- 1Dipartimento di Scienze AgroAlimentari, Ambientali e Animali, Università degli Studi di Udine, Udine, Italy
- 2Faculty of Science and Technology, Free University of Bozen-Bolzano, Bolzano, Italy
- 3Dipartimento di Biotecnologie, Università di Verona, Verona, Italy
Improvement of plant iron nutrition as a consequence of metal complexation by humic substances (HS) extracted from different sources has been widely reported. The presence of humified fractions of the organic matter in soil sediments and solutions would contribute, depending on the solubility and the molecular size of HS, to build up a reservoir of Fe available for plants which exude metal ligands and to provide Fe-HS complexes directly usable by plant Fe uptake mechanisms. It has also been shown that HS can promote the physiological mechanisms involved in Fe acquisition acting at the transcriptional and post-transcriptional level. Furthermore, the distribution and allocation of Fe within the plant could be modified when plants were supplied with water soluble Fe-HS complexes as compared with other natural or synthetic chelates. These effects are in line with previous observations showing that treatments with HS were able to induce changes in root morphology and modulate plant membrane activities related to nutrient acquisition, pathways of primary and secondary metabolism, hormonal and reactive oxygen balance. The multifaceted action of HS indicates that soluble Fe-HS complexes, either naturally present in the soil or exogenously supplied to the plants, can promote Fe acquisition in a complex way by providing a readily available iron form in the rhizosphere and by directly affecting plant physiology. Furthermore, the possibility to use Fe-HS of different sources, size and solubility may be considered as an environmental-friendly tool for Fe fertilization of crops.
Introduction
Soil HS are generally considered as the result of the partial degradation and re-synthesis of organic material, especially of plant residues. They originate from polymerization/polycondensation of phenolic compounds, mainly deriving from microbial lignin degradation. As a consequence, soil HS have a strong aromatic nature; nonetheless, during the condensation process a number of organic molecules including aliphatic chains, peptides, amino acids, fatty acids and sugars can be incorporated, thus forming substances from medium to high molecular weight (Stevenson, 1994). Soil HS could also originate from associations of relatively small humic molecules linked together by hydrophobic interactions and hydrogen bonds (Piccolo, 2002). Humic molecules of different molecular masses can bind together forming a supramolecular humic network; the degree of aggregation may depend on the pH, ionic strength and mineral composition of the solution (Garcia-Mina, 2007; Esfahani et al., 2015).
These processes imply that HS of different molecular size and solubility are present in the soil. Some fractions are present in the soil solution, thus being able to directly interact with plant roots (Chen and Schnitzer, 1978; Gerke, 1997). These latter soluble HS are considered as part of the DOM (Bolan et al., 2011).
Humic substances are routinely extracted from the soil with alkaline solutions and then can be operationally fractionated, based on their different water solubility, into humic (HA) and fluvic (FA) acids (Stevenson, 1994).
Due to their heterogeneity, the molecular structure of soil HS cannot be unequivocally identified. Nevertheless, it has been clearly defined that the presence of some functional groups within their structure are responsible for the observed indirect and direct effects on plant growth and nutrition (Nebbioso and Piccolo, 2011; Muscolo et al., 2013; García et al., 2016a). Indirect effects refer to changes in the chemical and physical properties of soil and rhizosphere, while direct ones indicate actions on plasma membrane (PM)-bound activities and plant metabolic pathways (Varanini and Pinton, 2001; Nardi et al., 2002; Zandonadi et al., 2013; Canellas and Olivares, 2014; Rose et al., 2014; Olaetxea et al., 2018).
The occurrence of HS in soils, as representative of natural organic matter evolution, has been questioned; rather it has been proposed that they are the result of the alkali-based extraction procedure (Lehmann and Kleber, 2015). While this aspect is still under debate (Gerke, 2018; Olk et al., 2019), it is noteworthy that humic-like molecules have been extracted from soils treated with mild extractants (Hayes, 2006), found in aquatic environments (Alberts and Takács, 2004), peat water extracts and soil leachates (Pinton et al., 1997; Vujinovic et al., 2013).
Despite being the chemical nature of HS still controversial, it has been unequivocally demonstrated that organic materials of different origin can provide available Fe to plants as a results of Fe complexation by humic molecules (Chen et al., 2004a; Bocanegra et al., 2006; Kovács et al., 2013; Cieschi et al., 2017). Furthermore, soluble Fe-HS complexes could be formed and directly used by the plants (Pandeya et al., 1998; Pinton et al., 1999). The capacity of HS to complex metals and affect the mechanisms of nutrient acquisition and plant metabolism provide evidence for a multifaceted role of these organic fractions on Fe nutrition.
In the present work, we will summarize recent reports on the role of HS in plant Fe nutrition that can be attributed to their chelating and biostimulant effect, with a special emphasis on effects exerted by the water-soluble fractions.
Effects of Humic Substances on Iron Availability
Humic substances are able to form stable complexes with metal micronutrients, due to the presence in their structure of oxygen-, nitrogen- and sulfur-containing functional groups. This, in turn, would help maintaining micronutrients in solution and/or in bioavailable forms at pH values found in most soils (Senesi, 1992; Tipping, 2002). In the case of Fe, highly stable HS complexes mainly involve O-containing groups (carboxylic and phenolic groups) (Senesi, 1992; Tipping, 2002). More recently it was shown that carboxylic acids in aliphatic domains are also involved in Fe(III)-HS complexation (Fuentes et al., 2013).
The stability order of the complexes formed between metals and humic acids has been determined through potentiometric titration and follows the Irving-Williams series. Evaluation of stability constants for metal-HS complexes (Garcia-Mina et al., 2004) showed values somewhat lower than those observed for complexes between Fe and synthetic chelating agents (e.g., EDTA, EDDHA; Lucena, 2003) or organic compounds of biological origin (e.g., organic acids, siderophores, PS, phenols) (von Wirén et al., 2000; Crowley, 2001; Ryan et al., 2001; Mimmo et al., 2014).
Stability and solubility of the complexes are both affected by pH and molar ratio between micronutrients and HS (Chen et al., 2004a; Garcia-Mina, 2006). A high stability would be favored in the 5–9 pH range by a low metal:HS ratio, while a high solubility would be favored by alkaline pH and a low metal:HS ratio. This implies that plants growing in calcareous soils with limited Fe availability could benefit from the formation of stable and soluble Fe-HS complexes (Cieschi and Lucena, 2018), as well as of insoluble complexes with high molecular weight HS (Colombo et al., 2014).
