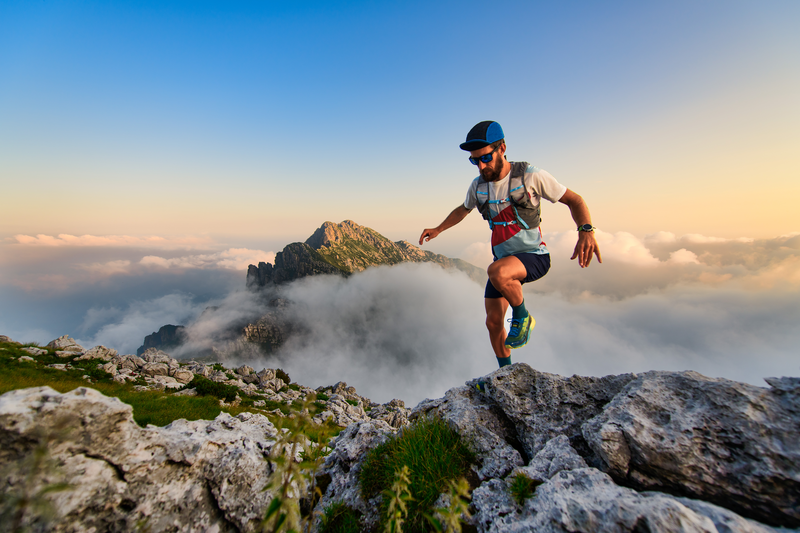
94% of researchers rate our articles as excellent or good
Learn more about the work of our research integrity team to safeguard the quality of each article we publish.
Find out more
MINI REVIEW article
Front. Plant Sci. , 09 April 2019
Sec. Plant Metabolism and Chemodiversity
Volume 10 - 2019 | https://doi.org/10.3389/fpls.2019.00439
This article is part of the Research Topic Polyamines in Plant Biotechnology, Food Nutrition and Human Health View all 25 articles
Polyamines (PAs) are related to many aspects of the plant’s life cycle, including responses to biotic and abiotic stress. On the other hand, halophytic plants are useful models for studying salt tolerance mechanisms related to the adaptive strategies that these plants present in adverse environments. Furthermore, some halophytes have high economic value, being recommended instead of glycophytes as alternative agricultural crops in salt-affected coastal zones or saline farmlands. In recent years, the understanding of the role of PAs in salt-tolerant plants has greatly advanced. This mini review reports on the advances in the knowledge of PAs and their participation in achieving better salt tolerance in 10 halophytes. PAs are associated with responses to heavy metals in phytoremediation processes using certain salt-tolerant species (Atriplex atacamensis, A. halimus, Inula chrithmoides, and Kosteletzkya pentacarpos). In crops with exceptional nutritional properties such as Chenopodium quinoa, PAs may be useful markers of salt-tolerant genotypes. The signaling and protection mechanisms of PAs have been investigated in depth in the extreme halophyte Mesembryanthemum crystallinum and Thellungiella spp., enabling genetic manipulation of PA biosynthesis. In Prosopis strombulifera, different biochemical and physiological responses have been reported, depending on the type of salt (NaCl, Na2SO4). Increases in spermidine and spermine have been positively associated with stress tolerance as these compounds provide protection in Cymodocea nodosa, and Solanum chilense, respectively. In addition, abscisic acid and salicylic acid can improve the beneficial effect of PAs in these plants. Therefore, these results indicate the great potential of PAs and their contribution to stress tolerance.
Halophytes comprise a group of plants able to complete their life cycle under saline environmental conditions of around 200 mM NaCl or even more (Flowers and Colmer, 2008; Golldack et al., 2014). Representing roughly 1% of the total world flora, they are distributed mainly in arid and wetlands saline areas, as well as in temperate zones (Gul et al., 2013; Kumari et al., 2015). These plants provide useful models to understand the mechanisms of adaptation to saline stress (Hurst et al., 2004; Arbona et al., 2010). The salt-tolerance strategies include: accumulation or exclusion of ions, control and compartmentalization of ion uptake, maintenance of osmotic balance (Shabala and Mackay, 2011; Bose et al., 2014), biosynthesis of compatible solutes (Flowers and Colmer, 2015; Slama et al., 2015), shift in the photosynthetic pathway (Uzilday et al., 2015), activation and synthesis of antioxidant compounds (Ozgur et al., 2013), induction and modulation of plant hormones (Gupta and Huang, 2014; Fahad et al., 2015), and the modulation of polyamines (PAs) (Shi and Chan, 2014; Liu et al., 2015). The use of mutants, transgenic plants, or exogenous PAs has revealed the key role of these compounds in multiple responses to salt stress (Liu et al., 2014; Parvin et al., 2014; Pathak et al., 2014).
Polyamines are polybasic aliphatic amines, widely distributed in all living organisms and involved in multiple cellular functions (Kusano et al., 2008; Hussain et al., 2011; Gupta et al., 2013; Tiburcio et al., 2014). The most commonly occurring PAs, found in halophytes, are the diamines putrescine (Put) and cadaverine (Cad), the triamine spermidine (Spd), and the tetramine spermine (Spm) (Shevyakova et al., 2006b; Kuznetsov et al., 2007). These compounds may serve as bioindicators of stress-tolerant lines (Simon-Sarkadi et al., 2007), stabilize biological membranes, regulate ion homeostasis, delay senescence processes (Lutts et al., 2013), regulate membrane transport through a direct interaction with plasma membrane or vacuolar transporters (Pottosin and Shabala, 2014), protect photosynthetic tissues (Malliarakis et al., 2015), regulate the antioxidant system and free-radical machinery (Sudhakar et al., 2015), participate in abiotic stress signaling (Pál et al., 2015), and protect against metal toxicity (Ghabriche et al., 2017; Zhou et al., 2018a,b). Furthermore, PAs also activate genes for stress response and interact with other metabolic pathways by establishing hormonal cross-talk (Pál et al., 2015; Llanes et al., 2018). Most studies on PAs have been made in glycophytes. The present review attempts to consolidate our understanding of the effects of PAs and their role in the physiology of 10 halophytes (Table 1), helping to elucidate the potential mechanisms of these growth regulators in halophytic plants.
