- Department of Applied Biology, College of Agriculture and Life Sciences, Chonnam National University, Gwangju, South Korea
Recent advances in methylated RNA immunoprecipitation followed by sequencing and mass spectrometry have revealed widespread chemical modifications on mRNAs. Methylation of RNA bases such as N6-methyladenosine (m6A) and 5-methylcytidine (m5C) is the most prevalent mRNA modifications found in eukaryotes. In recent years, cellular factors introducing, interpreting, and deleting specific methylation marks on mRNAs, designated as “writers (methyltransferase),” “readers (RNA-binding protein),” and “erasers (demethylase),” respectively, have been identified in plants and animals. An emerging body of evidence shows that methylation on mRNAs affects diverse aspects of RNA metabolism, including stability, splicing, nucleus-to-cytoplasm export, alternative polyadenylation, and translation. Although our understanding for roles of writers, readers, and erasers in plants is far behind that for their animal counterparts, accumulating reports clearly demonstrate that these factors are essential for plant growth and abiotic stress responses. This review emphasizes the crucial roles of epitranscriptomic modifications of RNAs in new layer of gene expression regulation during the growth and response of plants to abiotic stresses.
Introduction
Epigenetic regulation of gene expression via DNA methylation and histone modifications is an important strategy for living organisms to achieve fine-tuned regulation of developmental processes or responses to environmental cues. Similar to DNA methylation in epigenetic regulation, posttranscriptional RNA modifications are emerging as important “epitranscriptomic” regulatory networks in recent years (Saletore et al., 2012; Meyer and Jaffrey, 2014). Over 150 different chemical modifications on mRNAs, tRNAs, and rRNAs are currently known for all kingdoms of life (Cantara et al., 2010; Boccaletto et al., 2018). Among diverse modifications found on mRNAs, N6-methyladenosine (m6A) is the most prevalent modification in both plants and animals (Liu and Pan, 2016; Covelo-Molares et al., 2018). Recent advances in methylation RNA sequencing (Met RNA-seq) and deep RNA sequencing have revealed transcriptome-wide m6A methylation patterns in plants as well as in animals (Luo et al., 2014; Wang et al., 2015a; Cui et al., 2017). These modifications within mRNAs can affect multiple steps of transcript’s fate, including splicing (Haussmann et al., 2016; Xiao et al., 2016), nucleus-to-cytoplasm export (Zheng et al., 2013), RNA turnover (Du et al., 2016; Mauer et al., 2017; Wei et al., 2018), and translation (Meyer et al., 2015; Wang et al., 2015b; Choi et al., 2016).
The level and status of RNA methylation in cells depend on two crucial proteins: RNA methyltransferase (MT) designated as “writer” and RNA demethylase (DMT) designated as “eraser” (Figure 1). In addition to these two essential proteins required for the addition and removal of methyl groups on RNAs, a third protein designated as “reader” is involved in the recognition and processing of methylated RNAs (reviewed in Meyer and Jaffrey, 2014). In animals, genes encoding m6A writer (Ping et al., 2014; Schwartz et al., 2014), reader (Luo and Tong, 2014; Xu et al., 2014), and eraser (Jia et al., 2011; Zheng et al., 2013) proteins have been identified and characterized (Table 1). Notably, mutants lacking specific m6A writer, reader, or eraser have displayed abnormal development and altered response to hypoxia and high temperatures, suggesting crucial roles of RNA methylation in animal development and adaptation to changing environmental cues (reviewed in Meyer and Jaffrey, 2014; Yue et al., 2015; Hsu et al., 2017).
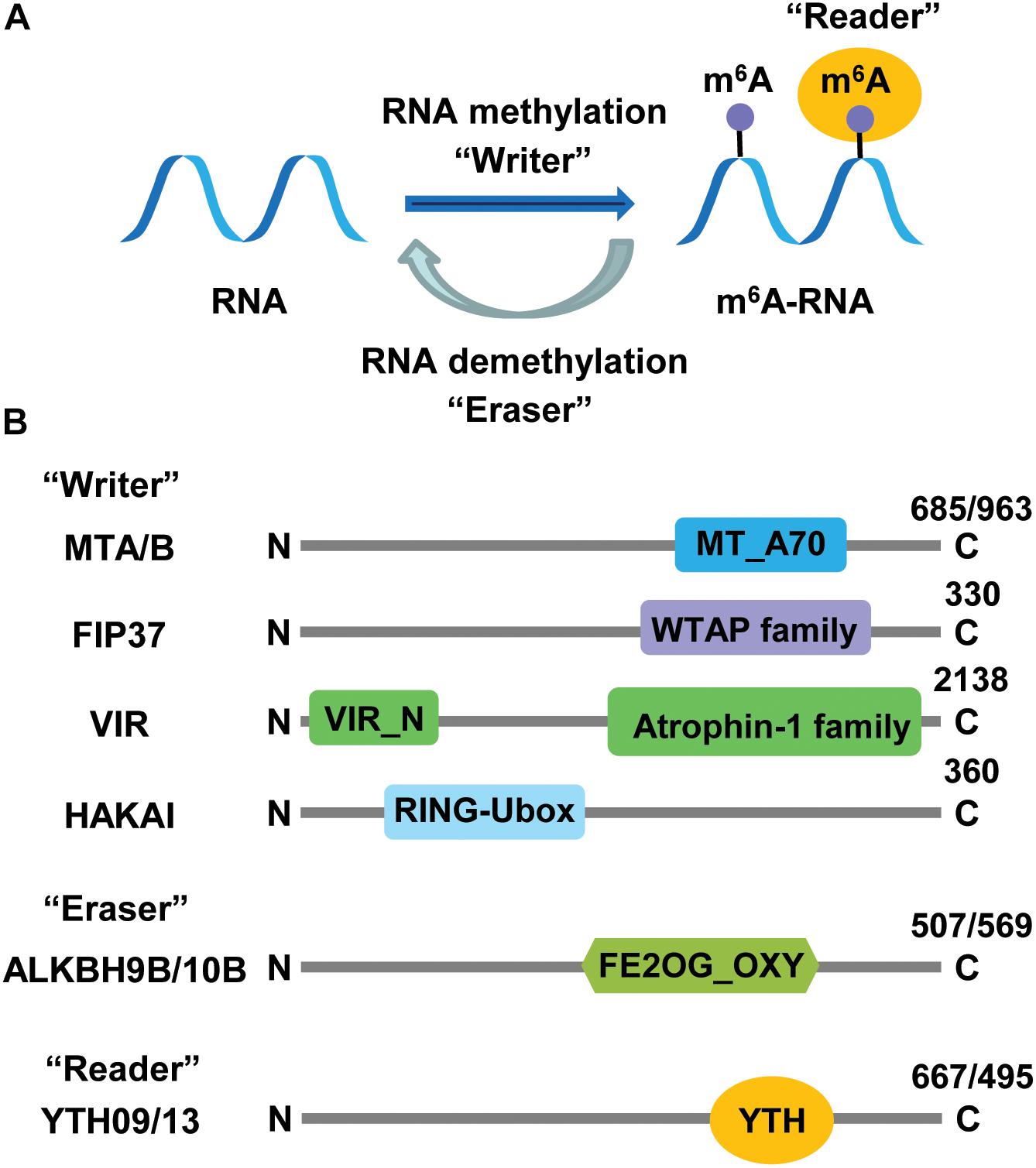
Figure 1. Roles and structural characteristics of m6A RNA methylation-related proteins. (A) Cellular factors introducing, deleting, and interpreting m6A marks are methyltransferase (“writers”), demethylase (“eraser”), and RNA-binding protein (“reader”), respectively. (B) The writer complex consists of five components: MTA/B (methyltransferase A/B), FIP37 (FKBP12 interacting protein 37), VIR (Virilizer), HAKAI (for “destruction” in Japanese, a c-Cb1-like protein), erasers belong to AlkB-homology (ALKBH) family proteins, and readers are YT512-B homology domain (YTHD) proteins. Numbers at the C terminus indicate the number of amino acid residues in each Arabidopsis protein. MT_A70, methytransferase_A70; FE2OG_OXY, Fe2+ 2-oxoglutarate dioxygenase domain; WTAP, WT1-associated protein.
