- 1Department of Ecology, College of Urban and Environmental Sciences, Peking University, Beijing, China
- 2College of Life Sciences, Capital Normal University, Beijing, China
- 3State Key Laboratory of Systematic and Evolutionary Botany, Institute of Botany, Chinese Academy of Sciences, Beijing, China
- 4Key Laboratory of Plant-Soil Interactions of the Ministry of Education, College of Resources and Environmental Sciences, China Agricultural University, Beijing, China
Allocation of biomass to different organs is a fundamental aspect of plant responses and adaptations to changing environmental conditions, but how it responds to nitrogen (N) and phosphorus (P) availability remains poorly addressed. Here we conducted greenhouse fertilization experiments using Arabidopsis thaliana, with five levels of N and P additions and eight repeat experiments, to ascertain the effects of N and P availability on biomass allocation patterns. N addition increased leaf and stem allocation, but decreased root and fruit allocation. P addition increased stem and fruit allocation, but decreased root and leaf allocation. Pooled data of the five levels of N addition relative to P addition resulted in lower scaling exponents of stem mass against leaf mass (0.983 vs. 1.226; p = 0.000), fruit mass against vegetative mass (0.875 vs. 1.028; p = 0.000), and shoot mass against root mass (1.069 vs. 1.324; p = 0.001). This suggested that N addition relative to P addition induced slower increase in stem mass with increasing leaf mass, slower increase in reproductive mass with increasing vegetative mass, and slower increase in shoot mass with increasing root mass. Further, the levels of N or P addition did not significantly affect the allometric relationships of stem mass vs. leaf mass, and fruit mass vs. vegetative mass. In contrast, increasing levels of N addition increased the scaling exponent of shoot to root mass, whereas increasing levels of P addition exerted the opposite influence on the scaling exponent. This result suggests that increasing levels of N addition promote allocation to shoot mass, whereas the increasing levels of P addition promote allocation to root mass. Our findings highlight that biomass allocation of A. thaliana exhibits a contrasting response to N and P availability, which has profound implications for forecasting the biomass allocation strategies in plants to human-induced nutrient enrichment.
Introduction
Allocation of biomass to different organs is a core component of plant life history and plays a pivotal role in the trade-off between resource acquisition and utilization (Bazzaz and Grace, 1997; Weiner, 2004; Eziz et al., 2017). Nutrient availability is one important factor driving the variability of biomass allocation (Müller et al., 2000; Poorter and Nagel, 2000; Fujita et al., 2014). Quantitative assessments of the impacts of nutrient availability on biomass allocation patterns could be instrumental in elucidating the responses and adaptations of plant growth and ecophysiological processes under global nutrient change (Müller et al., 2000; Shipley and Meziane, 2002; McCarthy and Enquist, 2007).
In recent decades, nitrogen (N) and phosphorus (P) fertilizers and depositions induced by human activities have enhanced the availability of N and P in terrestrial ecosystems (Galloway et al., 2008; Du et al., 2016). As two functionally coupled macroelements in biological systems, N and P have a critical bearing on plant growth and metabolic activities (Lambers et al., 2008; Ågren, 2008), and frequently limit primary productivity across various ecosystems (Aerts and Chapin, 2000; Elser et al., 2007). Previous studies have reported the effects of nutrient or N availability on biomass allocation (e.g., Bazzaz and Grace, 1997; Müller et al., 2000; Shipley and Meziane, 2002; Poorter et al., 2012; Fujita et al., 2014), with little knowledge about the responses of biomass allocation to varying P availability (but see Luo et al., 2016). Thus, considering the tightly coupled relationship between N and P and the imbalance of N vs. P inputs induced by human activities, it is imperative to disentangle the effects of N and P availability on biomass allocation concurrently.
Biomass allocation is often assessed as the fraction of each organ’s biomass to total plant biomass, which potentially reflects plant strategies to cope with resource limitation or disturbance (Poorter and Nagel, 2000; Weiner, 2004; Poorter et al., 2012). For instance, plants grown in nutrient-rich conditions relative to nutrient-poor conditions usually show lower root mass fraction (RMF, the proportion of biomass invested in roots) but higher leaf mass fraction (LMF, the proportion of biomass invested in leaves) and stem mass fraction (SMF, the proportion of biomass invested in stems), thereby increasing light interception and photosynthesis (Müller et al., 2000; de Groot et al., 2002; Poorter et al., 2012). Nutrient deficiency could increase plant reproductive allocation as plants adapted to poor conditions should maintain enough fecundity for survival (Sultan, 2001; Wang et al., 2016). On the other hand, allometric relationships of biomass among different organs are often used to explore the mechanisms of change in biomass allocation (Enquist and Niklas, 2002; Weiner, 2004; Niu et al., 2009; Poorter et al., 2015). Allometric analysis is usually considered in terms of allometric partitioning theory, which states that the responses of proportional biomass allocation to environmental factors are best explained by the changes in plant size along a fixed allometric trajectory (i.e., fixed scaling relationship) (Müller et al., 2000; Weiner, 2004; McCarthy and Enquist, 2007). Through synthesizing data of biomass from global seed plants, Enquist and Niklas (2002) concluded that leaf biomass should scale with the 3/4 power of both stem and root biomass, whereas root biomass should scale isometrically with stem biomass. Weiner et al. (2009) reviewed the relationship between reproductive and vegetative biomass, and revealed that short-lived herbaceous plants often exhibited a simple and linear relationship, whereas larger and longer-lived plants generally had an allometric relationship with a scaling exponent less than one.
To date, whether and how nutrient availability affects biomass allometric relationships among different organs remains contentious. Some studies suggested that biomass allocation patterns could be best explained by the fixed allometric strategies under changing nutrient conditions (Müller et al., 2000; Fortunel et al., 2009; Peng and Yang, 2016). For instance, Müller et al. (2000) observed that the allometric scaling relationship of aboveground against belowground biomass was unaffected by N addition in 21 out of 27 species. Similarly, through field investigations or meta-analysis, several studies have found that allometric relationships between aboveground and belowground biomass were reported to be isometric and rarely altered by nutrient availability (Fortunel et al., 2009; Yang et al., 2009; Peng and Yang, 2016). However, some studies emphasized that the bivariate scaling exponent of biomass between organs varied with nutrient availability (Shipley and Meziane, 2002; Huang et al., 2009; Luo et al., 2016; Yan B.G. et al., 2016). For instance, Shipley and Meziane (2002) found that the scaling exponent of aboveground against belowground biomass for 22 herbaceous species was altered by N addition. In an arid-hot grassland, the allometric relationship between stem and leaf biomass varied across a soil nutrient gradient, which influenced plant adaptation and distribution (Yan B.G. et al., 2016). These conflicting findings warrant more in-depth studies before drawing general conclusions. Besides, effects of N and P availability on the allometric relationships of biomass among different organs are rarely compared in concert (but see Luo et al., 2016).
In this study, we examined the effects of N and P availability on the biomass allocation of Arabidopsis thaliana, which is a model annual plant for molecular biological studies (Meinke et al., 1998). We conducted a series of greenhouse fertilization experiments with five levels of N and P additions and eight repeat experiments. Here we chose A. thaliana as the target species, mainly because it has a short lifespan and could be planted for several consecutive experiments during a short period. Specifically, this study aimed to address the following questions: (i) how do N and P availability affect the biomass allocation fractions? and (ii) how do N and P availability regulate the allometric relationships of biomass among different organs? Considering the differential roles of N and P in physiological functions and metabolic processes in plants, we hypothesize that (i) N addition increase leaf and stem allocation but decrease root and fruit allocation, whereas P addition increase stem and fruit allocation but decrease root and leaf allocation; (ii) N addition relative to P addition results in lower scaling exponents of stem mass against leaf mass, fruit mass against vegetative mass, and shoot mass against root mass.