Shevyakova et al. (2006b) were the first to investigate the free PA content in Mesembryanthemum crystallinum L. (the common ice plant) a C3-CAM (crassulacean acid metabolism) plant (Adams et al., 1998; Winter and Holtum, 2007), under severe salinity conditions. The dynamics of Spm accumulation resembled that of phosphoenolpyruvate carboxylase (PEPC), a key enzyme of the water-saving CAM pathway; this could indicate an indirect involvement of PAs in plant adaptation to stress. The gradual increase in Spm could be due to several causes: the conversion of Spd into Spm, the release of free Spm (active) from its conjugates, changes in the biosynthetic rate, and the degradation and/or competition between PAs and ethylene based on alterations in sulfur metabolism, necessary for S-adenosyl methionine (SAM) synthesis (a precursor of both PAs and ethylene). Shevyakova et al. (2006a) and Stetsenko et al. (2009) showed the accumulation of free and conjugated forms of PAs, although the latter predominated under saline conditions. However, for Cad, conjugated forms underwent a transition into the free form. These results indicate considerable quantitative organ-specific changes in the pool of free and conjugated forms, regulated by the combined action of H2O2-peroxidase and the oxidative degradation of PAs. 1.3-diaminopropane (Dap) was located in roots and was related with protection of membranes. Kuznetsov et al. (2007) suggested that accumulation of Cad could compensate for the Put deficit, acting as a DNA protector and a free-radical scavenger. Shevyakova et al. (2011) also demonstrated an antioxidant role of PAs. Spd diminished the expression of ferritin gene and consequently the production of reactive oxygen species (ROS). Shevyakova et al. (2013) found a protective effect of ABA related to the regulation of proline (Pro), PAs, and cytokinin contents. Under saline conditions, ABA treatment increased free PA content, in roots, whereas that in leaves, could regulate conjugate forms, thus controlling the PA biological functions. ABA may also be related to the transport of Cad from roots (decreased levels) to leaves (increased levels). The mechanisms of signaling and protection of PAs in M. crystallinum were reported by Surówka et al. (2016). These researchers studied (under exogenous application of H2O2) the diurnal rhythm cycle of antioxidants and osmotic compounds (transition C3-CAM), which could be responsible for the maintenance of the water-potential gradient, ROS homeostasis, and the prevention of oxidative damage. Among these components, PAs (accumulation of Put and Spd but lower amounts of Spm and agmatine) showed a daily pattern of accumulation, similar to malate, suggesting a metabolic connection between the two routes. On the other hand, the accumulation of these amines could be related to mitigate oxidative stress, and maintenance maximum photosystem II (PS II) photochemical efficiency (Fv/Fm) (Kotakis et al., 2014). These mechanisms regulate cellular metabolism and trigger signaling cascades leading to stress acclimation.
Radyukina et al. (2007) studying a new model plant Thellungiella halophila, found a high capability of accumulation of Na+ and Cl− ions, in their roots and leaves, under progressing salinity. In addition, they observed Pro accumulation due to enhanced expression of biosynthetic genes, accumulation of Spd and later Spm, increased expression of SAM synthetase and Spd synthase genes, and high constitutive levels of peroxidase that palliates the severity of oxidative stress. Later, Zhou et al. (2015) studied a novel Spd synthase gene (EsSPDS1), which was cloned and characterized from the halophytic plant Eutrema salsugineum. The expression of this gene was induced by polyethylene glycol (PEG), NaCl and ABA treatments. In transgenic tobacco plants, EsSPDS1-overexpressing lines exhibited drought tolerance via the reduction of water loss and the increase of sugar and Pro, favoring osmoregulation, stabilizing protein structure, and reducing oxidative potential. Overexpression of EsSPDS1 lowered the levels of malondialdehyde (MDA), diminished ion leakage, and also activated the antioxidant enzymes superoxide dismutase (SOD) and catalase (CAT), which mitigated oxidative stress. These data suggest that the activation of the EsSPDS1 gene could be an option for developing drought-tolerant plants. Ping Lee et al. (2016), working with diverse accessions of Thellungiella spp. collected from different geographical origins in many saline habitats, found that all accessions survived and grew with up to 700 mM NaCl. Salt stress induced the accumulation of high amounts of osmolytes. With respect to PAs, Put levels increased linearly with salt concentrations in all accessions. These researchers indicated a pre-adaptation to salt stress and a stronger metabolic reaction (e.g., accumulation of osmolytes) compared to its close relative Arabidopsis thaliana, a glycophyte. The protective role of Put in response to UV-B irradiation, in leaves of T. salsuginea and other species, was investigated by Radyukina et al. (2017), who concluded that the contribution of bound and free PAs is determined by the species studied and was organ-specific (roots versus leaves).
