- The National Field Station of Freshwater Ecosystem of Liangzi Lake, College of Life Sciences, Wuhan University, Wuhan, China
The strategy of producing rapid initial growth and establishing early in the growing season is important, and it is employed by invasive macrophytes. Elodea nuttallii and Egeria densa, two Hydrocharitaceae species, became weeds after invading many countries in recent years. Comparative studies on their invasive traits in relation to native species during winter and spring are limited. In the present study, we compared the growth performance of these two exotic species with a perennial native species, Potamogeton maackianus, in different water depths (1, 2, and 3 m) during winter (January and February) and spring (March and April). Three morphological traits (shoot number, root number and shoot length), total biomass, relative growth rate (RGR) and two physiological photosynthetic traits (total chlorophyll content and the maximum quantum yield of PSII [Fv/Fm]) were measured for each macrophyte. All three species could overwinter as entirely leafy plants. Biomass, RGR, morphological traits and physiological traits were all different among species. However, water depths had a significant effect only on morphological traits. At all water depths, E. nuttallii had significantly higher values for morphological traits, total biomass and RGR than P. maackianus, while E. densa had significantly fewer roots and a lower total chlorophyll content than P. maackianus. Except for Fv/Fm at a 3 m water depth, morphological and physiological photosynthetic traits, biomass and RGR of E. nuttallii were significantly higher than those of E. densa. In addition, a large number of adventitious roots developed from E. nuttallii but not from the other two species. These results indicate that the advantages of E. nuttallii to grow in winter and spring may make it more prone to expansion than E. densa in China.
Introduction
Currently, with the process of economic globalization and trade liberalization, many species are imported in places that are out of their indigenous geographical ranges, and some of them develop into invasive species (Richardson et al., 2000). With its negative effects, biological invasion of exotic species has become a universal ecological problem (Mack et al., 2000). Compared to controlling outbreaks of invaders, prevention is more feasible and economical (Waage and Reaser, 2001). Therefore, it is important to predict the potential invasiveness of species (Rejmánek and Richardson, 1996; Barrat-segretain et al., 2002), which can help us set priorities for the control of introduced invasive species (Rejmánek and Richardson, 1996).
In freshwater ecosystems, invasive plants are alien species that possess advantages for proliferation (produce large numbers of sexual and/or vegetative propagules), dispersal (multiple means and long-distance dispersal) and rapid colonization (Richardson et al., 2000; Kercher and Zedler, 2004). Numerous studies have considered invasive aquatic plants with regards to their growth, regeneration capacity, photosynthesis traits, genetic, reproductive, overwintering strategies and management (Lui et al., 2005; Hussner, 2009; Zhang et al., 2010; Wersal and Madsen, 2011; You et al., 2014; Liu et al., 2016; Hussner et al., 2017). Water depth could affect morphological traits, photosynthetic traits, reproduction and distribution of native or exotic aquatic macrophytes (Chambers and Kaiff, 1985; Strand and Weisner, 2001; Xiao et al., 2010; Fan et al., 2015; Liu et al., 2016; Xu et al., 2016; Han et al., 2018; Li et al., 2018; Zhao et al., 2018). On the other hand, overwintering is important for the invasion of exotic species. The strategy of invasive macrophytes to produce rapid initial growth and establish early in the growing season may increase their competitive ability and allow them to successfully replace native species (Nichols and Shaw, 1986). In addition, the response of submerged macrophytes to the environment might differ depending on the timing of environmental changes and the growth phases of plants (Cao et al., 2015). However, the influence of water depth on the growth of invasive submerged species during the early growth season has not been well elucidated.
Elodea nuttallii (Planch.) H. St John is a submerged macrophyte and is native to temperate North America (Cook and Urmi-König, 1985). It has been defined as an invasive species and is now receiving increasing attention for its rapid and lasting invasion of many freshwater habitats throughout Europe, Asia and Australia (Kunii, 1981, 1982, 1984; Barrat-segretain et al., 2002; Zehnsdorf et al., 2015). Another submerged macrophyte, Egeria densa (Planch.), which is native of South America, became a weed after it was introduced to North America, Europe and New Zealand (Cook and Urmi-König, 1984; Champion and Tanner, 2000; Gassmann et al., 2006). It can form monodominant and dense stands which have negative effects on native water ecosystems (Yarrow et al., 2009; Fujiwara et al., 2016). E. nuttallii and E. densa are both species that can tolerate and survive cold temperature, even surviving under ice cover (Kunii, 1984; Yarrow et al., 2009). Moreover, both species can tolerate a wide range of water depths (Vöge, 1994; Carrillo et al., 2006). E. nuttallii was introduced to China approximately 30 years ago (Xu et al., 2007). Previous studies considered the influence of E. nuttallii on the native ecosystems of China (Zhang et al., 1999) and found that this species had begun to invade the local aquatic community of the East Taihu Lake (Gu et al., 2005) and expanded in other sites in recent years (Yu et al., 2018). E. densa was introduced in China as an ornamental plant in recent years, but it has escaped and naturalized in fields, with a limited scope (Yu et al., 2018).
The objective of this study was to compare the growth under various water depths (1, 2, and 3 m) of these two species during winter and spring to that of native species Potamogeton maackianus, which is a primary submerged macrophyte in the Yangtze Plain of China that can grow in the winter (Ni, 2001; Su et al., 2019). We hypothesized that (1) not both of exotic species may have advantages in growth traits over native species and (2) water depths would influence the growth of all three macrophytes during winter and spring, and the effects of water depths were expected to be different for native and exotic species.
Materials and Methods
Plant Materials and Study Sites
This study was conducted at The National Field Station of Freshwater Ecosystem of Liangzi Lake, Wuhan University, China (30°5′–30°18′N, 114°21′–114°39′E). In early November 2013, all samples of two exotic species (E. nuttallii and E. densa) and one native species, P. maackianus were collected from the field station. We chose 37 apical shoots of each plant for the experiment, which were collected from parent individuals with an equal length of 15 cm. For each species, we recorded the fresh weight of all shoots, and we used the fresh and dry (after drying at 70°C for 72 h) weights of 16 apical shoots for the transformation. The mean dry weights of the shoots of E. nuttallii, E. densa, and P. maackianus were 0.024 ± 0.001, 0.116 ± 0.009, and 0.066 ± 0.005 g (mean ± SD), respectively.
Experimental Design
We set three water depths (1, 2, and 3 m) in this experiment because the actual mean water depth is 3.0 m in Liangzi Lake (Xu et al., 2018). The experiment was conducted in outdoor cement pools (4 m × 4 m × 4 m; length × width × height) filled with lake water [TN 0.71 ± 0.01 mg L-1,TP 0.04 ± 0.01 mg L-1, measured with a YSI Professional Plus water quality meter (YSI Inc., Yellow Springs, OH, United States)]. Seven cement pools were used and one pool represented a replicate. We placed three steel tubes (>4 m; length) on each cement pool at the same intervals and hung three rubber buckets (25 cm × 15 cm; diameter × depth) on each steel tube with the same intervals (Figure 1). One apical shoot was planted in a rubber bucket filled with homogeneous mud sediment [TN 0.74 mg g-1, TP 0.03 mg g-1, measured with IL500 Automatic Analyzer (Hach Corp., Loveland, CO, United States)] from Liangzi Lake and placed at the water level of 0.5 m for a month to root and acclimatize. A month later, we used ropes to control three water depths (1, 2, and 3 m) (Figure 1).
