- College of Life Sciences, Shaanxi Normal University, Xi’an, China
Fargesia is ecologically and economically important in mountainous forests. Many Fargesia species are also important sources of food for some endangered animals such as the giant panda. Recent molecular phylogenetic analyses have revealed Fargesia as a polyphyletic group despite some unclear lineage affinities. In the present study, we reconstructed the phylogeny of Fargesia and its allies, including Thamnocalamus, Arundinaria (incl. Bashania), Yushania, Indocalamus, Ampelocalamus and Phyllostachys, from a plastome sequence matrix that contained 20 Fargesia and five Yushania species as ingroups, 16 species from nine other bamboo genera plus Oryza sativa and Zea mays as outgroups. Fargesia and its allies were broken into eight clades. Several Fargesia species were clustered into the Thamnocalamus clade and the Drepanostachyum + Himalayacalamus clade that rendered the polyphyly of Fargesia. The remaining six clades, including the Fargesia spathe clade, the Phyllostachys clade, Arundinaria fargesii, the Ampelocalamus clade, the Fargesia grossa clade, and the Fargesia macclureana clade, were identified. Molecular phylogenetic analyses supported that Yushania should be included in Fargesia (s.l.) which had synapomorphy of expanded leaf sheaths in varying degree at the basis of inflorescences, and further divided into the Fargesia spathe clade, the Fargesia grossa clade, and the Fargesia macclureana clade. All sampled species of Yushania were nested within the Fargesia grossa clade. The probable model of the origin of the species in the Fargesia spathe clade with spathe-like leaf sheath syndrome was proposed. Moreover, the formation of the spathe-like leaf sheath syndrome may be correlated with cold climatic conditions in Quaternary. Our results provide new sight into the phylogenetic relationship within Fargesia.
Introduction
Bamboos, especially the woody bamboos, are notoriously difficult for identification and classification due to diversified morphology of vegetative characters and flowering intervals as long as 10–120 or even up to 150 years (McClure, 1966; Janzen, 1976; Ramanayake and Weerawardene, 2003; Stapleton et al., 2009; Attigala et al., 2016; Zhang et al., 2019). Previous phylogenetic studies have shown that the temperate woody bamboos are monophyletic (Hodkinson et al., 2010; Triplett and Clark, 2010; Bamboo Phylogeny Group, 2012; Saarela et al., 2018), although the woody bamboos as a whole are paraphyletic (Triplett and Clark, 2010; Bamboo Phylogeny Group, 2012; Triplett et al., 2014). Molecular phylogenies are often incongruent with morphologically-based classification schemes, leading to difficulty in understanding the evolution of morphological traits in many bamboo clades (Triplett and Clark, 2010; Triplett et al., 2014; Attigala et al., 2016). For example, the tribe Arundinarieae have been divided into 12 or 13 major lineages based on plastid or nuclear DNA markers, respectively, for which no morphological synapomorphies have been identified (Zhang et al., 2012; Attigala et al., 2014, 2016; Ma et al., 2014). At the generic level, the problem is more severe, where morphological intermediacy, insufficient informative characters, and hybridization/introgression contribute to the lack of agreement between morphological based classification schemes and recent molecular phylogenies (Triplett and Clark, 2010; Zhang et al., 2012; Yang et al., 2013).
Fargesia Franch. was considered to be a distinct genus in tribe Arundinarieae (Clayton and Renvoize, 1986; Soderstrom and Ellis, 1987; Keng, 1992; Yi, 1996; Li, 1997; Ohrnberger, 1999; Yi et al., 2008; Triplett and Clark, 2010; Bamboo Phylogeny Group, 2012; Kellogg, 2015) based on vegetative morphological traits (Wang and Ye, 1981; Yi, 1982, 1988a, 1996; Keng, 1983; McNeely, 1999; Guo et al., 2001, 2002; Guo and Li, 2004; Li et al., 2006; Yi et al., 2008). Fargesia species play an important role in the subtropical ecosystem and also as the main food of the giant panda and some other endangered animals (Yi, 1985a, 1996; McNeely, 1999; Guo and Li, 2004; Yi et al., 2008). The species of Fargesia share the following diagnostic characters: pachymorph rhizomes with a short neck, 7–15 branches per node on culm, racemose or paniculate inflorescences, ebracteate, inflorescences subtended by a series of delicate or spathe-like sheaths, and pistils with two or three stigmas (Franchet, 1893; Wang and Ye, 1981; Yi, 1982, 1983b, 1988a, 1996; Keng, 1987; Li et al., 2006; Yi et al., 2008; Kellogg, 2015). However, due to considerable morphological diversity and complex evolutionary history of bamboos, Fargesia had faced a protracted period of taxonomic uncertainty on the generic boundary, with some species transferred into or out of other genera of Sino-Himalayan pachymorph-rhizomed bamboos based on morphological evidence, including Thamnocalamus (Soderstrom, 1979a,b; Soderstrom and Ellis, 1982; Clayton and Renvoize, 1986; Chao and Renvoize, 1989; Demoly, 1991; Chao and Tang, 1993; Stapleton, 1994a; Li et al., 2006), Yushania (Soderstrom and Ellis, 1987; Stapleton, 1994a), Borinda (Stapleton, 1994a; Stapleton et al., 2005), Drepanostachyum (Stapleton, 1994b; Li, 2003; Li et al., 2006; Stapleton et al., 2005) or Himalayacalamus (Stapleton, 1994b; Ohrnberger, 1996; Li et al., 2006) and the leptomorph-rhizomed genus Arundinaria (Soderstrom and Ellis, 1988; Stapleton, 1994a; Li et al., 2006). Previous studies suggested that the spathe-like leaf sheaths subtending at the basis of inflorescences (Yi, 1988a) and the number of stigmas may be useful for delimitation of Fargesia and other alpine bamboos (Guo et al., 2002; Guo and Li, 2004), but the phylogenetic relationships among them are still unclear. Subsequently, Borinda was treated as a synonym for Fargesia based on nuclear phylogenies (Guo et al., 2001, 2002; Guo and Li, 2004). Fargesia species were nested within the Phyllostachys clade of tribe Arundinarieae which included more than 15 genera based on multi-locus plastid markers or complete chloroplast genome (Triplett and Clark, 2010; Zeng et al., 2010; Attigala et al., 2014; Ma et al., 2014; Zhang et al., 2016). However, by adding the nuclear markers and multiplying the sampling size, the phylogenetic relationship between Fargesia and closely taxa, including Yushania (Hodkinson et al., 2010; Wang et al., 2017; Zhang et al., 2019), Phyllostachys (Ma et al., 2014; Wang et al., 2017) Chimonocalamus (Yang et al., 2013; Wang et al., 2017), Bashania (Zeng et al., 2010; Yang et al., 2013; Wang et al., 2017), Thamnocalamus (Guo et al., 2002; Guo and Li, 2004; Zhang et al., 2019), Drepanostachyum (Wang et al., 2017; Zhang et al., 2019), and Himalayacalamus (Wang et al., 2017; Zhang et al., 2019), could not be resolved. For instance, Phyllostachys species were sister to Fargesia based on plastomes (Ma et al., 2014; Attigala et al., 2016), but it had no affinity with Fargesia in the RAD-seq data (Wang et al., 2017).
The infrageneric classification of Fargesia was established mainly based on vegetative characteristics including the shape of buds and culm sheath blades, and the number and thickness of branches (Yi, 1988a). However, high rates of morphological divergence may result in unstable generic/infrageneric classifications due to parallel evolution or morphological homoplasy (Wei et al., 2018), which prevail in vegetative traits of bamboos (Guo and Li, 2004; Attigala et al., 2016; Zhang et al., 2019). Therefore, it needs to be tested whether the current morphologically based infrageneric classification of Fargesia (Yi, 1988a, 1996; Yi and Jiang, 2010; Bamboo Phylogeny Group, 2012) is credible. Recent systematic studies revealed that the Fargesia + Yushania clade based on RAD-data (Wang et al., 2017) or plastid regions and nrITS (Zhang et al., 2019), including most Fargesia species and all sampled Yushania species, could be further divided into the Fargesia spathe clade and the non-spathe clade according to their morphological traits of inflorescences and distribution rather than the key vegetative characteristics listed by Yi (1988a) for the subdivision of Fargesia (Zhang et al., 2019). Moreover, the species in the Fargesia spathe clade shared a spathe-like leaf sheath syndrome, i.e., a series of spathe-like leaf sheaths that surround compressed inflorescences with the top spathe generally longer than the inflorescences and the spikelets exserted partially and uni-laterally, which was hypothesized to be an adaptation to cold environments in the north (Zhang et al., 2019). However, the phylogenetic relationships among major subclades of the non-spathe clade including the rest of Fargesia species and all sampled Yushania species are still poorly understood, with species from both genera being intermingled with other taxa such as Arundinaria, Indocalamus, and Chimonocalamus (Ma et al., 2014; Wang et al., 2017).
Sparse taxon sampling and insufficient informative characters had been the problems for investigating the phylogeny of Fargesia. Thus, a high-quality phylogeny, including more representative taxa and a large number of genetic markers, is necessary to understand the evolutionary history of Fargesia. Full plastome sequencing has been shown to be useful in resolving complex evolutionary relationships in closely related species (Njuguna et al., 2013; Williams et al., 2016; Uribe-Convers et al., 2017; Fonseca and Lohmann, 2018). Despite the existence of previous phylogenetic analyses based on the plastomes of Arundinarieae (Ma et al., 2014; Attigala et al., 2016; Saarela et al., 2018), the phylogenetic relationships among Fargesia species are still poorly resolved. Our aims were to (1) test the monophyly of Fargesia; (2) establish a phylogenetic framework for Fargesia and closely related taxa; (3) evaluate the utility of whole chloroplast genome sequencing in providing a high-resolution estimation of the infrageneric phylogeny within Fargesia.
Materials and Methods
Taxon Sampling
Three to five fresh leaf samples from each individual (clumps) were collected in their native habitats and dried in silica gel and stored at room temperature for DNA extraction (Table 1). Twenty-six species, including the type species for each section and series in Yi’s (1988a) classification of Fargesia, were selected for Illumina sequencing. We also included the representatives of the major subclades in the Fargesia + Yushania clade based on the previous study by Zhang et al. (2019) and outgroups representing genera within the Phyllostachys clade of the Arundinarieae (since their phylogenetic relationship is unclear with Fargesia; see Ma et al., 2014) (Table 1). All vouchers were deposited in the Herbarium of Shaanxi Normal University (SANU, Xi’an, China). Seventeen previously published plastomes were downloaded from the NCBI GenBank database1 (Table 1).
Plastomes Sequencing, Assembly, and Annotation
Silica-dried leaf material was sent to the Center for Genetic and Genomic Analysis (Genesky Biotechnologies Inc., Shanghai) for library preparation and Illumina sequencing. Total genomic DNA was isolated using a DNeasy Plant Mini Kit (Qiagen, CA, United States). Short-insert (330 bp) libraries were constructed using the TruSeqTM DNA sample preparation kit (Illumina, United States) for Illumina HiSeq sequencing. We sequenced at least 2 GB of raw data for each bamboo species with an average read length of 150 bp. All of the sequencing reactions were conducted on the Illumina HiSeq 2 × 150 platform. The obtained raw reads were quality-trimmed using CLC Genomics Workbench v.8.5.1 (CLC Bio, Aarhus, Denmark)2 with default parameters. After trimming, high-quality paired-end reads were MIRA mapping assembly using MIRA v4.0.2 (Chevreux et al., 2004) to create initial reference sequences. These newly created reference sequences were baiting and iterative mapping with MITObim v1.8 (Hahn et al., 2013) to assemble the complete plastome sequences, and the previous published complete plastome sequences of Fargesia spathacea (GenBank no., JX513417), Yushania levigata (GenBank no., JX513426), Pleioblastus maculatus (GenBank no., JX513424), Ampelocalamus calcareus (GenBank no., KJ496369), and Arundinaria fargesii (GenBank no., JX513413) were used as seed reference. Raw reads were then remapped to newly assembled complete plastome sequences using Geneious v R9.0.2 (Kearse et al., 2012) under default parameters to check for misassemblies. Small gaps (46–193 bp) or ambiguous nucleotides in the remapped sequences were further confirmed by designed PCR amplifications (Supplementary Table S1) and sent to Sangon Biotech (Shanghai) Co., Ltd for Sanger sequencing. Gene annotations were assigned using DOGMA (Wyman et al., 2004). Inverted repeat (IR) boundaries were determined using the BLAST method (Altschul et al., 1990)3 and verified using Geneious v R9.0.2. The genome map of Fargesia denudata was drawn using the online program OGDRAW v 1.1 (Lohse et al., 2013).