Materials and Methods
Growth Conditions and Experimental Design
In this study, we performed the greenhouse N and P fertilization experiments using A. thaliana from ecotype “Columbia” (Yan et al., 2015; Yan Z.B. et al., 2016; Yan et al., 2018), and repeated eight such experiments consecutively. We carried out all these experiments under nearly the same greenhouse conditions and similar experimental processes. These experiments were implemented in a phytotron with 16-h light/8-h dark photoperiod at approximately 20°C and 60–70% relative humidity. Light was provided by one yellow sodium fluorescent lamp and five white fluorescent lamps. Before sowing, seeds were surface sterilized using 70% (v/v) ethanol-0.5% (v/v) Tween 20 and then stratified in a 0.1% (w/v) agar solution at 4°C in the dark for 4 days. Hereafter, seeds were sown in plastic pots with 24 small divisions in favor of raising 24 individuals per pot. These pots were filled with sterilized vermiculite (medium particle size) containing few mineral nutrients, and the base of each pot was periodically watered by respective nutrient solutions with various N and P concentrations. Altogether, there were 27 pots from nine nutrient treatments with three replicates for each. To diminish the impacts of microenvironment, we randomly rearranged these pots every 2 days.
The experiments included nine nutrient treatments, which consisted of five levels of N addition (1, 2, 4, 8, and 12 mmol N L-1, added as NH4NO3) at the intermediate level of P addition (0.25 mmol P L-1) and five levels of P addition (0.0625, 0.125, 0.25, 0.5, and 1.0 mmol L-1, added as KH2PO4 and NaH2PO4) at the intermediate level of N addition (4 mmol N L-1), respectively. We designed the levels of N and P addition mainly based on the dose-responses in our preliminary experiments (Yan et al., 2015). The intermediate level of nutrient addition was defined as the level when the shoot mass reached to the maximum value along the increasing levels of nutrient addition. The five levels of N addition were indicated by N1, N2, N3, N4, and N5, and the five levels of P addition were indicated by P1, P2, P3, P4, and P5. The intermediate levels of N and P additions were indicated by N3 and P3, respectively. N addition experiments shared the same treatment of N3P3 with P addition experiments. All nutrient solutions contained the same concentrations of other macro- and microelements (i.e., all nutrient solutions per litter equally contained 2 mmol CaCl2, 0.75 mmol K2SO4, 0.65 mmol MgSO4, 0.1 mmol Fe-EDTA, 0.01 mmol H3BO3, 1 μmol MnSO4, 1 μmol ZnSO4, 0.1 μmol CuSO4, and 0.035 μmol Na2MoO4) except for N and P. The pH of each nutrient solution was adjusted to 5.8. Elemental composition and concentrations of the nutrient solutions conformed to Hoagland’s formula (Hoagland and Arnon, 1950), and were then modified according to our preliminary experiments (Yan et al., 2015).
Sampling and Measurement
We sampled plants at the end of main stem inflorescences in A. thaliana when the fruits are mostly mature (designated as the “fruit maturity stage”). Sampling date was adjusted to match the individual ontogenetic stage, considering that plant growth rates varied among treatments. Leaves, stems and fruits were divided, and then oven-dried at 65°C to constant weight. Aboveground mass of each individual plant was obtained by pooling the mass of leaves, stems, and fruits together. Vegetative mass of each individual plant was obtained by pooling the mass of leaf and stem together. Biomass allocation fraction for each organ was calculated by the organ’s mass as a fraction of aboveground mass. Four individuals with almost similar growth for each replicate were sampled and measured. Root biomass allocation was not analyzed in current fertilization experiments, because of lack of root biomass data. To replenish the understanding of root biomass allocation under N and P additions, we used biomass data including roots from our preliminary experiment to examine effects of N and P additions on root to shoot (R:S) ratio and allometric relationship of shoot mass against root mass in A. thaliana.
Data Analyses
We conducted two types of analyses in this study. Firstly, changes of biomass allocation fractions along the levels of N and P additions were examined using one-way analysis of variance (ANOVA) and the least significant difference post hoc test. Secondly, we performed standardized major axis (SMA) regression analyses to explore allometric relationships of biomass among different organs under influences of the type and level of N and P additions. A likelihood ratio test was used to indicate the heterogeneity of the scaling exponents among different groups (Warton et al., 2006). Effects of the type of nutrient addition on those relationships were analyzed using pooled data of the five levels of N or P addition. Effects of the levels of nutrient addition on those relationships were explored using data from each level of N or P addition. Data was log10-transformed before SMA regression analysis. We conducted all statistical analyses using combined data from the eight repeat experiments in R 2.15.2 (R Development Core Team, 2012).
Results
N and P availability exhibited contrasting effects on biomass allocation fractions of A. thaliana (Figure 1). With increasing levels of N addition, LMF, and SMF initially increased and then leveled off at higher addition levels, whereas root to shoot (R:S) ratio and fruit mass fraction (FMF) initially decreased sharply and then leveled off at higher levels of N addition (Figures 1A, 2A and Supplementary Table S1). In contrast, with increasing levels of P addition, LMF and R:S ratio showed a rapid decrease at lower addition levels and then leveled off, whereas SMF and FMF presented a rapid increase at lower addition levels and then leveled off (Figures 1B, 2B and Supplementary Table S1).
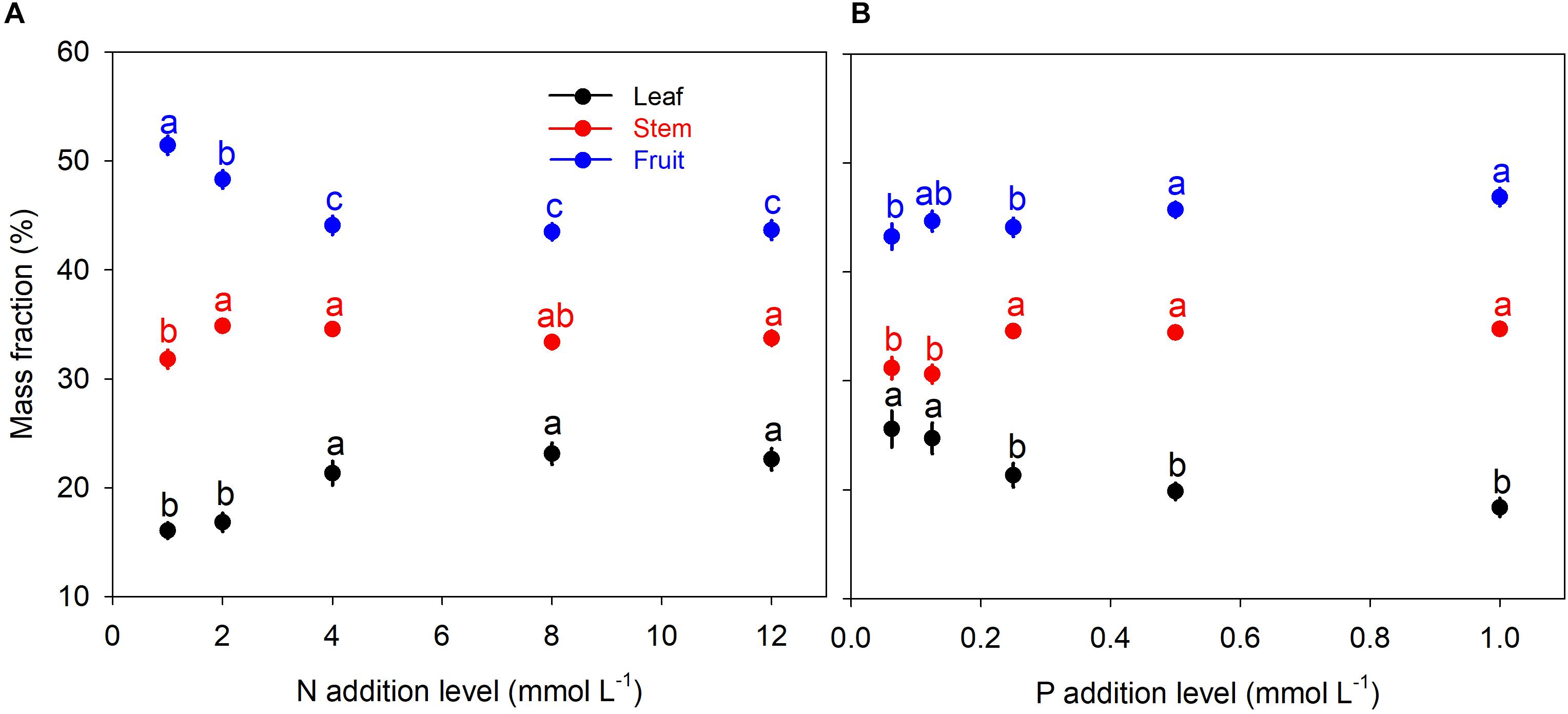
Figure 1. Biomass allocation fractions under different levels of N and P additions. (A) N additions; (B) P additions. Points and error bars denote the means and standard errors of biomass allocation fractions, respectively. Different letters above the error bars indicate significant difference (p < 0.05) among five levels of N or P addition based on one-way analysis of variance (ANOVA) and the least significant difference post hoc test.