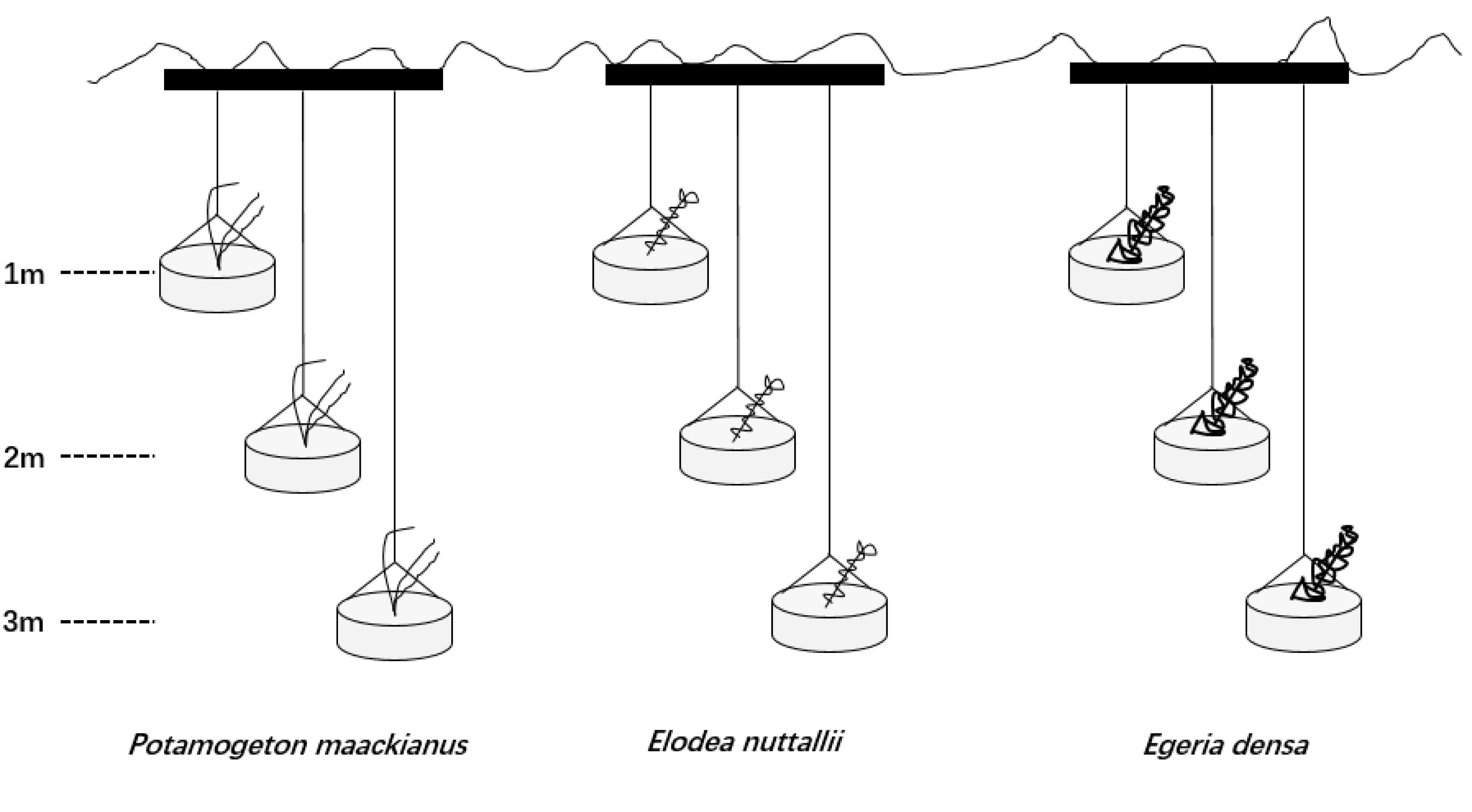
Figure 1. Design of the experiments showing three of the 9 containers in which the three species were planted.
A total of 21 individuals of each species were used. During the experiment, lake water was added to cement pools twice a week to compensate for evaporation loss. The water temperature and light intensity were recorded twice a week, and the average daily temperature of each month from January to April was 7.22 ± 0.5, 7.12 ± 1.96, 10.57 ± 1.9, and 16.74 ± 2.9°C (mean ± 1SE); photosynthetically active radiation (PAR) at noon at different water depths (1, 2, and 3 m) was 108.6 ± 90.1, 65.8 ± 57.0, and 14.8 ± 13.4 μmol m-2 s-1, respectively (means ± 1SE). The experiment lasted 5 months (from 22 November 2013 to 22 April 2014, including a month for acclimation).
Trait Measurement
The shoot number, root number (rooted in sediment), shoot length and two physiological photosynthetic traits (total chlorophyll content, maximum quantum yield of PSII [Fv/Fm]) were measured for each macrophyte. Adventitious roots (water roots) number of E. nuttallii were also recorded. All biomass was obtained after drying plants in an oven at 70°C for 72 h. Relative growth rate (RGR) was calculated as RGR = (lnw2 – lnw1)/(t2 – t1), where w1 and w2 stand for initial and final dry weight, respectively, and (t2 – t1) represents the experimental duration of each species from 22 November 2013 to 22 April 2014. A portion of 0.1 g of leaves (fresh weight) obtained from each plant was used for the measurement of chlorophyll content. Plant material was ground to a fine powder, and then extracted with 95% ethanol. The total chlorophyll content (the summed values of chlorophyll a and b) was determined by spectrophotometer, according to Lichtenthaler (1987). Chlorophyll fluorescence on the fully developed, healthy leaves from the top was measured after harvesting. Before measurements, leaves were covered for at least 20 min to allow for dark adaptation, then the maximum (Fm) and the minimum (Fo) fluorescence yields were measured with the saturation pulse method (Schreiber et al., 2000), with a portable chlorophyll fluorometer (DIVING-PAM, Walz, Effeltrich, Germany). Variable fluorescence (Fv) was calculated as Fm–Fo; consequently, the maximum quantum yield of PSII (Fv/Fm) was obtained.
Statistical Analysis
Differences in growth traits were analyzed first by analysis of covariance (ANCOVA) with initial biomass as the covariate. Initial biomass did not significantly affect any growth traits (all P > 0.05). Then, two-way ANOVA with water depths and species as the main factors was performed to determine the main effects and interactive effects on all growth traits. If a significant treatment effect was detected, post hoc pairwise comparisons of means were made to examine differences between treatments using studentized Tukey’s HSD for multiple comparisons. One-way ANOVA was performed to determine the effects of water depth on the water root number of E. nuttallii. Data were log-transformed (shoot and root number, total biomass and RGR) or square root-transformed (shoot length) to ensure normality of residuals and homogeneity of variances, and homogeneity was tested using Levene’s test. All data analyses were conducted using SPSS 18.0 (SPSS, Chicago, IL, United States).