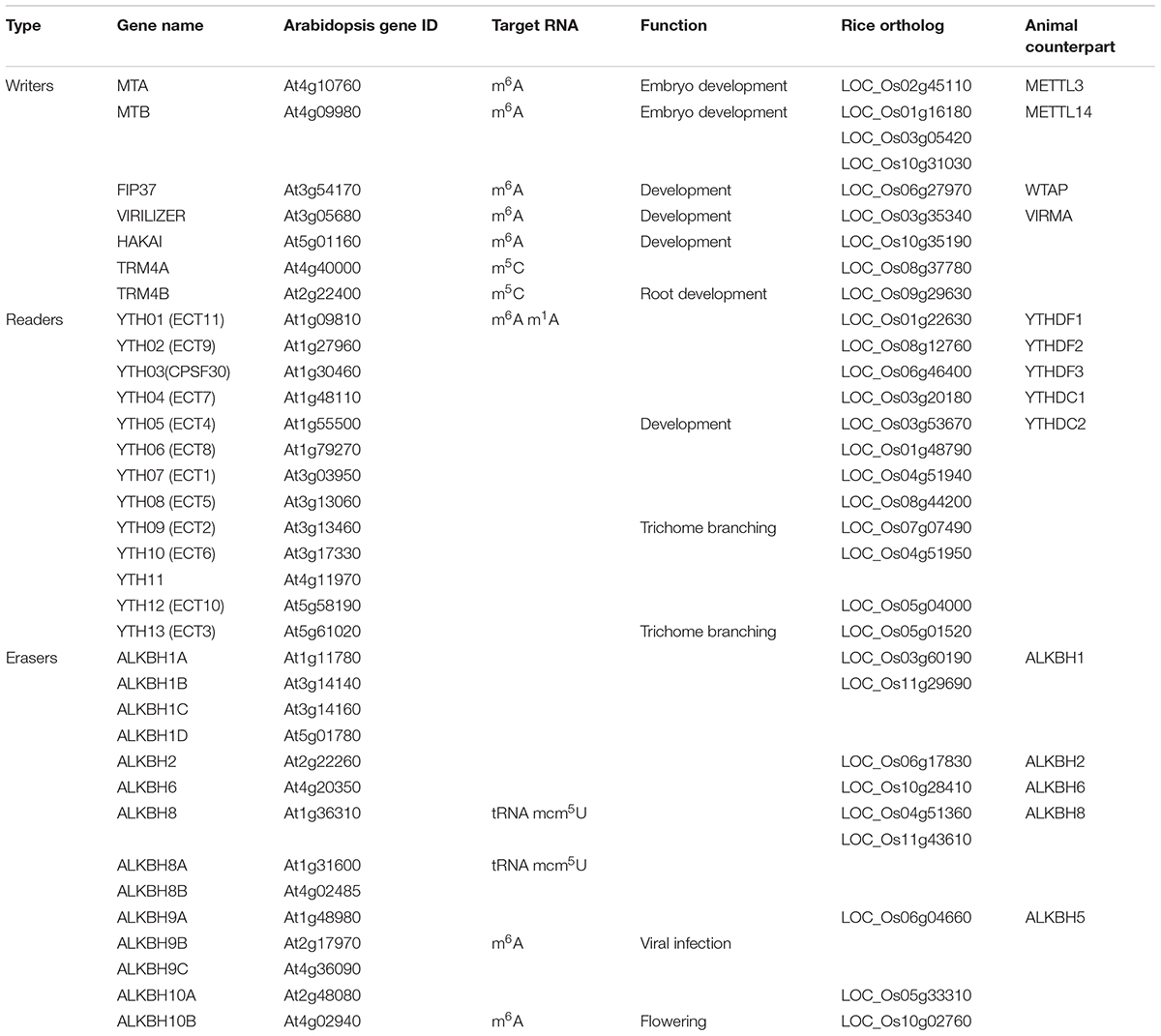
Table 1. List of writers, readers, and erasers involved in RNA methylation in Arabidopsis thaliana and rice (Oryza sativa).
Although these recent studies clearly point to the importance of RNA methylation and essential roles of writers, readers, and erasers in the development of animals, functions of these proteins in plants are just beginning to be uncovered. Arabidopsis contains functional orthologs of m6A writer complex components, erasers, and reader proteins, some of which have been found to play essential roles in normal plant development (Bodi et al., 2012; Shen et al., 2016; Růžička et al., 2017; Arribas-Hernández et al., 2018; Scutenaire et al., 2018; Wei et al., 2018). All these aforementioned studies have emphasized the essential roles of RNA methylation in plant development. However, the identity and functions of most writers, readers, and erasers in plants are currently unclear. In this review, we systematically identified potential m6A writers, readers, and erasers in Arabidopsis and rice (Oryza sativa) by comparing sequence homology to animal counterparts. We also reviewed multiple functions and potential significance of m6A RNA methylation in the development and response of plants to diverse abiotic stresses.
Diverse Modifications for Eukaryotic RNAs
Over 150 different internal modifications on RNAs have been identified (Cantara et al., 2010; Boccaletto et al., 2018), with different degree, topology, and kinds of modifications between mRNAs, tRNAs, and rRNAs. For instance, approximately 17% of total nucleotides in tRNAs are modified, whereas only 2% of nucleotides in rRNAs are modified (Jackman and Alfonzo, 2013). Among diverse modifications identified for tRNAs and rRNAs, 2′-O-ribose methylation and pseudouridilation of rRNAs and 5-methylcytosine (m5C) and 1-methylguanidine (m1G) of tRNAs are the most abundant (Chou et al., 2017). Despite emerging roles of mRNA modifications in its processing and function, mRNA is less densely modified compared to tRNAs and rRNAs (Gilbert et al., 2016). Only a handful of different methylations have been identified so far in mRNAs, with N6-methyladenosine (m6A) being the most abundant (Liu and Pan, 2016; Covelo-Molares et al., 2018). These methylations of bases can influence the structure of RNAs by increasing its hydrophobicity and disrupting the canonical Watson-Crick base pairing (Oerum et al., 2017; Väre et al., 2017).
Importantly, all organisms have evolved to cluster methylation marks in functionally critical positions rather than randomly distributing them along RNA molecules. Most of these modified bases in rRNAs are located at the interface between ribosomal large and small subunits corresponding to P-site and A-site (Sharma and Lafontaine, 2015; Sloan et al., 2017). Wobble positions 34 and 37 of the anticodon loop in tRNAs are the most frequently and diversely modified (Väre et al., 2017). These conserved modification patterns reflect the essential role of RNA methylation in ribosome structure and biogenesis, codon recognition and decoding, and translation initiation or elongation (Jackman and Alfonzo, 2013; Chou et al., 2017; Sloan et al., 2017; Väre et al., 2017). Similar to rRNAs and tRNAs, mRNAs are also methylated in specific regions. For instance, m6A maps preferentially to the transcription start site, the stop codon, and the 3′ UTR (Dominissini et al., 2012; Luo et al., 2014; Meyer and Jaffrey, 2014), while m5C is predominantly found in 3′ UTR and coding regions (Squires et al., 2012; David et al., 2017). Several studies have shown that m1A methylation is frequently found in the start codon and the first splicing site which influences translation (Dominissini et al., 2016; Safra et al., 2017). Clearly, the degree, topology, and non-random distribution of RNA modifications are crucial for its specific cellular functions.
Writers, Erasers, and Readers Involved in m6A RNA Methylation and Recognition
Writers
Genes encoding m6A writer complexes have been identified and characterized firstly in animals. Several proteins including methyltransferase-like 3 (METTL3) and METTL14, Wilms’ tumor 1-associating protein (WTAP), and Vir like m6A methyltransferase-associated (VIRMA; KIAA1429) are known to form multicomponent m6A writer complexes in animals (Shah and Clancy, 1992; Ping et al., 2014; Schwartz et al., 2014; Table 1).
Methyltransferase-like 3 is the principal enzyme exerting methyltransferase activity, while METTL14 has a supporting role forming a METTL3-METTL14 heterodimer (Sledz and Jinek, 2016; Wang et al., 2016). After the identification of METTL3 in mammals as a homolog of yeast methyltransferase IME4 (Shah and Clancy, 1992), its orthologs were identified in different species including Arabidopsis and Drosophila. At present, Arabidopsis orthologs of animal m6A writer components have been identified, including MTA (ortholog of METTL3) and MTB (ortholog of METTL14).
Wilms’ tumor 1-associating protein functions as a stabilizer for the heterodimer localized in nuclear speckle (Ping et al., 2014; Lence et al., 2016). VIRMA plays a role in guiding the methyltransferase complex to the selective target region of mRNAs (Niessen et al., 2001; Yue et al., 2018). Arabidopsis VIR and FIP37 were identified as a ortholog of VIRMA and WTAP, respectively (Zhong et al., 2008; Bodi et al., 2012; Shen et al., 2016; Růžička et al., 2017).
Recently, zinc finger CCCH domain-containing protein 13 (ZC3H13), the latest component of methyltransferase complex, was found to be essential for localization of methyltransferase complex in mammals and Drosophila (Guo et al., 2018; Knuckles et al., 2018). However, the existence and molecular function of ZC3H13 in plants remain unknown. Interestingly, Arabidopsis contains E3 ubiquitin ligase HAKAI as an additional m6A writer component (Růžička et al., 2017; Table 1). Although knockdown of its expression can decrease m6A level (Růžička et al., 2017), the primary role of HAKAI in methyltransferase complexes has yet to be investigated.
Erasers
Removal of methylation marks on RNAs is carried out by α-ketoglutarate-dependent dioxygenase (AlkB) homolog (ALKBH) proteins that can erase alkyl and methyl groups from DNAs, RNAs, and proteins (Fedeles et al., 2015; Alemu et al., 2016). Mammals have nine ALKBH family members: ALKBH1 to ALKBH8 and fat mass- and obesity-associated protein (FTO) (Ougland et al., 2015; Table 1). Although ALKBH2 and ALKBH3 have been identified as main DNA repair enzymes, ALKBH3 also shows activity on m1A and m3C of RNAs (Ueda et al., 2017). Interestingly, ALKBH1 acts on a wide range of substrates in DNAs, RNAs, and histones (Westbye et al., 2008; Ougland et al., 2012; Wu et al., 2016). In addition to its role in cytoplasm, human ALKBH1 targets several m1A methylated tRNAs in mitochondria, influencing the organellar translation and function (Kawarada et al., 2017; Müller et al., 2018). ALKBH8, another tRNA DMT, interestingly contains both methyltransferase and demethylase domains, unlike other family members (Pastore et al., 2012).
