatic conditions with the covering materials used in the protected environment—that can deplete the intensity and the quality of the light spectrum inside the greenhouse (Toor et al., 2006; Gent, 2007; Mariz-Ponte et al., 2019). Light quality and intensity are indeed major constraints influencing quality parameters in tomato fruit (Slimestad and Verheul, 2005). Generally, °Brix and titratable acidity are positively correlated with increasing light intensity (Claussen et al., 2006) and temperature (Adams et al., 2001). High light intensity and the modulation of UV-B in the light spectra enhance flavonoid accumulation in tomato fruit tissues. Low night temperature, which often occurs in non-heated greenhouses during winter, drastically affects plant growth and crop yield (Jing et al., 2016).
The genetic background is a critical factor that can significantly influence fruit quality (Steward et al., 2000; Vallverdú-Queralt et al., 2011). In the present work the response to salt stress of long-storage tomato landraces (or traditional varieties) were assessed. In particular, the effects of moderate and high concentrations of sodium chloride (60 and 120 mM NaCl) on yield and quality-related fruit metabolites were evaluated on three Italian tomato landraces from different geographic origin (Ciettaicale, Corleone and Linosa). These landraces are traditionally used to prepare fresh sauce or dried fruits stored in olive oil. A tomato ancient variety (UC-82B) was included in the experiment as commercial control. According to our preliminary screening of a tomato landrace collection, the local accessions selected for this study represented promising candidates for traits related to abiotic stress tolerance, which are notably found in other Mediterranean tomato landraces (Galmes et al., 2013; Patanè et al., 2016). Indeed, compared with other processing tomato genotypes, Ciettaicale previously showed an interesting tolerance profile to salt and drought stress at vegetative stage (Moles et al., 2016; Moles et al., 2018), whereas Linosa exhibited a high nitrogen use efficiency (Abenavoli et al., 2016; Lupini et al., 2017). These promising findings prompted to carry out experiments in autumn-winter off-season climatic conditions, which allowed us to adopt a hydroponic irrigation system with different salt concentrations (60 and 120 mM NaCl can be considered as 10% and 20% seawater dilutions, respectively) that normally are considered high for a moderate salt-sensitive crop such as tomato (Cuartero et al., 2006). This information is essential for selecting potential metabolic traits to be used as biomarkers on which to focus for new breeding strategies.
Materials and Methods
Plant Material and Growth Condition
Three Southern Italy tomato landraces and a standard tomato variety were used as genetic material in this study. Among the landraces, Ciettaicale (from Basilicata region) and Linosa (from Pelagic Islands, Sicily) belong to the category of tomatoes with indeterminate growth habit and with pear/globose fruits, whereas Corleone (from Sicily region) is an indeterminate tomato type with flattened/ribbed fruits. The commercial variety UC-82B (supplied by the Tomato Genetics Resource Center, Department of Plant Sciences, University of California-Davis, CA, USA) belongs to the category of determinate tomatoes with pear/globose fruits. Plants were grown in rockwool cube (Rockwool B.V., The Netherlands), in an open nutrient solution system at a plant density of approximately 3 m−2 in a glasshouse at the University of Pisa (Italy) from September 2016 to February 2017. The plants (16 individuals for each of the 4 genotypes for each of 3 experimental conditions, divided in eight pairs which were arranged in a randomized block design on the glasshouse benches, for a total of 192 plants) were grown vertically trimming the plant below the fourth fruit truss. Climatic parameters were continuously monitored by means of a weather station located inside the glasshouse. The mean air temperature and relative humidity were 17.3°C and 74.6%, respectively (Tmin = 11.4°C and Tmax = 27.7°C; RHmin = 50.2% and RHmax = 96.7%). Mean values of daily inside global radiation was 3.44 MJm−2 (GRmin = 1.02 MJm−2 and GRmax = 10.30 MJm−2). Two salinity levels of nutrient solution were used with electrical conductivities (EC) of 8.3 and 14.6 mS cm−1, which corresponded roughly to 60 and 120 mM NaCl, respectively. The concentration of nutrient solution was as reported by Incerti et al. (2007). Salt stress was applied 3 weeks after planting; the process was stepped up in roughly 2.1 mS cm−1 (20 mM NaCl) daily increments to avoid osmotic shock. Irrigation was controlled by a timer that opened the irrigation lines for 1 min up to 12 times per day, depending on growing stage and environmental conditions.
Biometrical Measurements, Fruit Yield and Sampling
Fresh fruit yield (FW) was determined based on total fruits picked two times per week in February from all the three trusses of each plant and genotype under different experimental treatments. The red-ripe fruits from the second truss (which consistence was evaluated by penetrometer) were separately collected early in the morning, weighted and randomly grouped in six biological replicates (15-20 whole fruits composed a single biological replicate) to be ground altogether at 4°C (homogenate) and then used for laboratory determinations. An aliquot of the homogenate was added to the remaining harvested material and stored at 80°C for 2 weeks to record fruit dry weight (DW). Aliquots of the homogenate were separately stored and placed at 80°C for 1 week for cation quantifications; additional aliquots were used to determine °Brix and titratable acidity (Beckles, 2012); finally, other samples were collected in tubes and stored at -80°C for further metabolic analyses (per biological replicate, four technical replicates were analysed). At the end of experiment, leaf area was estimated using a digital planimeter; then, shoot organs (leaves and stems) were weighted (shoot FW) and put in 80°C for 2 weeks to record the dry weight (shoot DW).
Total Soluble Sugar Measurements
Glucose, fructose and sucrose were extracted from tomato fruit homogenate aliquots according to the protocol described in Hostettler et al. (2011), and then quantified enzymatically according to Thalmann et al. (2016).
Cation Determination
Fruit dried samples were powdered and mineralized (60 min at 220°C) using a solution of HNO3:HClO4 (2.5:1, v/v). Sodium (Na+), potassium (K+) and calcium (Ca2+) were determined using an atomic absorption spectrometer (Varian AA 24FS, Australia).
Lycopene Determination
Lycopene content was assayed according to Giovannetti et al. (2012). Briefly, an aliquot of tomato fruit homogenate was extracted in a solution of acetone:ethanol:hexane (1:2:1, v/v/v) and agitated on an orbital shaker for 15 min. Then one volume of distilled water was added, followed by 5 min agitation. After centrifugation, the hexane phase was measured at 503 nm, blanked with pure hexane.
Total Flavonoids, Total Phenols and Total Antioxidant Activity Contents
Tomato fruit homogenates were mixed in 70% (v/v) methanol and agitated overnight at 4°C in the dark. After incubation, the extracts were centrifuged at 12000 x g for 15 min at 4°C and the supernatants were utilized for the analyses indicated below. Total soluble phenols content (TPHE) and total flavonoids content (TFL) were assayed using the respective protocols reported in Caser et al. (2016). Total antioxidant capacity (TAC) was determined by the 2,2-diphenyl-1-picrylhydrazyl (DPPH) assay as previously reported in Moles et al. (2016), with some modifications. Briefly, an aliquot of the methanolic fruit extract was added to 0.1 mM methanolic DPPH solution. After 30 min of incubation at room temperature in the dark, absorbance was measured at 515 nm, and the results were expressed as µmol of Trolox per gram of plant material on dry basis.
Metabolite Profiling
Tomato fruit homogenate (100 mg) was extracted with 100% methanol. The samples were ground using a mixer mill with 1.25–1.65 mm glass beads for 1.5 min at 30 Hz, centrifuged at 15,000 × g at 4°C and the supernatants collected. All samples were de-salinized over a silica-based classic cartridge (WAT051910, Waters) according to the manufacturer instructions. The eluted samples were concentrated using a Savant SpeedVac concentrator (Thermo Fisher Scientific) at 42°C. Prior to ultra-performance liquid chromatography–tandem mass spectrometry (UPLC-MS/MS) analysis the samples were re-suspended in 80% methanol, 0.1% formic acid. After sonication for 5 min, the samples were centrifuged at 15,000 × g, at 4°C for 5 min, and transferred to liquid chromatography vials. Samples were analysed on a UPLC (Dionex UltiMate 3000, Thermo Fisher Scientific) coupled to a Bruker compact electrospray ionisation (ESI)-quadrupole-time-of-flight tandem-mass spectrometer (Bruker Daltonics). The UPLC separation was performed at 28°C with a C18 reverse-phase column (ACQUITY UPLC™ BEH C18, 1.7 µm, 2.1 × 150 mm, Waters) using the following gradient of solvent B [acetonitrile with 0.1% (v/v) formic acid] and solvent A [water with 0.1% (v/v) formic acid]: 0–0.5 min, 5% B; 0.5–12 min, 5–100% B; 12–14 min, 100% B; 14–16 min, 100–5% B. The flow rate was set to 0.3 mL min−1 and 5 µl of each sample was injected. The ESI source was operated in positive mode and parameters were set as follows: gas temperature, 220°C; drying gas, 9 L min−1; nebuliser, 2.2 bar; capillary voltage, 4,500 V; end plate offset, 500 V. The instrument was set to acquire an m/z range of 50–1,300. Conditions for MS/MS were set as described by Christ et al. (2016). All data were recalibrated internally using pre-run injection of 10 mM sodium hydroxide in 0.2% formic acid, 49.8% water, 50% isopropanol (v/v/v). DataAnalysis v.4.2 and TargetAnalysis v.1.3 softwares (Bruker Daltonics) were used to analyse the data. Metabolites were identified and annotated by comparison with MS and MS/MS data spectra either generated by authentic reference standards or deposited in the literature and databases, such as PubChem and MoTo (Moço et al., 2006).
Statistical Analysis
A randomized block design as previously described was performed. Data were subjected to two-way analysis of variance (ANOVA) and the mean values were compared using Duncan’s test (P < 0.05) to check the significant differences. For the metabolomics data, in order to examine the differences between the treatments, the percentage contribution of each compound to the average dissimilarity between the aforementioned factors was calculated using similarity percentage analysis (SIMPER) (Vaníčková et al., 2015). A cut-off was imposed where ∑δi% reached 70%. Also, the differences in the chemical composition and extra characteristics of the samples from the study were analysed by principal component analysis (PCA). Prior to PCA, peak areas were subjected to logarithmic transformation; intraspecific scaling was performed by dividing each treatment/experimental condition score by its standard deviation; the data were centred by treatment scores. In PCA analyses, hierarchical clustering based on Pearson correlation showed that treatments with similar chemical profiles cluster together. All computations were performed with R 3.5.3 language and environment (R Core Team, 2019) and the R packages FactoMineR (Le et al., 2008) and vegan (Oksanen et al., 2019).
Results
We assessed the effects of moderate and high salt treatments (60 and 120 mM NaCl) applied during an off-season greenhouse experiment, thereby evaluating yield and quality-related fruit metabolites in three Italian tomato landraces (Ciettaicale, Corleone and Linosa) and in a commercial variety (UC-82B). Analysis of all the biometric and metabolic traits revealed a significant (P < 0.05) genotype × treatment interaction. Following that, subsequent data were presented in treatment combinations.
Biometrical Measurements and Fruit Yield
To investigate whether (or how) salinity stress impacted on leaf growth, we measured leaf area and epigeal biomass at the end of the experiment. In the landraces leaf area did not decrease upon treatment with 60 mM NaCl (Supplementary Figure 1A), while a significant reduction in this parameter was recorded under 120 mM NaCl, particularly in Ciettaicale (approximately 46% smaller than its control). By contrast, the commercial variety UC-82B decreased leaf area about 30% and 60% under 60 and 120 mM NaCl, respectively. However, a common reduction in the vegetative biomass (shoot FW and DW) was recorded in all the tomato genotypes comparing to their respective controls (Supplementary Figures 1B, C).
Although salinity treatments differently affected fruit size (Figure 1 and Table 1), the number of fruits was unaffected (Table 1). Ciettaicale and UC-82B already reduced fruit yield FW under 60 mM NaCl (Figure 2A). By contrast, Corleone and Linosa decreased fruit yield FW only under 120 mM NaCl by 47% and 20%, respectively. Moreover, Ciettaicale, Linosa and UC-82B did not exhibit significant differences when considering fruit yield DW (Figure 2B), except Corleone which significantly increased fruit yield DW under 60 mM NaCl (about 14%) and decreased under 120 mM Na Cl by 27% compared to the control.