Most of the species for human consumption belong to glycophytes (salt-sensitive plants), so halophytes can be used as an alternative to traditional agricultural crops, especially in countries with severe climatic conditions (Panta et al., 2014). Quinoa (Chenopodium quinoa Willd.) is a halophytic Andean plant, belonging to the Amaranthaceae, with remarkable tolerance to abiotic stress (Bosque-Sánchez et al., 2003; Martínez et al., 2009). This plant has excellent nutritional properties, such as a high protein content and excellent amino acid composition (Repo-Carrasco et al., 2003; Aloisi et al., 2016). Orsini et al. (2011), studying a quinoa accession (BO78) from southern Chile, demonstrated an inverse relationship of Put with tissue levels of Na+ or K+, thus relating this diamine to the maintenance of a suitable cation/anion balance. A more complete study on four quinoa genotypes, located in coastal-central and southern Chile along a latitudinal gradient, was undertaken by Ruiz-Carrasco et al. (2011), comparing their growth, physiological, and molecular responses, at seedling stage, under saline conditions. With respect to PA levels, all salt-treated quinoa genotypes followed the same pattern, i.e., a sharp decline in Put levels, accompanied by an increase in the ratio of (Spd + Spm) to Put, Spd and Spm being more closely related to vital physiological processes. These researchers concluded that salt-tolerant genotypes might have high levels of both Pro and PAs (Spd/Spm) and could offer a protective function, being useful indicators of salinity adaptation, and potential genotypes for crop improvement. Pottosin and Shabala (2014) and Pottosin et al. (2014) found that PAs were related to the regulation of slow-(SV) and fast-(FV) vacuolar cation channels, as well as vacuolar and (PM) +H pumps and Ca+2 pumps of the plasma membrane. Activation of Ca+2 efflux by PAs and contrasting effects of PAs on net H+ fluxes and membrane potential could contribute to modulate transport processes across the plasma membrane in stress conditions. More recently, Ruiz et al. (2017) studied the PA content and expression of genes involved in PA metabolism in R49 (salares ecotype and northern Chile) and Villarrica (VR, coastal lowlands ecotype, and southern Chile), in response to saline stress. A salt-induced increase was found in the (Spd + Spm): Put ratio, in VR the increase in the ratio occurs later than in R49, although it was significantly higher, distinguishing quinoa genotypes from different habitats. At the gene expression level, it was confirmed that lower Put levels may have resulted from diamine oxidase (DAO) up-regulation, arginine decarboxylase (ADC1) down-regulation, and (ADC2) up-regulation under saline conditions. In both landraces, the higher expression of Spm synthase (SPMS) relative to control was consistent with Spm accumulation. In addition, these authors suggested that the PA response found in both landraces could be a consequence of the early up-regulation of 9-Cis-epoxycarotenoid dioxygenase (NCED), a key enzyme in ABA biosynthesis, which preceded that of the PA biosynthesis genes, and the increase of ABA levels. Pál et al. (2015) have proposed a positive feedback loop in the response to abiotic stress between ABA and PAs.
In recent years, tomato has lost notable growth and yield, especially in arid and semi-arid zones due to intensive irrigation and soil salinization (Munns and Tester, 2008; Gharbi et al., 2017). Comparisons of the glycophyte Solanum lycopersicum and its wild relative halophyte S. chilense have revealed different ways of tolerating stress, the higher tolerance being related to enhanced levels of salicylic acid (SA), ethylene, and PAs (Gharbi et al., 2016). These researchers have shown an outstanding osmotic adjustment in leaves and greater ethylene production in salt-treated plants of S. chilense, correlated with increases, in both shoot and root, of K+ concentrations, as occurs in other species such as Arabidopsis (Yang et al., 2013). With respect to its biosynthesis, ethylene overproduction proved to be related to 1-aminocyclopropane-1-carboxylic acid synthase (ACCS2) gene induction, which has not been reported in S. lycopersicum. Jiang et al. (2013) showed that ethylene may contribute to Na+/K+ homeostasis, under saline stress. Endogenous SA increased in the halophyte in relation to greater stomatal conductance (gs), improving the metabolism under stressful conditions. Exogenous SA application and salt may act in synergy on osmolyte synthesis, and a positive linear correlation was recorded between SA content and ethylene synthesis for S. chilense. PA levels proved consistently higher in the halophyte than in the glycophyte. Saline stress lowered Put levels and reduced the expression of genes coding for ornithine decarboxylase ODC, ADC1, and ADC2 in the halophyte, whereas NaCl increased the expression of those genes in S. lycopersicum. Conversely, the tetraamine Spm was only increased in S. chilense. Hu et al. (2012) considered high Spd and Spm content to have protective functions in the glycophyte tomato. The application of SA raised PA levels in the aerial part of S. chilense (increased ADC1 and ADC2 gene expression) so that Put production was partly used for Spd synthesis, but this treatment had no impact in S. lycopersicum. However, when the plants were treated with NaCl + SA, the induction of gene expression was not correlated with the accumulation of products, implying a post-transcriptional regulation of PA metabolism. Finally, NaCl slightly induced the expression of the SAM decarboxylase (SAMDC) gene in S. chilense, while a higher expression was registered in S. lycopersicum, suggesting differential responses to salinity in the two species.
Two of the halophytes most widely studied for their high levels of resistance to salinity stress and drought (Jordan et al., 2002; Martínez et al., 2005) have been the xero-halophytes Atriplex atacamensis Phil., a native shrub from Atacama Desert (Chile), and A. halimus, a species of Mediterranean areas (Amaranthaceae family). These tolerate salinity by excreting ions (Cl− and Na+) through the trichomes located on the surface of their leaves, known as salt bladders, which allow the expulsion of certain amounts of heavy metals (Ben Hassine et al., 2009; Vromman et al., 2011). Given their high biomass production, and capacity to tolerate elevated concentrations of toxic ions (Lutts et al., 2004; Manousaki and Kalogerakis, 2009; Vromman et al., 2011), these could be promising species for phytoremediation. Data reported by Ben Hassine et al. (2009) in A. halimus, suggest that free PAs, mainly Spd and Spm, may be involved in the excretion processes and in the regulation of ion fluxes through salt bladders. These researchers suggested that the increase in endogenous PAs (interacting with ATPase or ion channels involved in Na+ and Cl− fluxes between epidermis and basal cells of bladders) could reduce Na+ influx into mesophyll cells, thereby re-directing Na+ flux toward salt bladders. In addition, ABA contributes to PA synthesis and the conversion from bound and conjugated to free soluble forms of PAs, thus favoring salt excretion. Lefèvre et al. (2009, 2010) also found high levels of Put and Spd in a water-stress resistant cell line of A. halimus, when tissues were maintained on a medium containing cadmium (Cd), whereas Spm and Dap were higher in the drought stress-sensitive line. PAs are effective in decreasing ionic toxicity, reducing oxidative stress, and restricting the harmful impact of Cd. On the other hand, A. atacamensis is able to grow with high external arsenic (As) concentrations in their natural habitat. Vromman et al. (2011), studying the root and leaves of this halophyte, found an increase in free soluble PAs in As-exposed plants. Specifically, Spd and Spm increased at the expense of Put. These researchers hypothesize that these polycationic molecules may assist in arsenate sequestration in the stressed tissues, and therefore should be tested in field trials for the phytomanagement of As-contaminated sites.
