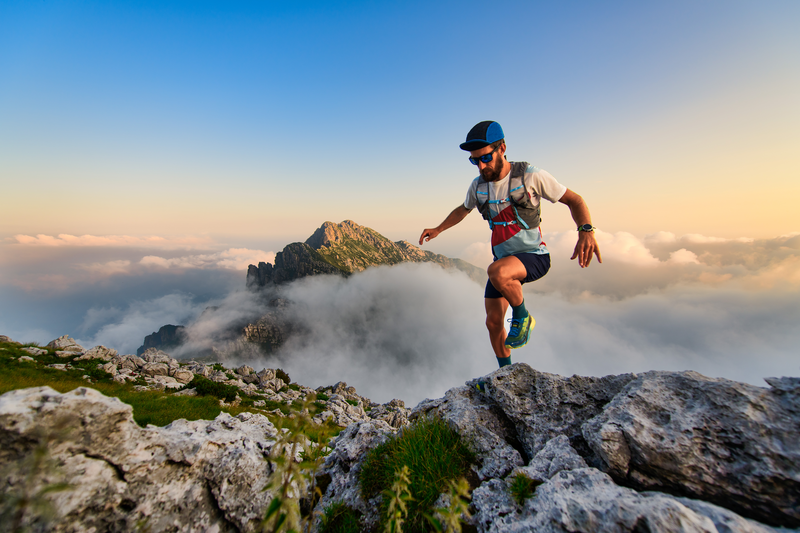
94% of researchers rate our articles as excellent or good
Learn more about the work of our research integrity team to safeguard the quality of each article we publish.
Find out more
REVIEW article
Front. Plant Sci. , 29 August 2019
Sec. Plant Physiology
Volume 10 - 2019 | https://doi.org/10.3389/fpls.2019.01022
Diverse proteins are found modified with glycosylphosphatidylinositol (GPI) at their carboxyl terminus in eukaryotes, which allows them to associate with membrane lipid bilayers and anchor on the external surface of the plasma membrane. GPI-anchored proteins (GPI-APs) play crucial roles in various processes, and more and more GPI-APs have been identified and studied. In this review, previous genomic and proteomic predictions of GPI-APs in Arabidopsis have been updated, which reveal their high abundance and complexity. From studies of individual GPI-APs in Arabidopsis, certain GPI-APs have been found associated with partner receptor-like kinases (RLKs), targeting RLKs to their subcellular localization and helping to recognize extracellular signaling polypeptide ligands. Interestingly, the association might also be involved in ligand selection. The analyses suggest that GPI-APs are essential and widely involved in signal transduction through association with RLKs.
The GPI oligosaccharide structure is ubiquitous among eukaryotes with a common minimal backbone consisting of three mannoses, one non-N-acetylated glucosamine (GlcN), and inositol phospholipid, which covalently links the carboxyl terminus (C terminus) of GPI-APs to the lipid bilayer (Figure 1A) (Stevens, 1995; Oxley and Bacic, 1999; Kinoshita and Fujita, 2016). Catalyzed by a series of enzyme complexes, GPI biosynthesis starts with a lipid molecule at the rough side of the endoplasmic reticulum (ER), and this then flips and the synthesis is completed on the luminal side of the ER (Figure 1C) (Stevens, 1995; Takeda and Kinoshita, 1995; Kinoshita and Fujita, 2016). The typical GPI-AP precursors possess a common structure that lead them to be modified by GPI moieties inside endomembrane systems: amino-terminal (N-terminal) hydrophobic signal peptides lead them to enter the ER lumen, and during translation and maturation, C-terminal hydrophobic signals are recognized and cleaved at the ω position by a series of catalytic complexes, where the peptide bond is replaced by a bond with ethanolamine phosphate (Figures 1A–C) (Eisenhaber et al., 1998; Kinoshita, 2014a; Kinoshita and Fujita, 2016).
Figure 1 Biosynthesis of GPI moiety and GPI-AP. (A) Minimal oligosaccharide structure of GPI modification, D-Manα (1–2)-D-Manα (1–6)-D-Manα (1–4)-D-GlcN-inositol, which covalently links the C-terminus of GPI-AP and lipid molecule. (B) Common structure of GPI-AP precursor. The hydrophobic regions at the N and C termini are in black and the spacer region is in light gray. (C) Biosynthesis of GPI-APs. ① Precursor of GPI-AP enters ER, ② C terminus of GPI-AP precursor is recognized when entering ER, ③ Oligosaccharide structure of GPI modification is synthesized separately, ④ Recognized C terminus of GPI-AP is cleaved and covalently linked to the GPI moiety.
The GPI moiety allows these GPI-APs, which possess no transmembrane region, to be anchored to membrane lipid bilayers. Compared to transmembrane association, GPI anchoring has its advantages: GPI-AP shedding and release due to the presence of GPI-specific phospholipases (PLC) makes this association reversible in mammalian cells (Orihashi et al., 2012; Fujihara and Ikawa, 2016). In plants, although similar shedding and release mechanisms are indicated as various GPI-APs were identified in cell walls, thus far, no GPI-specific PLC has been identified yet (Bayer et al., 2006; Yeats et al., 2018). However, a bacterial phosphatidylinositol-specific PLC (PI-PLC) has been used for shedding GPI-APs from lipid bilayers in vitro and identifying them by further proteomic analysis in Arabidopsis (Borner et al., 2003; Elortza et al., 2003; Takahashi et al., 2016; Yeats et al., 2018).
GPI-APs and their GPI moieties were demonstrated to be crucial for diverse developmental processes in mammals and in plants, because development was found to be broadly and severely affected if GPI moiety biosynthesis is disrupted (Kawagoe et al., 1996; Gillmor et al., 2005; Kinoshita, 2014b; Bundy et al., 2016).
As the most noticeable feature, GPI anchoring was thought to be essential for the functions of GPI-APs, and their enzymatic activities or subcellular localizations could be altered by the removal of the GPI moiety (Tozeren et al., 1992; Butikofer et al., 2001; Davies et al., 2010). However, there are exceptions: the GPI anchoring of ZERZAUST and FLA4/SOS5 was shown to be dispensable for their functions in Arabidopsis (Vaddepalli et al., 2017; Xue et al., 2017).
GPI moieties also play crucial roles for driving the transient, relatively ordered membrane domains rich in sphingolipids and sterols, which are called lipid rafts or microdomains, to their target regions (Saha et al., 2016; Sezgin et al., 2017; Hellwing et al., 2018; Lebreton et al., 2018). In mammalian and yeast cells, GPI-APs are co-clustered and organized in a mixture of monomers and cholesterol-dependent nanoclusters in the same lipid raft. These exit the ER in vesicles distinct from other secretory proteins and are predominantly sorted to the apical surface to serve in protein trafficking and signaling transduction (Eisenhaber et al., 1998; Morsomme et al., 2003; Legler et al., 2005; Muniz and Zurzolo, 2014; Miyagawa-Yamaguchi et al., 2015; Sezgin et al., 2017). In Arabidopsis, although GPI modification was found essential for protein delivery from the ER to ht eplasmodesmata (Zavaliev et al., 2016), the lipid raft mechanism has not been well revealed yet.
To identify GPI-APs, various bioinformatics tools were developed, generally depending on the prediction of a specific hydrophobic region at the C terminus. Examples are big-PI Plant Predictor (http://mendel.imp.ac.at/sat/gpi/gpi_server.html) (Eisenhaber et al., 1998), PredGPI (http://gpcr2.biocomp.unibo.it/gpipe/info.htm) (Pierleoni et al., 2008), GPI-SOM (http://gpi.unibe.ch/) (Fankhauser and Maser, 2005), and fragAnchor (Poisson et al., 2007). According to the latest genomic scanning by these tools, among lower and higher eukaryotes, about 0.21% to 2.01% of total proteins from diverse families are predicted to be modified by GPI moieties, and the percentage in Arabidopsis is 0.83% (Eisenhaber et al., 2001; Poisson et al., 2007). In the meantime, proteomic assays, which depend on cleavage from membranes by bacterial PI-PLC treatment in vitro and enrichment in particular membrane fractions, were performed to compare proteomic data to bioinformatic data. To date, more than 300 GPI-APs have been identified in Arabidopsis (Borner et al., 2002; Borner et al., 2003; Elortza et al., 2003; Bayer et al., 2006; Takahashi et al., 2016).
Arabidopsis GPI-APs identified in 2003 (Borner et al., 2003; Elortza et al., 2003) and 2016 (Takahashi et al., 2016) are assembled in Tables 1 and 2 , respectively, and their functions are discussed.
Table 1 A review of predicted GPI-APs updated from (Borner et al., 2003; Elortza et al., 2003).
In Table 1, 248 genes predicted to encode GPI-APs in 2003 have been listed. Some corrections have been made, as some of them could not be found in databases or turned out to encode non-coding RNA. However, according to more recent experimental data, genes not included in 2003 also turned out to encode GPI-APs, such as At1g09460, At2g30933, At2g03505, and At4g13600 (Simpson et al., 2009), LORELEI (Tsukamoto et al., 2010), and XYP2 (Motose et al., 2004). Interestingly, due to recent achievements on alternative splicing, transcriptional variants of SKS3 (Zhou, 2019a) and CRK10 (Grojean and Downes, 2010) have been found to encode GPI-APs besides their ordinarily reported proteins (Figure 2). Alternative splicing largely enhanced the diversity of transcriptome and proteome, and more and more genes (up to 80% according to recent RNA-seq achievements) have been found to be alternatively spliced in Arabidopsis, which could greatly increase the abundance of GPI-APs (Wang et al., 2009; Filichkin et al., 2010; Severing et al., 2011; Reddy et al., 2013; Lee and Rio, 2015; Bush et al., 2017).
Figure 2 GPI-anchored SKS3 and CRK10 encoded by alternatively spliced transcriptional variants. (A) Alternative splicing of SKS3. (B) Alternative splicing of CRK10. ExD, extracellular domain; TM, transmembrane domain; KD, intracellular kinase domain. Exons are dark gray, introns are black lines, and untranslated transcribed regions are light gray.
In addition, 163 GPI-APs were predicted in 2016, and those not included in Table 1 are listed in Table 2. In this study, a large proportion of possible GPI-APs were discounted as typical GPI-APs in spite of being predicted to possess a GPI signal at the C terminus by various bioinformatics tools. Some of those discounted were transmembrane proteins, such as PIN3 and PIN4 and some receptor-like kinases (RLKs), and the other were cytoplasmic proteins without N-terminal secretory signal peptide, such as SNARE family proteins (listed at the end of Table 2).
GPI-APs listed in Tables 1 and 2 are from diverse families, such as cell wall structure proteins, proteases, enzymes, receptor-like proteins (RLPs), lipid transfer proteins, and GPI-anchored peptides, which imply a functional diversity of GPI-APs: indeed, they were found functional in most processes, such as cell wall composition, cell wall component synthesis, cell polar expansion, stress responses, hormone signaling responses, pathogen responses, stomatal development, pollen tube elongation, and double fertilization in Arabidopsis.