Humic substances can affect Fe availability also through the stabilization of amorphous Fe oxides by high molecular weight humic fractions (Schwertmann, 1991). The poorly crystalline Fe phases, co-precipitated with insoluble HS (IHS) and maintained for a long period in this form, can represent a reservoir of iron suitable, via ligand mobilization, for plant Fe nutrition (Colombo et al., 2012, 2014).
The ability of HS to complex Fe can also be important for phosphorous nutrition, since phosphate can be bound to HS by Fe bridges (Gerke, 2010; Urrutia et al., 2013). This process would increase phosphate availability; in fact, complexation of Fe by ligands released by plant roots could promote uptake of both nutrients (Gerke, 1993; Urrutia et al., 2014).
Humic substances are known to be redox reactive and capable of chemically reducing metals including Fe3+ (Skogerboe and Wilson, 1981; Struyk and Sposito, 2001). Reduction of Fe3+ occurs at significant levels at pH values lower than 4; at higher pH values reduction is limited by formation of complexes between Fe3+ and humic molecules. It has been shown that dissolved and solid-phase HS can accelerate Fe(III)-oxide reduction in sediments (Nevin and Lovley, 2002; Roden et al., 2010) and bioreduction of Fe(III) minerals in soils (Rakshit et al., 2009), by shuttling electrons from bacteria to oxide surfaces.
Role of Humic Substances as Natural Chelates
Besides delaying the Fe crystallization processes, HS can contribute to Fe nutrition via formation of water-soluble Fe-HS complexes, which can move in the soil and reach the roots (Pandeya et al., 1998; Garcia-Mina et al., 2004; Chen et al., 2004b). These complexes would act as natural Fe-chelates interacting with plant uptake mechanisms. Using a water-extractable humic fraction (WEHS), purified from a water extract of sphagnum peat, it was demonstrated that a Fe-WEHS complex could be obtained by interaction between the humic fraction and a poorly soluble Fe form (Cesco et al., 2000). Fe-WEHS complex could, in turn, be used by Fe-deficient Strategy-I and Strategy-II plants. Uptake by Strategy-I plants could occur via the Fe(III) reduction-based mechanism (Pinton et al., 1999), while in Strategy-II plants, a ligand exchange between WEHS and PS was conceivably involved (Cesco et al., 2002). Uptake of 59Fe from 59Fe-WEHS complex was measured even at pH values compatible with those found in calcareous soils (Cesco et al., 2002; Tomasi et al., 2013) and the same held true for root Fe(III) reduction in Strategy-I plants (Tomasi et al., 2013; Zamboni et al., 2016). The recovery of Fe-deficient plants following the treatment with Fe-WEHS was paralleled by a stimulation of the acidification capacity of roots, a component of the Fe-deficiency response in Strategy-I plants (Pinton et al., 1999; Tomasi et al., 2013).
Iron from 59Fe-WEHS complex appeared to be accumulated in higher amount within the plant as compared with other natural chelates, such as 59Fe-citrate or 59Fe-PS (Tomasi et al., 2013; Zamboni et al., 2016). Furthermore, a higher translocation of Fe to the leaves was observed in Fe-deficient Strategy-I plants supplied with 59Fe-WEHS (Tomasi et al., 2009; Zanin et al., 2015) as compared with the other two natural Fe-chelates. This behavior was accompanied by an increase of Fe content in the xylem sap (Tomasi et al., 2009). In 59Fe-WEHS-treated cucumber plants Fe was more rapidly allocated into the leaf veins and transferred to interveinal cells (Zanin et al., 2015). Similar effects were reported by Bocanegra et al. (2006) who observed a rapid translocation of Fe from roots to leaves of plants treated with a low molecular weight humic fraction. These results indicate that HS could affect Fe nutrition not only by increasing the metal availability in the soil and in the rhizosphere, but also acting on the mechanisms involved in its uptake and its translocation within the plant.
Supply of HS or Fe-HS complexes has also been shown to affect expression of genes related to Fe-uptake mechanisms. Providing a Fe-WEHS complex to Fe-deficient tomato plants induced an up-regulation of root Fe(III)-chelate reductase (LeFRO1) and Fe transporter genes, LeIRT1 and LeIRT2 (Tomasi et al., 2013). The increase in transcript abundance was faster and reached a higher level than when Fe-citrate or Fe-PS were used. Aguirre et al. (2009) showed that the treatment of cucumber plants with HS purified from leonardite induced a transient up-regulation of genes involved in the Strategy-I uptake mechanism, that is CsHA2, CsFRO1 and CsIRT1, in cucumber roots. These effects were associated with an increase of the root Fe(III) chelate-reductase activity. Billard et al. (2013) showed that a humic fraction isolated from black peat could induce the up-regulation of the IRT1 gene in both the roots and leaves of rapeseed plants. These results were correlated to a significant increase of the Fe concentration in leaves.
Interestingly, also genes involved in Fe uptake in leaves (CsFRO1, CsIRT1, CsNRAMP) were up-regulated following Fe-WEHS supply to Fe-deficient cucumber plants, as compared with Fe-PS-fed plants (Zanin et al., 2015). The localization of CsFRO1, CsIRT1 transcripts was evident next to the midveins, while CsNRAMP expression was detected in the overall mesophyll region, supporting a role of this later gene in the Fe distribution within the whole leaf tissue.
Genome-wide transcriptional analysis revealed that the early response to Fe supply of Fe-deficient tomato plants was strongly influenced by the nature of the chelating agent (Zamboni et al., 2016). In fact, Fe-citrate and Fe-PS modulated, respectively the expression of 728 and 408 genes, showing a fast down-regulation of molecular mechanisms induced by Fe deficiency. On the other hand, Fe-WEHS did not determine relevant changes in the root transcriptome with respect to the Fe-deficient plants, suggesting that roots did not sense the restored cellular Fe accumulation. This behavior would account for the higher Fe accumulation in Fe-WEHS treated plants.
Effects of Humic Substances on Root Growth and Functions
Treatments of plants with HS have been shown to induce changes in root morphology and modulate plant membrane activities related to nutrient acquisition, pathways of primary and secondary metabolism, hormonal and reactive oxygen balance (Varanini and Pinton, 2001; Nardi et al., 2002; Canellas and Olivares, 2014; Olaetxea et al., 2018; Figure 1). These effects, which vary depending on the origin, molecular size and chemical characteristics of HS, suggest an action of these organic fractions on growth promotion and stress resistance in plants.
