- 1Key Laboratory of Molecular Epigenetics of MOE and Institute of Genetics and Cytology, Northeast Normal University, Changchun, China
- 2National Demonstration Center for Experimental Biology Education, Northeast Normal University, Changchun, China
The flavonoids are important and nourishing compounds for plants and human. The transcription regulation of anthocyanin and proanthocyanidin (PA) biosynthesis was extensively studied in dicot compared with monocot plants. In this study, we characterized the functionality of an R2R3-MYB gene FhMYB5 from the monocotyledonous flowering plant of Iridaceae, Freesia hybrida. Multiple sequence alignment and phylogenetic analysis implied that FhMYB5 was clustered into grapevine VvMYB5b subclade. Correlation analysis indicated that the spatio-temporal expression patterns of FhMYB5 coincided well with anthocyanin and PA accumulations in Freesia per se. Furthermore, transient transfection assays in Freesia protoplasts revealed that the late flavonoid biosynthetic genes (e.g., DFR and LDOX) were slightly up-regulated by FhMYB5 alone, whereas both early and late biosynthetic genes were significantly activated when FhMYB5 were co-infected with either of the two IIIf clade bHLH genes, FhTT8L and FhGL3L. Moreover, these results were further confirmed by co-transfection of FhMYB5 with either of the bHLH genes aforementioned into protoplasts expressing GUS reporter gene driven by Freesia promoters. In addition, the overexpression of FhMYB5 in tobacco and Arabidopsis could also significantly up-regulate the expression of genes participating in the general flavonoid pathway. In conclusion, FhMYB5 was proved to function in the general flavonoid pathway in Freesia. The results implied a function conservation of flavonoid biosynthesis related MYB regulators in angiosperm plants.
Introduction
Anthocyanins and proanthocyanidins (PAs) are two major classes of flavonoids in plants. Anthocyanins, responsible for the wide range of colors including red, blue, violet and purple in plants, are implicated in protecting plants against damaging coldness and UV irradiation and facilitating pollination and seed distribution (Shao et al., 2018). PAs, also known as condensed tannins (CTs), are widely characterized by their abilities of anti-microbial pathogens, insect pests, and herbivores (Shao et al., 2018). Evolutionarily, the two kinds of specialized metabolites emerged at different times. The anthocyanins are widely spread in angiosperms while the PAs could be traced back in ferns (Koes et al., 1994). There is no doubt that the evolutionary emergence of anthocyanins and PAs have aided the plants in environment adaptation. Besides, there are growing evidences showing that adequate dietary intake of foods rich in powerful antioxidants, e.g., anthocyanins and PAs, can notably reduce the risk of sufferings from cardiovascular and immune diseases (Bagchi et al., 2003; Jang et al., 2010). Thus, the health-promoting athocyanins and PAs have hitherto attracted the attentions of both scientists and breeders (Albert, 2015; An et al., 2015, 2017; Huang et al., 2015; Gao et al., 2016).
The biosynthesis of anthocyanins and PAs has been widely investigated in plants over the past few decades. Two typical classes of genes, structural genes encoding enzymes accounting for anthocyanin and PA accumulations directly and regulatory genes encoding transcription factors regulating the structural genes at the transcriptional level, have been characterized in numerous plants (Hichri et al., 2011a; Jaakola, 2013; Liu et al., 2015; Xu et al., 2015; Zhao and Tao, 2015). More precisely, at least two groups of structural genes, namely the early and late biosynthetic genes (EBGs and LBGs) have been characterized in flavonoid biosynthesis (Lepiniec et al., 2006; Petroni and Tonelli, 2011). The EBGs contribute to the biosynthesis of dihydroflavonols which are the common precursors of several kinds of flavonoids, whereas the LBGs are more specific to anthocyanin and PA biosynthesis. Furthermore, these structural genes are finely regulated by transcription factors encoded by regulatory genes (Broun, 2005; Xu et al., 2015). Recent studies have pinpointed that a series of regulatory genes encoding R2R3-MYB regulators, basic helix–loop–helix (bHLH) transcription factors, WD40 proteins and other regulators are involved in the regulation of anthocyanin and PA biosynthesis (Jaakola, 2013; Xu et al., 2015). In the model plant Arabidopsis, partially redundant R2R3-MYB regulators AtMYB11, AtMYB12, and AtMYB111 can activate the EBGs, while a ternary complex composed of R2R3-MYB regulator AtPAP1, bHLH factor AtTT8 or AtGL3, and WD40 protein AtTTG1 mainly regulate the expressions of the LBGs in anthocyanin biosynthetic pathway (Baudry et al., 2004; Stracke et al., 2007; Gonzalez et al., 2008; Zhou et al., 2012; Jaakola, 2013; Xu et al., 2015). Meanwhile, another R2R3-MYB factor AtTT2 mainly regulates the PA-specific AtBAN by forming another complex with AtTT8 and AtTTG1 (Hichri et al., 2011a; Zhao and Tao, 2015). To date, the evolutionarily conserved regulatory protein complex partners have been widely characterized in various species such as petunia (Petunia hybrida), pear (Pyrus bretschneideri Rehd.), grape (Vitis vinifera), apple (Malus domestica), strawberry (Fragaria x ananassa) and other angiosperms, mainly in core eudicot plants (Petroni and Tonelli, 2011; Jaakola, 2013; Schaart et al., 2013; An et al., 2015; Pillet et al., 2015; Zhai et al., 2016). In the MBW complex, the widely accepted mainstream opinion is that MYB protein determines the target genes to be specially activated. Generally, the function of a specific MYB protein is usually limited to a specialized pathway, such as AtPAP1 for anthocyanins and AtTT2 for PAs (Liu et al., 2015). In contrast, some general MYB regulators have also been found in addition to these specialized MYB regulators, although the numbers are limited. VvMYB5a and VvMYB5b isolated from grapevine, and EsMYB9 characterized from Epimedium sagittatum are examples of this kind of MYB regulators controlling more than one branches of the flavonoid pathway (Deluc et al., 2006, 2008; Hichri et al., 2011b; Huang et al., 2012, 2017). Though, numerous MYB members have been well studied in several monocotyledon plants, such as Zea mays, Oryza sativa, Lilium spp. and Phalaenopsis spp., they were proved to regulate the specialized flavonoid biosynthesis (Grotewold et al., 1994; Maeda et al., 2014; Yamagishi et al., 2014; Hsu et al., 2015). Few general MYB regulators have been isolated from monocot plants, which is a constraint to clarify their functional conservation or difference in angiosperms.
Freesia hybrida, a monocotyledonous flowering plant of Iridaceae, is distinguished for its abundant flower colors and fragrances. Recent studies revealed that there were five kinds of anthocyanin aglycons, namely delphinidin, petunidin, malvinidin, peonidin, and cyanidin, and two kinds of flavonols, namely kaempferol and quercetin, as well as PAs in F. hybrida “Red River®” (Sun et al., 2015, 2016; Li et al., 2016). To date, six structural genes and two IIIf bHLH regulatory genes have been functionally characterized (Sui et al., 2011; Sun et al., 2015, 2016, 2017; Li et al., 2016, 2017; Ju et al., 2018). In this study, the first MYB regulatory gene FhMYB5 was isolated and performed as candidate gene involved in flavonoid biosynthesis in the Freesia flowers. Spatiotemporal expression analysis implied a potential correlation of FhMYB5 with both anthocyanin and PA accumulations in Freesia per se. Transient expression assays indicated that FhMYB5 was an activator and participated in both anthocyanin and PA biosynthesis by interacting with bHLH co-factors. This was further validated by introducing FhMYB5 into tobacco as well as Arabidopsis, representatives of Asterids and Rosids, respectively. This study not only clarified the molecular mechanisms of flavonoid biosynthesis in Freesia, but also preliminarily indicated the evolutionary conservation of flavonoid biosynthesis related MYB proteins between monocots and dicots.
Materials and Methods
Plant Materials and Growth Conditions
One cultivar of F. hybrida, named Red River® was used in this study. Bulbs were grown in sandy soil in a greenhouse at 15°C under 14 h/10 h (light/dark) photoperiod. The soil was watered aperiodically to keep moist before anthesis (approximately one time a week). Young inflorescence segments were surface sterilized and employed as explants to induce calli following the highly efficient systems established in wild species and modern cultivars (Gao et al., 2010; Uwagaki et al., 2015), and the calli were subjected to protoplast isolation and transient transfection analysis. Moreover, flowers at different developmental stages and tissues stripped from the fully blooming flowers were randomly sampled and immediately frozen in liquid nitrogen before storage at -80°C, which were subsequently used for gene cloning, spatio-temporal expression analysis as well as promoter isolation.
Nicotiana tabacum (cv. K326) and Arabidopsis thaliana (Columbia-0) were grown in a greenhouse at 22°C under a 16 h light/8 h dark regime. The tobacco flowers fully blossomed at the first day were harvested and divided into five tissues, i.e., toruses, calyxes, petals, stamens and pistils. And the fully expanded mature leaves, the fourth or fifth leaf from the apical meristem, were sampled. The samples were immediately frozen in liquid nitrogen and stored at -80°C before further analysis. Moreover, 4-week-old Arabidopsis leaves were chosen for flavonoid accumulation analysis, gene expression analysis and protoplast isolation.
DNA and RNA Extraction and cDNA Synthesis
Plant samples grounded into powder in liquid nitrogen were processed by NuClean Plant Genomic DNA Kit (CWBIO, Beijing, China) for DNA extraction referring to the manufacturer’s instruction. Total RNA was isolated from various tissues using the OminiPlant RNA Kit (CWBIO, Beijing, China) followed by DNase I digestion to remove DNA contamination according to the manufacturer’s instruction. The purity and concentration of RNA was then assessed by NanoDrop 1000 spectrophotometry (Thermo Scientific, United States). 1 μg of total RNA was reversely transcribed into cDNA using UEIris II RT-PCR System for First-Strand cDNA Synthesis Kit (US Everbright® Inc., Suzhou, China).
Gene and Promoter Cloning
Freesia transcriptomic database was constructed by the high throughput sequencing of RNA samples extracted from flower tissues including toruses, calyxes, petals, stamens, and pistils and flowers at different developmental stages. In situ TBLASTN screen was conducted using Vitis VvMYB5a (GenBank accession number: AAS68190) as probe bait. The candidate gene was amplified using specific primers (Supplementary Table S1) designed according to the predicted sequence, and cloned into pGEM-Teasy vector (Promega, Madison, WI, United States) for sequencing confirmation. The verified gene sequence was subsequently subjected to manual BLASTX search of NCBI database for further analysis (Supplementary Table S2).
The promoters of Freesia anthocyanin biosynthetic genes were cloned from genomic DNA isolated with Genome Walking Kit (Takara, Dalian, China). At least three specific reverse primers (SP primers) for each gene were designed for TAIL-PCR with arbitrary degenerate primers (AP primers supplied in the kit) to isolate upstream sequences (promoters) according to the manufacturer’s instructions. The PCR products were sub-cloned into pGEM-Teasy vector and sequenced. Finally, 1,000–1,500-bp upstreams of the ATG transcriptional start site were tentatively employed as promoter sequences. The primers were presented in Supplementary Table S1.
Plant Transformation
About 5- to 6-week-old tobacco was transformed and regenerated according to Sparkes et al. (2006). The binary vectors were introduced into Agrobacterium tumefaciens strain GV3101 and injected into tobacco leaves with syringes. About 5 days later, the infiltrated leaves were sterilized and cut into pieces. The leaf squares were then placed on the shooting medium with 50 μg mL-1 kanamycin until shoots appeared. Three to four weeks later, the shoots were transferred onto the rooting medium, and roots would appear within 7–10 days. The rooted plantlets were deemed as T0 generations and then transferred into pots and grown in the greenhouse. Fully expanded mature leaves and blooming flowers of T0 tobacco plants were collected for further analysis.
About 5- to 6-week-old Arabidopsis with a few mature flowers was transformed with GV3101 containing FhMYB5 by the floral dip method (Clough and Bent, 1998). Selection of the T1 transformants was carried out on 1/2 MS supplemented with 50 μg mL-1 kanamycin, and the survivals were transferred to soil and cultivated in the greenhouse to confirm the expression of the exogenous FhMYB5 gene by RT-PCR.