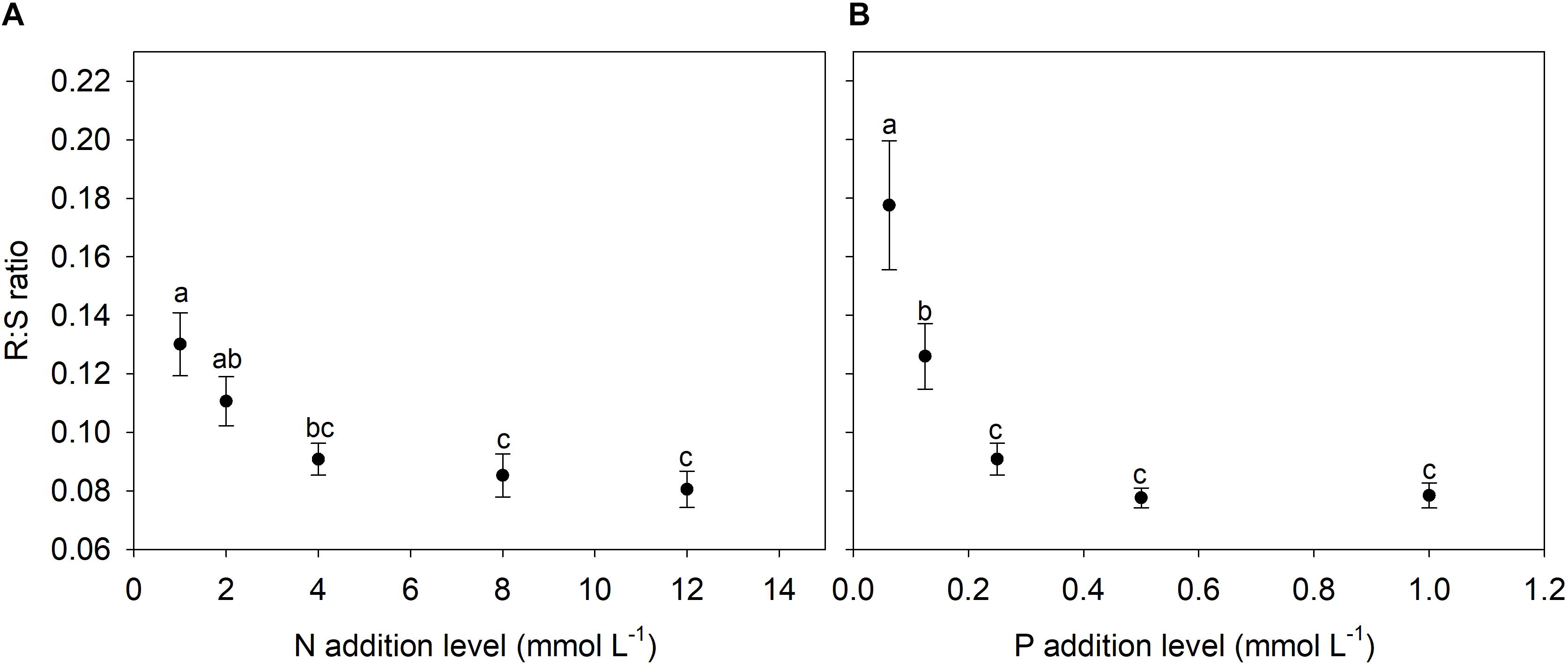
Figure 2. Changes in root to shoot (R:S) ratio with levels of N and P additions. (A) N additions; (B) P additions. Points and error bars denote the means and standard errors of R:S ratio, respectively. Different letters above the error bars indicate significant difference (p < 0.05) among five levels of N or P addition based on one-way analysis of variance (ANOVA) and the least significant difference post hoc test.
The type of nutrient addition regulated allometric relationships of biomass among different organs (Figures 3, 4). In comparison with pooled data of the five levels of P addition, pooled data of the five levels of N addition showed significantly lower scaling exponents of stem mass against leaf mass (0.983 vs. 1.226; p = 0.000), fruit mass against vegetative mass (0.875 vs. 1.028; p = 0.000), and shoot mass against root mass (1.069 vs. 1.324; p = 0.001) (Figures 3, 4). These results suggested that N additions relative to P additions caused slower increase in stem mass with increasing leaf mass, slower increase in reproductive mass with increasing vegetative mass, and slower increase in shoot mass with increasing root mass.
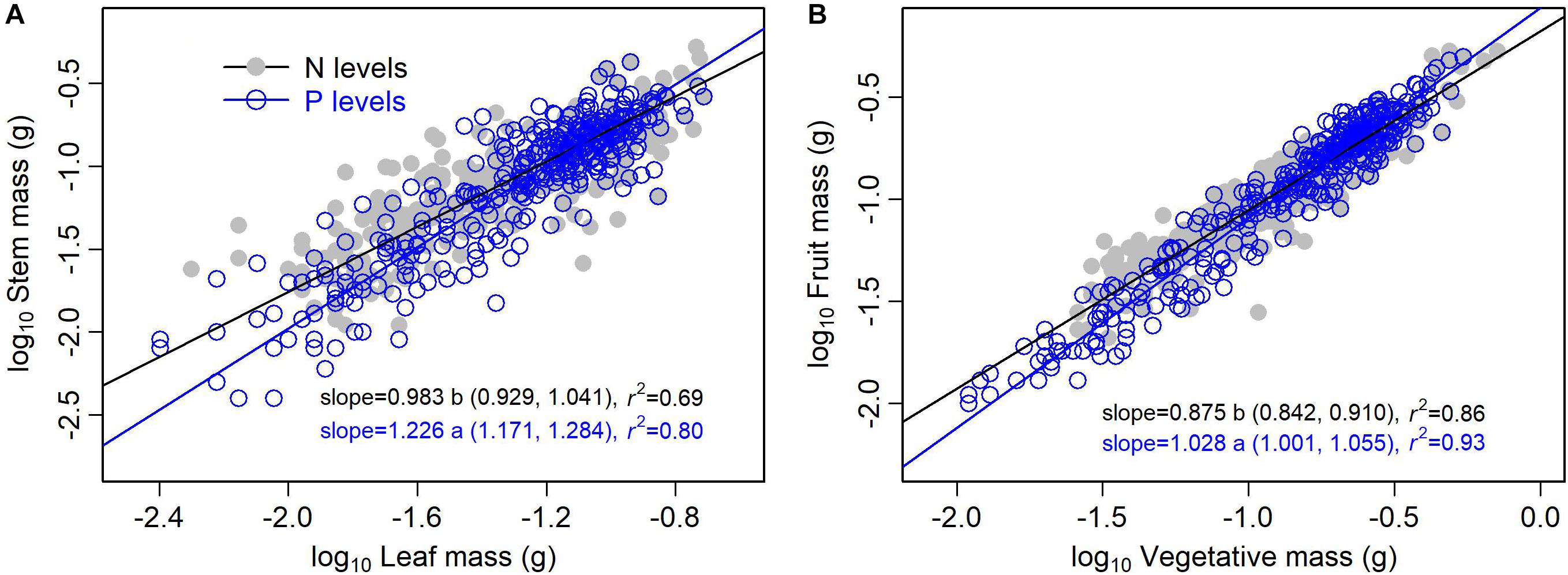
Figure 3. Effects of the type of nutrient addition (i.e., N additions vs. P additions) on the allometric relationships for (A) stem mass vs. leaf mass, and (B) fruit mass vs. vegetative mass. SMA regression is used to determine the significant line (p < 0.05). Relationships for N (or P) levels are examined using data pooled from the five levels of N (or P) addition. Numbers in square brackets are the lower and upper 95% confident intervals of the SMA slopes. Different letters after scaling slopes indicate significant difference (p < 0.05) based on a likelihood ratio test.