Results
All morphological traits were significantly different between species and water treatments (Table 1). All morphological traits (shoot number, shoot length and root number) of E. nuttallii were significantly higher than those of E. densa and P. maackianus at all water depths (Figures 2A–C). The shoot number of P. maackianus at 2 and 3 m depths and the shoot length of P. maackianus at the 2 m depth were significantly higher than those of E. densa (Figures 2A,B). The root number of E. densa was significantly lower than that of P. maackianus at all depths (Figure 2C). With increasing water depth, the shoot number and shoot length of E. nuttallii decreased and increased, respectively, while depth had no significant effect on the shoot traits of E. densa and P. maackianus (Figures 2A,B). Water depth had no significant effect on the root number of E. nuttallii and E. densa, while the root number of P. maackianus at the 3 m depth was significantly lower than that of plants at the 1 and 2 m depths (Figure 2C). Depth had no significant effect on the water root number (F = 0.192, P = 0.827) of E. nuttallii, and the mean number of water roots was 54.71 ± 9.85 per plant among all three water depths.
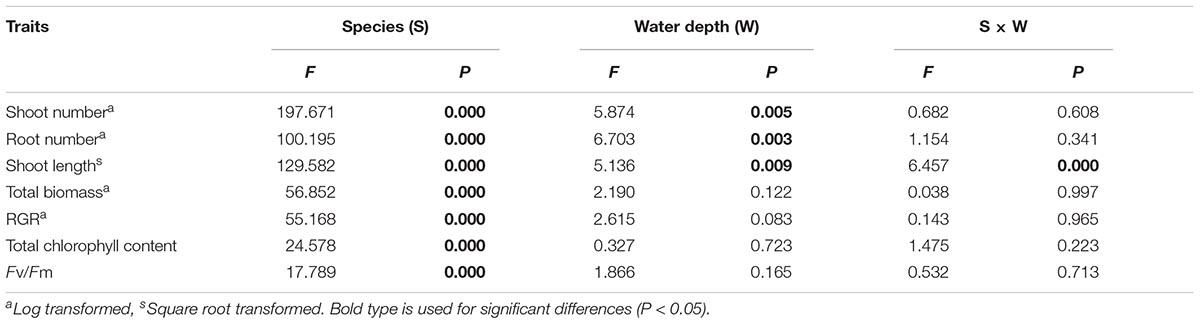
Table 1. F values and P values for species and water depth treatments for shoot number, root number, shoot length, total biomass, RGR, total chlorophyll content and Fv/Fm by two-way ANOVA (n = 7).
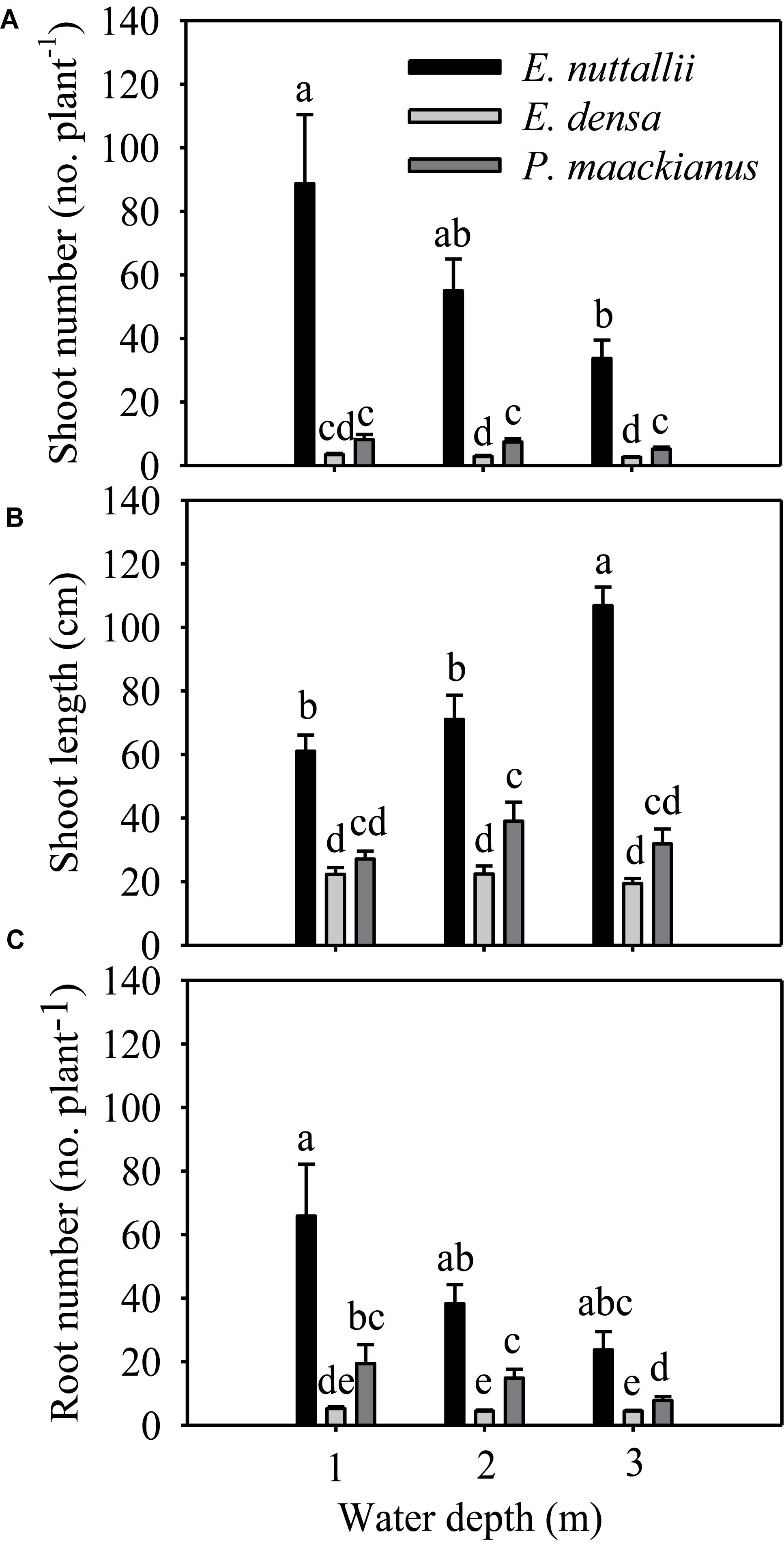
Figure 2. Changes in the morphological traits (shoot number A, shoot length B, and root number C) of the three macrophytes, data are presented as the means ± 1SE (n = 7). Means with different small letters are significantly different at P < 0.05 among different treatments.
Total biomass and RGR were significantly different among species but not among different water depths (Table 1). The total biomass and RGR of E. nuttallii were significantly higher than those of E. densa and P. maackianus at all water depths (Figures 3A,B). There were no significant differences in total biomass and RGR between E. densa and P. maackianus (Figures 3A,B).