Another halophyte common in Mediterranean zones is Inula chrithmoides, which belongs to the Asteraceae family. It is a perennial coastal species found on sea cliffs and in salt marshes. Abdel-Wahhab et al. (2008) found medicinal applications by analyzing their metabolite production. This species is found in areas contaminated by Cd, nickel, and salt (Zurayk et al., 2001). In absence of Cd, Ghabriche et al. (2017) have shown that NaCl (100 mM) strongly boosted plant growth and improved the Na+: K+ ratio in shoot of I. chrithmoides. Meanwhile, in Cd-treated plants, NaCl protected this species from ionic toxicity and helped reduce Cd absorption and translocation. PAs increased in response to both NaCl and CdCl2 (mainly the bound PA fraction) in leaves and roots, and this increase could be related to protective functions of PAs on endogenous cellular structures. In rice, Li et al. (2013) found that Cd decreased the activities of glutamine synthetase and glutamate synthase, enzymes of N assimilation pathway, and therefore increased NH4+ and arginine content (a precursor of Put). Thus, Ghabriche et al. (2017) proposed that the Cd-induced alteration of the N cycle was the main factor responsible for altering PA levels in I. chrithmoides.
The halophyte Kosteletzkya pentacarpos (formerly K. virginica) is a perennial wetland species (Malvaceae family) and a promising plant for saline agriculture (He et al., 2003). This plant grows in coastal areas contaminated by industrial activity (Lutts and Lefèvre, 2015). Zhou et al. (2018a,b) found that salt and heavy metals altered the endogenous PA content, providing protection under these stress conditions. Heavy metals augmented ethylene synthesis, but NaCl depressed it in plants exposed to Cd or the combined treatment (Cd + Zn) but not with Zn. PA concentrations were altered by Cd (Spd and Spm decreased concomitantly with Put accumulation). These researchers concluded that PA profile could be the result of ethylene overproduction in Cd-treated plants because SAM is a precursor of ethylene, Spd, and Spm. Inhibition of ethylene synthesis increased Spd and Spm level and reduced Cd accumulation.
In Cymodocea nodosa, a seagrass, PAs are distributed in all plant tissues, but mainly the apical section of the rhizome (Marián et al., 2000). Zarranz Elso et al. (2012) showed a decline in PAs during embryo development (free PAs), and an increase during germination, indicating the key role of these growth regulators in the first steps of the zygotic and germination stages. Exogenous Spd helps improve Fv/Fm-values, exerting a protective role in maintaining the photosynthetic apparatus. Ioannidis and Kotzabasis (2007), Hamdani et al. (2011), and Malliarakis et al. (2015) found that application of PAs (Spd and Spm) could restore PSII efficiency, via electrostatic interactions with extrinsic and intrinsic, and PSII thylakoid proteins, thereby stabilizing them. These results indicate that C. nodosa requires elevated PA levels to survive in high salinity. Prosopis strombulifera, a common spiny shrub, belonging to the family Fabaceae (subfamily Mimosoideae) is distributed in arid regions (of North and South America). Reginato (2009) and Reginato et al. (2012) considered this plant to be a true “euhalophyte” for NaCl, being less tolerant to Na2SO4, although a partial improvement was found with two salt mixtures. Put accumulation exerted a beneficial effect in NaCl-treated plants, stimulating growth and the antioxidative defense system. However, low Put levels, in leaves, were correlated with inhibition of shoot growth, associated with Na2SO4 toxicity. Cad and Dap were related with stress symptoms, and possibly, the activities of PA oxidases.
MB and M-PC revised the Bibliography. MB wrote the manuscript.
This research was supported by Spanish Ministry of Science and Innovation (CGL2006-08830, “The involvement of growth regulators in plant responses to salinity”).
The authors declare that the research was conducted in the absence of any commercial or financial relationships that could be construed as a potential conflict of interest.
Abdel-Wahhab, M. A., Abdel-Azim, S. H., and El-Nekeety, A. A. (2008). Inula crithmoides extract protects against ochratoxin A-induced oxidative stress, clastogenic and mutagenic alterations in male rats. Toxicon 52, 566–573. doi: 10.1016/j.toxicon.2008.07.006
Adams, P., Nelson, D. E., Yamada, S., Chmara, W., Jensen, R. G., Bohnert, H. J., et al. (1998). Growth and development of Mesembryanthemum crystallinum (Aizoaceae). New Phytol. 138, 171–190. doi: 10.1046/j.1469-8137.1998.00111.x
Aloisi, I., Parrotta, L., Ruíz, K. B., Landi, C., Bini, L., Cai, G., et al. (2016). New insight into quinoa seed quality under salinity: changes in proteomic and amino acid profiles, phenolic content, and antioxidant activity of protein extracts. Front. Plant Sci. 7:656. doi: 10.3389/fpls.2016.00656
Arbona, V., Argamasilla, R., and Gómez-Cadenas, A. (2010). Common and divergent physiological, hormonal and metabolic responses of Arabidopsis thaliana and Thellungiella halophila to water and salt stress. J. Plant Physiol. 167, 1342–1350. doi: 10.1016/j.jplph.2010.05.012
Ben Hassine, A., Ghanem, M. E., Bouzid, S., and Lutts, S. (2009). Abscisic acid has contrasting effects on salt excretion and polyamine concentrations of an inland and a coastal population of the Mediterranean xero-halophyte species Atriplex halimus. Ann. Bot. 104, 925–936. doi: 10.1093/aob/mcp174
Bose, J., Rodrigo-Moreno, A., Lai, D., Xie, Y., Shen, W., and Shabala, S. (2014). Rapid regulation of the plasma membrane H+-ATP-asa activity is essential to salinity tolerance in two halophytes species, Atriplex lentiformis and Chenopodium quinoa. Ann. Bot. 115, 481–494. doi: 10.1093/aob/mcu219
Bosque-Sánchez, H., Lemeur, R., Van Damme, P., and Jacobsen, S. E. (2003). “Ecophysical analysis of drought and salinity stress of quinoa (Chenopodium quinoa Willd.),” in Quinoa-An Andean Food Group, eds S. E. Jacobsen and A. Mujica (New York, NY: Marcel Dekker Inc.,), 111–119.