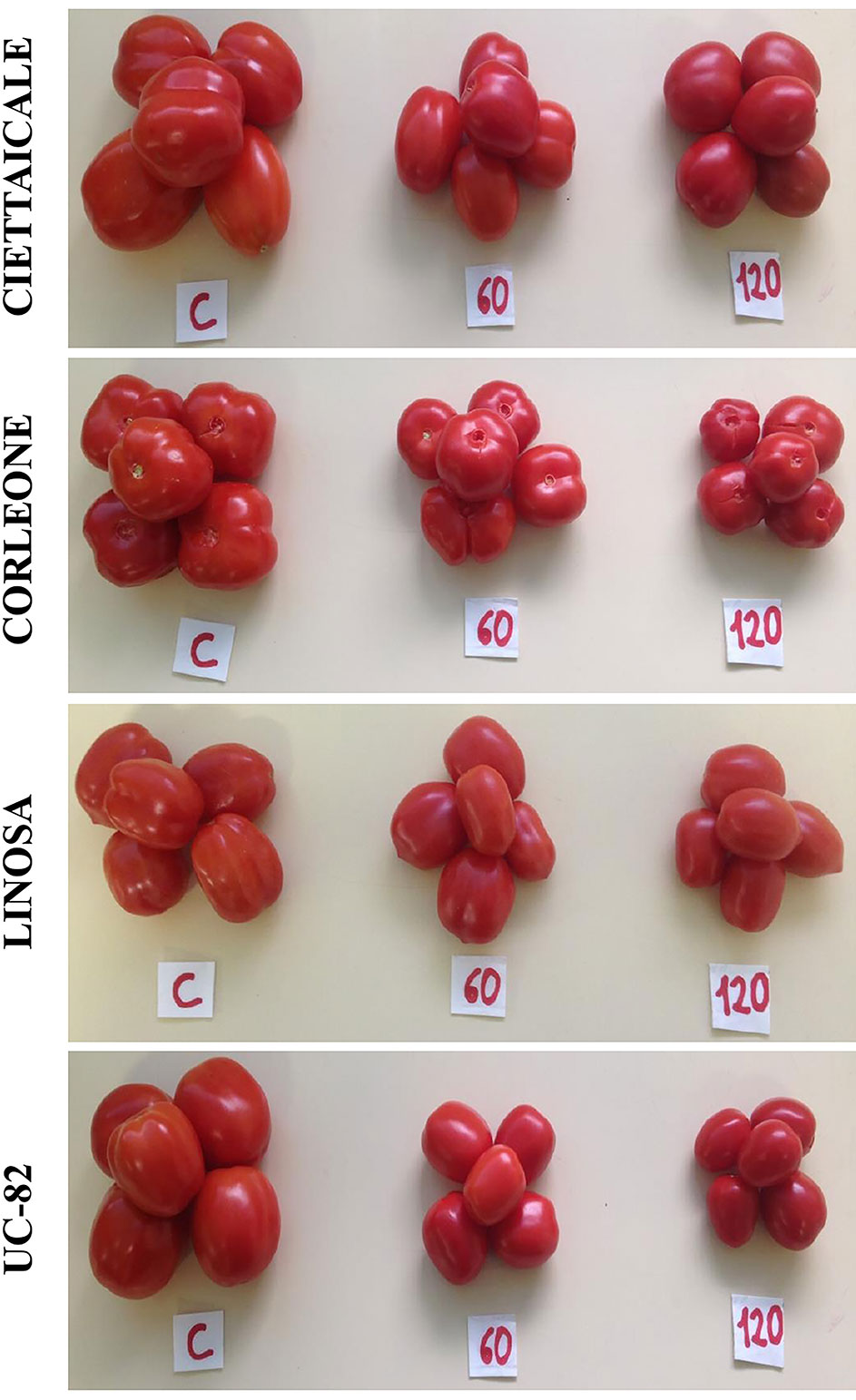
Figure 1 Effect of different salt concentrations (60 mM and 120 mM NaCl) on fruit size of three tomato landraces (Ciettaicale, Corleone and Linosa) and a commercial variety (UC-82B) compared to respective control condition (C).
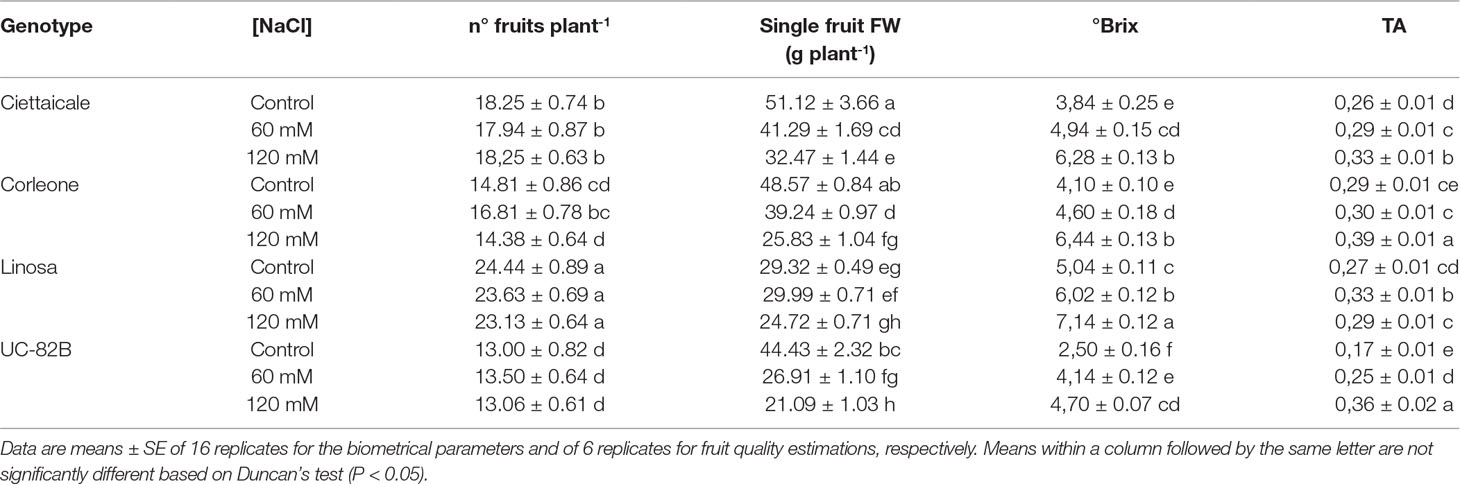
Table 1 Effect of different salt concentrations (60 mM and 120 mM NaCl) on number of fruits, single fruit fresh weight (FW), fruit total soluble solids (°Brix) and titratable acidity (TA) of three tomato landraces (Ciettaicale, Corleone and Linosa) and a commercial variety (UC-82B).
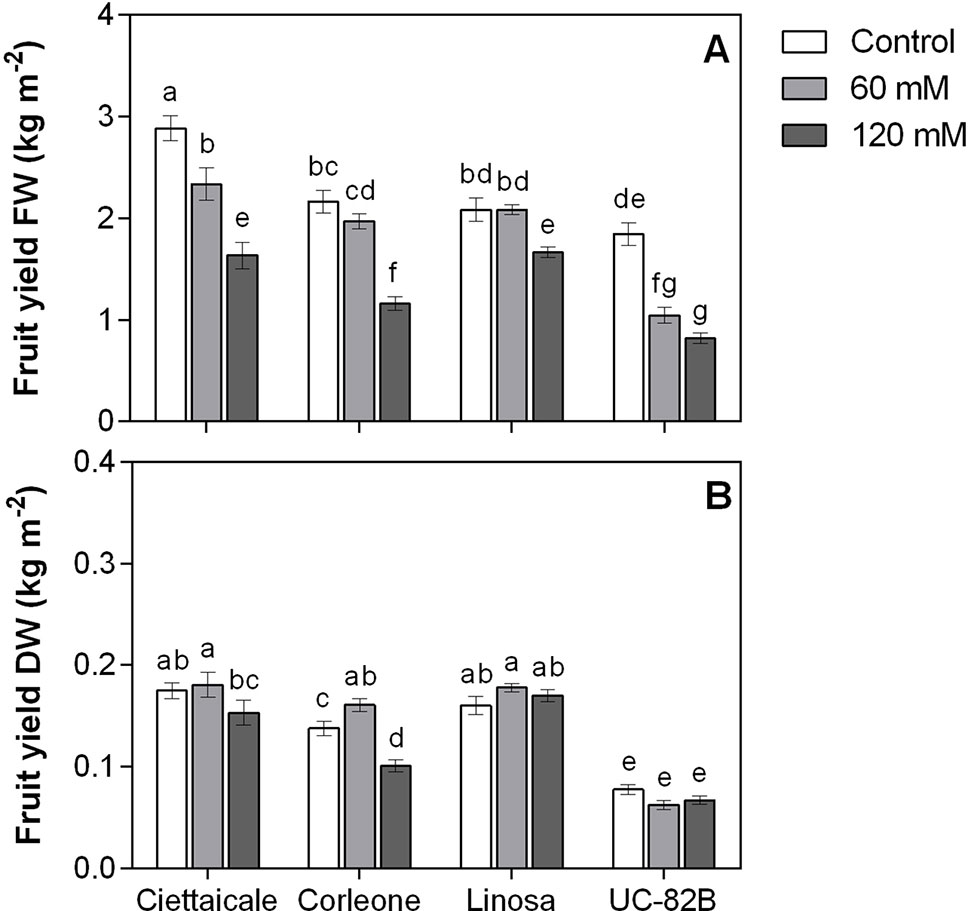
Figure 2 Effect of different salt concentrations (60 mM and 120 mM NaCl) on fruit yield of three tomato landraces (Ciettaicale, Corleone and Linosa) and a commercial variety (UC-82B). (A) Yield fresh weight (FW) and (B) yield dry weight (DW). Error bars represent the standard error of the mean (n = 16). Bars with same letters are not statistically different from one another according to Duncan’s test (P < 0.05).
Soluble Sugars Content
In all genotypes, 120 mM NaCl treatment caused significant increases in both fruit °Brix and titratable acidity (Table 1). We did not detect sucrose in fruit samples, but different trends in glucose and fructose contents among tomato genotypes were observed (Figure 3). Under 120 mM NaCl, landraces had increased glucose and fructose contents, while no significant changes in glucose and fructose levels were observed in the commercial variety under the salt treatment.
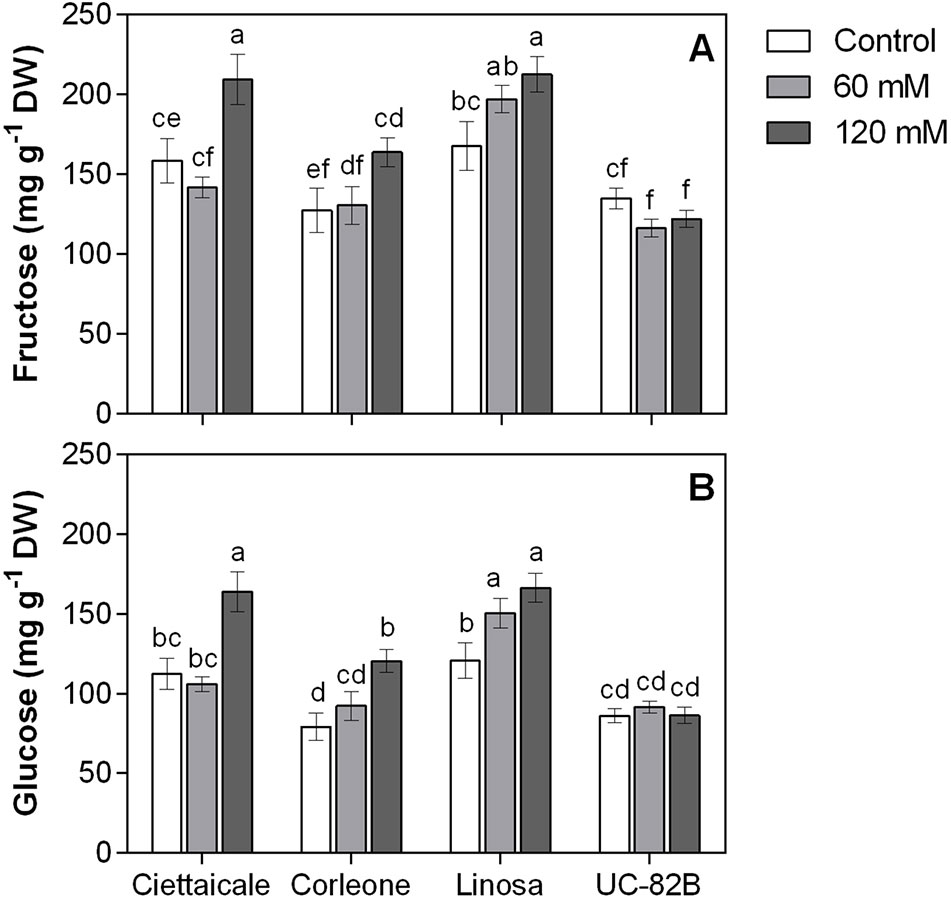
Figure 3 Effect of different salt concentrations (60 mM and 120 mM NaCl) on fruit hexose contents of three tomato landraces (Ciettaicale, Corleone and Linosa) and a commercial variety (UC-82B). (A) Fructose content and (B) glucose content. Error bars represent the standard error of the mean (n = 6). Bars with same letters are not statistically different from one another according to Duncan’s test (P < 0.05).