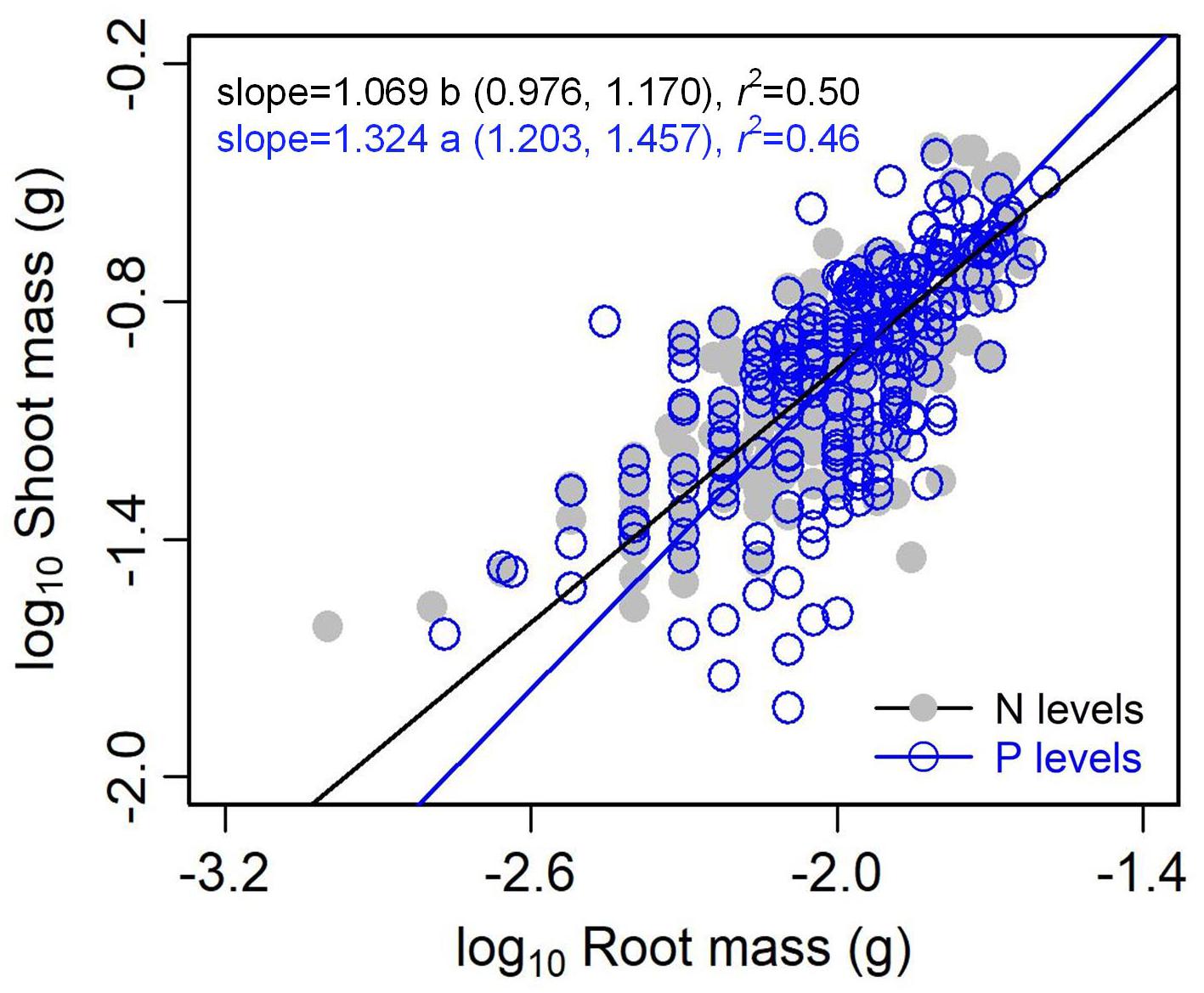
Figure 4. Effects of the type of nutrient addition (i.e., N addition vs. P addition) on the allometric relationships between shoot mass and root mass. SMA regression is used to determine the significant line (p < 0.05). Relationships for N (or P) levels are examined using data pooled from the five levels of N (or P) addition. Numbers in square brackets are the lower and upper 95% confident intervals of the RMA slopes. Different letters after scaling slopes indicate significant difference (p < 0.05) based on a likelihood ratio test.
The levels of N or P addition exerted little influences on allometric relationships of stem mass vs. leaf mass and fruit mass vs. vegetative mass (Table 1 and Figure 5). Scaling exponents and normalization constants of stem mass against leaf mass showed the insignificant difference among the five levels of N (or P) addition (Table 1 and Figures 5A,C). Similarly, scaling exponents and normalization constants of fruit mass against vegetative mass also exhibited the insignificant difference among the five levels of N (or P) addition (Table 1 and Figures 5B,D). In contrast, increasing levels of N addition increased the scaling exponent of shoot to root mass, whereas increasing levels of P addition exerted the opposite influence on the scaling exponent (Table 2). This result suggested that increasing levels of N addition promoted allocation to shoot mass, whereas increasing levels of P addition promoted allocation to root mass.
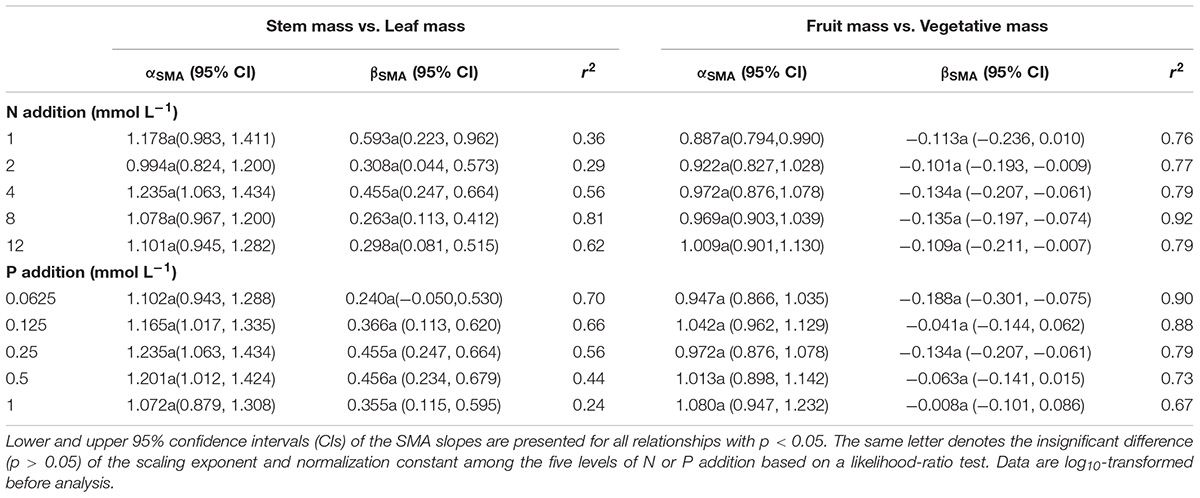
Table 1. Summary of the SMA regressions about the allometric relationships of biomass among different organs [e.g., log10 Stem mass = α∗log10 (Leaf mass)+β] for various levels of N or P addition.