Quantitative Real-Time PCR Analysis
Specific quantitative real-time PCR primers were designed to detect the spatial and temporal expression patterns of FhMYB5. Meanwhile, primers for anthocyanin biosynthetic genes from tobacco and Arabidopsis were also designed to detect gene expression levels by quantitative real-time PCR (Supplementary Table S1). RT-qPCR assays were carried out in 20 μL reactions with SYBR Green Mixture (US Everbright® Inc., Suzhou, China) using an ABI7500 RT-qPCR system. Each PCR mixture contained 1 μL of cDNA, 10 μL of 2 × Taq DNA polymerase premix and each primer at 0.25 μM. The reaction mixtures were heated to 95°C for 5 min, 45 cycles at 95°C for 10 s, 60°C for 10 s and 72°C for 20 s. The relative transcript levels were normalized by Freesia 18S rRNA, tobacco Tubulin and Arabidopsis Actin, respectively, and calculated using the 2-ΔΔCT analysis method (Livak and Schmittgen, 2001). All biological replicates were analyzed in triplicate.
Constructs
Vectors used in this study were constructed using Minerva Super Fusion Cloning Kit (US Everbright® Inc., Suzhou, China). The modified pUC19 vectors with HA, GD, GFP(N) or GFP(C) tag, which were driven by double 35S promoter of CaMV and terminated by NOS terminator, were all linearized by NdeI and AflII (Wang et al., 2015). To generate constructs for plant transformation, the complete coding sequence of FhMYB5 cDNA was amplified with specific primers designed to introduce 15-bp overlap regions with the digested pUC19 vector and assembled by Minerva Super Fusion Cloning Kit, creating the pUC19-HA-FhMYB5 plasmid. The pUC19-HA-FhMYB5 vector was then digested by EcoRI and the fragments containing 35S promoter, HA tag, FhMYB5 and NOS terminator were cloned into binary vector pPZP211 by T4 DNA ligase (TransGen Biotech, Beijing, China), creating pPZP211-HA-FhMYB5 plasmid, which was then introduced into Agrobacterium tumefaciens GV3101 strain before plant transformation. For plasmids used in plant protoplast transient transfection assays, corresponding sequence of each gene was constructed in the backbone of pUC19 according to the protocol mentioned above to generate vectors such as pUC19-GFP(N)-FhMYB5, pUC19-GFP(C)-FhTT8L, pUC19-GFP(C)-FhGL3L, pUC19-HA-AtTT2, pUC19-GD-NtAN1 and pUC19-GD-NtJAF13. As for GUS reporter plasmids used in transient transfection assays, the former constructed AtDFR-pro:GUS vector used in earlier studies was digested by PstI and SacI (Li et al., 2017). The -1,500- to -1000-bp upstreams of Freesia anthocyanin biosynthetic genes from the initiation codon “ATG” were assembled into the digested GUS vector following the methods aforementioned. All the other constructs used in this study have been described previously (Li et al., 2016, 2017).
Transient Transfection Assays
All the plasmids used in transient transfection assays were extracted from Escherichia coli using GoldHi EndoFree Plasmid Maxi Kit (CWBIO, Beijing, China) and then concentrated by isopropanol and 5 M NaCl solution. The volume ratio between plasmids, isopropanol and NaCl was 1:1.42:0.42. The mixture was then incubated at room temperature for 10 min followed by centrifugation at 4,000 rpm for 20 min. The pellet was washed at least once by 75% ethanol before dissolved by moderate ultrapure water. The enriched plasmids were subjected to the further transfection assays.
Protoplasts isolation and transfection assays were performed as described in earlier studies (Yoo et al., 2007; Zhou et al., 2014; Li et al., 2016, 2017). Briefly, 3- to 4-week-old Arabidopsis leaves were employed for the protoplasts isolation, while 3-week-old subcultured calli were collected to isolate protoplasts from Freesia. Different combinations of 10 μg aliquot of effector and reporter plasmids were transfected into protoplasts by PEG3350. After 20–22 h incubation at 22°C in the dark, the transfected protoplasts were gathered by centrifugation and subjected to GUS activity detection using a SynergyTM HT microplate reader (BioTEK, United States) or total RNA extraction with the OminiPlant RNA Kit (CWBIO, Beijing, China) for gene expression analysis. The transient transfection assays were repeated in triplicate with three biological replicates.
Anthocyanin and Proanthocyanidin Analysis
Total anthocyanin and PA contents were determined in both transgenic and control plants as described in previous study (Li et al., 2016). For anthocyanin measurement, 0.1–0.15 g of each sample was ground into powder in liquid nitrogen and soaked in 1 mL acidic methanol (containing 1% HCl) at 4°C overnight. The solution was centrifuged at 12,000 rpm for 1 min to collect the supernatant. 400 μL of supernatant was mixed with 600 μL of acidic methanol. The absorbance of each sample was measured at 530 and 657 nm using a spectrophotometer (BioTEK, United States), respectively. The relative content of anthocyanin was quantified as (A530–0.25 × A657) g-1 fresh weight (FW). For measurement of PA, 0.1–0.15 g of samples were extracted with 1 mL of 70% (V/V) aqueous acetone solution containing 0.1% (W/V) ascorbic acid at 4°C for 1 h. After centrifugation at 12,000 rpm for 5 min, the supernatant was transferred to a new tube and the precipitate was soaked in another 1 mL of 70% (V/V) aqueous acetone solution containing 0.1% (W/V) ascorbic acid at 4°C overnight. The 2 mL of supernatant was mixed with 1.5 mL of diethyl ether and kept at -20°C in the dark for the separation of two phases. ∼330 μL of lower phase was mixed with 84 μL of DMACA (dimethylaminocinnamaldehyde) regent and 165 μL of methanol, kept at room temperature for 20 min to detect the soluble PA. The DMACA reagent containing 2% (w/v) DMACA in a cold mixture of methanol and 6 M HCl (1:1, v/v) was prepared immediately before use. The PA content was quantified as A640 g-1 fresh weight (FW). For catechin and epicatechin analysis, 0.1 g of samples were processed as the method of PA measurement aforementioned, the ∼400 μL of lower phase after extraction with diethyl ether was concentrated for 1 h using Vacuum Centrifugal Evaporator (CVE-2000, EYELA, Tokyo, Japan). The concentrated solution was injected through 0.22 μm filtrum and analyzed using an ACCHROM XUnion C18 column (250 mm × 4.6 mm, 5 mm). The column was maintained at 30°C and eluted with solvent A (0.1% phosphoric acid in H2O) and B (acetonitrile) following the conditions: 0–16 min, 5–10% B; 16–23 min, 10–13% B; 23–43 min, 13–17% B; 43–48 min, 17–22% B; 48–60 min, 22–30% B; 60–65 min, 30–60% B; 65–72 min, 60–100% B; 72–82 min, 100–5% B; 82–100 min, 5% B. The flow rate was set at 1 mL min-1. Detection was monitored at 280 nm. The standard catechin and epicatechin were purchased from Sigma Chemical Company (St. Louis, MO, United States). For anthocyanin and PA measurement in Freesia protoplasts, nine replicates of protoplasts transfected by the same combination of constructs were gathered by centrifugation and extracted by acidic methanol or acetone solution aforementioned, respectively. The relative contents of anthocyanin and PA were quantified as A530–0.25 × A657 or A640, respectively. All biological replicates were analyzed in triplicate.
The histochemical staining of PA in tobacco flowers was conducted following the earlier studies with some modifications (Harborne, 1989). The tissues were stained with 0.3 % (w/v) DMACA dissolved in a solution of ethanol: 6 M HCl (1:1) for 30 min, and then washed by 70% ethanol.
Results
Isolation and Characterization of FhMYB5 From Freesia hybrida
Initially, the amino acid sequence of Vitis VvMYB5a was used as a query to search homologous sequences participating in flavonoid biosynthesis in Freesia by mining the Freesia transcriptome database through in situ TBLASTN program. Consequently, one sequence was seemed to be a general pathway regulator and tentatively named as FhMYB5 for further functional identification. This 888-bp open reading frame of FhMYB5 encodes a protein of 295 amino acid residues with a predicted molecular mass of ∼33 kD (Supplementary Table S2).
FhMYB5 protein sequence was aligned with other known R2R3-MYB regulators from various plants, which revealed that the deduced FhMYB5 contained the R2R3 imperfect repeats responsible for target DNA binding near its amino-terminal extremity (Figure 1A). The DNA binding domain also contained the conserved motif [D/E]Lx2[R/K]x3Lx6Lx3R which was required to interact with bHLH co-factors in R3 domain (Dubos et al., 2010; Liu et al., 2015). Moreover, the alignment also revealed the appearance of conserved peptidic motifs in the C-terminal regions such as C1 (Lx3GIDPxTHKPL) which was initially found in MYB subgroup 4 (Kranz et al., 2010). In addition to C1, another conserved motif C3 (DDxF[S/P]SFL[N/D]SLIN[E/D]), whose physiological function has not been characterized yet, was also present in FhMYB5.
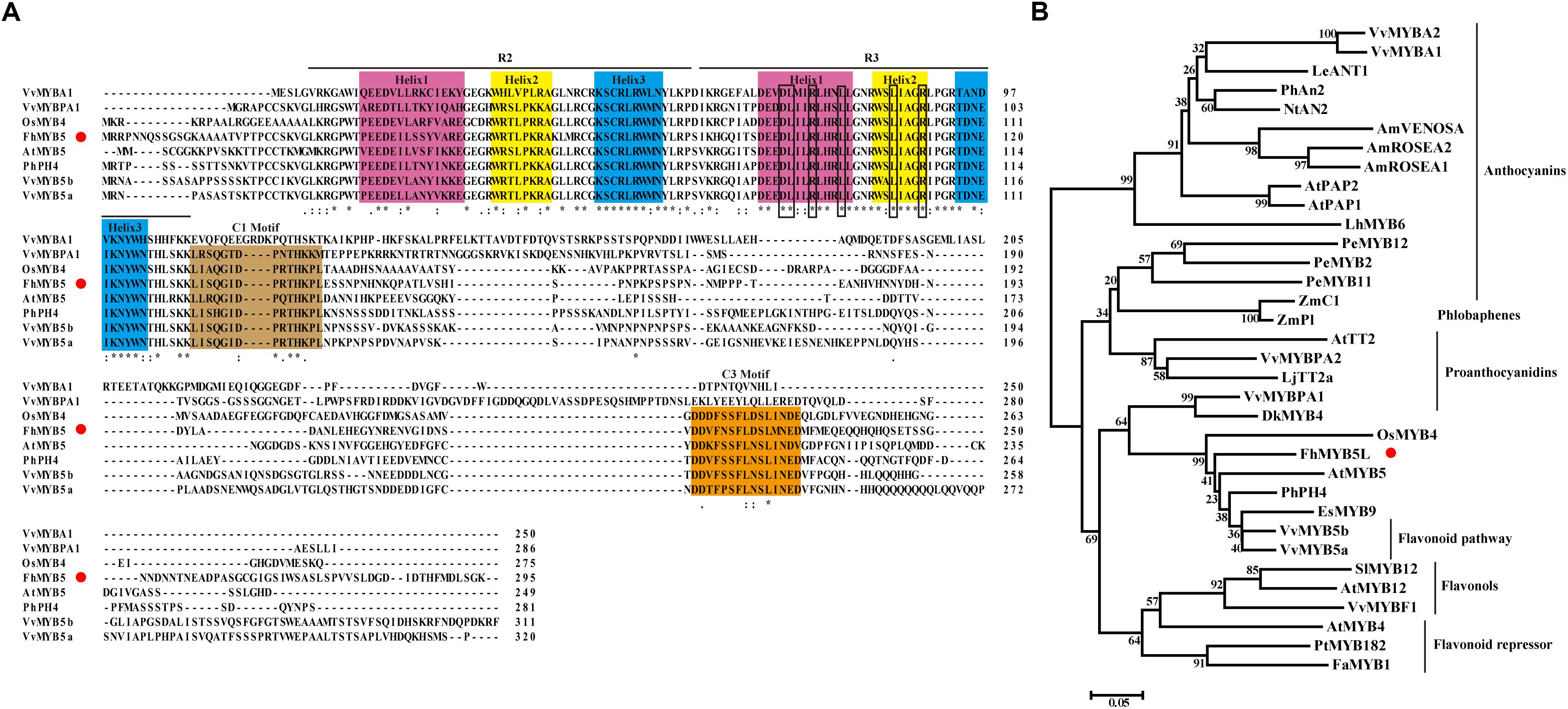
Figure 1. Molecular and phylogenic analysis of FhMYB5. (A) Multiple alignment of the full amino acid sequence of FhMYB5 with MYB proteins from other plants. Number indicated the position of the last amino acid in each line. ∗, identical amino acids; : or ., similar amino acids. The three helixes in either R2 or R3 repeats were shaded in different colors. Rectangular frames were used to indicate the specific motif [D/E]Lx2[R/K]x3Lx6Lx3R responsible for interacting with bHLH factors. The conserved C1 and C3 motifs were also well-marked by colorful backgrounds. Red circle was used for signalizing FhMYB5. (B) Phylogenetic analysis of MYB proteins from different plants. The tree was constructed using the neighbor-joining method by the MEGA 6.0 software. The numbers indicated bootstrap values for 1,000 replicates. FhMYB5 was indicated by red circle. The GenBank accession numbers of the MYB proteins were as follows: Freesia hybrida FhMYB5 (MK168337); Antirrhinum majus AmROSEA1 (ABB83826), AmROSEA2 (ABB83827), AmVENOSA (ABB83828); Arabidopsis thaliana AtPAP1 (AAG42001), AtPAP2 (AAG42002), AtTT2 (Q2FJA2), AtMYB12 (CAB09172), AtMYB4 (AAC83582), AtMYB5 (AAC49311); Vitis vinifera VvMYBF1 (FJ948477), VvMYB5a (AAS68190), VvMYB5b (Q58QD0), VvMYBPA1 (AM259485), VvMYBPA2 (ACK56131), VvMYBA1 (BAD18977), VvMYBA2 (BAD18978); Nicotiana tabacum NtAN2 (ACO52470); Epimedium sagittatum EsMYB9 (AFH03061); Lycopersicon esculentum LeANT1 (AAQ55181); Petunia hybrida PhAn2 (AAF66727), PhPH4 (AAY51377); Lilium hybrid LhMYB6 (BAJ05399); Phalaenopsis equestris PeMYB2 (KF769467), PeMYB11 (KF769476), PeMYB12 (KF769477); Zea mays ZmC1 (AAA33482), ZmP1 (AAA19819); Lotus japonicus LjTT2a (BAG12893); Diospyros kaki DkMYB4 (BAI49721); Oryza sativa OsMYB4 (BAA23340); Fragaria ananassa FaMYB1 (AAK84064); Populus tremula x Populus tremuloides PtrMYB182 (KP723392); Solanum lycopersicum SlMYB12 (ACB46530).