Only two m6A erasers, ALKBH5 and FTO, have been identified in animals so far. Both enzymes were originally shown to be involved in demethylation of m6A (Jia et al., 2011; Zheng et al., 2013). However, recent studies have suggested that FTO has a much higher activity toward N6, 2′-O-dimethyladenosine (m6Am) compared to that for m6A (Meyer and Jaffrey, 2017; Mauer et al., 2017; Mauer and Jaffrey, 2018). ALKBH5 and FTO have been found to be involved in alternative splicing, 3′-UTR processing, mRNA stability, translation, and amino-acids deprivation response pathway (Zheng et al., 2013; Zhao et al., 2014; Bartosovic et al., 2017; Tang et al., 2018). Arabidopsis contains several putative m6A eraser ALKBH family proteins (Table 1), among which only two eraser proteins ALKBH9B and ALKBH10B have been functionally characterized in viral infection and floral transition (Duan et al., 2017; Martínez-Pérez et al., 2017). In summary, although increasing number of erasers targeting specific methylation marks have been identified, the activity and substrate RNAs of most ALKBH family members in plants and animals are yet to be determined.
Readers
Interpretation of methylation marks is tightly related to posttranscriptional regulation of mRNA metabolism which requires reader proteins to recognize methylated transcripts and ultimately determine their fates. In recent years, several RNA-binding proteins (RBPs) that can recognize m6A marks on mRNAs have been identified in animals by RNA-protein immunoprecipitation using synthetic m6A-containing RNAs (Dominissini et al., 2012; Xu et al., 2014; Arguello et al., 2017; Edupuganti et al., 2017; Wu et al., 2017). YT521-B homology (YTH) domain family (YTHDF) protein was first identified as an m6A-binding protein (Xu et al., 2014). Recently, human and mouse YTHDF proteins including YTHDF1, YTHDF2, YTHDF3, YTHDC1, and YTHDC2 were found to possess a specific binding pocket for m6A nucleotides and exhibit significantly high affinity to methylated RNAs, suggesting their role as m6A readers (Dominissini et al., 2012; Hsu et al., 2017; Xiang et al., 2017; Liao et al., 2018). YHHDF2 can bind to m6A-modified RNAs and play a distinct role in mRNA degradation by recruiting the CCR4-NOT deadenylase complex (Wang et al., 2014; Zhou et al., 2015; Du et al., 2016). YTHDF1 was found to recognize the 5′UTR of m6A-modified mRNAs in the cytosol, which promotes translation of target transcripts in a cap-independent manner (Wang et al., 2015b; Shi et al., 2017). YTHDC1 is involved in exon-selective gene splicing in the nucleus (Xiang et al., 2017). Interestingly, YTHDC2 also contains RNA helicase domain (Jain et al., 2018). Arabidopsis and rice genomes encode 13 and 12 YTHD proteins, respectively (Li et al., 2014a; Table 1). Contrary to extensive study on YTHD proteins in animals, only three evolutionarily conserved c-terminal region (ECT) family proteins have recently been functionally characterized in Arabidopsis as YTHD homologs (Arribas-Hernández et al., 2018; Scutenaire et al., 2018; Wei et al., 2018; Table 1).
Besides YTHD proteins, two other proteins containing different RNA-binding domains that can recognize m6A marks in animal cells have been reported. One is a heterogeneous nuclear ribonucleoprotein A2/B1 (HNRNPA2B1) which regulate RNA splicing in the nucleus through a well-characterized RNA-recognition motif (Alarcon et al., 2015). Notably, instead of directly binding to m6A site as YTHD proteins, HNRNPA2B1 might bind to altered structures right after the m6A site (Alarcon et al., 2015). Insulin-like growth factor 2 mRNA-binding protein (IGF2BP) contains tandem K-homology (KH) domains to recognize m6A sites and enhance target mRNA stability, storage, and translation in an m6A-dependent manner (Nicastro et al., 2015; Huang et al., 2018). Eukaryotic initiation factor 3 (eIF3) can also promote translation of mRNAs depending on m6A modification (Meyer et al., 2015). Clearly, more reader proteins recognizing other RNA modifications as well as m6A marks should be uncovered to fully understand cellular roles of epitranscriptomic RNA modifications in both plants and animals.
RNA Methylation in Animal Development and Diseases
m6A methylation has been demonstrated to affect all fates of mRNA metabolism, including pre-mRNA processing and intron splicing in the nucleus, nucleus-to cytoplasm RNA export, translation, and RNA decay in the cytoplasm (Figure 2). Analysis of different mettl mutants demonstrated the essential role of m6A methylation in cell development, proliferation, differentiation, and motility by regulating mRNA stability and splicing pattern of diverse transcripts (Wang et al., 2014; Chen et al., 2015; Geula et al., 2015; Park and Hong, 2017; Widagdo and Anggono, 2018). Loss of FTO can inhibit differentiation of primary myoblasts and skeletal muscle in mice, suggesting that m6A demethylase FTO plays a crucial role in somatic and neural stem cell differentiation (Wang et al., 2017). A larger number of gene encoding clock genes and clock output genes are enriched in m6A methylation (Fustin et al., 2013; Hastings, 2013) and changes in m6A levels can affect circadian rhythms, cellular growth, and survival (Fustin et al., 2018).
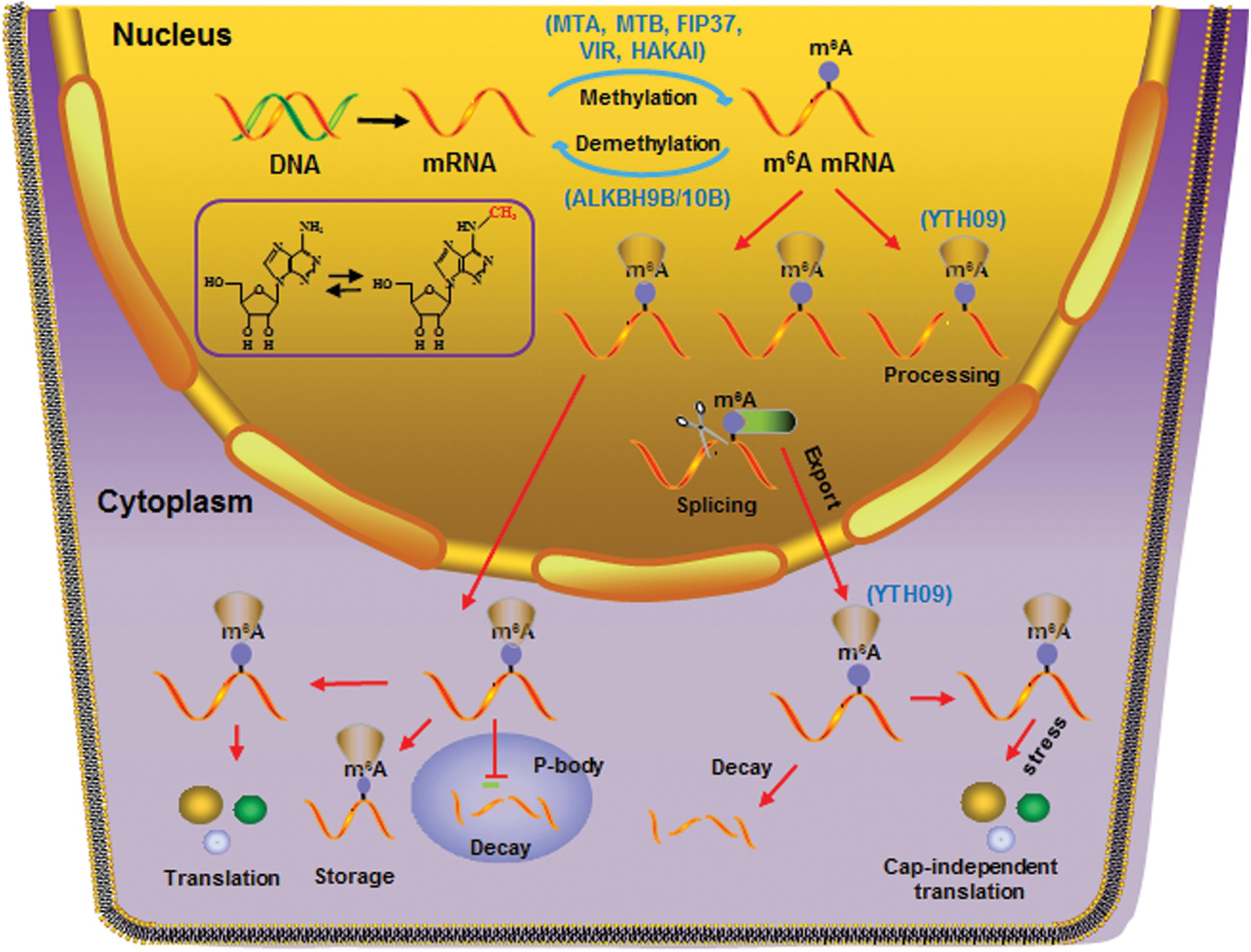
Figure 2. Diverse cellular processes affected by m6A RNA methylation. Splicing of mRNAs in the nucleus and diverse RNA metabolism in cytoplasm, including cap-dependent and cap-independent translation, RNA decay in cytosol and P-body, and RNA storage, is affected by m6A RNA methylation. Specific “reader” proteins recognizing m6A marks on mRNAs play essential roles in these cellular processes. Writers (MTA, MTB, FIP37, VIR, and HAKAI), erasers (ALKBH9B/10B), and reader (YTH09) identified in Arabidopsis are shown.