Data Partitioning and Alignment of Plastomes
We employed six datasets to reconstruct the phylogenetic trees: (1) the complete chloroplast genome sequences that excluded one copy of the IR region; (2) coding sequences including exons of protein-coding genes, tRNAs, and rRNAs (3) non-coding sequences including intergenic regions and introns; (4) the large single copy region (LSC), (5) the small single copy region (SSC) and (6) one inverted repeats region (IR). All 43 sequences from these six datasets were aligned using the MAFFT plugin (Katoh et al., 2002) in Geneious v.R9.0.2 with default parameters. As there is a negative effect of an increasing density of gap characters in multiple sequence alignments (Dwivedi and Gadagkar, 2009) and biases may be introduced when indels are included without precautions in Bayesian and Maximum Likelihood phylogenies (Warnow, 2012; Simmons, 2014), poorly aligned regions contained one or more gaps introduced by the alignments were excluded from the datasets (Attigala et al., 2016). Two different partitioning strategies were explored to access heterogeneity in the processes of molecular evolution for different sites in a sequence alignment. The first strategy included two partitions: coding sequences and non-coding sequences. The second strategy included three partitions: LSC, SSC, and one IR regions we then used PartitionFinder v 1.1.1 (Lanfear et al., 2012) to compare and evaluate partitioning schemes under 56 models (models = all) according to AICc values.
Phylogenetic Analysis
We used DAMBE v6.4.29 to assess substitution saturation of the matrices before phylogenetic analyses (Xia et al., 2003; Xia and Lemey, 2009). Six datasets mentioned above with unpartitioning strategies were analyzed using Maximum likelihood (ML), Maximum parsimony (MP), and Bayesian inference (BI). ML analysis with rapid bootstrapping and 1000 replications (RAxML; Stamatakis et al., 2008) was performed using the General Time Reversible + gamma model (GTR-GAMMA model) via the CIPRES Science Gateway server (Miller et al., 2010). MP analysis was implemented in PAUP∗ 4.0b10 (Swofford, 2002) with 1000 random addition sequences and tree bisection-reconnection (TBR) branch swapping. A full heuristic bootstrap was conducted for MP with 1000 bootstrap replicates. The appropriate model for each dataset was selected according to AIC value (Posada and Crandall, 1998) implemented in jModelTest v2.1.4 (Darriba et al., 2012). GTR+I+G was found to be the most appropriate nucleotide substitution model for 43 complete chloroplast genome sequences, 41 complete chloroplast genome sequences, coding sequences, non-coding sequences, and LSC sequences; TVM+I G for SSC sequences; TVM+I for IR sequences; TIM1+I+G for 39 complete chloroplast genome sequences. BI analysis was performed using MrBayes v3.2.6 (Ronquist and Huelsenbeck, 2003). Two independent Markov chain Monte Carlo (MCMC) runs were executed, each with three heated and one cold chain for two million generations, sampling every 1000 generations, and a chain-heating temperature of 0.1. Twenty-five percent of trees were discarded as burn-in and the remaining samples were then summarized and a majority-rule consensus tree was constructed. Convergence of runs was also checked using Tracer v 1.6 (Rambaut et al., 2014) to assess the effective sample size (ESS) > 200 for all parameters.
Topology Hypothesis Testing
Taxon removal experiments can aid in the investigation of whether distantly related outgroups have a biased attraction to long branches within the ingroups (Bergsten, 2005; Attigala et al., 2016). To test if outgroup selection affected the topology of the ingroups, we recovered phylogenetic trees with two datasets: (1) removing Zea mays and Oryza sativa to include only species of bamboos; (2) further removing species of Bambuseae to leave only species of Fargesia and its allies. Assessments of competing hypotheses (e.g., the monophyly of the Fargesia spathe and non-spathe clades) of the phylogeny were conducted with the approximately unbiased (AU) test (Shimodaira, 2002) as implemented in CONSEL (Shimodaira and Hasegawa, 2001). The site-wise log likelihoods for the trees were calculated using TREE-PUZZLE v5.3 (Schmidt et al., 2002). Neighbor-Net algorithm based on uncorrected P-distances was performed with SplitsTree v4.14.4 (Huson et al., 2008). To better visualize and evaluate the phylogenetic signal conflicts between ingroup taxa, we excluded all outgroup taxa in the SplitsTree analysis.
Calibration and Estimation of the Divergence Time
A sequence matrix including 43 complete plastomes was created for divergence time dating. A molecular clock test was performed under the GTR model to assess the applicability of the strict molecular clock model. Our results showed that ΔlnL = 429 (P < 0.05), so the relaxed log-normal molecular clock was used. Divergence time dating was analyzed using BEAST v2.4.2 (Bouckaert et al., 2014) on the Cipres web server (Miller et al., 2010) with a Yule tree prior. We selected a Bambusoideae cf. Chusquea fossil (ca. 35 Mya) to calibrate the crown Bambusoideae (Strömberg, 2005). Runs were performed for 60 million generations, and trees were sampled every 1000 generations. Tracer v 1.6 (Rambaut et al., 2014) was used to check that all ESS values were > 200. The maximum clade credibility tree was generated by TreeAnnotator with mean node heights and 95% highest posterior density intervals. The temporal dynamics of the diversification of the major clades within Fargesia was visualized by constructing lineages through time (LTT) plots implemented by the APE package in R v.3.2.4 (Paradis et al., 2004).
Morphological Character Evolution
Ancestral character state reconstruction was performed in Mesquite v 3.5 (Maddison and Maddison, 2018) using Likelihood ancestral state with Mk1 model (Lewis, 2001). The vegetative and the reproductive characters were then optimized on the BI tree inferred from the 41 plastome data. Information on morphological character states of inflorescences was generated based on published literature (Li et al., 2006; Yi et al., 2008; Zhang and Ren, 2016) and herbarium specimens in cases of the traits are missing in the published literature (Zhang Yu-QuE612, Zhang Yu-QuC305, Zhang Yu-QuA071) (Table 1 and Supplementary Table S2). These characters were (1) type of culm, (2) type of rhizomes, (3) type of leaf sheaths at the basis of inflorescences, (4) type of inflorescences, and (5) number of stigmas. Character states were scored as follows: Type of culm: (0) culm unicaepitose (clump forming due to rhizomes with short necks), (1) culm diffuse (spaced culm due to rhizomes with short necks); type of rhizomes: (0) sympodium (rhizomes pachymorph), (1) amphipodium, (2) monopodium (rhizomes leptomorph); type of leaf sheaths at the basis of inflorescences: (0) spathe-like leaf sheath syndrome, (1) open inflorescences with expanded leaf sheaths, (2) open inflorescences with non-expanded leaf sheaths, (3) absence of leaf sheaths at the basis of inflorescences; type of inflorescences: (0) semelauctant inflorescences, (1) iterauctant inflorescences; number of stigmas: (0) pistils with three stigmas, (1) two stigmas, (2) two or three stigmas.
Results
General Features of Plastomes
We sequenced and assembled 26 plastomes from Fargesia and its allies (Table 1). All sequenced genomes were similar in total size, structure, and gene content relative to previously published bamboo plastomes (Supplementary Figure S1) (Zhang et al., 2011; Burke et al., 2012; Wu and Ge, 2012; Ma et al., 2014; Attigala et al., 2016; Saarela et al., 2018). The size of the IR, large single copy (LSC) and small single copy (SSC) regions ranged from 21,793 bp (Ampelocalamus calcareus) to 21,813 (F. communis), 83,112 (F. collaris) to 83,418 (Am. calcareus), and 12,648 (Am. calcareus) to 12,882 (F. damuniu), respectively (Table 1). We identified 112 genes comprising 4 ribosomal RNAs, 31 transfer RNAs, and 77 protein-coding genes. Nineteen genes were duplicated in IR regions, and thus each chloroplast genome harbored 131 genes in total.
Plastome Phylogenomics
The multiple sequence alignment of all 43 species (including both newly generated and previously published plastomes) was 124,853 bp after excluding one of the IR regions. After removal of alignment columns with gaps, the alignment length was reduced to 108,515 bp, including 8841 (8.15%) variable sites and 2187 (2.02%) parsimony-informative sites (Table 2 and Supplementary Table S3). The aligned sequences of six sequence datasets mentioned above were little saturated and were thus useful for phylogenetic analyses (Supplementary Table S4). The less partitioned model was found to be better than the highly partitioned model (Supplementary Table S5). The topologies estimated from the complete chloroplast sequences (Supplementary Figure S2F) were broadly similar to those estimated using the other datasets (Supplementary Figures S2A–E) but recovered relationships received much higher support values than those inferred from the five other datasets. Across these five datasets, the phylogenetic relationships inferred from the LSC sequences were largely resolved, while the SSC and IR datasets resolved only some of the lineages. Thus, we limited the discussion of phylogenetic trees estimated using partitioned analyses (Supplementary Figure S2).
In the phylogenetic tree based on complete plastid genome sequences (Figure 1A), Bambusa oldhamii and Dendrocalamus latiflorus was recovered as a clade (MLB/MPB/PP = 100/100/100; Figure 1A) sister to the remaining bamboo species. Ampelocalamus calcareus represent the earliest diverging lineage within Arundinarieae. Then Pleioblastus clade and Chimonocalamus longiusculus were recovered the distinct lineage, respectively. Eight major clades were recovered among Fargesia (Yi, 1988a) and its allies: the Thamnocalamus clade, the Drepanostachyum + Himalayacalamus clade, the Fargesia macclureana clade, the Fargesia grossa clade, the Ampelocalamus clade, Arundinaria fargesii, the Phyllostachys clade, and the Fargesia spathe clade (Figure 1A). The Thamnocalamus clade, which contained F. damuniu and Thamnocalamus spathiflorus, was supported by a high bootstrap value (MLB/MPB/PP = 100/ 100/1.00; Figure 1A). Similarly, high support (MLB/MPB/PP = 100/100/1.00; Figure 1A) was provided for Drepanostachyum + Himalayacalamus clade, which contained F. gyirongensis and F. collaris. The Ampelocalamus clade combined with the Fargesia spathe clade, the Phyllostachys clade, and Arundinaria fargesii to form a strongly supported monophyletic group (MLB/MPB/PP = 93/95/1.00; Figure 1A), but the phylogenetic relationships involving Arundinaria fargesii, the Phyllostachys clade, and the Fargesia spathe clade were not fully resolved. The Fargesia spathe clade, which including all species of Fargesia with the spathe-like leaf sheath syndrome (Figure 1B), is a monophyletic lineage with moderate support (MLB/MPB/PP = 82/86/1.00; Figure 1A), but the resolution among species within the clade was relatively low. The Phyllostachys clade, which contained Ph. propinqua, Ph. edulis, Ph. nigra var. henonis, and Ph. sulphurea showed strong support (MLB/MPB/PP = 100/100/1.00; Figure 1A). Phyllostachys species shows leptomorph rhizomes, inflorescences subtended by 2-keeled prophylls and 2–6 scale-like bracts (Figure 1F) while Arundinaria fargesii shows prominent sheath scars and initially compressed inflorescences with non-expanding leaf sheaths (Figure 1G). The Ampelocalamus clade, containing Am. saxatilis (= Drepanostachyum saxatile) and Am. melicoideus (= D. melicoideum), represented a distinct clade (MLB/MPB/PP = 99/95/1.00; Figure 1A). Am. melicoideus in Ampelocalamus clade in the present study have panicles fascicled on leafless flowering branches and 2–3 bracts on the basis of inflorescences (Figure 1E). The remaining Fargesia species formed two paraphyletic clades, including F. canaliculata, F. edulis, F. fungosa, F. yunnanensis, and F. grossa with Indocalamus longiauritus (Figure 1D) and the species from Yushania in a strongly supported the Fargesia grossa clade (MLB/MPB/PP = 98/95/1.00; Figure 1A). F. macclureana, F. hygrophila, F. communis, F. albocerea, Fargesia sp. and Arundinaria faberi formed another strongly supported the Fargesia macclureana clade (MLB/MPB/PP = 78/80/1.00; Figure 1A).