In order to better define FhMYB5, a phylogenetic analysis of 33 plant MYB proteins associated with different functions was constructed by the neighbor-joining method and the proteins were clearly defined into different clades according to their functions (Figure 1B). As results, FhMYB5 belonged to none of the clades of proteins specially regulating one branch of flavonoid pathway, but fell within the cluster of Vitis VvMYB5a and VvMYB5b which functioned in general flavonoid pathway. In conclusion, sequence analysis indicated that FhMYB5 was a member of R2R3-MYB family, and more precisely, belonged to a small group of MYB family characterized by conserved C1 and C3 motifs which might be involved in general flavonoid biosynthesis.
Expression of FhMYB5 Correlates With Anthocyanin and Proanthocyanidin Accumulation in F. hybrida
To verify the relationship between the expression levels of FhMYB5 gene and the flavonoid accumulation, we examined the anthocyanin and PA accumulation together with the expression profiles of the FhMYB5 gene in five flower developmental stages and eight vegetative or reproductive tissues. As results showed in Supplementary Figure S1 and our earlier studies, anthocyanin levels increased gradually and peaked at blooming flowers, while the abundance of PAs fluctuated at a relative stable level during flower development in the Freesia cultivar, Red River® (Li et al., 2016). As for different tissues, anthocyanins and PAs were mainly accumulated in petals and toruses, respectively. RT-qPCR analyses showed that the expression level of FhMYB5 also increased gradually during the five developmental stages, resembling anthocyanin biosynthesis pattern. Moreover, FhMYB5 was highly expressed in both petals and toruses, from which we inferred that FhMYB5 might also be involved in the biosynthesis of other flavonoid compounds other than anthocyanins, such as PAs (Figure 2).
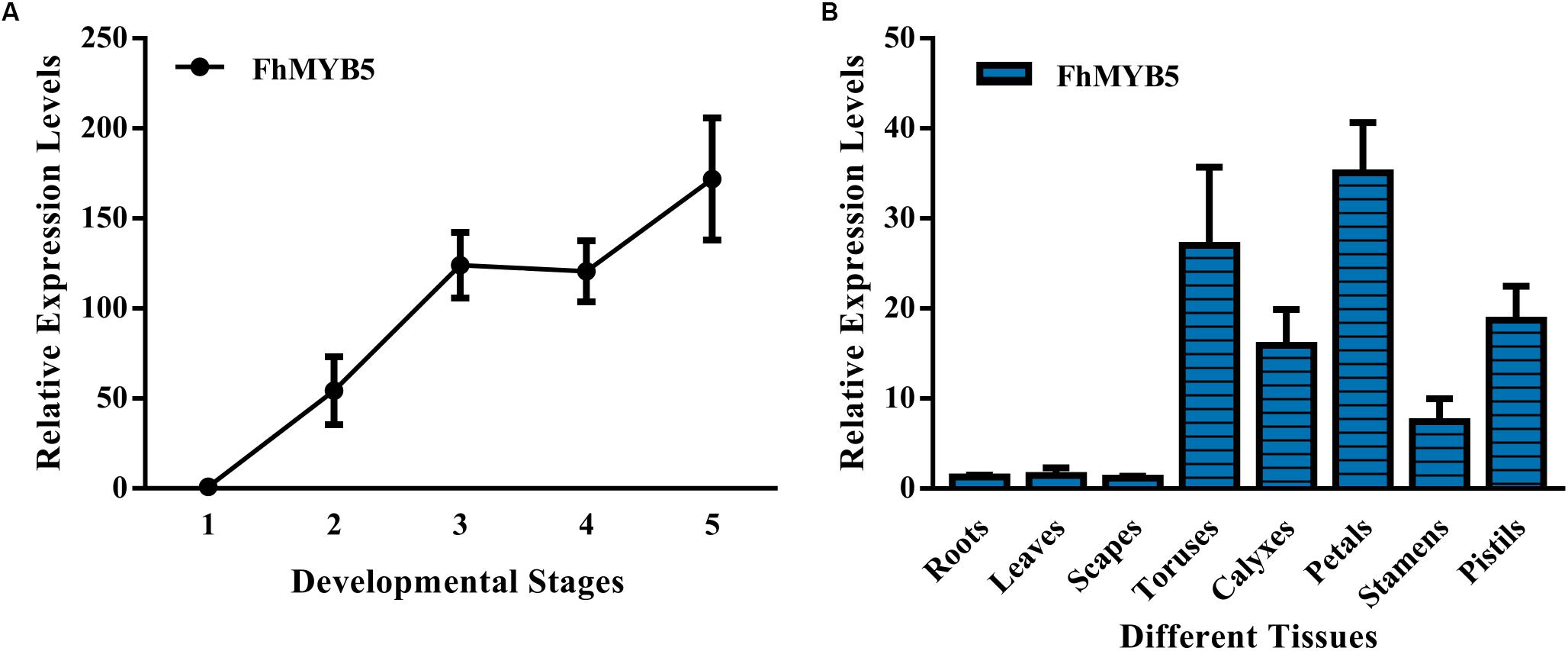
Figure 2. Expression profiles of FhMYB5 in Freesia hybrida. (A) Relative expression levels of FhMYB5 at five developmental stages. Stage 1, unpigmented buds; Stage 2, slightly pigmented buds; Stage 3, pigmented buds; Stage 4, flowers just before complete opening; Stage 5, fully opened flowers. (B) Relative expression levels of FhMYB5 in different tissues. Data represent means ± SD of three biological replicates.
Taken together, these data suggested that the expression of FhMYB5 was associated with anthocyanin and PA accumulation in Freesia. However, the mechanism of how FhMYB5 controls anthocyanin and PA biosynthesis remains unclear.
FhMYB5 Is an Activator and May Function With Co-factors
Transient protoplast transfection assays were conducted to determine the trans-regulation properties of FhMYB5 in Freesia cells. As shown in Figure 3A, protoplasts transformed with GD-FhMYB5 effector construct exhibited a drastic increment in beta-glucuronidase activity compared to protoplasts expressing GD only. As a consequence, FhMYB5 could activate reporter gene in protoplasts and thus was confirmed to be an activator with striking trans-activation property.
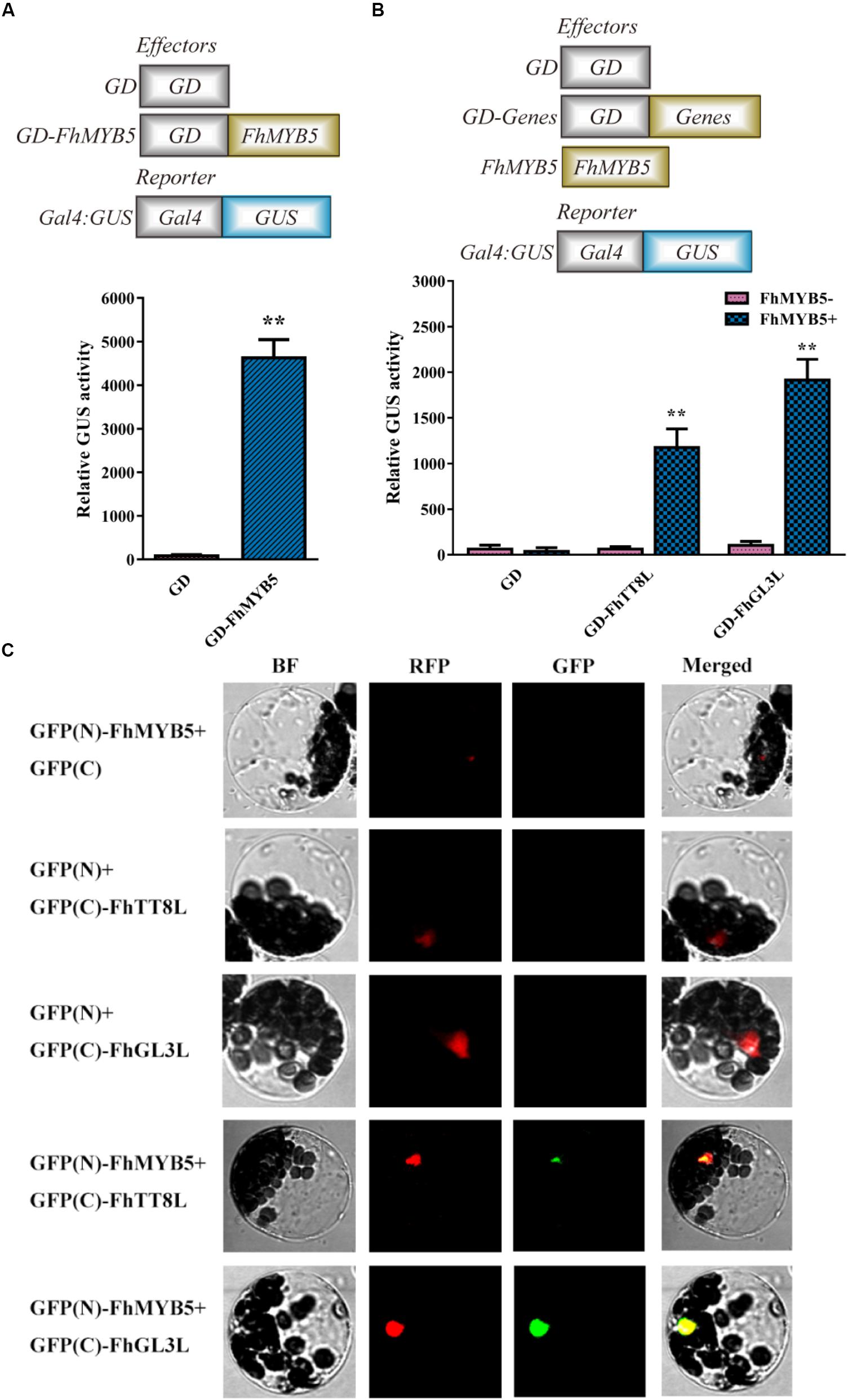
Figure 3. Trans-activation ability of FhMYB5 and cooperative interaction between FhMYB5 and bHLH proteins in transient protoplasts experiments. (A) FhMYB5 showed high trans-activation ability. (B) FhMYB5 could interact with FhTT8L and FhGL3L based on the GAL4-GUS system. Different combinations of effector and reporter constructs (diagrammed at the x-axis) were co-transformed into Freesia protoplasts. After 20–22 h incubation, the GUS activity was measured. The experiments were replicated at least thrice. Data represented means ± SD of three replicates. T-test was carried out to analyze the significant difference (∗ represented P < 0.05; ∗∗ represented P < 0.01). (C) FhMYB5 could interact with FhTT8L and FhGL3L based on the BiFC experiments. Different combinations of constructs were co-transformed into Arabidopsis protoplasts. Moreover, a chimeric red fluorescent protein (RFP) construct containing a nuclear localization signal was included in all the transformation as a positive control. After 20–22 h incubation, the fluorescence was detected. BF, bright field.