Notably, recent studies have demonstrated that alteration in m6A levels is closely associated with various diseases, especially cancer (reviewed in Dai et al., 2018; Pan et al., 2018). For example, FTO affects m6A level and translation of Angptl4 mRNA, which regulates fatty acid mobilization in adipocytes and body weight (Wang et al., 2015a). Low m6A level in total RNA is related to type 2 diabetes mellitus (Shen et al., 2014). Considering that aberrant cell growth and differentiation cause cancer, it is worth noting that cancer cells may improve their survival rate and progression by modulating aberrant methylation of target RNAs. Several studies have shown that expression of FTO or ALKBH5 can decrease m6A level, resulting in enhanced cancer cell growth (Zhang et al., 2016; Li et al., 2017; Zhang et al., 2017). METTL3 acts as an oncogene in cancer cells, enhancing the translation of cancer-inducing genes by interacting with translation initiation factor (Lin et al., 2016).
In addition to m6A methylation, m5C is also involved in cell development and diseases. This modification is deeply associated with testis differentiation and tumor cell proliferation. A previous study has shown that NOP2/sun RNA methyltransferase family member 2 (NSUN2), an m5C writer, is highly expressed in tumor cells and its depletion decreases levels of Ddx4, Miwi, and Tudor domain-containing proteins, suggesting an essential role of m5C RNA methylation in male germ cell differentiation (Frye and Watt, 2006). Moreover, loss of NSUN2 causes an accumulation of progenitors, decreases in upper-layer neurons, and increases in tRNA fragment accumulation in the brain, resulting in damage to neural stem cell differentiation and motility (Flores et al., 2017). Although these studies clearly demonstrate the importance of m6A and m5C in cell proliferation and diseases, biological functions of other RNA methylations in animal development and pathogenesis are yet to be elucidated.
RNA Methylation in Plant Development and Abiotic Stress Responses
Although our understanding of writers, readers, and erasers in plants is far behind their animal counterparts, accumulating reports clearly demonstrate that these factors are essential for plant growth and abiotic stress responses. Herein, we will summarize and discuss characterized and potential writers, readers, and erasers (Table 1) in plants.
m6A Writers
Genome-wide m6A methylation patterns have been mapped in barley, Arabidopsis, and rice (Li et al., 2014b; Luo et al., 2014). However, key enzymes responsible for this methylation have only been studied in Arabidopsis. Analysis of mta (Arabidopsis ortholog of human METTL3) knockdown mutants has revealed that MTA is required for m6A mRNA methylation and essential for normal growth and development, such as shoot and root growth as well as leaf and floral development (Zhong et al., 2008; Bodi et al., 2012). Moreover, MTA was found to interact with MTB, an Arabidopsis ortholog of human METTLl4. Knockdown of MTB showed a similar but less severe phenotype compared to mta mutants, indicating that both writers are essential for plant development (Růžička et al., 2017). The Arabidopsis m6A writer complex also includes an ortholog of human WTAP named FIP37. Depletion of FIP37 results in embryo lethality while its partial loss causes huge overproliferation of shoot meristems by increasing the stability of shootmeristemless (STM) and WUSCHEL (WUS) (Shen et al., 2016). Vir and Hakai are other m6A writer components in Arabidopsis. They affects root and shoot growth as well as cotyledon development, similar to other m6A writer mutant phenotypes (Růžička et al., 2017). However, the molecular mechanism underlying Vir and Hakai functions is yet to be elucidated.
Despite increasing understanding of the roles of m6A writers in plant growth and development, reports demonstrating their involvement and functions in plant response to abiotic stresses are lacking. Our analysis of publically available microarray data using GENEVESTIGATOR revealed that expressions levels of writers in Arabidopsis and rice are differently modulated by diverse abiotic stresses (Figure 3). In Arabidopsis, levels of most m6A writer components were not significantly modulated by abiotic stresses. Levels of MTA and FIP37 were only marginally increased by cold and heat stress, respectively. In rice, the level of OsFIP was increased by cold stress whereas levels of OsMTA, OsMTB, and OsVIR were decrease by cold, drought, or salt stress. The constant expression of m6A writer components under normal and stress conditions suggests the fundamental role of m6A methylation in plant development and stress responses.
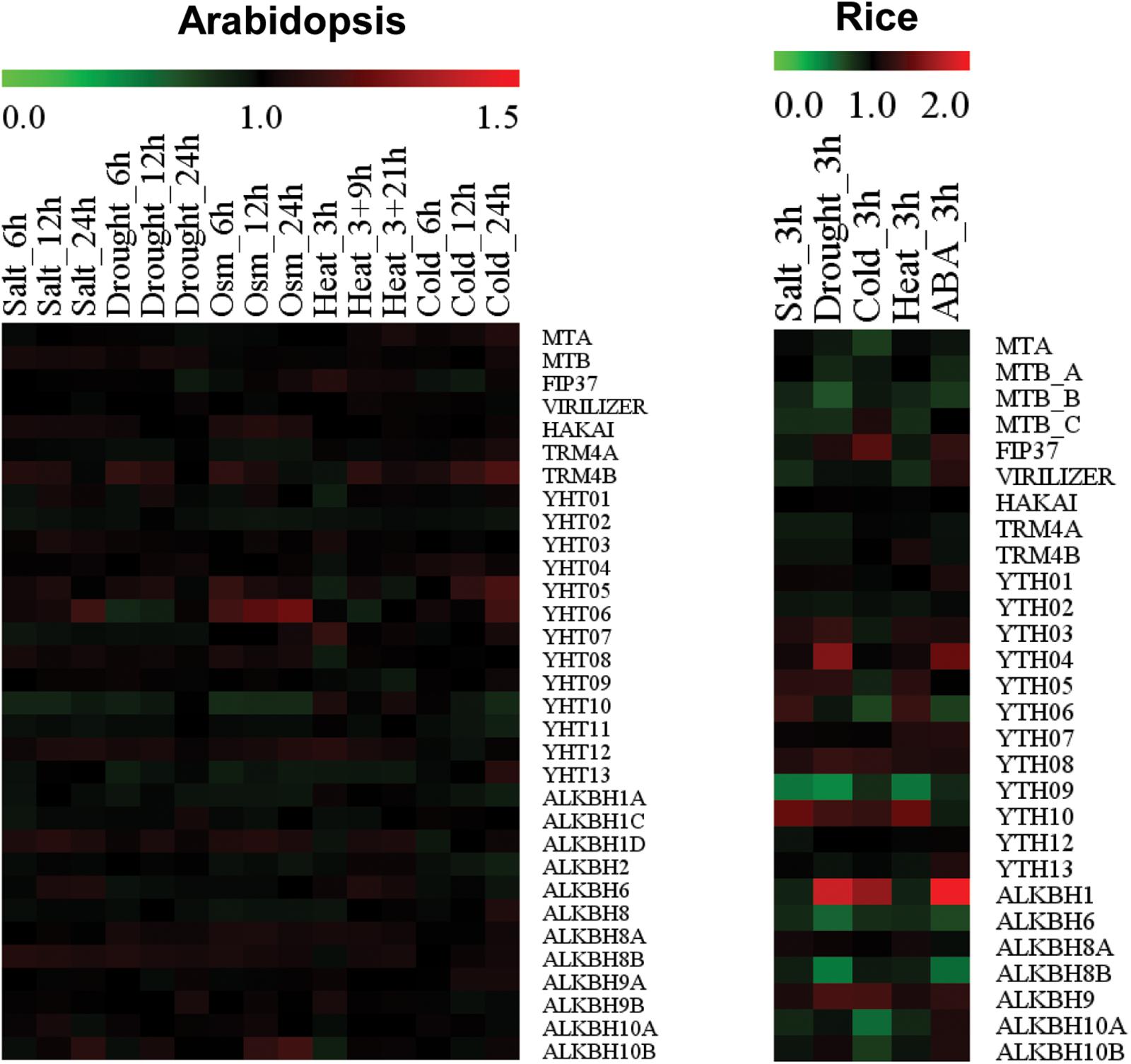
Figure 3. Heatmap showing stress-responsive expression patterns of writers, readers, and erasers in Arabidopsis and rice. Red or green colors represent upregulated and downregulated expression level, respectively. Microarray data were obtained from GENEVESTIGATOR, and expression levels of each gene under stress conditions were calculated relative to control levels.
m5C Writers
Although m5C methylation in DNA has been studied for many years, its cellular and molecular functions in RNAs is just beginning to be uncovered. Due to advancement in RNA sequencing, m5C RNA methylation could be mapped to mRNAs in both animals and plants (Schaefer et al., 2009; Hussain et al., 2013; Song et al., 2018). Overall, m5C RNA methylation is a less abundant modification of mRNA than m6A methylation. In Arabidopsis, two enzymes, TRM4A and TRM4B, are responsible for m5C RNA methylation. Both enzymes are orthologs of human m5C methyltransferase NSuns2. However, TRM4A contributes to tRNA m5C methylation while TRM4B targets mRNA for m5C methylation. Loss of TRM4A does not exhibit any visible phenotype while loss of Trm4B reduces root length, suggesting the role of mRNA m5C methylation in root development regulation (David et al., 2017). In accordance to this, loss of m5C RNA methylation affects the stability of short hypocotyl 2 (SHY2) and indoleacetic acid-induced protein 16 (IAA16), two critical genes related to root development (Cui et al., 2017). Our analysis showed that expression levels of Arabidopsis TRM4B are marginally increased by cold stress, although they decrease under heat stress. In contrast, expression levels of rice TRM4A and TRM4B are not altered in response to abiotic stresses (Figure 3). Although these expression patterns suggest potential roles of m5C writers in abiotic stress response, the relevance of m5C methylation to abiotic stress responses awaits further investigation.