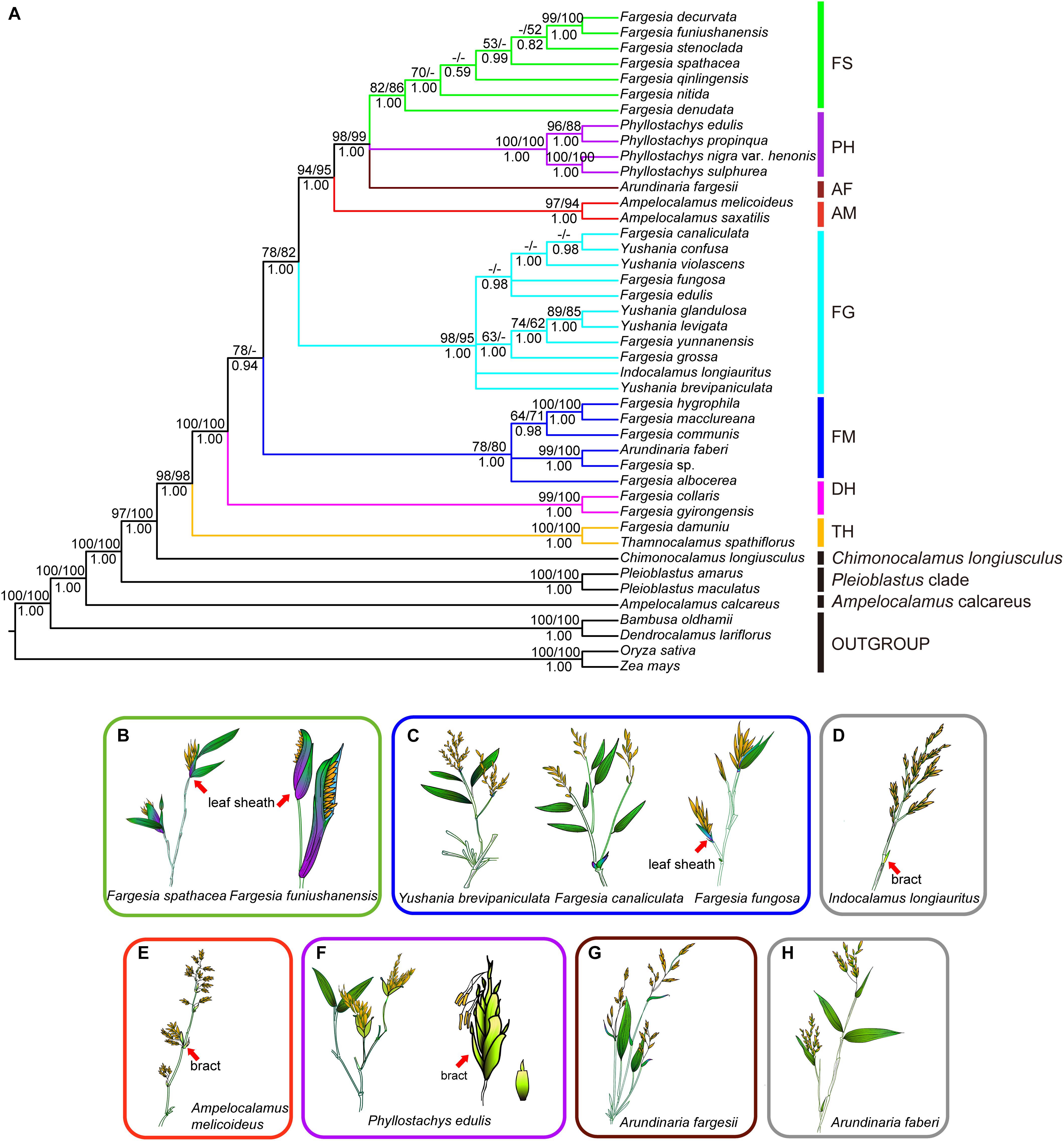
Figure 1. Phylogeny of Fargesia and its allies and floral morphology of representatives of major clades. (A) Phylogeny of 43 species of Fargesia and its allies inferred from Bayesian analysis of plastome sequences. Numbers above branches are ML bootstrap values/MP bootstrap values. Numbers below the branches are posterior probability (PP). Dashes represent nodes unresolved or without bootstrap support in the ML or MP trees or contradicted by the BI trees with PPs < 0.50. Roman numerals represent the revealed clades. FS, Fargesia spathe clade; PH, Phyllostachys clade; AF, Arundinaria fargesii; AM, Ampelocalamus clade; FG, Fargesia grossa clade; FM, Fargesia macclureana clade; DH, Drepanostachyum + Himalayacalamus clade; TH, Thamnocalamus clade. (B) Fargesia spathacea and Fargesia funiushanensis, (C) Yushania brevipaniculata, Fargesia canaliculata, and Fargesia fungosa, (D) Indocalamus longiauritus, (E) Ampelocalamus melicoideus, (F) Phyllostachys edulis, (G) Arundinaria fargesii, (H) Arundinaria faberi. Pictures of inflorescences are referred from Flora Republicae Popularis Sinicae (Yi, 1996), Flora of China (Li et al., 2006; Zhang and Ren, 2016).
Topology Testing
Since the tree topologies of the major clades based on two taxon removal experiments showed no changes when outgroups were removed (Supplementary Figures S3, S4), we concluded that the tree topology was not affected by long branch attraction.
The incompletely resolved relationships in the network suggested substantial conflicting signals (Figure 2). All species were clearly sorted into respective clades, and the clades were almost distinct. However, the relationships among the Fargesia macclureana clade, the Drepanostachyum + Himalayacalamus clade, and the Thamnocalamus clade were less clear. We used the AU test to evaluate alternative tree topologies for the relationships among the major clades of Fargesia and its allies (Supplementary Figure S5). The results based on the complete plastomes sequences and non-coding sequences rejected the monophyly of the Fargesia spathe clade (Supplementary Figure S5A), the Fargesia grossa clade and the Fargesia macclureana clade and the monophyly of the Fargesia grossa clade and the Fargesia macclureana clade (Supplementary Figures S5A,C). However, the results based on coding sequences failed to reject two topologies (Supplementary Figure S5B) involving the Fargesia grossa clade, the Fargesia macclureana clade, and the Drepanostachyum + Himalayacalamus clade.
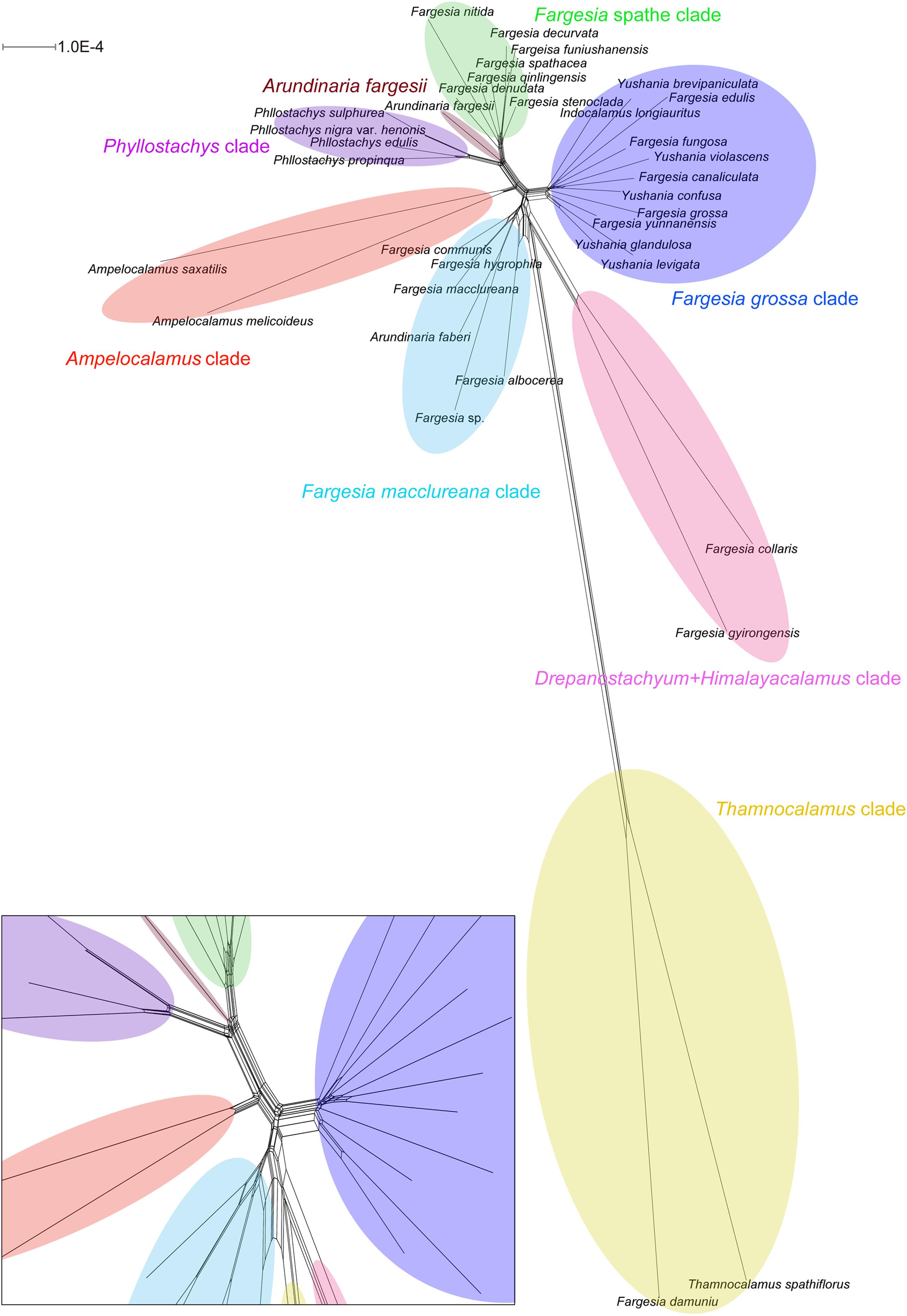
Figure 2. Neighbor-net analyses of Fargesia based on 35 plastome sequences. Clades revealed are labeled with different colors. Lower left part is a zoom-in of the network at the root.
Divergence Time Estimates
The Bayesian topology (Figure 3A) was similar to the congruent topologies from the MP, ML, and BI analyses (Figure 1A), and differed only in the placement of a few statistically unsupported nodes (e.g., Arundinaria fargesii and the Phyllostachys clade). The crown node of the Arundinarieae was dated to 13.17 Mya (95%HPD: 11.30–15.03; late Miocene); the crown node of eight clades (i.e., FS, AF, PH, AM, FG, FM, DH, TH, see Figure 1A) was dated to 7.92 Mya (95%HPD: 5.36–10.77); the crown node of six clades (i.e., FS, AF, PH, AM, FG, FM) was dated to 4.83 Mya (95%HPD: 2.19–7.30); and the Fargesia spathe clade crown was dated to 2.28 Mya (95%HPD: 0.93–4.13). In the diversification analyses, the slope of the six clades (i.e., FS, AF, PH, AM, FG, FM) of Fargesia LTT plots became steeper during ca. 3–5 Mya (Figure 3B).