Fahad, S., Hussain, S., Matloob, A., Khan, F. A., Khaliq, A., Saud, S., et al. (2015). Phytohormones and plant responses to salinity stress: a review. Plant Growth Regul. 75, 391–404. doi: 10.1007/s10725-014-0013-y
Flowers, T. J., and Colmer, T. D. (2008). Salinity tolerance in halophytes. New Phytol. 179, 945–963. doi: 10.1111/j.1469-8137.2008.02531.x
Flowers, T. J., and Colmer, T. D. (2015). Plant salt tolerance: adaptation in halophytes. Ann. Bot. 115, 327–331. doi: 10.1093/aob/mcu267
Ghabriche, R., Ghnaya, T., Mnasri, M., Zaier, H., Baioui, R., Vromman, D., et al. (2017). Polyamine and tyramine involvement in NaCl-induced improvement of Cd resistance in the halophyte Inula chrithmoides L. J. Plant Physiol. 216, 136–144. doi: 10.1016/j.jplph.2017.05.018
Gharbi, E., Martínez, J. P., Benahmed, H., Dailly, H., Quinet, M., and Lutts, S. (2017). The salicylic acid analog 2,6-dichloroisonicotinic acid has specific impact on the response of the halophyte plant species Solanum chilense to salinity. Plant Growth Regul. 82, 517–525. doi: 10.1007/s10725-017-0278-z
Gharbi, E., Martínez, J. P., Benahmed, H., Fauconnier, M. L., Lutts, S., and Quinet, M. (2016). Salicylic acid differently impacts ethylene and polyamine synthesis in the glycophyte Solanum lycopersicum and the wild-related halophyte Solanum chilense exposed to mild salt stress. Physiol. Plant 158, 152–167. doi: 10.1111/ppl.12458
Golldack, D., Li, C., Mohan, H., and Probst, N. (2014). Tolerance to drought and salt stress in plants: unraveling the signaling networks. Front. Plant Sci. 5:151. doi: 10.3389/fpls.2014.00151
Gul, B., Ansari, R., Flowers, T. J., and Khan, M. A. (2013). Germination strategies of halophyte seeds under salinity. Environ. Exp. Bot. 92, 4–18. doi: 10.1016/j.envexpbot.2012.11.006
Gupta, B., and Huang, B. (2014). Mechanism of salinity tolerance in plants: physiological, biochemical, and molecular characterization. Int. J. Genom. 2014, 1–18. doi: 10.1155/2014/701596
Gupta, K., Dey, A., and Gupta, B. (2013). Plant polyamines in abiotic stress responses. Acta Physiol. Plant. 35, 2015–2036. doi: 10.1007/s11738-013-1239-4
Hamdani, S., Yaakoubi, H., and Carpentier, R. (2011). Polyamines interaction with thylakoid proteins during stress. J. Photochem. Photobiol. B Biol. 104, 314–319. doi: 10.1016/j.jphotobiol.2011.02.007
He, Z., Ruan, C., Quin, P., Seliskar, D. M., and Gallagher, J. L. (2003). Kosteletzkya virginica, a halophytic species with potential for agroecotechnology in Jiangsu Province. China. Ecol. Eng. 21, 271–276. doi: 10.1016/j.ecoleng.2004.01.001
Hu, X., Zhang, Y., Shi, Y., Zhang, Z., Zou, Z., Zhang, H., et al. (2012). Effect of exogenous spermidine on polyamine content and metabolism in tomato exposed to salinity-alkalinity mixed stress. Plant Physiol. Biochem. 57, 200–209. doi: 10.1016/j.plaphy.2012.05.015
Hurst, A. C., Grams, T. E. E., and Ratajczak, R. (2004). Effects of salinity, high irradiance, ozone, and ethylene on mode of photosynthesis: oxidative stress and oxidative damage in the C3/CAM intermediate plant Mesembryanthemum crystallinum L. Plant Cell Environ. 27, 187–197. doi: 10.1046/j.0016-8025.2003.01133.x
Hussain, S. S., Ali, M., Ahmad, M., and Siddique, K. H. (2011). Polyamines: natural and engineered abiotic and biotic stress tolerance in plants. Biotechnol. Adv. 29, 300–311. doi: 10.1016/j.biotechadv.2011.01.003
Ioannidis, N. E., and Kotzabasis, K. (2007). Effects of polyamines on the functionality of photosynthetic membrane in vivo and in vitro. Biochem. Biophys. Acta 1767, 1372–1382. doi: 10.1016/j.bbabio.2007.10.002
Jiang, C., Belfield, E. J., Cao, Y., Smith, J. A., and Harberd, N. P. (2013). An Arabidopsis soil-salinity-tolerance mutation confers ethylene-mediated enhancement of sodium/potassium homeostasis. Plant Cell 25, 3535–3552. doi: 10.1105/tpc.113.115659
Jordan, T. E., Muñoz, N., Hein, M., Lowenstein, T., Godfrey, I., and Yu, J. (2002). Active faulting and folding without topographic expression in an evaporative basin. Chile. Geol. Oceanogr. Am. Bull. 114, 1406–1421. doi: 10.1130/0016-7606(2002)114<1406:AFAFWT>2.0.CO;2
Kotakis, C., Theodoropoulou, E., Tassis, K., Oustamanolakis, C., Ioannidis, N. E., and Kotzabasis, K. (2014). Putrescine, a fast-acting switch for tolerance against osmotic stress. J. Plant Physiol. 171, 48–51. doi: 10.1016/j.jplph.2013.09.015
Kumari, A., Das, P., Parida, A. K., and Agarwal, P. K. (2015). Proteomics, metabolomics, and ionomics perspectives of salinity tolerance in halophytes. Front. Plant Sci. 6:537. doi: 10.3389/fpls.2015.00537
Kusano, T., Berberich, T., Tateda, C., and Takahashi, Y. (2008). Polyamines: essential factors for growth and survival. Planta 228, 367–381. doi: 10.1007/s00425-008-0772-7