m6A Erasers
Among protein factors involved in RNA methylation in plants, erasers are so far the least studied, although new knowledge is gained rapidly. Thirteen Arabidopsis ALKBH family members have been identified by bioinformatic analysis (Mielecki et al., 2012). However, only a few of them have been studied so far (Table 1). Among them, ALKBH9A, 9B, 9C, 10A, and 10B show the highest amino acid sequence similarity with human ALKBH5. Other family members are numbered based on their sequence similarity to human orthologs (Table 1). Like animal counterparts, most erasers are localized in the nucleus and cytoplasm whereas ALKBH1D is also present in chloroplasts. Interestingly, some of them show relocalization to the nucleus in response to methylating agents (Mielecki et al., 2012). ALKBH10B was identified as the principal mRNA m6A eraser influencing floral transition by controlling transcript levels of SPL3, SPL9, and FLOWERING LOCUS T (Duan et al., 2017). Another demethylase, ALKBH9B, was shown to revert m6A from single-stranded RNA in vitro (Martínez-Pérez et al., 2017). Although alkbh9b knockout mutants do not show differences in plant RNA m6A methylation level (Duan et al., 2017), its depletion results in hypermethylation of alfalfa mosaic virus (AMV) RNA, mediating systemic infection by interacting with viral cap proteins (Martínez-Pérez et al., 2017).
Expression of ALKBH9A is highly induced in roots under salt stress but not in response to ABA (Ma et al., 2006). Its level is much lower than ALKBH9 and ALKBH10 under normal conditions (Duan et al., 2017). ALKBH10A is down-regulated by heat stress (Merret et al., 2015) whereas ALKBH10B is up-regulated in response to karrikins (Nelson et al., 2010). Although these previous studies suggest a specific role of ALKBHs in stress responses as well as plant development, nothing is known about their actual roles. Our analysis showed that expression levels of ALKBH members were marginally up- or down-regulated in Arabidopsis by different abiotic stresses (Figure 3). Notably, levels of ALKBH1 in rice were highly increased upon drought, cold, or ABA treatment whereas expression levels of ALKBH6, ALKBH8B, and ALKBH10A were decreased by drought, ABA, or cold (Figure 3). These data suggest that ALKBHs could play important roles in abiotic stress responses, although this awaits further investigation.
m6A Readers
Although several RBPs interpreting m6A marks have been identified in animals, roles of only three YTHD m6A reader proteins have very recently been determined in Arabidopsis (Arribas-Hernández et al., 2018; Scutenaire et al., 2018; Wei et al., 2018). YTHD09 (ECT2) is involved in trichome development. Moreover, cytoplasmic-localized YTHD09 relocates to stress granules upon heat exposure, suggesting its role in mRNA fate control under stress conditions (Scutenaire et al., 2018). By using single and double mutants, it has been demonstrated that YTHD09 (ECT2), YTHD13 (ECT3), and ECT4 regulate the timing and execution of plant organogenesis (Arribas-Hernández et al., 2018). Moreover, a molecular study revealed that ECT2 targets a large number of m6A-containing transcripts, including TTG1, ITB1, and DIS2, which are involved in trichome development (Wei et al., 2018). Further sequencing analysis suggested that ECT2 increases the stability of these transcripts and influences trichome development (Wei et al., 2018). Although these studies clearly point to important roles of YTHD readers in plant development, more in-depth and focused efforts are needed to identify and characterize potential reader proteins (Table 1) that can recognize not only m6A modification, but also other methylation marks in plants.
No reports demonstrating the involvement or functions of any RNA methylation reader proteins in plant response to abiotic stresses have been published so far. However, a previous study and our current analysis showed that the expression of YTHDs in Arabidopsis and rice is highly regulated by different abiotic stresses (Li et al., 2014a; Figure 3). In Arabidopsis, levels of YTHD05, YTHD06, and YTHD07 are increased by heat, cold, hypoxia, or submergence stress. In contrast, the expression level of YTHD10 decreases under cold, drought, salt, or osmotic stress whereas YTHD08 level is reduced by heat stress. In rice, YTHDs responded differently to various abiotic stresses (Li et al., 2014a; Figure 3). Expression levels of YTHD05, YTHD06, YTHD07, and YTHD09 are downregulated by cold stress whereas levels of YTHD03 and YTHD08 increase under submergence and heat stress, respectively. Notably, none of these rice YTHDs showed altered expression under salt stress whereas YTHD01, YTHD02, YTHD03, YTHD04, or YTHD08 does not respond to cold stress. The fact that m6A reader proteins respond more to abiotic stresses than writers and erasers suggests that decoding of methylation marks is much more important than introducing or removing these marks during stress adaptation process in plants. It would be interesting to characterize roles of reader proteins in RNA metabolism and its consequence in stress responses.
Concluding Remarks and Perspectives
Chemical modifications of RNAs are invaluable ways to expand decoding capacity of RNA transcripts beyond genetic information inherent to genome sequences. They are crucial for posttranscriptional gene regulatory events such as mRNA splicing, stability, and translation. The ability to regulate the fate of RNA molecules through nucleotide modifications is vital to plant survival and fitness under adverse as well as favorable environmental conditions. Despite the increasing discovery of cellular components essential for chemical modification and decoding of modified RNA molecules, our knowledge regarding physiological roles of proteins involved in these processes is far from sufficient. Several key questions remain to be further investigated. Are there any other internal RNA modifications not identified so far? How these components are regulated depending on developmental stages and/or in response to changing environmental cues? What guides the specificity of interactions between these components with target transcripts? Are these components conserved between dicots and monocots, especially in crop species? Addressing these questions will greatly expand our knowledge on the process of chemical modifications of RNAs and its effects on plant survival and fitness under stressful conditions. Such studies could provide potential new targets for engineering crop plants with higher adaptability to adverse environmental conditions.
Author Contributions
HK designed the concept. JH and SM compiled and analyzed data. JH, SM, and HK contributed to the writing of this review.
Funding
This work was supported by grants from the Next-Generation BioGreen21 Program (PJ01314701 and PJ01312201), funded by Rural Development Administration, Republic of South Korea.
Conflict of Interest Statement
The authors declare that the research was conducted in the absence of any commercial or financial relationships that could be construed as a potential conflict of interest.
References
Alarcon, C. R., Goodarzi, H., Lee, H., Liu, X., Tavazoie, S., and Tavazoie, S. F. (2015). HNRNPA2B1 is a mediator of m6A-dependent nuclear RNA processing events. Cell 162, 1299–1308. doi: 10.1016/j.cell.2015.08.011
Alemu, E. A., He, C., and Klungland, A. (2016). ALKBHs-facilitated RNA modifications and de-modifications. DNA Repair 44, 87–91. doi: 10.1016/j.dnarep.2016.05.026
Arguello, A. E., Deliberto, A. N., and Kleiner, R. (2017). RNA chemical proteomics approach reveals the N6-methyladenosine (m6A)-regulated protein-RNA interactome. J. Am. Chem. Soc. 139, 17249–17252. doi: 10.1021/jacs.7b09213
