- 1Department of Ecology and Genetics, University of Oulu, Oulu, Finland
- 2Climate laboratory Holt, Department of Arctic and Marine Biology, UiT The Arctic University of Norway, Tromsø, Norway
- 3Norwegian Institute of Bioeconomy Research (NIBIO), Ås, Norway
Ripening of non-climacteric bilberry (Vaccinium myrtillus L.) fruit is characterized by a high accumulation of health-beneficial anthocyanins. Plant hormone abscisic acid (ABA) and sucrose have been shown to be among the central signaling molecules coordinating non-climacteric fruit ripening and anthocyanin accumulation in some fruits such as strawberry. Our earlier studies have demonstrated an elevation in endogenous ABA level in bilberry fruit at the onset of ripening indicating a role for ABA in the regulation of bilberry fruit ripening. In the present study, we show that the treatment of unripe green bilberry fruits with exogenous ABA significantly promotes anthocyanin biosynthesis and accumulation both in fruits attached and detached to the plant. In addition, ABA biosynthesis inhibitor, fluridone, delayed anthocyanin accumulation in bilberries. Exogenous ABA also induced the expression of several genes involved in cell wall modification in ripening bilberry fruits. Furthermore, silencing of VmNCED1, the key gene in ABA biosynthesis, was accompanied by the down-regulation in the expression of key anthocyanin biosynthetic genes. In contrast, the treatment of unripe green bilberry fruits with exogenous sucrose or glucose did not lead to an enhancement in the anthocyanin accumulation neither in fruits attached to plant nor in post-harvest fruits. Moreover, sugars failed to induce the expression of genes associated in anthocyanin biosynthesis or ABA biosynthesis while could elevate expression of some genes associated with cell wall modification in post-harvest bilberry fruits. Our results demonstrate that ABA plays a major role in the regulation of ripening-related processes such as anthocyanin biosynthesis and cell wall modification in bilberry fruit, whereas sugars seem to have minor regulatory roles in the processes. The results indicate that the regulation of bilberry fruit ripening differs from strawberry that is currently considered as a model of non-climacteric fruit ripening. In this study, we also identified transcription factors, which expression was enhanced by ABA, as potential regulators of ABA-mediated bilberry fruit ripening processes.
Introduction
Fleshy fruits and berries have important roles in human health and nutrition, and therefore their ripening regulation have been intensively studied. Development and subsequent ripening of fleshy fruits are complex processes including major metabolic and structural changes, such as accumulation of pigments, flavor and aroma compounds as well as changes in fruit texture. These processes are controlled by a series of signaling events regulated by plant hormones. Fleshy fruits are physiologically defined as either climacteric or non-climacteric according to the differences in respiration rate and production of plant hormone ethylene during ripening (Osorio et al., 2013). A burst of ethylene accompanied by an increase in the respiration rate at the onset of ripening has long been known to be a critical signal controlling ripening of climacteric fruit, such as tomato, mango, melon, apple, and peach. In contrast, ripening mechanisms of non-climacteric fruits, lacking the burst of respiration and ethylene production, have remained less understood (Cherian et al., 2014).
Studies have shown that plant hormone abscisic acid (ABA), in addition to its central role in plant growth and development and in the adaptation to stress conditions (Vishwakarma et al., 2017), is a major regulator of non-climacteric fruit ripening (Cherian et al., 2014; Leng et al., 2014). ABA has been indicated as a ripening promoter in many non-climacteric fruits, such as strawberry (Jia et al., 2011; Li et al., 2011; Kadomura-Ishikawa et al., 2015), grape (Koyama et al., 2010; Jia et al., 2017), sweet cherry (Luo et al., 2014; Shen et al., 2014), cucumber (Wang et al., 2013), citrus (Zhang et al., 2014), pear (Dai et al., 2014), and litchi (Singh et al., 2014) but also for climacteric fruits, such as tomato, peach, melon, and mango (Zhang et al., 2009; Soto et al., 2013; Sun et al., 2013; Zaharah et al., 2013; Mou et al., 2015). The direct molecular level evidence for the role of ABA in fruit ripening was shown in strawberry by suppressing the expression of the key ABA biosynthetic gene, FaNCED1, blocking ABA biosynthesis and leading to partly uncolored strawberry fruits that could be rescued by exogenous ABA (Jia et al., 2011).
Progress has been made during recent years in the understanding of the molecular mechanisms underlying ABA perception and signal transduction during non-climacteric fruit ripening (Li et al., 2011; Cherian et al., 2014; Leng et al., 2014). The ABA receptors were identified by down-regulating the expression of receptors FaPYR1 and FaCHLH/ABAR that led to delay in strawberry fruit ripening and fruit coloring that could not be rescued by exogenous ABA (Chai et al., 2011; Jia et al., 2011). Studies have also identified some ABA-regulated fruit ripening-related transcription factors (TFs) belonging to different gene families, such as MADS, MYB, and bZIP (Daminato et al., 2013; Nicolas et al., 2014; Medina-Puche et al., 2016). However, the ABA-mediated regulatory network promoting non-climacteric fruit ripening still remains poorly understood.
Sugars have traditionally been considered as a carbon and energy source for plants, and in fruits sugars have been thought merely to affect fruit quality. A growing number of studies have indicated that sugars, such as glucose and sucrose, can act as signaling molecules and possess hormone-like signaling functions in plant development and stress responses (Van den Ende and El-Esawe, 2014; Huang et al., 2016). Especially sucrose-specific signaling pathway has been proposed in the regulation of anthocyanin biosynthesis, and in Arabidopsis anthocyanin biosynthesis was shown to be up-regulated by sucrose (Solfanelli et al., 2006; Loreti et al., 2008). Fruit ripening signals have been studied extensively in strawberry, which is currently considered as a model of non-climacteric fruit ripening (Li et al., 2011; Cherian et al., 2014). In strawberry, studies have demonstrated that especially sucrose but also glucose promotes fruit ripening (Jia et al., 2011, 2013a,b). Currently, sucrose in co-operation with ABA are indicated as the core signaling molecules regulating strawberry fruit ripening (Jia et al., 2011, 2013b). The coordinated regulation of fruit ripening by ABA and sucrose has recently been suggested also for grapes (Jia et al., 2017).
Bilberry (Vaccinium myrtillus L.) is one of the most abundant wild berries in the Northern Europe and it is valued for its nutraceutical and health-beneficial properties (Kolehmainen et al., 2012; Jimenez-Garcia et al., 2013; Törrönen et al., 2013). Ripening of non-climacteric bilberry fruit is characterized by a high accumulation of health-beneficial anthocyanins both in peel and flesh providing deep blue color to the ripe fruits. Anthocyanins are biosynthesized via the well-known phenylpropanoid/flavonoid pathway consisting of a number of enzymatic steps that catalyze a sequential reaction leading to the production of different anthocyanin classes (Supplementary Figure S1). Our earlier studies have identified altogether 33 different anthocyanins in bilberry fruits belonging to delphinidin, cyanidin, petunidin, peonidin, and malvidin classes (Zoratti et al., 2014).
So far, there are no studies concerning the role of ABA or sugars on the regulation of fruit ripening and anthocyanin biosynthesis in bilberry fruit. Furthermore, earlier studies have given contradictory results on the role of ABA in the anthocyanin accumulation in other Vaccinium species (Percival and MacKenzie, 2007; Forney et al., 2009; Buran et al., 2012; Oh et al., 2018). In our previous study, an increase in endogenous ABA level accompanied by an increase in the expression of VmNCED1, the key gene in ABA biosynthesis, was demonstrated at the onset of bilberry fruit ripening suggesting a role for ABA in bilberry ripening regulation (Karppinen et al., 2013).
The aim of the current study was to examine the role of ABA and various sugars on bilberry fruit ripening and ripening-related processes. For this purpose, the effect of exogenous ABA and sugars on bilberry fruit ripening and anthocyanin accumulation was examined in both pre- and post-harvest experiments. The effects of the post-harvest treatments on the expression of the key genes in anthocyanin, ABA and sucrose biosynthesis as well as the expression of the genes encoding major cell wall modifying enzymes was studied. The role of ABA in anthocyanin biosynthesis was further examined by silencing VmNCED1 in ripening bilberry fruits. Finally, we also identified potential TFs in ABA-regulated bilberry fruit ripening processes.
Materials and Methods
Plant Material
Bilberry (V. myrtillus L.) plant material used for the experiments was originated from the natural forest stands in Oulu (65°01′ N, 25°28′ E) and Tromsø (69°42′ N, 18°51′ E). For the virus-induced gene silencing (VIGS) experiments, bilberry plants with their root system were harvested at the stage when fruits were unripe and green. The plants were placed in boxes (50 cm × 70 cm) with forest peat soil and watered well. The five developmental stages of bilberry fruit were collected as described earlier (Karppinen et al., 2013).