Cation Content
Salt treatments differently affected the fruit contents of Na+, K+ and Ca2+, depending on the genotype (Table 2). Ciettaicale showed the highest fold increase, reaching 3.4 fold more under 60 mM NaCl and 5.3 fold more under 120 mM NaCl compared to the control. Under 120 mM NaCl, the lowest Na+ concentration was found in Linosa fruits (2.5 fold more than the control), while the highest Na+ level was recorded in UC-82B (4 fold more than the control). Notably, Ciettaicale accumulated more K+ under both salinity treatments than in control, and more K+ was also found in Linosa under 60 mM NaCl. However, 120 mM salt induced a decrease of K+ concentration in Corleone and UC-82B. Commercial variety fruits showed reduced Ca2+ content already at 60 mM NaCl, while Linosa had less Ca2+ only under 120 mM compared to respective controls. Overall, Linosa maintained higher K+/Na+ and Ca2+/Na+ ratios at 60 mM and 120 mM NaCl compared to the other genotypes, while Ciettaicale and UC-82B more markedly decreased Ca2+/Na+ ratio with increasing salinity level.
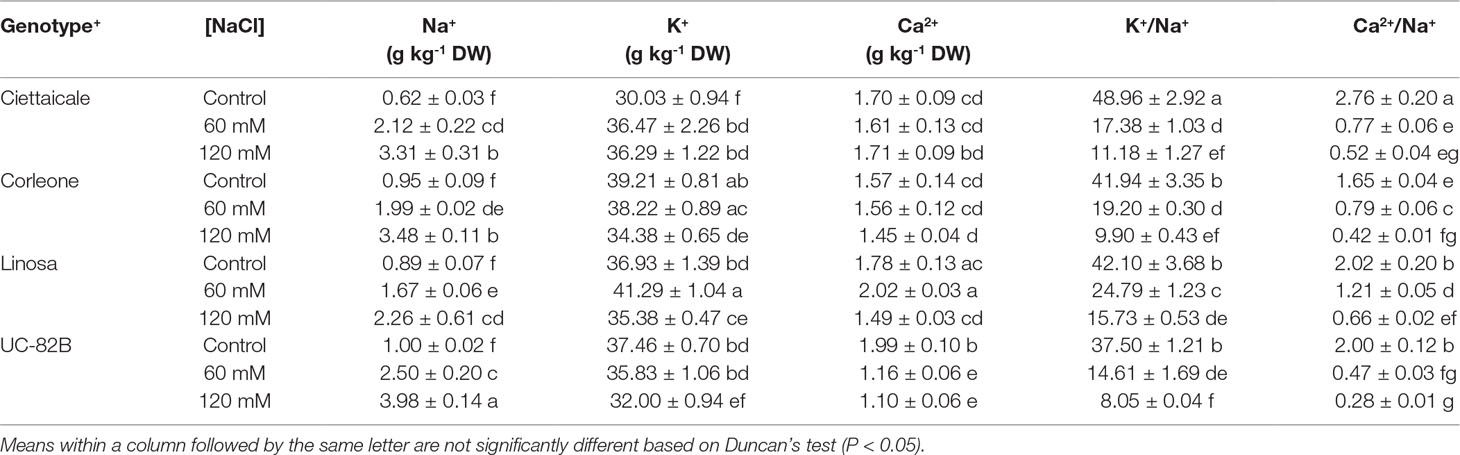
Table 2 Effect of different salt concentrations (60 and 120 mM NaCl) on fruit cation contents of three tomato landraces (Ciettaicale, Corleone and Linosa) and a commercial variety (UC-82B). Data are means ± SE of six replicates.
Lycopene
Landraces treated with 60 mM NaCl did not show changes in lycopene content (Figure 4). However, Corleone fruits under 120 mM NaCl contained about 40% less lycopene compared to controls. Notably, UC-82B in control conditions showed the highest values of fruit lycopene among the tomato genotypes, but exhibited a progressive decrease in lycopene content already at 60 mM NaCl (-38%) and more marked at 120 mM NaCl (-55%) compared to the control.
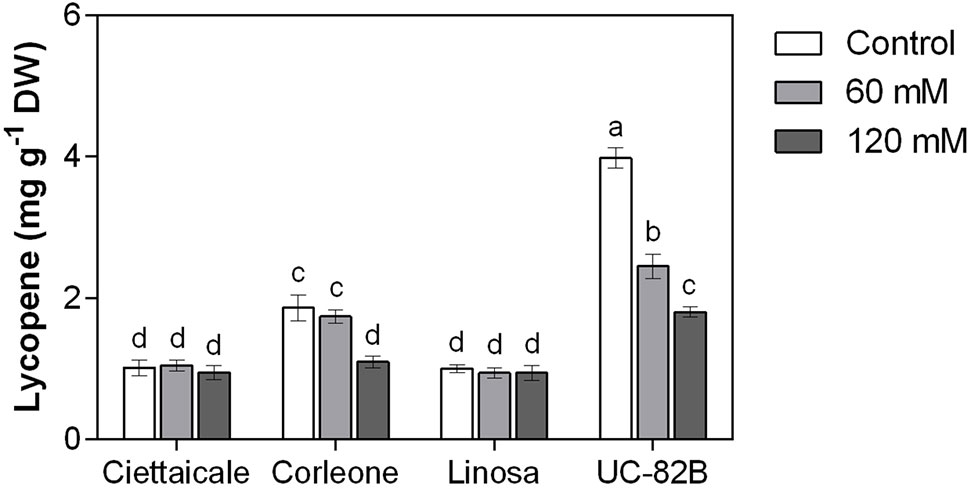
Figure 4 Effect of different salt concentrations (60 mM and 120 mM NaCl) on fruit lycopene content of three tomato landraces (Ciettaicale, Corleone, and Linosa) and a commercial variety (UC-82B). Error bars represent the standard error of the mean (n = 6). Bars with same letters are not statistically different from one another according to Duncan’s test (P < 0.05).
Total Flavonoids, Total Phenols, and Total Antioxidant Activity
Salt conditions did not affect TFL in Ciettaicale and Linosa (Figure 5A). An increase (+ 21%) in TFL was recorded in Corleone fruits under 120 mM NaCl compared to controls. Conversely, salinity negatively affected TFL in UC-82B. No differences in TPHE in the landraces under 60 mM NaCl were recorded (Figure 5B). Under the same stress condition, UC-82B reduced TPHE by around 29% compared to its control. The highest salt concentration caused a decrease in fruit TPHE with a similar magnitude in Corleone, Linosa and UC-82B compared to respective controls (-26%, -28% and -33%, respectively). At harvesting point, fruits of Ciettaicale and Linosa maintained roughly control TAC values under 60 mM NaCl (Figure 5C). Corleone and UC-82B had decreased TAC under 60 mM (-17% and -29% compared to their respective controls). However, a common reduction in TAC was observed in all genotypes under 120 mM compared to controls, i.e. from -17% in Linosa to -48% in UC-82B.
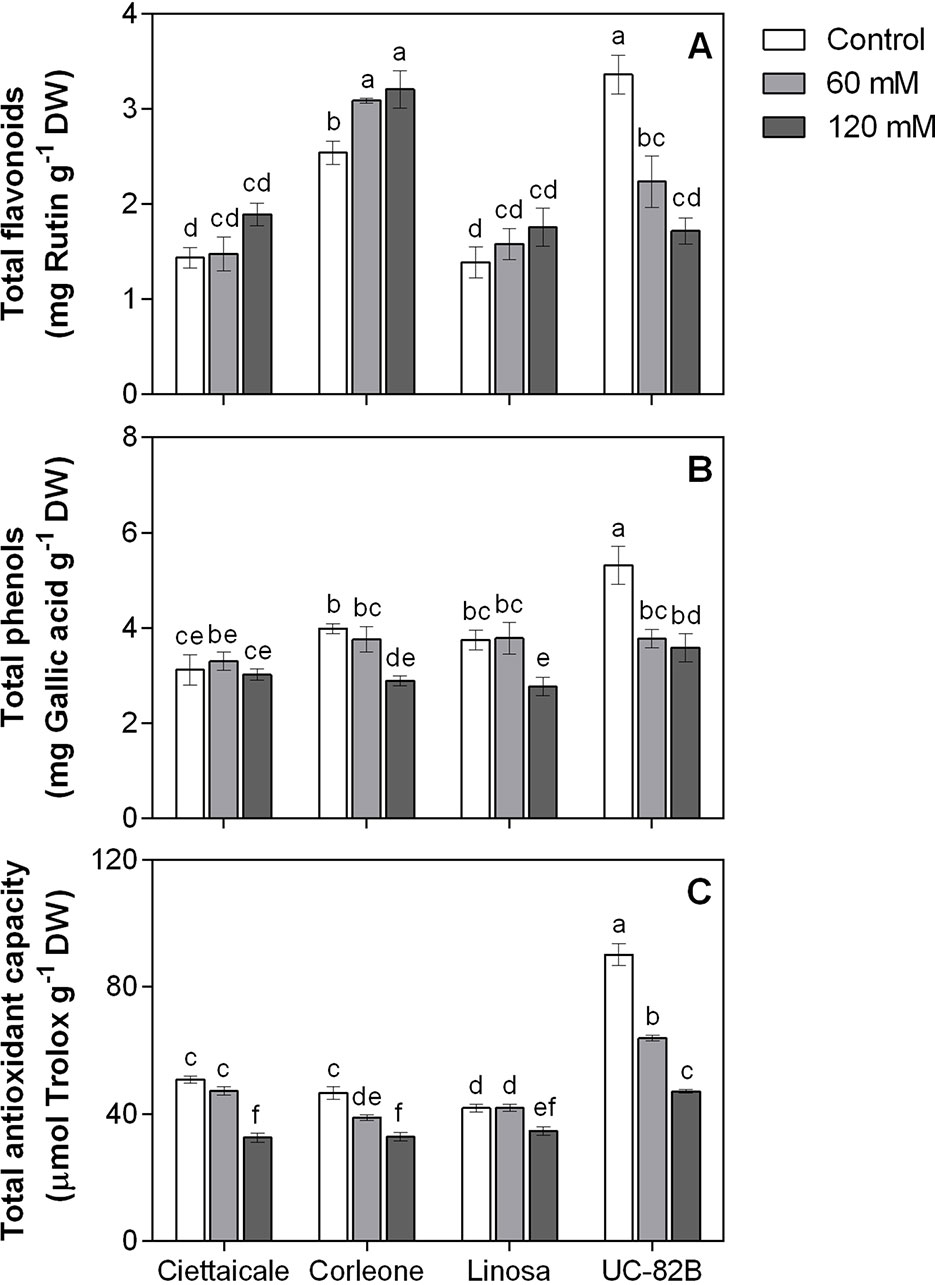
Figure 5 Effect of different salt concentrations (60 mM and 120 mM NaCl) on fruit phenolic content and antioxidant capacity of three tomato landraces (Ciettaicale, Corleone and Linosa) and a commercial variety (UC-82B). (A) Total flavonoids, (B) total phenols and (C) total antioxidant capacity. Error bars represent the standard error of the mean (n = 6). Bars with same letters are not statistically different from one another according to Duncan’s test (P < 0.05).
Metabolite Profiling
An untargeted UPLC-MS/MS analysis profiled the same sample sets as described above. We were able to identify 32 metabolites (Table 3) based on accurate mass measurements and MS/MS spectra from biological standards or publicly available data, namely literature and/or databases such as PubChem and Moto. ANOVA results are reported in Supplementary Table 1 and graphically represented in Figure 6. Most metabolites detected were phenylpropanoids (10 hydroxycinnamic acids and 7 flavonoids) and glycoalkaloids (8). The remainder metabolites were assigned as phenylamides (4), amino acids (2) and vitamins (1).