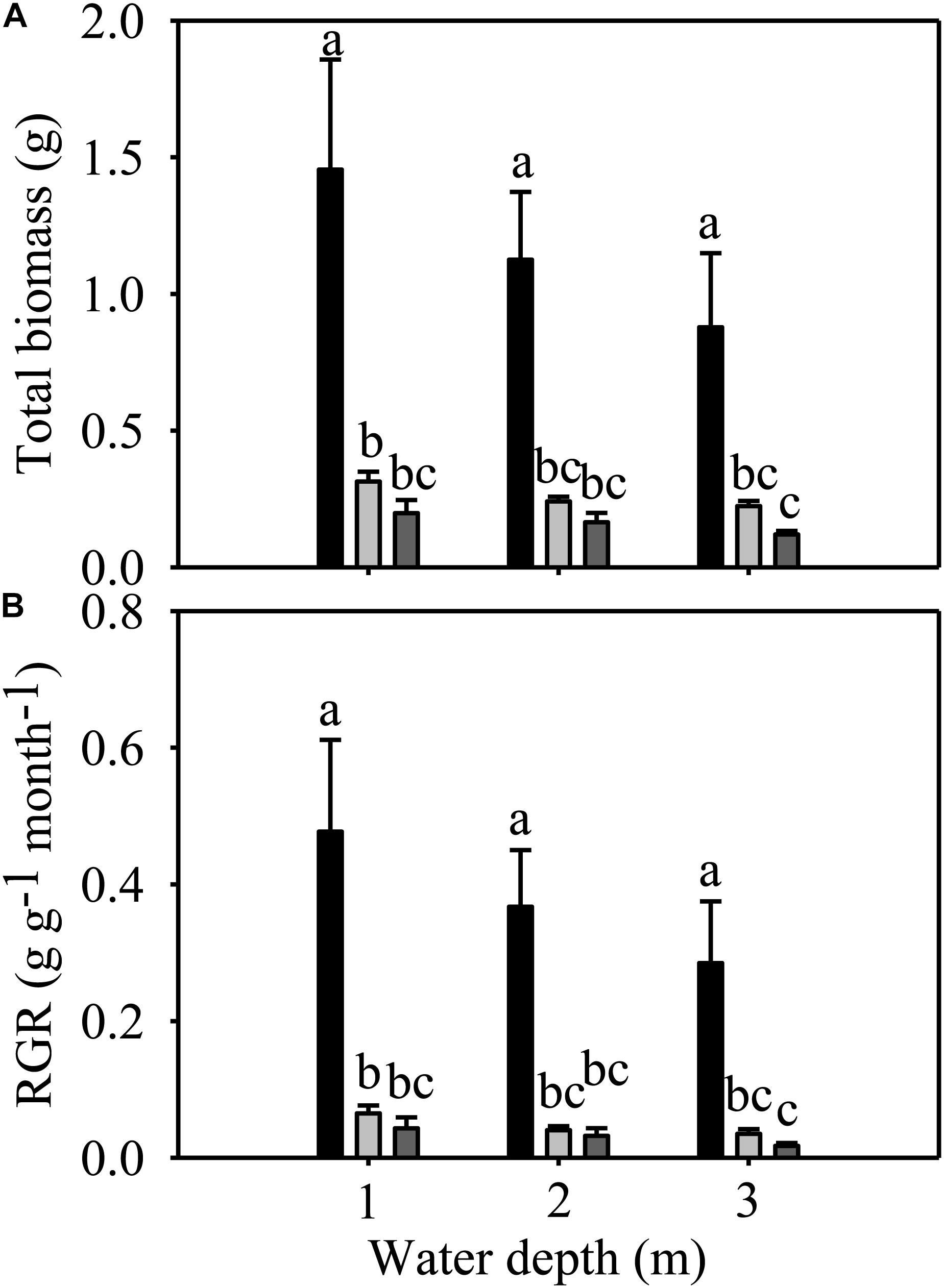
Figure 3. Changes in the total biomass (A) and RGR (B) of three macrophytes. The data are presented as the mean ± 1SE (n = 7). Means with different small letters are significantly different at P < 0.05 among different treatments.
Total Chl and Fv/Fm were significantly different among species but not among different water depth treatments (Table 1). At all three water depths, the total Chl of E. nuttallii was not significantly different from that of P. maackianus, while the total Chl of E. densa was significantly lower than that of the other two species (Figure 4A). At the 1 m depth, both Fv/Fm of E. nuttallii and E. densa were similar to P. maackianus, while Fv/Fm of E. nuttallii was significantly higher than that of E. densa (Figure 4B). At the 2 m depth, E. densa and P. maackianus had no difference in Fv/Fm, while both values were significantly lower than that of E. nuttallii (Figure 4B). The difference in Fv/Fm among the three species disappeared when plants were grown at a depth of 3 m (Figure 4B).
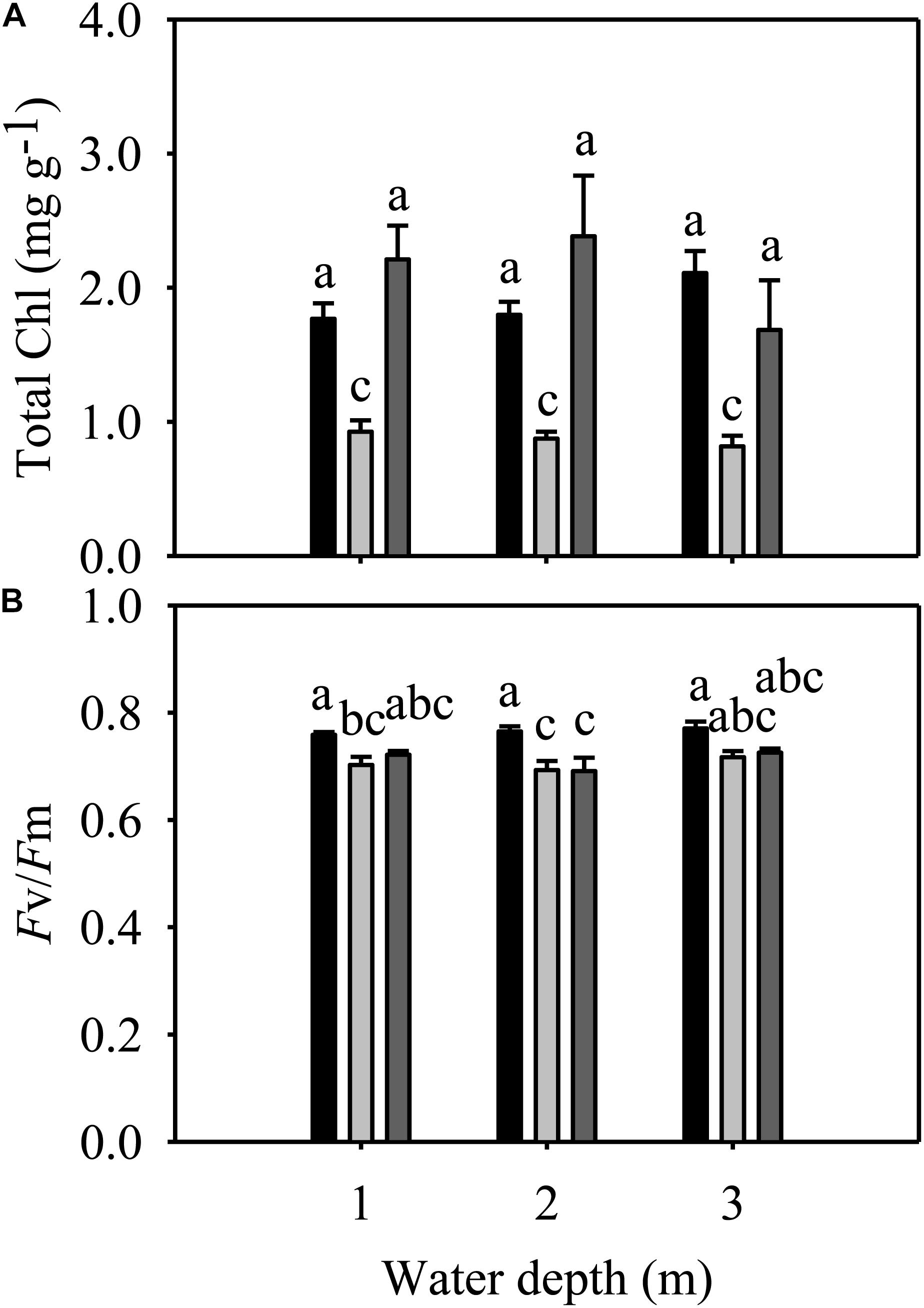
Figure 4. Changes in the total chlorophyll content (A) and Fv/Fm (B) of three macrophytes. The data presented are the mean ± 1SE (n = 7). Means with different small letters are significantly different at P < 0.05 among different treatments.