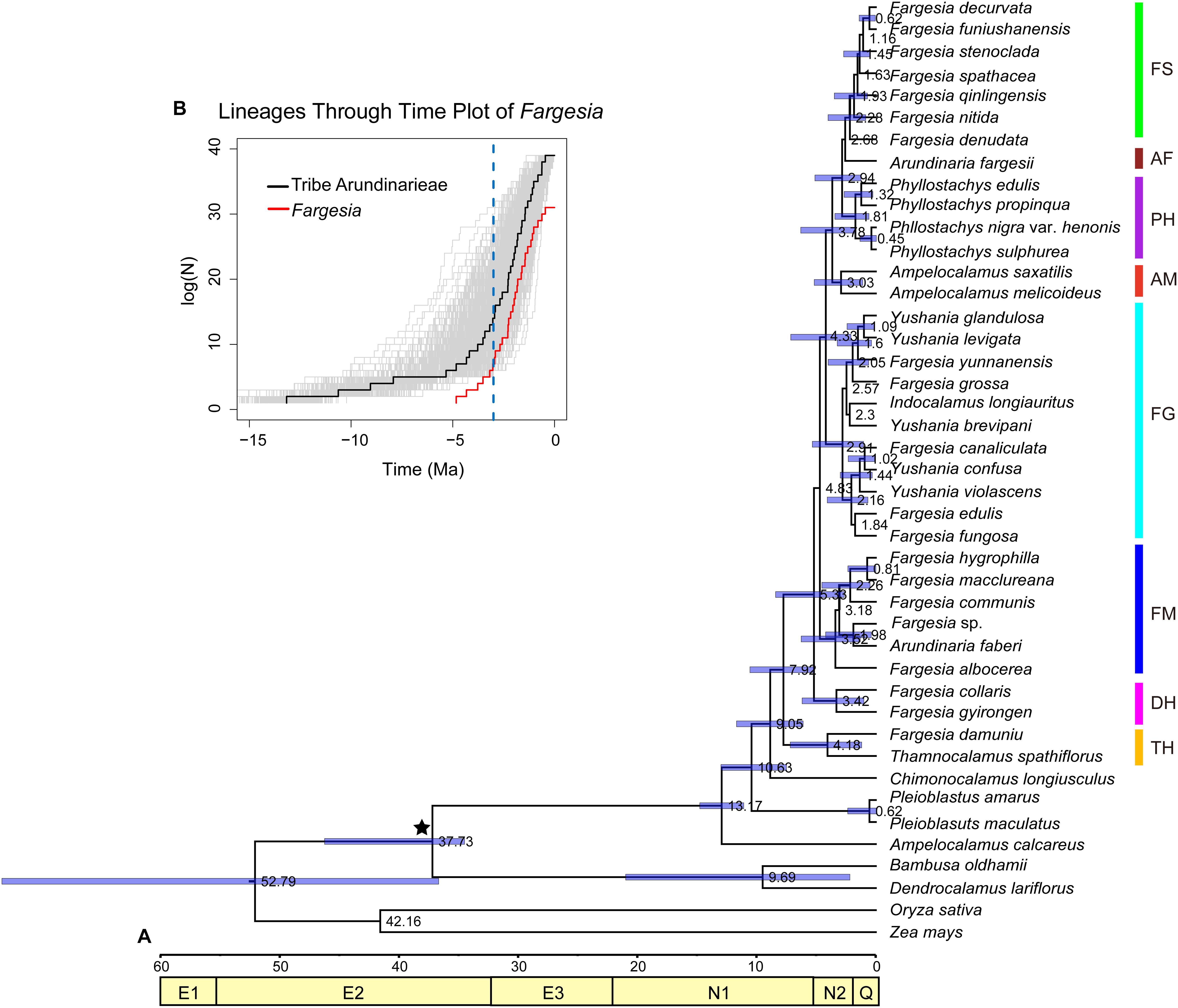
Figure 3. Divergence time estimates of Fargesia and its allies. (A) Chronogram of Fargesia and its allies based on the plastomes. E1, Paleocene; E2, Eocene; E3, Oligocene; N1, Miocene; N2, Pliocene; Q, Quaternary. Calibration points are indicated with black asterisks. (B) Lineage through time (LTT) plots for six major clades within Fargesia and tribe Arundinarieae using R-APE package. The vertical dashed lines indicate FS, Fargesia spathe clade; PH, Phyllostachys clade; AF, Arundinaria fargesii; AM, Ampelocalamus clade; FG, Fargesia grossa clade; FM, Fargesia macclureana clade; DH, Drepanostachyum + Himalayacalamus clade; TH, Thamnocalamus clade.
Morphological Characters Evolution
Most extant and ancestral taxa have cespitose culms and sympodium. Diffused culms and amphipodium (e.g., Ar. faberi and l. longiauritus) or monopodium (e.g., Phyllostachys species) have evolved independently in several species of different lineages in parallel (Figures 4A,B). Spathe-like leaf sheath syndrome is only restricted to the Fargesia spathe clade (Figure 4C), whereas species in the Fargesia grossa clade and the Fargesia macclureana clade have open inflorescences with expanded leaf sheaths in varying degree (Figures 1C,D,H). The semelauctant inflorescences seem to have evolved from iterauctant inflorescences, followed by a reversal in Phyllostachys species (Figure 4D). The ancestral state of the number of stigmas in Arundinarieae was ambiguous due to some floral characters are unknown in outgroups (Figure 4E). The selecting of different outgroups may affect the evolutionary trend of the number of stigmas. The situation is more complex within Fargesia (s.l.) due to the presence of both pistils with two stigmas and three stigmas taxa in the Fargesia spathe clade, the Fargesia grossa clade and the Fargesia macclureana clade. Thus, the pistils with two or three stigmas might arise independently in Fargesia (s.l.).
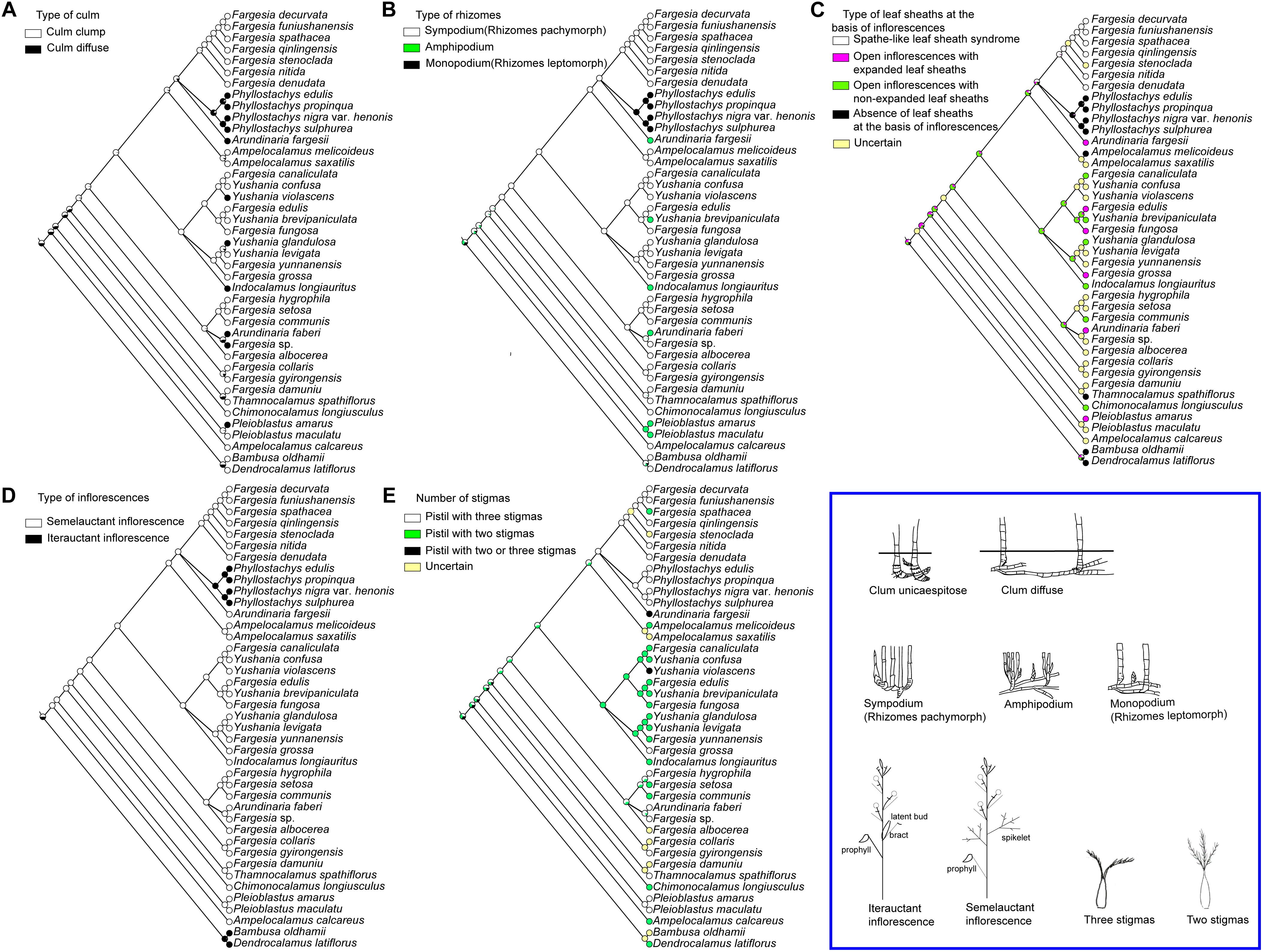
Figure 4. Optimization of morphological characters inferred on a 41-complete plastome sequences BI tree in Mesquite (A) type of culm, (B) type of rhizomes, (C) morphology of leaf sheath at the basis of inflorescences, (D) type of inflorescences and (E) number of stigmas. The illustrations of each morphological characters are shown in the blue box at the lower right.
Discussion
Polyphyly of Fargesia
Our phylogenetic analyses based on 43 complete plastome sequences, which represented a greater diversity known in Fargesia and its closely related species, confirmed that Fargesia (Yi, 1988a) is not monophyletic, which was consistent with previous analyses (Guo et al., 2002; Guo and Li, 2004; Triplett and Clark, 2010; Zeng et al., 2010; Wang et al., 2017; Zhang et al., 2019). In the present study, Fargesia damuniu and T. spathiflorus formed a monophyletic clade, which is in line with the previous recognition of a strongly supported the Thamnocalamus clade consisting of F. damuniu (Zhang et al., 2019) or F. crassinoda (Guo and Li, 2004; Zhang et al., 2019) and other Thamnocalamus species. The phylogenetic tree based on plastomes also supported that F. gyirongensis and F. collaris would be included in Drepanostachyum + Himalayacalamus clade. Phylogenetic relationships among the Drepanostachyum + Himalayacalamus clade, the Fargesia grossa clade, and the Fargesia macclureana clade varied depending on data partitioning. Differing rates, insufficient phylogenetic signals, and patterns of nucleotide substitution may be the cause of conflicts between partitioned data and unpartition data, as summarized by Ma et al. (2014). The isolated position of the Drepanostachyum + Himalayacalamus clade in the present study coincides well with the results from other studies that used nuclear genes (Hodkinson et al., 2010; Yang et al., 2013; Wang et al., 2017; Zhang et al., 2019) or plastid genes (Triplett and Clark, 2010; Zeng et al., 2010; Zhang et al., 2019). Furthermore, the morphology evidence (Keng, 1983; Ohrnberger, 1996; Li et al., 2006; Liu et al., 2017) supported F. damuniu, F. gyirongensis, and F. collaris should not be considered as Fargesia species.
Meanwhile, the phylogenetic relationships recovered in the present study corroborated Yushania species nested with Fargesia (Guo et al., 2001, 2002; Guo and Li, 2004; Yang et al., 2013; Wang et al., 2017; Zhang et al., 2019). Yushania was considered to be the most closely allied genus to Fargesia with similar vegetative characters, inflorescence types and habitats (Keng, 1957, 1984, 1987; Wang and Ye, 1980, 1981; Yi, 1982, 1985a, 1988a, 1996; Keng and Wen, 1989; Stapleton, 1994a, 1998; Wang, 1997; Guo et al., 2001, 2002; Guo and Li, 2004; Li et al., 2006). Although that opinion was supported by molecular systematic studies, the results were weakly supported and fluctuated depending on the gene and taxon sampling (Guo et al., 2001, 2002; Guo and Li, 2004; Triplett and Clark, 2010; Zhang et al., 2012; Ma et al., 2014). The recent studies based on a wider taxon sampling revealed that the sampled species of Yushania were nested within Fargesia (Wang et al., 2017; Zhang et al., 2019). Our backbone topology based on plastome data further revealed that all sampled Yushania species were nested within the Fargesia grossa clade. This result was congruent with the convergence of morphological traits. When Yushania was published, Keng (1957) stated that Yushania was different with Fargesia in aspects of rhizomes, simple inflorescences with only three spikelets and the acute lemma. Among those three traits, only the morphology of the rhizome was considered as the actual difference between Fargesia and Yushania (Yi, 1996; Li et al., 2006). Actually, the length and thickness of culm-necks in Fargesia and Yushania were considered as one of the highly variable traits (Wang et al., 2017) as the intermediate states were common (Yi, 1996; Guo and Li, 2004; Li et al., 2006). The pulvini at the point of the inflorescence branches were considered as an identifying characteristic of Yushania (Stapleton, 1994a), except for Y. lineolate which do not have pulvini, while Fargesia species have a synflorescence without pulvini except for F. yunnanensis with pulvini at the base of the inflorescence branches (Yi, 1996). A very small bract at the base of each of inflorescence branches in Yushania, which was considered as bract (Wang and Ye, 1981; Keng, 1982) or leaf sheath (Wang and Ye, 1980; Yi, 1982, 1985a,b, 1988a, 1996; Li et al., 2006; Yi et al., 2008; Yi and Jiang, 2010), is another identifying trait between these two genera (Stapleton, 1994a). However, a small bract exists at the base of each of inflorescence branches in the lower part of inflorescences in F. lincangensis and F. yunnanensis indicating the transition of this trait (Yi, 1996; Yi et al., 2008). Therefore, Fargesia and Yushania could not be distinguished by above-mentioned morphological traits.