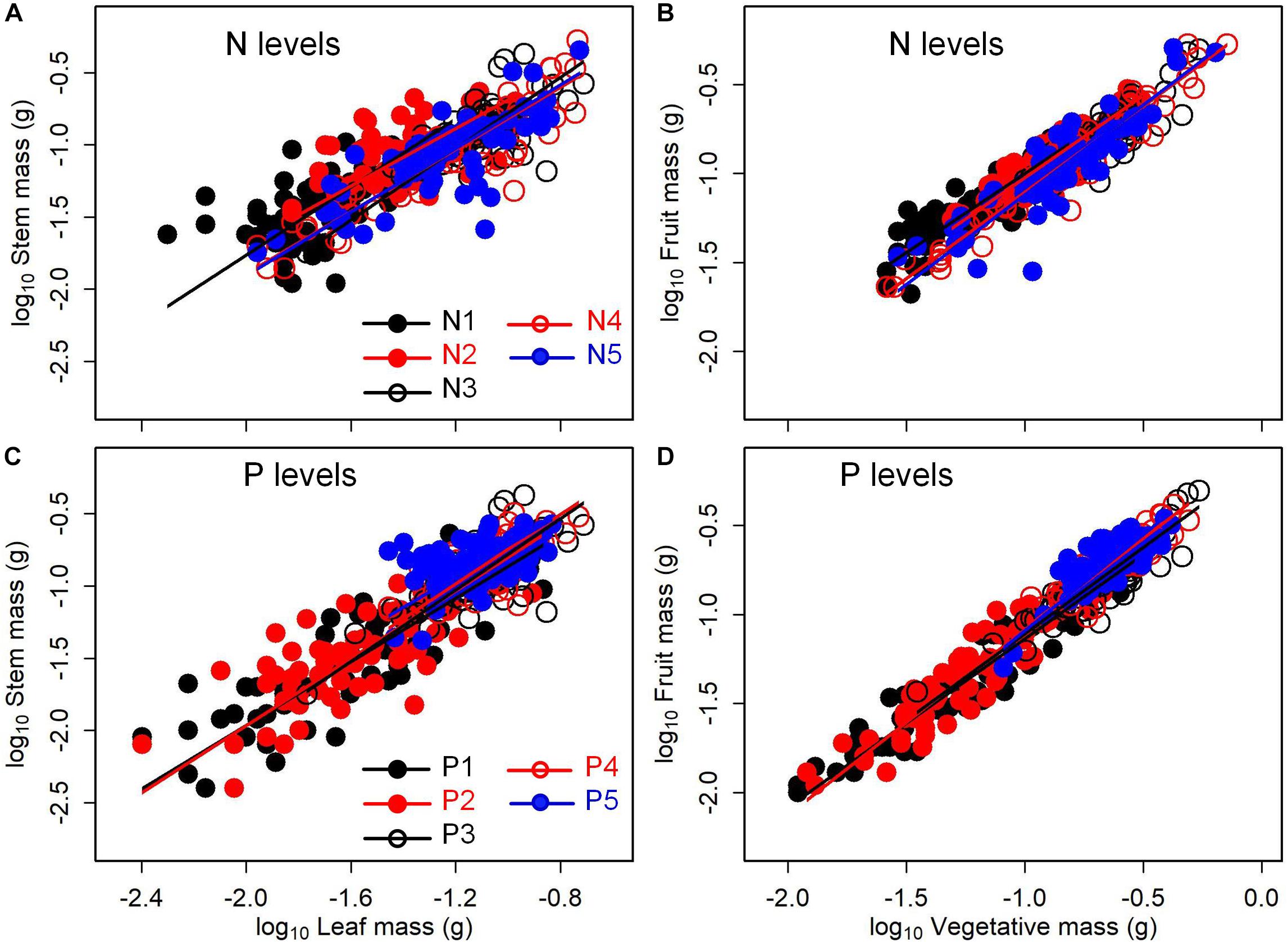
Figure 5. Effects of the levels of N and P addition on the allometric relationships for (A,C) stem mass vs. leaf mass, and (B,D) fruit mass vs. vegetative mass. SMA regression is used to determine significant line (p < 0.05) for each level of N or P addition.
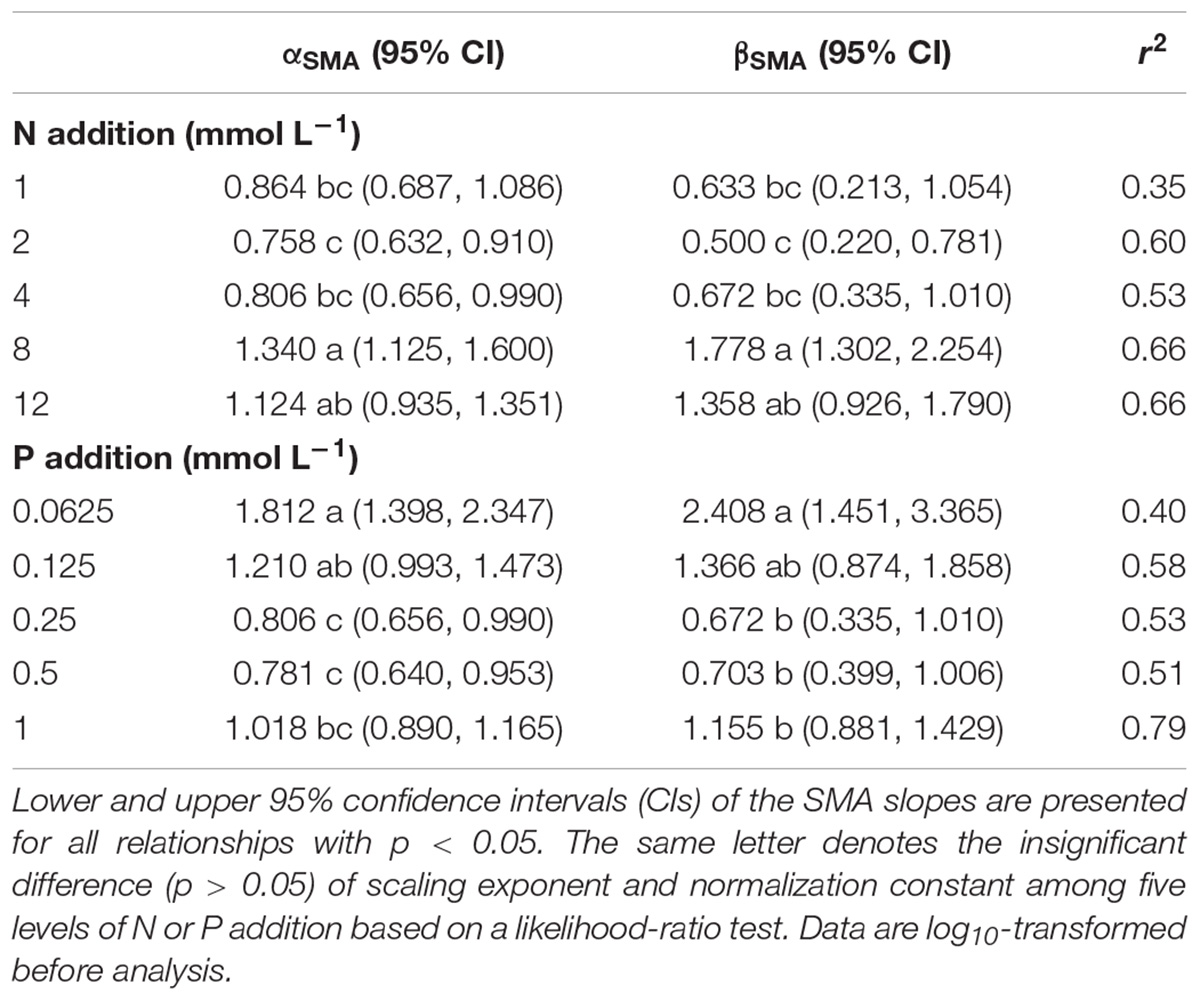
Table 2. Summary of the SMA regressions about the allometric relationships between shoot mass and root mass [e.g., log10 Shoot mass = α∗log10 (Root mass)+β] for various levels of N or P addition.