In many model plant systems, it has been established that the combinatorial interactions between MYB and bHLH transcription factors within the MBW complex are crucial for the regulation of the flavonoid pathway (Hichri et al., 2011a; Zhou et al., 2012; Jaakola, 2013; Liu et al., 2015; Xu et al., 2015; Zhao and Tao, 2015). Previously, we have characterized two bHLH regulators regulating anthocyanin and PA biosynthesis in Freesia (Li et al., 2016). Thus, a transient protoplasts assay was conducted to assess whether FhMYB5 could interact with FhTT8L or FhGL3L in Freesia cells. As shown in Figure 3B, co-transfection of FhMYB5 effector plasmid and GAL4-GUS reporter construct, together with the bHLH protein FhTT8L or FhGL3L, resulted in notable increase of beta-glucuronidase activity, as compared to the control (GAL4-GUS reporter construct with bHLH factor). Moreover, the interactions between FhMYB5 and FhTT8L or FhGL3L were also confirmed by bimolecular fluorescence complementation (BiFC) assays. As shown in Figure 3C, the green fluorescence could only be detected in combinations of FhMYB5 and FhTT8L or FhGL3L. Altogether, these results demonstrated significant interactions between FhMYB5 and FhTT8L or FhGL3L, implying positive roles of FhMYB5 in anthocyanin and PA biosynthesis.
Overexpression of FhMYB5 in Freesia Affects the General Flavonoid Pathway
To identify the potential target genes of FhMYB5 in Freesia, we analyzed the ability of the protein to activate Freesia genes encoding enzymes of the flavonoid pathway by transient transforming FhMYB5 alone or in combination with FhTT8L or FhGL3L together into Freesia protoplasts. RNA was extracted from the protoplasts and the expression levels of flavonoid biosynthetic genes were evaluated by RT-qPCR. As results shown in Figure 4, FhMYB5 could only slightly activate FhCHI and the late biosynthetic genes including FhDFR1/2, FhDFR3, FhLDOX1 and FhLDOX2 when overexpressed alone in Freesia. However, the co-expression of FhMYB5 and FhbHLH gene could highly up-regulate the expression of the general flavonoid biosynthetic genes, including FhCHS, FhCHI, FhF3H, FhF3’H, FhF3’5’H, FhDFR, and the PA biosynthetic gene FhLAR (changing fold was lower compared with other genes). These results suggested that FhMYB5 might function in the common steps of the flavonoid pathway and PA biosynthetic pathway. Moreover, the anthocyanin or PA contents in Freesia protoplasts were subsequently detected, a slight but significant increase in PA accumulation was observed when FhMYB5 and FhTT8L were co-transfected (Figure 4D). Nevertheless, the short time transient protoplast transfection assays might not be a strong system to supervise the flavonoid accumulations which need a substantial time to be synthesized.
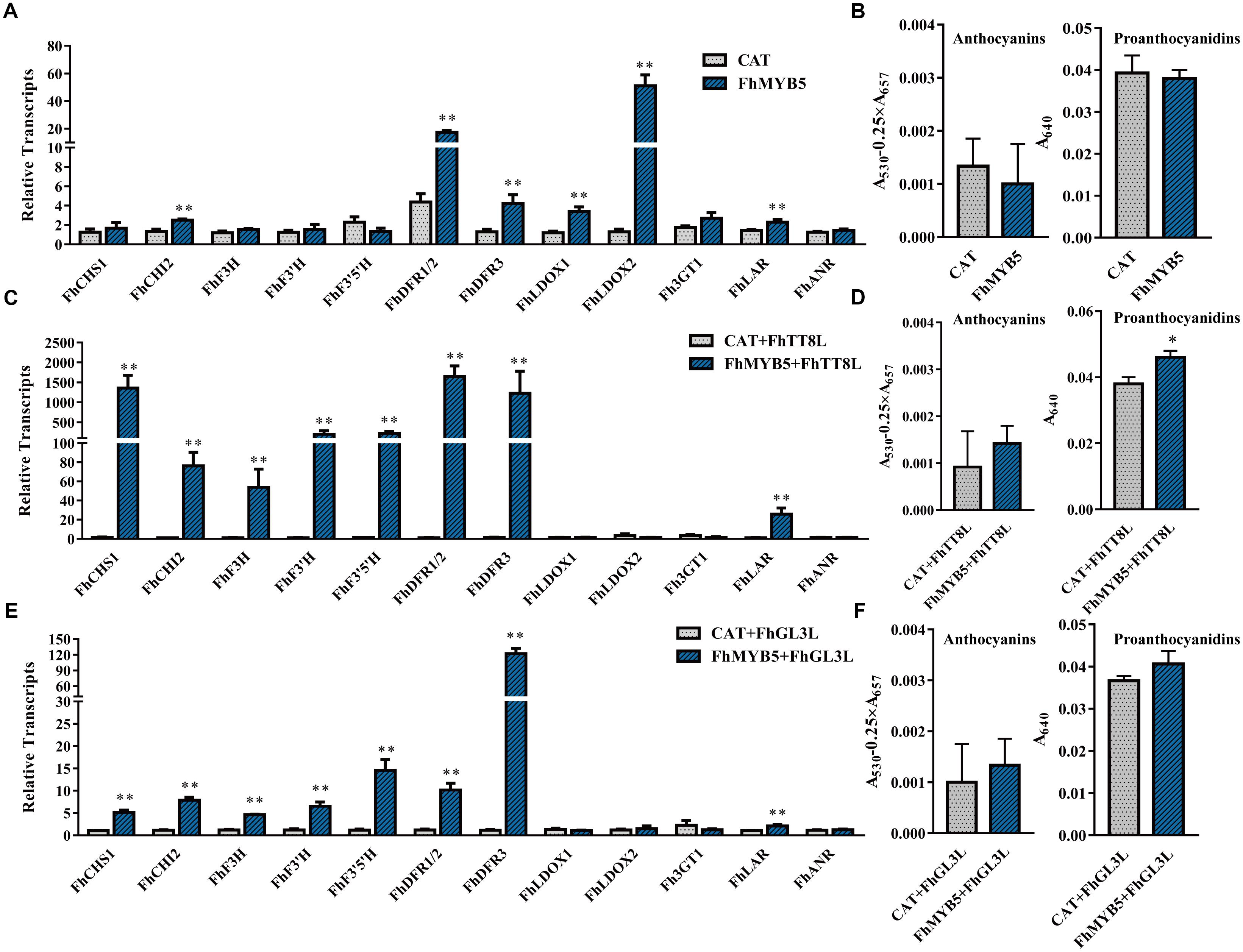
Figure 4. Relative expression levels of flavonoid biosynthetic genes and anthocyanin or PA accumulation in Freesia protoplasts overexpressing FhMYB5. (A) Freesia protoplasts isolated from calli tissues were transfected with FhMYB5, an irrelevant construct CAT (CHLORAMPHENICOL ACETYLTRANSFERASE) was used as negative control. (B) The accumulation of anthocyanin and PA in Freesia protoplasts overexpressing CAT and FhMYB5. (C) Relative gene expression levels in Freesia protoplasts overexpressing CAT and FhTT8L, FhMYB5, and FhTT8L. (D) The accumulation of anthocyanin and PA in Freesia protoplasts overexpressing CAT and FhTT8L, FhMYB5, and FhTT8L. (E) Relative gene expression levels in Freesia protoplasts overexpressing CAT and FhGL3L, FhMYB5, and FhGL3L. (F) The accumulation of anthocyanin and PA in Freesia protoplasts overexpressing CAT and FhGL3L, FhMYB5, and FhGL3L. The protoplasts were incubated in darkness at room temperature for 20∼22 h, and then harvested for RNA extraction or anthocyanin and PA accumulation. Data represented means ± SD of three replicates. T-test was carried out to analyze the significant difference (∗ represented P < 0.05; ∗∗ represented P < 0.01).
To further confirm the ability of FhMYB5 to activate the promoters of Freesia flavonoid biosynthetic genes, we constructed the GUS reporter constructs containing the respective promoter of structural genes. Figure 5 summarized the results obtained with different combinations of FhMYB5 and FhbHLH proteins and indicated that no obvious activation effects of FhMYB5 to the flavonoid biosynthetic genes were found in the absence of FhbHLH co-factors. In contrast, significant inductions of the promoters of flavonoid biosynthetic genes, including FhCHS1, FhCHI2, FhF3H, FhF3’H, FhF3’5’H, FhDFR3 and FhLDOX1, were strikingly detected in protoplasts co-transformed with FhMYB5 and FhTT8L or FhGL3L proteins, which was consistent to the RT-PCR analysis mentioned above. In addition, the promoter of Fh3GT1 could also be slightly activated. Unfortunately, we failed to isolate the promoter of FhLAR after numerous attempts and the promoter of ANR leading to the synthesis of PAs could not be activated by any combinations. Accordingly, FhANR was not highly expressed in PA hyper-accumulation tissues in contrast to FhLAR (unpublished data). These findings further indicated that FhMYB5 could mainly enhance the expression of structural genes from the common steps of the flavonoid pathway.