Among these GPI-APs, the arabinogalactan protein (AGP) family, LORELEI family, COBRA family, and some RLPs, were better characterized. AGP family proteins are ubiquitous cell wall components anchoring on the plasma membrane throughout the Plant Kingdom and abundantly decorated at their Hyp residues by arabinogalactan polysaccharides, which make them be one of the most complex families of macromolecules in plants and play roles in various processes (Schultz et al., 2000; Ellis et al., 2010; Marzec et al., 2015; Showalter and Basu, 2016; Losada and Herrero, 2019; Palacio-Lopez et al., 2019). COBRA families were reported to be involved in various processes by regulating cell wall synthesis in plants (Hochholdinger et al., 2008; Cao et al., 2012; Niu et al., 2015; Niu et al., 2018). LORELEI family proteins associate with cell surface RLK, which is essential not only for ligand recognition but also for RLK transport (Capron et al., 2008; Duan et al., 2010; Tsukamoto et al., 2010; Meng et al., 2012; Yu et al., 2012; Li et al., 2015; Li et al., 2016; Liao et al., 2017; Stegmann et al., 2017; Feng et al., 2018; Guo et al., 2018; Yin et al., 2018).
In Arabidopsis, hundreds of RLKs, which possess extracellular ligand recognition domains and intracellular kinase domains, control a wide range of processes, including development, disease resistance, hormone perception, and self-incompatibility (Shiu and Bleecker, 2001; Muschietti and Wengier, 2018; Wei and Li, 2018). Their association with extracellular ligands, including phytohormones, signaling polypeptides, and pathogen molecules, leads to the phosphorylation of the intracellular kinase domain, which consequently activate cytoplasmic signaling components and switch on response mechanisms (Figure 3A) (Pearce et al., 2001; Asai et al., 2002; Geldner and Robatzek, 2008; Murphy et al., 2012; Breiden and Simon, 2016; Yamaguchi et al., 2016; Chardin et al., 2017).
Figure 3 RLK-mediated signaling pathway and various associations between RLKs and GPI-APs. (A) Association with extracellular ligand activates and phosphorylates the intracellular kinase domain of RLK, which activates intracellular signaling components to regulate various processes. (B) GPI-AP is required for ligand recognition and its association with RLK. (C) GPI-AP is required not only for ligand recognition and its association with RLK but also for RLK localization by chaperoning its transport, and those un-chaperoned would reside in ER. (D) GPI-APs are required for ligand selection.
By summarizing the functional mechanism of those listed GPI-APs in Tables 1 and 2, a group of GPI-APs from various families was found to share a common mechanism of action involving RLK-related signal transduction (Table 3). The same mechanism has been reported in mammalian cells, for example, that GPI-anchored CD14 possessing leucine-rich repeats (LRR) region associates with not only Toll-like receptor TLR4 to perceive their polypeptide ligand lipopolysaccharide (LPS) leading them to activate mitogen-activated protein kinase (MAPK) cascades (Wright et al., 1990; Schumann, 1992; Zanoni et al., 2011; Li X. et al., 2015) but also TLR3 to perceive viral double-stranded RNA (dsRNA) leading them to activate (Vercammen et al., 2008). This common mechanism found in both animals and plants suggests that important and common roles are played by GPI-APs in signal transduction (Figure 3B).
Interestingly, the association between GPI-AP and RLK could be involved in not only ligand recognition but also RLK transport and subcellular localization. One of the best characterized GPI-APs, LORELEI, not only participates in ligand recognition by associating with FERONIA but also plays a crucial role in chaperoning the transport of FERONIA from the ER to the plasma membrane (Capron et al., 2008; Duan et al., 2010; Tsukamoto et al., 2010; Meng et al., 2012; Yu et al., 2012; Li et al., 2015; Li et al., 2016; Liao et al., 2017; Stegmann et al., 2017; Feng et al., 2018; Guo et al., 2018; Yin et al., 2018) (Figure 3C). This special chaperone and transport mechanism might be due to the GPI-APs becoming involved with lipid rafts, which determine distinct protein sorting and protein traffic (Eisenhaber et al., 1998; Legler et al., 2005; Miyagawa-Yamaguchi et al., 2015; Sezgin et al., 2017).
GPI-APs appear to be important not only for ligand recognition but also essential for ligand selection. For example, RLK FERONIA recognizes ligands RALF1 or RALF22/23 when associated with GPI-anchored LORELEI or LRX5, respectively (Li C et al., 2015; Li et al., 2016; Zhao et al., 2018). This potential GPI-AP-dependent selection mechanism could greatly enhance the ligand recognition abundance of RLK but could also mediate the cross-talk between various signaling perception and transduction (Figure 3D).
The associations between GPI-AP and RLK could be structure independent, such as SKU5-TMK1, LRE/LLGs-FERONIA, FLA4-FEI1/FEI2, ENODL14-FERONIA, and LRX5-FERONIA, or structure dependent, such as TMM and ERECTA both possessing LRR structure at the extracellular domain and LYM1/LYM3 and CERK1 both possessing LyM structure at extracellular domain in Arabidopsis. Interestingly, the same structure dependence is also present in one of the best characterized GPI-APs in mammalian cells, CD-14, and together with its partner receptor kinases TLR3 and TLR4 all possess an LRR structure.
The structure-dependent associations between GPI-APs and RLKs largely increased the curiosities of the group of GPI-anchored RLPs, which shared the same structures or sequence similarities with RLKs but lack intracellular kinase domains. They might recognize specific RLKs depending on sequence and structure similarities and form heterodimers with various RLKs in the ER or Golgi bodies and then chaperone them to specific plasma membrane regions through GPI-AP-driven lipid rafts. On arrival, they select and recognize ligands and activate the intracellular signaling components.
Whether the GPI-anchored RLKs encoded by transcriptional variants, such as GPI-CRK10 and its variant of CRK10, can form homodimers based on the same extracellular domains and play a role in RLK regulation, is a very interesting question.
Previous genomic and proteomic assays that predicted and identified GPI-APs from Arabidopsis have been listed. Due to recent experimental data and knowledge of alternative splicing, more and more GPI-APs have been identified, suggesting that GPI-APs in Arabidopsis might be more abundant than we expected.
Previous studies on those listed GPI-APs from diverse families were discussed, and they were found to be involved in diverse biological processes, including cell wall composition, cell wall component synthesis, cell polar expansion, hormone signaling response, stress response, pathogen response, stomata development, pollen tube elongation, and double fertilization. Those reports demonstrated the functional diversity and indispensability of GPI-APs in Arabidopsis.
Among these reports, direct associations were found between various GPI-APs and their partner cell surface RLKs, demonstrating not only participation in their ligand recognition but also essential roles in RLK transport and localization. Localization might due to specific protein sorting and protein traffic driven by GPI-AP-related lipid rafts. Surprisingly, GPI-APs have also been shown to participate in ligand selection, which made one RLK and its downstream intracellular target activated by various ligands. Such protein cross-reactivity greatly enhanced the ligand recognition abundance of RLKs, which can also be considered as a common mechanism of cross-talk between various ligands or various signaling pathways.
In this review, the most predicted or identified GPI-APs in Arabidopsis were listed and discussed, and a common involvement of them in signing transduction was summarized. This involvement could be very helpful for understanding the ligand-RLK signaling transduction in plants, especially for understanding the polar localization of RLKs, and the crosstalk between various ligand-RLK signaling transduction. It would be interesting to identify more associations between various GPI-APs and RLKs and study their recognition and selection of ligands and downstream intracellular signaling components in Arabidopsis.
KZ wrote this manuscript.
The authors declare that the research was conducted in the absence of any commercial or financial relationships that could be construed as a potential conflict of interest.