Discussion
N and P Availability Differently Altered Biomass Allocation Fractions
In this study, we found that N addition increased LMF but decreased FMF, whereas P addition showed the opposite effects on these two. Similarly, Wang et al. (2016) also found that N addition induced the decrease in reproductive allocation of Chloris virgata. Fujita et al. (2014) found that plants distributed in P-deficient communities showed low investment of resources in sexual reproductive tissues. One potential cause for a contrasting response may be that N and P have differential roles in physiological functions and metabolic processes in plants. Large proportion of acquired N is allocated into photosynthetic apparatus (Yasumura et al., 2007; Ghimire et al., 2017), and N could then more strongly constrain photosynthesis relative to reproduction (Fortunel et al., 2009). Accordingly, N addition could promote carbon assimilation capacity and leaf expansion (Lambers et al., 2008), resulting in an increase in the LMF. In contrast, plant invests up to c. 50–60% of acquired P in reproductive tissues, which is larger for P than for N (Güsewell, 2004; Kerkhoff et al., 2006). Accordingly, P deficiency tends to reduce the reproductive allocation (Güsewell, 2004; Fujita et al., 2014).
In addition, differences in the phenological response of plants to N and P availability could regulate biomass allocation pattern in plants (Bazzaz and Grace, 1997; McConnaughay and Coleman, 1999). The delay of flowering implies that plants postpone the switch from vegetative growth to reproductive growth, resulting in the decreasing allocation of biomass to reproductive organs (Zhang et al., 2014). Our results showed that low P availability delayed the flowering date in A. thaliana (Supplementary Figure S2), which may further decrease reproductive allocation. In contrast, we found that N availability exerted insignificant effect on flowering date of A. thaliana (Supplementary Figure S2). Several other studies found that the responses of plant flowering date and reproductive allocation fractions to N addition differed among various species (Tian et al., 2012; Zhang et al., 2014). Thus, observed pattern regarding the effects of N availability on reproductive allocation fraction of A. thaliana may be species-specific and should be cautioned when extending to other species.
Consistent with previous studies regarding the effects of nutrient availability on SMF (de Groot et al., 2002; Poorter et al., 2012), we found that both N and P additions increased SMF of A. thaliana. Generally, stem is regarded as an effectively luxury organ for plants grown in nutrient poor environments, and thus plants distributed in such conditions usually reduce the SMF (Yan B.G. et al., 2016). In contrast, plants grown in high nutrient supply often need to allocate more biomass into stem in order to provide mechanical supports for the enhanced aboveground biomass. Moreover, nutrient addition that induced crowded conditions in field plant populations could promote SMF for coping with light competition (Niu et al., 2008; Zhang et al., 2014; Yan B.G. et al., 2016). R:S ratio of A. thaliana decreased with increasing levels of N and P additions, which was in accordance with previous findings (Bazzaz and Grace, 1997; Müller et al., 2000; de Groot et al., 2002; Poorter et al., 2012).
Besides, we found another intriguing result that pattern of biomass allocation did not change after moderate additions of N and P (Figure 1). Effects of N and P additions on biomass allocation fractions were only significant when N and P availability limited the plant growth (Figure 1). Beyond the threshold of nutrient availability, further addition of N or P exited insignificant influence on biomass allocation fractions (Figure 1). Thus, effect of nutrient addition on biomass allocation pattern should depend on substrate nutrient limitation status.
Peng et al. (2019) has conducted a similar N and P fertilization experiment to explore the responses of stoichiometry and nutrient resorption efficiency of Amaranthus mangostanus. Using their biomass data, we further analyzed effects of N and P additions on biomass allocation of A. mangostanus. Results showed that N and P additions decreased R:S ratio, whereas N (or P) addition increased (or decreased) LMF (Supplementary Figures S1a,b). These results are in accordance with our study. However, response of SMF to N and P additions was very complex, and was adjusted by the interaction of N and P additions (Supplementary Figure S1c). In contrast with N addition, P addition exerted larger and positive influence on FMF (Supplementary Figure S1d), which is partly consistent with our findings. Overall, effects of N and P additions on biomass allocation to root, leaf and reproductive tissues of A. thaliana is mostly applicable to those of A. mangostanus. Further study is needed to test the generality of biomass allocation pattern across species with different life strategies.
N and P Availability Contrastingly Influenced Biomass Allometric Relationships
Shift in scaling exponents of biomass allometric relationships suggests an independent change in functional tradeoff among organs, which is very meaningful to understand how plant balance fixed biomass in limitation of different available resources (Weiner, 2004; Poorter et al., 2015). In this study, we found that the type of nutrient addition regulated scaling exponent of stem mass against leaf mass. That is, allometric relationship of stem mass against leaf mass was nearly isometric for pooled data of the five levels of N addition, whereas the relationship was allometric with scaling exponent of 1.226 (i.e., stem ∝ leaf1.226) for pooled data of the five levels of P addition. This difference in the scaling exponent might be attributed to the disproportionate increase in leaf mass under N addition relative to P addition. Both LMF and SMF increased with increasing level of N addition, while LMF decreased and SMF increased with increasing level of P addition. Thereby, plants under P additions relative to N additions should exhibit a lower investment in leaf mass per additional unit of stem mass accumulated. These findings indicated that there was plasticity in allometric relationship between stem mass and leaf mass for A. thaliana. In addition, previous important recognition of isometry between leaf and stem biomass for herbaceous species (Niklas and Cobb, 2006; Enquist et al., 2007) didn’t conform to the result under P additions. As A. thaliana is a rosette forb and primary function of its stem is to support reproductive tissues, allometric relationship between stem mass and leaf mass might thus be different from previous traditional viewpoint.
Relationship between reproductive and vegetative mass is a fundamental component of plants’ reproductive strategy (Reekie and Bazzaz, 2005). Multiple environmental factors might change allometric coefficients of the relationship (Weiner et al., 2009). In our study, we found that the type of nutrient addition significantly regulated scaling exponent of the relationship. Namely, scaling exponent of fruit mass (i.e., reproductive mass) against vegetative mass for pooled data of the five levels of N addition was significantly lower than that for pooled data of the five levels of P addition (0.875 vs. 1.028, p < 0.05). This difference might be attributed to a contrasting roles of N and P on the trade-off between reproductive and vegetative growth. N addition facilitated a higher investment of biomass in vegetative organ, whereas P addition favored a higher investment of biomass in reproductive organ (Lambers et al., 2008; Fujita et al., 2014; Kitayama et al., 2015). Thereby, plants under N addition relative to P addition should exhibit a lower investment in reproductive mass per additional unit of vegetative mass accumulated. These findings further indicated that there was plasticity in the allometric relationship between reproductive mass and vegetative mass for A. thaliana.
Compared with the type of nutrient addition, the levels of N or P addition exerted little impacts on scaling exponents and normalization constants of allometric relationships of stem mass vs. leaf mass and fruit mass vs. vegetative mass (Table 1 and Figure 5). Similarly, through conducting experimental manipulations with species from the Mediterranean old-field succession, Müller et al. (2000) found common allometric relationships of biomass among different plant structures under several levels of N addition. Fortunel et al. (2009) found that allometric relationships between reproductive and leaf biomass had no difference in either slope or normalization constant among different levels of N addition, suggesting the little plasticity of reproductive strategies. Peng and Yang (2016) synthesized data from global N fertilization experiments and found that the levels of N addition didn’t alter allometric relationship between aboveground and belowground biomass. However, we found that increasing levels of N addition increased scaling exponent of shoot mass to root mass, whereas increasing levels of P addition exerted the opposite influence on the scaling exponent (Table 2). Consistently, Luo et al. (2016) found that scaling exponent of shoot to root mass increased with N:P supply ratio. These results suggested that plants under higher N:P supply ratio exhibited a bias toward elevated aboveground allocation, because root growth is more severely suppressed than shoot growth under P limitation (Güsewell, 2005; Venterink and Güsewell, 2010).