Pre-harvest Treatments With ABA and Sugars
In order to study the effect of exogenous ABA on bilberry fruits still attached to plants, an experiment was conducted on field conditions with bilberries growing on the natural forest stand in Oulu, Finland July 2014. For the treatments, ABA [(±)-abscisic acid; Sigma, St. Louis, MO, United States] at concentrations of 0.5 and 2 mM with 0.5% (v/v) Tween 20 were utilized. A solution containing water with 0.5% (v/v) Tween 20 was used as a control treatment. The solutions were applied individually on unripe green bilberry fruits by spraying until run-off with a hand-held sprayer on alternate days for 6 days (three times) in the late afternoon to minimize ABA photo-degradation. Approximately 50 berries were utilized per treatment with four replicates by employing around 15 m2 areas adjacent to each other. Berries were evaluated for their color 7 days from the beginning of the first treatment. Berry samples were collected after 0, 24, 48, 96, and 168 h (7 days) from the first treatment, immediately frozen in liquid nitrogen and stored at -80°C until used for RNA extraction and determination of anthocyanin content.
The effect of glucose and sucrose on bilberry fruits still attached to plants was studied on field conditions with bilberries growing on the natural forest stand in Tromsø, Norway August 2015. Sucrose and glucose at concentration of 200 mM with 0.5% (v/v) Tween 20 were used. A solution containing water with 0.5% (v/v) Tween 20 was used as a control treatment. The solutions were applied individually on unripe green bilberry fruits similarly as described above by spraying until run-off with a hand-held sprayer on alternate days for 6 days (three times). Approximately 50 berries per treatment were utilized with four replicate areas as described above. When obvious induction in berry ripening was not detected after 7 days from the first treatment, the treatments were repeated (three times on alternate days). Berries were evaluated for their color and collected after 0, 7, and 19 days from the beginning of the first treatment, immediately frozen in liquid nitrogen and stored at -80°C until used for the determination of anthocyanin content.
Post-harvest Treatments With ABA and Sugars
For studying the effect of ABA and sugars on detached bilberry fruits, fruits at unripe green stage were harvested from natural forest stand in Oulu, Finland July 2017. Fruits of similar size and color with absence of physical injuries or insect infections were selected for the experiment. The experiment was set-up aseptically under a laminar flow. After rinsing the fruits three times with sterile distilled water, the fruits were randomly divided and immersed with their pedicels (Roubelakis-Angelakis and Kliewer, 1986; Jia et al., 2013a) into the following filter sterilized solutions in sterile Petri plates: 0.5 and 2 mM ABA [(±)-abscisic acid; Sigma], 50 and 200 mM sucrose (VWR International, Lutterworth, United Kingdom), 50 and 200 mM glucose (Sigma), 50 and 200 mM fructose (Merck, United States), 200 μM fluridone (Sigma), 0.5 mM ABA + 200 mM sucrose, and water (control). All solutions contained 0.5% (v/v) Tween 20. Three replicate Petri plates with approximately 50 berries per plate were employed. The plates were placed at 18°C under 30 μmol m-2 s-1 light. The berries were evaluated for their color on the 4th and 6th day from the beginning of the experiment. Berry samples were collected after 0, 24, 48, 96, and 144 h (6 days) from the beginning of the experiment, immediately frozen in liquid nitrogen and stored at -80°C until used for RNA extraction and determination of anthocyanin content.
Construction of VmNCED1 VIGS Vector and Agrobacterium-Mediated Infiltration
A 165 bp cDNA fragment of VmNCED1 (GenBank accession no. JX982599) was PCR-amplified using forward primer 5′-GGATCCCGATCAGCAAGTGGTGTTTA-3′ (BamH1 site is underlined) and reverse primer 5′-TGGAAGCTTAATGTATCCGGACACTCG-3′ (HindIII site is underlined) under standard PCR conditions. The PCR product was gel-purified, digested with BamHI and HindIII and ligated into pTV00 vector. The resulting pTV00-VmNCED1 vector was confirmed by sequencing and transformed into Agrobacterium tumefaciens strain GV3101 by the freeze-thaw method.
Agrobacterium-mediated infiltration by syringe injection with a needle into bilberry fruits was performed as described earlier by Jaakola et al. (2010). Briefly, a 5 ml cultures of Agrobacterium strain GV3101 containing pTV00-VmNCED1 and strain C58c1 containing pBINTRA6 were grown overnight at 28°C in liquid Luria-Bertani (LB) medium (pH 5.6) with appropriate antibiotics. The overnight cultures were inoculated into 50 ml of LB medium containing 10 mM MES, 20 μM acetosyringone and appropriate antibiotics and grown at 28°C until the OD600 of the cultures reached 1.0–1.3. The cells were collected by centrifugation (3500 rpm, 5 min, 20°C), resuspended in infiltration buffer (10 mM MgCl2, 10 mM MES, 200 μM acetosyringone) to a OD600 of approximately 1.5 and incubated at room temperature at least for 2 h. Agrobacterium mixture containing pTV00-VmNCED1 and pBINTRA6 (1:1 ratio) was injected into unripe green bilberry fruits at two spots on the same side of the berry by a 1-ml syringe with a needle. As a control, only Agrobacterium with pBINTRA6 was injected into the fruits. The bilberry plants were placed at 18°C with 60% humidity and 125 μmol m-2 s-1 light intensity. Fruits were evaluated 4 weeks after injection, then frozen in liquid nitrogen and stored at -80°C until used for RNA extraction.
Isolation of RNA and cDNA Preparation
Total RNA was isolated from berries according to the method described earlier for bilberry (Jaakola et al., 2001). The cDNA was synthesized from the total RNA by using SuperScript III reverse transcriptase (Invitrogen, Carlsbad, CA, United States) according to the manufacturer’s instructions. The cDNA was purified from the contaminating genomic DNA by using the method described by Jaakola et al. (2004).
Relative Quantification of Gene Expression
Real-time quantitative reverse transcription PCR (qRT-PCR) analyses were performed with a LightCycler 480 instrument and software (Roche Applied Sciences, Indianapolis, IN, United States). The transcript abundance of the genes was detected using a LightCycler® SYBR Green I Master qPCR Kit (Roche). The qRT-PCR conditions were an initial incubation at 95°C for 10 min followed by 40 cycles at 95°C for 10 s, 60°C for 20 s, and 72°C for 10 s. The studied genes we identified from the publicly available Vaccinium transcriptome databases. The gene-specific primer sequences used for the qRT-PCR analyses are listed in Supplementary Table S1. Glyceraldehyde-3-phosphate dehydrogenase (VmGAPDH; GenBank accession no. AY123769) was employed as a reference gene for the relative quantification of the PCR products. The results were calculated with LightCycler® 480 software (Roche), using the calibrator-normalized PCR efficiency-corrected method (Technical note No. LC 13/2001, Roche). The amplification of only one product in qRT-PCR was confirmed by a melting curve analysis.
Determination of Total Anthocyanins
Frozen berries were ground to fine powder with a mortar and pestle in the presence of liquid nitrogen. Berry powder of 0.1 g was extracted with methanol acidified with 0.1% HCl (v/v) by sonication in the dark for 10 min followed by shaking at room temperature in the dark for 1 h. After centrifugation, the supernatant was collected and the total anthocyanin content was determined according to the pH differential method (Lee et al., 2005, 2008) that has been tested for bilberry material (Dandena et al., 2012). Analyses were performed with three to four biological replicates. The results were expressed as mg (cyanidin-3-glucoside equivalent) g-1 fresh weight.
Statistical Analysis
The quantitative results of gene expression and measurements of anthocyanins in bilberry fruits were analysed either with Student’s t-Test or one-way analysis of variance (ANOVA) followed by Tukey’s HSD test by using SPSS Statistics program, version 25 (IBM, New York, NY, United States).
Results
Effect of Pre-harvest Treatments With ABA and Sugars on Bilberry Fruit Ripening and Anthocyanin Accumulation
To investigate the role of ABA on bilberry fruit ripening and anthocyanin accumulation, exogenous ABA was sprayed three times on alternate days on unripe green bilberry fruits still attached to plants. Seven days after the first treatment with 0.5 mM ABA, and especially with 2 mM ABA, most of the fruits had turned red/blue indicating fruit ripening and anthocyanin accumulation while most of the control fruits treated with water were still green (Figure 1A). The anthocyanin content was significantly higher at day seven in ABA treated fruits compared to control fruits sprayed with water (Figure 1B). Also, both the ABA treatments up-regulated the expression of the anthocyanin biosynthetic genes VmCHS, VmANS, and VmUFGT during the 7 days experiment (Figure 1C).