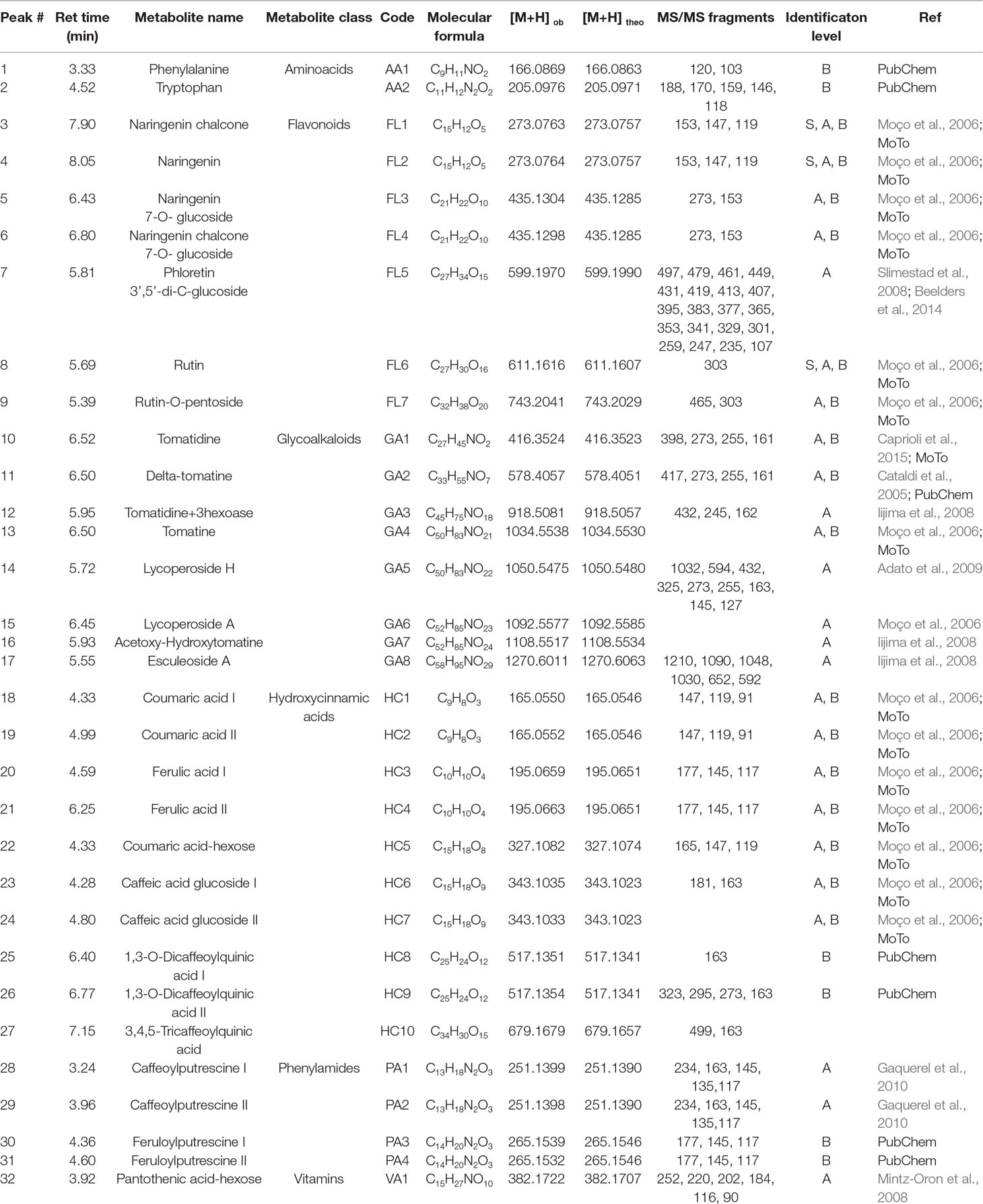
Table 3 UPLC-MS/MS analysis of plant specialized metabolites responsive to salinity stress found in this work. For the identification level: A, literature; B, database, S, standard.
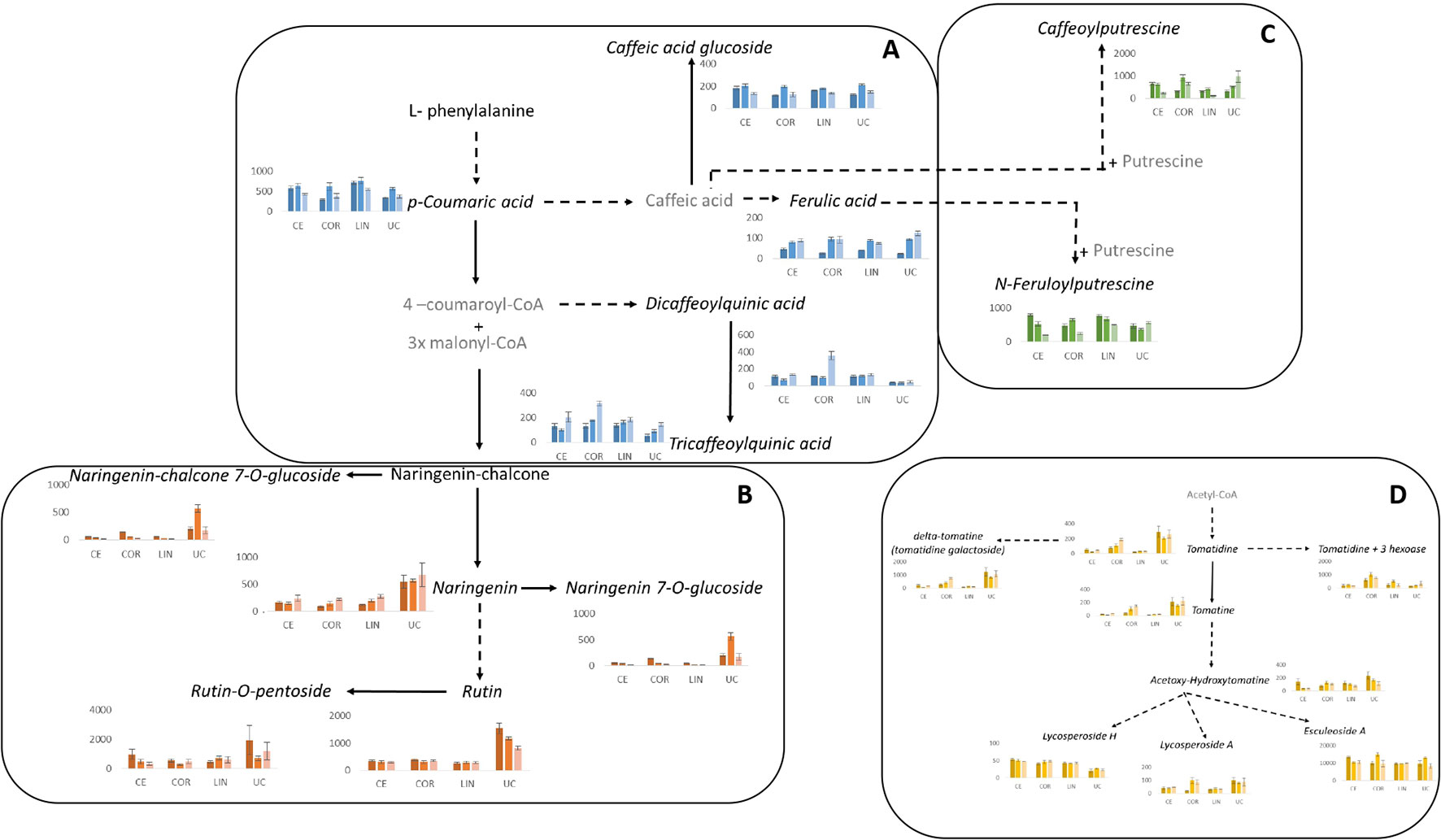
Figure 6 Pathways overview of the plant specialized metabolites responsive to salinity stress. (A) Hydroxycinnamic acids, (B) flavonoids, (C) phenylamides and (D) glycoalkaloids. Metabolites that were identified in the present study are represented in black, whereas non identified metabolites are in grey. Metabolites that showed statistically significant changes (genotype x salinity treatment) are represented in italic and graphical representations are presented. The graphs represent the mean of relative ion intensity/dry weight of six biological replicates ± SE. Dark, middle and light colours (blue, orange, green and yellow) represent control, 60 and 120 mM NaCl, respectively; CE, Ciettaicale; LIN, Linosa; COR, Corleone; UC, commercial variety UC-82B.
Among the hydroxycinnamic acids (Figure 6A), coumaric acid (detected as two isomers) was the compound that presented the most intense mass signal (Supplementary Table 1). We could observe only a significant decrease of coumaric acid II under 120 mM NaCl in UC-82B. Interestingly, a similar trend was observed for coumaric acid-hexose accumulation in Linosa. Two ferulic acid isomers were detected: ferulic acid I significantly increased in all genotypes under salt stress compared to control conditions, whereas ferulic acid II did not significantly change in the case of Ciettaicale and Corleone upon salt stress. Linosa and UC-82B displayed a similar trend of reduction of the intensity of ferulic acid II mass signal upon 120 mM NaCl. The 1,3-O-dicaffeoylquinic acid I showed a remarkably low mass signal intensity in UC-82B fruits as compared to the landraces. At 120 mM NaCl, Corleone fruits showed a 3 fold increase of 1,3-O-dicaffeoylquinic acid I mass signal intensity. Also, 3,4,5-tricaffeoylquinic acid showed a significant increase in mass signal intensity in Corleone fruits upon 120 mM NaCl.
Apart from hydroxycinnamic acids, the phenylamides conjugated with caffeic acid (caffeoylputrescine I and II isomers) and ferulic acid (feruloylputrescine I and II isomers) were also identified (Figure 6C and Supplementary Table 1). Caffeoylputrescine I was significantly reduced in Ciettaicale and Linosa upon 120 mM NaCl, whereas the same salt concentration induced an increase of this compound in UC-82B fruits. Feruloylputrescine I was significantly reduced under 120 mM NaCl for the three landraces. Feruloylputrescine II increased in Corleone and UC-82B fruits upon salt stress, but in Linosa fruits the opposite trend was observed.
Regarding the flavonoids identified, rutin was the compound that exhibited the most intense mass signal (Figure 6B and Supplementary Table 1). Interestingly, UC-82B fruits showed the highest signal for all the flavonoids detected. Nevertheless, rutin and rutin-O-pentoside did not significantly change upon salt stress in all landraces, whereas UC-82B fruits showed a significant decrease of rutin upon 60 and 120 mM NaCl.
The list of identified compounds also includes 8 glycoalkaloids that presented a variable accumulation pattern in the studied conditions (Figure 6D and Supplementary Table 1). Esculeoside A was the glycoalkaloid that showed the highest mass signal intensity. Under control conditions, Ciettaicale accumulated significantly higher amounts of esculeoside A than all the other genotypes, while 60 mM NaCl promoted its accumulation in Corleone and UC-82B fruits. Under 120 mM NaCl all genotypes accumulated similar levels of esculeoside A. Tomatidine content was significantly higher in the commercial variety and Corleone under 120 mM NaCl, when compared to Ciettaicale and Linosa. Tomatidine+3hexoase showed the highest mass intensity signal in Corleone fruits upon 60 mM NaCl treatment. Tomatine, delta-tomatine and lycosperoside A were significantly high in the commercial variety in all conditions and in Corleone upon 120 mM NaCl. On the other hand, lycoperoside H was significantly higher in all landraces compared to UC-82B variety, and its content was not modulated by salt treatment.
We also identified the amino acids phenylalanine and tryptophan, and the vitamin derivative, pantothenic acid–hexose (Supplementary Table 1). Phenylalanine showed a marked increase in mass signal intensity upon 60 mM NaCl treatment in Ciettaicale fruits, whereas for the other genotypes the levels remained unchanged. The lowest mass signal intensity of tryptophan was observed in Corleone at 120 mM NaCl treatment, whereas the other genotypes showed the same mass signal intensity for this compound. Finally, the levels of pantothenic acid-hexose were high upon 60 mM NaCl in all genotypes except for Linosa, which showed unaltered levels of this metabolite in all treatments.
Moreover, the UPLC-MS/MS data were used in a further analysis known as SIMPER (Supplementary Table 2). The objective of this analysis was to find key compounds that allow for differentiation of one experimental condition from another when compared in a pair-wise analysis. The results display the contribution of each compound to the average overall dissimilarity of the two compared samples. A cut-off is imposed when ∑δi% reaches 70%. The metabolomic profiles of the experimental treatments differed qualitatively. In the first analyses, we identified the compounds that were consistently present in all the three salinity comparisons (control vs 60, control vs 120, and 60 vs 120), regardless of the genotypes. Among them, comparing conspecific different salinity treatments among all the genotypes, we identified the persistence of seven compounds: feruloylputrescine II (PA4), caffeoylputrescine I (PA1), naringenin (FL2), rutin-O-pentoside (FL7), naringenin chalcone (FL1), esculeoside A (GA8) and tomatidine+3hexoase (GA3).