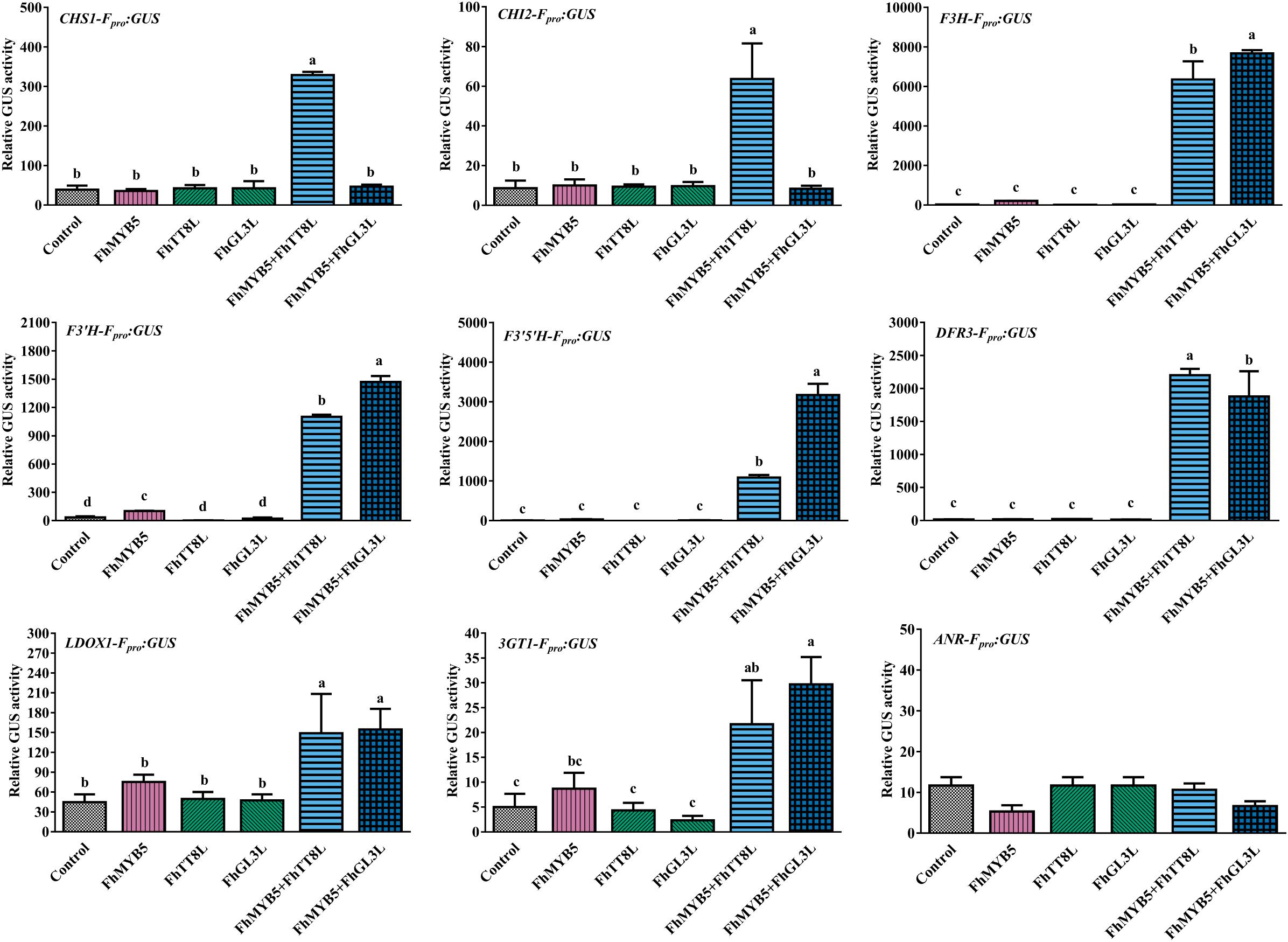
Figure 5. Activation of flavonoid biosynthetic gene promoters by FhMYB5 and FhbHLH regulators. Control indicated the activity of the respective promoter in the absence of FhMYB5 and FhbHLH factors. FhMYB5 was transfected alone or co-transfected with FhTT8L or FhGL3L as effectors. The Arabidopsis protoplasts were incubated in darkness at room temperature for 20–22 h, and then harvested for GUS activity determinant. Each column represented the mean value of three replicates with error bars indicating SD. One-way ANOVA was carried out to compare statistical differences (Ducan, P < 0.05).
Taken together, gene expression assays and promoter activation data indicated that FhMYB5 could mainly activate the expression of genes encoding enzymes of the general flavonoid pathway involved in synthesis of anthocyanins and PAs.
Overexpression of FhMYB5 Impacts on Accumulations of Anthocyanin and Proanthocyanidin in Tobacco and Arabidopsis
To ascertain the putative function for FhMYB5 in planta and investigate whether it is functionally conserved in angiosperms, we firstly employed tobacco, represents of the Asterids plant, as a model system for heterologous expression experiments. Transgenic tobacco plants overexpressing FhMYB5 under the control of the cauliflower mosaic virus 35S promoter showed no obvious differences in vegetative tissues compared to the control lines. However, the most important modifications were observed in stamens which were obviously pigmented in transgenic plants (Figure 6A). Moreover, another observation was the hyper-accumulation of PAs in transgenic lines revealed by the DMACA regent which provided a blue staining when interacted with PAs (Figure 6B). In order to confirm whether the phenotypes of transgenic lines resulted from the expression of the exogenous genes, the presence of FhMYB5 was confirmed by RT-PCR (Figure 6C). Moreover, the relative quantifications of anthocyanin and PA content were accomplished by spectrophotometry detection in different tissues. The most conspicuous increase of anthocyanin content was observed in stamens as expected, while slight increase was also detected in petals (Figure 6D). As for PA accumulation, the increase was almost obvious in all the tissues detected (Figure 6E). Expression analysis performed on RNA extracted from stamens of transgenic and control lines indicated that FhMYB5 could activate the expression of several structural genes associated with the flavonoid pathway, including NtCHS, NtF3H, NtF3’H, NtDFR, NtLDOX, NtUFGT and NtLAR (Figure 6F). In contrast, the structural genes were differently regulated in other tissues of the transgenic plants. An exception was observed for NtLAR which was particularly up-regulated in all the tissues detected (Supplementary Figure S2). As NtLAR synthesized catechins while NtANR synthesized epicatechins, which promoted us to detect whether the PA hyper-accumulation in FhMYB5 overexpressors was resulted from catechins accumulation, further HPLC assays of the stamens were carried out and results indicated that catechins were significantly synthesized (Supplementary Figure S3). To further decipher the mechanism of FhMYB5 in regulating tobacco flavonoid biosynthesis, we carried out additional analyses on the interaction between FhMYB5 and the earlier-characterized flavonoid biosynthesis related bHLH factors using GAL4-based transient protoplast transfection system aforementioned. Interestingly, neither NtAN1 nor NtJAF13 could interact with FhMYB5 (Figure 6G). Taken together, these results indicated the regulatory mechanisms of FhMYB5 might be different with recently characterized anthocyanin or PA related MYB regulators in tobacco per se.
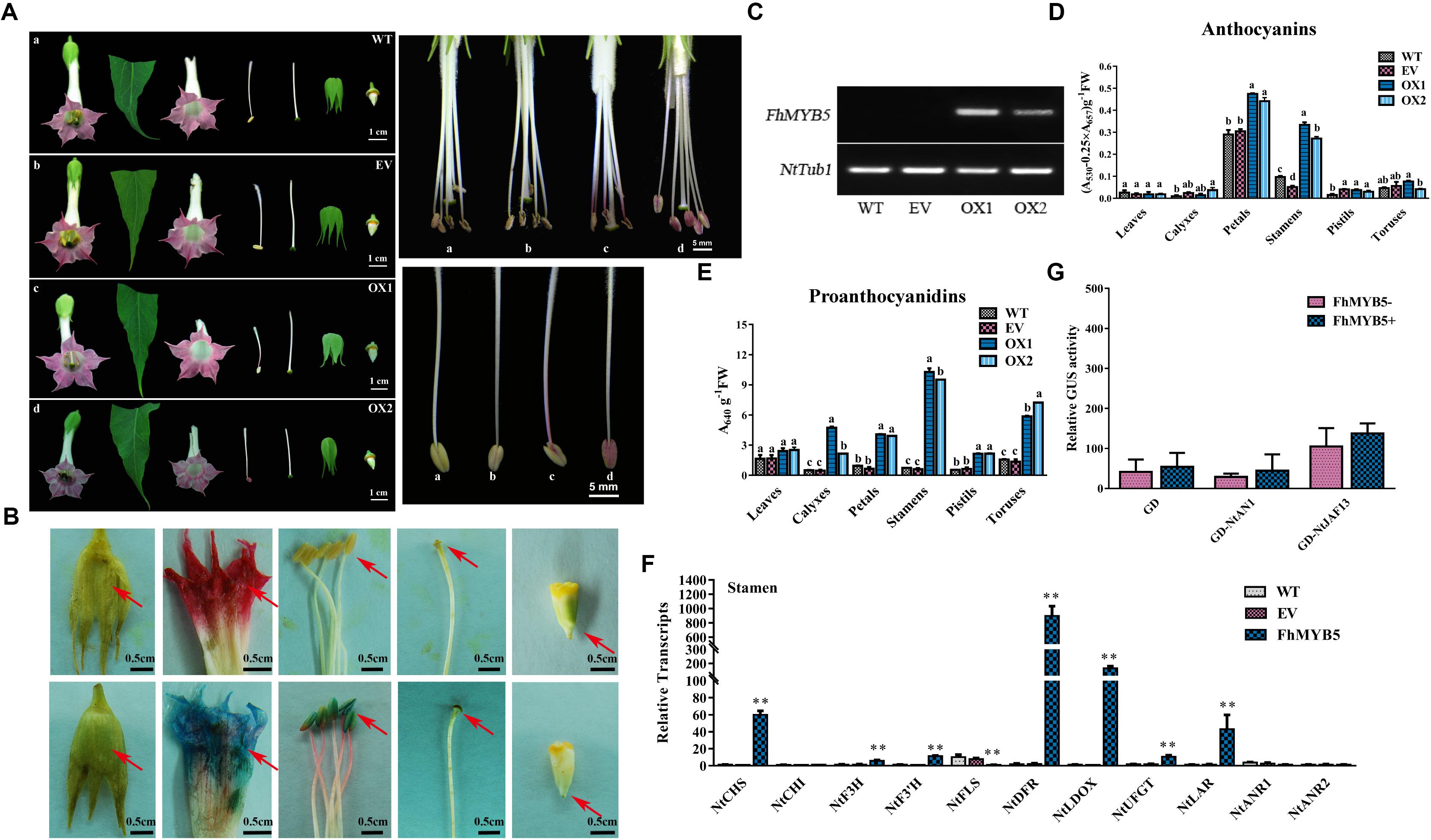
Figure 6. Phenotypic and molecular analysis of transgenic tobacco flowers overexpressing FhMYB5. (A) Flowers of transgenic plants showed an increased pigmentation in stamen compared to control flowers. WT, wild type plant; EV, plant expressing empty vector; OX, plant overexpressing FhMYB5. (B) DMACA staining of flower tissues showed proanthocyanidin accumulation when compared to control lines. (C) RT-PCR indicated the expression of FhMYB5 in transgenic tobacco. (D,E) The detection of anthocyanins and proanthocyanidins in control and transgenic plants. One-way ANOVA was carried out to confirm statistical differences (Ducan, P < 0.05). (F) The relative expression levels of flavonoid genes in tobacco stamen detected by RT-qPCR. The relative expression levels of genes were normalized by tobacco Tubulin. The assays were repeated three times with three biological replicates. Data represented means ± SD of three replicates. T-test was carried out to analyze the significant difference (∗ represented P < 0.05; ∗∗ represented P < 0.01). (G) Transient transfection assays indicated neither NtAN1 nor NtJAF13 could interact with FhMYB5 in Arabidopsis protoplasts. Transformed protoplasts were cultured for 20–22 h at room temperature and harvested for GUS activity determinant. Data represented means ± SD of three replicates. T-test was carried out to analyze the significant difference (∗ represented P < 0.05; ∗∗ represented P < 0.01).
In addition, to further confirm the regulatory function of FhMYB5 in Rosids plants, Arabidopsis was selected to perform the transformation assays. However, no obvious phenotype was observed yet. About 4-week-old Arabidopsis leaves of T1 progeny were selected for measuring anthocyanin and PA content and the results showed that leaves of FhMYB5 overexpressors accumulated more PAs than the control (Figure 7A). Raised transcript levels of flavonoid biosynthetic genes, e.g., AtPAL1, AtPAL2, AtC4H, AtCHS, AtCHI, AtF3H, AtF3’H and AtBAN were revealed by RT-qPCR, implying FhMYB5 had an extensive effect on both EBGs and LBGs (Figure 7B). In addition, significant interactions between FhMYB5 and Arabidopsis bHLH proteins were observed (Figure 7C). We also analyzed the ability of FhMYB5 to activate heterologous promoters using the transient expression assay aforementioned. Resembling the anthocyanin-specific AtPAP1, FhMYB5 is able to activate AtDFR at the presence of bHLH proteins (Figure 7D). In addition, it could also dramatically activate AtBAN promoter either alone or co-transfected with AtTT8 (Figure 7E). However, FhMYB5 appeared to play subordinate roles in the control of the At3GT promoter where the level of activation was very low (Figure 7F). These results reinforced the preliminary conclusion that FhMYB5 appeared to implicate in the control of general flavonoid biosynthesis in Freesia and functioned when heterologously expressed in other plants.