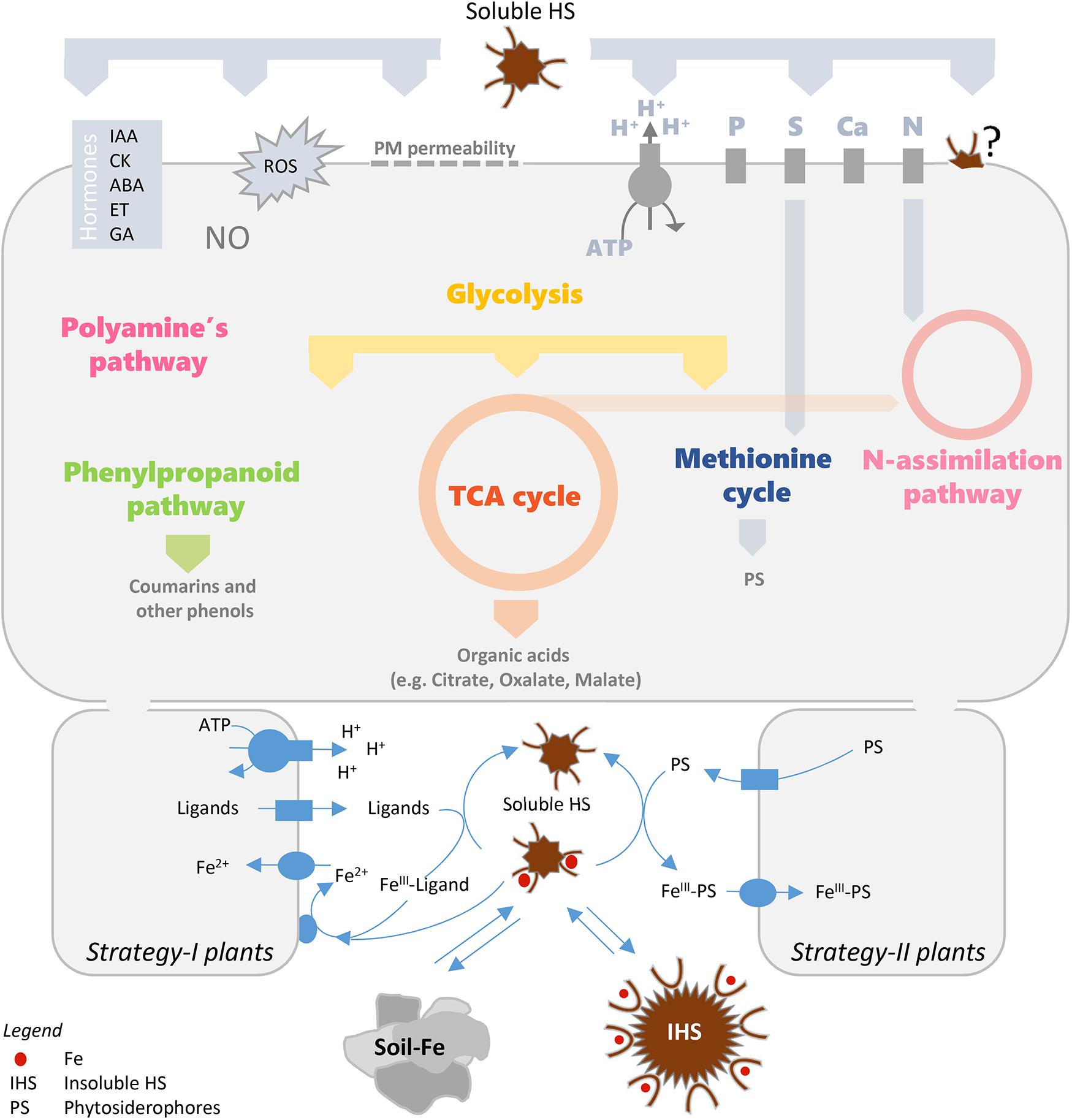
Figure 1. Physiological and molecular plant responses induced by HS (in the upper part) and role of Fe-HS complexes on Fe plant nutrition (in the lower part). Schematic drawings of Strategy-I and Strategy-II components on PM of root cells are shown (for Strategy I: a proton pump, a ligand efflux transporter, an Fe transporter and an FeIII-chelate reductase are depicted in the left; for Strategy II: a ligand efflux transporter and an Fe-chelate transporter are depicted in the right). ABA, abscisic acid; CK, cytokinin; ET, ethylene; GA, gibberellic acid; IAA, indole-3-acetic acids; IHS, insoluble HS; NO, nitric oxide; PS, phytosiderophores; ROS, reactive oxygen species; TCA, tricarboxylic acid; PM, plasma membrane; GS/GOGAT, glutamine synthase/glutamine oxoglutarate aminotransferase).
Many authors observed that plants treated with HS of different origin were able to induce proliferation of lateral roots and root hairs (Canellas et al., 2002; Nardi et al., 2002). This behavior has been related to the activation of signaling pathways involving phytohormones, especially auxin, nitric oxide, Ca2+ and ROS (Trevisan et al., 2010; Zandonadi et al., 2010; Mora et al., 2012; Ramos et al., 2015; García et al., 2016b,c). Up-regulation of auxin-regulated genes (Trevisan et al., 2011) and modulation of genes coding for enzymes involved in hormone metabolisms (Zanin et al., 2018) suggest that HS might influence the steady-state equilibrium of different plant hormones. However, stimulation of root growth was observed also independently of hormonal changes (Schmidt et al., 2007; Mora et al., 2012), suggesting that other signals might be involved in the morphological modifications elicited by HS.
A recognized target of HS action is the root PM H+-ATPase (Zandonadi et al., 2016). Evidence for activation of the PM proton pump has been observed both at transcriptional and post-transcriptional level and related to proton extrusion (Varanini et al., 1993; Canellas et al., 2002) and uptake of ions, such as nitrate (Pinton et al., 1999; Quaggiotti et al., 2004; Tavares et al., 2017), phosphate (Jindo et al., 2016) and sulfate (Jannin et al., 2012). Besides ion uptake, HS have been shown to promote nitrogen assimilation (Mora et al., 2010; Jannin et al., 2012; Vaccaro et al., 2015; Zanin et al., 2018), carbon metabolism (glycolysis and Krebs cycle; Nardi et al., 2007; Trevisan et al., 2011) and synthesis of secondary metabolites, such as phenylpropanoids (Schiavon et al., 2010; Jannin et al., 2012; García et al., 2016c).