The PCA (Figures 7A, B) performed on metabolomics data showed that the first two dimensions (PC1 and PC2) account for 47.5% of the total variance (total inertia). The first axis (PC1) explains 30.9% of the total variance and the second axis (PC2) 16.6%. The contribution of individual compounds to sample differentiation is displayed as a correlation circle (Figure 7A) where normalized vectors graphically represent the quantitative variables. The length and the direction of the vectors directly correlate with their significance within each treatment. A positive correlation between compounds is greater when the angle between their directions is smaller (close to 0 degree), whereas the correlation is negative if the angle reaches 180 degrees. No linear dependence exists if the angle is exactly 90 degrees. Overall, in our dataset we observed strong positive correlation between caffeic acid glucoside II (HC7) and coumaric acid II (HC2), caffeoylputrescine I (PA1) and caffeoylputrescine II (PA2), and 1,3-O-dicaffeoylquinic acid II (HC9), 3,4,5-tricaffeoylquinic acid (HC10) and phenylalanine (AA1). From the spatial distribution of the treatments (Figure 7B), we observed that Corleone distinguished itself the most. At 60 mM NaCl, Corleone showed a distinct metabolomic profile, while it shared more similarities under control conditions and at 120 mM NaCl. Ciettaicale and Linosa clustered separately but formed one cluster regardless of the salinity level. Furthermore, the commercial variety UC-82B under 120 mM NaCl showed a distinct profile compared to the control and mild salinity level. In the PCA analysis performed on the biochemical and biometric characteristics (Figures 7C, D), the landraces segregated into two main groups mainly according to the salinity level, whereas the standard variety clustered separately. In particular, untreated UC-82B was uniquely distinct, while the salt-treated ones grouped together forming one cluster.
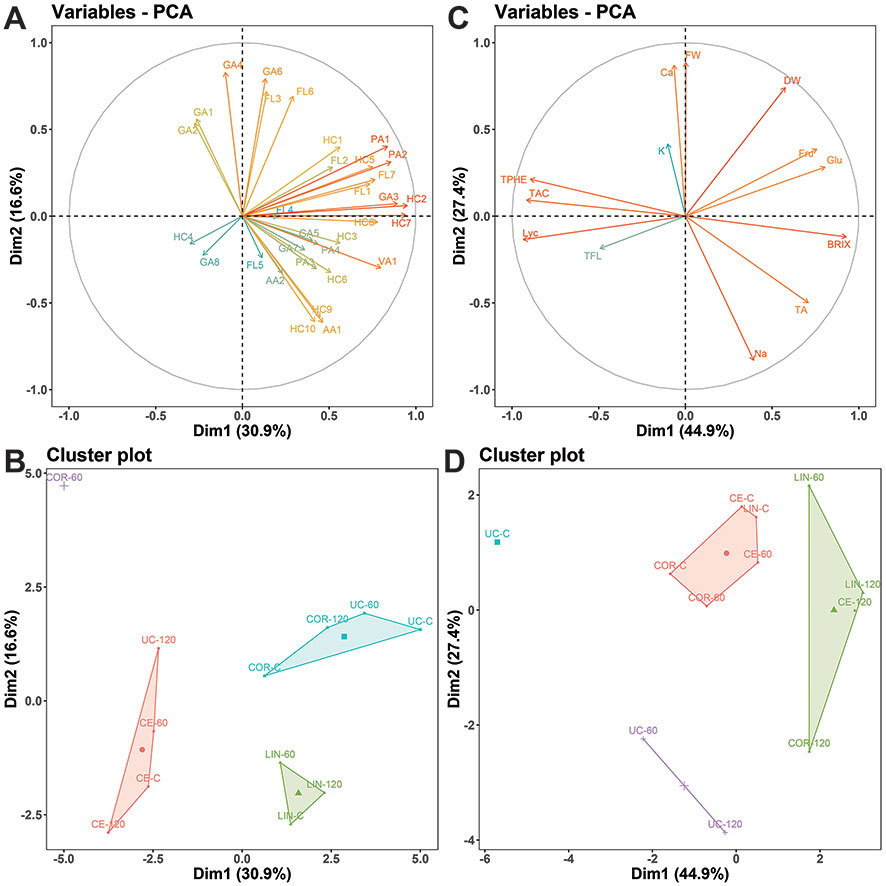
Figure 7 Graphical representations of Principal Component Analysis (PCA) results. (A and C) Vector representation of the contribution of each metabolite identified by UPLC-MS/MS (A) and each laboratory determination/assay (C) to the distinction of experimental treatments. The coordinates of each variable are the correlation coefficients with the two-first principal components. Between vectors and between a vector and an axis, there is positive correlation if the angle is less than 90 degrees whereas the correlation is negative if the angle reaches 180 degrees. There is no linear dependence if the angle is 90 degrees. (B and D) Cluster map of PCA results of UPLC-MS/MS data (B) and laboratory determinations/assays (D) obtained from experimental treatments. For UPLC-MS/MS metabolite codes see Table 3; FW, yield fresh weight; DW, yield dry weight; Na, sodium; K, potassium; Ca, calcium; Fru, fructose; Glu, glucose; BRIX, total soluble solids; TA, titratable acidity; LYC, lycopene; TPHE, total phenols; TFL, total flavonoids; TAC, total antioxidant capacity; CE, Ciettaicale; COR, Corleone; LIN, Linosa; UC, commercial variety UC-82B; C, control condition; 60 and 120 mM NaCl used as experimental treatments, respectively.
Discussion
Tomato landraces are a valuable resource for many traits of agronomic interest. This is mostly due to their resilience against abiotic stresses, which contributes to yield stability and adaptation to low input and/or adverse growth conditions. Landraces are also associated with distinctive organoleptic and nutritional quality traits and could exhibit peculiar and often contrasting metabolic profiles (Baldina et al., 2016; Gascuel et al., 2017; Patanè et al., 2017; Siracusa et al., 2018). High contents in functional compounds are frequent traits found in Mediterranean traditional tomato varieties (Pinela et al., 2012; Berni et al., 2018), but often no differences in sensory profile have been identified between commercial varieties and landraces (Ruiz et al., 2005; Sinesio et al., 2007; Casals et al., 2011). Moreover, the promoting effect on fruit quality metabolites is frequently ascribed to the concentration effect and not to the absolute accumulation (Zushi and Matsuzoe, 2015). However, different studies consistently concluded that the interaction between genotype and environment is the key component able to modulate the expression of specific metabolic patterns (Berni et al., 2019).
In this study we compared the effects of moderate and high concentrations of sodium chloride on yield and quality-related ions and metabolites in three tomato landraces (Ciettaicale, Corleone and Linosa) and a commercial variety (UC-82B). We showed that salinity promoted the anticipation of fruit ripening in all genotypes, but differentially caused fresh fruit yield losses. Notably, at 60 mM NaCl all landraces showed better performance in terms of yield FW compared to the commercial variety. The most interesting results were the absence of yield loss in Corleone and Linosa at the aforementioned salinity level and the observation that Linosa reduced only around 20% fresh fruit production under 120 mM NaCl. The capacity to maintain an adequate yield has also been found in a Kenyan tomato landrace grown in site soil polluted by high salty water irrigation (Agong et al., 1997).
Yield and functional quality traits can be influenced by salinity mainly due to sodium competition for other cations, such as K+ and Ca2+ (Adams, 1991; Petersen et al., 1998, Pompeiano et al., 2017). Among the genotypes, Linosa maintained higher K+/Na+ and Ca2+/Na+ ratios along the salt gradient. Calcium participates both in the alleviation of sodium toxicity and in the fruit size development (Plieth, 2005; Manaa et al., 2013). This observation could support the ability of Linosa to maintain an adequate yield under salt stress. Additionally, fresh tomato fruits with high Ca2+ content represent a natural mineral supply indispensable in human dietary (Soetan et al., 2010). On the contrary, in the commercial variety the Ca2+/Na+ ratio was more affected by the salinity gradient, leading to a more marked reduction of fruit size and weight. Several studies reported that calcium deficiency affects tomato fruit development (Park et al., 2005), often resulting with the appearance of the blossom-end rot (Taylor and Locascio, 2004). However, in the present study, as well as in the winter greenhouse experiment conducted by Zushi and Matsuzoe (2009), the fruits of all genotypes were not affected by this marketable injury.
The increases in soluble solids and titratable acidity are common responses of tomato fruits under salt stress (Balibrea et al., 2003; Zushi and Matsuzoe, 2015). High values of these parameters have been found in Spanish tomato landraces under salt stress (Massaretto et al., 2018). Soluble solids content (°Brix) mainly estimated the sugar amounts in tomato fruit pulp, but also organic acids, amino acids, soluble pectins, phenolic compounds and minerals (Beckles, 2012). Nevertheless, the sugar/acid ratio generally increases during summer and decreases during winter. Primary metabolism is more affected when light and temperature changes occur during early fruit development than when environmental conditions mutate during the ripening phase (Gautier et al., 2008). Under 120 mM NaCl all genotypes showed higher °Brix content compared to the respective controls. High °Brix improves the taste of tomato fruits and is a desirable trait for the processing of tomato products (De Pascale et al., 2001). Also 120 mM NaCl promoted the accumulation of fructose and glucose in the landraces, but not in the commercial variety. Zushi and Matsuzoe (2015) reported that the tomato cultivar Mini Carol accumulated more fruit glucose and fructose under 50 mM NaCl, while the tomato cultivar House Momotaro increased total soluble sugars only under 100 mM NaCl. The authors concluded that the salt effect on sugar levels depends essentially on the genotype. Interestingly, sucrose was detectable only in traces in the fruits of any of the studied tomato accessions, suggesting that salt stress promotes invertase activity and consequently the release of hexoses during tomato ripening (Balibrea et al., 2003). Also, low solar radiation conditions, such as the ones experienced in our study, could affect sugar concentration in sink tissues due to a limitation in carbon fixation/transport in/from source leaves (Hu et al., 2006).
The content of lycopene, the main carotenoid that confers the red pigmentation to the tomato fruit, is a genotype-dependent trait. Lycopene metabolism can be modulated by water deficit (Atkinson et al., 2011; Coyago-Cruz et al., 2017), low light radiation as well as low temperature (Dumas et al., 2003; Jarquín-Enríquez et al., 2013). Even though several tomato landraces have been identified with constitutive high carotenoid content (Pinela et al., 2012; Figàs et al., 2015; Zushi and Matsuzoe, 2015), the studied landraces showed lower fruit lycopene content under control conditions compared to the commercial variety. Upon 60 mM NaCl treatment, the landraces roughly maintained control values of lycopene, while the commercial variety had a significantly reduced content. Ciettaicale and Linosa displayed no changes in lycopene amount under 120 mM NaCl. The decrease in K+ content, as we observed in Corleone and UC-82B under 120 mM NaCl, could affect lycopene production. Indeed, K+ plays a role as cofactor of several enzymes involved in the biosynthesis of isopentenyl diphosphate, the first precursor of carotenoids in the mevalonate pathway (Trudel and Ozbun, 1971). Li and Yuan (2013) and Coyago-Cruz et al. (2019) suggested that the fruit ripening-related accumulation of lycopene can also be influenced due to limited amount of sucrose. Overall, our results were in agreement with those found by Petersen et al. (1998), which reported that salinity did not promote an increase of lycopene levels per dry weight in tomato fruits. Nevertheless, moderate salinity stress has previously been used to improve lycopene content in tomato (De Pascale et al., 2001; Kubota et al., 2012).
Phenolic acids and flavonoids represent a complex class of compounds with specific biological activity. Their profile in tomato fruits have been widely investigated and often used as taxonomical markers to discriminate tomato varieties (Minoggio et al., 2003; Vallverdú-Queralt et al., 2011). Flavonoids content has been positively correlated with environmental radiation, resulting in seasonal changes (Incerti et al., 2009). For example, one light-dependent effect is the up-regulation of the gene expression of chalcone synthase, the first committed enzyme in flavonoid biosynthesis (Feinbaum and Ausubel, 1988). Flavonoids have been found highly concentrated in epidermal and placental tissues of tomato fruits, acting as chemical defences against pathogens and UV radiation (Slimestad et al., 2008). Flavonoids, also known as vitamin P, have recently been targeted as important functional compounds with benefits for human health (Perez-Vizcaino and Fraga, 2018). New emerging studies showed that flavonoids may interfere with the signalling of several kinases that in turn modulate cellular functions by altering the phosphorylation state of target molecules and by modulating gene expression (Williams et al., 2004; Van Der Rest et al., 2006).