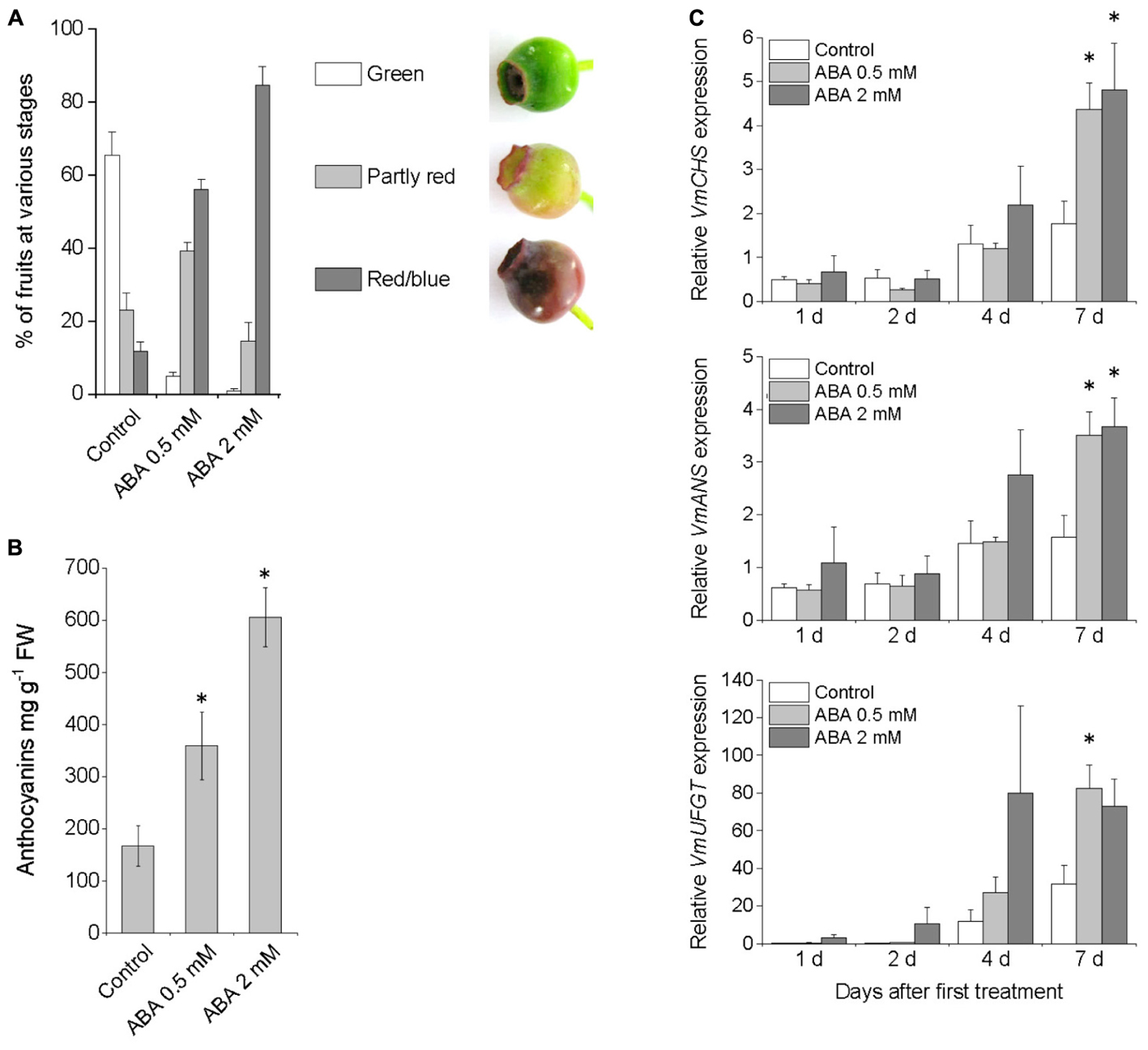
FIGURE 1. Effect of pre-harvest treatment with ABA on bilberry fruit color (A), anthocyanin content (B), and expression of anthocyanin biosynthetic genes (C). Unripe green berries attached to plants were sprayed with 0.5 mM ABA, 2 mM ABA or water (control). Fruit color and anthocyanin content was evaluated after 7 days from the beginning of the experiment. Total anthocyanin content is expressed as milligrams of cyanidin-3-glucoside equivalents g-1 FW. Relative expression of the genes was quantified by qRT-PCR and normalized to VmGAPDH. Values represent means ± SEs of four replicates. Asterisks indicate significant differences from control in Student’s t-Test (P ≤ 0.05).
Glucose and sucrose were similarly applied by spraying on attached unripe green bilberry fruits to investigate their effect on bilberry fruit ripening and anthocyanin accumulation. Seven days after the beginning of the experiment, the fruits were still green in color and neither 200 mM glucose nor 200 mM sucrose had induced significant changes in the fruit anthocyanin content compared to control fruits treated with water (Figure 2A). Therefore, the treatments were repeated second time by again spraying berries with sugars three times on alternate days. After 19 days of the first treatment, there were only slightly more red/blue berries in the sugar treatments compared to control berries treated with water (Figure 2B). There were no significant differences between the treatments in the anthocyanin content of the berries at day 19, however, there was a high variation between individual berries in the response to sucrose (Figure 2A).
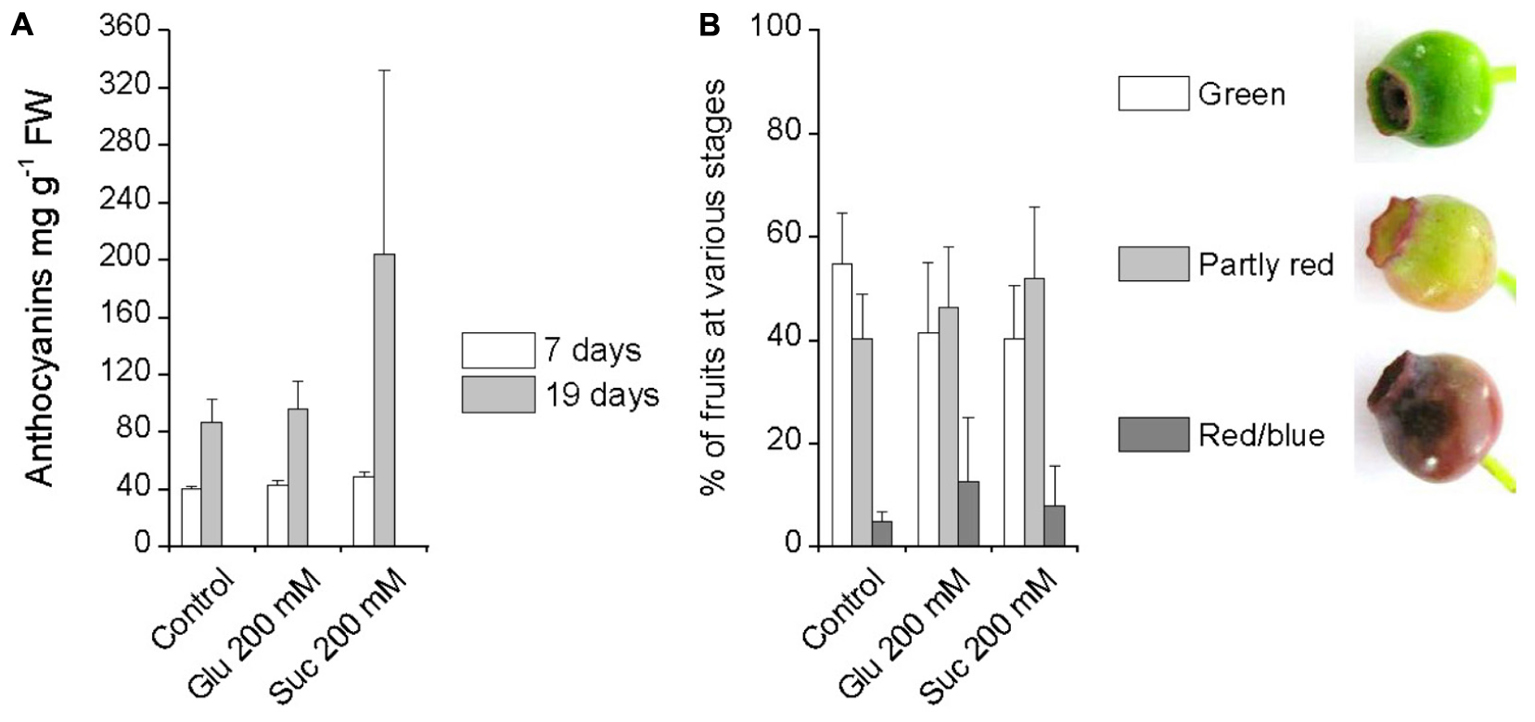
FIGURE 2. Effect of pre-harvest treatments with glucose and sucrose on bilberry fruit anthocyanin content (A) and fruit color (B). Unripe green berries attached to plants were sprayed with 200 mM glucose, 200 mM sucrose or water (control). Total anthocyanin content in fruits was measured after 7 and 19 days from the beginning of the experiment and is expressed as milligrams of cyanidin-3-glucoside equivalents g-1 FW. Fruit color was evaluated after 19 days from the beginning of the experiment. Values represent means ± SEs of four replicates.