In addition to the stimulation of proton release, HS have been shown to affect rhizodeposition. Humic acids promoted release of anionic species close to region of root acidification [apolar sugars from maize roots (Puglisi et al., 2009), impacting soil microbial community in the rhizosphere (Puglisi et al., 2013)]. Increase in root growth was accompanied by a greater release of low molecular weight exudates from maize plants treated with HS (Canellas et al., 2019). On the other hand, it has been reported that organic acids, such as those released by the roots, could disaggregate supramolecular structure of HS releasing low molecular weight humic fractions (Piccolo et al., 2003), which in turn might exert their effects on roots. Regarding this point is noteworthy that accumulation of HS at the root surface and in the apoplast has been observed (García et al., 2012; Kulikova et al., 2014). Furthermore, HS fractions obtained from rhizospheric soil showed different chemical characteristics to those isolated from bulk soil (D’Orazio and Senesi, 2009).
Effects of HS on root growth (signaling pathways), ion uptake (primary and secondary membrane transporters), primary metabolism (nitrogen and carbon), secondary metabolism (phenylpropanoids) and root exudation might be important for Fe acquisition and could improve the response of plants to Fe deprivation (Figure 1).
Conclusion and Perspective
Plenty of papers in the last decades have proven the capability of HS, isolated from different organic sources, to affect plant growth, nutrition and metabolism.
In natural soils, these substances, due to their heterogeneity and polydispersity, can be present as co-precipitates with mineral parts (e.g., Fe-oxides and clays) or in the solution where they contribute a considerable portion of the DOM.
Low-molecular-weight and water-soluble fractions have been shown to affect functionality of ion transporters operating on the PM of root cells, acting both at transcriptional and post-transcriptional level. This evidence has been achieved mostly using controlled experimental conditions, such as isolated HS and hydroponically grown plants. Conceivably, these HS could directly interact with plant roots, microorganisms and soil particles in the rhizosphere. Thus, study of structural and chemical characteristics of HS present in soil solution and in the rhizosphere are needed to allow the transfer of knowledge obtained in controlled systems to real soil/rhizosphere conditions. This would help to shed light on the direct contribution of HS to plant nutrition and growth and on their usefulness in the field. Evidence of a relationship between chemical structural characteristics of HS obtained from different sources and having variable molecular complexity and the biological effects they exert on plants has been already provided. This kind of studies can now be performed using new analytical techniques thus allowing a full characterization of HS based on their origin, either natural or anthropogenic.
Concerning Fe nutrition, these aspects would be very useful considering the dual role that has been attributed to HS, as chelating compounds and biostimulants (Table 1). The capability of HS to form stable complexes with Fe and to directly affect Fe-acquisition mechanisms would account for the relative contribution of Fe-HS complexes to plant Fe nutrition as compared to other Fe-complexes naturally occurring in the rhizosphere.
It is noteworthy that HS induce a “nutrient acquisition response” even when plants are adequately supplied or during the recovery from a deficiency status, affecting functionality and regulation of nutrient uptake mechanisms. The signaling network at the basis of this behavior starts to be elucidated. Furthermore, it has been suggested that the cross-interaction between root exudates and HS might be part of the cross-talk between plant and soil. These features would favor a prompt adaptation of plants to a specific environment.
Another point of interest studying the behavior of HS is their possible use to develop environmentally friendly fertilization tools, being crucial in terms of circular economy. Although their chemical structure is not yet fully understood and the direct transfer of results obtained in controlled conditions to real soil has been questioned, it is quite clear that HS isolated from different organic sources, when added to nutrient solution or to the soil can favor plant nutrition, and especially nitrogen and Fe accumulation. This implies that humic fractions with different chemical and biological properties could be used to tailor HS-based fertilizers with high use efficiency. This tool could be particularly relevant for precision agriculture aimed at limiting external inputs and optimizing the use of natural resources by crops.
Author Contributions
LZ, NT, and RP wrote the manuscript and SC and ZV critically revised the manuscript. All authors approved the final version of the manuscript.
Funding
This work was supported by Dipartimento di Scienze AgroAlimentari, Ambientali e Animali (Di4A), University of Udine (PRID Startup 2018: DOM-BIO).
Conflict of Interest Statement
The authors declare that the research was conducted in the absence of any commercial or financial relationships that could be construed as a potential conflict of interest.
The reviewer YP declared a shared affiliation, though no other collaboration, with one of the authors SC to the handling Editor.
Abbreviations
ABA, abscisic acid; CK, cytokinins; DOM, dissolved organic matter; ET, ethylene; FA, fluvic acids; FRO, ferric chelate reductase; GA, gibberellic acid; HA, humic acids; HS, humic substances; IAA, indole-3-acetic acids; IHS, insoluble HS; IRT, iron transporter; NO, nitric oxide; NRAMP, natural resistance-associated macrophage proteins; PS, phytosiderophores; ROS, reactive oxygen species; TCA, tricarboxylic acid; WEHS, water extractable humic substances.
References
Abros’kin, D. P., Fuentes, M., Garcia-Mina, J. M., Klyain, O. I., Senik, S. V., Volkov, D. S., et al. (2016). The effect of humic acids and their complexes with iron on the functional status of plants grown under iron deficiency. Euras. Soil Sci. 49, 1099–1108. doi: 10.1134/s1064229316100021
Aguirre, E., Leménager, D., Bacaicoa, E., Fuentes, M., Baigorri, R., Zamarreño, A. M., et al. (2009). The root application of a purified leonardite humic acid modifies the transcriptional regulation of the main physiological root responses to Fe deficiency in Fe-sufficient cucumber plants. Plant Physiol. Biochem. 47, 215–223. doi: 10.1016/j.plaphy.2008.11.013
Alberts, J. J., and Takács, M. (2004). Comparison of the natural fluorescence distribution among size fractions of terrestrial fulvic and humic acids and aquatic natural organic matter. Org. Geochem. 35, 1141–1149. doi: 10.1016/j.orggeochem.2004.06.010
Billard, V., Etienne, P., Jannin, L., Garnica, M., Cruz, F., Garcia-Mina, J. M., et al. (2013). Two biostimulants derived from algae or humic acid induce similar responses in the mineral content and gene expression of winter oilseed rape (Brassica napus L.). J. Plant Growth Regul. 33, 305–316. doi: 10.1007/s00344-013-9372-2
Bocanegra, M. P., Lobartini, J. C., and Orioli, G. A. (2004). Fe-humate as a source of iron for plants. Commun. Soil Sci. Plant Anal. 35, 2567–2576. doi: 10.1081/lcss-200030378
Bocanegra, M. P., Lobartini, J. C., and Orioli, G. A. (2006). Plant uptake of iron chelated by humic acids of different molecular weights. Commun. Soil Sci. Plan. 37, 239–248. doi: 10.1080/00103620500408779
Bolan, N. S., Adriano, D. C., Kunhikrishnan, A., James, T., McDowell, R., and Senesi, N. (2011). Dissolved organic matter: biogeochemistry, dynamics, and environmental significance in soils. Adv. Agron. 110, 1–75.