Yi (1988a) assumed that on the premise of the similar floral morphology and the same position of a series of leaf sheaths subtending at the base of inflorescences, the small or delicate leaf sheaths in Yushania might evolve from the small or large leaf sheaths in Fargesia. Fargesia species have a series of leaf sheaths which expand to varying degrees, or even to spathes subtending at the basis of inflorescences (Wang and Ye, 1980, 1981; Keng, 1982; Yi, 1982, 1985a,b, 1988a, 1996; Wang, 1997; Li et al., 2006; Yi et al., 2008; Yi and Jiang, 2010) and spikelets are exserted partially and uni-laterally from leaf sheaths (Keng, 1982; Yi et al., 2008), while those of Yushania have small or delicate leaf sheaths which are not spathe-like (Wang and Ye, 1980, 1981; Keng, 1982; Yi, 1982, 1985a,b, 1988a, 1996; Wang, 1997; Li et al., 2006; Yi et al., 2008; Yi and Jiang, 2010) and all spikelets are exserted from leaf sheaths (Keng, 1982). However, the transitional states of this trait exist in F. yunnanensis and Y. brevipaniculata. All spikelets of F. yunnanensis are exserted from the delicate leaf sheaths (Yi, 1988a, 1996), while the spikelets of Y. brevipaniculata are exserted slightly from the non-expanded leaf sheaths (Yi, 1996). Based on basic morphological and positional similarities and the present phylogenetic analyses, we considered that a series of leaf sheaths, regardless of size, at the base of inflorescences might be a homological and synapomorphic trait in Fargesia and Yushania (Figures 1B,C, 4C). Combined with the results from our data and previous phylogenetic studies (Wang et al., 2017; Zhang et al., 2019), we suggested that Yushania might be included in Fargesia (s.l.).
Phylogenetic Relationship Among Fargesia (s.l.) and Its Allies
Previous molecular systematic studies using plastid gene sequences revealed that Fargesia was included in the Phyllostachys clade of the Arundinarieae along with Yushania, Phyllostachys, Pleioblastus, Indocalamus, Arundinaria (incl. Bashania), Drepanostachyum and Himalayacalamus (Triplett and Clark, 2010; Zeng et al., 2010; Zhang et al., 2012, 2016; Ma et al., 2014; Attigala et al., 2016). Moreover, studies using nuclear markers and RAD-seq data found that Fargesia (incl. Yushania) were not monophyletic and formed a polytomy with some species from Chimonocalamus and Arundinaria subg. Sarocalamus (Wang et al., 2017), which had some conflicts in the plastome phylogenomics (Ma et al., 2014).
In the present study, Arundinaria fargesii, Phyllostachys clade and Ampelocalamus clade are successive sisters to the Fargesia spathe clade, which was incongruent with nuclear regions (Zhang et al., 2012; Wang et al., 2017). Arundinaria fargesii was sister to the Fargesia spathe clade based on our plastomes analyses, in line with previous plastid markers analyses (Zhang et al., 2012) or plastome phylogenomics (Ma et al., 2014). However, Ar. fargesii may be a hybrid between F. decurvata and Bashania aristata based on GBSSI analyses or originated from hybridization between Chinese Pleioblastus and Indocalamus based on morphological features (Zhang et al., 2012). Thus, hybridization or plastid introgression may be a reasonable explanation for different phylogenetic positions of Ar. fargesii.
Phyllostachys species were clustered within the Fargesia (s.l.) in our plastomes analyses, while they grouped together with other species with leptomorph rhizomes such as Brachystachyum and Shibataea based on GBSSI gene tree (Zhang et al., 2012) or Pleioblastus, Acidosasa, Indosasa, and Shibataea based on RAD-seq data (Wang et al., 2017), which confirmed an isolated position from species of Fargesia (s.l.). Phyllostachys species have leptomorph rhizomes and iterauctant inflorescences which have few morphological similarities compared to species of Fargesia (s.l.). Therefore, plastid introgression or lineage sorting may be alternative causes for the incongruence.
The previous molecular studies revealed that Am. melicoideus and other Ampelocalamus species (excluding Am. calcareus and Am. actinotrichus) grouped together with Drepanostachyum and Himalayacalamus based on nuclear gene markers (Guo et al., 2002; Guo and Li, 2004; Yang et al., 2013). The plastomes of Ampelocalamus, Fargesia, Yushania, and Arundinaria (incl. Bashania) might share the same origin. Plastid capture or incomplete lineage sorting could be an explanation for the incongruent phylogenetic position of Ampelocalamus species between plastid gene markers and nuclear gene markers (Yang et al., 2013).
The inflorescence traits also provided a clue for the differences among Fargesia (s.l.), Phyllostachys species, Arundinaria fargesii and Ampelocalamus species such as semelauctant synflorescences or iterauctant synflorescence, leafy or leafless flowering branches, and the position of inflorescence-affiliated leaf sheaths, prophylls, and bracts (Figures 1A,B,E,G, 4C). For example, the inflorescences of Am. melicoideus interrupted clustered on leafless flowering branches subtended by two or three bracts and one prophyll (Yi, 1996), which differ in leafy flowering branches subtended by a series of expanded leaf sheaths in varying degree of Fargesia (s.l.) species. However, morphological differences among these taxa might implicate intermediate forms. For example, inflorescence branches of Ar. fargesii subtended by very small remnants of sheaths or rings of hairs, pulvinate, which is similar to species in Fargesia (s.l.). Moreover, these intermediate forms may be the result of hybridization given the nested positions of complete plastomes phylogeny in the present study, which is similar to Ar. fargesii discussed above or what was found in the Thamnocalamus group (incl. Fargesia and Yushania)and Arundinaria groups (incl. Bashania) (Triplett and Clark, 2010).
Differing from the situation of Ar. fargesii, Phyllostachys and Ampelocalamus, Arundinaria faberi and Indocalamus longiauritus was nested within the Fargesia grossa clade and the Fargesia macclureana clade, respectively, in the present study. Arundinaria faberi (=Bashania fangiana) was considered to belong to Arundinaria subgenus Sarocalamus (Li et al., 2006) or alpine Bashania (Zhang et al., 2012), while Ar. fargesii was put into Arundinaria subgenus Bashania (Li et al., 2006). Even though they were put into Bashania in Floral Reipublicae Popularis Sinicae (Yi, 1996) due to similar morphology, molecular evidence suggested that no affiliation between these species was revealed (Li et al., 2006; Zeng et al., 2010; Zhang et al., 2012). Arundinaria faberi was clustered within the Fargesia macclureana clade in our plastome analyses, while Ar. faberi or other alpine Bashania species were sister to species in the Fargesia spathe clade rather than the species in the Fargesia grossa clade on the GBSSI gene tree (Yang et al., 2013) and RAD-seq analyses (Wang et al., 2017). In morphology, however, Ar. faberi have open inflorescences initially at the tips of leafy branches and later on leafless branches, and the inflorescence branches subtended by long hairs and delicate leaf sheaths (Yi, 1996; Li et al., 2006), which is similar to species in the Fargesia macclureana clade. Arundinaria faberi and species in Fargesia (s.l.) overlap in distribution which may provide a potential condition for hybridization. In addition, incomplete lineage sorting during speciation may be an alternative interpretation, as well as Ampelocalamus species.
The placement of I. longiauritus may stay incertae sedis due to a putative hybridization event between Pseudosasa guanxianensis and Bashania qingchengshanensis based on previous multiple plastid regions (Zeng et al., 2010) and GBSSI gene (Zhang et al., 2012). A lack of informative characters and taxa sampling may be responsible for the disparity between our results and previous plastid phylogenies (Zeng et al., 2010; Zhang et al., 2012; Ma et al., 2014). Indocalamus longiauritus has amphipodium rhizomes with spaced culms and open inflorescences with tiny bracts subtending at the basis of inflorescence branches (Figure 1D; Li et al., 2006; Yi et al., 2008), which has some morphological differences compared with Fargesia (s.l.). Moreover, the geographic distribution of I. longiauritus overlaps less with that of species of Fargesia (s.l.) (Yi, 1996; Li et al., 2006; Yi et al., 2008). Thus, incomplete lineage sorting or homoplasy may be another cause for the present phylogenetic disparities among I. longiauritus species in Fargesia grossa clade based on plastomes and nuclear markers.
Our results also indicated the Arundinarieae began to diversify around 13.17 Mya and Fargesia (s.l.) along with the Phyllostachys clade, Ampelocalamus clade, Ar. fargesii, Ar. Faberi, and I. longiauritus undergo a rapid late Pleistocene radiation at 4.83 Mya (95%HPD: 2.19–7.30). This is close to the recent recognition of a relatively recent dispersal event in the Pliocene within Phyllostachys clade of the Arundinarieae ranging from an estimated 2.38 to 3.10 Mya (Zhang et al., 2016). Arundinarieae may have experienced rapid radiation, especially within the Arundinaria clade, Phyllostachys clade and Shibataea clade of Arundinarieae, starting from ca. 7–8 Mya (Zhang et al., 2016). A bottleneck to resolving the phylogenetic uncertainty among Fargesia (s.l.) and its allies might be the low information and different genetic background of molecular markers (nrDNA and plastid genes), like other clades in Arundinarieae (Triplett and Clark, 2010; Zeng et al., 2010; Zhang et al., 2012; Yang et al., 2013; Ma et al., 2014; Attigala et al., 2016).
Using a combination of phylogenetic estimation and morphological evolution, we revealed that Arundinaria fargesii, Phyllostachys species, and Ampelocalamus species might not be included in Fargesia (s.l.) due to recent radiation, plastid capture, incomplete lineage sorting, and hybridization/introgression among different clades within Fargesia (s.l.). We need more species Arundinaria (incl. Bashania) and Indocalamus with more nuclear markers and morphological analyses to amend Arundinaria (incl. Bashania) and Indocalamus and further disentangle the phylogenetic relationships among Ar. faberi, I. longiauritus and Fargesia (s.l.). Thus, we temporarily excluded them from Fargesia (s.l.) to discuss the infrageneric phylogenetic relationships within Fargesia (s.l.) below.
Infrageneric Phylogenetic Relationships Within Fargesia (s.l.)
Fargesia was first described by Franchet (1893) based on F. spathacea, a species with spathe-like leaf sheath syndrome. However, Yi (1983a) considered that other alpine bamboo species which have the inflorescences subtending by a series of expanded leaf sheaths in varying degree should be added in Fargesia. Therefore, Yi (1983a,b,c, 1985a,b,c, 1986, 1988a,b, 1989, 1990, 1991a,b, 1992a,b, 2000a,b,c) and others (Lu, 1981; Wang and Ye, 1981; Wen, 1984, 1989; Keng, 1987; Yi and Shao, 1987; Yi and Long, 1989; Yi et al., 2005, 2006, 2007a,b, 2008; Yi and Zhu, 2012; Yang and Yi, 2013) published ca. 100 new species of Fargesia. Subsequently, Yi (1985a,b, 1988a) amended this genus and established an infrageneric classification of Fargesia including two sections (Yi, 1985a,b) and six series (Yi, 1988a) based on morphology of buds, branches and culm sheaths among which only two sections were accepted (Ohrnberger, 1999; Yi et al., 2008). The morphological continuity observed in the field (Zhang et al., 2014) and molecular systematic studies (Zhang et al., 2019) have been revealed these key morphological characters are not objective.
Our analyses highlight species with spathe-like leaf sheath syndrome formed the Fargesia spathe clade with high supported in line with previous nuclear molecular studies (Guo et al., 2001; Guo and Li, 2004; Wang et al., 2017; Zhang et al., 2019). Here, we speculate that the spathe-like leaf sheath syndrome may be a morphological synapomorphy of Fargesia (s. s.) species that can be used to distinguish the species of the Fargesia spathe clade from those of other Fargesia clades. On the other hand, species assigned to the non-spathe clade by an earlier study (see Zhang et al., 2019) fell into two strongly supported paraphyletic clades, the Fargesia grossa clade and the Fargesia macclureana clade. The result of the AU test also demonstrated the non-monophyly of the Fargesia grossa clade and the Fargesia macclureana clade. The use of whole plastid genome sequences has the potential to increase the resolution of phylogenetic analyses at low taxonomic levels or among recently diverged species (Njuguna et al., 2013; Williams et al., 2016; Sancho et al., 2017; Uribe-Convers et al., 2017; Fonseca and Lohmann, 2018). Taxon sampling (including ingroups and outgroups) is important for phylogenetic inference, particularly for the complex temperate woody bamboos. The non-spathe clade may be further broken, even more clades will be recovered as sampling more Fargesia and Yushania species in the future.