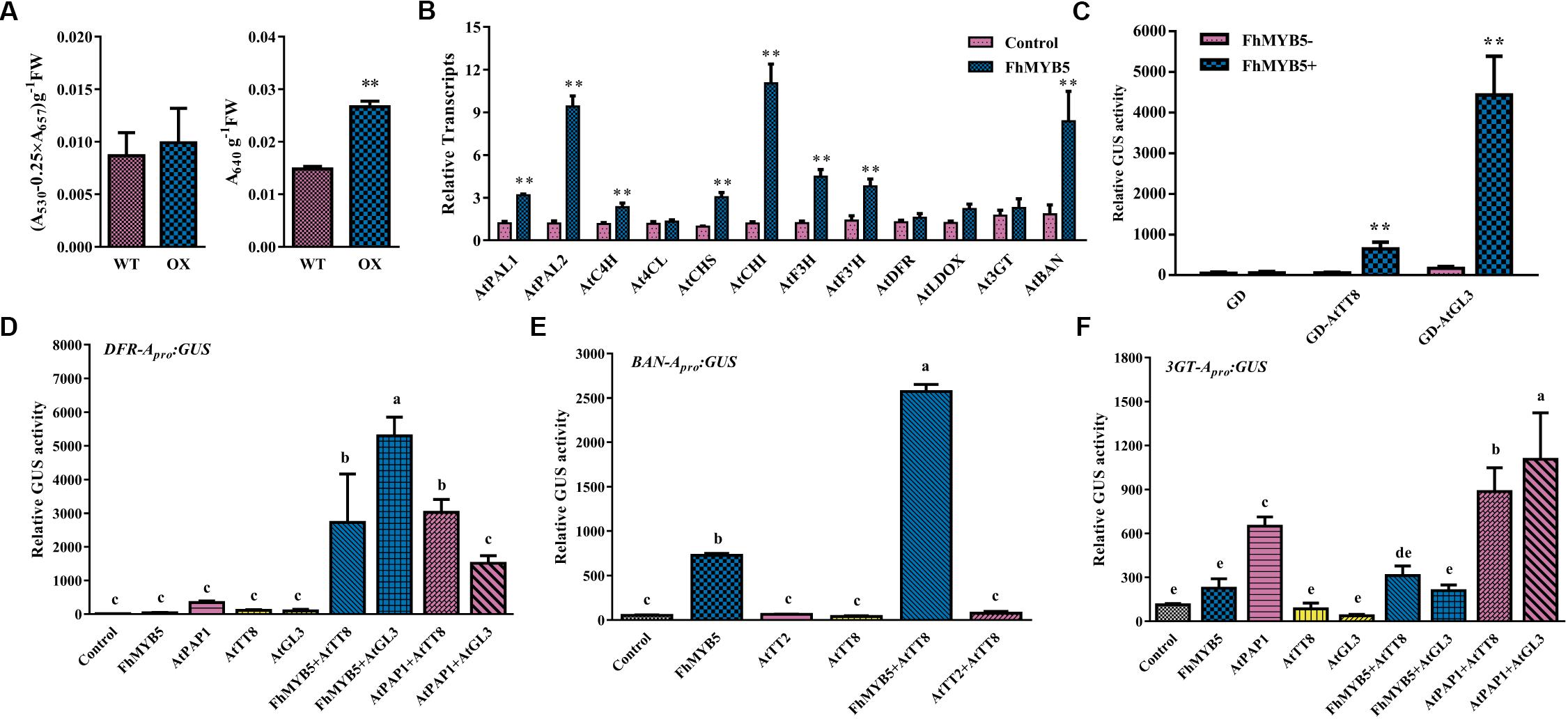
Figure 7. FhMYB5 could interact with Arabidopsis bHLH factors in regulating flavonoid biosynthesis related genes. (A) The contents of anthocyanins and proanthocyanidins in wild type and transgenic plants. T-test was carried out to analyze the significant difference (∗ represented P < 0.05; ∗∗ represented P < 0.01). (B) Gene expression levels of wild type and transgenic Arabidopsis overexpressing FhMYB5. Total RNA was extracted from rosette leaves of 4-week-old Arabidopsis and RT-qPCR was performed to detect gene expression levels. T-test was carried out to analyze the significant difference (∗ represented P < 0.05; ∗∗ represented P < 0.01). (C) FhMYB5 could interact with AtTT8 and AtGL3. GD tagged bHLH proteins were co-transfected into Arabidopsis protoplasts with reporter construct GAL4-GUS in the absence or presence of FhMYB5. T-test was carried out to analyze the significant difference (∗ represented P < 0.05; ∗∗ represented P < 0.01). (D–F) Promoter activation of AtDFR, AtBAN and At3GT in Arabidopsis protoplasts expressing different combinations of effector and reporter constructs. The promoter activation abilities were quantified by relative GUS activities. One-way ANOVA was carried out to compare statistical differences (Ducan, P < 0.05). All the transformed protoplasts were cultured at room temperature for 20–22 h in the darkness. Data represented means ± SD of three replicates.
Discussion
FhMYB5 Belongs to VvMYB5b Subclade
Since the first MYB regulator COLORED1 (C1) was identified in the aleurone of maize kernels, a tremendous amount of MYB proteins have been investigated in numerous plant species such as Arabidopsis thaliana, Zea mays, Petunia hybrida, Oryza sativa, Antirrhinum majus, Populus tremuloides, Vitis vinifera L., and Malus domestica, etc. Four major MYB groups have been recognized based on the number and placement of MYB domains and the R2R3-MYB proteins have been further classified into 28 subgroups according to the conserved amino acid sequence motifs in their C terminus (Stracke et al., 2001; Dubos et al., 2010). The extensive functional characterizations demonstrated that most MYB proteins played central roles in plant-specific processes as a member of regulatory networks controlling development, primary and secondary metabolism and being in response to biotic and abiotic stresses (Dubos et al., 2010).
In this study, we firstly isolated a flavonoid biosynthesis related R2R3-MYB regulator in F. hybrida, designated as FhMYB5, whose protein sequence displayed 68% similarity to AtPAP1. The high levels of similarity were relevant considering the significant similarities within R2R3 domain (Supplementary Table S2). Protein structure and phylogenic analysis could help predict functions of the new found MYB, as primary protein structures and biological functions were usually clustered within the same subgroups. Firstly, the existence of the conserved motif [D/E]Lx2[R/K]x3Lx6Lx3R implied that FhMYB5 might have the ability to interact with bHLH regulators. This was further validated by transient protoplast assay in our study (Figure 3). Actually, it seems that the interaction was indispensable for FhMYB5 in activating the promoters of Freesia genes (Figure 5). Secondly, the conserved C1 motif (Lx3GIDPxTHKPL) closely related to amino acid residues, which were found in the maize transcriptional activator C1, could also be found in FhMYB5. The C1 motif is common in MYB subgroup 4, most of which tend to be negative regulators of phenylpropanoid-derived compound synthesis (Pérez-Díaz et al., 2015; Yoshida et al., 2015). Considering the existence of C1 motif, we arbitrarily regarded FhMYB5 as a phenylpropanoid-related suppressor. However, trans-activation and genetic transformation assays verified that FhMYB5 turned out to be a flavonoid biosynthesis related activator. Moreover, FhMYB5 also contained the conserved motif C3 (DDxF[S/P]SFL[N/D]SLIN[E/D]) which only appeared in VvMYB5b cluster including AtMYB5, OsMYB4, PH4, BNLGHi233, EsMYB9, VvMYB5a and VvMYB5b as described by Deluc et al. (2006; 2008; Huang et al., 2012, 2017). Functionally, the proteins in this cluster seemed to be involved in the control of various processes like cell fate determination in Arabidopsis for AtMYB5 (Li et al., 2009), chilling tolerance in Oryza sativa for OsMYB4 (Vannini et al., 2010), vacuolar acidification in Petunia hybrida for PH4 (Quattrocchio et al., 2006), anthocyanin and flavonol biosynthesis in Epimedium sagittatum for EsMYB9 (Huang et al., 2017), and phenylpropanoid pathway in Vitis vinifera for VvMYB5a and VvMYB5b (Deluc et al., 2006, 2008). Additional data were also provided to indicate the functional conservation between FhMYB5 and VvMYB5b, such as the appearance of the same phenotypic changes in transgenic plants, activation of flavonoid biosynthetic genes, the accumulation of anthocyanin and PA-derived compounds and the promoter activation capacity to induce promoters of the general flavonoid pathway genes. Thus, these functional characterization data reinforced that FhMYB5 was a new member of the VvMYB5b cluster (Figure 1).
FhMYB5 Is Mainly Responsible for the General Flavonoid Genes to Regulate the Biosynthesis of Anthocyanin and Proanthocyanidin
The expression levels of FhMYB5 increased gradually during Freesia flower development and the highest level was detected in petals, which correlated well with anthocyanin accumulation (Li et al., 2016). When overexpressed in Freesia protoplasts, FhMYB5 could highly activate most of flavonoid biosynthetic genes transiently except the anthocyanin specific structural gene Fh3GT1 (Figure 4). This could be partly confirmed by the promoter activation experiment which indicated a lower activation of Fh3GT1 promoter (Figure 5). In grapevine, VvMYB5b was also able to activate several genes of the general pathway, but not the anthocyanin specific UFGT in promoter activation experiments in grape cells (Deluc et al., 2008). In addition to the anthocyanin accumulated tissues, e.g., petal, FhMYB5 was also found to be highly expressed in toruses where PAs were hyper-accumulated, indicating a positive correlation between FhMYB5 and PAs (Figure 2). Generally in plants, PAs are condensed from two kinds of monomers, catechins synthesized by LAR and epicatechin synthesized by ANR (Xie et al., 2003; Bogs et al., 2005; Tsai et al., 2006). The homologs of LAR and ANR were also isolated from Freesia (unpublished data). Transient expression assays indicated that FhMYB5 could only activate FhLAR expression in Freesia cells. However, the increase fold was also significantly lower when compared with the general flavonoid genes. Similar results were also observed in earlier studies about VvMYB5a and VvMYB5b. Though VvMYB5b could activate the expression of VvLAR and VvANR, and VvMYB5a appeared only implicated in VvLAR regulation, the activation levels were low when compared with general flavonoid genes (Deluc et al., 2008). Altogether, it might be reasonable to deduce that FhMYB5 could play roles in the regulation of both anthocyanins and PAs. More precisely, considering the differences of activation levels, FhMYB5 is mainly responsible for the regulation of the general flavonoid genes.
Recently, Uwagaki et al. (2015) has successfully established a method for Agrobacterium-mediated transformation of cormlet-derived calli of F. hybrida. Unfortunately, it usually took 18–24 months from inoculation to generate flowered plantlets and the regenerate rate was low. As the MYB proteins within a given subgroup were quite well conserved across angiosperm species, the in planta function of FhMYB5 in flavonoid biosynthesis was herein further validated in tobacco and Arabidopsis, respectively. As expected, FhMYB5 could mainly induce expression of the general flavonoid genes. Moreover, the gene expression analysis in transgenic tissues of tobacco further consolidated the fact that NtLAR is preferentially the potential target gene of FhMYB5 as NtLAR is up-regulated in all the transgenic tissues (Figure 6 and Supplementary Figure S1). Further analysis of catechin and epicatechin contents also consolidated that FhMYB5 mainly promoted catechin accumulation in tobacco stamens (Supplementary Figure S3). However, as lack of LAR homologs in Arabidopsis, FhMYB5 turned to activate AtBAN expression in transgenic plants or protoplasts alternatively (Figure 7). In addition, previous studies revealed that the AtBAN promoter could hardly be activated unless the co-expression of AtTT2, AtTT8, and AtTTG1 (Baudry et al., 2004). Unexpectedly, FhMYB5 could significantly activate AtBAN either alone or co-expressed with AtTT8, implying a stronger role of FhMYB5 in PA biosynthesis of Arabidopsis. However, there might be other MYB factors participating in Freesia anthocyanin and PA synthesis as Fh3GT1 and FhANR could hardly be activated by FhMYB5. Similarly, besides VvMYB5a and VvMYB5b in grapevine, there were also other MYB regulators including anthocyanin specific VvMYBA1 and VvMYBA2, PA specific VvMYBPA1 and VvMYBPA2, working coordinately on anthocyanin and PA regulation (Kobayashi et al., 2002, 2004; Bogs et al., 2007; Terrier et al., 2009). Actually, we have also cloned three PAP1-like and four TT2-like transcription factors in Freesia but their roles remain to be further unveiled (unpublished data).
The Target Genes of FhMYB5 May Be Affected by Different Co-factors
The ternary complex composed of MYB and bHLH transcription factors, together with WD40 proteins (designated as MBW complex) has been extensively elucidated, and the MYB proteins play critical roles in the determination of cis-elements and thus contribute to the selection of target genes (Hartmann et al., 2005; Park et al., 2010; Jaakola, 2013; Xu et al., 2015). However, more and more studies indicated that the affinity between MYB proteins and cis-element might partly depend on the nature of co-factors.