Conclusion
This study ascertained contrasting effects of N and P availability on biomass allocation of A. thaliana. N addition increased leaf and stem allocation, but decreased root and fruit allocation. P addition increased stem and fruit allocation, but decreased root and leaf allocation. Pooled data of the five levels of N addition relative to P addition resulted in lower scaling exponents of stem mass against leaf mass, fruit mass against vegetative mass, and shoot mass against root mass. Further, the levels of N or P addition did not significantly affect allometric relationships of stem mass vs. leaf mass, and fruit mass vs. vegetative mass. In contrast, increasing levels of N addition increased scaling exponent of shoot to root mass, whereas increasing levels of P addition exerted opposite influence on scaling exponent. Our findings highlight that biomass allocation of A. thaliana is differentially influenced by N and P availability, which could foster the understanding about response and acclimation of biomass allocation strategies in plants to varying nutrient conditions. We also point out that the types and levels of nutrient availability should be considered, respectively, when exploring effects of nutrient availability on biomass allometric relationships. However, whether the observed pattern in A. thaliana can be extended to other species with different functional traits necessitates further studies.
Author Contributions
JF designed the research. ZY and JF performed the research and analyzed the data. All authors wrote the manuscript.
Funding
China Postdoctoral Science Foundation (Project Nos. 2017M620004 and 2018T110008), the National Key Research and Development Program of China (2017YFC0503900), and the National Natural Science Foundation of China (Project Nos. 31621091 and 31330012).
Conflict of Interest Statement
The authors declare that the research was conducted in the absence of any commercial or financial relationships that could be construed as a potential conflict of interest.
Acknowledgments
The authors thank N. Y. Kim, H. Y. Guan, W. J. Fang, Q. Cai, and H. Y. Huang for assistance with laboratory work.
Supplementary Material
The Supplementary Material for this article can be found online at: https://www.frontiersin.org/articles/10.3389/fpls.2019.00598/full#supplementary-material
References
Aerts, R., and Chapin, F. S. III (2000). The mineral nutrition of wild plants revisited: a re-evaluation of processes and patterns. Adv. Ecol. Res. 30, 1–67. doi: 10.1016/S0065-2504(08)60016-1
Ågren, G. I. (2008). Stoichiometry and nutrition of plant growth in natural communities. Annu. Rev. Ecol. Evol. Syst. 39, 153–170. doi: 10.1146/annurev.ecolsys.39.110707.173515
de Groot, C. C., Marcelis, L. F. M., van den Boogaard, R., and Lambers, H. (2002). Interactive effects of nitrogen and irradiance on growth and partitioning of dry mass and nitrogen in young tomato plants. Funct. Plant Biol. 29, 1319–1328. doi: 10.1071/FP02087
Du, E. Z., de Vries, W., Han, W. X., Liu, X. J., Yan, Z. B., and Jiang, Y. (2016). Imbalanced phosphorus and nitrogen deposition in China’s forests. Atmos. Chem. Phys. 16, 8571–8579. doi: 10.5194/acp-16-8571-2016
Elser, J. J., Bracken, M. E. S., Cleland, E. E., Gruner, D. S., Harpole, W. S., Hillebrand, H., et al. (2007). Global analysis of nitrogen and phosphorus limitation of primary producers in freshwater, marine and terrestrial ecosystems. Ecol. Lett. 10, 1135–1142. doi: 10.1111/j.1461-0248.2007.01113.x
Enquist, B. J., Allen, A. P., Brown, J. H., Gillooly, J. F., Kerkhoff, A. J., Niklas, K. J., et al. (2007). Biological scaling: does the exception prove the rule? Nature 445, E9–E10. doi: 10.1038/nature05548
Enquist, B. J., and Niklas, K. J. (2002). Global allocation rules for patterns of biomass partitioning in seed plants. Science 295, 1517–1520. doi: 10.1126/science.1066360
Eziz, A., Yan, Z. B., Tian, D., Han, W. X., Tang, Z. Y., and Fang, J. Y. (2017). Drought effect on plant biomass allocation: a meta-analysis. Ecol. Evol. 7, 11002–11010. doi: 10.1002/ece3.3630
Fortunel, C., Violle, C., Roumet, C., Buatois, B., Navas, M., and Garnier, E. (2009). Allocation strategies and seed traits are hardly affected by nitrogen supply in 18 species differing in successional status. Perspect. Plant Ecol. Evol. Syst. 11, 267–283. doi: 10.1016/j.ppees.2009.04.003
Fujita, Y., Venterink, H. O., van Bodegom, P. M., Douma, J. C., Heil, G. W., Hölzel, N., et al. (2014). Low investment in sexual reproduction threatens plants adapted to phosphorus limitation. Nature 505, 82–86. doi: 10.1038/nature12733
Galloway, J. N., Townsend, A. R., Erisman, J. W., Bekunda, M., Cai, Z. C., Freney, J. R., et al. (2008). Transformation of the nitrogen cycle: recent trends, questions, and potential solutions. Science 320, 889–892. doi: 10.1126/science.1136674
Ghimire, B., Riley, W. J., Koven, C. D., Kattge, J., Rogers, A., Reich, P. B., et al. (2017). A global trait-based approach to estimate leaf nitrogen functional allocation from observations. Ecol. Appl. 27, 1421–1434. doi: 10.1002/eap.1542
Güsewell, S. (2005). Responses of wetland graminoids to the relative supply of nitrogen and phosphorus. Plant Ecol. 176, 35–55. doi: 10.1007/s11258-004-0010-8
Güsewell, S. (2004). N:P ratios in terrestrial plants: variation and functional significance. New Phytol. 164, 243–266. doi: 10.1078/1433-8319-0000022
Hoagland, D. R., and Arnon, D. I. (1950). The Water-Culture Method for Growing Plants Without Soil. Berkeley, CA: University of California.
Huang, Y. X., Zhao, X. Y., Zhou, D. W., Zhao, H. L., Zhang, H. X., Zuo, X. A., et al. (2009). Allometry of Salsola collina in response to soil nutrients, water supply and population density. Nord. J. Bot. 27, 539–547. doi: 10.1111/j.1756-1051.2009.00382.x
Kerkhoff, A. J., Fagan, W. F., Elser, J. J., and Enquist, B. J. (2006). Phylogenetic and growth form variation in the scaling of nitrogen and phosphorus in the seed plants. Am. Nat. 168, E103–E122. doi: 10.1086/507879
Kitayama, K., Tsujii, Y., Aoyagi, R., and Aiba, S. (2015). Long-term C, N and P allocation to reproduction in Bornean tropical rain forests. J. Ecol. 103, 606–615. doi: 10.1111/1365-2745.12379
Lambers, H., Chapin, F. S. I. I. I., and Pons, T. L. (2008). Plant Physiological Ecology. New York, NY: Springer-Verlag.