Discussion
Previous studies revealed that some exotic aquatic plants survival increased with increasing winter temperatures (Hussner and Lösch, 2005; You et al., 2014; Liu et al., 2016), which may be responsible for facilitating their invasion. Our results revealed that both exotic macrophytes could overwinter as entire plants. Compared with invasive species that cannot overwinter, overwintering ability may aid these species in invasion.
The shoot number, shoot length, root number, total biomass and RGR of E. nuttallii were significantly higher than those of E. densa and P. maackianus at all water depths (Figures 2A–C, 3A,B). This suggests that the recovery of E. nuttallii happens earlier than the other two species, consistent with the findings of E. nuttallii compared with E. canadensis (Ozimek et al., 1993). This may be due to the extension of the growth season of E. nuttallii, beginning in winter with the cumulative daily water temperatures over 4°C (Kunii, 1981); the critical temperature for its active growth lies between 8.2 and 12.0°C (Kunii, 1982), which is lower than the temperature for active growth of many other submerged plants (Ozimek et al., 1993). It was also found that E. nuttallii grows well and has a competitive advantage over native species at low water temperatures in Japan (Kunii, 1981, 1982, 1984). The strategy adopted by invasive macrophytes to produce rapid initial growth and establish early in the growing season is a much less conservative life strategy than that of the species that grow and reproduce until midsummer (Nichols and Shaw, 1986); thus, our results suggested that the early development of E. nuttallii might be another important means to promote its colonization. A similar phenomenon of the winter growth of E. nuttallii has been reported in its related species E. canadensis (Haag, 1979). E. densa did not show advanced winter growth, compared to E. nuttallii and the indigenous P. maackianus, in the present study. The winter growth of E. densa is slow and this species tends to store starch for subsequent spring growth (Yarrow et al., 2009). It can recover from winter senescence and quickly reinvade water bodies through energy stored in basal stems and the root crown (Pennington and Sytsma, 2009; Cabrera et al., 2013), but the optimal temperature range for its active growth is 14 to 25°C (Pennington, 2008). However, the water temperature was only up to 14°C in late April, which could explain why E. densa grew slowly and did not recover during the experimental period.
Once the invasion of exotic macrophytes has taken place, subsequent colony growth proceeds primarily by stem fragmentation (Hofstra et al., 1999), owing to clonal fragmentation being one of the essential means of duplication and dispersal of aquatic plants (Barrat-segretain, 1996). The three macrophytes in our study mainly reproduce vegetatively through clonal growth of stolons or fragments (Cook and Urmi-König, 1984; Li et al., 2004; Nagasaka, 2004). Therefore, the number of propagules could be reflected by their shoot number and shoot length. In our study, the shoot number and shoot length of E. nuttallii were significantly higher than that of P. maackianus, whereas the reverse was true for E. densa under most treatment conditions (Figures 2A,B), which might aid E. nuttallii and inhibit E. densa in occupying niches early in the spring. The number of invaders (propagule pressure) is one of the traits that influence the ability of species to invade new communities (Lonsdale, 1999; Xie et al., 2013). Furthermore, E. nuttallii may produce a canopy shading out and inhibiting other species due to its shoot length (Barrat-segretain and Elger, 2004); a similar study suggested that E. nuttallii invests more in shoot elongation than E. canadensis and thus has a greater advantage for light capture (Szabó et al., 2019). We suggested that the earlier the start of the vegetative period, the higher the capability for vegetative reproduction, and a greater shoot length might contribute to E. nuttallii being more invasive than E. densa during winter and spring.
The roots of submerged plants could maintain many of the functions of terrestrial roots, such as the absorption and transportation of nutrients and water and the supply of shoots with anchorage and phytohormones (Agami and Waisel, 1986; Hussner et al., 2009). The root number of E. densa was significantly lower than that of the other two macrophytes in all treatments, while that of E. nuttallii was the highest among the three species at all water depths (Figure 2C). Since this species can absorb and accumulate large quantities of nitrogen through its roots (Ozimek et al., 1993), the greater amount of roots of E. nuttallii may result in an advantage in nitrogen absorption over the other two species. A previous study also found that another invasive species, E. canadensis, allocated more biomass to roots during adverse environmental conditions (heat wave) to absorb more nutrients (Cao et al., 2015). Furthermore, adventitious roots were of greater importance in P-storage under high resource availabilities (Garbey et al., 2004). The development of adventitious roots may aid the survival through the uptake of water column nutrients, which may be a mechanism for survival during adverse conditions, a means of long distance dispersal of fragments, or it may offer a competitive advantage over species that rely on sediment nutrients (Wersal and Madsen, 2011). Moreover, the development of adventitious roots may contribute to the ability to spread and colonize in local habitats or in new habitats once fragments occur, because shoots are very often broken and fragmented by animals and water flow (Barrat-segretain, 1996), in plants that possess higher survival rates (Barrat-segretain et al., 2002). Adventitious roots (water roots) developed from shoots of E. nuttallii in our experiment, as observed by previous studies, were located at nodes of lateral branches (Ozimek et al., 1993; Barrat-segretain, 2005). Therefore, the development of more roots and adventitious roots in E. nuttallii may contribute to its rapid growth and distribution.
Since the conditions of light and substrate availability change and waves may occur with increasing water depth (Duarte and Kalff, 1990), and light intensity underwater decreases significantly (Zhu et al., 2012), water depth has an important influence on the growth, reproduction and distribution of aquatic macrophytes (Chambers and Kaiff, 1985; Xiao et al., 2010; Zhu et al., 2012; Fan et al., 2015; Li et al., 2018; Zhao et al., 2018). Contrary to our hypothesis, our results showed that water depth had less influence on most traits of E. nuttallii and P. maackianus, except that deeper water (3 m) decreased the shoot number of E. nuttallii and root number of P. maackianus, and increased the shoot length of E. nuttallii. Similar results were reported for other species, also including P. maackianus (Strand and Weisner, 2001; Yang et al., 2004; Zhu et al., 2012; Fan et al., 2015). In addition, in this study, water depth had no influence on any traits of E. densa (Figures 2–4). Previous studies found that these three species could tolerate a wide range of water depths and adapt well under low light conditions (Barko and Smart, 1981; Vöge, 1994; Ni, 2001; Carrillo et al., 2006), which probably accounts for the indistinct differences in their growth among different water depths in our study. In addition, plant growth was not essentially sensitive to light intensity at cool temperatures (Rajan et al., 1973). The Fv/Fm ratio is normally within the range of 0.75–0.85, and the decline in this ratio indicates photoinhibitory damage caused by environmental stresses (Bolhár-Nordenkampf et al., 1989). For example, water depth (≥3 m) severely reduced the Fv/Fm of the submerged plants Vallisneria natans, indicating that flooding treatments imposed severe stress on the fitness of this species (Han et al., 2018), or shallow water decreased Fv/Fm of the submerged plants Ottelia acuminata because of the strong light (Zhao et al., 2018). However, water depth had no significant effects on the Fv/Fm of the three macrophytes in our study (Figure 4B). Similarly, there was no significant change in the total Chl content of the three species among different water depth treatments (Figure 4A). The maintenance of the photosynthetic pigment concentration (Chl concentration) explains the stability of Fv/Fm (Meneguelli-Souza et al., 2016). These results suggested that 2 m or 3 m water depths did not cause damage to these three macrophytes, because they can tolerate a wide range of water depths (Vöge, 1994; Carrillo et al., 2006; Zhu et al., 2012; Li et al., 2013). Contrary to our second hypothesis, the growth of these three macrophytes was less influenced by water depth.