The results presented in this study indicated that a single gene FhMYB5 might impact not only on one branch of the flavonoid pathway, but on nearly the whole pathway. However, unlike other MYB regulators such as AtPAP1 in Arabidopsis, which appeared to function on the same targets in all vegetative and reproductive tissues when overexpressed, the function of FhMYB5 appeared variable when overexpressed in tobacco, depending on the tissue studied (Supplementary Figure S1) (Zhou et al., 2008; Shi and Xie, 2010). And it seemed that other potential co-factors remaining uncharacterized might exist in tobacco as FhMYB5 could regulate flavonoid biosynthesis independent of NtAN1 or NtJAF13 (Figure 6G). Consequently, it is possible that a specific interacting partner might exist in special tissues and the lack of a specific co-factor might explain the phenotypic differences of different tissues. The phenomena were also observed in earlier studies about VvMYB5a and VvMYB5b (Deluc et al., 2006, 2008). Moreover, as shown in Figure 4, the activation of flavonoid biosynthetic genes by FhMYB5 in promoter activation experiments in Freesia cells largely depended on the bHLH co-factors. This also substantiated the versatile roles of FhMYB5 in planta as it could interact with different co-factors like FhTT8L or FhGL3L or other proteins. Moreover, parameters including cell and tissue type, developmental stage, and environmental conditions could also affect the pattern of MYB regulation. Nevertheless, the functional identification of FhMYB5 in this article underlined the potential of elucidating the mechanisms of flavonoid biosynthesis in F. hybrida and the evolutionary conservation of MYB regulators in angiosperm plants.
Conclusion
In this study, the first MYB regulatory gene FhMYB5 was isolated and performed as candidate gene implicated in flavonoid biosynthesis in Freesia flowers. The results provided substantial proof that FhMYB5 was able to regulate various branches of the flavonoid pathway in Freesia protoplast transient transfection system, as well as in tobacco and Arabidopsis when overexpressed and seemed mainly to be anthocyanin and PA biosynthesis related regulator. However, compared with the relative low activation levels of Fh3GT1 and FhLAR, the strong expression of genes encoding enzymes of the general flavonoid pathway was found, indicating that FhMYB5 might be involved in the control of the general flavonoid pathway.
Author Contributions
YL, XS, LZ, RG, and SY performed most of the experiments. SW analyzed the results. XG designed the experiments, wrote and edited the manuscript. LW designed the experiments and revised the manuscript. All authors read and approved the final manuscript.
Funding
This work was supported by the National Natural Science Foundation of China (31570295), the National Key R&D Program of China (2016YFD0101900), The Program for Introducing Talents to Universities (B07017), and the Fundamental Research Funds for the Central Universities. The funders had no role in study design, data collection and analysis, decision to publish, or preparation of the manuscript.
Conflict of Interest Statement
The authors declare that the research was conducted in the absence of any commercial or financial relationships that could be construed as a potential conflict of interest.
Supplementary Material
The Supplementary Material for this article can be found online at: https://www.frontiersin.org/articles/10.3389/fpls.2018.01935/full#supplementary-material
References
Albert, N. W. (2015). Subspecialization of R2R3-MYB repressors for anthocyanin and proanthocyanidin regulation in forage legumes. Front. Plant Sci. 6:1165. doi: 10.3389/fpls.2015.01165
An, J. P., Qu, F. J., Yao, J. F., Wang, X. N., You, C. X., Wang, X. F., et al. (2017). The bZIP transcription factor MdHY5 regulates anthocyanin accumulation and nitrate assimilation in apple. Hortic. Res. 4:17023. doi: 10.1038/hortres.2017.23
An, X. H., Tian, Y., Chen, K. Q., Liu, X. J., Liu, D. D., Xie, X. B., et al. (2015). MdMYB9 and MdMYB11 are involved in the regulation of the JA-induced biosynthesis of anthocyanin and proanthocyanidin in apples. Plant Cell Physiol. 56:650. doi: 10.1093/pcp/pcu205
Bagchi, D., Sen, C. K., Ray, S. D., Das, D. K., Bagchi, M., Preuss, H. G., et al. (2003). Molecular mechanisms of cardioprotection by a novel grape seed proanthocyanidin extract. Mutat. Res. 523, 87–97. doi: 10.1016/S0027-5107(02)00324-X
Baudry, A., Heim, M. A., Dubreucq, B., Caboche, M., Weisshaar, B., and Lepiniec, L. (2004). TT2, TT8, and TTG1 synergistically specify the expression of BANYULS and proanthocyanidin biosynthesis in Arabidopsis thaliana. Plant J. 39, 366–380. doi: 10.1111/j.1365-313X.2004.02138.x
Bogs, J., Downey, M. O., Harvey, J. S., Ashton, A. R., Tanner, G. J., and Robinson, S. P. (2005). Proanthocyanidin synthesis and expression of genes encoding leucoanthocyanidin reductase and anthocyanidin reductase in developing grape berries and grapevine leaves. Plant Physiol. 139, 652–663.
Bogs, J., Jaffé, F. W., Takos, A. M., Walker, A. R., and Robinson, S. P. (2007). The grapevine transcription factor VvMYBPA1 regulates proanthocyanidin synthesis during fruit development. Plant Physiol. 143, 1347–1361. doi: 10.1104/pp.105.064238
Broun, P. (2005). Transcriptional control of flavonoid biosynthesis: a complex network of conserved regulators involved in multiple aspects of differentiation in Arabidopsis. Curr. Opin. Plant Biol. 8, 272–279. doi: 10.1016/j.pbi.2005.03.006
Clough, S. J., and Bent, A. F. (1998). Floral dip: a simplified method for Agrobacterium -mediated transformation of Arabidopsis thaliana. Plant J. 16, 735–743.
Deluc, L., Barrieu, F., Marchive, C., Lauvergeat, V., Decendit, A., Richard, T., et al. (2006). Characterization of a grapevine R2R3-MYB transcription factor that regulates the phenylpropanoid pathway. Plant Physiol. 140, 499–511. doi: 10.1104/pp.105.067231
Deluc, L., Bogs, J., Walker, A. R., Ferrier, T., Decendit, A., Merillon, J. M., et al. (2008). The transcription factor VvMYB5b contributes to the regulation of anthocyanin and proanthocyanidin biosynthesis in developing grape berries. Plant Physiol. 147, 2041–2053. doi: 10.1104/pp.108.118919
Dubos, C., Stracke, R., Grotewold, E., Weisshaar, B., Martin, C., and Lepiniec, L. (2010). MYB transcription factors in Arabidopsis. Trends Plant Sci. 15, 573–581. doi: 10.1016/j.tplants.2010.06.005
Gao, L., Yang, H., Liu, H., Yang, J., and Hu, Y. (2016). Extensive transcriptome changes underlying the flower color intensity variation in Paeonia ostii. Front. Plant Sci. 6:1205. doi: 10.3389/fpls.2015.01205
Gao, X., Yang, D., Cao, D., Ao, M., Sui, X., Wang, Q., et al. (2010). In vitro micropropagation of freesia hybrida and the assessment of genetic and epigenetic stability in regenerated plantlets. J. Plant Growth Regul. 29, 257–267. doi: 10.1007/s00344-009-9133-4
Gonzalez, A., Zhao, M., Leavitt, J. M., and Lloyd, A. M. (2008). Regulation of the anthocyanin biosynthetic pathway by the TTG1/bHLH/Myb transcriptional complex in Arabidopsis seedlings. Plant J. 53, 814–827. doi: 10.1111/j.1365-313X.2007.03373.x
Grotewold, E., Drummond, B. J., Bowen, B., and Peterson, T. (1994). The myb -homologous P gene controls phlobaphene pigmentation in maize floral organs by directly activating a flavonoid biosynthetic gene subset. Cell 76, 543–553. doi: 10.1016/0092-8674(94)90117-1
Harborne, J. B. (1989). Methods in Plant Biochemistry. Plant Phenolics, Vol. 1. San Diego, CA: Academic Press.
Hartmann, U., Sagasser, M., Mehrtens, F., Stracke, R., and Weisshaar, B. (2005). Differential combinatorial interactions of cis-acting elements recognized by R2R3-MYB, BZIP, and BHLH factors control light-responsive and tissue-specific activation of phenylpropanoid biosynthesis genes. Plant Mol. Biol. 57, 155–171. doi: 10.1007/s11103-004-6910-0
Hichri, I., Barrieu, F., Bogs, J., Kappel, C., Delrot, S., and Lauvergeat, V. (2011a). Recent advances in the transcriptional regulation of the flavonoid biosynthetic pathway. J. Exp. Bot. 62, 2465–2483. doi: 10.1093/jxb/erq442
Hichri, I., Deluc, L., Barrieu, F., Bogs, J., Mahjoub, A., Regad, F., et al. (2011b). A single amino acid change within the R2 domain of the VvMYB5b transcription factor modulates affinity for protein partners and target promoters selectivity. BMC Plant Biol. 11:117. doi: 10.1186/1471-2229-11-117
Hsu, C. C., Chen, Y. Y., Tsai, W. C., Chen, W. H., and Chen, H. H. (2015). Three R2R3-MYB transcription factors regulate distinct floral pigmentation patterning in phalaenopsis orchids. Plant Physiol. 168, 175–191. doi: 10.1104/pp.114.254599
Huang, W., Lv, H., and Wang, Y. (2017). Functional characterization of a novel R2R3-MYB transcription factor modulating the flavonoid biosynthetic pathway from Epimedium sagittatum. Front. Plant Sci. 8:1274. doi: 10.3389/fpls.2017.01274
Huang, W., Sun, W., Lv, H., Xiao, G., Zeng, S., and Wang, Y. (2012). Isolation and molecular characterization of thirteen R2R3-MYB transcription factors from Epimedium sagittatum. Int. J. Mol. Sci. 14, 594–610. doi: 10.3390/ijms14010594
Huang, W., Zeng, S., Xiao, G., Wei, G., Liao, S., Chen, J., et al. (2015). Elucidating the biosynthetic and regulatory mechanisms of flavonoid-derived bioactive components in Epimedium sagittatum. Front. Plant Sci. 6:689. doi: 10.3389/fpls.2015.00689
Jaakola, L. (2013). New insights into the regulation of anthocyanin biosynthesis in fruits. Trends Plant Sci. 18, 477–483. doi: 10.1016/j.tplants.2013.06.003
Jang, H., Ha, U. S., Kim, S. J., Yoon, B. I., Han, D. S., Yuk, S. M., et al. (2010). Anthocyanin extracted from black soybean reduces prostate weight and promotes apoptosis in the prostatic hyperplasia-induced rat model. J. Agric. Food Chem. 58, 12686–12691. doi: 10.1021/jf102688g
Ju, Z., Sun, W., Meng, X., Liang, L., Li, Y., Zhou, T., et al. (2018). Isolation and functional characterization of two 5-O-glucosyltransferases related to anthocyanin biosynthesis from Freesia hybrida. Plant Cell Tissue Organ Cult. 135, 99–110. doi: 10.1007/s11240-018-1447-0
Kobayashi, S., Gotoyamamoto, N., and Hirochika, H. (2004). Retrotransposon-induced mutations in grape skin color. Science 304, 982–982. doi: 10.1126/science.1095011
Kobayashi, S., Ishimaru, M., Hiraoka, K., and Honda, C. (2002). Myb-related genes of the Kyoho grape (Vitis labruscana) regulate anthocyanin biosynthesis. Planta 215, 924–933. doi: 10.1007/s00425-002-0830-5
Koes, R. E., Quattrocchio, F., and Mol, J. N. M. (1994). The flavonoid biosynthetic pathway in plants: function and evolution. Bioessays 16, 123–132. doi: 10.1002/bies.950160209