The Fargesia grossa clade in the present study included some species of Fargesia (incl. Borinda), all species of Yushania and Indocalamus longiauritus. Those species have open inflorescences subtended by expanded leaf sheaths and pachymorph rhizomes with a long (5–20 cm) and thick (0.25–7 cm) neck (Li et al., 2006; Yi et al., 2008), except for I. longiauritus. The Fargesia macclureana clade in the present study included F. macclureana, F. albocerea, F. hygrophila, F. communis, Fargesia sp. and Ar. faberi. In this clade, all species have lax inflorescences subtended by unexpanded leaf sheaths and pachymorph rhizomes with a short (3–11 cm) and thin (0.4–2.3 cm) neck (Li et al., 2006; Yi et al., 2008), expect for Ar. faberi. The range of the thickness and the length of the culm neck and the degree of the openness of the leaf sheaths at the basis of the inflorescences are overlapped in different species of two clades, so that these morphological traits may not be sufficient to distinguish between these two clades. These differences may be explained, at least in part, by the absence of morphological autapomorphies, high levels of homoplasy in these two clades.
Temperate woody bamboos might flower frequently at the beginning of the rapid divergence, but climate fluctuation might influence their subsequent life cycles (Zhang et al., 2012). Thus, long generation times might mean that temperate bamboos have a relatively recent diversification compared to annual flowering plants or tropical bamboos (Stapleton et al., 2009). Basic knowledge on the genetics and inflorescence morphology of Fargesia is still lacking due in part to their unusual life cycle, with the vegetative phase ranging from a few to 120 or even 150 years (Janzen, 1976; Campbell, 1987). Our estimation of the divergence times for Fargesia (s.l.) and its allies implies that Fargesia (s.l.) might also undergo rapid radiation, which accounts for the difficulties of reconstructing the phylogeny of Fargesia to some degree. As plastomes only provide maternally inherited signals, further study with more nuclear DNA makers and an expanded sampling of taxa is necessary for a clearer elucidating involving the phylogenetic placement of major clades within Fargesia (s.l.) and complicated evolutionary history of Fargesia (s.l.).
A Hypothesis: Putative Hybrid Origin of the Fargesia Spathe Clade
Putative relictual lineages, transitional series, and hybrids are common in the Arundinarieae, which experienced rapid radiation (Triplett and Clark, 2010; Zhang et al., 2012, 2016). In particular, hybridization has been a key factor in bamboo diversification (Triplett et al., 2014). Incongruence between trees based on nuclear and uniparentally inherited organellar DNA has been reported to be caused by hybridization or gene introgression (Hodkinson et al., 2010; Yang et al., 2013). Arundinaria fargesii and Ar. faberi may have different phylogenetic relationships with species in the Fargesia spathe clade due to hybridization or gene introgression accounting for discrepancies between the findings of our plastome-based study and those of Ma et al. (2014) and nuclear phylogenies (Zhang et al., 2012; Wang et al., 2017). Additionally, species in Fargesia (incl. Yushania) are so widely distributed that substantial geographic overlap likely exists between the hybrids and putative parental species from Arundinaria (Li et al., 2006), thus providing the potential for hybridization or gene introgression.
Morphological evidence along with molecular phylogenies suggested that transitional state or hybrids might be common in the Yushania, and Arundinaria sp. nested within Fargesia (Zhang et al., 2012; Yang et al., 2013; Ma et al., 2014; Wang et al., 2017). Wang et al. (2017) revealed that Fargesia and Yushania may have an intermediate morphology regarding the length of culm necks and the open degree of spathe subtending; the resulting taxonomic uncertainty might be due to hybridization. The derived spathe-like leaf sheath syndrome might be interpreted as a significant indicator. Evolution of the morphology of the leaf sheaths at the basis of inflorescences or the bracts at the basis of branches has remained puzzling as comparable morphologies in related taxa were unknown until the present discovery of a relationship among Fargesia (s.l.) and Arundinaria. Arundinaria species have initially compressed inflorescences subtended by reduced leaf sheaths (Li et al., 2006; Kellogg, 2015). Species in the Fargesia grossa clade and the Fargesia macclureana clade have open inflorescences with a series of expanded leaf sheaths in varying degree but never spathe-like at the basis of inflorescence branches. In other words, the species in the Fargesia spathe clade retain partial morphological similarity to Arundinaria and species in Fargesia (s.l.) without spathe-like leaf sheath syndrome.
The spathe-like leaf sheath syndrome might be an adaptive trait facing decreasing temperature, as flower buds could be protected by the expanded spathes. Our divergence time estimation showed that the Fargesia spathe clade originated and diversified in ca. 2.28 Mya, in the Quaternary. Climatic oscillations in the Quaternary likely had tremendous effects on current plant distribution (Hewitt, 2000, 2004). When temperature decreased in glacial times, species capable of enduring cold likely expanded northward, as in the case of the Fargesia spathe clade. The fact that this clade is currently distributed in the north compared to Fargesia species without spathe-like leaf sheath syndrome also supports the hypothesis. Our data thus present an interesting scenario concerning the evolution of the Fargesia spathe clade: hybridization or gene introgression between Arundinaria species and species that bore a preliminary spathe-like leaf sheath syndrome species in the Fargesia grossa clade or the Fargesia macclureana clade (i.e., the species have a series of leaf sheaths subtending at the basis of inflorescences or bracts at the basis of branches, which could be further expanded or elongated) produced an advanced spathe-like leaf sheath syndrome, which conferred the ability for the species in the Fargesia spathe clade to expand northward in the Quaternary. However, this hypothesis needs further validation from multiple nuclear marker-based phylogenies and ecological niche modeling.
We assembled and annotated 26 plastomes, which allowed us to infer a well-supported phylogenetic backbone for Fargesia (Yi, 1988a) and its allies. Our results revealed that all sampled species of Yushania were nested with most of Fargesia and we propose a new Fargesia (s.l.) and further divided into the Fargesia spathe clade, the Fargesia grossa clade and the Fargesia macclureana clade. Phyllostachys clade, Arundinaria fargesii, and Ampelocalamus clade are inserted in Fargesia (s.l.), sister to the Fargesia spathe clade. The derived spathe-like leaf sheath syndrome was believed to be unique to the Fargesia spathe clade, which probably originated from hybridization or gene introgression between the Fargesia grossa clade or the Fargesia macclureana clade and Arundinaria species. Moreover, climatic oscillations in the Quaternary may also contribute to the formation of the derived spathe-like leaf sheath syndrome. Elucidating the complex evolutionary history of Fargesia and its allies remains difficult as multiple biological factors may play complex roles among lineages. Future work with multiple independent molecular regions will help to test different hypotheses of biological factors generated by the current study.
Data Availability
The datasets generated for this study can be found in GenBank (National Center for Biotechnology Information) with the accession numbers MH988715–MH988740.
Author Contributions
Y-QZ and X-CX collected the materials and conducted the field investigations. YZ conducted the experiments and analyzed the data. YR, J-QZ, and YZ wrote the manuscript. All authors have read and approved the final manuscript.
Funding
This study was co-supported by the National Natural Science Foundation of China (No. 31570221) and the Fundamental Research Funds for the Central Universities (2017TS028 to YZ).
Conflict of Interest Statement
The authors declare that the research was conducted in the absence of any commercial or financial relationships that could be construed as a potential conflict of interest.
Supplementary Material
The Supplementary Material for this article can be found online at: https://www.frontiersin.org/articles/10.3389/fpls.2019.00981/full#supplementary-material
FIGURE S1 | Gene map of the chloroplast genome of Fargesia denudata. Individual genes are labeled and those belonging to different functional groups are color coded. Dashed area in the inner circle indicates the GC content of the genome.
FIGURE S2 | Phylogeny of 43 taxa dataset inferred from Bayesian analysis based on different chloroplast genome partitions. Numbers above branches are ML bootstrap values/MP bootstrap values. Numbers below the branches are posterior probabilities. Dashes represent nodes unresolved or without bootstrap support in the ML or MP trees or contradicted by the BI trees with PPs < 0.50. (A) coding sequences, (B) non-coding sequences, (C) large single-copy sequences, (D) small single-copy sequences, (E) inverted repeat region sequences, (F) complete plastome sequences.
FIGURE S3 | Phylogeny of 41 taxa dataset inferred from Bayesian analysis of complete plastome sequences. Numbers above branches are ML bootstrap values/MP bootstrap values. Numbers below the branches are posterior probabilities. Dashes represent nodes unresolved or without bootstrap support in the ML or MP trees or contradicted by the BI trees with PPs < 0.50. Roman numerals represent the revealed clades. FS, Fargesia spathe clade; PH, Phyllostachys clade; AF, Arundinaria fargesii; AM, Ampelocalamus clade; FG, Fargesia grossa clade; FM, Fargesia macclureana clade; DH, Drepanostachyum + Himalayacalamus clade; TH, Thamnocalamus clade.
FIGURE S4 | Phylogeny of 39 taxa dataset inferred from Bayesian analysis of complete plastome sequences. Numbers above branches are ML bootstrap values/MP bootstrap values. Numbers below the branches are posterior probabilities. Dashes represent nodes unresolved or without bootstrap support in the ML or MP trees or contradicted by the BI trees with PPs < 0.50. Roman numerals represent the revealed clades. FS, Fargesia spathe clade; PH, Phyllostachys clade; AF, Arundinaria fargesii; AM, Ampelocalamus clade; FG, Fargesia grossa clade; FM, Fargesia macclureana clade; DH, Drepanostachyum + Himalayacalamus clade; TH, Thamnocalamus clade.
FIGURE S5 | The hypothetical topologies approximately unbiased (AU) test in CONSEL based on (A) complete plastome sequences, (B) coding sequences and (C) non-coding sequences. FS, Fargesia spathe clade; PH, Phyllostachys clade; AF, Arundinaria fargesii; AM, Ampelocalamus clade; FG, Fargesia grossa clade; FM, Fargesia macclureana clade; DH, Drepanostachyum + Himalayacalamus clade; TH, Thamnocalamus clade. Bold lines illustrate the lineages that were constrained as monophyletic. The optimal topologies according to the p-value of the approximately unbiased test are shaded.
TABLE S1 | The primers newly designed in this study for gap closure and validation of the assembled chloroplast genomes.
TABLE S2 | Data matrix of the five morphological characters used in the morphological evolution of Fargesia and the outgroup. Bold indicated morphological character states of inflorescences observed based on herbarium specimens.
TABLE S3 | The full alignment of complete plastome sequences from the 43 species excluding the gaps (NEXUS format).
TABLE S4 | The results of substitution saturation tests implemented in DAMBE.
TABLE S5 | Comparison of partitioning strategies used for the data sets implemented in PartitionFinder v.1.1.1 (Lanfear et al., 2012).
Footnotes
- ^ https://www.ncbi.nlm.nih.gov/
- ^ http://www.qiagenbioinformatics.com/product-downloads/
- ^ https://blast.ncbi.nlm.nih.gov/Blast.cgi
References
Altschul, S. F., Gish, W., Miller, W., Myers, E. W., and Lipman, D. J. (1990). Basic local alignment search tool. J. Mol. Biol. 215, 403–410. doi: 10.1016/S0022-2836(05)80360-2
Attigala, L., Triplett, J. K., Kathriarachchi, H. S., and Clark, L. G. (2014). A new genus and a major temperate bamboo lineage of the Arundinarieae (Poaceae: Bambusoideae) from Sri Lanka based on a multi-locus plastid phylogeny. Phytotaxa 174, 187–205. doi: 10.11646/phytotaxa.174.4.1
Attigala, L., Wysocki, W. P., Duvall, M. R., and Clark, L. G. (2016). Phylogenetic estimation and morphological evolution of Arundinarieae (Bambusoideae: Poaceae) based on plastome phylogenomic analysis. Mol. Phylogenet. Evol. 101, 111–121. doi: 10.1016/j.ympev.2016.05.008
Bamboo Phylogeny Group (2012). “An updated tribal and subtribal classification for the Bambusoideae (Poaceae),” in Proceedings of the Ninth World Bamboo Congress, eds J. Gielis and G. Potters (Belgium: World Bamboo Organization), 3–27.
Bergsten, J. (2005). A review of long-branch attraction. Cladistics 21, 163–193. doi: 10.1111/j.1096-0031.2005.00059.x
Bouckaert, R., Heled, J., Kühnert, D., Vaughan, T., Wu, C. H., Xie, D., et al. (2014). BEAST 2: a software platform for Bayesian evolutionary analysis. PLoS Comput. Biol. 10:e1003537. doi: 10.1371/journal.pcbi.1003537
Burke, S. V., Grennan, C. P., and Duvall, M. R. (2012). Plastome sequences of two New World bamboos – Arundinaria gigantea and Cryptochloa strictiflora (Poaceae) – extend phylogenomic understanding of Bambusoideae. Am. J. Bot. 99, 1951–1961. doi: 10.3732/ajb.1200365
Campbell, J. J. N. (1987). The history of sino-himalayan bamboo flowering, droughts and sun-spots. J. Bamboo Res. 5, 1–15.