Abdin, M. Z., Kiran, U., Alam, A. (2011). Analysis of osmotin, a PR protein as metabolic modulator in plants. Bioinformation 5 (8), 336–340. doi: 10.6026/97320630005336
Abrash, E. B., Bergmann, D. C. (2010). Regional specification of stomatal production by the putative ligand CHALLAH. Development 137 (3), 447–455. doi: 10.1242/dev.040931
Acosta-Garcia, G., Vielle-Calzada, J. P. (2004). A classical arabinogalactan protein is essential for the initiation of female gametogenesis in Arabidopsis. Plant Cell 16 (10), 2614–2628. doi: 10.1105/tpc.104.024588
Asai, T., Tena, G., Plotnikova, J., Willmann, M. R., Chiu, W. L., Gomez-Gomez, L., et al. (2002). MAP kinase signalling cascade in Arabidopsis innate immunity. Nature 415 (6875), 977–983. doi: 10.1038/415977a
Barozzi, F., Papadia, P., Stefano, G., Renna, L., Brandizzi, F., Migoni, D., et al. (2018). Variation in membrane trafficking linked to SNARE AtSYP51 interaction with aquaporin NIP1;1. Front. Plant Sci. 9, 1949. doi: 10.3389/fpls.2018.01949
Basu, D., Tian, L., Debrosse, T., Poirier, E., Emch, K., Herock, H., et al. (2016). Glycosylation of a fasciclin-like arabinogalactan-protein (SOS5) mediates root growth and seed mucilage adherence via a cell wall receptor-like kinase (FEI1/FEI2) pathway in Arabidopsis. PLoS One 11 (1), e0145092. doi: 10.1371/journal.pone.0145092
Bayer, E. M., Bottrill, A. R., Walshaw, J., Vigouroux, M., Naldrett, M. J., Thomas, C. L., et al. (2006). Arabidopsis cell wall proteome defined using multidimensional protein identification technology. Proteomics 6 (1), 301–311. doi: 10.1002/pmic.200500046
Benedetti, M., Verrascina, I., Pontiggia, D., Locci, F., Mattei, B., De Lorenzo, G., et al. (2018). Four Arabidopsis berberine bridge enzyme-like proteins are specific oxidases that inactivate the elicitor-active oligogalacturonides. Plant J. 94 (2), 260–273. doi: 10.1111/tpj.13852
Ben-Tov, D., Abraham, Y., Stav, S., Thompson, K., Loraine, A., Elbaum, R., et al. (2015). COBRA-LIKE2, a member of the glycosylphosphatidylinositol-anchored COBRA-LIKE family, plays a role in cellulose deposition in Arabidopsis seed coat mucilage secretory cells. Plant Physiol. 167 (3), 711–724. doi: 10.1104/pp.114.240671
Ben-Tov, D., Idan-Molakandov, A., Hugger, A., Ben-Shlush, I., Gunl, M., Yang, B., et al. (2018). The role of COBRA-LIKE 2 function, as part of the complex network of interacting pathways regulating Arabidopsis seed mucilage polysaccharide matrix organization. Plant J. 94 (3), 497–512. doi: 10.1111/tpj.13871
Bhave, N. S., Veley, K. M., Nadeau, J. A., Lucas, J. R., Bhave, S. L., Sack, F. D. (2009). Too many mouths promotes cell fate progression in stomatal development of Arabidopsis stems. Planta 229 (2), 357–367. doi: 10.1007/s00425-008-0835-9
Borner, G. H., Lilley, K. S., Stevens, T. J., Dupree, P. (2003). Identification of glycosylphosphatidylinositol-anchored proteins in Arabidopsis. A proteomic and genomic analysis. Plant Physiol. 132 (2), 568–577. doi: 10.1104/pp.103.021170
Borner, G. H., Sherrier, D. J., Stevens, T. J., Arkin, I. T., Dupree, P. (2002). Prediction of glycosylphosphatidylinositol-anchored proteins in Arabidopsis. A genomic analysis. Plant Physiol. 129 (2), 486–499. doi: 10.1104/pp.010884
Breiden, M., Simon, R. (2016). Q&A: how does peptide signaling direct plant development? BMC Biol. 14, 58. doi: 10.1186/s12915-016-0280-3
Bundy, M. G., Kosentka, P. Z., Willet, A. H., Zhang, L., Miller, E., Shpak, E. D. (2016). A mutation in the catalytic subunit of the glycosylphosphatidylinositol transamidase disrupts growth, fertility, and stomata formation. Plant Physiol. 171 (2), 974–985. doi: 10.1104/pp.16.00339
Bush, S. J., Chen, L., Tovar-Corona, J. M., Urrutia, A. O. (2017). Alternative splicing and the evolution of phenotypic novelty. Philos. Trans. R. Soc. Lond. B. Biol. Sci. 372 (1713). doi: 10.1098/rstb.2015.0474
Butikofer, P., Malherbe, T., Boschung, M., Roditi, I. (2001). GPI-anchored proteins: now you see ‘em, now you don’t. FASEB J. 15 (2), 545–548. doi: 10.1096/fj.00-0415hyp
Cagnola, J. I., Dumont de Chassart, G. J., Ibarra, S. E., Chimenti, C., Ricardi, M. M., Delzer, B., et al. (2018). Reduced expression of selected FASCICLIN-LIKE ARABINOGALACTAN PROTEIN genes associates with the abortion of kernels in field crops of Zea mays (maize) and of Arabidopsis seeds. Plant Cell Environ. 41 (3), 661–674. doi: 10.1111/pce.13136
Cao, Y., Tang, X., Giovannoni, J., Xiao, F., Liu, Y. (2012). Functional characterization of a tomato COBRA-like gene functioning in fruit development and ripening. BMC Plant Biol. 12, 211. doi: 10.1186/1471-2229-12-211
Capron, A., Gourgues, M., Neiva, L. S., Faure, J. E., Berger, F., Pagnussat, G., et al. (2008). Maternal control of male-gamete delivery in Arabidopsis involves a putative GPI-anchored protein encoded by the LORELEI gene. Plant Cell 20 (11), 3038–3049. doi: 10.1105/tpc.108.061713
Chardin, C., Schenk, S. T., Hirt, H., Colcombet, J., Krapp, A. (2017). Review: mitogen-activated protein kinases in nutritional signaling in Arabidopsis. Plant Sci. 260, 101–108. doi: 10.1016/j.plantsci.2017.04.006
Chen, J., Chen, X., Zhang, Q., Zhang, Y., Ou, X., An, L., et al. (2018). A cold-induced pectin methyl-esterase inhibitor gene contributes negatively to freezing tolerance but positively to salt tolerance in Arabidopsis. J. Plant Physiol. 222, 67–78. doi: 10.1016/j.jplph.2018.01.003
Cheng, Y., Zhou, W., El Sheery, N. I., Peters, C., Li, M., Wang, X., et al. (2011). Characterization of the Arabidopsis glycerophosphodiester phosphodiesterase (GDPD) family reveals a role of the plastid-localized AtGDPD1 in maintaining cellular phosphate homeostasis under phosphate starvation. Plant J. 66 (5), 781–795. doi: 10.1111/j.1365-313X.2011.04538.x
Coimbra, S., Costa, M., Jones, B., Mendes, M. A., Pereira, L. G. (2009). Pollen grain development is compromised in Arabidopsis agp6 agp11 null mutants. J. Exp. Bot. 60 (11), 3133–3142. doi: 10.1093/jxb/erp148
Coimbra, S., Costa, M., Mendes, M. A., Pereira, A. M., Pinto, J., Pereira, L. G. (2010). Early germination of Arabidopsis pollen in a double null mutant for the arabinogalactan protein genes AGP6 and AGP11. Sex. Plant Reprod. 23 (3), 199–205. doi: 10.1007/s00497-010-0136-x
Costa, M., Nobre, M. S., Becker, J. D., Masiero, S., Amorim, M. I., Pereira, L. G., et al. (2013). Expression-based and co-localization detection of arabinogalactan protein 6 and arabinogalactan protein 11 interactors in Arabidopsis pollen and pollen tubes. BMC Plant Biol. 13, 7. doi: 10.1186/1471-2229-13-7
Davies, A., Kadurin, I., Alvarez-Laviada, A., Douglas, L., Nieto-Rostro, M., Bauer, C. S., et al. (2010). The alpha2delta subunits of voltage-gated calcium channels form GPI-anchored proteins, a posttranslational modification essential for function. Proc. Natl. Acad. Sci. U.S.A. 107 (4), 1654–1659. doi: 10.1073/pnas.0908735107
De Benedictis, M., Bleve, G., Faraco, M., Stigliano, E., Grieco, F., Piro, G., et al. (2013). AtSYP51/52 functions diverge in the post-Golgi traffic and differently affect vacuolar sorting. Mol. Plant 6 (3), 916–930. doi: 10.1093/mp/sss117
Debono, A., Yeats, T. H., Rose, J. K., Bird, D., Jetter, R., Kunst, L., et al. (2009). Arabidopsis LTPG is a glycosylphosphatidylinositol-anchored lipid transfer protein required for export of lipids to the plant surface. Plant Cell 21 (4), 1230–1238. doi: 10.1105/tpc.108.064451
Dinneny, J. R., Long, T. A., Wang, J. Y., Jung, J. W., Mace, D., Pointer, S., et al. (2008). Cell identity mediates the response of Arabidopsis roots to abiotic stress. Science 320 (5878), 942–945. doi: 10.1126/science.1153795
Duan, Q., Kita, D., Li, C., Cheung, A. Y., Wu, H. M. (2010). FERONIA receptor-like kinase regulates RHO GTPase signaling of root hair development. Proc. Natl. Acad. Sci. U.S.A. 107 (41), 17821–17826. doi: 10.1073/pnas.1005366107
Edstam, M. M., Edqvist, J. (2014). Involvement of GPI-anchored lipid transfer proteins in the development of seed coats and pollen in Arabidopsis thaliana. Physiol. Plant. 152 (1), 32–42. doi: 10.1111/ppl.12156
Eisenhaber, B., Bork, P., Eisenhaber, F. (1998). Sequence properties of GPI-anchored proteins near the omega-site: constraints for the polypeptide binding site of the putative transamidase. Protein Eng. 11 (12), 1155–1161. doi: 10.1093/protein/11.12.1155
Eisenhaber, B., Bork, P., Eisenhaber, F. (2001). Post-translational GPI lipid anchor modification of proteins in kingdoms of life: analysis of protein sequence data from complete genomes. Protein Eng. 14 (1), 17–25. doi: 10.1093/protein/14.1.17
Ellis, M., Egelund, J., Schultz, C. J., Bacic, A. (2010). Arabinogalactan-proteins: key regulators at the cell surface? Plant Physiol. 153 (2), 403–419. doi: 10.1104/pp.110.156000
Elortza, F., Nuhse, T. S., Foster, L. J., Stensballe, A., Peck, S. C., Jensen, O. N. (2003). Proteomic analysis of glycosylphosphatidylinositol-anchored membrane proteins. Mol. Cell. Proteomics 2 (12), 1261–1270. doi: 10.1074/mcp.M300079-MCP200
Escobar-Restrepo, J. M., Huck, N., Kessler, S., Gagliardini, V., Gheyselinck, J., Yang, W. C., et al. (2007). The feronia receptor-like kinase mediates male-female interactions during pollen tube reception. Science 317 (5838), 656–660. doi: 10.1126/science.1143562
Eudes, A., Mouille, G., Thevenin, J., Goyallon, A., Minic, Z., Jouanin, L. (2008). Purification, cloning and functional characterization of an endogenous beta-glucuronidase in Arabidopsis thaliana. Plant Cell Physiol. 49 (9), 1331–1341. doi: 10.1093/pcp/pcn108
Ezaki, B., Sasaki, K., Matsumoto, H., Nakashima, S. (2005). Functions of two genes in aluminium (Al) stress resistance: repression of oxidative damage by the AtBCB gene and promotion of efflux of Al ions by the NtGDI1gene. J. Exp. Bot. 56 (420), 2661–2671. doi: 10.1093/jxb/eri259