Kuznetsov, V., Shorina, M., Aronova, E., Stetsenko, L., Rakitin, V., and Shevyakova, N. (2007). NaCl- and ethylene-dependent cadaverine accumulation and its possible protective role in the adaptation of the common ice plant to salt stress. Plant Sci. 172, 363–370. doi: 10.1016/j.plantsci.2006.09.012
Lefèvre, I., Marchal, G., Ghanem, M. E., Correal, E., and Lutts, S. (2010). Cadmium has contrasting effects on polyethylene glycol-sensitive and resistant cell lines in the Mediterranean halophyte species Atriplex halimus L. J. Plant Physiol. 167, 365–374. doi: 10.1016/j.jplph.2009.09.019
Lefèvre, I., Marchal, G., Meerts, P., Corréal, E., and Lutts, S. (2009). Chloride salinity reduces cadmium accumulation by the Mediterranean halophyte species Atriplex halimus L. Environ. Exp. Bot. 65, 142–152. doi: 10.1016/j.envexpbot.2008.07.005
Li, B., He, W. X., Wang, C. Q., Li, S., and Xu, Q. (2013). Effects of cadmium stress on ammonium assimilation enzymes and polyamine in the roots of rice (Oryza sativa) with different cadmium resistance. Int. J. Appl. Environ. Sci. 17, 2203–2214.
Liu, J. H., Wei, W., Wu, H., Gong, X., and Moriguchi, T. (2015). Polyamines function in stress tolerance: from synthesis to regulation. Front. Plant Sci. 6:827. doi: 10.3389/fpls.2015.00827
Liu, T., Dobashi, H., Kim, D. W., Sagor, G. H. M., Niitsu, M., Berberich, T., et al. (2014). Arabidopsis mutant plants with diverse defects in polyamine metabolism show unequal sensitivity to exogenous cadaverine probably based on their spermine content. Physiol. Mol. Biol. Plants 20, 151–159. doi: 10.1007/s12298-014-0227-5
Llanes, A., Reginato, M., Devinar, G., and Luna, V. (2018). What is known about phytohormones in halophytes? A review. Biologia 73, 727–742. doi: 10.2478/s11756-018-0093-7
Lutts, S., Hausman, J. F., Quinet, M., and Lefèvre, I. (2013). “Polyamines and their roles in the alleviation of ion toxicities in plants,” in Ecophysiology and Responses of Plants Under Salt Stress, eds P. Ahmad, M. M. Azooz, and M. N. V. Prasad (New York, NY: Springer-Verlag), 315–353.
Lutts, S., and Lefèvre, I. (2015). How can we take advantage of halophyte properties to cope with heavy metal toxicity in salt-affected areas? Ann. Bot. 115, 509–528. doi: 10.1093/aob/mcu264
Lutts, S., Lefèvre, I., Delpérée, C., Kivits, S., Dechamps, C., Robledo, A., et al. (2004). Heavy metal accumulation by the halophyte species Mediterranean saltbush. J. Environ. Qual. 33, 1271–1279. doi: 10.2134/jeq2004.1271
Malliarakis, D., Tsiavos, T., Ionnadis, N. E., and Kotzabasis, K. (2015). Spermine and lutein quench chlorophyll fluorescence in isolated PSII antenna complexes. J. Plant Physiol. 183, 108–113. doi: 10.1016/j.jplph.2015.06.006
Manousaki, E., and Kalogerakis, N. (2009). Phytoextraction of Pb and Cd by the Mediterranean saltbush (Atriplex halimus L.): metal uptake in relation to salinity. Environ. Sci. Pollut. Res. Int. 16, 844–854. doi: 10.1007/s11356-009-0224
Marián, F. D., García-Jiménez, P., and Robaina, R. R. (2000). Polyamine levels in the seagrass Cymodocea nodosa. Aquat. Bot. 68, 179–184. doi: 10.1016/S0304-3770(00)00111-X
Martínez, E. A., Veas, E., Jorquera, C., San Martín, R., and Jara, P. (2009). Re-introduction of Chenopodium quinoa Willd. into arid Chile: cultivation of two lowland races under extremely low irrigation. J. Agron. Crop. Sci. 195, 1–10. doi: 10.1111/j.1439-037X.2008.00332.x
Martínez, J. P., Kinet, J. M., Bajji, M., and Lutts, S. (2005). NaCl alleviates polyethylene glycol-induced water stress in the halophyte species Atriplex halimus L. J. Exp. Bot. 56, 2421–2431. doi: 10.1093/jxb/eri235
Munns, R., and Tester, M. (2008). Mechanisms of salinity tolerance. Annu. Rev. Plant Biol. 59, 651–681. doi: 10.1146/annurev.arplant.59.032607.092911
Orsini, F., Accorsi, M., Gianquinto, G., Dinelli, G., Antognoni, F., Ruiz Carrasco, K. B., et al. (2011). Beyond the ionic and osmotic response to salinity in Chenopodium quinoa: functional elements of successful halophytism. Funct. Plant Biol. 38, 818–831. doi: 10.1071/FP11088
Ozgur, R., Uzilday, B., Sekmen, A. H., and Turkan, I. (2013). Reactive oxygen species regulation and antioxidant defence in halophytes. Funct. Plant Biol. 40, 832–847. doi: 10.1071/FP12389
Pál, M., Szalai, G., and Janda, T. (2015). Speculation: polyamines are important in abiotic stress signaling. Plant Sci. 237, 16–23. doi: 10.1016/j.plantsci.2015.05.003
Panta, S., Flowers, T., Lane, P., Doyle, R., Haros, G., and Shabala, S. (2014). Halophyte agriculture: success stories. Environ. Exp. Bot. 107, 71–83. doi: 10.1016/j.envexpbot.2014.05.006
Parvin, S., Lee, O. R., Sathiyaraj, G., Khorolragchaa, A., Kim, Y. J., and Yang, D. C. (2014). Spermidine alleviates the growth of saline-stressed ginseng seedlings through antioxidative defense system. Gene 537, 70–78. doi: 10.1016/j.gene.2013.12.021