Effect of Post-harvest Treatments With ABA and Sugars on Bilberry Fruit Ripening and Anthocyanin Accumulation
To examine in more detail the role of ABA and sugars on bilberry fruit ripening and anthocyanin accumulation and verify the results attained with attached fruits, unripe green bilberry fruits were harvested and submerged into various post-harvest treatments in Petri plates. The treatments were: ABA (0.5 and 2 mM), glucose (50 and 200 mM), fructose (50 and 200 mM), sucrose (50 and 200 mM), 0.5 mM ABA + 200 mM sucrose, 200 μM fluridone (ABA biosynthesis inhibitor) or water (control). An increase in red coloration in bilberry fruits treated with 2 mM ABA was obvious already after 1 day from the beginning of the treatment. After 4 days, all the fruits in 2 mM ABA treatment and most of the fruits in 0.5 mM ABA and 0.5 mM ABA + 200 mM sucrose treatments had obtained red coloration indicating anthocyanin accumulation (Figures 3A,B). The fruits treated with water (control), fluridone or different types and concentrations of sugars were still mostly unripe and green with only few individual berries obtained some red coloration (Figures 3A,B). The berries treated with either 0.5 mM or 2 mM ABA had significantly higher levels of anthocyanins compared to control berries in water after 4 days from the beginning of the experiment (Figure 3C). The treatment either with glucose, fructose or sucrose, except 50 mM glucose, did not significantly increase the anthocyanin content in bilberry fruits (Figure 3C). The increase with 50 mM glucose was due to increase in anthocyanin accumulation in few individual berries and the increase was not seen with 200 mM glucose. Berries treated with fluridone had significantly lower level of anthocyanins compared to control fruits in water (Figure 3C). Furthermore, the fruits treated with 0.5 mM ABA + 200 mM sucrose did not have significantly higher anthocyanin level compared to the berries treated only with 0.5 mM ABA, suggesting that sucrose does not significantly enhance the effect of ABA in bilberry anthocyanin accumulation (Figure 3C).
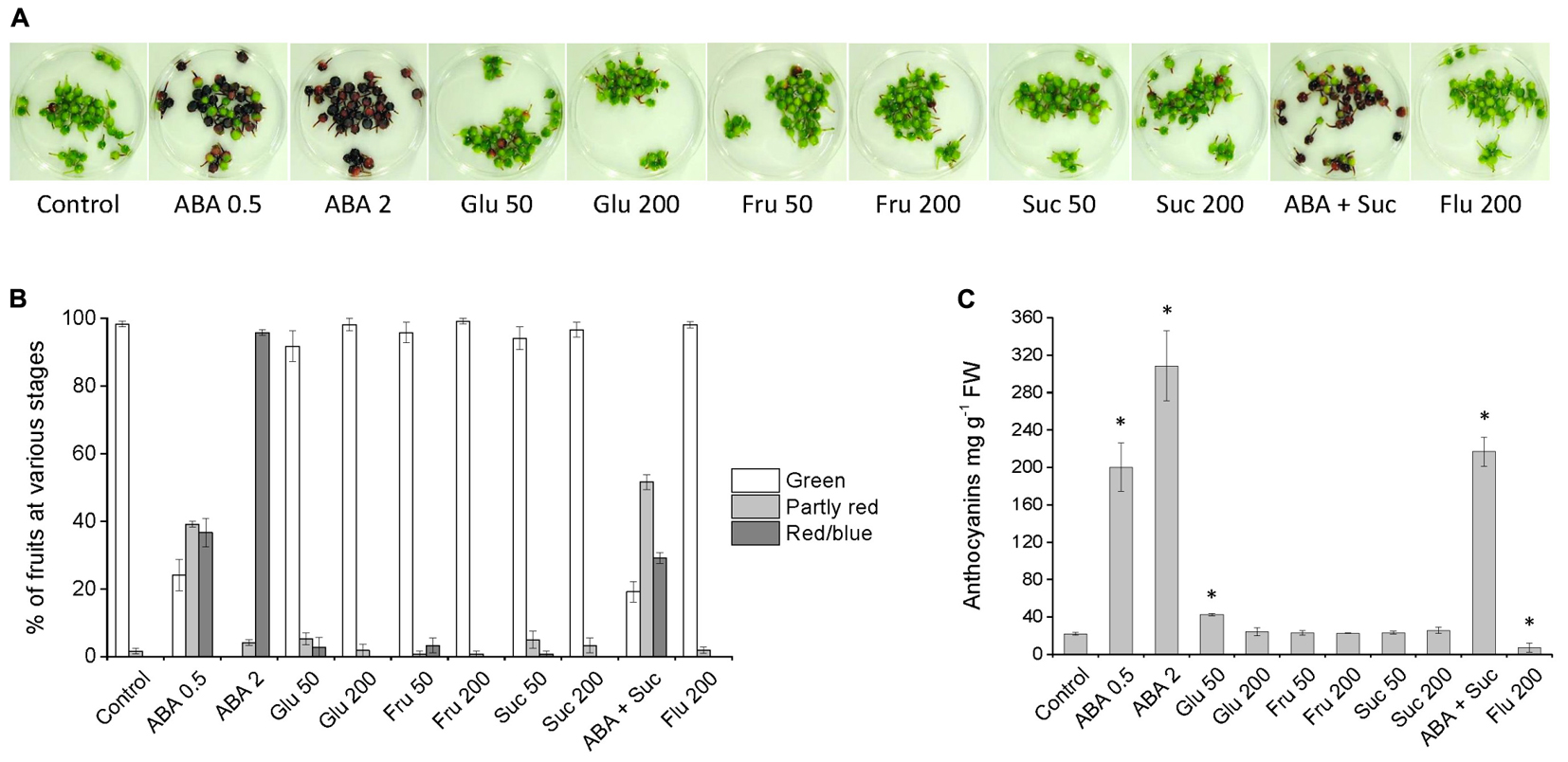
FIGURE 3. Effect of post-harvest ABA and sugar treatments on bilberry fruit color and anthocyanin accumulation. Detached unripe green berries were immersed into solutions containing ABA (0.5 and 2 mM), glucose (50 and 200 mM), fructose (50 and 200 mM), sucrose (50 and 200 mM), 0.5 mM ABA + 200 mM sucrose, 200 μM fluridone or water (control). After 4 days from the beginning of the experiment, fruits in Petri plates (A) were evaluated for their color (B) and measured for their anthocyanin content (C). Total anthocyanin content is expressed as milligrams of cyanidin-3-glucoside equivalents g-1 FW. Values represent means ± SEs of three replicates. Asterisks indicate significant differences from control in Student’s t-Test (P ≤ 0.05).
After 6 days from the beginning of the experiment, almost all the ABA treated berries had turned fully red/blue while most of the berries in the treatment with water (control), fluridone or sugars were still unripe and green (Supplementary Figure S2). None of the berries in fluridone treatment had reached the fully red/blue coloration. The anthocyanin content in berries treated with 0.5 mM ABA had highly increased from day 4. Instead, the anthocyanin accumulation in berries in 2 mM ABA treatment had slowed down from day 4 and the anthocyanin content was significantly lower in berries in 2 mM ABA compared to berries in 0.5 mM ABA treatment (Supplementary Figure S2) indicating over-ripening of the berries in 2 mM ABA treatment and cease in anthocyanin biosynthesis.