Most of the flavonoids found in tomato are mainly present as O-glycosides, but also as non-conjugated forms (aglycones). The main flavonoid reported in tomato fruits is rutin (quercetin 3-rutinoside) (Le Gall et al., 2003). However, naringenin chalcone and its more stable cyclized form naringenin, as well as naringenin-7-O-glucoside, have been also detected as additional frequent flavonoids in fresh tomato skins (Moço et al., 2006) and have been investigated in vitro as potential anti-allergic compounds (Yamamoto et al., 2004). In addition, the presence of the aglycones kaempferol, quercetin and naringenin is also noticed, but with a discrepancy in the amount among experimental studies (Stewart et al., 2000). Overall, the content of flavonoids in terms of quantity and quality greatly varies depending on genotype, growth conditions, stage of ripeness and tissue, as well as on the detection method (Slimestad and Verheul, 2005; Slimestad et al., 2008). In this study, most of the abovementioned flavonoids were identified with the exception of the aglycones, possibly due to the absence of acid hydrolysis of the analysed sample extracts. The commercial variety clearly differed from the landraces for rutin and rutin-O-pentoside contents both under control and salt. Overall, the TFL content, as well as rutin content, in the commercial variety decreased according to the salt gradient. In Ciettaicale and Linosa, TFL content was not affected by salinity, while 120 mM NaCl induced an accumulation of TFL in Corleone fruits.
Tomato fruits also contain hydroxycinnamic acids and respective quinic acid ester derivatives. During ripening, an increase of these esters generally occurs, especially in the pulp (Whitaker and Stommel, 2003). Caffeic acid and its quinic acid ester (chlorogenic acid), as well as ferulic acid and coumaric acid, are detected in quite high levels in tomato fruits. In particular, chlorogenic acid and its derivatives reduce the incidence of fungal disease in tomato (Ruelas et al., 2006; Wojciechowska et al., 2014). Moreover, hydroxycinnamic acids can modulate auxin and ethylene metabolism, which are both involved in fruit size development and ripening (Fleuriet and Macheix, 1981). The highest salt stress condition promoted di- and tricaffeoylquinic acid accumulation in Corleone compared to the commercial variety. Coumaric acid (I+II) showed a similar profile of accumulation in all genotypes under 60 mM NaCl, while a decreasing trend was observed in Linosa and in the commercial variety under 120 mM NaCl compared to the respective controls. Cinnamate 3-hydroxylase, a key limiting enzyme for hydroxycinnamic acid biosynthesis (Ferrer et al., 2008), was shown to be up-regulated at transcriptional and protein levels by salinity (Martinez et al., 2016). Our data suggest that cinnamate 3-hydroxylase may be differentially regulated in the landraces compared to the commercial variety, but further investigations need to be conducted to validate this hypothesis.
The observed decrease in TPHE in salt-treated plants may be the result of the reallocation of phenolic compounds to lignin polymers as a protective mechanism (Humphreys and Chapple, 2002). However, decreased values of phenolic contents are often observed under low temperature (Rivero et al., 2001; Gautier et al., 2008).
Tomato, a fully-fledged member of the Solanaceae, produces steroidal glycoalkaloids, which belong to the terpenoid family. Tomatine represents the main glycoalkaloid in tomato fruit. From a pharmaceutical point of view, dietary tomatine leads to a reduction of plasma cholesterol content thanks to the capacity of this terpenoid to form insoluble complexes with cholesterol, which are poorly absorbed from the intestinal tract (Friedman, 2013). During fruit ripening, tomatine is normally converted in esculeoside, while concomitantly lycopene contents increases (Katsumata et al., 2011). However, as for many other metabolites, this relationship is deeply affected by agronomic practices, genotype and environmental conditions (Koh et al., 2013). The pattern of accumulation of glycoalkaloids was variable in the landraces and in the commercial variety upon salt stress. The levels of esculeoside A were almost similar in all genotypes. Although salinity was shown to induce accumulation of these compounds in tomato leaves (Han et al., 2016), this trend was only confirmed for Corleone.
The class of polyamines, which includes putrescine, spermidine and spermine, is required for tomato fruit development (Cohen et al., 1982). These metabolites can donate amino groups to different other plant compounds, such as hydroxycinnamic acids. Hydroxycinnamic amides seem to play a key role in the reproductive tissues since their catabolism provides nitrogenous and phenolic carbon skeletons for reproductive development (Balint et al., 1987). Caffeoylputrescine and feruloylputrescine isomer contents showed different trends in the genotypes along the salt gradient. Since polyamines compete with ethylene for the biosynthetic precursor S-adenosylmethionine, high content of hydroxycinnamic amides may delay the fruit softening-inhibiting production of ethylene (Liu et al., 2006).
Overall, despite the salinity-induced rearrangement in the stoichiometry of the antioxidant metabolites identified by UPLC-MS/MS, UC-82B and Corleone progressively decreased fruit TAC with increasing salt concentrations, while in Ciettaicale and Linosa TAC only declined under 120 mM NaCl.
Conclusion
The combination of moderate/high salt concentrations with low light irradiance differently affected the yield and the metabolism of the studied tomato genotypes. Despite these non-optimal environmental conditions for tomato cultivation, the Italian landraces showed a different behaviour as compared to the commercial variety UC-82B under moderate salinity stress, showing a tolerable compromise between yield and quality attributes. Salt stress markedly reduced yield and functional metabolite contents in the commercial variety. Among the landraces investigated, Linosa showed better performance in terms of yield/quality parameters under 60 mM NaCl. However, off-season high salinity stress (120 mM NaCl) significantly reduced the antioxidant activity both in UC-82B and in the landraces. In conclusion, these data point to the use of tomato landrace germplasm as a suitable strategy to counteract detrimental environmental factors, such as salinity and off-season cropping, and also as resource of metabolic biomarkers which can be used to improve commercial varieties.
Data Availability
All datasets for this study are included in the manuscript and the Supplementary files.
Author Contributions
TM, RBF, LM, LI, and FS conceived and designed the experiments with the help of all authors. TM, RBF, LM, GC, AS, and LP performed the experiments. TM, RBF, AnP, AL, and LI analyzed the data. TM, RBF, and AnP drafted the manuscript. All authors led the critical review of the final version.
Funding
This research has been financially supported by the Swiss National Science Foundation (grant no. 31003A-166539/1 to DS). AnP was supported by the project no. LQ1605 from the National Program of Sustainability II (MEYS CR).
Conflict of Interest Statement
The authors declare that the research was conducted in the absence of any commercial or financial relationships that could be construed as a potential conflict of interest.
Acknowledgments
Special thanks to Mr. Giovanni Infantino (De Angelis S.r.l, Tolve, Potenza, Italy) for providing us Ciettaicale seeds.
Supplementary Material
The Supplementary Material for this article can be found online at: https://www.frontiersin.org/articles/10.3389/fpls.2019.01078/full#supplementary-material
References
Abenavoli, M. R., Longo, C., Lupini, A., Araniti, F., Mercati, F., Princi, M. P., et al. (2016). Phenotyping two tomato genotypes with different nitrogen use efficiency. Plant Physiol. Biochem. 107, 21–32. doi: 10.1016/j.plaphy.2016.04.021
Adams, P. (1991). Effects of increasing the salinity of the nutrient solution with major nutrients or sodium chloride on the yield, quality and composition of tomatoes grown in rockwool. J. Hort. Sci. 66, 201–207. doi: 10.1080/00221589.1991.11516145
Adams, S. R., Cockshull, K. E., Cave, C. R. J. (2001). Effect of temperature on the growth and development of tomato fruits. Ann. Bot. 88, 869–877. doi: 10.1006/anbo.2001.1524
Adato, A., Mandel, T., Mintz-Oron, S., Venger, I., Levy, D., Yativ, M., et al. (2009). Fruit-surface flavonoid accumulation in tomato is controlled by a SlMYB12-regulated transcriptional network. PLoS Genet. 5 (12), e1000777. doi: 10.1371/journal.pgen.1000777
Agong, S. G., Schittenhelm, S., Friedt, W. (1997). Assessment of tolerance to salt stress in Kenyan tomato germplasm. Euphytica 95, 57–66. doi: 10.1023/A:1002933325347
Asensio, E., Sanvicente, I., Mallor, C., Menal-Puey, S. (2019). Spanish traditional tomato: effects of genotype, location and agronomic conditions on the nutritional quality and evaluation of consumer preferences. Food Chem. 270, 452–458. doi: 10.1016/j.foodchem.2018.07.131
Atkinson, N. J., Dew, T. P., Orfila, C., Urwin, P. E. (2011). Influence of combined biotic and abiotic stress on nutritional quality parameters in tomato (Solanum lycopersicum). J. Agr. Food Chem. 59, 9673–9682. doi: 10.1021/jf202081t
Baldina, S., Picarella, M. E., Troise, A. D., Pucci, A., Ruggieri, V., Ferracane, R., et al. (2016). Metabolite profiling of Italian tomato landraces with different fruit types. Front. Plant Sci. 7, 664. doi: 10.3389/fpls.2016.00664
Balibrea, M. E., Cuartero, J., Bolarín, M. C., Pérez-Alfocea, F. (2003). Sucrolytic activities during fruit development of Lycopersicon genotypes differing in tolerance to salinity. Physiol. Plant. 118, 38–46. doi: 10.1034/j.1399-3054.2003.00084.x
Balint, R., Cooper, G., Staebell, M., Filner, P. (1987). N-caffeoyl-4-amino-n-butyric acid, a new flower-specific metabolite in cultured tobacco cells and tobacco plants. J. Biol. Chem. 262, 11026–11031.
Barbagallo, R. N., Chisari, M., Branca, F., Spagna, G. (2008). Pectin methylesterase, polyphenol oxidase and physicochemical properties of typical long-storage cherry tomatoes cultivated under water stress regime. J. Sci. Food Agr. 88, 389–396. doi: 10.1002/jsfa.3098
Barone, D., Cito, L., Tommonaro, G., Abate, A. A., Penon, D., De Prisco, R., et al. (2018). Antitumoral potential, antioxidant activity and carotenoid content of two Southern Italy tomato cultivars extracts: San Marzano and Corbarino. J. Cell. Physiol. 233, 1266–1277. doi: 10.1002/jcp.25995
Beckles, D. M. (2012). Factors affecting the postharvest soluble solids and sugar content of tomato (Solanum lycopersicum L.) fruit. Postharvest Biol. Technol. 63 (1), 129–140. doi: 10.1016/j.postharvbio.2011.05.016
Berni, R., Romi, M., Parrotta, L., Cai, G., Cantini, C. (2018). Ancient tomato (Solanum lycopersicum L.) varieties of Tuscany have high contents of bioactive compounds. Hortic. 4 (4), 51. doi: 10.3390/horticulturae4040051
Berni, R., Romi, M., Cantini, C., Hausman, J. F., Guerriero, G., Cai, G. (2019). Functional molecules in locally-adapted crops: the case study of tomatoes, onions, and sweet cherry fruits from Tuscany in Italy. Front. Plant Sci. 9, 1983. doi: 10.3389/fpls.2018.01983
Beelders, T., De Beer, D., Stander, M. A., Joubert, E. (2014). Comprehensive phenolic profiling of Cyclopia genistoides (L.) Vent. Molecules 19, 11760–11790. doi: 10.3390/molecules190811760
Borguini, R. G., da Silva Torres, E. A. F. (2009). Tomatoes and tomato products as dietary sources of antioxidants. Food Rev. Int. 25, 313–325. doi: 10.1080/87559120903155859
Canene-Adams, K., Campbell, J. K., Zaripheh, S., Jeffery, E. H., Erdman, J. W. (2005). The tomato as a functional food. J. Nutr. 135, 1226–1230. doi: 10.1093/jn/135.5.1226
Caprioli, G., Cahill, M., Logrippo, S., James, K. (2015). Elucidation of the mass fragmentation pathways of tomatidine and β-1-hydroxytomatine using orbitrap mass spectrometry. Nat. Prod. Commun. 10, 575–576. doi: 10.1177/1934578X1501000409
Casals, J., Pascual, L., Cañizares, J., Cebolla-Cornejo, J., Casañas, F., Nuez, F. (2011). The risks of success in quality vegetable markets: possible genetic erosion in Marmande tomatoes (Solanum lycopersicum L.) and consumer dissatisfaction. Sci. Hort. 130, 78–84. doi: 10.1016/j.scienta.2011.06.013
Casals, J., Rull, A., Bernal, M., González, R., del Castillo, R. R., Simó, J. (2018). Impact of grafting on sensory profile of tomato landraces in conventional and organic management systems. Hort. Environ. Biotechnol. 59, 597–606. doi: 10.1007/s13580-018-0086-z
Caser, M., D’Angiolillo, F., Chitarra, W., Lovisolo, C., Ruffoni, B., Pistelli, L., et al. (2016). Water deficit regimes trigger changes in valuable physiological and phytochemical parameters in Helichrysum petiolare Hilliard & B.L. Ind. Crop. Prod. 83, 680–692. doi: 10.1016/j.indcrop.2015.12.053
Cataldi, T. R. I., Lelario, F., Bufo, S. A. (2005). Analysis of tomato glycoalkaloids by liquid chromatography coupled with electrospray ionization tandem mass spectrometry. Rapid Commun. Mass Spectrom. 19 (21), 3103–3110. doi: 10.1002/rcm.2176
Christ, B., Hauenstein, M., Hörtensteiner, S. (2016). A liquid chromatography–mass spectrometry platform for the analysis of phyllobilins, the major degradation products of chlorophyll in Arabidopsis thaliana. Plant J. 88, 505–518. doi: 10.1111/tpj.13253
Claussen, W., Brückner, B., Krumbein, A., Lenz, F. (2006). Long-term response of tomato plants to changing nutrient concentration in the root environment: the role of proline as an indicator of sensory fruit quality. Plant Sci. 171, 323–331. doi: 10.1016/j.plantsci.2006.04.002
Cohen, E., Arad, S. M., Heimer, Y. M., Mizrahi, Y. (1982). Participation of ornithine decarboxylase in early stages of tomato fruit development. Plant Physiol. 70, 540–543. doi: 10.1104/pp.70.2.540
Coyago-Cruz, E., Corell, M., Stinco, C. M., Hernanz, D., Moriana, A., Meléndez-Martínez, A. J. (2017). Effect of regulated deficit irrigation on quality parameters, carotenoids and phenolics of diverse tomato varieties (Solanum lycopersicum L.). Food Res. Int. 96, 72–83. doi: 10.1016/j.foodres.2017.03.026
Coyago-Cruz, E., Corell, M., Moriana, A., Mapelli-Brahm, P., Hernanz, D., Stinco, C. M., et al. (2019). Study of commercial quality parameters, sugars, phenolics, carotenoids and plastids in different tomato varieties. Food Chem. 277, 480–489. doi: 10.1016/j.foodchem.2018.10.139
Cuartero, J., Bolarín, M. C., Asíns, M. J., Moreno, V. (2006). Increasing salt tolerance in the tomato. J. Exp. Bot. 57 (5), 1045–1058. doi: 10.1093/jxb/erj102
D’Amico, M. L., Izzo, R., Tognoni, F., Pardossi, A., Navari-Izzo, F. (2003). Application of diluted sea water to soilless culture of tomato (Lycopersicon esculentum Mill.): effects on plant growth, yield, fruit quality and antioxidant capacity. J. Food Agr. Environ. 1, 112–116.