Canellas, L. P., and Olivares, F. L. (2014). Physiological responses to humic substances as plant growth promoter. Chem. Biol. Technol. Agric. 1, 1–11.
Canellas, L. P., Olivares, F. L., Canellas, N. O. A., Mazzei, P., and Piccolo, A. (2019). Humic acids increase the maize seedlings exudation yield. Chem. Biol. Technol. Agric. 6:3.
Canellas, L. P., Olivares, F. L., Okorokova-Façanha, A. L., and Façanha, A. R. (2002). Humic acids isolated from earthworm compost enhance root elongation, lateral root emergence, and plasma membrane HC-ATPase activity in maize roots. Plant Physiol. 130, 1951–1957. doi: 10.1104/pp.007088
Cesco, S., Nikolic, M., Römheld, V., Varanini, Z., and Pinton, R. (2002). Uptake of 59Fe from soluble 59Fe-humate by cucumber and barley plants. Plant Soil 241, 121–128.
Cesco, S., Römheld, V., Varanini, Z., and Pinton, R. (2000). Solubilization of iron by water-extractable humic substances. J. Plant Nutr. Soil Sci. 163, 285–290. doi: 10.1002/1522-2624(200006)163:3<285::aid-jpln285>3.0.co;2-z
Chen, Y., Clapp, C. E., and Magen, H. (2004a). Mechanisms of plant growth stimulation by humic substances: the role of organo-iron complexes. Soil Sci. Plant Nutr. 50, 1089–1095. doi: 10.1080/00380768.2004.10408579
Chen, Y., De Nobili, M., and Aviad, T. (2004b). “Stimulatory effects of humic substances on plant growth,” in Soil Organic Matter in Sustainable Agriculture, eds F. Magdoff and R. R. Weil (New York, NY: CRC Press), 103–130.
Chen, Y., and Schnitzer, M. (1978). The surface tension of aqueous solutions of soil humic substances. Soil Sci. 125, 7–15. doi: 10.1097/00010694-197801000-00002
Cieschi, M. T., Caballero-Molada, M., Menéndez, N., Naranjo, M. A., and Lucena, J. J. (2017). Long-term effect of a leonardite iron humate improving Fe nutrition as revealed in silico, in vivo, and in field experiments. J. Agr. Food Chem. 65, 6554–6563. doi: 10.1021/acs.jafc.7b01804
Cieschi, M. T., and Lucena, J. J. (2018). Iron and humic acid accumulation on soybean roots fertilized with leonardite iron humates under calcareous conditions. J. Agr. Food Chem. 66, 13386–13396. doi: 10.1021/acs.jafc.8b04021
Colombo, C., Palumbo, G., He, J. Z., Pinton, R., and Cesco, S. (2014). Review on iron availability in soil: interaction of Fe minerals, plants, and microbes. J Soils Sed. 14, 538–548. doi: 10.1007/s11368-013-0814-z
Colombo, C., Palumbo, G., Sellitto, V. M., Rizzardo, C., Tomasi, N., Pinton, R., et al. (2012). Characteristics of insoluble, high molecular weight Fe-humic substances used as plant Fe sources. Soil Sci. Soc. Am. J. 76, 1246–1256.
Crowley, D. (2001). “Function of siderophores in the plant rhizosphere,” in The Rhizosphere: Biochemistry and Organic Substances at the Soil-Plant Interface, eds R. Pinton, Z. Varanini, and P. Nannipieri (New York, NY: Marcel Dekker), 223–261.
D’Orazio, V., and Senesi, N. (2009). Spectroscopic properties of humic acids isolated from the rhizosphere and bulk soil compartments and fractionated by size-exclusion chromatography. Soil Biol. Biochem. 41, 1775–1781. doi: 10.1016/j.soilbio.2008.02.001
Esfahani, M. R., Stretz, H. A., and Wells, M. J. M. (2015). Abiotic reversible self-assembly of fulvic and humic acid aggregates in low electrolytic conductivity solutions by dynamic light scattering and zeta potential investigation. Sci. Total Environ. 537, 81–92. doi: 10.1016/j.scitotenv.2015.08.001
Fuentes, M., Olaetxea, M., Baigorri, R., Zamarreño, A. M., Etienne, P., Laîné, P., et al. (2013). Main binding sites involved in Fe(III) and Cu(II) complexation in humic-based structures. J. Geochem. Exp. 129, 14–17. doi: 10.1016/j.gexplo.2012.12.015
García, A. C., de Souza, L. G. A., Pereira, M. G., Castro, R. N., García-Mina, J. M., Zonta, E., et al. (2016a). Structure-property-function relationship in humic substances to explain the biological activity in plants. Sci. Rep. 6:e20798. doi: 10.1038/srep20798
García, A. C., Olaetxea, M., Santos, L. A., Mora, V., Baigorri, R., Fuentes, M., et al. (2016b). Involvement of hormone-and ROS-signaling pathways in the beneficial action of humic substances on plants growing under normal and stressing conditions. BioMed. Res. Int. 2016:3747501. doi: 10.1155/2016/3747501
García, A. C., Santos, L. A., de Souza, L. G. A., Tavares, O. C. H., Zonta, E., Gomes, E. T. M., et al. (2016c). Vermicompost humic acids modulate the accumulation and metabolism of ROS in rice plants. J. Plant Physiol. 192, 56–63. doi: 10.1016/j.jplph.2016.01.008
García, A. C., Santos, L. A., Izquierdo, F. G., Sperandio, M. V. L., Castro, R. N., and Berbara, R. L. L. (2012). Vermicompost humic acids as an ecological pathway to protect rice plant against oxidative stress. Ecol. Eng. 47, 203–208. doi: 10.1016/j.ecoleng.2012.06.011
Garcia-Mina, J. M. (2006). Stability, solubility and maximum metal binding capacity in metal–humic complexes involving humic substances extracted from peat and organic compost. Org. Geochem. 37, 1960–1972. doi: 10.1016/j.orggeochem.2006.07.027
Garcia-Mina, J. M. (2007). Advantages and limitations of the use of an extended polyelectrolyte model to describe the proton-Binding process in macromolecular systems. application to a poly(acrylic acid) and a humic acid. J. Phys. Chem. B 111, 4488–4494. doi: 10.1021/jp0689518