Conclusion
All three species could overwinter as whole leafy plants. The growth and photosynthesis of the three species were less influenced by water depths. The results verified our first hypothesis that not both exotic species showed advantages over the native species. E. nuttallii presented superior advantages over others observed in its considerable growth and shoot increase (such as the larger RGR, more shoots and roots, and higher shoot length) in late winter and spring, which might help it to establish in its niche early and express its invasive traits, thus favoring its expansion and potential outbreak. By contrast, E. densa was disadvantaged compared with the other two macrophytes because of its weak growth and photosynthetic rate in winter and early spring.
Author Contributions
YW, DY, and CL designed the research and executed the research project. YW, XC, JL, YH and QH collected the field data. YW and CH analyzed the data and wrote the manuscript. HD revised the paper critically.
Funding
This work was supported by the Major Science and Technology Program for Water Pollution Control and Treatment (2015ZX07503-005) and the Special Foundation of National Science and Technology Basic Research (2013FY112300).
Conflict of Interest Statement
The authors declare that the research was conducted in the absence of any commercial or financial relationships that could be construed as a potential conflict of interest.
The handling Editor is currently organizing a Research Topic with one of the authors CL, and confirms the absence of any other collaboration.
Acknowledgments
The authors gratefully acknowledge the support Ligong Wang provided for the laboratory and field work, and the useful suggestions provided by Shufeng Fan, Chengxing Xia, Tong Wang, Xiaolong Huang, and Haihao Yu.
References
Agami, M., and Waisel, Y. (1986). The ecophysiology of roots of submerged vascular plants. Physiol. Végétale 24, 607–624.
Barko, J. W., and Smart, R. M. (1981). Comparative influences of light and temperature on the growth and metabolism of selected submersed freshwater macrophytes. Ecol. Monogr. 51, 219–235.
Barrat-segretain, M. H. (1996). Strategies of reproduction, dispersion, and competition in river plants: a review. Vegetatio 123, 13–37. doi: 10.1007/BF00044885
Barrat-segretain, M. H. (2005). Competition between invasive and indigenous species: impact of spatial pattern and developmental stage. Plant Ecol. 180, 153–160. doi: 10.1007/s11258-004-7374-7
Barrat-segretain, M. H., and Elger, A. (2004). Experiments on growth interactions between two invasive macrophyte species. J. Veg. Sci. 15, 109–114. doi: 10.1111/j.1654-1103.2004.tb02243.x
Barrat-segretain, M. H., Elger, A., Sagnes, P., and Puijalon, S. (2002). Comparison of three life-history traits of invasive Elodea canadensis Michx. and Elodea nuttallii (Planch.) H. St. John. Aquat. Bot. 74, 299–313. doi: 10.1016/S0304-3770(02)00106-7
Bolhár-Nordenkampf, H. R., Long, S. P., Baker, N. R., Öquist, G., Schreiber, U., and Lechner, E. G. (1989). Chlorophyll fluorescence as a probe of the photosynthetic competence of leaves in the field: a review of current instrumentation. Funct. Ecol. 3, 497–514.
Cabrera, W. G., Dalto, Y. M., Mattioli, F. M., Carruthers, R. I., and Anderson, L. W. (2013). Biology and ecology of Brazilian elodea (Egeria densa) and its specific herbivore, Hydrellia sp., in Argentina. Biocontrol 58, 133–147. doi: 10.1007/s10526-012-9475-x
Cao, Y., Neif,ÉM., Li, W., Coppens, J., Filiz, N., Lauridsen, T. L., et al. (2015). Heat wave effects on biomass and vegetative growth of macrophytes after long-term adaptation to different temperatures: a mesocosm study. Clim. Res. 66, 265–274. doi: 10.3354/cr01352
Carrillo, Y., Guarín, A., and Guillot, G. (2006). Biomass distribution, growth and decay of Egeria densa in a tropical high-mountain reservoir (NEUSA, Colombia). Aquat. Bot. 85, 7–15. doi: 10.1016/j.aquabot.2006.01.006
Chambers, P. A., and Kaiff, J. (1985). Depth distribution and biomass of submersed aquatic macrophyte communities in relation to Secchi depth. Can. J. Fish. Aquat. Sci. 42, 701–709. doi: 10.1139/f85-090
Champion, P. D., and Tanner, C. C. (2000). Seasonality of macrophytes and interaction with flow in a New Zealand lowland stream. Hydrobiologia 441, 1–12. doi: 10.1023/A:1017517303221
Cook, C. D. K., and Urmi-König, K. (1984). A revision of the genus Egeria (hydrocharitaceae). Aquat. Bot. 19, 73–96. doi: 10.1016/0304-3770(84)90009-3
Cook, C. D. K., and Urmi-König, K. (1985). A revision of the genus Elodea (Hydrocharitaceae). Aquat. Bot. 21, 111–156. doi: 10.1016/0304-3770(85)90084-1
Duarte, C. M., and Kalff, J. (1990). Patterns in the submerged macrophyte biomass of lakes and the importance of the scale of analysis in the interpretation. Can. J. Fish. Aquat. Sci. 47, 357–363. doi: 10.1139/f90-037
Fan, S., Yu, H., Liu, C., Yu, D., Han, Y., and Wang, L. (2015). The effects of complete submergence on morphological and biomass allocation response of invasive plant Alternanthera philoxeroides. Hydrobiologia 746, 159–169. doi: 10.1007/s10750-014-2005-3
Fujiwara, A., Matsuhashi, S., Doi, H., Yamamoto, S., and Minamoto, T. (2016). Use of environmental DNA to survey the distribution of an invasive submerged plant in ponds. Freshw. Sci. 35, 748–754.