Fahlberg, P., Buhot, N., Johansson, O. N., Andersson, M. X. (2019). Involvement of lipid transfer proteins in resistance against a non-host powdery mildew in Arabidopsis thaliana. Mol. Plant Pathol. 20 (1), 69–77. doi: 10.1111/mpp.12740
Fankhauser, N., Maser, P. (2005). Identification of GPI anchor attachment signals by a Kohonen self-organizing map. Bioinformatics 21 (9), 1846–1852. doi: 10.1093/bioinformatics/bti299
Feng, W., Kita, D., Peaucelle, A., Cartwright, H. N., Doan, V., Duan, Q., et al. (2018). The FERONIA receptor kinase maintains cell-wall integrity during salt stress through Ca(2+) signaling. Curr. Biol. 28 (5), 666–75 e5. doi: 10.1016/j.cub.2018.01.023
Filichkin, S. A., Priest, H. D., Givan, S. A., Shen, R., Bryant, D. W., Fox, S. E., et al. (2010). Genome-wide mapping of alternative splicing in Arabidopsis thaliana. Genome Res. 20 (1), 45–58. doi: 10.1101/gr.093302.109
Foresti, O., Silva, L. L., Denecke, J. (2006). Overexpression of the Arabidopsis syntaxin PEP12/SYP21 inhibits transport from the prevacuolar compartment to the lytic vacuole in vivo. Plant Cell 18 (9), 2275–2293. doi: 10.1105/tpc.105.040279
Fujihara, Y., Ikawa, M. (2016). GPI-AP release in cellular, developmental, and reproductive biology. J. Lipid Res. 57 (4), 538–545. doi: 10.1194/jlr.R063032
Fulton, L., Batoux, M., Vaddepalli, P., Yadav, R. K., Busch, W., Andersen, S. U., et al. (2009). Detorqueo, Quirky, and Zerzaust represent novel components involved in organ development mediated by the receptor-like kinase Strubbelig in Arabidopsis thaliana. PLoS Genet. 5 (1), e1000355. doi: 10.1371/journal.pgen.1000355
Gao, H., Li, R., Guo, Y. (2017). Arabidopsis aspartic proteases A36 and A39 play roles in plant reproduction. Plant Signal. Behav. 12 (4), e1304343. doi: 10.1080/15592324.2017.1304343
Gao, H., Zhang, Y., Wang, W., Zhao, K., Liu, C., Bai, L., et al. (2017). Two membrane-anchored aspartic proteases contribute to pollen and ovule development. Plant Physiol. 173 (1), 219–239. doi: 10.1104/pp.16.01719
Geisler, M., Nadeau, J., Sack, F. D. (2000). Oriented asymmetric divisions that generate the stomatal spacing pattern in Arabidopsis are disrupted by the too many mouths mutation. Plant Cell 12 (11), 2075–2086. doi: 10.1105/tpc.12.11.2075
Geisler, M., Yang, M., Sack, F. D. (1998). Divergent regulation of stomatal initiation and patterning in organ and suborgan regions of the Arabidopsis mutants too many mouths and four lips. Planta 205 (4), 522–530. doi: 10.1007/s004250050351
Geldner, N., Robatzek, S. (2008). Plant receptors go endosomal: a moving view on signal transduction. Plant Physiol. 147 (4), 1565–1574. doi: 10.1104/pp.108.120287
Gillmor, C. S., Lukowitz, W., Brininstool, G., Sedbrook, J. C., Hamann, T., Poindexter, P., et al. (2005). Glycosylphosphatidylinositol-anchored proteins are required for cell wall synthesis and morphogenesis in Arabidopsis. Plant Cell 17 (4), 1128–1140. doi: 10.1105/tpc.105.031815
Griffiths, J. S., Crepeau, M. J., Ralet, M. C., Seifert, G. J., North, H. M. (2016). Dissecting seed mucilage adherence mediated by FEI2 and SOS5. Front. Plant Sci. 7, 1073. doi: 10.3389/fpls.2016.01073
Grojean, J., Downes, B. (2010). Riboswitches as hormone receptors: hypothetical cytokinin-binding riboswitches in Arabidopsis thaliana. Biol. Direct 5, 60. doi: 10.1186/1745-6150-5-60
Guan, P., Wang, J., Xie, C., Wu, C., Yang, G., Yan, K., et al. (2019). SES1 positively regulates heat stress resistance in Arabidopsis. Biochem. Biophys. Res. Commun. 513 (3), 582–588. doi: 10.1016/j.bbrc.2019.04.015
Guenin, S., Mareck, A., Rayon, C., Lamour, R., Assoumou Ndong, Y., Domon, J. M., et al. (2011). Identification of pectin methylesterase 3 as a basic pectin methylesterase isoform involved in adventitious rooting in Arabidopsis thaliana. New Phytol. 192 (1), 114–126. doi: 10.1111/j.1469-8137.2011.03797.x
Gunl, M., Pauly, M. (2011). AXY3 encodes a alpha-xylosidase that impacts the structure and accessibility of the hemicellulose xyloglucan in Arabidopsis plant cell walls. Planta 233 (4), 707–719. doi: 10.1007/s00425-010-1330-7
Guo, H., Nolan, T. M., Song, G., Liu, S., Xie, Z., Chen, J., et al. (2018). FERONIA receptor kinase contributes to plant immunity by suppressing jasmonic acid signaling in Arabidopsis thaliana. Curr. Biol. 28 (20), 3316–3324. doi: 10.1016/j.cub.2018.07.078
Guo, L., Yang, H., Zhang, X., Yang, S. (2013). Lipid transfer protein 3 as a target of MYB96 mediates freezing and drought stress in Arabidopsis. J. Exp. Bot. 64 (6), 1755–1767. doi: 10.1093/jxb/ert040
Harpaz-Saad, S., Western, T. L., Kieber, J. J. (2012). The FEI2-SOS5 pathway and CELLULOSE SYNTHASE 5 are required for cellulose biosynthesis in the Arabidopsis seed coat and affect pectin mucilage structure. Plant Signal. Behav. 7 (2), 285–288. doi: 10.4161/psb.18819
Hart, P. J., Nersissian, A. M., Herrmann, R. G., Nalbandyan, R. M., Valentine, J. S., Eisenberg, D. (1996). A missing link in cupredoxins: crystal structure of cucumber stellacyanin at 1.6 A resolution. Protein Sci. 5 (11), 2175–2183. doi: 10.1002/pro.5560051104
Hayashi, S., Ishii, T., Matsunaga, T., Tominaga, R., Kuromori, T., Wada, T., et al. (2008). The glycerophosphoryl diester phosphodiesterase-like proteins SHV3 and its homologs play important roles in cell wall organization. Plant Cell Physiol. 49 (10), 1522–1535. doi: 10.1093/pcp/pcn120
Hellwing, C., Schoeniger, A., Roessler, C., Leimert, A., Schumann, J. (2018). Lipid raft localization of TLR2 and its co-receptors is independent of membrane lipid composition. PeerJ. 6, e4212. doi: 10.7717/peerj.4212
Hijazi, M., Roujol, D., Nguyen-Kim, H., Del Rocio Cisneros Castillo, L., Saland, E., Jamet, E., et al. (2014). Arabinogalactan protein 31 (AGP31), a putative network-forming protein in Arabidopsis thaliana cell walls? Ann. Bot. 114 (6), 1087–1097. doi: 10.1093/aob/mcu038
Hochholdinger, F., Wen, T. J., Zimmermann, R., Chimot-Marolle, P., da Costa e Silva, O., Bruce, W., et al. (2008). The maize (Zea mays L.) roothairless3 gene encodes a putative GPI-anchored, monocot-specific, COBRA-like protein that significantly affects grain yield. Plant J. 54 (5), 888–898. doi: 10.1111/j.1365-313X.2008.03459.x
Hong, D., Jeon, B. W., Kim, S. Y., Hwang, J. U., Lee, Y. (2016). The ROP2-RIC7 pathway negatively regulates light-induced stomatal opening by inhibiting exocyst subunit Exo70B1 in Arabidopsis. New Phytol. 209 (2), 624–635. doi: 10.1111/nph.13625
Hou, Y., Guo, X., Cyprys, P., Zhang, Y., Bleckmann, A., Cai, L., et al. (2016). Maternal ENODLs are required for pollen tube reception in Arabidopsis. Curr. Biol. 26 (17), 2343–2350. doi: 10.1016/j.cub.2016.06.053
Hwang, J. U., Jeon, B. W., Hong, D., Lee, Y. (2011). Active ROP2 GTPase inhibits ABA- and CO2-induced stomatal closure. Plant Cell Environ. 34 (12), 2172–2182. doi: 10.1111/j.1365-3040.2011.02413.x
Ichikawa, M., Nakai, Y., Arima, K., Nishiyama, S., Hirano, T., Sato, M. H. (2015). A VAMP-associated protein, PVA31 is involved in leaf senescence in Arabidopsis. Plant Signal. Behav. 10 (3), e990847. doi: 10.4161/15592324.2014.990847
Ito, S., Suzuki, Y., Miyamoto, K., Ueda, J., Yamaguchi, I. (2005). AtFLA11, a fasciclin-like arabinogalactan-protein, specifically localized in sclerenchyma cells. Biosci. Biotechnol. Biochem. 69 (10), 1963–1969. doi: 10.1271/bbb.69.1963
Jakoby, M. J., Weinl, C., Pusch, S., Kuijt, S. J., Merkle, T., Dissmeyer, N., et al. (2006). Analysis of the subcellular localization, function, and proteolytic control of the Arabidopsis cyclin-dependent kinase inhibitor ICK1/KRP1. Plant Physiol. 141 (4), 1293–1305. doi: 10.1104/pp.106.081406
Jeon, B. W., Hwang, J. U., Hwang, Y., Song, W. Y., Fu, Y., Gu, Y., et al. (2008). The Arabidopsis small G protein ROP2 is activated by light in guard cells and inhibits light-induced stomatal opening. Plant Cell 20 (1), 75–87. doi: 10.1105/tpc.107.054544
Jeong, I. S., Lee, S., Bonkhofer, F., Tolley, J., Fukudome, A., Nagashima, Y., et al. (2018). Purification and characterization of Arabidopsis thaliana oligosaccharyltransferase complexes from the native host: a protein super-expression system for structural studies. Plant J. 94 (1), 131–145. doi: 10.1111/tpj.13847
Ji, H., Wang, Y., Cloix, C., Li, K., Jenkins, G. I., Wang, S., et al. (2015). The Arabidopsis RCC1 family protein TCF1 regulates freezing tolerance and cold acclimation through modulating lignin biosynthesis. PLoS Genet. 11 (9), e1005471. doi: 10.1371/journal.pgen.1005471
Johnson, K. L., Kibble, N. A., Bacic, A. (2011). Schultz CJ. A fasciclin-like arabinogalactan-protein (FLA) mutant of Arabidopsis thaliana, fla1, shows defects in shoot regeneration. PLoS One 6 (9), e25154. doi: 10.1371/journal.pone.0025154
Jolivet, P., Roux, E., D’Andrea, S., Davanture, M., Negroni, L., Zivy, M., et al. (2004). Protein composition of oil bodies in Arabidopsis thaliana ecotype WS. Plant Physiol. Biochem. 42 (6), 501–509. doi: 10.1016/j.plaphy.2004.04.006
Jones, M. A., Raymond, M. J., Smirnoff, N. (2006). Analysis of the root-hair morphogenesis transcriptome reveals the molecular identity of six genes with roles in root-hair development in Arabidopsis. Plant J. 45 (1), 83–100. doi: 10.1111/j.1365-313X.2005.02609.x
Jorda, L., Sopena-Torres, S., Escudero, V., Nunez-Corcuera, B., Delgado-Cerezo, M., Torii, K. U., et al. (2016). ERECTA and BAK1 receptor like kinases interact to regulate immune responses in Arabidopsis. Front. Plant Sci. 7, 897. doi: 10.3389/fpls.2016.00897
Kawagoe, K., Kitamura, D., Okabe, M., Taniuchi, I., Ikawa, M., Watanabe, T., et al. (1996). Glycosylphosphatidylinositol-anchor-deficient mice: implications for clonal dominance of mutant cells in paroxysmal nocturnal hemoglobinuria. Blood 87 (9), 3600–3606.