Garcia-Mina, J. M., Antolín, M. C., and Sanchez-Diaz, M. (2004). Metal-humic complexes and plant micronutrient uptake: a study based on different plant species cultivated in diverse soil types. Plant Soil 258, 57–68. doi: 10.1023/b:plso.0000016509.56780.40
Gerke, J. (1993). Solubilization of Fe(III) from humic-Fe complexes, humic/Fe-oxide mixtures and from poorly ordered Fe-oxide by organic acids - consequences for P adsorption. Z. Pflanzenernähr. Bodenk. 156, 253–257. doi: 10.1002/jpln.19931560311
Gerke, J. (1997). Aluminum and iron(III) species in the soil solution including organic complexes with citrate and humic substances. J. Plant Nutr. Soil Sci. 160, 427–432. doi: 10.1002/jpln.19971600313
Gerke, J. (2010). Humic (organic matter)-Al(Fe)-phosphate complexes: an underestimated phosphate form in soils and source of plant-available phosphate. Soil Sci. 175, 417–425. doi: 10.1097/ss.0b013e3181f1b4dd
Gerke, J. (2018). Concepts and misconceptions of humic substances as the stable part of soil organic matter: a review. Agronomy 8:76. doi: 10.3390/agronomy8050076
Gumiński, S., Sulej, J., and Glabiszewski, J. (1983). Influence of sodium humate on the uptake of some ions by tomato seedlings. Acta Soc. Bot. Pol. 52, 149–164. doi: 10.5586/asbp.1983.017
Hayes, M. H. B. (2006). Solvent systems for the isolation of organic components from soils. Soil Sci. Soc. Am. J. 70, 986–994.
Jannin, L., Arkoun, M., Ourry, A., Laîné, P., Goux, D., Garnica, M., et al. (2012). Microarray analysis of humic acid effects on Brassica napus growth: involvement of N, C and S metabolisms. Plant Soil 359, 297–319. doi: 10.1007/s11104-012-1191-x
Jindo, K., Soares, T. S., Peres, L. E. P., Azevedo, I. G., Aguiar, N. O., Mazzei, P., et al. (2016). Phosphorus speciation and high-affinity transporters are influenced by humic substances. J. Plant Nutr. Soil Sci. 179, 206–214. doi: 10.1002/jpln.201500228
Kovács, K., Czech, V., Fodor, F., Solti, A., Lucena, J. J., Santos-Rosell, S., et al. (2013). Characterization of fe–leonardite complexes as novel natural iron fertilizers. J. Agr. Food Chem. 61, 12200–12210. doi: 10.1021/jf404455y
Kulikova, N. A., Badun, G. A., Korobkov, V. I., Chernysheva, M. G., Tsvetkova, E. A., Abroskin, D. P., et al. (2014). Accumulation of coal humic acids by wheat seedlings: direct evidence using tritium autoradiography and occurrence in lipid fraction. J. Plant Nutr. Soil Sci. 177, 875–883. doi: 10.1002/jpln.201300648
Lehmann, J., and Kleber, M. (2015). The contentious nature of soil organic matter. Nature 528, 60–68. doi: 10.1038/nature16069
Lucena, J. J. (2003). Fe chelates for remediation of Fe chlorosis in strategy I plants. J. Plant Nutr. 26, 1969–1984. doi: 10.1002/jsfa.5726
Mimmo, T., Del Buono, D., Terzano, R., Tomasi, N., Vigani, G., Crecchio, C., et al. (2014). Rhizospheric organic compounds in the soil–microorganism–plant system: their role in iron availability. Eur. J. Soil Sci. 65, 629–642. doi: 10.1111/ejss.12158
Mora, V., Bacaicoa, E., Zamarreño, A. M., Aguirre, E., Garnica, M., Fuentes, M., et al. (2010). Action of humic acid on promotion of cucumber shoot growth involves nitrate-related changes associated with the root-to-shoot distribution of cytokinins, polyamines and mineral nutrients. J. Plant Physiol. 167, 633–642. doi: 10.1016/j.jplph.2009.11.018
Mora, V., Baigorri, R., Bacaicoa, E., Zamarreño, A. M., and García-Mina, J. M. (2012). The humic acid-induced changes in the root concentration of nitric oxide, IAA and ethylene do not explain the changes in root architecture caused by humic acid in cucumber. Environ. Exp. Bot. 76, 24–32. doi: 10.1016/j.envexpbot.2011.10.001
Muscolo, A., Sidari, M., and Nardi, S. (2013). Humic substance: relationship between structure and activity. deeper information suggests univocal findings. J. Geochem. Explor. 129, 57–63. doi: 10.1016/j.gexplo.2012.10.012
Nardi, S., Muscolo, A., Vaccaro, S., Baiano, S., Spaccini, R., and Piccolo, A. (2007). Relationship between molecular characteristics of soil humic fractions and glycolytic pathway and Krebs cycle in maize seedlings. Soil Biol. Biochem. 39, 3138–3146. doi: 10.1016/j.soilbio.2007.07.006
Nardi, S., Pizzeghello, D., Muscolo, A., and Vianello, A. (2002). Physiological effects of humic substances on higher plants. Soil Biol. Biochem. 34, 1527–1536. doi: 10.1016/s0038-0717(02)00174-8
Nebbioso, A., and Piccolo, P. (2011). Basis of a humeomics science: chemical fractionation and molecular characterization of humic biosuprastructures. Biomacromolecules 12, 1187–1199. doi: 10.1021/bm101488e
Nevin, K. P., and Lovley, D. R. (2002). Mechanisms for Fe(III) oxide reduction in sedimentary environments. Geomicrobiol. J. 19, 141–159. doi: 10.1080/01490450252864253
Olaetxea, M., De Hita, D., Garcia, C. A., Fuentes, M., Baigorri, R., Mora, V., et al. (2018). Hypothetical framework integrating the main mechanisms involved in the promoting action of rhizospheric humic substances on plant root- and shoot- growth. Appl. Soil Ecol. 123, 521–537. doi: 10.1016/j.apsoil.2017.06.007
Olk, D. C., Bloom, P. R., Perdue, E. M., McKnight, D. M., Chen, Y., Farenhorst, A., et al. (2019). Environmental and agricultural relevance of humic fractions extracted by alkali from soils and natural waters. J. Environ. Qual. 48, 217–232. doi: 10.2134/jeq2019.02.0041
Pandeya, S. B., Singh, A. K., and Dhar, P. (1998). Influence of fulvic acid on transport of iron in soils and uptake by paddy seedlings. Plant Soil 198, 117–125.