Garbey, C., Murphy, K. J., Thiébaut, G., and Muller, S. (2004). Variation in P-content in aquatic plant tissues offers an efficient tool for determining plant growth strategies along a resource gradient. Freshw. Biol. 49, 346–356. doi: 10.1111/j.1365-2427.2004.01188.x
Gassmann, A., Cock, M. W., Shaw, R., and Evans, H. (2006). The potential for biological control of invasive alien aquatic weeds in Europe: a review. Hydrobiologia 570, 217–222. doi: 10.1007/978-1-4020-5390-0_31
Gu, X., Zhang, S. Z., Bai, X. L., Hu, W., and Wang, X. R. (2005). Evolution of community structure of aquatic macrophytes in East Taihu Lake and its wetlands. Acta Ecol. Sin. 25, 1541–1548. doi: 10.3321/j.issn:1000-0933.2005.07.002
Haag, R. W. (1979). The ecological significance of dormancy in some rooted aquatic plants. J. Ecol. 67, 727–738. doi: 10.2307/2259122
Han, Y. Q., Wang, L. G., You, W. H., Yu, H. H., Xiao, K. Y., and Wu, Z. H. (2018). Flooding interacting with clonal fragmentation affects the survival and growth of a key floodplain submerged macrophyte. Hydrobiologia 806, 67–75. doi: 10.1007/s10750-017-3356-3
Hofstra, D. E., Clayton, J., Green, J. D., and Auger, M. (1999). Competitive performance of Hydrilla verticillata in New Zealand. Aquat. Bot. 63, 305–324. doi: 10.1016/S0304-3770(98)00125-9
Hussner, A. (2009). Growth and photosynthesis of four invasive aquatic plant species in Europe. Weed Res. 49, 506–515. doi: 10.1111/j.1365-3180.2009.00721.x
Hussner, A., and Lösch, R. (2005). Alien aquatic plants in a thermally abnormal river and their assembly to neophyte-dominated macrophyte stands (River Erft, Northrhine-Westphalia). Limnologica 35, 18–30. doi: 10.1016/j.limno.2005.01.001
Hussner, A., Meyer, C., and Busch, J. (2009). The influence of water level and nutrient availability on growth and root system development of Myriophyllum aquaticum. Weed Res. 49, 73–80. doi: 10.1111/j.1365-3180.2008.00667.x
Hussner, A., Stiers, I., Verhofstad, M. J. J. M., Bakker, E. S., Grutters, B. M. C., Haury, J., et al. (2017). Management and control methods of invasive alien freshwater aquatic plants: a review. Aquat. Bot. 136, 112–137. doi: 10.1016/j.aquabot.2016.08.002
Kercher, S., and Zedler, J. (2004). Multiple disturbances accelerate invasion of reed canary grass (Phalaris arundinacea L.) in a mesocosm study. Oecologia 138, 455–464. doi: 10.1007/s00442-003-1453-7
Kunii, H. (1981). Characteristics of the winter growth of detached Elodea nuttallii (Planch.) St. John in Japan. Aquat. Bot. 11, 57–66. doi: 10.1016/0304-3770(81)90046-2
Kunii, H. (1982). The critical water temperature for the active growth of Elodea nuttallii (Planch.) St. John. Jpn. J. Ecol. 32, 111–112. doi: 10.18960/seitai.32.1_111
Kunii, H. (1984). Seasonal growth and profile structure development of Elodea nuttallii (Planch.) St. John in pond Ojaga-Ike, Japan. Aquat. Bot. 18, 239–247. doi: 10.1016/0304-3770(84)90065-2
Li, L., Lan, Z. C., Chen, J. K., and Song, Z. P. (2018). Allocation to clonal and sexual reproduction and its plasticity in Vallisneria spinulosa along a water-depth gradient. Ecosphere 9:e02070. doi: 10.1002/ecs2.2070
Li, W., Cao, T., Ni, L. Y., Zhang, X. L., Zhu, G. R., and Xie, P. (2013). Effects of water depth on carbon, nitrogen and phosphorus stoichiometry of five submersed macrophytes in an in situ experiment. Ecol. Eng. 61, 358–365. doi: 10.1016/j.ecoleng.2013.09.028
Li, W., Xia, L. Q., Li, J. Q., and Wang, G. X. (2004). Genetic diversity of Potamogeton maackianus in the yangtze river. Aquat. Bot. 80, 227–240. doi: 10.1016/j.aquabot.2004.07.003
Lichtenthaler, H. K. (1987). Chlorophylls and carotenoids: pigments of photosynthetic biomembranes. Method Enzymol. 148, 350–382. doi: 10.1016/0076-6879(87)48036-1
Liu, J. C., Chen, X. W., Wang, Y. L., Li, X., Yu, D., and Liu, C. H. (2016). Response differences of Eichhornia crassipes to shallow submergence and drawdown with an experimental warming in winter. Aquat. Ecol. 50, 307–314. doi: 10.1007/s10452-016-9579-y
Lonsdale, W. M. (1999). Global patterns of plant invasions and the concept of invasibility. Ecology 80, 1522–1536. doi: 10.1890/0012-9658(1999)080[1522:GPOPIA]2.0.CO;2
Lui, K., Thompson, F., and Eckert, C. (2005). Causes and consequences of extreme variation in reproductive strategy and vegetative growth among invasive populations of a clonal aquatic plant, Butomus umbellatus L. (Butomaceae). Biol. Invasions 7, 427–444. doi: 10.1007/s10530-004-4063-3
Mack, R. N., Simberloff, D., Lonsdale, W. M., Evans, H., Clout, M., and Bazzaz, F. A. (2000). Biotic invasions: causes, epidemiology, global, consequences, and control. Ecol. Appl. 10, 689–710. doi: 10.1890/1051-0761(2000)010[0689:BICEGC]2.0.CO;2
Meneguelli-Souza, A. C., Vitória, A. P., Vieira, T. O., Degli-Esposti, M. S. O., and Souza, C. M. M. (2016). Ecophysiological responses of Eichhornia crassipes (Mart.) solms to As5+ under different stress conditions. Photosynthetica 54, 243–250. doi: 10.007/s11099-015-0174-6
Nagasaka, M. (2004). Changes in biomass and spatial distribution of Elodea nuttallii (Planch.) St. John, an invasive submerged plant, in oligomesotrophic Lake Kizaki from 1999 to 2002. Limnology 5, 129–139. doi: 10.1007/s10201-004-0129-2
Ni, L. Y. (2001). Growth of Potamogeton maackianus under low-light stress in eutrophic water. J. Freshw. Ecol. 16, 249–256. doi: 10.1080/02705060.2001.9663809
Nichols, S. A., and Shaw, B. H. (1986). Ecological life histories of the three aquatic nuisance plants, Myriophyllum spicatum, Potamogeton crispus and Elodea canadensis. Hydrobiologia 131, 3–21. doi: 10.1007/BF00008319
Ozimek, T., Van Donk, E., and Gulati, R. D. (1993). Growth and nutrient uptake by two species of Elodea in experimental conditions and their role in nutrient accumulation in a macrophyte-dominated lake. Hydrobiologia 251, 13–18. doi: 10.1007/978-94-011-1602-2_2
Pennington, T. G. (2008). Seasonal Changes in Allocation, Growth, and Photosynthetic Responses of the Submersed Macrophyte Egeria Densa Planch. (Hydrocharitaceae) From Oregon and California. Ph. D Thesis, Portland State University, Portland.