Chao, C. S., and Renvoize, S. A. (1989). Revision of species described under Arundinaria (Gramineae) in South-East Asia and Africa. Kew Bull. 44, 349–367. doi: 10.2307/4110809
Chao, C. S., and Tang, G. G. (1993). The present status and problems of bamboo classification in China. J. Nanjing Forestry Univ. 17, 1–8. doi: 10.3969/j.jssn.1000-2006.1993.04.001
Chevreux, B., Pfisterer, T., Drescher, B., Driesel, A. J., Müller, W. E. G., Wetter, T., et al. (2004). Using the miraEST assembler for reliable and automated mRNA transcript assembly and SNP detection in sequenced SETs. Genome Res. 14, 1147–1159. doi: 10.1101/gr.1917404
Clayton, W. D., and Renvoize, S. A. (1986). Genera Graminum: Grasses of the World. London: Her Majesty’s Stationary Office.
Darriba, D., Taboada, G. L., Doallo, R., and Posada, D. (2012). jModelTest 2: more models, new heuristics and parallel computing. Nat. Methods 9:772. doi: 10.1038/nmeth.2109
Demoly, J. P. (1991). Recensement des bambous cultivés en Europe. Bambou-Bull. Assoc. Eur. Bambou Sec. France 8, 20–28.
Dwivedi, B., and Gadagkar, S. R. (2009). Phylogenetic inference under varying proportions of indel-induced alignment gaps. BMC Evol. Biol. 9:211. doi: 10.1186/1471-2148-9-211
Fonseca, L. H. M., and Lohmann, L. G. (2018). Combining high-throughput sequencing and targeted loci data to infer the phylogeny of the “Adenocalymma-Neojobertia” clade (Bignonieae. Bignoniaceae). Mol. Phylogenet. Evol. 123, 1–15. doi: 10.1016/j.ympev.2018.01.023
Franchet, M. A. (1893). Fargesia, nouveau genre de Bambuseés de la Chine. Bull. Mens. Soc. Linn. Paris 2, 1067–1069.
Guo, Z. H., and Li, D. Z. (2004). Phylogenetics of the Thamnocalamus group and its allies (Gramineae: Bambusoideae): inference from the sequences of GBSSI gene and ITS spacer. Mol. Phylogenet. Evol. 30, 1–12. doi: 10.1016/S1055-7903(03)00161-1
Guo, Z. H., Chen, Y. Y., and Li, D. Z. (2002). Phylogenetic studies on Thamnocalamus group and its allies (Poaceae: Bambusoideae) based on nrITS sequence data. Mol. Phylogenet. Evol. 22, 20–30. doi: 10.1006/mpev.2001.1039
Guo, Z. H., Chen, Y. Y., Li, D. Z., and Yang, J. B. (2001). Genetic variation and evolution of alpine Bamboos (Poaceae: Bambusoideae) using DNA sequence data. J. Plant Res. 114, 315–322. doi: 10.1007/PL00013993
Hahn, C., Bachmann, L., and Chevreux, B. (2013). Reconstructing mitochondrial genomes directly from genomic next-generation sequencing reads-a baiting and iterative mapping approach. Nucleic Acids Res. 41:e129. doi: 10.1093/nar/gkt371
Hewitt, G. (2000). The genetic legacy of the quaternary ice ages. Nature 405, 907–913. doi: 10.1098/rstb.2003.1388
Hewitt, G. M. (2004). Genetic consequences of climatic oscillations in the Quaternary. Philos. T. R. Soc. B 359, 183–195. doi: 10.1098/rstb.2003.1388
Hodkinson, T. R., Chonghaile, G. N., Sungkaew, S., Chase, M. W., Salamin, N., and Stapleton, C. M. A. (2010). Phylogenetic analyses of plastid and nuclear DNA sequences indicate a rapid late Miocene radiation of the temperate bamboo tribe Arundinarieae (Poaceae, Bambusoideae). Plant Ecol. Divers. 3, 109–120. doi: 10.1080/17550874.2010.521524
Huson, D. H., Kloepper, T., and Bryant, D. (2008). SplitsTree 4.0-Computation of phylogenetic trees and networks. Bioinformatics 14, 68–73.
Janzen, D. H. (1976). Why bamboos wait so long to flower. Ann. Rev. Ecol. Syst. 7, 347–391. doi: 10.1146/annurev.es.07.110176.002023
Katoh, K., Misawa, K., Kuma, K., and Miyata, T. (2002). MAFFT: a novel method for rapid multiple sequence alignment based on a fast Fourier transformation. Nucleic Acids Res. 30, 3059–3066. doi: 10.1093/nar/gkf436
Kearse, M., Moir, R., Wilson, A., Stones-Havas, S., Cheung, M., Sturrock, S., et al. (2012). Geneious Basic: an integrated and extendable desktop software platform for the organization and analysis of sequence data. Bioinformatics 28, 1647–1649. doi: 10.1093/bioinformatics/bts199
Kellogg, E. A. (2015). “Flowering plants, monocots, Poaceae,” in The Families and Genera of Vascular Plants, Vol. XIII, ed. K. Kubitzki (Basel: Springer International Publishing), 1–416. doi: 10.1007/978-3-319-15332-2
Keng, P. C. (1957). One new genus and two new species of Chinese bamboos. Acta Phytotax. Sin. 6, 355–360.
Keng, P. C. (1983). A revision of the genera of bamboos from the world. III. J. Bamboo Res. 2, 11–27.
Keng, P. C. (1987). On the nomenclature of the high-alpine bamboos from China. J. Bamboo Res. 6, 19–26.
Keng, P. C., and Wen, T. H. (1989). A preliminary study on bamboo classification according to the vegetative characters. J. Bamboo Res. 8, 17–29.
Lanfear, R., Calcott, B., Ho, S. Y. W., and Guindon, S. (2012). PartitionFinder: combined selection of partitioning schemes and substitution models for phylogenetic analyses. Mol. Biol. Evol. 29, 1695–1701. doi: 10.1093/molbev/mss020
Lewis, P. O. (2001). A likelihood approach to estimating phylogeny from discrete morphological character data. Syst. Biol. 50, 913–925. doi: 10.1080/106351501753462876
Li, D. Z. (1997). “The Flora of China Bambusoideae project-problems and current understanding of bamboo taxonomy in China,” in The Bamboos, ed. G. P. Chapman (London: Academic Press), 61–81.
Li, D. Z., Guo, Z. H., and Stapleton, C. M. A. (2006). “Fargesia, Yushania,” in Flora of China (Poaceae), Vol. 22, eds Z. Y. Wu and P. H. Raven (Beijing: Science Press), 74–96.
Liu, S. L., Yang, R. J., Yang, J., Yi, T. P., Song, H. X., Jiang, M. Y., et al. (2017). Differentiating thamnocalamus munro from fargesia franchet emend. Yi (Bambusoideae, Poaceae): novel evidence from morphological and neural-network analyses. Sci. Rep. 7:4192. doi: 10.1038/s41598-017-04613-9
Lohse, M., Drechsel, O., Kahlau, S., and Bock, R. (2013). Organellar Genome DRAW-a suite of tools for generating physical maps of plastid and mitochondrial genomes and visualizing expression data sets. Nucleic Acids Res. 41, W575–W581. doi: 10.1093/nar/gkt289
Ma, P. F., Zhang, Y. X., Zeng, C. X., Guo, Z. H., and Li, D. Z. (2014). Chloroplast phylogenomic analyses resolve deep-level relationships of an intractable bamboo tribe Arundinarieae (Poaceae). Syst. Biol. 63, 933–950. doi: 10.1093/sysbio/syu054
Maddison, W. P., and Maddison, D. R. (2018). Mesquite, Version 3.5: A Modular System for Evolutionary Analysis. Available at: http://www.mesquiteproject.org (accessed May 4, 2018).
McNeely, J. A. (1999). Biodiversity and bamboo genetic resources in Asia: in situ, community-based and ex situ approaches to conservation. Chin. Biodivers. 7, 38–51.
Miller, M. A., Pfeiffer, W., and Schwartz, T. (2010). “Creating the CIPRES science gateway for inference of large phylogenetic trees,” in Proceedings of the Gateway Computing Environments Workshop (GCE), New Orleans, LA, 1–8.
Njuguna, W., Liston, A., Cronn, R., Ashman, T. L., and Bassil, N. (2013). Insights into phylogeny, sex function and age of Fragaria based on whole chloroplast genome sequencing. Mol. Phylogenet. Evol. 66, 17–29. doi: 10.1016/j.ympev.2012.08.026
Ohrnberger, D. (1999). The Bamboos of the World: Annotated Nomenclature and Literature of the Species and the Higher and Lower Taxa. Amsterdam: Elsevier Science.
Paradis, E., Claude, J., and Strimmer, K. (2004). APE: analyses of phylogenetics and evolution in R language. Bioinformatics 20, 289–290. doi: 10.1093/bioinformatics/btg412
Posada, D., and Crandall, K. A. (1998). jModeltest: testing the model of DNA substitution. Bioinformatics 14, 817–818. doi: 10.1093/bioinformatics/14.9.817
Ramanayake, S. M. S. D., and Weerawardene, T. E. (2003). Flowering in a bamboo, Melocanna baccifera (Bambusoideae: Poaceae). Bot. J. Linn. Soc. 143, 287–291. doi: 10.1046/j.1095-8339.2003.00216.x
Rambaut, A., Suchard, M. A., Xie, D., and Drummond, A. J. (2014). Tracer version 1.6. Available at: http://beast.bio.ed.ac.uk/Tracer (accessed December 11, 2013).
Ronquist, F., and Huelsenbeck, J. P. (2003). MrBayes 3: Bayesian phylogenetic inference under mixed models. Bioinformatics 19, 1572–1574. doi: 10.1093/bioinformatics/btg180
Saarela, J. M., Burke, S. V., Wysocki, W. P., Barrett, M. D., Clark, L. G., and Craine, J. M. (2018). A 250 plastome phylogeny of the grass family (Poaceae): topological support under different data partitions. PeerJ 6:e4299. doi: 10.7717/peerj.4299
Sancho, R., Cantalapiedra, C. P., López-Alvarez, D., Gordon, S. P., Vogel, J. P., Catalán, P., et al. (2017). Comparative plastome genomics and phylogenomics of Brachypodium: flowering time signatures, introgression and recombination in recently diverged ecotypes. New Phytol 218, 1631–1644. doi: 10.1111/nph.14926
Schmidt, H. A., Strimmer, K., Vingron, M., and von Haeseler, A. (2002). TREE-PUZZLE: maximum likelihood phylogenetic analysis using quartets and parallel computing. Bioinformatics 18, 502–504. doi: 10.1093/bioinformatics/18.3.502
Shimodaira, H. (2002). An approximately unbiased test of phylogenetic tree selection. Syst. Biol. 51, 492–508. doi: 10.1080/10635150290069913
Shimodaira, H., and Hasegawa, M. (2001). CONSEL: for assessing the confidence of phylogenetic tree selection. Bioinformatics 17, 1246–1247. doi: 10.1093/bioinformatics/17.12.1246
Simmons, M. P. (2014). A confounding effect of missing data on character conflict in maximum likelihood and bayesian MCMC phylogenetic analyses. Mol. Phylogenet. Evol. 80, 267–280. doi: 10.1016/j.ympev.2014.08.021
Soderstrom, T. R. (1979a). Another name for the umbrella Bamboo. Brittonia 31, 495. doi: 10.2307/2806007
Soderstrom, T. R., and Ellis, R. P. (1982). Taxonomic status of the endemic South African bamboo, Thamnocalamus tessellatus. Bothalia 14, 53–67. doi: 10.4102/abc.v14i1.1135
Soderstrom, T. R., and Ellis, R. P. (1988). The woody bamboos (Poaceae: Bambuseae) of Sri Lanka: a morphological-anatomical study. Smithsonian Contr. Bot. 72, 1–75. doi: 10.5479/si.0081024X.72
Soderstrom, T. R., and Ellis, R. P. (1987). “The position of bamboo genera and allies in a system of grass classification,” in Grass Systematics and Evolution (Washington, DC: Smithsonian Institution Press), 225–238.