Piccolo, A. (2002). The supramolecular structure of humic substances: a novel understanding of humus chemistry and implications in soil science. Adv. Agron. 75, 57–134. doi: 10.1016/s0065-2113(02)75003-7
Piccolo, A., Conte, P., Spaccini, R., and Chiarella, M. (2003). Effects of some dicarboxylic acids on the association of dissolved humic substances. Biol. Fertil. Soils 37, 255–259.
Pinton, R., Cesco, S., De Nobili, M., Santi, S., and Varanini, Z. (1997). Water and pyrophosphate-extractable humic substances fractions as a source of iron for Fe-deficient cucumber plants. Biol. Fertil. Soils 26, 23–27. doi: 10.1007/s003740050337
Pinton, R., Cesco, S., Iacolettig, G., Astolfi, S., and Varanini, Z. (1999). Modulation of NO3- uptake by water-extractable humic substances: involvement of root plasma membrane H+ ATPase. Plant Soil 215, 155–161.
Puglisi, E., Fragoulis, G., Ricciuti, P., Cappa, F., Spaccini, R., Piccolo, A., et al. (2009). Effects of a humic acid and its size-fractions on the bacterial community of soil rhizosphere under maize (Zea mays L.). Chemosphere 77, 829–837. doi: 10.1016/j.chemosphere.2009.07.077
Puglisi, E., Pascazio, S., Suciu, N., Cattani, I., Fait, G., Spaccini, R., et al. (2013). Rhizosphere microbial diversity as influenced by humic substance amendments and chemical composition of rhizodeposits. J. Geochem. Explor. 129, 82–94. doi: 10.1016/j.gexplo.2012.10.006
Quaggiotti, S., Ruperti, B., Pizzeghello, D., Francioso, O., Tugnoli, V., and Nardi, S. (2004). Effect of low molecular size humic substances on nitrate uptake and expression of genes involved in nitrate transport in maize (Zea mays L.). J. Exp. Bot. 55, 803–813. doi: 10.1093/jxb/erh085
Rakshit, S., Uchimiya, M., and Sposito, G. (2009). Iron(III) bioreduction in soil in the presence of added humic substances. Soil Sci. Soc. Am. J. 73, 65–71.
Ramos, A. C., Dobbss, L. B., Santos, L. A., Fernandes, M. S., Olivares, F. L., Aguiar, N. O., et al. (2015). Humic matter elicits proton and calcium fluxes and signalling dependent on Ca2+-dependent protein kinase (CDPK) at early stages of lateral plant root development. Chem. Biol. Tech. Agr. 2:3. doi: 10.1186/s40538-014-0030-0
Roden, E. E., Kappler, A., Bauer, I., Jiang, J., Paul, A., Stoesser, R., et al. (2010). Extracellular electron transfer through microbial reduction of solid-phase humic substances. Nat. Geosci. 3:417. doi: 10.1038/ngeo870
Rose, M. T., Patti, A. F., Little, K. R., Brown, A. L., Jackson, W. R., and Cavagnaro, T. R. (2014). A meta-analysis and review of plant-growth response to humic substances: practical implications for agriculture. Adv. Agron. 124, 37–89. doi: 10.1016/b978-0-12-800138-7.00002-4
Ryan, P. R., Delhaize, E., and Jones, D. L. (2001). Function and mechanism of organic anion exudation from plant roots. Annu. Rev. Plant Phys. 52, 527–560.
Schiavon, M., Pizzeghello, D., Muscolo, A., Vaccaro, S., Francioso, O., and Nardi, S. (2010). High molecular size humic substances enhance phenylpropanoid metabolism in maize (Zea mays L.). J. Chem. Ecol. 36, 662–669. doi: 10.1007/s10886-010-9790-6
Schmidt, W., Santi, S., Pinton, R., and Varanini, Z. (2007). Water-extractable humic substances alter root development and epidermal cell pattern in Arabidopsis. Plant Soil 300, 259–267. doi: 10.1007/s11104-007-9411-5
Schwertmann, U. (1991). Solubility and dissolution of iron oxides. Plant Soil 130, 1–25. doi: 10.1007/bf00011851
Senesi, N. (1992). “Metal-Humic substance complexes in the environment. Molecular and mechanistic aspects by multiple spectroscopic approach,” in Biogeochemistry of Trace Metals, ed. D. M. Adriano (Boca Raton: Lewis Publishers), 429–495.
Skogerboe, R. K., and Wilson, S. A. (1981). Reduction of ionic species by fulvic acid. Wilson A. Anal. Chem. 53, 228–232. doi: 10.1021/ac00225a023
Stevenson, F. J. (1994). Humus Chemistry. Genesis, Composition, Reactions, 2nd Edn. New York, NY: Wiley.
Struyk, Z., and Sposito, G. (2001). Redox properties of standard humic acids. Geoderma 102, 329–346. doi: 10.1016/s0016-7061(01)00040-4
Tavares, O. C. H., Santos, L. A., Ferreira, L. M., Sperandio, M. V. L., da Rocha, J. G., Garcia, A. C., et al. (2017). Humic acid differentially improves nitrate kinetics under low and high-affinity systems and alters the expression of plasma membrane H+-ATPases and nitrate transporters in rice. Ann. Appl. Biol. 170, 89–103. doi: 10.1111/aab.12317
Tipping, E. (2002). Cation Binding by Humic Substances. Cambridge: Cambridge University Press, 1–434.