Arribas-Hernández, L., Bressendorff, S., Hansen, M. H., Poulsen, C., Erdmann, S., and Brodersen, P. (2018). An m6A-YTH module controls developmental timing and morphogenesis in Arabidopsis. Plant Cell 30, 952–967. doi: 10.1105/tpc.17.00833
Bartosovic, M., Molares, H. C., Gregorova, P., Hrossova, D., Kudla, G., and Vanacova, S. (2017). N6-methyladenosine demethylase FTO targets pre-mRNAs and regulates alternative splicing and 3′-end processing. Nucleic Acids Res. 45, 11356–11370. doi: 10.1093/nar/gkx778
Boccaletto, P., Machnicka, M. A., Purta, E., Piatkowski, P., Baginski, B., Wirecki, T. K., et al. (2018). MODOMICS: a database of RNA modification pathways. 2017 update. Nucleic Acids Res. 46, D303–D307. doi: 10.1093/nar/gkx1030
Bodi, Z., Zhong, S., Mehra, S., Song, J., Graham, N., Li, H., et al. (2012). Adenosine methylation in Arabidopsis mRNA is associated with the 3′ end and reduced levels cause developmental defects. Front. Plant Sci. 3:48. doi: 10.3389/fpls.2012.00048
Cantara, W. A., Crain, P. F., Rozenski, J., McCloskey, J. A., Harris, K. A., Zhang, X., et al. (2010). The RNA modification database RNAMDB: 2011 update. Nucleic Acids Res. 39, D195–D201. doi: 10.1093/nar/gkq1028
Chen, T., Hao, Y. J., Zhang, Y., Li, M. M., Wang, M., Han, W., et al. (2015). m6A RNA methylation is regulated by microRNAs and promotes reprogramming to pluripotency. Cell Stem Cell 16, 289–301. doi: 10.1016/j.stem.2015.01.016
Choi, J., Ieong, K. W., Demirci, H., Chen, J., Petrov, A., Prabhakar, A., et al. (2016). N6-methyladenosine in mRNA disrupts tRNA selection and translation-elongation dynamics. Nat. Struct. Mol. Biol. 23, 110–115. doi: 10.1038/nsmb.3148
Chou, H. J., Donnard, E., Gustafsson, H. T., Garber, M., and Rando, O. J. (2017). Transcriptome-wide analysis of roles for tRNA modifications in translational regulation. Mol. Cell 68, 978–992. doi: 10.1016/j.molcel.2017.11.002
Covelo-Molares, H., Bartosovic, M., and Vanacova, S. (2018). RNA methylation in nuclear pre-mRNA processing. Wiley Interdiscip. Rev. RNA 9:e1489. doi: 10.1002/wrna.1489
Cui, X., Liang, Z., Shen, L., Zhang, Q., Bao, S., Geng, Y., et al. (2017). 5-Methylcytosine RNA methylation in Arabidopsis thaliana. Mol. Plant 10, 1387–1399. doi: 10.1016/j.molp.2017.09.013
Dai, D., Wang, H., Zhu, L., Jin, H., and Wang, X. (2018). N6-methyladenosine links RNA metabolism to cancer progression. Cell Death Dis. 9:124. doi: 10.1038/s41419-017-0129-x
David, R., Burgess, A., Parker, B., Li, J., Pulsford, K., Sibbritt, T., et al. (2017). Transcriptome-wide mapping of RNA 5-methylcytosine in Arabidopsis mRNAs and noncoding RNAs. Plant Cell 29, 445–460. doi: 10.1105/tpc.16.00751
Dominissini, D., Moshitch-Moshkovitz, S., Schwartz, S., Salmon-Divon, M., Ungar, L., Osenberg, S., et al. (2012). Topology of the human and mouse m6A RNA methylomes revealed by m6A-seq. Nature 485, 201–206. doi: 10.1038/nature11112
Dominissini, D., Nachtergaele, S., Moshitch-Moshkovitz, S., Peer, E., Kol, N., Benhaim, M. S., et al. (2016). The dynamic N1-methyladenosine methylome in eukaryotic messenger RNA. Nature 530, 441–446. doi: 10.1038/nature16998
Du, H., Zhao, Y., He, J., Zhang, Y., Xi, H., Liu, M., et al. (2016). YTHDF2 destabilizes m6A-containing RNA through direct recruitment of the CCR4–NOT deadenylase complex. Nat. Commun. 7:12626. doi: 10.1038/ncomms12626
Duan, H.-C., Wei, L.-H., Zhang, C., Wang, Y., Chen, L., Lu, Z., et al. (2017). ALKBH10B is an RNA N6-methyladenosine demethylase affecting Arabidopsis floral transition. Plant Cell 29, 2995–3011. doi: 10.1105/tpc.16.00912
Edupuganti, R. R., Geiger, S., Lindeboom, R., Shi, H., Hsu, P. J., Lu, Z., et al. (2017). N6-methyladenosine (m6A) recruits and repels proteins to regulate mRNA homeostasis. Nat. Struct. Mol. Biol. 24, 870–878. doi: 10.1038/nsmb.3462
Fedeles, B. I., Singh, V., Delaney, J. C., Li, D., and Essigmann, J. M. (2015). The AlkB family of Fe(II)/α-ketoglutarate-dependent dioxygenases: repairing nucleic acid alkylation damage and beyond. J. Biol. Chem. 290, 20734–20742. doi: 10.1074/jbc.R115.656462
Flores, J. V., Cordero-Espinoza, L., Oeztuerk-Winder, F., Andersson-Rolf, A., Selmi, T., Blanco, S., et al. (2017). Cytosine-5 RNA methylation regulates neural stem cell differentiation and motility. Stem Cell Rep. 8, 112–124. doi: 10.1016/j.stemcr.2016.11.014
Frye, M., and Watt, F. M. (2006). The RNA methyltransferase Misu (NSun2) mediates Myc-induced proliferation and is upregulated in tumors. Curr. Biol. 16, 971–981. doi: 10.1016/j.cub.2006.04.027
Fustin, J. M., Doi, M., Yamaguchi, Y., Hida, H., Nishimura, S., Yoshida, M., et al. (2013). RNA-methylation-dependent RNA processing controls the speed of the circadian clock. Cell 155, 793–806. doi: 10.1016/j.cell.2013.10.026
Fustin, J. M., Kojima, R., Itoh, K., Chang, H. Y., Ye, S., Zhuang, B., et al. (2018). Two Ck1δ transcripts regulated by m6A methylation code for two antagonistic kinases in the control of the circadian clock. Proc. Natl. Acad. Sci. U.S.A. 115, 5980–5985. doi: 10.1073/pnas.1809838115
Geula, S., Moshitch-Moshkovitz, S., Dominissini, D., Mansour, A. A., Kol, N., Salmon-Divon, M., et al. (2015). m6A mRNA methylation facilitates resolution of naive pluripotency toward differentiation. Science 347, 1002–1006. doi: 10.1126/science.1261417
Gilbert, W. V., Bell, T. A., and Schaening, C. (2016). Messenger RNA modifications: form, distribution and function. Science 352, 1408–1412. doi: 10.1126/science.aad8711
Guo, J., Tang, H.-W., Li, J., Perrimon, N., and Yan, D. (2018). Xio is a component of the Drosophila Sex determination pathway and RNA N6-methyladenosine methyltransferase complex. Proc. Natl. Acad. Sci. U.S.A. 115, 3674–3679. doi: 10.1073/pnas.1720945115
Hastings, M. H. (2013). m6A mRNA methylation: a new circadian pacesetter. Cell 155, 740–741. doi: 10.1016/j.cell.2013.10.028
Haussmann, I. U., Bodi, Z., Sanchez-Moran, E., Mongan, N. P., Archer, N., Fray, R. G., et al. (2016). m6A potentiates Sxl alternative pre-mRNA splicing for robust Drosophila sex determination. Nature 540, 301–304. doi: 10.1038/nature20577
Hsu, P. J., Zhu, Y., Ma, H., Guo, Y., Shi, X., Liu, Y., et al. (2017). Ythdc2 is an N6-methyladenosine binding protein that regulates mammalian spermatogenesis. Cell Res. 27, 1115–1127. doi: 10.1038/cr.2017.99
Huang, H., Weng, H., Sun, W., Qin, X., Shi, H., Wu, H., et al. (2018). Recognition of RNA N6-methyladenosine by IGF2BP proteins enhances mRNA stability and translation. Nat. Cell Biol. 20, 285–296. doi: 10.1038/s41556-018-0045-z
Hussain, S., Tuorto, F., Menon, S., Blanco, S., Cox, C., Flores, J. V., et al. (2013). The mouse cytosine-5 RNA methyltransferase NSun2 is a component of the chromatoid body and required for testis differentiation. Mol. Cell. Biol. 33, 1561–1570. doi: 10.1128/MCB.01523-12
Jackman, J. E., and Alfonzo, J. D. (2013). Transfer RNA modifications: nature’s combinatorial chemistry playground. Wiley Interdiscip. Rev. RNA 4, 35–48. doi: 10.1002/wrna.1144
Jain, D., Puno, M. R., Meydan, C., Lailler, N., Mason, C. E., Lima, C. D., et al. (2018). ketu mutant mice uncover an essential meiotic function for the ancient RNA helicase YTHDC2. eLife 7:e30919. doi: 10.7554/eLife.30919
Jia, G., Fu, Y., Zhao, X., Dai, Q., Zheng, G., Yang, Y., et al. (2011). N6-Methyladenosine in nuclear RNA is a major substrate of the obesity-associated FTO. Nat. Chem. Biol. 7, 885–887. doi: 10.1038/nchembio.687
Kawarada, L., Suzuki, T., Ohira, T., Hirata, S., Miyauchi, K., and Suzuki, T. (2017). ALKBH1 is an RNA dioxygenase responsible for cytoplasmic and mitochondrial tRNA modifications. Nucleic Acids Res. 45, 7401–7415. doi: 10.1093/nar/gkx354
Knuckles, P., Lence, T., Haussmann, I. U., Jacob, D., Kreim, N., Carl, S. H., et al. (2018). Zc3h13/Flacc is required for adenosine methylation by bridging the mRNA-binding factor Rbm15/Spenito to the m6A machinery component Wtap/Fl(2)d. Genes Dev. 32, 415–429. doi: 10.1101/gad.309146