Pennington, T. G., and Sytsma, M. D. (2009). Seasonal changes in carbohydrate and nitrogen concentrations in Oregon and California populations of Brazilian egeria (Egeria densa). Invasive Plant Sci. Manag. 2, 120–129. doi: 10.1614/IPSM-08-101.1
Rajan, A. K., Betteridge, B., and Blackman, G. E. (1973). Differences in the interacting effects of light and temperature on growth of four species in the vegetative phase. Ann. Bot. 37, 287–316. doi: 10.1093/oxfordjournals.aob.a084693
Rejmánek, M., and Richardson, D. M. (1996). What attributes make some plant species more invasive? Ecology 77, 1655–1661. doi: 10.2307/2265768
Richardson, D. M., Pyšek, P., Rejmánek, M., Barbour, M. G., Paneta, F. D., and West, C. J. (2000). Naturalization and invasion of alien plants: concepts and definitions. Divers. Distrib. 6, 93–107. doi: 10.1046/j.1472-4642.2000.00083.x
Schreiber, U., Bilger, W., Hormann, H., and Neubauer, C. (2000). “Chlorophyll fluorescence as a diagnostic tool: basics and some aspects of practical relevance,” in Photosynthesis: A Comprehensive Treatise, ed. A. S. Raghavendra (Cambridge: Cambridge University Press), 320–336.
Strand, J. A., and Weisner, S. E. B. (2001). Morphological plastic responses to water depth and wave exposure in an aquatic plant (Myriophyllum spicatum). J. Ecol. 89, 166–175. doi: 10.1046/j.1365-2745.2001.00530.x
Su, H. J., Wu, Y., Xia, W. L., Yang, L., Chen, J. F., Han, W. X., et al. (2019). Stoichiometric mechanisms of regime shifts in freshwater ecosystem. Water Research 149, 302–310. doi: 10.1016/j.watres.2018.11.024
Szabó, S., Peeters, E. T. H. M., Várbíró, G., Borics, G., and Lukács, B. A. (2019). Phenotypic plasticity as a clue for invasion success of the submerged aquatic plant Elodea nuttallii. Plant Biol. 21, 54–63. doi: 10.1111/plb.12918
Vöge, M. (1994). Tauchbeobachtungen in Siedlungsgewässern von Elodea nuttallii (Planch.) St. John. Tuexenia 14, 335–342.
Waage, J. K., and Reaser, J. K. (2001). A global strategy to defeat invasive species. Science 292:1486. doi: 10.1126/science.292.5521.1486a
Wersal, R. M., and Madsen, J. D. (2011). Comparative effects of water level variations on growth characteristics of Myriophyllum aquaticum. Weed Res. 51, 386–393. doi: 10.1111/j.1365-3180.2011.00854.x
Xiao, C., Wang, X. F., Xia, J., and Liu, G. (2010). The effect of temperature, water level and burial depth on seed germination of Myriophyllum spicatum and Potamogeton malaianus. Aquat. Bot. 92, 28–32. doi: 10.1016/j.aquabot.2009.09.004
Xie, D., Yu, D., You, W. H., and Xia, C. X. (2013). The propagule supply, litter layers and canopy shade in the littoral community influence the establishment and growth of Myriophyllum aquaticum. Biol. Invasions 15, 113–123. doi: 10.1007/s10530-012-0272-3
Xu, J. W., Li, W., Liu, G. H., Zhang, L. J., and Liu, W. Z. (2007). Inter-specific competition between two submerged macrophytes, Elodea nuttallii and Hydrilla verticillata. J. Plant Ecol. 31, 83–92. doi: 10.17521/cjpe.2007.0011
Xu, W. W., Hu, W. P., Deng, J. C., Zhu, J. G., and Li, Q. Q. (2016). How do water depth and harvest intensity affect the growth and reproduction of Elodea nuttallii (Planch.) St. John? J. Plant Ecol. 9, 212–223. doi: 10.1093/jpe/rtv048
Xu, X., Huang, X. L., Zhang, Y. L., and Yu, D. (2018). Long-term changes in water clarity in Lake Liangzi determined by remote sensing. Remote Sens. 10:1441. doi: 10.3390/rs10091441
Yang, Y. Q., Yu, D., Li, Y., Xie, Y. H., and Geng, X. H. (2004). Phenotypic plasticity of two submersed plants in response to flooding. J. Freshw. Ecol. 19, 69–76. doi: 10.1080/02705060.2004.9664514
Yarrow, M., Marin, V. H., Finlayson, M., Tironi, A., Delgado, L. E., and Fischer, F. (2009). The ecology of Egeria densa Planchon (Liliopsida: Alismatales): a wetland ecosystem engineer. Rev. Chil. Hist. Nat. 82, 299–313. doi: 10.4067/S0716-078X2009000200010
You, W. H., Yu, D., Xie, D., Yu, L. F., Xiong, W., and Han, C. M. (2014). Responses of the invasive aquatic plant water hyacinth to altered nutrient levels under experimental warming in China. Aquat. Bot. 119, 51–56. doi: 10.1016/j.aquabot.2014.06.004
Yu, H. H., Wang, L. G., Liu, C. H., and Fan, S. F. (2018). Coverage of native plants is key factor Influencing the invasibility of freshwater ecosystems by exotic plants in China. Front. Plant Sci. 9:250. doi: 10.3389/fpls.2018.00250
Zehnsdorf, A., Hussner, A., Eismann, F., Rönicke, H., and Melzer, A. (2015). Management options of invasive Elodea nuttallii and Elodea canadensis. Limnologica 51, 110–117. doi: 10.1016/j.limno.2014.12.010
Zhang, S. Z., Wang, G. X., Pu, P. M., and Chhigira, T. (1999). Succession of hydrophytic vegetation and swampy tendency in the East Taihu Lake. J. Plant Resour. Environ. 8, 1–6.
Zhang, Y. Y., Zhang, D. Y., and Barrett, S. C. H. (2010). Genetic uniformity characterizes the invasive spread of water hyacinth (Eichhornia crassipes), a clonal aquatic plant. Mol. Ecol. 19, 1774–1786. doi: 10.1111/j.1365-294X.2010.04609.x
Zhao, F. B., Zhang, W., Liu, Y. H., and Wang, L. Q. (2018). Responses of growth and photosynthetic fluorescent characteristics in Ottelia acuminata to a water-depth gradient. J. Freshw. Ecol. 33, 285–297. doi: 10.1080/02705060.2018.1443841
Keywords: invasive, submerged, water depth, overwinter, morphological, photosynthesis
Citation: Wang Y, Chen X, Liu J, Hong Y, He Q, Yu D, Liu C and Dingshanbayi H (2019) Greater Performance of Exotic Elodea nuttallii in Response to Water Level May Make It a Better Invader Than Exotic Egeria densa During Winter and Spring. Front. Plant Sci. 10:144. doi: 10.3389/fpls.2019.00144
Received: 31 July 2018; Accepted: 28 January 2019;
Published: 25 February 2019.
Edited by:
Te Cao, Institute of Hydrobiology (CAS), ChinaReviewed by:
Heyun Wang, Hubei University of Technology, ChinaWei Li, Nanchang Institute of Technology, China
Copyright © 2019 Wang, Chen, Liu, Hong, He, Yu, Liu and Dingshanbayi. This is an open-access article distributed under the terms of the Creative Commons Attribution License (CC BY). The use, distribution or reproduction in other forums is permitted, provided the original author(s) and the copyright owner(s) are credited and that the original publication in this journal is cited, in accordance with accepted academic practice. No use, distribution or reproduction is permitted which does not comply with these terms.
*Correspondence: Chunhua Liu, bGl1Y2hoQDE2My5jb20=