Tomasi, N., De Nobili, M., Gottardi, S., Zanin, L., Mimmo, T., Varanini, Z., et al. (2013). Physiological and molecular aspects of Fe acquisition by tomato plants from natural Fe complexes. Biol. Fertil. Soil 49, 187–200. doi: 10.1007/s00374-012-0706-1
Tomasi, N., Mimmo, T., Terzano, R., Alfeld, M., Janssens, K., Zanin, L., et al. (2014). Nutrient accumulation in leaves of Fe-deficient cucumber plants treated with natural Fe complexes. Biol. Fert. Soils 50, 973–982. doi: 10.1007/s00374-014-0919-6
Tomasi, N., Rizzardo, C., Monte, R., Gottardi, S., Jelali, N., Terzano, R., et al. (2009). Micro-analytical, physiological and molecular aspects of Fe acquisition in leaves of Fe-deficient tomato plants re-supplied with natural Fe-complexes in nutrient solution. Plant Soil 325, 25–38. doi: 10.1007/s11104-009-0069-z
Trevisan, S., Botton, A., Vaccaro, S., Vezzaroa, A., Quaggiotti, S., and Nardi, S. (2011). Humic substances affect Arabidopsis physiology by altering the expression of genes involved in primary metabolism, growth and development. Environ. Exp. Bot. 74, 45–55. doi: 10.1016/j.envexpbot.2011.04.017
Trevisan, S., Pizzeghello, D., Ruperti, B., Francioso, O., Sassi, A., Palme, K., et al. (2010). Humic substances induce lateral root formation and expression of the early auxin-responsive IAA19 gene and DR5 synthetic element in Arabidopsis. Plant Biol. 12, 604–614. doi: 10.1111/j.1438-8677.2009.00248.x
Urrutia, O., Erro, J., Guardado, I., Mandado, M., and Garcia-Mina, J. M. (2013). Theoretical chemical characterization of phospho-metal humic complexes and relationships with their effects on both phosphorus soil fixation and phosphorus availability for plants. J. Sci. Food Agric. 93, 293–303. doi: 10.1002/jsfa.5756
Urrutia, O., Erro, J., Guardado, I., San Francisco, S., Mandado, M., Baigorri, R., et al. (2014). Physico-chemical characterization of humic-metalphosphate complexes and their potential application to the manufacture of new types of phosphate-based fertilizers. J. Plant Nutr. Soil Sci. 177, 128–136. doi: 10.1002/jpln.201200651
Vaccaro, S., Ertani, A., Nebbioso, A., Muscolo, A., Quaggiotti, S., Piccolo, A., et al. (2015). Humic substances stimulate maize nitrogen assimilation and amino acid metabolism at physiological and molecular level. Chem. Biol. Technol. Agric. 2:5. doi: 10.1186/s40538-015-0033-5
Varanini, Z., and Pinton, R. (2001). “Direct versus indirect effects of soil humic substances on plant growth and nutrition,” in The Rizosphere, eds R. Pinton, Z. Varanini, and P. Nannipieri (Basel: Marcel Dekker), 141–158.
Varanini, Z., Pinton, R., De Biasi, M. G., Astolfi, S., and Maggioni, A. (1993). Low molecular weight humic substances stimulate H+-ATPase activity of plasma membrane vesicles isolated from oat (Avena sativa L.) roots. Plant Soil 153, 61–69. doi: 10.1007/bf00010544
von Wirén, N., Khodr, H., and Hider, R. C. (2000). Hydroxylated phytosiderophore species possess an enhanced chelate stability and affinity for Iron(III). Plant Physiol. 124, 1149–1158. doi: 10.1104/pp.124.3.1149
Vujinovic, T., Contin, M., Cesco, S., Pinton, R., Tomasi, N., Ceccon, P., et al. (2013). “Characterization of humic fractions in leachates from soil under organic and conventional management and their interactions with the root zone,” in Functions of Natural Organic Matter in Changing Environment, eds J. Xu, J. Wu, and Y. He (Dordrecht: Springer).
Zamboni, A., Zanin, L., Tomasi, N., Avesani, L., Pinton, R., Varanini, Z., et al. (2016). Early transcriptomic response to Fe supply in Fe-deficient tomato plants is strongly influenced by the nature of the chelating agent. BMC Genomics 17:35. doi: 10.1186/s12864-015-2331-5
Zandonadi, D. B., Santos, M. P., Busato, J. G., Peres, L. E. P., and Façanha, A. R. (2013). Plant physiology as affected by humified organic matter. Theor. Exp. Plant Phys. 25, 12–25.
Zandonadi, D. B., Santos, M. P., Caixeta, L. S., Marinho, E. B., Peres, L. E. P., and Façanha, A. R. (2016). Plant proton pumps as markers of biostimulant action. Sci. Agric. 73, 24–28. doi: 10.1590/0103-9016-2015-0076
Zandonadi, D. B., Santos, M. P., Dobbss, L. B., Olivares, F. L., Canellas, L. P., Binzel, M. L., et al. (2010). Nitric oxide mediates humic acids induced root development and plasma membrane H+-ATPase activation. Planta 231, 1025–1036. doi: 10.1007/s00425-010-1106-0
Zanin, L., Tomasi, N., Rizzardo, C., Gottardi, S., Terzano, R., Alfeld, M., et al. (2015). Iron allocation in leaves of Fe-deficient cucumber plants fed with natural Fe complexes. Physiol. Plant. 154, 82–94. doi: 10.1111/ppl.12296
Keywords: Fe complex, Fe chelates, fulvic acids, root uptake, strategy I, strategy II, water-extractable humic substances (WEHS)
Citation: Zanin L, Tomasi N, Cesco S, Varanini Z and Pinton R (2019) Humic Substances Contribute to Plant Iron Nutrition Acting as Chelators and Biostimulants. Front. Plant Sci. 10:675. doi: 10.3389/fpls.2019.00675
Received: 05 February 2019; Accepted: 06 May 2019;
Published: 22 May 2019.
Edited by:
Thomas J. Buckhout, Humboldt University of Berlin, GermanyReviewed by:
Marta Fuentes, University of Navarra, SpainYoury Pii, Free University of Bozen-Bolzano, Italy
Copyright © 2019 Zanin, Tomasi, Cesco, Varanini and Pinton. This is an open-access article distributed under the terms of the Creative Commons Attribution License (CC BY). The use, distribution or reproduction in other forums is permitted, provided the original author(s) and the copyright owner(s) are credited and that the original publication in this journal is cited, in accordance with accepted academic practice. No use, distribution or reproduction is permitted which does not comply with these terms.
*Correspondence: Roberto Pinton, roberto.pinton@uniud.it