Stamatakis, A., Hoover, P., and Rougemont, J. (2008). A rapid bootstrap algorithm for the RAxML web servers. Syst. Biol. 57, 758–771. doi: 10.1080/10635150802429642
Stapleton, C. M. A. (1994a). The bamboos of the Nepal and Bhutan, Part II: Arundinaria, Thamnocalamus, Borinda and Yushania (Gramineae: Poaceae, Bambusoideae). Edinb. J. Bot. 51, 275–295. doi: 10.1017/s0960428600000883
Stapleton, C. M. A. (1994b). The bamboos of the Nepal and Bhutan, Part III: Drepanostachyum, Himalayacalamus, Ampelocalamus, Neomicrocalamus and Chimonobambusa (Gramineae: Poaceae, Bambusoideae). Edinb. J. Bot. 51, 301–330. doi: 10.1017/s0960428600001815
Stapleton, C. M. A. (1998). New combination in Borinda (Gramineae–Bambusoideae). Kew Bull. 53, 453–459.
Stapleton, C. M. A., Chonghaile, G. N., and Hodkinson, T. R. (2009). Molecular phylogeny of Asian woody bamboos: review for the flora of China. Bamb. Sci. Cul., J. Am. Bamb. Soc. 22, 5–25.
Stapleton, C. M. A., Li, D. Z., and Xia, N. H. (2005). New combinations for Chinese bamboos (Poaceae. Bambuseae). Novon 15, 599–601.
Strömberg, C. A. E. (2005). Decoupled taxonomic radiation and ecological expansion of open-habitat grasses in the Cenozoic of North America. Proc. Natl. Acad. Sci. U.S.A. 102, 11980–11984. doi: 10.1073/pnas.0505700102
Swofford, D. L. (2002). PAUP∗. Phylogenetic Analysis using Parsimony (∗and other methods), Version 4.0b10. Sunderland: Sinauer Associates.
Triplett, J. K., and Clark, L. G. (2010). Phylogeny of the temperate bamboos (Poaceae: Bambusoideae: Bambuseae) with an emphasis on Arundinaria and allies. Syst. Bot. 35, 102–120. doi: 10.1600/036364410790862678
Triplett, J. K., Clark, L. G., Fisher, A. E., and Wen, J. (2014). Independent allopolyploidization events preceded speciation in the temperate and tropical woody bamboos. New Phytol. 204, 66–73. doi: 10.1111/nph.12988
Uribe-Convers, S., Carlsen, M. M., Lagomarsino, L. P., and Muchhala, N. (2017). Phylogenetic relationships of Burmeistera (Campanulaceae: Lobelioideae): combining whole plastome with targeted loci data in a recent radiation. Mol. Phylogenet. Evol. 107, 551–563. doi: 10.1016/j.ympev.2016.12.011
Wang, X. Q., Ye, X. Y., Zhao, L., Li, D. Z., Guo, Z. H., and Zhuang, H. F. (2017). Genome-wide RAD sequencing data provide unprecedented resolution of the phylogeny of temperate bamboos (Poaceae: Bambusoideae). Sci. Rep. 7:11546. doi: 10.1038/s41598-017-11367-x
Wang, Z. P. (1997). A proposal concerning a system of classification of Bambusoideae from China. J. Bamboo Res. 16, 1–6.
Wang, Z. P., and Ye, G. H. (1980). On the problems of the classification of Chinese bamboos with creeping rhizomes. Acta Phytotax. Sin. 18, 283–291.
Wang, Z. P., and Ye, G. H. (1981). Miscellaneous notes on Chinese Bambusoideae. J. Nanjing Univ. 1, 91–108.
Warnow, T. (2012). Standard maximum likelihood analyses of alignments with gaps can be statistically inconsistent. PLoS Curr. 4:1308. doi: 10.1371/currents.RRN1308
Wei, R., Ebihara, A., Zhu, Y. M., Zhao, C. F., Hennequin, S., and Zhang, X. C. (2018). A total-evidence phylogeny of the lady fern genus Athyrium Roth (Athyriaceae) with a new infrageneric classification. Mol. Phylogenet. Evol. 119, 25–36. doi: 10.1016/j.ympev.2017.10.019
Wen, T. H. (1984). New taxa of Bambusoideae in China (I). J. Bamboo Res. 3, 23–47. doi: 10.3897/phytokeys.109.27566
Williams, A. V., Miller, J. T., Small, I., Nevill, P. G., and Boykin, L. M. (2016). Integration of complete chloroplast genome sequences with small amplicon datasets improves phylogenetic resolution in Acacia. Mol. Phylogenet. Evol. 96, 1–8. doi: 10.1016/j.ympev.2015.11.021
Wu, Z. Q., and Ge, S. (2012). The phylogeny of the BEP clade in grasses revisited: evidence from the whole-genome sequences of chloroplasts. Mol. Phylogenet. Evol. 62, 573–578. doi: 10.1016/j.ympev.2011.10.019
Wyman, S. K., Jansen, R. K., and Boore, J. L. (2004). Automatic annotation of organellar genomes with DOGMA. Bioinformatics 20, 3252–3255. doi: 10.1093/bioinformatics/bth352
Xia, X., and Lemey, P. (2009). “Assessing substitution saturation with DAMBE,” in The Phylogenetic Handbook: a Practical Approach to DNA and Protein Phylogeny, ed. L. Philippe (London: Cambridge University Press), 615–630. doi: 10.1017/cbo9780511819049.022
Xia, X., Xie, Z., Salemi, M., Chen, L., and Wang, Y. (2003). An index of substitution saturation and its application. Mol. Phylogenet. Evol. 26, 1–7. doi: 10.1016/S1055-7903(02)00326-3
Yang, H., Zhang, Y. X., Yang, J. B., and Li, D. Z. (2013). The monophyly of Chimonocalamus and conflicting gene trees in Arundinarieae (Poaceae: Bambusoideae) inferred from four plastid and two nuclear markers. Mol. Phylogenet. Evol 68, 340–356. doi: 10.1016/j.ympev.2013.04.002
Yang, L., and Yi, T. P. (2013). A new species of Fargesia Franch. emend Yi from western Guizhou of China (Babusoideae). Bull. Bot. Res. 33, 513–515.
Yi, T. P. (1982). A revision of the genera of Fargesia group in China. J. Sichuan Forestry Sci. Techn. 2, 54–59.
Yi, T. P. (1983b). New species of Fargesia Franchet and Yushania Keng f. from Tibet. J. Bamboo Res. 2, 18–53.
Yi, T. P. (1985a). Classification and distribution of the food bamboos of the Giant Panda (I). J. Bamboo Res. 4, 11–27.
Yi, T. P. (1985b). Classification and distribution of the food bamboos of the Giant Panda (II). J. Bamboo Res. 4, 20–45.
Yi, T. P. (1988a). A study on the genus Fargesia from China. J. Bamboo Res. 7, 1–119. doi: 10.1016/j.ympev.2016.12.026
Yi, T. P. (1988b). Four new species of bamboo from South Yunnan. China. Acta Bot. Yunnan 10, 437–443.
Yi, T. P. (1991a). A new species of alpine bamboo from Sichuan-Fargesia parvifolia. J. Bamboo Res. 10, 15–18.
Yi, T. P. (1992a). New bamboos of Fargesia and Chimonobambusa from Sichuan. Acta Bot. Yunnan. 14, 135–138.
Yi, T. P. (1996). “Fargesia, Yushania,” in Flora Reipublicae Popularis Sinicae, Vol. 9, eds P. C. Keng and Z. P. Wang (Beijing: Science Press), 387–560.
Yi, T. P. (2000a). A new species of Fargesia from northeastern Sichuan, China. Acta Bot. Yunnan. 22, 251–254.
Yi, T. P. (2000b). New materials of alpine bamboos from Southwest China. J. Sichuan Forestry Sci. Techn. 21, 1–6.
Yi, T. P. (2000c). Some new taxa of Bambusoideae in western Sichuan. China. J. Bamboo Res. 1, 19–26.
Yi, T. P., and Jiang, X. L. (2010). Staple food bamboo species of the giant panda and their biodiversity. J. Sichuan Forestry Sci. Techn. 4, 1–20.
Yi, T. P., and Long, T. L. (1989). Two new species of bamboos for Giant Panda. J. Bamboo Res. 8, 30–36.
Yi, T. P., and Zhu, X. B. (2012). A new species and two combinations of Bambusoideae (Poaceae). J. Sichuan Forestry Sci. Techn. 33, 8–11.
Yi, T. P., Shi, J. Y., and Ma, L. S. (2008). Iconographia Bambusoidearum Sinicarum. Beijing: Science Press.
Yi, T. P., Shi, J. Y., Ma, L. S., Yang, L., and Wang, H. T. (2007a). A report on a new bamboo species in northeast Yunnan, China. J. Sichuan Forestry Sci. Techn. 28, 1–3.
Yi, T. P., Shi, J. Y., and Yang, L. (2007b). Alpine new bamboos from Sichuan, Tibet and Chongqing China. Bull. Bot. Res. 27, 515–520.
Yi, T. P., Shi, J. Y., Ma, L. S., Wang, H. T., and Yang, L. (2005). Two new species and a new combination of bamboo from Sichuan and Yunnan, China. J. Sichuan Forestry Sci. Techn. 26, 33–42.
Yi, T. P., Shi, J. Y., Ma, L. S., Wang, H. T., and Yang, L. (2006). A new species and a new forma of Fargesia Franch. emend. Yi from Yunnan, China. J. Sichuan Forestry Sci. Techn. 27, 47–48.
Zeng, C. X., Zhang, Y. X., Triplett, J. K., Yang, J. B., and Li, D. Z. (2010). Large multi-locus plastid phylogeny of the tribe Arundinarieae (Poaceae: Bambusoideae) reveals ten major lineages and low rate of molecular divergence. Mol. Phylogenet. Evol. 56, 821–839. doi: 10.1016/j.ympev.2010.03.041
Zhang, X. Z., Zeng, C. X., Ma, P. F., Haevermans, T., Zhang, Y. X., Zhang, L. N., et al. (2016). Multi-locus plastid phylogenetic biogeography supports the Asian hypothesis of the temperate woody bamboos (Poaceae: Bambusoideae). Mol. Phylogenet. Evol. 96, 118–129. doi: 10.1016/j.ympev.2015.11.025
Zhang, Y. J., Ma, P. F., and Li, D. Z. (2011). High-throughput sequencing of six bamboo chloroplast genomes: phylogenetic implications for temperate woody bamboos (Poaceae: Bambusoideae). PLoS One 6:e20596. doi: 10.1371/journal.pone.0020596
Zhang, Y. Q., and Ren, Y. (2016). Supplementary description of flowers and flowering branches of four Fargesia and one Drepanostachyum species (Bambusoideae, Poaceae), and notes on their taxonomy. . Nord. J. Bot 34, 565–572. doi: 10.1111/njb.00975
Zhang, Y. Q., Wang, X. M., Wu, A. L., and Ren, Y. (2014). Merging Fargesia dracocephala into Fargesia decurvata (Bambusoideae, Poaceae): implications from morphological and ITS sequence analyses. PLoS One 9:e101362. doi: 10.1371/journal.pone.0101362
Zhang, Y. Q., Zhou, Y., Hou, X. Q., Huang, L., Kang, J. Q., Zhang, J. Q., et al. (2019). Phylogeny of Fargesia (Poaceae: Bambusoideae) and infrageneric adaptive divergence inferred from three cpDNA and nrITS sequence data. Plant Syst. Evol. 305, 61. doi: 10.1007/s00606-018-1551-y
Keywords: Fargesia, inflorescences, phylogeny, plastome, spathe-like leaf sheath syndrome, Yushania
Citation: Zhou Y, Zhang Y-Q, Xing X-C, Zhang J-Q and Ren Y (2019) Straight From the Plastome: Molecular Phylogeny and Morphological Evolution of Fargesia (Bambusoideae: Poaceae). Front. Plant Sci. 10:981. doi: 10.3389/fpls.2019.00981
Received: 08 March 2019; Accepted: 11 July 2019;
Published: 06 August 2019.
Edited by:
Michael R. McKain, The University of Alabama, United StatesReviewed by:
Angela Jean McDonnell, Chicago Botanic Garden, United StatesDaryl Lam, The University of Alabama, United States
Copyright © 2019 Zhou, Zhang, Xing, Zhang and Ren. This is an open-access article distributed under the terms of the Creative Commons Attribution License (CC BY). The use, distribution or reproduction in other forums is permitted, provided the original author(s) and the copyright owner(s) are credited and that the original publication in this journal is cited, in accordance with accepted academic practice. No use, distribution or reproduction is permitted which does not comply with these terms.
*Correspondence: Jian-Qiang Zhang, anF6aGFuZ0Bzbm51LmVkdS5jbg==; Yi Ren, cmVueWlAc25udS5lZHUuY24=