Pathak, M. R., Teixeira, J. A., and Wani, S. H. (2014). Polyamines in response to abiotic stress tolerance through transgenic approaches. GM Crops Food 5, 87–96. doi: 10.4161/gmcr.28774
Ping Lee, Y., Funk, C., Erban, A., Kopka, J., Köhl, K. I., Zuther, K., et al. (2016). Salt stress responses in a geographycally diverse collection of Eutrema/Thellungiella spp. accessions. Funct. Plant Biol. 43, 590–606. doi: 10.1071/FP15285
Pottosin, I., and Shabala, S. (2014). Polyamines control of cation transport across plant membranes: implications for ion homeostasis and abiotic stress signaling. Front. Plant Sci. 5:154. doi: 10.3389/fpls.2014.00154
Pottosin, I., Velarde-Buendía, A. M., Bose, J., Fuglsang, A. T., and Shabala, S. (2014). Polyamines cause plasma membrane depolarization, activate Ca+2-, and modulate H+-ATPase pump activity in pea roots. J. Exp. Bot. 65, 2463–2472. doi: 10.1093/jxb/eru133
Radyukina, N. L., Ivanov, Y. V., Mapelli, S., Mikheeva, L. E., and Karbysheva, E. A. (2017). Effects of medium-wave ultraviolet radiation on levels and spectrum of polyamines in leaves and roots of wild-growing plants. Moscow University Biol. Sci. Bull. 72, 155–158. doi: 10.3103/S0096392517030087
Radyukina, N. L., Kartashov, A. V., Ivanov, Y. V., Shevyakova, N. I., and Kuznetsov, V. Vl (2007). Functioning of defense systems in halophytes and glycophytes under progressing salinity. Russ. J. Plant Physiol. 54, 806–815. doi: 10.1134/S1021443707060131
Reginato, M. A. (2009). Response of the Halophyte Prosopis Strombulifera to Different Saline Media. Modifications of the Morphophysiological Parameters and their Hormonal Regulation. Master’s thesis, University of Córdoba, Argentina.
Reginato, M. A., Abdalla, G. I., Miersch, O., Ruiz, O. A., Moschetti, E., and Luna, V. (2012). Changes in the levels of jasmonates and free polyamines induced by Na2SO4 and NaCl in roots and leaves of the halophyte Prosopis strombulifera. Biologia 67, 689–697. doi: 10.2478/s11756-012-0052-7
Repo-Carrasco, R., Espinoza, C., and Jacobsen, S. E. (2003). Nutritional value and use of the Andean crops quinoa (Chenopodium quinoa) and kañiwa (Chenopodium pallidicaule). Food Rev. Int. 19, 179–189. doi: 10.1081/FRI-120018884
Ruiz, K. B., Rapparini, F., Bertazza, G., Silva, H., Torrigiani, P., and Biondi, S. (2017). Comparing salt-induced responses at the transcript level in a salares and coastal-lowlands landrace of quinoa (Chenopodium quinoa Willd). Environ. Exp. Bot. 139, 127–142. doi: 10.1016/j.envexpbot.2017.05.003
Ruiz-Carrasco, K., Antognoni, F., Coulibaly, A. K., Lizardi, S., Covarrubias, A., Martínez, E., et al. (2011). Variation in salinity tolerance of four lowland genotypes of quinoa (Chenopodium quinoa Willd.) as assessed by growth, physiological traits, and sodium transporter gene expression. Plant Physiol. Biochem. 49, 1333–1341. doi: 10.1016/j.plaphy.2011.08.005
Shabala, S., and Mackay, A. (2011). Ion transport in halophytes. Adv. Bot. Res. 57, 151–199. doi: 10.1016/B978-0-12-387692-8.00005-9
Shevyakova, N. I., Eshinimaeva, B. T., and Kuznetsov, V. Vl (2011). Expression of ferritin gene in Mesembryanthemum crystallinum plants under different supply with iron and different intensity of oxidative stress. Russ. J. Plant Physiol. 58, 768–775. doi: 10.1134/S1021443711050219
Shevyakova, N. I., Musatenko, L. I., Stetsenko, L. A., Rakitin, V. Y., Vedenicheva, N. P., and Kuznetsov, V. Vl (2013). Effect of ABA on the contents of proline, polyamines, and cytokinins in the common ice plants under salt stress. Russ. J. Plant Physiol. 60, 741–748. doi: 10.1134/S1021443713060125
Shevyakova, N. I., Rakitin, V. Y., Stetsenko, L. A., Aronova, E. E., and Kuznetsov, V. Vl (2006a). Oxidative stress and fluctuations of free and conjugated polyamines in the halophyte Mesembryanthemum crystallinum L. under NaCl salinity. Plant Growth Regul. 50, 69–78. doi: 10.1007/s10725-006-9127-1
Shevyakova, N. I., Shorina, M. V., Rakitin, V. Y., and Kuznetsov, V. Vl (2006b). Stress-dependent accumulation of spermidine and spermine in the halophyte Mesembryanthemum crystallinum under salinity conditions. Russ. J. Plant Physiol. 53, 739–745. doi: 10.1134/S1021443706060021
Shi, H., and Chan, Z. (2014). Improvement of plant abiotic stress tolerance through modulation of the polyamine pathway. J. Integr. Plant Biol. 56, 114–121. doi: 10.1111/jipb.12128
Simon-Sarkadi, L., Kocsy, G., Sebestyén, Z., and Galiba, G. (2007). Deletions of chromosome 5A affect free amino acid and polyamine levels in wheat subjected to salt stress. Environ. Exp. Bot. 60, 193–201. doi: 10.1016/j.envexpbot.2006.10.002
Slama, I., Abdelly, C., Bouchereau, A., Flowers, T., and Savouré, A. (2015). Diversity, distribution and roles of osmoprotective compounds accumulated in halophytes under abiotic stress. Ann. Bot. 115, 433–447. doi: 10.1093/aob/mcu239