Expression of Anthocyanin Biosynthetic Genes in Response to Post-harvest Treatments With ABA and Sugars
The transcript levels of anthocyanin biosynthetic genes (Supplementary Figure S1) were examined by qRT-PCR in the detached berries during 4 days in different treatments in Petri plates. Significant induction in the expression of all anthocyanin biosynthetic genes was detected in berries in ABA treatments indicating a major role for ABA as a positive regulator of bilberry anthocyanin biosynthesis (Figure 4). Especially the transcripts levels of VmCHS, VmF3H, VmF3′H, VmF3′5′H, VmANS, and VmUFGT were significantly elevated (P ≤ 0.001) by both ABA treatments at day 4 and 2 mM ABA treatment already at day 2. For example, after 4 days in 2 mM ABA treatment the up-regulation of VmCHS, VmF3′5′H, and VmUFGT were 180-, 460-, and 850-fold, respectively, compared to water control. Instead, although slight elevation in gene expression was detected in sugar treatments at day 2 and 4 compared to control fruits, no obvious induction in the expression of the anthocyanin biosynthetic genes in fruits was detected (Figure 4). In fact, the expression of the anthocyanin biosynthetic genes was more or less decreased during the 4 days in sugar treatments indicating that sugars have less obvious positive signaling role to induce bilberry anthocyanin biosynthesis compared to ABA (Figure 4).
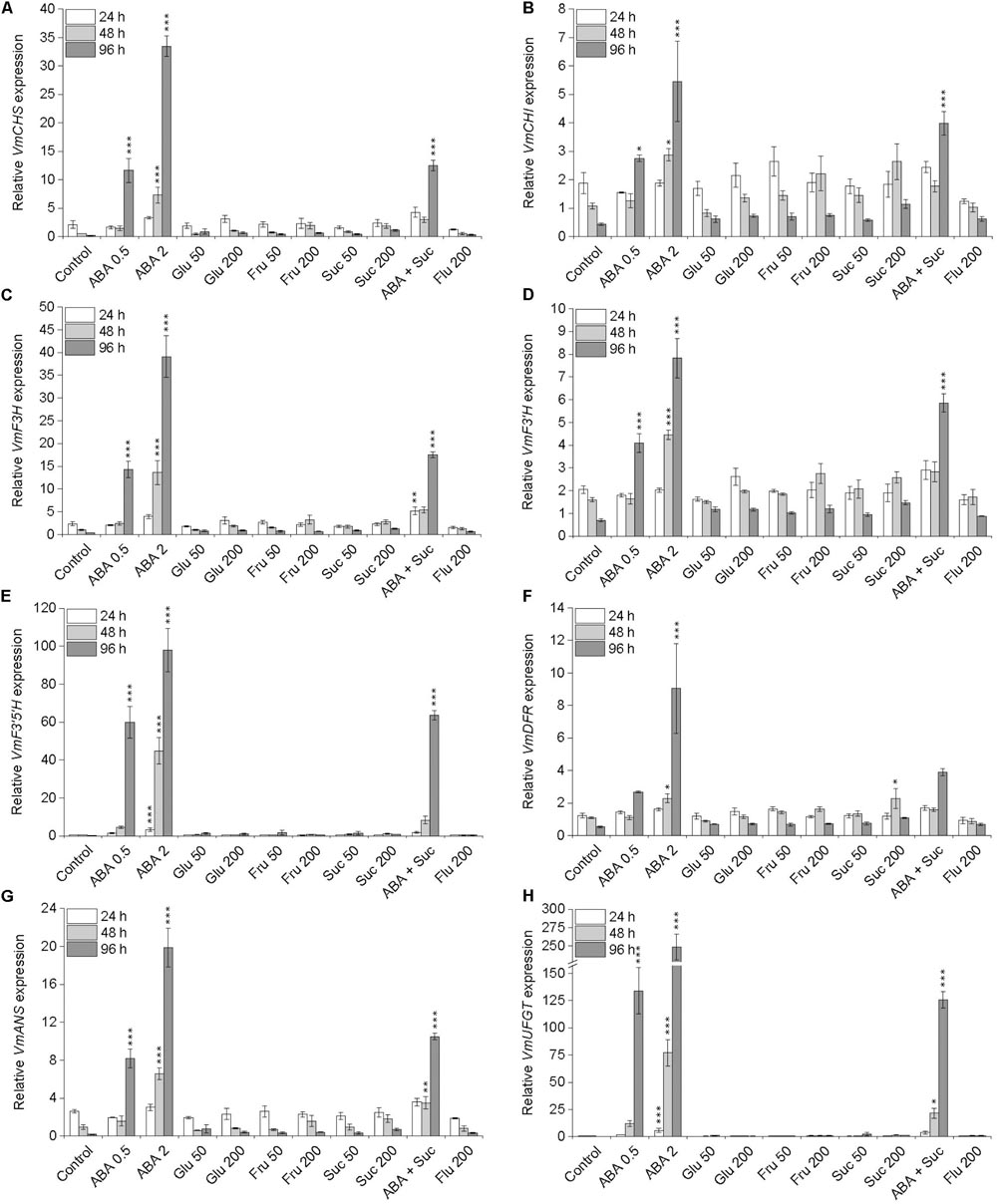
FIGURE 4. Effect of post-harvest ABA and sugar treatments on the expression of anthocyanin biosynthetic genes VmCHS (A), VmCHI (B), VmF3H (C), VmF3′H (D), VmF3′5′H (E), VmDFR (F), VmANS (G), and VmUFGT (H) in bilberry fruit. The treatments were: ABA (0.5 and 2 mM), glucose (50 and 200 mM), fructose (50 and 200 mM), sucrose (50 and 200 mM), 0.5 mM ABA + 200 mM sucrose, 200 μM fluridone or water (control). Relative expression of the genes was quantified by qRT-PCR and normalized to VmGAPDH. Values represent means ± SEs of three replicates. Asterisks indicate significant differences from respective control (∗P ≤ 0.05, ∗∗P ≤ 0.01, ∗∗∗P ≤ 0.001, one-way ANOVA with Tukey’s HSD test).
Expression of ABA and Sucrose Biosynthetic Genes in Response to Post-harvest Treatments With ABA and Sugars
In order to examine the interaction between ABA and sucrose, the transcript levels of ABA and sucrose biosynthetic genes was analyzed in post-harvest bilberry fruits in the different treatments. Bilberry fruits in 0.5 and 2 mM ABA treatments had significantly elevated transcript levels of VmNCED1, the key gene in the ABA biosynthetic pathway, compared to control fruits in water (Figure 5A) indicating autocatalytic biosynthesis of ABA. In the berries in treatments with different sugars the expression of VmNCED1 was not induced but decreased during the 4 days experiment. The expression of the two key genes in the sucrose metabolism, VmSS and VmSPS was also studied, especially in response to ABA treatments. The expression of VmSS was significantly elevated in berries in 2 mM ABA treatment compared to control berries after 4 days indicating sucrose degradation while no significant increase in the expression of the gene was observed in other treatments (Figure 5B). The expression profiles of the three identified bilberry SPS genes slightly differentiated from each other. The expression of VmSPS1 and VmSPS2 showed initially up-regulation in berries in ABA treatments and then down-regulation after 96 h compared to control berries, while the VmSPS3 expression was significantly down-regulated by ABA (Figures 5C–E) indicating that ABA does not advance sucrose formation in bilberry fruits.