Khan, J. A., Wang, Q., Sjolund, R. D., Schulz, A., Thompson, G. A. (2007). An early nodulin-like protein accumulates in the sieve element plasma membrane of Arabidopsis. Plant Physiol. 143 (4), 1576–1589. doi: 10.1104/pp.106.092296
Kim, D. S., Kim, J. B., Goh, E. J., Kim, W. J., Kim, S. H., Seo, Y. W., et al. (2011). Antioxidant response of Arabidopsis plants to gamma irradiation: genome-wide expression profiling of the ROS scavenging and signal transduction pathways. J. Plant Physiol. 168 (16), 1960–1971. doi: 10.1016/j.jplph.2011.05.008
Kim, H., Lee, S. B., Kim, H. J., Min, M. K., Hwang, I., Suh, M. C. (2012). Characterization of glycosylphosphatidylinositol-anchored lipid transfer protein 2 (LTPG2) and overlapping function between LTPG/LTPG1 and LTPG2 in cuticular wax export or accumulation in Arabidopsis thaliana. Plant Cell Physiol. 53 (8), 1391–1403. doi: 10.1093/pcp/pcs083
Kinoshita, T. (2014a). Enzymatic mechanism of GPI anchor attachment clarified. Cell Cycle 13 (12), 1838–1839. doi: 10.4161/cc.29379
Kinoshita, T. (2014b). Biosynthesis and deficiencies of glycosylphosphatidylinositol. Proc. Jpn. Acad., Ser. B, Phys. Biol. Sci. 90 (4), 130–143. doi: 10.2183/pjab.90.130
Kinoshita, T., Fujita, M. (2016). Biosynthesis of GPI-anchored proteins: special emphasis on GPI lipid remodeling. J. Lipid Res. 57 (1), 6–24. doi: 10.1194/jlr.R063313
Ko, J. H., Kim, J. H., Jayanty, S. S., Howe, G. A., Han, K. H. (2006). Loss of function of COBRA, a determinant of oriented cell expansion, invokes cellular defence responses in Arabidopsis thaliana. J. Exp. Bot. 57 (12), 2923–2936. doi: 10.1093/jxb/erl052
Kobe, B., Kajava, A. V. (2001). The leucine-rich repeat as a protein recognition motif. Curr. Opin. Struct. Biol. 11 (6), 725–732. doi: 10.1016/S0959-440X(01)00266-4
Lebreton, S., Zurzolo, C., Paladino, S. (2018). Organization of GPI-anchored proteins at the cell surface and its physiopathological relevance. Crit. Rev. Biochem. Mol. Biol. 53 (4), 403–419. doi: 10.1080/10409238.2018.1485627
Lee, Y., Rio, D. C. (2015). Mechanisms and regulation of alternative pre-mRNA splicing. Annu. Rev. Biochem. 84, 291–323. doi: 10.1146/annurev-biochem-060614-034316
Lee, S. B., Suh, M. C. (2018). Disruption of glycosylphosphatidylinositol-anchored lipid transfer protein 15 affects seed coat permeability in Arabidopsis. Plant J. 96 (6), 1206–1217. doi: 10.1111/tpj.14101
Lee, J. S., Hnilova, M., Maes, M., Lin, Y. C., Putarjunan, A., Han, S. K., et al. (2015). Competitive binding of antagonistic peptides fine-tunes stomatal patterning. Nature 522 (7557), 439–443. doi: 10.1038/nature14561
Lee, J. S., Kuroha, T., Hnilova, M., Khatayevich, D., Kanaoka, M. M., McAbee, J. M., et al. (2012). Direct interaction of ligand-receptor pairs specifying stomatal patterning. Genes Dev. 26 (2), 126–136. doi: 10.1101/gad.179895.111
Legler, D. F., Doucey, M. A., Schneider, P., Chapatte, L., Bender, F. C., Bron, C. (2005). Differential insertion of GPI-anchored GFPs into lipid rafts of live cells. FASEB J. 19 (1), 73–75. doi: 10.1096/fj.03-1338fje
Lerouxel, O., Mouille, G., Andeme-Onzighi, C., Bruyant, M. P., Seveno, M., Loutelier-Bourhis, C., et al. (2005). Mutants in defective glycosylation, an Arabidopsis homolog of an oligosaccharyltransferase complex subunit, show protein underglycosylation and defects in cell differentiation and growth. Plant J. 42 (4), 455–468. doi: 10.1111/j.1365-313X.2005.02392.x
Leshem, Y., Golani, Y., Kaye, Y., Levine, A. (2010). Reduced expression of the v-SNAREs AtVAMP71/AtVAMP7C gene family in Arabidopsis reduces drought tolerance by suppression of abscisic acid-dependent stomatal closure. J. Exp. Bot. 61 (10), 2615–2622. doi: 10.1093/jxb/erq099
Levitin, B., Richter, D., Markovich, I., Zik, M. (2008). Arabinogalactan proteins 6 and 11 are required for stamen and pollen function in Arabidopsis. Plant J. 56 (3), 351–363. doi: 10.1111/j.1365-313X.2008.03607.x
Li, S., Ge, F. R., Xu, M., Zhao, X. Y., Huang, G. Q., Zhou, L. Z., et al. (2013). Arabidopsis COBRA-LIKE 10, a GPI-anchored protein, mediates directional growth of pollen tubes. Plant J. 74 (3), 486–497. doi: 10.1111/tpj.12139
Li, X., Wang, D., Chen, Z., Lu, E., Wang, Z., Duan, J., et al. (2015). Galphai1 and Galphai3 regulate macrophage polarization by forming a complex containing CD14 and Gab1. Proc. Natl. Acad. Sci. U.S.A. 112 (15), 4731–4736. doi: 10.1073/pnas.1503779112
Li, C., Wu, H. M., Cheung, A. Y. (2016). FERONIA and her pals: functions and mechanisms. Plant Physiol. 171 (4), 2379–2392. doi: 10.1104/pp.16.00667
Li, C., Yeh, F. L., Cheung, A. Y., Duan, Q., Kita, D., Liu, M. C., et al. (2015). Glycosylphosphatidylinositol-anchored proteins as chaperones and co-receptors for FERONIA receptor kinase signaling in Arabidopsis. eLife 4. doi: 10.7554/eLife.06587
Li, J., Yu, M., Geng, L. L., Zhao, J. (2010). The fasciclin-like arabinogalactan protein gene, FLA3, is involved in microspore development of Arabidopsis. Plant J. 64 (3), 482–497. doi: 10.1111/j.1365-313X.2010.04344.x
Liang, X., Ding, P., Lian, K., Wang, J., Ma, M., Li, L., et al. (2016). Arabidopsis heterotrimeric G proteins regulate immunity by directly coupling to the FLS2 receptor. eLife 5, e13568. doi: 10.7554/eLife.13568
Liao, H., Tang, R., Zhang, X., Luan, S., Yu, F. (2017). FERONIA receptor kinase at the crossroads of hormone signaling and stress responses. Plant Cell Physiol. 58 (7), 1143–1150. doi: 10.1093/pcp/pcx048
Lin, W. D., Liao, Y. Y., Yang, T. J., Pan, C. Y., Buckhout, T. J., Schmidt, W. (2011). Coexpression-based clustering of Arabidopsis root genes predicts functional modules in early phosphate deficiency signaling. Plant Physiol. 155 (3), 1383–1402. doi: 10.1104/pp.110.166520
Lin, G., Zhang, L., Han, Z., Yang, X., Liu, W., Li, E., et al. (2017). A receptor-like protein acts as a specificity switch for the regulation of stomatal development. Genes Dev. 31 (9), 927–938. doi: 10.1101/gad.297580.117
Link, M., Rausch, T., Greiner, S. (2004). In Arabidopsis thaliana, the invertase inhibitors AtC/VIF1 and 2 exhibit distinct target enzyme specificities and expression profiles. FEBS Lett. 573 (1–3), 105–109. doi: 10.1016/j.febslet.2004.07.062
Liu, Y., Huang, X., Li, M., He, P., Zhang, Y. (2016). Loss-of-function of Arabidopsis receptor-like kinase BIR1 activates cell death and defense responses mediated by BAK1 and SOBIR1. New Phytol. 212 (3), 637–645. doi: 10.1111/nph.14072
Losada, J. M., Herrero, M. (2019). Arabinogalactan proteins mediate intercellular crosstalk in the ovule of apple flowers. Plant Reprod. 32, 291–305. doi: 10.1007/s00497-019-00370-z
Luo, H., Lyznik, L. A., Gidoni, D., Hodges, T. K. (2000). FLP-mediated recombination for use in hybrid plant production. Plant J. 23 (3), 423–430. doi: 10.1046/j.1365-313x.2000.00782.x
MacMillan, C. P., Mansfield, S. D., Stachurski, Z. H., Evans, R., Southerton, S. G. (2010). Fasciclin-like arabinogalactan proteins: specialization for stem biomechanics and cell wall architecture in Arabidopsis and Eucalyptus. Plant J. 62 (4), 689–703. doi: 10.1111/j.1365-313X.2010.04181.x
Marzec, M., Szarejko, I., Melzer, M. (2015). Arabinogalactan proteins are involved in root hair development in barley. J. Exp. Bot. 66 (5), 1245–1257. doi: 10.1093/jxb/eru475
Membre, N., Bernier, F., Staiger, D., Berna, A. (2000). Arabidopsis thaliana germin-like proteins: common and specific features point to a variety of functions. Planta 211 (3), 345–354. doi: 10.1007/s004250000277
Meng, X., Chen, X., Mang, H., Liu, C., Yu, X., Gao, X., et al. (2015). Differential function of Arabidopsis SERK family receptor-like kinases in stomatal patterning. Curr. Biol. 25 (18), 2361–2372. doi: 10.1016/j.cub.2015.07.068
Meng, X., Wang, H., He, Y., Liu, Y., Walker, J. C., Torii, K. U., et al. (2012). A MAPK cascade downstream of ERECTA receptor-like protein kinase regulates Arabidopsis inflorescence architecture by promoting localized cell proliferation. Plant Cell 24 (12), 4948–4960. doi: 10.1105/tpc.112.104695
Miyagawa-Yamaguchi, A., Kotani, N., Honke, K. (2015). Each GPI-anchored protein species forms a specific lipid raft depending on its GPI attachment signal. Glycoconj. J. 32 (7), 531–540. doi: 10.1007/s10719-015-9595-5
Morsomme, P., Prescianotto-Baschong, C., Riezman, H. (2003). The ER v-SNAREs are required for GPI-anchored protein sorting from other secretory proteins upon exit from the ER. J. Cell Biol. 162 (3), 403–412. doi: 10.1083/jcb.200212101
Motose, H., Sugiyama, M., Fukuda, H. (2004). A proteoglycan mediates inductive interaction during plant vascular development. Nature 429 (6994), 873–878. doi: 10.1038/nature02613