Lence, T., Akhtar, J., Bayer, M., Schmid, K., Spindler, L., Ho, C. H., et al. (2016). m6A modulates neuronal functions and sex determination in Drosophila. Nature 540, 242–247. doi: 10.1038/nature20568
Li, D., Zhang, H., Hong, Y., Huang, L., Li, X., Zhang, Y., et al. (2014a). Genome-wide identification, biochemical characterization, and expression analyses of the YTH domain-containing RNA-binding protein family in Arabidopsis and rice. Plant Mol. Biol. Rep. 32, 1169–1186. doi: 10.1007/s11105-014-0724-2
Li, Y., Wang, X., Li, C., Hu, S., Yu, J., and Song, S. H. (2014b). Transcriptome-wide N6-methyladenosine profiling of rice callus and leaf reveals the presence of tissue-specific competitors involved in selective mRNA modification. RNA Biol. 11, 1180–1188. doi: 10.4161/rna.36281
Li, Z., Weng, H., Su, R., Weng, X., Zuo, Z., Li, C., et al. (2017). FTO plays an oncogenic role in acute myeloid leukemia as a N6-methyladenosine RNA demethylase. Cancer Cell 31, 127–141. doi: 10.1016/j.ccell.2016.11.017
Liao, S. H., Sun, H. B., and Xu, C. (2018). YTH domain: a family of N6-methyladenosine (m6A) readers. Genomics Proteomics Bioinformatics 16, 99–107. doi: 10.1016/j.gpb.2018.04.002
Lin, S., Choe, J., Du, P., Triboulet, R., and Gregory, R. I. (2016). The m6A methyltransferase METTL3 promotes translation in human cancer cells. Mol. Cell 62, 335–345. doi: 10.1016/j.molcel.2016.03.021
Liu, N., and Pan, T. (2016). N6-methyladenosine–encoded epitranscriptomics. Nat. Struct. Mol. Biol. 23, 98–102. doi: 10.1038/nsmb.3162
Luo, G. Z., MacQueen, A., Zheng, G., Duan, H., Dore, L. C., Lu, Z., et al. (2014). Unique features of the m6A methylome in Arabidopsis thaliana. Nat. Commun. 5:5630. doi: 10.1038/ncomms6630
Luo, S., and Tong, L. (2014). Molecular basis for the recognition of methylated adenines in RNA by the eukaryotic YTH domain. Proc. Natl. Acad. Sci. U.S.A. 111, 13834–13839. doi: 10.1073/pnas.1412742111
Ma, S., Gong, Q., and Bohnert, H. J. (2006). Dissecting salt stress pathways. J. Exp. Bot. 57, 1097–1107. doi: 10.1093/jxb/erj098
Martínez-Pérez, M., Aparicio, F., López-Gresa, M. P., Bellés, J. M., Sánchez-Navarro, J. A., and Pallás, V. (2017). Arabidopsis m6A demethylase activity modulates viral infection of a plant virus and the m6A abundance in its genomic RNAs. Proc. Natl. Acad. Sci. U.S.A. 114, 10755–10760. doi: 10.1073/pnas.1703139114
Mauer, J., and Jaffrey, S. R. (2018). FTO, m6Am, and the hypothesis of reversible epitranscriptomic mRNA modifications. FEBS Lett. 592, 2012–2022. doi: 10.1002/1873-3468.13092
Mauer, J., Luo, X., Blanjoie, A., Jiao, X., Grozhik, A. V., Patil, D. P., et al. (2017). Reversible methylation of m6Am in the 5′ cap controls mRNA stability. Nature 541, 371–375. doi: 10.1038/nature21022
Merret, R., Nagarajan, V. K., Carpentier, M.-C., Park, S., Favory, J.-J., Descombin, J., et al. (2015). Heat-induced ribosome pausing triggers mRNA co-translational decay in Arabidopsis thaliana. Nucleic Acids Res. 43, 4121–4132. doi: 10.1093/nar/gkv234
Meyer, K. D., and Jaffrey, S. R. (2014). The dynamic epitranscriptome: N6-methyladenosine and gene expression control. Nat. Rev. Mol. Cell Biol. 15, 313–326. doi: 10.1038/nrm3785
Meyer, K. D., and Jaffrey, S. R. (2017). Rethinking m6A readers, writers and erasers. Annu. Rev. Cell Dev. Biol. 33, 319–342. doi: 10.1146/annurev-cellbio-100616-060758
Meyer, K. D., Patil, D. P., Zhou, J., Zinoviev, A., Skabkin, M. A., Elemento, O., et al. (2015). 5′ UTR m6A promotes cap-independent translation. Cell 163, 999–1010. doi: 10.1016/j.cell.2015.10.012
Mielecki, D., Zugaj, D. L., Muszewska, A., Piwowarski, J., Chojnacka, A., Mielecki, M., et al. (2012). Novel AlkB dioxygenases- alternative models for in silico and in vivo studies. PLoS One 7:e30588. doi: 10.1371/journal.pone.0030588
Müller, T. A., Struble, S. L., Meek, K., and Hausinger, R. P. (2018). Characterization of human AlkB homolog 1 produced in mammalian cells and demonstration of mitochondrial dysfunction in ALKBH1-deficient cells. Biochem. Biophys. Res. Commun. 495, 98–103. doi: 10.1016/j.bbrc.2017.10.158
Nelson, D. C., Flematti, G. R., Riseborough, J. A., Ghisalberti, E. L., Dixon, K. W., and Smith, S. M. (2010). Karrikins enhance light responses during germination and seedling development in Arabidopsis thaliana. Proc. Natl. Acad. Sci. U.S.A. 107, 7095–7100. doi: 10.1073/pnas.0911635107
Nicastro, G., Taylor, I. A., and Ramos, A. (2015). KH-RNA interactions: back in the groove. Curr. Opin. Struct. Biol. 30, 63–70. doi: 10.1016/j.sbi.2015.01.002
Niessen, M., Schneiter, R., and Nothiger, R. (2001). Molecular identification of virilizer, a gene required for the expression of the sex-determining gene sex-lethal in Drosophila melanogaster. Genetics 157, 679–688.
Oerum, S., Dégut, C., Barraud, P., and Tisné, C. (2017). m1A post-transcriptional modification in tRNAs. Biomolecules 7:E20. doi: 10.3390/biom7010020
Ougland, R., Lando, D., Jonson, I., Dahl, J. A., Moen, M. N., Nordstrand, L. M., et al. (2012). ALKBH1 is a histone H2A dioxygenase involved in neural differentiation. Stem Cells 30, 2672–2682. doi: 10.1002/stem.1228
Ougland, R., Rognes, T., Klungland, A., and Larsen, E. (2015). Non-homologous functions of AlkB homologs. J. Mol. Cell Biol. 7, 494–504. doi: 10.1093/jmcb/mjv029
Pan, Y., Ma, P., Liu, Y., Li, W., and Shu, Y. (2018). Multiple functions of m6A RNA methylation in cancer. J. Hematol. Oncol. 11:48. doi: 10.1186/s13045-018-0590-8
Park, C. H., and Hong, K. (2017). Epitranscriptome: m6A and its function in stem cell biology. Genes Genomics 39, 371–378. doi: 10.1007/s13258-016-0507-2
Pastore, C., Topalidou, I., Forouhar, F., Yan, A. C., Levy, M., and Hunt, J. F. (2012). Crystal structure and RNA biding properties of the RNA recognition motif (RRM) and AlkB domains in human AlkB homolog 8 (ABH8), an enzyme catalyzing tRNA hypermodification. J. Biol. Chem. 287, 2130–2143. doi: 10.1074/jbc.M111.286187
Ping, X. L., Sun, B. F., Wang, L., Xiao, W., Yang, X., Wang, W. J., et al. (2014). Mammalian WTAP is a regulatory subunit of the RNA N6-methyladenosine methyltransferase. Cell Res. 24, 177–189. doi: 10.1038/cr.2014.3
Růžička, K., Zhang, M., Campilho, A., Bodi, Z., Kashif, M., Saleh, M., et al. (2017). Identification of factors required for m6A mRNA methylation in Arabidopsis reveals a role for the conserved E3 ubiquitin ligase HAKAI. New Phytol. 215, 157–172. doi: 10.1111/nph.14586
Safra, M., Saschen, A., Nir, R., Winkler, R., Nachshon, A., Bar-Yaacov, D., et al. (2017). The m1A landscape on cytosolic and mitochondrial mRNA at single-base resolution. Nature 551, 251–255. doi: 10.1038/nature24456
Saletore, Y., Meyer, K., Korlach, J., Vilfan, I. D., Jaffrey, S., and Mason, C. E. (2012). The birth of the Epitranscriptome: deciphering the function of RNA modifications. Genome Biol. 13:175. doi: 10.1186/gb-2012-13-10-175
Schaefer, M., Pollex, T., Hanna, K., and Lyko, F. (2009). RNA cytosine methylation analysis by bisulfite sequencing. Nucleic Acids Res. 37:e12. doi: 10.1093/nar/gkn954
Schwartz, S., Mumbach, M. R., Jovanovic, M., Wang, T., Maciag, K., Bushkin, G. G., et al. (2014). Perturbation of m6A writers reveals two distinct classes of mRNA methylation at internal and 50 sites. Cell Rep. 8, 284–296. doi: 10.1016/j.celrep.2014.05.048
Scutenaire, J., Deragon, J. M., Jean, V., Benhamed, M., Raynaud, C., Favory, J. J., et al. (2018). The YTH domain protein ECT2 is an m6A reader required for normal trichome branching in Arabidopsis. Plant Cell 30, 986–1005. doi: 10.1105/tpc.17.00854
Shah, J. C., and Clancy, M. J. (1992). IME4, a gene that mediates MAT and nutritional control of meiosis in Saccharomyces cerevisiae. Mol. Cell. Biol. 12, 1078–1086. doi: 10.1128/mcb.12.3.1078
Sharma, S., and Lafontaine, D. L. J. (2015). View from a bridge’: a new prospective on eukaryotic rRNA base modificationTrends Biochem. Sci. 40, 560–575. doi: 10.1016/j.tibs.2015.07.008