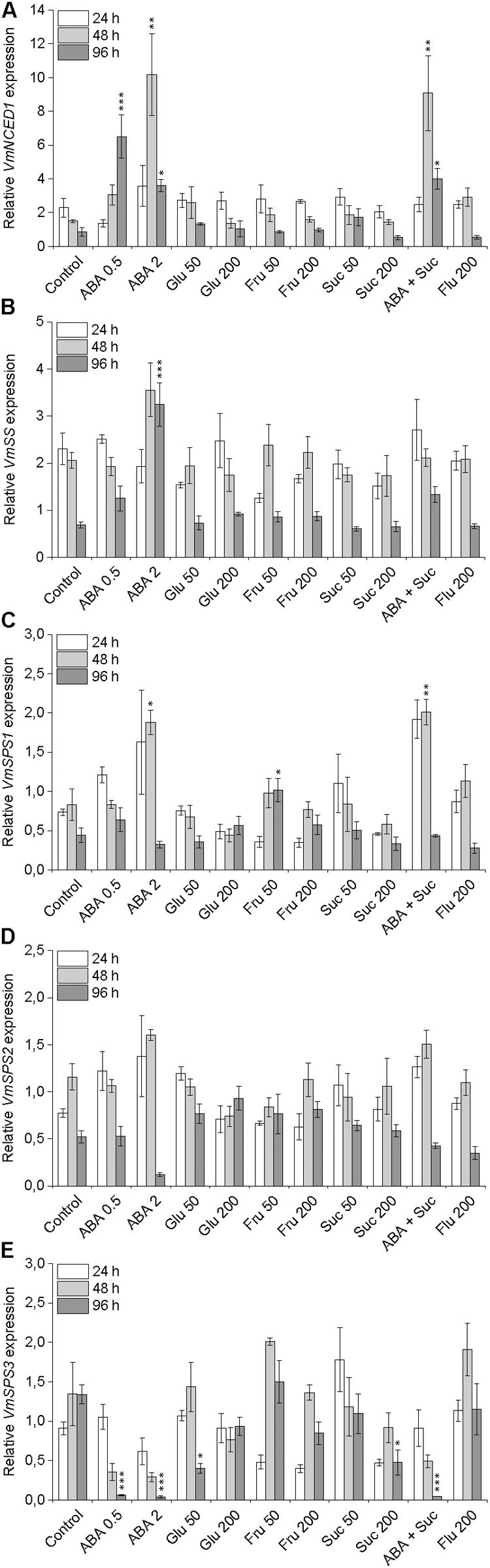
FIGURE 5. Effect of post-harvest ABA and sugar treatments on the expression of key ABA and sucrose biosynthetic genes VmNCED1 (A), VmSS (B), VmSPS1 (C), VmSPS2 (D), and VmSPS3 (E) in bilberry fruit. The treatments were: ABA (0.5 and 2 mM), glucose (50 and 200 mM), fructose (50 and 200 mM), sucrose (50 and 200 mM), 0.5 mM ABA + 200 mM sucrose, 200 μM fluridone or water (control). Relative expression of the genes was quantified by qRT-PCR and normalized to VmGAPDH. Values represent means ± SEs of three replicates. Asterisks indicate significant differences from respective control (∗P ≤ 0.05, ∗∗P ≤ 0.01, ∗∗∗P ≤ 0.001, one-way ANOVA with Tukey’s HSD test).
Silencing of VmNCED1 in Bilberry Fruit by Virus-Induced Gene Silencing (VIGS)
The effect of ABA on bilberry fruit ripening and anthocyanin biosynthesis was further studied by silencing VmNCED1, the key gene in ABA biosynthetic pathway. VmNCED1-VIGS vector was injected into unripe green bilberry fruits attached to bilberry plants. After 4 weeks of injection, chimeric fruits with green sectors at the site of injection were found (Figure 6A). The transcript levels of the VmNCED1 were confirmed to be suppressed in these fruits compared to control fruits as well as in green sectors of the chimeric fruits compared to red sectors (Figure 6B). The silencing of VmNCED1 was accompanied by the down-regulation in the expression of anthocyanin biosynthetic genes VmCHS, VmF3H, VmF3′5′H, VmANS, and VmUFGT in intact bilberry fruits injected with VmNCED1-VIGS vector as well as in green sectors of the fruits compared to red sectors (Figure 6B).
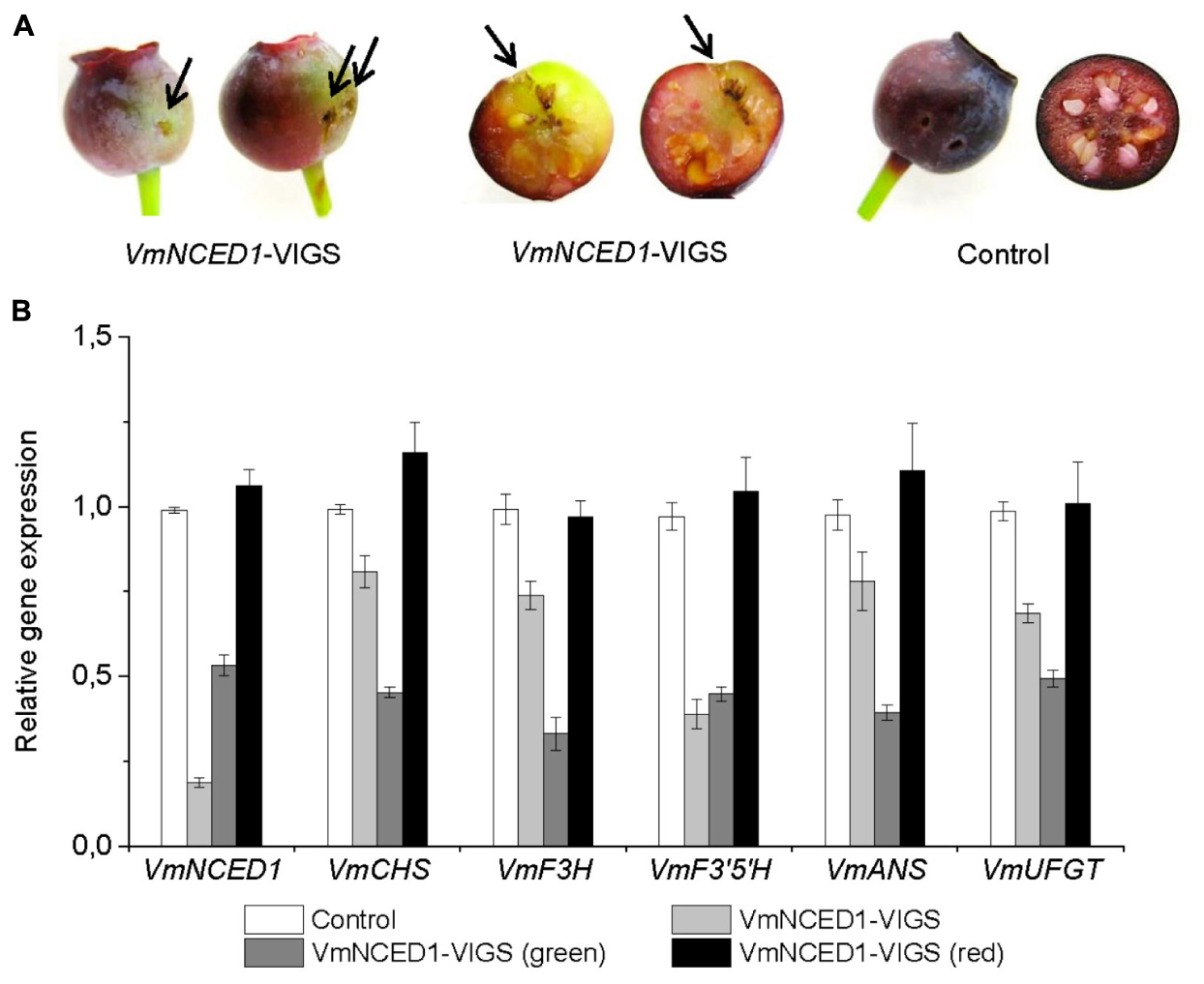
FIGURE 6. Effect of VmNCED1 silencing on anthocyanin biosynthesis in ripening bilberry fruit. Green unripe fruits still attached to the bilberry plants were injected with VmNCED1-VIGS vector or pBINTRA6 vector only (control). Arrows indicate injection sites. Fruits were evaluated 4 weeks after injection for color (A), and the expression of VmNCED1 and the key anthocyanin biosynthetic genes in intact fruits as well as in green and red sectors of chimeric fruits (B). Relative expression of the genes was quantified by qRT-PCR and normalized to VmGAPDH. Values represent means ± SDs of three replicates.
Expression of Genes Associated With Cell Wall Modification in Response to Post-harvest Treatments With ABA and Sugars
In order study the effect of ABA and sugars on other ripening-related processes, we analyzed the expression of several genes encoding cell wall modifying enzymes in bilberry fruit in response to the post-harvest treatments. As shown in Figure 7, ABA significantly increased the expression of some of the genes associated with cell wall modification, including genes indicated in pectin modification VmPL, VmRGLyase, VmβGAL1, and VmβGAL2 as well as genes involved in the depolymerization of hemicellulose VmXTH, VmCEL, and three expansins (VmEXP1, VmEXP2, and VmEXP3). The tested isoforms of PE, PG, or XYL responded to ABA treatment by down-regulating their expression. Instead, the sugar treatments could elevate expression of some genes associated with cell wall modification and the treatment with glucose and fructose significantly elevated the expression of VmPE1 and VmPG1 (Figure 7).