Muller, K., Levesque-Tremblay, G., Bartels, S., Weitbrecht, K., Wormit, A., Usadel, B., et al. (2013). Demethylesterification of cell wall pectins in Arabidopsis plays a role in seed germination. Plant Physiol. 161 (1), 305–316. doi: 10.1104/pp.112.205724
Muniz, M., Zurzolo, C. (2014). Sorting of GPI-anchored proteins from yeast to mammals—common pathways at different sites? J. Cell Sci. 127 (Pt 13), 2793–2801. doi: 10.1242/jcs.148056
Murphy, E., Smith, S., De Smet, I. (2012). Small signaling peptides in Arabidopsis development: how cells communicate over a short distance. Plant Cell 24 (8), 3198–3217. doi: 10.1105/tpc.112.099010
Muschietti, J. P., Wengier, D. L. (2018). How many receptor-like kinases are required to operate a pollen tube. Curr. Opin. Plant Biol. 41, 73–82. doi: 10.1016/j.pbi.2017.09.008
Nersissian, A. M., Immoos, C., Hill, M. G., Hart, P. J., Williams, G., Herrmann, R. G., et al. (1998). Uclacyanins, stellacyanins, and plantacyanins are distinct subfamilies of phytocyanins: plant-specific mononuclear blue copper proteins. Protein Sci. 7 (9), 1915–1929. doi: 10.1002/pro.5560070907
Niu, E., Fang, S., Shang, X., Guo, W. (2018). Ectopic expression of GhCOBL9A, a cotton glycosyl-phosphatidyl inositol-anchored protein encoding gene, promotes cell elongation, thickening and increased plant biomass in transgenic Arabidopsis. Mol. Genet. Genomics 293 (5), 1191–1204. doi: 10.1007/s00438-018-1452-3
Niu, E., Shang, X., Cheng, C., Bao, J., Zeng, Y., Cai, C., et al. (2015). Comprehensive analysis of the COBRA-Like (COBL) gene family in Gossypium identifies two COBLs potentially associated with fiber quality. PLoS One 10 (12), e0145725. doi: 10.1371/journal.pone.0145725
Orihashi, K., Tojo, H., Okawa, K., Tashima, Y., Morita, T., Kondoh, G. (2012). Mammalian carboxylesterase (CES) releases GPI-anchored proteins from the cell surface upon lipid raft fluidization. Biol. Chem. 393 (3), 169–176. doi: 10.1515/hsz-2011-0269
Oxley, D., Bacic, A. (1999). Structure of the glycosylphosphatidylinositol anchor of an arabinogalactan protein from Pyrus communis suspension-cultured cells. Proc. Natl. Acad. Sci. U.S.A. 96 (25), 14246–14251. doi: 10.1073/pnas.96.25.14246
Palacio-Lopez, K., Tinaz, B., Holzinger, A., Domozych, D. S. (2019). Arabinogalactan proteins and the extracellular matrix of charophytes: a sticky business. Front. Plant Sci. 10, 447. doi: 10.3389/fpls.2019.00447
Palareti, G., Legnani, C., Cosmi, B., Antonucci, E., Erba, N., Poli, D., et al. (2016). Comparison between different D-Dimer cutoff values to assess the individual risk of recurrent venous thromboembolism: analysis of results obtained in the DULCIS study. Int. J. Lab. Hematol. 38 (1), 42–49. doi: 10.1111/ijlh.12426
Paparella, C., Savatin, D. V., Marti, L., De Lorenzo, G., Ferrari, S. (2014). The Arabidopsis LYSIN MOTIF-CONTAINING RECEPTOR-LIKE KINASE3 regulates the cross talk between immunity and abscisic acid responses. Plant Physiol. 165 (1), 262–276. doi: 10.1104/pp.113.233759
Pariani, S., Contreras, M., Rossi, F. R., Sander, V., Corigliano, M. G., Simon, F., et al. (2016). Characterization of a novel Kazal-type serine proteinase inhibitor of Arabidopsis thaliana. Biochimie 123, 85–94. doi: 10.1016/j.biochi.2016.02.002
Parker, J. S., Cavell, A. C., Dolan, L., Roberts, K., Grierson, C. S. (2000). Genetic interactions during root hair morphogenesis in Arabidopsis. Plant Cell 12 (10), 1961–1974. doi: 10.1105/tpc.12.10.1961
Pearce, G., Moura, D. S., Stratmann, J., Ryan, C. A., Jr. (2001). RALF, a 5-kDa ubiquitous polypeptide in plants, arrests root growth and development. Proc. Natl. Acad. Sci. U.S.A. 98 (22), 12843–12847. doi: 10.1073/pnas.201416998
Pereira, A. M., Lopes, A. L., Coimbra, S. (2016b). JAGGER, an AGP essential for persistent synergid degeneration and polytubey block in Arabidopsis. Plant Signal. Behav. 11 (8), e1209616. doi: 10.1080/15592324.2016.1209616
Pereira, A. M., Nobre, M. S., Pinto, S. C., Lopes, A. L., Costa, M. L., Masiero, S., et al. (2016a). “Love Is Strong, and You’re so Sweet”: JAGGER is essential for persistent synergid degeneration and polytubey block in Arabidopsis thaliana. Mol. Plant 9 (4), 601–614. doi: 10.1016/j.molp.2016.01.002
Pierleoni, A., Martelli, P. L., Casadio, R. (2008). PredGPI: a GPI-anchor predictor. BMC Bioinformatics 9, 392. doi: 10.1186/1471-2105-9-392
Poisson, G., Chauve, C., Chen, X., Bergeron, A. (2007). FragAnchor: a large-scale predictor of glycosylphosphatidylinositol anchors in eukaryote protein sequences by qualitative scoring. Genomics Proteomics Bioinformatics 5 (2), 121–130. doi: 10.1016/S1672-0229(07)60022-9
Qin, C., Li, Y., Gan, J., Wang, W., Zhang, H., Liu, Y., et al. (2013). OsDGL1, a homolog of an oligosaccharyltransferase complex subunit, is involved in N-glycosylation and root development in rice. Plant Cell Physiol. 54 (1), 129–137. doi: 10.1093/pcp/pcs159
Qu, H., Guan, Y., Wang, Y., Zhang, S. (2017). PLC-mediated signaling pathway in pollen tubes regulates the gametophytic self-incompatibility of Pyrus species. Front. Plant Sci. 8, 1164. doi: 10.3389/fpls.2017.01164
Rasmussen, C. G., Humphries, J. A., Smith, L. G. (2011). Determination of symmetric and asymmetric division planes in plant cells. Annu. Rev. Plant Biol. 62, 387–409. doi: 10.1146/annurev-arplant-042110-103802
Reboul, R., Geserick, C., Pabst, M., Frey, B., Wittmann, D., Lutz-Meindl, U., et al. (2011). Down-regulation of UDP-glucuronic acid biosynthesis leads to swollen plant cell walls and severe developmental defects associated with changes in pectic polysaccharides. J. Biol. Chem. 286 (46), 39982–39992. doi: 10.1074/jbc.M111.255695
Reddy, A. S., Marquez, Y., Kalyna, M., Barta, A. (2013). Complexity of the alternative splicing landscape in plants. Plant Cell 25 (10), 3657–3683. doi: 10.1105/tpc.113.117523
Ringli, C., Baumberger, N., Keller, B. (2005). The Arabidopsis root hair mutants der2-der9 are affected at different stages of root hair development. Plant Cell Physiol. 46 (7), 1046–1053. doi: 10.1093/pcp/pci115
Roudier, F., Fernandez, A. G., Fujita, M., Himmelspach, R., Borner, G. H., Schindelman, G., et al. (2005). COBRA, an Arabidopsis extracellular glycosyl-phosphatidyl inositol-anchored protein, specifically controls highly anisotropic expansion through its involvement in cellulose microfibril orientation. Plant Cell 17 (6), 1749–1763. doi: 10.1105/tpc.105.031732
Roudier, F., Schindelman, G., DeSalle, R., Benfey, P. N. (2002). The COBRA family of putative GPI-anchored proteins in Arabidopsis. A new fellowship in expansion. Plant Physiol. 130 (2), 538–548. doi: 10.1104/pp.007468
Saha, S., Anilkumar, A. A., Mayor, S. (2016). GPI-anchored protein organization and dynamics at the cell surface. J. Lipid Res. 57 (2), 159–175. doi: 10.1194/jlr.R062885
Sampedro, J., Pardo, B., Gianzo, C., Guitian, E., Revilla, G., Zarra, I. (2010). Lack of alpha-xylosidase activity in Arabidopsis alters xyloglucan composition and results in growth defects. Plant Physiol. 154 (3), 1105–1115. doi: 10.1104/pp.110.163212
Sampedro, J., Valdivia, E. R., Fraga, P., Iglesias, N., Revilla, G., Zarra, I. (2017). Soluble and membrane-bound beta-glucosidases are involved in trimming the xyloglucan backbone. Plant Physiol. 173 (2), 1017–1030. doi: 10.1104/pp.16.01713
Schilling, S., Stenzel, I., von Bohlen, A., Wermann, M., Schulz, K., Demuth, H. U., et al. (2007). Isolation and characterization of the glutaminyl cyclases from Solanum tuberosum and Arabidopsis thaliana: implications for physiological functions. Biol. Chem. 388 (2), 145–153. doi: 10.1515/BC.2007.016
Schindelman, G., Morikami, A., Jung, J., Baskin, T. I., Carpita, N. C., Derbyshire, P., et al. (2001). COBRA encodes a putative GPI-anchored protein, which is polarly localized and necessary for oriented cell expansion in Arabidopsis. Genes Dev. 15 (9), 1115–1127. doi: 10.1101/gad.879101
Schmidt, A., Su, Y. H., Kunze, R., Warner, S., Hewitt, M., Slocum, R. D., et al. (2004). UPS1 and UPS2 from Arabidopsis mediate high affinity transport of uracil and 5-fluorouracil. J. Biol. Chem. 279 (43), 44817–44824. doi: 10.1074/jbc.M405433200
Schultz, C. J., Ferguson, K. L., Lahnstein, J., Bacic, A. (2004). Post-translational modifications of arabinogalactan-peptides of Arabidopsis thaliana. Endoplasmic reticulum and glycosylphosphatidylinositol-anchor signal cleavage sites and hydroxylation of proline. J. Biol. Chem. 279 (44), 45503–45511. doi: 10.1074/jbc.M407594200
Schultz, C. J., Johnson, K. L., Currie, G., Bacic, A. (2000). The classical arabinogalactan protein gene family of Arabidopsis. Plant Cell 12 (9), 1751–1768. doi: 10.1105/tpc.12.9.1751
Schumann, R. R. (1992). Function of lipopolysaccharide (LPS)-binding protein (LBP) and CD14, the receptor for LPS/LBP complexes: a short review. Res. Immunol. 143 (1), 11–15. doi: 10.1016/0923-2494(92)80074-U