Luo, X., Mazer, S. J., Guo, H., Zhang, N., Weiner, J., and Hu, S. J. (2016). Nitrogen : phosphorous supply ratio and allometry in five alpine plant species. Ecol. Evol. 6, 8881–8892. doi: 10.1002/ece3.2587
McCarthy, M. C., and Enquist, B. J. (2007). Consistency between an allometric approach and optimal partitioning theory in global patterns of plant biomass allocation. Func. Ecol. 21, 713–720. doi: 10.1111/j.1365-2435.2007.01276.x
McConnaughay, K. D. M., and Coleman, J. S. (1999). Biomass allocation in plants: ontogeny or optimality? A test along three resources gradients. Ecology 80, 2581–2593. doi: 10.2307/177242
Meinke, D. W., Cherry, J. M., Dean, C., Rounsley, S. D., and Koornneef, M. (1998). Arabidopsis thaliana: a model plant for genome analysis. Science 282, 662–682. doi: 10.1126/science.282.5389.662
Müller, I., Schmid, B., and Weiner, J. (2000). The effect of nutrient availability on biomass allocation patterns in 27 species of herbaceous plants. Perspect. Plant. Ecol. 3, 115–127. doi: 10.1078/1433-8319-00007
Niklas, K. J., and Cobb, E. D. (2006). Biomass partitioning and leaf N, P-stoichiometry: comparisons between tree and herbaceous current-year shoots. Plant Cell Environ. 29, 2030–2042. doi: 10.1111/j.1365-3040.2006.01578.x
Niu, K. C., Choler, P., Zhao, B. B., and Du, G. Z. (2009). The allometry of reproductive biomass in response to land use in Tibetan alpine grasslands. Funct. Ecol. 23, 274–283. doi: 10.1111/j.1365-2435.2008.01502.x
Niu, K. C., Luo, Y. J., Choler, P., and Du, G. Z. (2008). The role of biomass allocation strategy in diversity loss due to fertilization. Basic Appl. Ecol. 9, 485–493. doi: 10.1016/j.baae.2007.06.015
Peng, H. Y., Yan, Z. B., Chen, Y. H., Zhao, X. J., and Han, W. X. (2019). Effects of body size and root to shoot ratio on foliar nutrient resorption efficiency in Amaranthus mangostanus. Am. J. Bot. 106, 363–370. doi: 10.1002/ajb2.1246
Peng, Y. F., and Yang, Y. H. (2016). Allometric biomass partitioning under nitrogen enrichment: evidence from manipulative experiments around the world. Sci. Rep. 6:28918. doi: 10.1038/srep28918
Poorter, H., Jagodzinski, A. M., Ruiz-Peinado, R., Kuyah, S., Luo, Y., Oleksyn, J., et al. (2015). How does biomass distribution change with size and differ among species? An analysis for 1200 plant species from five continents. New Phytol. 208, 736–749. doi: 10.1111/nph.13571
Poorter, H., and Nagel, O. (2000). The role of biomass allocation in the growth response of plants to different levels of light, CO2, nutrients and water: a quantitative review. Aust. J. Plant Physiol. 27, 595–607. doi: 10.1071/PP99173_CO
Poorter, H., Niklas, K. J., Reich, P. B., Oleksyn, J., Poot, P., and Mommer, L. (2012). Biomass allocation to leaves, stems and roots: meta-analyses of interspecific variation and environmental control. New Phytol. 193, 30–50. doi: 10.1111/j.1469-8137.2011.03952.x
R Development Core Team (2012). R: A Languange and Environment for Statistical Computing. Vienna: R Foundation for Statistical Computing.
Reekie, E. G., and Bazzaz, F. A. (2005). Reproductive Allocation in Plants. Burlington, CA: Academic Press.
Shipley, B., and Meziane, D. (2002). The balanced growth hypothesis and the allometry of leaf and root biomass allocation. Funct. Ecol. 16, 326–331. doi: 10.1046/j.1365-2435.2002.00626.x
Sultan, S. E. (2001). Phenotypic plasticity for fitness components in Polygonum species of contrasting ecological breadth. Ecology 82, 328–343. doi: 10.2307/2679863
Tian, D. S., Pan, Q. M., Simmons, M., Chaolu, H. D., Du, B. H., Bai, Y. F., et al. (2012). Hierarchical reproductive allocation and allometry within a perennial bunchgrass after 11 years of nutrient addition. PLoS One 7:e42833. doi: 10.1371/journal.pone.0042833
Venterink, H. O., and Güsewell, S. (2010). Competitive interactions between two meadow grasses under nitrogen and phosphorus limitation. Funct. Ecol. 24, 877–886. doi: 10.1111/j.1365-2435.2010.01692.x
Wang, Y., Li, L., Zhou, D. W., and Weiner, J. (2016). The allometry of reproductive allocation in a Chloris virgata population in response to simulated atmospheric nitrogen deposition. Basic Appl. Ecol. 17, 388–395. doi: 10.1016/j.baae.2016.01.004
Warton, D. I., Wright, I. J., Falster, D. S., and Westoby, M. (2006). Bivariate line-fitting methods for allometry. Biol. Rev. Camb. Philos. Soc. 81, 259–291. doi: 10.1017/S1464793106007007
Weiner, J. (2004). Allocation, plasticity and allometry in plants. Perspect. Plant Ecol. 6, 207–215. doi: 10.1078/1433-8319-00083
Weiner, J., Campbell, L. G., Pino, J., and Echarte, L. (2009). The allometry of reproduction within plant populations. J. Ecol. 97, 1220–1233. doi: 10.1111/j.1365-2745.2009.01559.x
Yan, B. G., Ji, Z. H., Fan, B., Wang, X. M., He, G. X., Shi, L. T., et al. (2016). Plants adapted to nutrient limitation allocate less biomass into stems in an arid-hot grassland. New Phytol. 211, 1232–1240. doi: 10.1111/nph.13970
Yan, Z. B., Guan, H. Y., Han, W. X., Han, T. S., Guo, Y. L., and Fang, J. Y. (2016). Reproductive organ and young tissues show constrained elemental composition in Arabidopsis thaliana. Ann. Bot. 117, 431–439. doi: 10.1093/aob/mcv190
Yan, Z. B., Kim, N. Y., Han, W. X., Guo, Y. L., Han, T. S., Du, E. Z., et al. (2015). Effects of nitrogen and phosphorus supply on growth rate, leaf stoichiometry, and nutrient resorption of Arabidopsis thaliana. Plant Soil 388, 147–155. doi: 10.1007/s11104-014-2316-1
Yan, Z. B., Li, X. P., Tian, D., Han, W. X., Hou, H., Shen, H., et al. (2018). Nutrient addition affects scaling relationship of leaf nitrogen to phosphorus in Arabidopsis thaliana. Funct. Ecol. 32, 2689–2698. doi: 10.1007/s11104-014-2316-1
Yang, Y. H., Fang, J. Y., Ji, C. J., and Han, W. X. (2009). Above- and belowground biomass allocation in Tibetan grasslands. J. Veg. Sci. 20, 177–184. doi: 10.1111/j.1654-1103.2009.05566.x
Yasumura, Y., Hikosaka, K., and Hirose, T. (2007). Nitrogen resorption and protein degradation during leaf senescence in Chenopodium album grown in different light and nitrogen conditions. Funct. Plant Biol. 34, 409–417. doi: 10.1071/FP06307
Keywords: allometry, Arabidopsis thaliana, biomass allocation, fertilization experiment, N and P availability
Citation: Yan Z, Eziz A, Tian D, Li X, Hou X, Peng H, Han W, Guo Y and Fang J (2019) Biomass Allocation in Response to Nitrogen and Phosphorus Availability: Insight From Experimental Manipulations of Arabidopsis thaliana. Front. Plant Sci. 10:598. doi: 10.3389/fpls.2019.00598
Received: 13 October 2018; Accepted: 24 April 2019;
Published: 14 May 2019.
Edited by:
Sebastian Leuzinger, Auckland University of Technology, New ZealandReviewed by:
Rubén Retuerto, University of Santiago de Compostela, SpainNiu Kechang, Nanjing University, China
Hui Guo, Nanjing Agricultural University, China
Copyright © 2019 Yan, Eziz, Tian, Li, Hou, Peng, Han, Guo and Fang. This is an open-access article distributed under the terms of the Creative Commons Attribution License (CC BY). The use, distribution or reproduction in other forums is permitted, provided the original author(s) and the copyright owner(s) are credited and that the original publication in this journal is cited, in accordance with accepted academic practice. No use, distribution or reproduction is permitted which does not comply with these terms.
*Correspondence: Jingyun Fang, anlmYW5nQHVyYmFuLnBrdS5lZHUuY24=