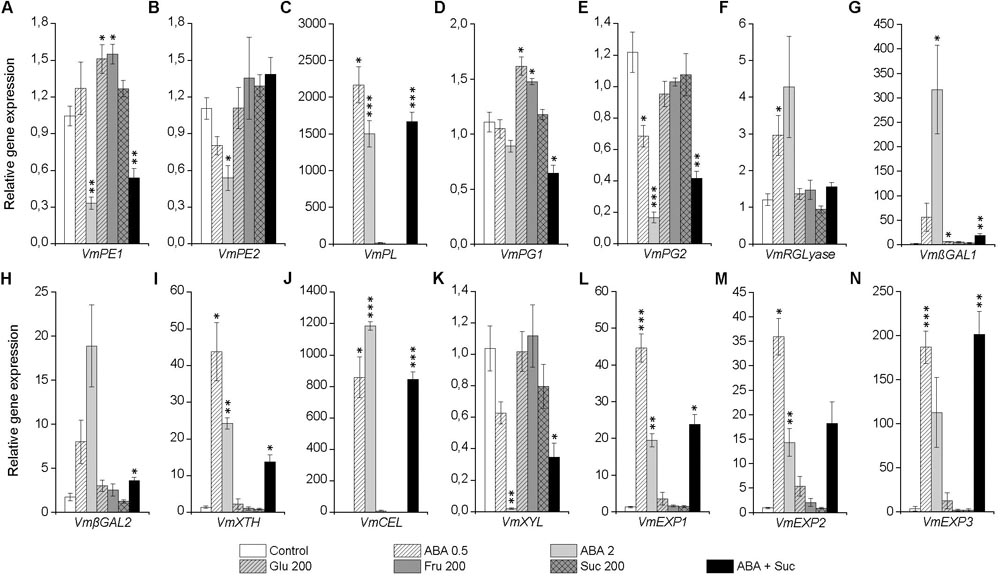
FIGURE 7. Effect of post-harvest ABA and sugar treatments on the expression cell wall modifying genes VmPE1 (A), VmPE2 (B), VmPL (C), VmPG1 (D), VmPG2 (E), VmRGLyase (F), VmβGAL1 (G), VmβGAL2 (H), VmXTH (I), VmCEL (J), VmXYL (K), VmEXP1 (L), VmEXP2 (M), and VmEXP3 (N) in bilberry fruit. The treatments were: ABA (0.5 and 2 mM), glucose (200), fructose (200 mM), sucrose (200 mM), 0.5 mM ABA + 200 mM sucrose, or water (control). Relative expression of the genes was quantified by qRT-PCR after 4 days of the beginning of the experiment and normalized to VmGAPDH. Values represent means ± SEs of three replicates. PE, pectin esterase; PL, pectate lyase; PG, polygalacturonase; RGLyase, rhamnogalacturonate lyase; βGAL, β-galactosidase; XTH, xyloglucan endotransglycosylase/hydrolase; CEL, endo-β-1,4 glucanase: XYL, β-xylosidase; EXP, expansin. Asterisks indicate significant differences from control in Student’s t-Test (∗P ≤ 0.05, ∗∗P ≤ 0.01, ∗∗∗P ≤ 0.001).
Effect of Post-harvest Treatments With ABA and Sugars on the Expression of Potential Bilberry Fruit Ripening Regulators
In order to get an insight into the ABA signaling transduction in bilberry fruit ripening-related processes, we identified from the publicly available Vaccinium transcriptome databases the closest homologs for the genes encoding TFs that have earlier been demonstrated to have a role in ripening regulation or anthocyanin biosynthesis in other fruits. From the tested TFs, expression of VmSCL8, VmMADS18, VmMADS9, VmSHP, and VmBL were significantly up-regulated by ABA indicating their potential involvement in ABA-regulated fruit ripening processes in bilberry (Figure 8A). Also VmTDR4, that has earlier been shown to be involved in anthocyanin accumulation in ripening bilberry fruit (Jaakola et al., 2010), was significantly up-regulated by ABA treatments. Expression of VmMADS18 and VmBL were also significantly up-regulated by fructose and sucrose, respectively (Figure 8A).
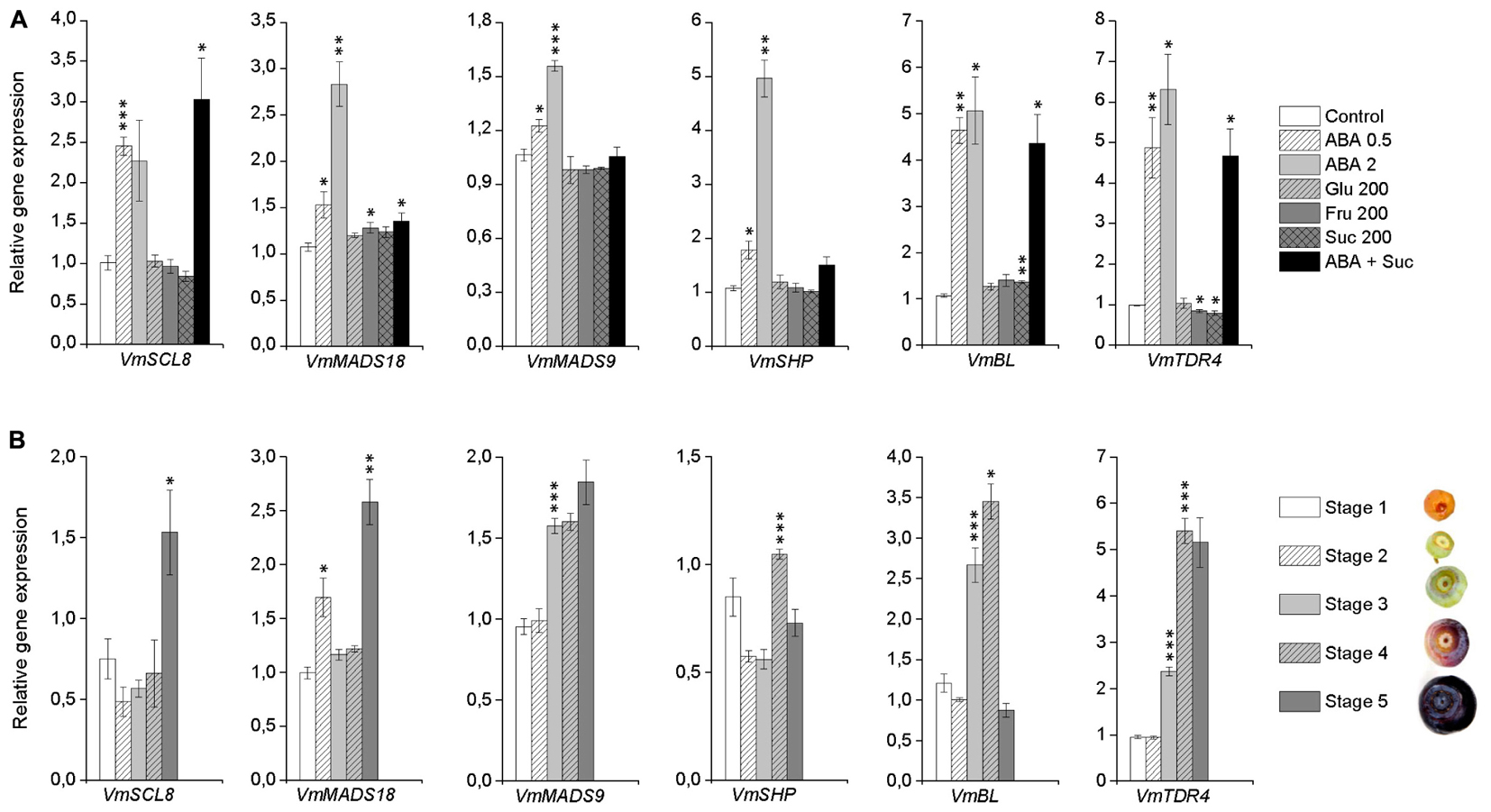
FIGURE 8. Expression of potential ripening-related transcription factors (TFs) in response to post-harvest ABA and sugar treatments (A) and during bilberry fruit development (B). The gene expression was analyzed 4 days after the beginning of the treatments. The treatments were: ABA (0.5 and 2 mM), glucose (200 mM), fructose (200 mM), sucrose (200 mM), 0.5 mM ABA + 200 mM sucrose, or water (control). Relative expression of the genes was quantified by qRT-PCR and normalized to VmGAPDH. Values in (A) represent means ± SEs of three replicates and asterisks significant differences from control in Student’s t-Test (∗P ≤ 0.05, ∗∗P ≤ 0.01, ∗∗∗P ≤ 0.001). Values in (B) represent means ± SEs of four replicates and asterisks significant increase from previous developmental stage in Student’s t-Test (∗P ≤ 0.05, ∗∗P ≤ 0.01, ∗∗∗P ≤ 0.001). Stages 1–5 indicate the bilberry fruit developmental stages from flower to ripe berry.