Sedbrook, J. C., Carroll, K. L., Hung, K. F., Masson, P. H., Somerville, C. R. (2002). The Arabidopsis SKU5 gene encodes an extracellular glycosyl phosphatidylinositol-anchored glycoprotein involved in directional root growth. Plant Cell 14 (7), 1635–1648. doi: 10.1105/tpc.002360
Seifert, G. J., Xue, H., Acet, T. (2014). The Arabidopsis thaliana faciclin like arabinogalactan protein 4 gene acts synergistically with abscisic acid signalling to control root growth. Ann. Bot. 114 (6), 1125–1133. doi: 10.1093/aob/mcu010
Senechal, F., L’Enfant, M., Domon, J. M., Rosiau, E., Crepeau, M. J., Surcouf, O., et al. (2015). Tuning of pectin methylesterification: pectin methylesterase inhibitor 7 modulates the processive activity of co-expressed pectin methylesterase 3 in a pH-dependent manner. J. Biol. Chem. 290 (38), 23320–23335. doi: 10.1074/jbc.M115.639534
Severing, E. I., van Dijk, A. D., van Ham, R. C. (2011). Assessing the contribution of alternative splicing to proteome diversity in Arabidopsis thaliana using proteomics data. BMC Plant Biol. 11 (1), 82. doi: 10.1186/1471-2229-11-82
Sezgin, E., Levental, I., Mayor, S., Eggeling, C. (2017). The mystery of membrane organization: composition, regulation and roles of lipid rafts. Nat. Rev. Mol. Cell Biol. 18 (6), 361–374. doi: 10.1038/nrm.2017.16
Shen, Q., Bourdais, G., Pan, H., Robatzek, S., Tang, D. (2017). Arabidopsis glycosylphosphatidylinositol-anchored protein LLG1 associates with and modulates FLS2 to regulate innate immunity. Proc. Natl. Acad. Sci. U.S.A. 114 (22), 5749–5754. doi: 10.1073/pnas.1614468114
Shimomura, S. (2006). Identification of a glycosylphosphatidylinositol-anchored plasma membrane protein interacting with the C-terminus of auxin-binding protein 1: a photoaffinity crosslinking study. Plant Mol. Biol. 60 (5), 663–677. doi: 10.1007/s11103-005-5471-1
Shirakawa, M., Ueda, H., Shimada, T., Koumoto, Y., Shimada, T. L., Kondo, M., et al. (2010). Arabidopsis Qa-SNARE SYP2 proteins localized to different subcellular regions function redundantly in vacuolar protein sorting and plant development. Plant J. 64 (6), 924–935. doi: 10.1111/j.1365-313X.2010.04394.x
Shiu, S. H., Bleecker, A. B. (2001). Plant receptor-like kinase gene family: diversity, function, and signaling. Sci. STKE 2001 (113), re22. doi: 10.1126/scisignal.1132001re22
Showalter, A. M., Basu, D. (2016). Glycosylation of arabinogalactan-proteins essential for development in Arabidopsis. Commun. Integr. Biol. 9 (3), e1177687. doi: 10.1080/19420889.2016.1177687
Siddique, S., Sobczak, M., Tenhaken, R., Grundler, F. M., Bohlmann, H. (2012). Cell wall ingrowths in nematode induced syncytia require UGD2 and UGD3. PLoS One 7 (7), e41515. doi: 10.1371/journal.pone.0041515
Silady, R. A., Ehrhardt, D. W., Jackson, K., Faulkner, C., Oparka, K., Somerville, C. R. (2008). The GRV2/RME-8 protein of Arabidopsis functions in the late endocytic pathway and is required for vacuolar membrane flow. Plant J. 53 (1), 29–41. doi: 10.1111/j.1365-313X.2007.03314.x
Simpson, C., Thomas, C., Findlay, K., Bayer, E., Maule, A. J. (2009). An Arabidopsis GPI-anchor plasmodesmal neck protein with callose binding activity and potential to regulate cell-to-cell trafficking. Plant Cell 21 (2), 581–594. doi: 10.1105/tpc.108.060145
Singh, S. K., Kumar, V., Srinivasan, R., Ahuja, P. S., Bhat, S. R., Sreenivasulu, Y. (2017). The TRAF mediated gametogenesis progression (TRAMGaP) gene is required for megaspore mother cell specification and gametophyte development. Plant Physiol. 175 (3), 1220–1237. doi: 10.1104/pp.17.00275
Smith, S. J., Kroon, J. T., Simon, W. J., Slabas, A. R., Chivasa, S. (2015). A novel function for Arabidopsis CYCLASE1 in programmed cell death revealed by isobaric tags for relative and absolute quantitation (iTRAQ) analysis of extracellular matrix proteins. Mol. Cell. Proteomics 14 (6), 1556–1568. doi: 10.1074/mcp.M114.045054
Sorek, N., Szemenyei, H., Sorek, H., Landers, A., Knight, H., Bauer, S., et al. (2015). Identification of MEDIATOR16 as the Arabidopsis COBRA suppressor MONGOOSE1. Proc. Natl. Acad. Sci. U.S.A. 112 (52), 16048–16053. doi: 10.1073/pnas.1521675112
Stegmann, M., Monaghan, J., Smakowska-Luzan, E., Rovenich, H., Lehner, A., Holton, N., et al. (2017). The receptor kinase FER is a RALF-regulated scaffold controlling plant immune signaling. Science 355 (6322), 287–289. doi: 10.1126/science.aal2541
Stevens, V. L. (1995). Biosynthesis of glycosylphosphatidylinositol membrane anchors. Biochem. J. 310 (Pt 2), 361–370. doi: 10.1042/bj3100361
Sun, W., Xu, J., Yang, J., Kieliszewski, M. J., Showalter, A. M. (2005). The lysine-rich arabinogalactan-protein subfamily in Arabidopsis: gene expression, glycoprotein purification and biochemical characterization. Plant Cell Physiol. 46 (6), 975–984. doi: 10.1093/pcp/pci106
Takahashi, D., Kawamura, Y., Uemura, M. (2016). Cold acclimation is accompanied by complex responses of glycosylphosphatidylinositol (GPI)-anchored proteins in Arabidopsis. J. Exp. Bot. 67 (17), 5203–5215. doi: 10.1093/jxb/erw279
Takahashi, K., Shimada, T., Kondo, M., Tamai, A., Mori, M., Nishimura, M., et al. (2010). Ectopic expression of an esterase, which is a candidate for the unidentified plant cutinase, causes cuticular defects in Arabidopsis thaliana. Plant Cell Physiol. 51 (1), 123–131. doi: 10.1093/pcp/pcp173
Takeda, J., Kinoshita, T. (1995). GPI-anchor biosynthesis. Trends Biochem. Sci. 20 (9), 367–371. doi: 10.1016/S0968-0004(00)89078-7
Tang, Y., He, R., Zhao, J., Nie, G., Xu, L., Xing, B. (2016). Oxidative stress-induced toxicity of CuO nanoparticles and related toxicogenomic responses in Arabidopsis thaliana. Environ. Pollut. 212, 605–614. doi: 10.1016/j.envpol.2016.03.019
Taylor-Teeples, M., Lin, L., de Lucas, M., Turco, G., Toal, T. W., Gaudinier, A., et al. (2015). An Arabidopsis gene regulatory network for secondary cell wall synthesis. Nature 517 (7536), 571–575. doi: 10.1038/nature14099
Theerakulpisut, P., Xu, H., Singh, M. B., Pettitt, J. M., Knox, R. B. (1991). Isolation and developmental expression of Bcp1, an anther-specific cDNA clone in Brassica campestris. Plant Cell 3 (10), 1073–1084. doi: 10.1105/tpc.3.10.1073
Touihri, S., Knoll, C., Stierhof, Y. D., Muller, I., Mayer, U., Jurgens, G. (2011). Functional anatomy of the Arabidopsis cytokinesis-specific syntaxin KNOLLE. Plant J. 68 (5), 755–764. doi: 10.1111/j.1365-313X.2011.04736.x
Tozeren, A., Sung, K. L., Sung, L. A., Dustin, M. L., Chan, P. Y., Springer, T. A., et al. (1992). Micromanipulation of adhesion of a Jurkat cell to a planar bilayer membrane containing lymphocyte function-associated antigen 3 molecules. J. Cell Biol. 116 (4), 997–1006. doi: 10.1083/jcb.116.4.997
Tsukamoto, T., Qin, Y., Huang, Y., Dunatunga, D., Palanivelu, R. (2010). A role for LORELEI, a putative glycosylphosphatidylinositol-anchored protein, in Arabidopsis thaliana double fertilization and early seed development. Plant J. 62 (4), 571–588. doi: 10.1111/j.1365-313X.2010.04177.x
Turupcu, A., Almohamed, W., Oostenbrink, C., Seifert, G. J. (2018). A speculation on the tandem fasciclin 1 repeat of FLA4 proteins in angiosperms. Plant Signal. Behav. 13 (9), e1507403. doi: 10.1080/15592324.2018.1507403
Vaddepalli, P., Fulton, L., Schneitz, K. (2019). Asymmetric redundancy of ZERZAUST and ZERZAUST HOMOLOG in different accessions of Arabidopsis thaliana. G3 (Bethesda) 9, 2245–2252. doi: 10.1534/g3.119.400211
Vaddepalli, P., Fulton, L., Wieland, J., Wassmer, K., Schaeffer, M., Ranf, S., et al. (2017). The cell wall-localized atypical beta-1,3 glucanase ZERZAUST controls tissue morphogenesis in Arabidopsis thaliana. Development 144 (12), 2259–2269. doi: 10.1242/dev.152231
Vercammen, E., Staal, J., Beyaert, R. (2008). Sensing of viral infection and activation of innate immunity by toll-like receptor 3. Clin. Microbiol. Rev. 21 (1), 13–25. doi: 10.1128/CMR.00022-07
Vogel, J. P., Raab, T. K., Schiff, C., Somerville, S. C. (2002). PMR6, a pectate lyase-like gene required for powdery mildew susceptibility in Arabidopsis. Plant Cell 14 (9), 2095–2106. doi: 10.1105/tpc.003509
Vogel, J. P., Raab, T. K., Somerville, C. R., Somerville, S. C. (2004). Mutations in PMR5 result in powdery mildew resistance and altered cell wall composition. Plant J. 40 (6), 968–978. doi: 10.1111/j.1365-313X.2004.02264.x
Wang, G., Ellendorff, U., Kemp, B., Mansfield, J. W., Forsyth, A., Mitchell, K., et al. (2008). A genome-wide functional investigation into the roles of receptor-like proteins in Arabidopsis. Plant Physiol. 147 (2), 503–517. doi: 10.1104/pp.108.119487
Wang, Z., Gerstein, M., Snyder, M. (2009). RNA-Seq: a revolutionary tool for transcriptomics. Nat. Rev. Genet. 10 (1), 57–63. doi: 10.1038/nrg2484