Shen, F., Huang, W., Huang, J. T., Xiong, J., Yang, Y., Wu, K., et al. (2014). Decreased N6-methyladenosine in peripheral blood RNA from diabetic patients is associated with FTO expression rather than ALK BH5. J. Clin. Endocrinol. Metab. 100, 148–154. doi: 10.1210/jc.2014-1893
Shen, L., Liang, Z., Gu, X., Chen, Y., Teo, Z. W. N., Hou, X., et al. (2016). N6-methyladenosine RNA modification regulates shoot stem cell fate in Arabidopsis. Dev. Cell 38, 186–200. doi: 10.1016/j.devcel.2016.06.008
Shi, H., Xiao, W., Lu, Z., Zhao, B. S., Ma, H., Hsu, P. J., et al. (2017). YTHDF3 facilitates translation and decay of N6-methyladenosine-modified RNA. Cell Res. 27, 315–328. doi: 10.1038/cr.2017.15
Sledz, P., and Jinek, M. (2016). Structural insights into the molecular mechanism of the m6A writer complex. eLife 5:e18434. doi: 10.7554/eLife.18434
Sloan, K. E., Warda, A. S., Sharma, S., Entian, K. D., Lafontaine, D. L. J., and Bohnsack, M. T. (2017). Tuning the ribosome: the influence of rRNA modification on eukaryotic ribosome biogenesis and function. RNA Biol. 14, 1138–1152. doi: 10.1080/15476286.2016.1259781
Song, J., Zhai, J., Bian, E., Song, Y., Yu, J., and Ma, C. (2018). Transcriptome-wide annotation of m5C RNA modifications using machine learning. Front. Plant Sci. 9:519. doi: 10.3389/fpls.2018.00519
Squires, J. E., Patel, H. R., Nousch, M., Sibbritt, T., Humphreys, D. T., Parker, B. J., et al. (2012). Widespread occurrence of 5-methylcytosine in human coding and non-coding RNA. Nucleic Acids Res. 40, 5023–5033. doi: 10.1093/nar/gks144
Tang, C., Klukovich, R., Peng, H., Wang, Z., Yu, T., Zhang, Y., et al. (2018). ALKBH5-dependent m6A demethylation controls splicing and stability of long 3′-UTR mRNAs in male germ cells. Proc. Natl. Acad. Sci. U.S.A. 115, E325–E333. doi: 10.1073/pnas.1717794115
Ueda, Y., Ooshio, I., Fusamae, Y., Kitae, K., Kawaguchi, M., Jingushi, K., et al. (2017). AlkB homolog 3-mediated tRNA demethylation promotes protein synthesis in cancer cells. Sci. Rep. 7:42271. doi: 10.1038/srep42271
Väre, V. Y., Eruysal, E. R., Narendran, A., Sarachan, K. L., and Agris, P. F. (2017). Chemical and conformational diversity of modified nucleosides affects tRNA structure and function. Biomolecules 7:E29. doi: 10.3390/biom7010029
Wang, C. Y., Shie, S. S., Wen, M. S., Hung, K. C., Hsieh, I. C., Yeh, T. S., et al. (2015a). Loss of FTO in adipose tissue decreases ANGPTL4 translation and alters triglyceride metabolism. Sci. Signal. 8:ra127. doi: 10.1126/scisignal.aab3357
Wang, X., Feng, J., Xue, Y., Guan, Z., Zhang, D., Liu, Z., et al. (2016). Structural basis of N6-adenosine methylation by the METTL3-METTL14 complex. Nature 534, 575–578. doi: 10.1038/nature18298
Wang, X., Huang, N., Yang, M., Wei, D., Tai, H., Han, X., et al. (2017). FTO is required for myogenesis by positively regulating mTOR-PGC-1α pathway-mediated mitochondria biogenesis. Cell Death Dis. 8:e2702. doi: 10.1038/cddis.2017.122
Wang, X., Lu, Z., Gomez, A., Hon, G. C., Yue, Y., Han, D., et al. (2014). N6-methyladenosine-dependent regulation of messenger RNA stability. Nature 505, 117–120. doi: 10.1038/nature12730
Wang, X., Zhao, B. S., Roundtree, I. A., Lu, Z., Han, D., Ma, H., et al. (2015b). N6-methyladenosine modulates messenger RNA translation efficiency. Cell 161, 1388–1399. doi: 10.1016/j.cell.2015.05.014
Wei, L.-H., Song, P., Wang, Y., Lu, Z., Tang, Q., Yu, Q., et al. (2018). The m6A reader ECT2 controls trichome morphology by affecting mRNA stability in Arabidopsis. Plant Cell 30, 968–985. doi: 10.1105/tpc.17.00934
Westbye, M. P., Feyzi, E., Aas, P. A., Vågbø, C. B., Talstad, V. A., Kavli, B., et al. (2008). Human AlkB homolog 1 is a mitochondrial protein that demethylates 3-methylcytosine in DNA and RNA. J. Biol. Chem. 283, 25046–25056. doi: 10.1074/jbc.M803776200
Widagdo, J., and Anggono, V. (2018). The m6A-epitranscriptomic signature in neurobiology: from neurodevelopment to brain plasticity. J. Neurochem. 147, 137–152. doi: 10.1111/jnc.14481
Wu, B., Li, L., Huang, Y., Ma, J., and Min, J. (2017). Readers, writers and erasers of N6-methylated adenosine modification. Curr. Opin. Struct. Biol. 47, 67–76. doi: 10.1016/j.sbi.2017.05.011
Wu, T. P., Wang, T., Seetin, M. G., Lai, Y., Zhu, S., Lin, K., et al. (2016). DNA methylation on N6-adenine in mammalian embryonic stem cells. Nature 532, 329–333. doi: 10.1038/nature17640
Xiang, Y., Laurent, B., Hsu, C. H., Nachtergaele, S., Lu, Z., Sheng, W., et al. (2017). RNA m6A methylation regulates the ultraviolet-induced DNA damage response. Nature 543, 573–576. doi: 10.1038/nature21671
Xiao, W., Adhikari, S., Dahal, U., Chen, Y. S., Hao, Y. J., Sun, B. F., et al. (2016). Nuclear m6A reader YTHDC1 regulates mRNA splicing. Mol. Cell 61, 507–519. doi: 10.1016/j.molcel.2016.01.012
Xu, C., Wang, X., Liu, K., Roundtree, I. A., Tempel, W., Li, Y., et al. (2014). Structural basis for selective binding of m6A RNA by the YTHDC1 YTH domain. Nat. Chem. Biol. 10, 927–929. doi: 10.1038/nchembio.1654
Yue, Y., Liu, J., Cui, X., Cao, J., Luo, G., Zhang, Z., et al. (2018). VIRMA mediates preferential m6A mRNA methylation in 3′UTR and near stop codon and associates with alternative polyadenylation. Cell Discov. 4:10. doi: 10.1038/s41421-018-0019-0
Yue, Y., Liu, J., and He, C. (2015). RNA N6-methyladenosine methylation in post-transcriptional gene expression regulation. Genes Dev. 29, 1343–1355. doi: 10.1101/gad.262766.115
Zhang, C., Samanta, D., Lu, H., Bullen, J. W., Zhang, H., Chen, I., et al. (2016). Hypoxia induces the breast cancer stem cell phenotype by HIF-dependent and ALKBH5-mediated m6A-demethylation of NANOG mRNA. Proc. Natl. Acad. Sci. U.S.A. 113, E2047–E2056. doi: 10.1073/pnas.1602883113
Zhang, S., Zhao, B. S., Zhou, A., Lin, K., Zheng, S., Lu, Z., et al. (2017). m6A demethylase ALKBH5 maintains tumorigenicity of glioblastoma stem-like cells by sustaining FOXM1 expression and cell proliferation program. Cancer Cell 31, 591–606. doi: 10.1016/j.ccell.2017.02.013
Zhao, X., Yang, Y., Sun, B. F., Shi, Y., Yang, X., Xiao, W., et al. (2014). FTO-dependent demethylation of N6-methyladenosine regulates mRNA splicing and is required for adipogenesis. Cell Res. 24, 1403–1419. doi: 10.1038/cr.2014.151
Zheng, G., Dahl, J. A., Niu, Y., Fedorcsak, P., Huang, C. M., Li, C., et al. (2013). ALKBH5 is a mammalian RNA demethylase that impacts RNA metabolism and mouse fertility. Mol. Cell 49, 18–29. doi: 10.1016/j.molcel.2012.10.015
Zhong, S., Li, H., Bodi, Z., Button, J., Vespa, L., Herzog, M., et al. (2008). MTA is an Arabidopsis messenger RNA adenosine methylase and interacts with a homolog of a sex-specific splicing factor. Plant Cell 20, 1278–1288. doi: 10.1105/tpc.108.058883
Keywords: abiotic stress, epitranscriptome, RNA metabolism, RNA methylation, RNA modification
Citation: Hu J, Manduzio S and Kang H (2019) Epitranscriptomic RNA Methylation in Plant Development and Abiotic Stress Responses. Front. Plant Sci. 10:500. doi: 10.3389/fpls.2019.00500
Received: 14 October 2018; Accepted: 01 April 2019;
Published: 17 April 2019.
Edited by:
Sang Yeol Lee, Gyeongsang National University, South KoreaReviewed by:
Andreas Bachmair, University of Vienna, AustriaByungho-Ho Kang, The Chinese University of Hong Kong, China
Copyright © 2019 Hu, Manduzio and Kang. This is an open-access article distributed under the terms of the Creative Commons Attribution License (CC BY). The use, distribution or reproduction in other forums is permitted, provided the original author(s) and the copyright owner(s) are credited and that the original publication in this journal is cited, in accordance with accepted academic practice. No use, distribution or reproduction is permitted which does not comply with these terms.
*Correspondence: Hunseung Kang, hskang@jnu.ac.kr
†These authors have contributed equally to this work