Kranz, H., Denekamp, M., Greco, R., Jin, H., Leyva, A., Meissner, R. C., et al. (2010). Towards functional characterisation of the members of the R2R3-MYB gene family from Arabidopsis thaliana. Plant J. 16, 263–276. doi: 10.1046/j.1365-313x.1998.00278.x
Lepiniec, L., Debeaujon, I., Routaboul, J.-M., Baudry, A., Pourcel, L., Nesi, N., et al. (2006). Genetics and biochemistry of seed flavonoids. Annu. Rev. Plant Biol. 57, 405–430. doi: 10.1146/annurev.arplant.57.032905.105252
Li, S. F., Milliken, O. N., Pham, H., Seyit, R., Napoli, R., Preston, J., et al. (2009). The Arabidopsis MYB5 transcription factor regulates mucilage synthesis, seed coat development, and trichome morphogenesis. Plant Cell 21, 72–89. doi: 10.1105/tpc.108.063503
Li, Y., Liu, X., Cai, X., Shan, X., Gao, R., Yang, S., et al. (2017). Dihydroflavonol 4-reductase genes from Freesia hybrida play important and partially overlapping roles in the biosynthesis of flavonoids. Front. Plant Sci. 8:428. doi: 10.3389/fpls.2017.00428
Li, Y., Shan, X., Gao, R., Song, Y., Wang, S., Xiang, G., et al. (2016). Two IIIf clade-bHLHs from Freesia hybrida play divergent roles in flavonoid biosynthesis and trichome formation when ectopically expressed in Arabidopsis. Sci. Rep. 6:30514. doi: 10.1038/srep30514
Liu, J., Osbourn, A., and Ma, P. (2015). MYB transcription factors as regulators of phenylpropanoid metabolism in plants. Mol. Plant 8:689. doi: 10.1016/j.molp.2015.03.012
Livak, K. J., and Schmittgen, T. D. (2001). Analysis of relative gene expression data using real-time quantitative PCR and the 2 -ΔΔ C T method. Methods 25, 402–408. doi: 10.1006/meth.2001.1262
Maeda, H., Yamaguchi, T., Omoteno, M., Takarada, T., Fujita, K., Murata, K., et al. (2014). Genetic dissection of black grain rice by the development of a near isogenic line. Breed. Sci. 64, 134–141. doi: 10.1270/jsbbs.64.134
Park, K. I., Ishikawa, N., Morita, Y., Choi, J. D., Hoshino, A., and Iida, S. (2010). A bHLH regulatory gene in the common morning glory, Ipomoea purpurea, controls anthocyanin biosynthesis in flowers, proanthocyanidin and phytomelanin pigmentation in seeds, and seed trichome formation. Plant J. 49, 641–654. doi: 10.1111/j.1365-313X.2006.02988.x
Pérez-Díaz, J. R., Pérez-Díaz, J., Madrid-Espinoza, J., González-Villanueva, E., Moreno, Y., and Ruiz-Lara, S. (2015). New member of the R2R3-MYB transcription factors family in grapevine suppresses the anthocyanin accumulation in the flowers of transgenic tobacco. Plant Mol. Biol. 90, 63–76. doi: 10.1007/s11103-015-0394-y
Petroni, K., and Tonelli, C. (2011). Recent advances on the regulation of anthocyanin synthesis in reproductive organs. Plant Sci. 181, 219–229. doi: 10.1016/j.plantsci.2011.05.009
Pillet, J., Yu, H. W., Chambers, A. H., Whitaker, V. M., and Folta, K. (2015). Identification of candidate flavonoid pathway genes using transcriptome correlation network analysis in ripe strawberry (Fragaria × ananassa) fruits. J. Exp. Bot. 66, 4455–4467. doi: 10.1093/jxb/erv205
Quattrocchio, F., Verweij, W., Kroon, A., Spelt, C., Mol, J., and Koes, R. (2006). PH4 of petunia is an R2R3 MYB protein that activates vacuolar acidification through interactions with basic-helix-loop-helix transcription factors of the anthocyanin pathway. Plant Cell 18, 1274–1291. doi: 10.1105/tpc.105.034041
Schaart, J. G., Dubos, C., Fuente, I. R. D. L., Houwelingen, A. M. M. L., Vos, R. C. H., Jonker, H. H., et al. (2013). Identification and characterization of MYB-bHLH-WD40 regulatory complexes controlling proanthocyanidin biosynthesis in strawberry (Fragaria x ananassa) fruits. New Phytol. 197, 454–467. doi: 10.1111/nph.12017
Shao, Y., Hu, Z., Yu, Y., Mou, R., Zhu, Z., and Beta, T. (2018). Phenolic acids, anthocyanins, proanthocyanidins, antioxidant activity, minerals and their correlations in non-pigmented, red, and black rice. Food Chem. 239:733. doi: 10.1016/j.foodchem.2017.07.009
Shi, M. Z., and Xie, D. Y. (2010). Features of anthocyanin biosynthesis in pap1 - D and wild-type Arabidopsis thaliana plants grown in different light intensity and culture media conditions. Planta 231, 1385–1400. doi: 10.1007/s00425-010-1142-9
Sparkes, I. A., Runions, J., Kearns, A., and Hawes, C. (2006). Rapid, transient expression of fluorescent fusion proteins in tobacco plants and generation of stably transformed plants. Nat. Protoc. 1, 2019–2025. doi: 10.1038/nprot.2006.286
Stracke, R., Ishihara, H., Huep, G., Barsch, A., Mehrtens, F., Niehaus, K., et al. (2007). Differential regulation of closely related R2R3-MYB transcription factors controls flavonol accumulation in different parts of the Arabidopsis thaliana seedling. Plant J. 50, 660–677. doi: 10.1111/j.1365-313X.2007.03078.x
Stracke, R., Werber, M., and Weisshaar, B. (2001). The R2R3-MYB gene family in Arabidopsis thaliana. Curr. Opin. Plant Biol. 4, 447–456. doi: 10.1016/S1369-5266(00)00199-0
Sui, X., Gao, X., Ao, M., Wang, Q., Yang, D., Wang, M., et al. (2011). cDNA cloning and characterization of UDP-glucose: anthocyanidin 3-O-glucosyltransferase in Freesia hybrida. Plant Cell Rep. 30, 1209–1218. doi: 10.1007/s00299-011-1029-7
Sun, W., Liang, L., Meng, X., Li, Y., Gao, F., Liu, X., et al. (2016). Biochemical and molecular characterization of a flavonoid 3-O-glycosyltransferase responsible for anthocyanins and flavonols biosynthesis in freesia hybrida. Front. Plant Sci. 7:410. doi: 10.3389/fpls.2016.00410
Sun, W., Meng, X., Liang, L., Jiang, W., Huang, Y., He, J., et al. (2015). Molecular and biochemical analysis of chalcone synthase from Freesia hybrid in flavonoid biosynthetic pathway. PLoS One 10:e0119054. doi: 10.1371/journal.pone.0119054
Sun, W., Meng, X., Liang, L., Li, Y., Zhou, T., Cai, X., et al. (2017). Overexpression of a Freesia hybrida flavonoid 3-O-glycosyltransferase gene, Fh3GT1, enhances transcription of key anthocyanin genes and accumulation of anthocyanin and flavonol in transgenic petunia (Petunia hybrida). In Vitro Cell. Dev. Biol. Plant. 53, 478–488. doi: 10.1007/s11627-017-9836-3
Terrier, N., Torregrosa, L., Ageorges, A., Vialet, S., Verriès, C., Cheynier, V., et al. (2009). Ectopic expression of VvMybPA2 promotes proanthocyanidin biosynthesis in grapevine and suggests additional targets in the pathway. Plant Physiol. 149, 1028–1041. doi: 10.1104/pp.108.131862
Tsai, C. J., Harding, S., Tschaplinski, T. J., Lindroth, R. L., and Yuan, Y. (2006). Genome-wide analysis of the structural genes regulating defense phenylpropanoid metabolism in Populus. New Phytol. 172, 47–62. doi: 10.1111/j.1469-8137.2006.01798.x
Uwagaki, Y., Matsuda, E., Komaki, M., Murahama, M., Otani, M., Nishizawa, N., et al. (2015). Agrobacterium-mediated transformation and regeneration of Freesia × hybrida. Plant Biotechnol. 32, 165–168. doi: 10.5511/plantbiotechnology.15.0301a
Vannini, C., Locatelli, F., Bracale, M., Magnani, E., Marsoni, M., Osnato, M., et al. (2010). Overexpression of the rice Osmyb4 gene increases chilling and freezing tolerance of Arabidopsis thaliana plants. Plant J. 37, 115–127. doi: 10.1046/j.1365-313X.2003.01938.x:
Wang, X., Wang, X., Hu, Q., Dai, X., Tian, H., Zheng, K., et al. (2015). Characterization of an activation-tagged mutant uncovers a role of GLABRA2 in anthocyanin biosynthesis in Arabidopsis. Plant J. 83, 300–311. doi: 10.1111/tpj.12887
Xie, D. Y., Sharma, S. B., Paiva, N. L., Ferreira, D., and Dixon, R. A. (2003). Role of anthocyanidin reductase, encoded by BANYULS in plant flavonoid biosynthesis. Science 299, 396–399. doi: 10.1126/science.1078540
Xu, W., Dubos, C., and Lepiniec, L. (2015). Transcriptional control of flavonoid biosynthesis by MYB-bHLH-WDR complexes. Trends Plant Sci. 20, 176–185. doi: 10.1016/j.tplants.2014.12.001
Yamagishi, M., Toda, S., and Tasaki, K. (2014). The novel allele of the LhMYB12 gene is involved in splatter-type spot formation on the flower tepals of Asiatic hybrid lilies (Lilium spp.). New Phytol. 201, 1009–1020. doi: 10.1111/nph.12572
Yoo, S. D., Cho, Y. H., and Sheen, J. (2007). Arabidopsis mesophyll protoplasts: a versatile cell system for transient gene expression analysis. Nat. Protoc. 2:1565. doi: 10.1038/nprot.2007.199
Yoshida, K., Ma, D., and Constabel, C. P. (2015). The MYB182 protein down-regulates proanthocyanidin and anthocyanin biosynthesis in poplar by repressing both structural and regulatory flavonoid genes. Plant Physiol. 167, 693–710. doi: 10.1104/pp.114.253674
Zhai, R., Wang, Z., Zhang, S., Meng, G., Song, L., Wang, Z., et al. (2016). Two MYB transcription factors regulate flavonoid biosynthesis in pear fruit (Pyrus bretschneideri Rehd.). J. Exp. Bot. 67, 1275–1284. doi: 10.1093/jxb/erv524
Zhao, D., and Tao, J. (2015). Recent advances on the development and regulation of flower color in ornamental plants. Front. Plant Sci. 6:261. doi: 10.3389/fpls.2015.00261
Zhou, L., Zheng, K., Wang, X., Tian, H., Wang, X., and Wang, S. (2014). Control of trichome formation in Arabidopsis by poplar single-repeat R3 MYB transcription factors. Front. Plant Sci. 5:262. doi: 10.3389/fpls.2014.00262
Zhou, L. L., Shi, M. Z., and Xie, D. Y. (2012). Regulation of anthocyanin biosynthesis by nitrogen in TTG1–GL3/TT8–PAP1-programmed red cells of Arabidopsis thaliana. Planta 236, 825–837. doi: 10.1007/s00425-012-1674-2
Keywords: general flavonoid pathway, MYB transcription factor, tobacco, transient transfection assay, protoplast
Citation: Li Y, Shan X, Zhou L, Gao R, Yang S, Wang S, Wang L and Gao X (2019) The R2R3-MYB Factor FhMYB5 From Freesia hybrida Contributes to the Regulation of Anthocyanin and Proanthocyanidin Biosynthesis. Front. Plant Sci. 9:1935. doi: 10.3389/fpls.2018.01935
Received: 19 September 2018; Accepted: 12 December 2018;
Published: 07 January 2019.
Edited by:
Deyu Xie, North Carolina State University, United StatesReviewed by:
Hiroshi Noguchi, Nihon Pharmaceutical University, JapanAi-Xia Cheng, Shandong University, China
Copyright © 2019 Li, Shan, Zhou, Gao, Yang, Wang, Wang and Gao. This is an open-access article distributed under the terms of the Creative Commons Attribution License (CC BY). The use, distribution or reproduction in other forums is permitted, provided the original author(s) and the copyright owner(s) are credited and that the original publication in this journal is cited, in accordance with accepted academic practice. No use, distribution or reproduction is permitted which does not comply with these terms.
*Correspondence: Li Wang, d2FuZ2xlZTU3QDE2My5jb20= Xiang Gao, Z2FveGlhbmc0MjRAMTYzLmNvbQ==
†These authors have contributed equally to this work