De Pascale, S., Maggio, A., Fogliano, V., Ambrosino, P., Ritieni, A. (2001). Irrigation with saline water improves carotenoids content and antioxidant activity of tomato. J. Hort. Sci. Biotechnol. 76, 447–453. doi: 10.1080/14620316.2001.11511392
Dumas, Y., Dadomo, M., Di Lucca, G., Grolier, P. (2003). Effects of environmental factors and agricultural techniques on antioxidant content of tomatoes. J. Sci. Food Agr. 83 (5), 369–382. doi: 10.1002/jsfa.1370
Feinbaum, R. L., Ausubel, F. M. (1988). Transcriptional regulation of the Arabidopsis thaliana Chalcone Synthase gene. Mol. Cell. Biol. 8, 1985–1992. doi: 10.1128/MCB.8.5.1985
Ferrer, J. L., Austin, M. B., Stewart, C., Noel, J. P. (2008). Structure and function of enzymes involved in the biosynthesis of phenylpropanoids. Plant Physiol. Biochem. 46 (3), 356–370. doi: 10.1016/j.plaphy.2007.12.009
Figàs, M. R., Prohens, J., Raigón, M. D., Fita, A., García-Martínez, M. D., Casanova, C., et al. (2015). Characterization of composition traits related to organoleptic and functional quality for the differentiation, selection and enhancement of local varieties of tomato from different cultivar groups. Food Chem. 187, 517–524. doi: 10.1016/j.foodchem.2015.04.083
Fleuriet, A., Macheix, J. J. (1981). Quinyl esters and glucose derivatives of hydroxycinnamic acids during growth and ripening of tomato fruit. Phytochemistry 20, 667–671. doi: 10.1016/0031-9422(81)85153-9
Friedman, M. (2013). Anticarcinogenic, cardioprotective, and other health benefits of tomato compounds lycopene, α-tomatine, and tomatidine in pure form and in fresh and processed tomatoes. J. Agric. Food Chem. 61 (40), 9534–9550. doi: 10.1021/jf402654e
Gaquerel, E., Heiling, S., Schoettner, M., Zurek, G., Baldwin, I. T. (2010). Development and validation of a liquid chromatography-electrospray ionization-time-of-flight mass spectrometry method for induced changes in Nicotiana attenuata leaves during simulated herbivory. J. Agric. Food Chem. 58, 9418–9427. doi: 10.1021/jf1017737
Galmes, J., Ochogavia, J. M., Gago, J., Roldan, E. J., Cifre, J., Conesa, M. A. (2013). Leaf responses to drought stress in Mediterranean accessions of Solanum lycopersicum: anatomical adaptations in relation to gas exchange parameters. Plant Cell Environ. 36, 920–935. doi: 10.1111/pce.12022
Gascuel, Q., Diretto, G., Monforte, A. J., Fortes, A. M., Granell, A. (2017). Use of natural diversity and biotechnology to increase the quality and nutritional content of tomato and grape. Front. Plant Sci. 8, 652. doi: 10.3389/fpls.2017.00652
Gautier, H., Diakou-Verdin, V., Bénard, C., Reich, M., Buret, M., Bourgaud, F., et al. (2008). How does tomato quality (sugar, acid and nutritional quality) vary with ripening stage, temperature, and irradiance? J. Agr. Food Chem. 56, 1241–1250. doi: 10.1021/jf072196t
Gent, M. P. N. (2007). Effect of degree and duration of shade on quality of greenhouse tomato. Hort Sci. 42, 514–520. doi: 10.21273/HORTSCI.42.3.514
Giovannetti, M., Avio, L., Barale, R., Ceccarelli, N., Cristofani, R., Iezzi, A., et al. (2012). Nutraceutical value and safety of tomato fruits produced by mycorrhizal plants. Br. J. Nutr. 107, 242–251. doi: 10.1017/S000711451100290X
Han, P., Wang, Z. J., Lavoir, A. V., Michel, T., Seassau, A., Zheng, W. Y., et al. (2016). Increased water salinity applied to tomato plants accelerates the development of the leaf miner Tuta absoluta through bottom-up effects. Sci. Rep. 6, 32403. doi: 10.1038/srep32403
Hostettler, C., Kölling, K., Santelia, D., Streb, S., Kötting, O., Zeeman, S. C. (2011). Analysis of starch metabolism in chloroplasts. Methods Mol. Biol. 775, 387–410. doi: 10.1007/978-1-61779-237-3_21
Hu, W. H., Zhou, Y. H., Du, Y. S., Xia, X. J., Yu, J. Q. (2006). Differential response of photosynthesis in greenhouse- and field-ecotypes of tomato to long-term chilling under low light. J. Plant Physiol. 163, 1238–1246. doi: 10.1016/j.jplph.2005.10.006
Humphreys, J. M., Chapple, C. (2002). Rewriting the lignin roadmap. Curr. Opin. Plant Biol. 5 (3), 224–229. doi: 10.1016/S1369-5266(02)00257-1
Iijima, Y., Nakamura, Y., Ogata, Y., Tanaka, K. I., Sakurai, N., Suda, K., et al. (2008). Metabolite annotations based on the integration of mass spectral information. Plant J. 54, 949–962. doi: 10.1111/j.1365-313X.2008.03434.x
Incerti, A., Navari-Izzo, F., Pardossib, A., Izzoa, R. (2009). Seasonal variations in polyphenols and lipoic acid in fruits of tomato irrigated with sea water. J. Sci. Food Agr. 89, 1326–1331. doi: 10.1002/jsfa.3589
Incerti, A., Navari-Izzo, F., Pardossi, A., Mensuali, A., Izzo, R. (2007). Effect of sea water on biochemical properties of fruit of tomato (Lycopersicon esculentum Mill.) genotypes differing for ethylene production. J. Sci. Food Agr. 87, 2528–2537. doi: 10.1002/jsfa.3020
Jarquín-Enríquez, L., Mercado-Silva, E. M., Maldonado, J. L., Lopez-Baltazar, J. (2013). Lycopene content and colour index of tomatoes are affected by the greenhouse cover. Sci. Hortic. 155, 43–48. doi: 10.1016/j.scienta.2013.03.004
Jing, P., Wang, D., Zhu, C., Chen, J. (2016). Plant physiological, morphological and yield-related responses to night temperature changes across different species and plant functional types. Front. Plant Sci. 7, 1774. doi: 10.3389/fpls.2016.01774
Katsumata, A., Kimura, M., Saigo, H., Aburaya, K., Nakano, M., Ikeda, T., et al. (2011). Changes in Esculeoside A content in different regions of the tomato fruit during maturation and heat processing. J. Agr. Food Chem. 59, 4104–4110. doi: 10.1021/jf104025p
Koh, E., Kaffka, S., Mitchell, A. E. (2013). A long-term comparison of the influence of organic and conventional crop management practices on the content of the glycoalkaloid α-tomatine in tomatoes. J. Sci. Food Agr. 93, 1537–1542. doi: 10.1002/jsfa.5951
Kubota, C., Kroggel, M., Torabi, M., Dietrich, K. A., Kim, H. J., Fonseca, J., et al. (2012). Changes in selected quality attributes of greenhouse tomato fruit as affected by pre-and postharvest environmental conditions in year-round production. Hort Sci. 47, 1698–1704. doi: 10.21273/HORTSCI.47.12.1698
Le, S., Josse, J., Husson, F. (2008). FactoMineR: an R package for multivariate analysis. J. Stat. Softw. 25 (1), 1–18. doi: 10.18637/jss.v025.i01
Le Gall, G., Dupont, M. S., Mellon, F. A., Davis, A. L., Collins, G. J., Verhoeyen, M. E., et al. (2003). Characterization and content of flavonoid glycosides in genetically modified tomato (Lycopersicon esculentum) fruits. J. Agr. Food Chem. 51, 2438–2446. doi: 10.1021/jf025995e
Li, L., Yuan, H. (2013). Chromoplast biogenesis and carotenoid accumulation. Arch. Biochem. Biophys. 539 (2), 102–109. doi: 10.1016/j.abb.2013.07.002
Liu, J. H., Honda, C., Moriguchi, T. (2006). Involvement of polyamine in floral and fruit development. JARQ 40 (1), 51–58. doi: 10.6090/jarq.40.51
Lupini, A., Princi, M. P., Araniti, F., Miller, A. J., Sunseri, F., Abenavoli, M. R. (2017). Physiological and molecular responses in tomato under different forms of N nutrition. J. Plant Physiol. 216, 17–25. doi: 10.1016/j.jplph.2017.05.013
Manaa, A., Faurobert, M., Valot, B., Bouchet, J.-P., Grasselly, D., Causse, M., et al. (2013). Effect of salinity and calcium on tomato fruit proteome. OMICS 17, 338–352. doi: 10.1089/omi.2012.0108
Mariz-Ponte, N., Martins, S., Gonçalves, A., Correia, C. M., Ribeiro, C., Dias, M. C., et al. (2019). The potential use of the UV-A and UV-B to improve tomato quality and preference for consumers. Sci. Hortic. 246, 777–784. doi: 10.1016/j.scienta.2018.11.058
Martinez, V., Mestre, T. C., Rubio, F., Girones-Vilaplana, A., Moreno, D. A., Mittler, R., et al. (2016). Accumulation of flavonols over hydroxycinnamic acids favors oxidative damage protection under abiotic stress. Front. Plant Sci. 7, 838. doi: 10.3389/fpls.2016.00838
Massaretto, I. L., Albaladejo, I., Purgatto, E., Flores, F. B., Plasencia, F., Egea-Fernández, J. M., et al. (2018). Recovering tomato landraces to simultaneously improve fruit yield and nutritional quality against salt stress. Front. Plant Sci. 9, 1778–1778. doi: 10.3389/fpls.2018.01778
Minoggio, M., Bramati, L., Simonetti, P., Gardana, C., Iemoli, L., Santangelo, E., et al. (2003). Polyphenol pattern and antioxidant activity of different tomato lines and cultivars. Ann. Nutr. Metab. 47, 64–69. doi: 10.1159/000069277
Mintz-Oron, S., Mandel, T., Rogachev, I., Feldberg, L., Lotan, O., Yativ, M., et al. (2008). Gene expression and metabolism in tomato fruit surface tissues. Plant Physiol. 147, 823–851. doi: 10.1104/pp.108.116004
Moço, S., Bino, R. J., Vorst, O., Verhoeven, H. A., de Groot, J., van Beek, T. A., et al. (2006). A Liquid Chromatography-Mass Spectrometry-based metabolome database for tomato. Plant Physiol. 141, 1205–1218. doi: 10.1104/pp.106.078428
Moles, T. M., Pompeiano, A., Huarancca Reyes, T., Scartazza, A., Guglielminetti, L. (2016). The efficient physiological strategy of a tomato landrace in response to short-term salinity stress. Plant Physiol. Biochem. 109, 262–272. doi: 10.1016/j.plaphy.2016.10.008
Moles, T. M., Mariotti, L., De Pedro, L. F., Guglielminetti, L., Picciarelli, P., Scartazza, A. (2018). Drought induced changes of leaf-to-root relationships in two tomato genotypes. Plant Physiol. Biochem. 128, 24–31. doi: 10.1016/j.plaphy.2018.05.008
Ndhlala, A. R., Moyo, M., Van Staden, J. (2010). Natural antioxidants: fascinating or mythical biomolecules? Molecules 15, 6905–6930. doi: 10.3390/molecules15106905
Oksanen, J., Blanchet, F. G., Friendly, M., Kindt, R., Legendre, P., McGlinn, D., et al. (2019). Vegan: Community Ecology Package. R package version 2.5-4.