Stetsenko, L. A., Rakitin, V. Y., Shevyakova, N. I., and Kuznetsov, V. Vl (2009). Organ-specific changes in the content of free and conjugated polyamines in Mesembryanthemum crystallinum plants under salinity. Russ. J. Plant Physiol. 56, 808–813. doi: 10.1134/S1021443709060107
Sudhakar, C., Veeranagamallaiah, G., Nareshkumlar, A., Sundhakarbabu, O., Sivakumar, M., Pandurangaiah, M., et al. (2015). Polyamine metabolism influences antioxidant defense mechanisms in foxtail millet (Setaria italica L.) cultivars with different salinity tolerance. Plant Cell Rep. 34, 141–156. doi: 10.1007/s00299-014-1695-3
Surówka, E., Dziurka, M., Kocurek, M., Goraj, S., Rapacz, M., and Miszalski, Z. (2016). Effects of exogenously applied hydrogen peroxide on antioxidant and osmoprotectant profiles and the C3-CAM shift in the halophyte Mesembryanthemum crystallinum L. J. Plant Physiol. 200, 102–110. doi: 10.1016/j.jplph.2016.05.021
Tiburcio, A. F., Altabella, T., Bitrián, M., and Alcázar, R. (2014). The roles of polyamines during the lifespan of plants: from development to stress. Planta 240, 1–18. doi: 10.1007/s00425-014-2055-9
Uzilday, B., Ozgur, R., Sekmen, A. H., Yildiztugay, E., and Turkan, I. (2015). Changes in the alternative electron sinks and antioxidant defence in chloroplasts of the extreme halophyte Eutrema parvulum (Thellungiella parvula) under salinity. Ann. Bot. 115, 449–463. doi: 10.1093/aob/mcu184
Vromman, D., Flores-Bavestrello, A., Šlejkovec, Z., Lapaille, S., Teixeira-Cardoso, C., Briceño, M., et al. (2011). Arsenic accumulation and distribution in relation to young seedling growth in Atriplex atacamensis Phil. Sci. Total Environ. 412–413, 286–295. doi: 10.1016/j.scitotenv.2011.09.085
Winter, K., and Holtum, J. A. M. (2007). Environment or development? Lifetime net CO2 exchange and control of the expression of crassulacean acid metabolism in Mesembryanthemum crystallinum. Plant Physiol. 143, 98–107. doi: 10.1104/pp.106.088922
Yang, L., Zu, Y. G., and Tang, Z. H. (2013). Ethylene improves Arabidopsis salt tolerance mainly via retaining K+ in shoots and roots rather than decreasing tissue Na+ content. Environ. Exp. Bot. 86, 60–69. doi: 10.1016/j.envexpbot.2010.08.006
Zarranz Elso, M., García-Jiménez, P., and Robaina, R. R. (2012). Endogenous polyamine content and photosynthetic performance under hypo-osmotic conditions reveal Cymodocea nodosa as an obligate halophyte. Aquat. Biol. 17, 7–17. doi: 10.3354/ab00454
Zhou, C., Sun, Y., Ma, Z., and Wang, J. (2015). Heterologous expression of EsSPDS1 in tobacco plants improves drought tolerance with efficient reactive oxygen species scavenging systems. South Afr. J. Bot. 96, 19–28. doi: 10.1016/j.sajb.2014.10.008
Zhou, M. X., Dailly, H., Renard, M. E., Han, R. M., and Lutts, S. (2018a). NaCl impact on Kosteletzkya pentacarpos seedlings simultaneously exposed to cadmium and zinc toxicities. Environ. Sci. Pollut. Res. Int. 25, 17444–17456. doi: 10.1007/s11356-018-1865-x
Zhou, M. X., Han, R., Ghnaya, T., and Lutts, S. (2018b). Salinity influences the interactive effects of cadmium and zinc on ethylene and polyamine synthesis in the halophyte plant species Kosteletzkya pentacarpos. Chemosphere 209, 892–900. doi: 10.1016/j.chemosphere.2018.06.143
Keywords: antioxidant system, extremophiles, ion sequestration, saline markers, wetlands species, xerohalophytes
Citation: Bueno M and Cordovilla M-P (2019) Polyamines in Halophytes. Front. Plant Sci. 10:439. doi: 10.3389/fpls.2019.00439
Received: 26 December 2018; Accepted: 22 March 2019;
Published: 09 April 2019.
Edited by:
Rubén Alcázar, University of Barcelona, SpainReviewed by:
Stefania Biondi, University of Bologna, ItalyCopyright © 2019 Bueno and Cordovilla. This is an open-access article distributed under the terms of the Creative Commons Attribution License (CC BY). The use, distribution or reproduction in other forums is permitted, provided the original author(s) and the copyright owner(s) are credited and that the original publication in this journal is cited, in accordance with accepted academic practice. No use, distribution or reproduction is permitted which does not comply with these terms.
*Correspondence: Milagros Bueno, bWJ1ZW5vQHVqYWVuLmVz
Disclaimer: All claims expressed in this article are solely those of the authors and do not necessarily represent those of their affiliated organizations, or those of the publisher, the editors and the reviewers. Any product that may be evaluated in this article or claim that may be made by its manufacturer is not guaranteed or endorsed by the publisher.
Research integrity at Frontiers
Learn more about the work of our research integrity team to safeguard the quality of each article we publish.