Wang, X., Zafian, P., Choudhary, M., Lawton, M. (1996). The PR5K receptor protein kinase from Arabidopsis thaliana is structurally related to a family of plant defense proteins. Proc. Natl. Acad. Sci. U.S.A. 93 (6), 2598–2602. doi: 10.1073/pnas.93.6.2598
Wei, Z., Li, J. (2018). Receptor-like protein kinases: key regulators controlling root hair development in Arabidopsis thaliana. J. Integr. Plant Biol. 60 (9), 841–850. doi: 10.1111/jipb.12663
Wei, P. C., Zhang, X. Q., Zhao, P., Wang, X. C. (2011). Regulation of stomatal opening by the guard cell expansin AtEXPA1. Plant Signal. Behav. 6 (5), 740–742. doi: 10.4161/psb.6.5.15144
Werner, A. K., Sparkes, I. A., Romeis, T., Witte, C. P. (2008). Identification, biochemical characterization, and subcellular localization of allantoate amidohydrolases from Arabidopsis and soybean. Plant Physiol. 146 (2), 418–430. doi: 10.1104/pp.107.110809
Willmann, R., Lajunen, H. M., Erbs, G., Newman, M. A., Kolb, D., Tsuda, K., et al. (2011). Arabidopsis lysin-motif proteins LYM1 LYM3 CERK1 mediate bacterial peptidoglycan sensing and immunity to bacterial infection. Proc. Natl. Acad. Sci. U.S.A. 108 (49), 19824–19829. doi: 10.1073/pnas.1112862108
Wright, S. D., Ramos, R. A., Tobias, P. S., Ulevitch, R. J., Mathison, J. C. (1990). CD14, a receptor for complexes of lipopolysaccharide (LPS) and LPS binding protein. Science 249 (4975), 1431–1433. doi: 10.1126/science.1698311
Xu, T., Dai, N., Chen, J., Nagawa, S., Cao, M., Li, H., et al. (2014). Cell surface ABP1-TMK auxin-sensing complex activates ROP GTPase signaling. Science 343 (6174), 1025–1028. doi: 10.1126/science.1245125
Xu, H., Knox, R. B., Taylor, P. E., Singh, M. B. (1995). Bcp1, a gene required for male fertility in Arabidopsis. Proc. Natl. Acad. Sci. U.S.A. 92 (6), 2106–2110. doi: 10.1073/pnas.92.6.2106
Xu, T., Wen, M., Nagawa, S., Fu, Y., Chen, J. G., Wu, M. J., et al. (2010). Cell surface- and rho GTPase-based auxin signaling controls cellular interdigitation in Arabidopsis. Cell 143 (1), 99–110. doi: 10.1016/j.cell.2010.09.003
Xue, H., Veit, C., Abas, L., Tryfona, T., Maresch, D., Ricardi, M. M., et al. (2017). Arabidopsis thaliana FLA4 functions as a glycan-stabilized soluble factor via its carboxy-proximal Fasciclin 1 domain. Plant J. 91 (4), 613–630. doi: 10.1111/tpj.13591
Xue, Y., Yang, Y., Yang, Z., Wang, X., Guo, Y. (2018). VAMP711 is required for abscisic acid-mediated inhibition of plasma membrane H(+)-ATPase activity. Plant Physiol. 178 (3), 1332–1343. doi: 10.1104/pp.18.00499
Yamaguchi, Y. L., Ishida, T., Sawa, S. (2016). CLE peptides and their signaling pathways in plant development. J. Exp. Bot. 67 (16), 4813–4826. doi: 10.1093/jxb/erw208
Yan, L., Cheng, X., Jia, R., Qin, Q., Guan, L., Du, H., et al. (2014). New phenotypic characteristics of three tmm alleles in Arabidopsis thaliana. Plant Cell Rep. 33 (5), 719–731. doi: 10.1007/s00299-014-1571-1
Yang, J., Showalter, A. M. (2007). Expression and localization of AtAGP18, a lysine-rich arabinogalactan-protein in Arabidopsis. Planta 226 (1), 169–179. doi: 10.1007/s00425-007-0478-2
Yang, J., Sardar, H. S., McGovern, K. R., Zhang, Y., Showalter, A. M. (2007). A lysine-rich arabinogalactan protein in Arabidopsis is essential for plant growth and development, including cell division and expansion. Plant J. 49 (4), 629–640. doi: 10.1111/j.1365-313X.2006.02985.x
Yang, Y., Zhang, Y., Ding, P., Johnson, K., Li, X., Zhang, Y. (2012). The ankyrin-repeat transmembrane protein BDA1 functions downstream of the receptor-like protein SNC2 to regulate plant immunity. Plant Physiol. 159 (4), 1857–1865. doi: 10.1104/pp.112.197152
Yang, J., Zhang, Y., Liang, Y., Showalter, A. M. (2011). Expression analyses of AtAGP17 and AtAGP19, two lysine-rich arabinogalactan proteins, in Arabidopsis. Plant. Biol. (Stuttg.) 13 (3), 431–438. doi: 10.1111/j.1438-8677.2010.00407.x
Yeats, T. H., Somerville, C. R. (2016). A dual mechanism of cellulose deficiency in shv3svl1. Plant Signal. Behav. 11 (9), e1218108. doi: 10.1080/15592324.2016.1218108
Yeats, T. H., Bacic, A., Johnson, K. L. (2018). Plant glycosylphosphatidylinositol anchored proteins at the plasma membrane-cell wall nexus. J. Integr. Plant Biol. 60 (8), 649–669. doi: 10.1111/jipb.12659
Yeats, T. H., Sorek, H., Wemmer, D. E., Somerville, C. R. (2016). Cellulose deficiency Is enhanced on hyper accumulation of sucrose by a H+-coupled sucrose symporter. Plant Physiol. 171 (1), 110–124. doi: 10.1104/pp.16.00302
Yin, Y., Qin, K., Song, X., Zhang, Q., Zhou, Y., Xia, X., et al. (2018). BZR1 transcription factor regulates heat stress tolerance through FERONIA receptor-like kinase-mediated reactive oxygen species signaling in tomato. Plant Cell Physiol. 59 (11), 2239–2254. doi: 10.1093/pcp/pcy146
Yu, F., Qian, L., Nibau, C., Duan, Q., Kita, D., Levasseur, K., et al. (2012). FERONIA receptor kinase pathway suppresses abscisic acid signaling in Arabidopsis by activating ABI2 phosphatase. Proc. Natl. Acad. Sci. U.S.A. 109 (36), 14693–14698. doi: 10.1073/pnas.1212547109
Zanoni, I., Ostuni, R., Marek, L. R., Barresi, S., Barbalat, R., Barton, G. M., et al. (2011). CD14 controls the LPS-induced endocytosis of Toll-like receptor 4. Cell 147 (4), 868–880. doi: 10.1016/j.cell.2011.09.051
Zavaliev, R., Dong, X., Epel, B. L. (2016). Glycosylphosphatidylinositol (GPI) modification serves as a primary plasmodesmal sorting signal. Plant Physiol. 172 (2), 1061–1073. doi: 10.1104/pp.16.01026
Zhang, X. Q., Wei, P. C., Xiong, Y. M., Yang, Y., Chen, J., Wang, X. C. (2011c). Overexpression of the Arabidopsis alpha-expansin gene AtEXPA1 accelerates stomatal opening by decreasing the volumetric elastic modulus. Plant Cell Rep. 30 (1), 27–36. doi: 10.1007/s00299-010-0937-2
Zhang, Y., Yang, J., Showalter, A. M. (2011a). AtAGP18 is localized at the plasma membrane and functions in plant growth and development. Planta 233 (4), 675–683. doi: 10.1007/s00425-010-1331-6
Zhang, Y., Yang, J., Showalter, A. M. (2011b). AtAGP18, a lysine-rich arabinogalactan protein in Arabidopsis thaliana, functions in plant growth and development as a putative co-receptor for signal transduction. Plant Signal. Behav. 6 (6), 855–857. doi: 10.4161/psb.6.6.15204
Zhang, Y., Yang, Y., Fang, B., Gannon, P., Ding, P., Li, X., et al. (2010). Arabidopsis snc2-1D activates receptor-like protein-mediated immunity transduced through WRKY70. Plant Cell 22 (9), 3153–3163. doi: 10.1105/tpc.110.074120
Zhao, C., Zayed, O., Yu, Z., Jiang, W., Zhu, P., Hsu, C. C., et al. (2018). Leucine-rich repeat extensin proteins regulate plant salt tolerance in Arabidopsis. Proc. Natl. Acad. Sci. U.S.A. 115 (51), 13123–13128. doi: 10.1073/pnas.1816991115
Zhao, P., Zhang, F., Liu, D., Imani, J., Langen, G., Kogel, K. H. (2017). Matrix metalloproteinases operate redundantly in Arabidopsis immunity against necrotrophic and biotrophic fungal pathogens. PLoS One 12 (8), e0183577. doi: 10.1371/journal.pone.0183577
Zhou, K. (2019a). GPI-anchored SKS proteins regulate root development through controlling cell polar expansion and cell wall synthesis. Biochem. Biophys. Res. Commun. 509 (1), 119–124. doi: 10.1016/j.bbrc.2018.12.081.
Keywords: glycosylphosphatidylinositol (GPI), GPI-anchored protein (GPI-AP), receptor-like kinase (RLK), ligand, signaling transduction
Citation: Zhou K (2019) Glycosylphosphatidylinositol-Anchored Proteins in Arabidopsis and One of Their Common Roles in Signaling Transduction. Front. Plant Sci. 10:1022. doi: 10.3389/fpls.2019.01022
Received: 06 May 2019; Accepted: 22 July 2019;
Published: 29 August 2019.
Edited by:
Els JM Van Damme, Ghent University, BelgiumReviewed by:
Daisuke Takahashi, Max Planck Institute of Molecular Plant Physiology, GermanyCopyright © 2019 Zhou. This is an open-access article distributed under the terms of the Creative Commons Attribution License (CC BY). The use, distribution or reproduction in other forums is permitted, provided the original author(s) and the copyright owner(s) are credited and that the original publication in this journal is cited, in accordance with accepted academic practice. No use, distribution or reproduction is permitted which does not comply with these terms.
*Correspondence: Ke Zhou, emhvdWtlMDUyNUBnbWFpbC5jb20=
Disclaimer: All claims expressed in this article are solely those of the authors and do not necessarily represent those of their affiliated organizations, or those of the publisher, the editors and the reviewers. Any product that may be evaluated in this article or claim that may be made by its manufacturer is not guaranteed or endorsed by the publisher.
Research integrity at Frontiers
Learn more about the work of our research integrity team to safeguard the quality of each article we publish.