Park, S., Cheng, N. H., Pittman, J. K., Yoo, K. S., Park, J., Smith, R. H., et al. (2005). Increased calcium levels and prolonged shelf-life in tomatoes expressing Arabidopsis H+/Ca2+ transporters. Plant Physiol. 139, 1194–1206. doi: 10.1104/pp.105.066266
Patanè, C., Scordi, D., Testa, G., Cosentino, S. L. (2016). Physiological screening for drought tolerance in Mediterranean long-storage tomato. Plant Sci. 249, 25–34. doi: 10.1016/j.plantsci.2016.05.006
Patanè, C., Pellegrino, A., Saita, A., Siracusa, L., Ruberto, G., Barbagallo, R. (2017). Mediterranean long storage tomato as a source of novel products for the agrifood industry: nutritional and technological traits. LWT - Food Sci. Technol. 85, 445–448. doi: 10.1016/j.lwt.2016.12.011
Perez-Vizcaino, F., Fraga, C. G. (2018). Research trends in flavonoids and health. Arch. Biochem. Biophys. 646, 107–112. doi: 10.1016/j.abb.2018.03.022
Petersen, K. K., Willumsen, J., Kaack, K. (1998). Composition and taste of tomatoes as affected by increased salinity and different salinity sources. J. Hort. Sci. Biotechnol. 73, 205–215. doi: 10.1080/14620316.1998.11510966
Pinela, J., Barros, L., Carvalho, A. M., Ferreira, I. C. F. R. (2012). Nutritional composition and antioxidant activity of four tomato (Lycopersicon esculentum L.) farmer’ varieties in Northeastern Portugal homegardens. Food Chem. Toxicol. 50, 829–834. doi: 10.1016/j.fct.2011.11.045
Plieth, C. (2005). Calcium: just another regulator in the machinery of life? Ann. Bot. 96, 1–8. doi: 10.1093/aob/mci144
Poiroux-Gonord, F., Bidel, L. P. R., Fanciullino, A. L., Gautier, H., Lauri-Lopez, F., Urban, L. (2010). Health benefits of vitamins and secondary metabolites of fruits and vegetables and prospects to increase their concentrations by agronomic approaches. J. Agr. Food Chem. 58, 12065–12082. doi: 10.1021/jf1037745
Pompeiano, A., Di Patrizio, E., Volterrani, M., Scartazza, A., Guglielminetti, L. (2016). Growth responses and physiological traits of seashore paspalum subjected to short-term salinity stress and recovery. Agr. Water Manag. 163, 57–65. doi: 10.1016/j.agwat.2015.09.004
Pompeiano, A., Landi, M., Meloni, G., Vita, F., Guglielminetti, L., Guidi, L. (2017). Allocation pattern, ion partitioning, and chlorophyll a fluorescence in Arundo donax L. Plant Biosyst. 151 (4), 613–622. doi: 10.1080/11263504.2016.1187680
R Core Team (2019). R: A language and environment for statistical computing. Vienna, Austria: R Foundation for Statistical Computing.
Rivero, R. M., Ruiz, J. M., García, P. C., López-Lefebre, L. R., Sánchez, E., Romero, L. (2001). Resistance to cold and heat stress: accumulation of phenolic compounds in tomato and watermelon plants. Plant Sci. 160, 315–321. doi: 10.1016/S0168-9452(00)00395-2
Ruelas, C., Tiznado-Hernández, M. E., Sánchez-Estrada, A., Robles-Burgueño, M. R., Troncoso-Rojas, R. (2006). Changes in phenolic acid content during Alternaria alternata infection in tomato fruit. J. Phytopathol. 154, 236–244. doi: 10.1111/j.1439-0434.2006.01090.x
Ruiz, J. J., Alonso, A., García-Martínez, S., Valero, M., Blasco, P., Ruiz-Bevia, F. (2005). Quantitative analysis of flavour volatiles detects differences among closely related traditional cultivars of tomato. J. Sci. Food Agr. 85, 54–60. doi: 10.1002/jsfa.1879
Sánchez-Rodríguez, E., Ruiz, J. M., Ferreres, F., Moreno, D. A. (2012). Phenolic profiles of cherry tomatoes as influenced by hydric stress and rootstock technique. Food Chem. 134, 775–782. doi: 10.1016/j.foodchem.2012.02.180
Santa-Cruz, A., Martinez-Rodriguez, M. M., Perez-Alfocea, F., Romero-Aranda, R., Bolarin, M. C. (2002). The rootstock effect on the tomato salinity response depends on the shoot genotype. Plant Sci. 162, 825–831. doi: 10.1016/S0168-9452(02)00030-4
Sinesio, F., Moneta, E., Peparaio, M. (2007). Sensory characteristics of traditional field grown tomato genotypes in Southern Italy. J. Food Qual. 30, 878–895. doi: 10.1111/j.1745-4557.2007.00161.x
Siracusa, L., Patanè, C., Rizzo, V., Cosentino, S. L., Ruberto, G. (2018). Targeted secondary metabolic and physico-chemical traits analysis to assess genetic variability within a germplasm collection of “long storage” tomatoes. Food Chem. 244, 275–283. doi: 10.1016/j.foodchem.2017.10.043
Slimestad, R., Verheul, M. J. (2005). Seasonal variations in the level of plant constituents in greenhouse production of cherry tomatoes. J. Agr. Food Chem. 53, 3114–3119. doi: 10.1021/jf047864e
Slimestad, R., Fossen, T., Verheul, M. J. (2008). The flavonoids of tomatoes. J. Agr. Food Chem. 56, 2436–2441. doi: 10.1021/jf073434n
Soetan, K. O., Olaiya, C. O., Oyewole, O. E. (2010). The importance of mineral elements for humans, domestic animals and plants. Afr. J. Food Sci 4, 200–222.
Stewart, A. J., Bozonnet, S., Mullen, W., Jenkins, G. I., Lean, M. E. J., Crozier, A. (2000). Occurrence of flavonols in tomatoes and tomato-based products. J. Agr. Food Chem. 48, 2663–2669. doi: 10.1021/jf000070p
Taylor, M. D., Locascio, S. J. (2004). Blossom-end rot: a calcium deficiency. J. Plant Nutr. 27 (1), 123–139. doi: 10.1081/PLN-120027551
Thalmann, M., Pazmino, D., Seung, D., Horrer, D., Nigro, A., Meier, T., et al. (2016). Regulation of leaf starch degradation by abscisic acid is important for osmotic stress tolerance in plants. Plant Cell 28, 1860–1878. doi: 10.1105/tpc.16.00143
Toor, R., Savage, G., Lister, C. (2006). Seasonal variations in the antioxidant composition of greenhouse grown tomatoes. J. Food Compos. Anal. 19, 1–10. doi: 10.1016/j.jfca.2004.11.008
Trudel, M. J., Ozbun, J. L. (1971). Influence of potassium on carotenoid content of tomato fruit. J. Am. Soc. Hortic. 96, 763–765.
Vallverdú-Queralt, A., Medina-Remón, A., Martínez-Huélamo, M., Jáuregui, O., Andres-Lacueva, C., Lamuela-Raventos, R. M. (2011). Phenolic profile and hydrophilic antioxidant capacity as chemotaxonomic markers of tomato varieties. J. Agr. Food Chem. 59, 3994–4001. doi: 10.1021/jf104400g
Van Der Rest, B., Danoun, S., Boudet, A. M., Rochange, S. F. (2006). Down-regulation of Cinnamoyl-CoA reductase in tomato (Solanum lycopersicum L.) induces dramatic changes in soluble phenolic pools. J. Exp. Bot. 57, 1399–1411. doi: 10.1093/jxb/erj120
Vaníčková, L., Břízová, R., Pompeiano, A., Ekesi, S., De Meyer, M. (2015). Cuticular hydrocarbons corroborate the distinction between lowland and highland Natal fruit fly (Tephritidae, Ceratitis rosa) populations. ZooKeys 540, 507–524. doi: 10.3897/zookeys.540.9619
Whitaker, B. D., Stommel, J. R. (2003). Distribution of hydroxycinnamic acid conjugates in fruit of commercial eggplant (Solanum melongena L.) cultivars. J. Agr. Food Chem. 51, 3448–3454. doi: 10.1021/jf026250b
Williams, R. J., Spencer, J. P. E., Rice-Evans, C. (2004). Flavonoids: antioxidants or signalling molecules? Free Radic. Biol. Med. 36 (7), 838–849. doi: 10.1016/j.freeradbiomed.2004.01.001
Wojciechowska, E., Weinert, C. H., Egert, B., Trierweiler, B., Schmidt-Heydt, M., Horneburg, B., et al. (2014). Chlorogenic acid, a metabolite identified by untargeted metabolome analysis in resistant tomatoes, inhibits the colonization by Alternaria alternata by inhibiting alternariol biosynthesis. Eur. J. Plant Pathol. 139, 735–747. doi: 10.1007/s10658-014-0428-3
Wu, M., Kubota, C. (2008). Effects of high electrical conductivity of nutrient solution and its application timing on lycopene, chlorophyll and sugar concentrations of hydroponic tomatoes during ripening. Sci. Hortic. 116, 122–129. doi: 10.1016/j.scienta.2007.11.014
Yamamoto, T., Yoshimura, M., Yamaguchi, F., Kouchi, T., Tsuji, R., Saito, M., et al. (2004). Anti-allergic activity of Naringenin Chalcone from a tomato skin extract. Biosci. Biotechnol. Biochem. 68, 1706–1711. doi: 10.1271/bbb.68.1706
Zushi, K., Matsuzoe, N. (2009). Seasonal and cultivar differences in salt-induced changes in antioxidant system in tomato. Sci. Hortic. 120, 181–187. doi: 10.1016/j.scienta.2008.10.005
Keywords: tomato, landraces, off-season, salinity, fruit quality, metabolites
Citation: Moles TM, Francisco RdB, Mariotti L, Pompeiano A, Lupini A, Incrocci L, Carmassi G, Scartazza A, Pistelli L, Guglielminetti L, Pardossi A, Sunseri F, Hörtensteiner S and Santelia D (2019) Salinity in Autumn-Winter Season and Fruit Quality of Tomato Landraces. Front. Plant Sci. 10:1078. doi: 10.3389/fpls.2019.01078
Received: 28 April 2019; Accepted: 07 August 2019;
Published: 24 September 2019.
Edited by:
George A. Manganaris, Cyprus University of Technology, CyprusReviewed by:
Eleni Tani, Agricultural University of Athens, GreeceAmalia Barone, University of Naples Federico II, Italy
Alexios A. Alexopoulos, Technological Educational Institute of Peloponnese, Greece
Copyright © 2019 Moles, de Brito Francisco, Mariotti, Pompeiano, Lupini, Incrocci, Carmassi, Scartazza, Pistelli, Guglielminetti, Pardossi, Sunseri, Hörtensteiner and Santelia. This is an open-access article distributed under the terms of the Creative Commons Attribution License (CC BY). The use, distribution or reproduction in other forums is permitted, provided the original author(s) and the copyright owner(s) are credited and that the original publication in this journal is cited, in accordance with accepted academic practice. No use, distribution or reproduction is permitted which does not comply with these terms.
*Correspondence: Tommaso Michele Moles, dG9tbWFzby5tb2xlc0BlbnYuZXRoei5jaA==; Rita de Brito Francisco, cmZyYW5jaXNjb0Bib3RpbnN0LnV6aC5jaA==; Lorenzo Mariotti, bG9yZW56by5tYXJpb3R0aUB1bmlwaS5pdA==