The expression of these TFs was further analyzed during bilberry fruit development and ripening. The expression of VmSCL8 and VmMADS18 was significantly elevated in ripe fruit (VmMADS18 also at stage 2) indicating a role in ripening processes at late stage of bilberry fruit ripening (Figure 8B). The expression of the genes VmMADS9, VmSHP, and VmBL was significantly up-regulated (P ≤ 0.001) earlier during the fruit development and at the onset of fruit ripening together with VmTDR4 (Figure 8B).
Discussion
ABA Is a Positive Regulator of Bilberry Fruit Ripening Processes
A central role for the plant hormone ABA in promoting fruit ripening has been demonstrated during recent years. Exogenous application of ABA has been shown in many studies to advance especially non-climacteric fruit ripening and the associated anthocyanin accumulation in grape berries (Wheeler et al., 2009; Koyama et al., 2010; Villalobos-González et al., 2016), strawberries (Chai et al., 2011; Jia et al., 2011; Kadomura-Ishikawa et al., 2015; Chen et al., 2016), sweet cherries (Luo et al., 2014; Shen et al., 2014), and litchi fruit (Wei et al., 2011; Singh et al., 2014). Moreover, treatments with inhibitors of ABA biosynthesis, such as fluridone and nordihydroguaiaretic acid (NDGA), delay fruit ripening and decrease anthocyanin accumulation (Jia et al., 2011; Shen et al., 2014; Kadomura-Ishikawa et al., 2015).
In bilberry, the accumulation of anthocyanin pigments is an important indicator of fruit ripening. Our previous studies have demonstrated an increase in ABA content and ABA biosynthesis at the onset of bilberry fruit ripening preceding anthocyanin accumulation (Karppinen et al., 2013, 2016). Similarly, Zifkin et al. (2012) demonstrated a substantial increase in ABA level in highbush blueberries (V. corymbosum) at the initiation of fruit ripening suggesting a role for ABA in fruit ripening regulation. However, contradictory results on the effect of ABA in anthocyanin accumulation have been reported when fruits of genus Vaccinium have been treated with ABA. Oh et al. (2018) demonstrated that exogenous application of ABA increased northern highbush blueberry (V. corymbosum) fruit coloration and accumulation of anthocyanins, especially malvidin, delphinidin, and petunidin glycosides. Instead, exogenous ABA application delayed ripening of southern highbush blueberries (V. darrowii; Buran et al., 2012) while ABA had no effect on the anthocyanin accumulation in lowbush blueberry (V. angustifolium; Percival and MacKenzie, 2007) or in white cranberries (V. macrocarpon; Forney et al., 2009).
The data from the current study clearly demonstrates that ABA induces bilberry fruit anthocyanin biosynthesis. Exogenous ABA applied to unripe bilberry fruits either as pre- or post-harvest treatment promoted berry coloration and anthocyanin accumulation. In addition, the post-harvest treatment with ABA biosynthesis inhibitor, fluridone, delayed bilberry fruit coloration and reduced fruit anthocyanin content. The anthocyanin accumulation in bilberry fruit was supported by the gene expression data demonstrating that all the genes related to anthocyanin biosynthesis were significantly induced by ABA. Especially the expression of VmUFGT and VmF3′5′H were highly induced in bilberry fruit by post-harvest ABA treatment. At the branch point in flavonoid biosynthetic route, F3′H leads to cyanidin-derived anthocyanins while F3′5′H activity leads to delphinidin-derived compounds (Supplementary Figure S1). Earlier, exogenous ABA treatment has been shown to modify fruit anthocyanin profile (Koyama et al., 2010; Singh et al., 2014; Ju et al., 2016). Our results may imply that in bilberry ABA promotes especially biosynthesis of delphinidin-derived compounds similarly as reported in highbush blueberries by Oh et al. (2018). Also in grape berries, the treatment with high ABA concentration increased the ratio of delphinidin-derived anthocyanins to cyanidin-derived anthocyanins (Ju et al., 2016). The earlier reported contradictory results concerning the role of ABA as a regulator of anthocyanin accumulation among different Vaccinium fruits may reflect the differences among the species or a dose of ABA. Also, timing of ABA application has been reported to be critical in ripening promotion among other fruits (Soto et al., 2013; Wang et al., 2013; Luo et al., 2014).
Our results of silencing of VmNCED1 gene also evidenced the role of ABA in bilberry anthocyanin biosynthesis. 9-cis-epoxycarotenoid dioxygenase (NCED), catalyzing the oxidative cleavage of 9-cis-isomers of violaxanthin and neoxanthin to xanthoxin, is considered as the key enzyme responsible for ABA biosynthesis (Leng et al., 2014), also in fruits of genus Vaccinium (Zifkin et al., 2012; Karppinen et al., 2013). In the present study, silencing of VmNCED1 in bilberry fruits by virus-induced gene silencing (VIGS) resulted in chimeric fruits with green sectors at the site of infection. The silencing of VmNCED1 was accompanied by the down-regulation in the expression of the key anthocyanin biosynthesis genes. Our results are similar to previously reported. Previously, Shen et al. (2014) showed that silencing of PacNCED1 led to the decrease in anthocyanin biosynthesis and resulted in partly colorless sweet cherries. In strawberries, the down-regulation of FaNCED1 by VIGS was demonstrated to reduce ABA accumulation, delay fruit ripening and anthocyanin biosynthesis, and to lead partly uncolored fruits (Jia et al., 2011; Medina-Puche et al., 2014; Kadomura-Ishikawa et al., 2015). Furthermore, the suppression of SlNCED1 expression in tomato has been shown to slow down ripening, elongate fruit shelf life and enhance fruit firmness (Sun et al., 2012; Ji et al., 2014).
In the current study, post-harvest ABA treatment was also found to increase expression of VmNCED1 indicating that ABA regulates its own biosynthesis in bilberry fruit. Earlier, similar results have been reported and externally applied ABA has been found to elevate NCED gene expression and ABA synthesis in grapes (Wheeler et al., 2009), cucumber (Wang et al., 2013), and sweet cherry (Luo et al., 2014). Up-regulation of FaNCED1 expression by ABA has also been demonstrated in strawberry fruits (Chen et al., 2016; Medina-Puche et al., 2016) and it was suggested that the autocatalytic biosynthesis of ABA may be necessary for the induction of high increase in ABA production at fruit ripening (Medina-Puche et al., 2016).
Both transcriptomic and proteomic level studies have revealed that ABA regulates many aspects of non-climacteric fruit ripening (Giribaldi et al., 2010; Li et al., 2015; Medina-Puche et al., 2016; Rattanakon et al., 2016). In addition to pigment formation, fruit ripening is associated with other ripening-related processes, including fruit softening. Structural changes in cell wall polysaccharides pectin, hemicellulose and cellulose due to the action of hydrolytic enzymes and expansins lead to a loss of firmness of fruit pulp at late stage of fruit ripening (Goulao and Oliveira, 2008; Payasi et al., 2009). Studies in non-climacteric grapes (Koyama et al., 2010), strawberry (Li et al., 2014), and cherries (Luo et al., 2014) have implicated that the fruit ripening-related softening is regulated by ABA, and in northern highbush blueberry exogenous ABA was shown to decrease fruit firmness (Oh et al., 2018). In climacteric tomato, involvement of ABA in cell wall degradation was proven by silencing of SlNCED1 leading to higher pectin content, enhancement in fruit firmness and down-regulation in expression of genes encoding cell wall degrading enzymes (Sun et al., 2012).
Bilberry fruit has a short post-harvest shelf life due to relatively rapid softening. However, gene expression associated with ripening-related fruit softening has not been studied previously in bilberry or other Vaccinium species. In the present study, post-harvest ABA treatment during bilberry fruit ripening led to the induction in the expression of genes associated with cell wall modifications. Among these, ABA induced genes encoding pectin-modifying enzymes VmPL, VmRGLyase, VmβGAL1, and VmβGAL2 as well as genes involved in depolymerization of hemicellulose, including VmXTH, VmCEL, and three expansins (VmEXP1, VmEXP2, and VmEXP3). Earlier, fruit softening related gene expression has been studied extensively in strawberry having also a short shelf life. Indications that ABA activates expression of FaPL, FaCEL, FaRGlyase, FcXTH1, FaβGal4, FaXYL1 and expansins have been reported (Bustamante et al., 2009; Molina-Hidalgo et al., 2013; Opazo et al., 2013; Chen et al., 2016; Medina-Puche et al., 2016; Paniagua et al., 2016). However, earlier studies have shown that the textural changes occurring during fruit ripening are characteristic to particular fruit species, and are due to differences in type and extent of cell wall modifications and the expression of the modifying enzymes during ripening (Goulao and Oliveira, 2008; Payasi et al., 2009). Overall, our results indicate that the expression of many genes involved in cell wall disassembly is enhanced during bilberry fruit ripening by ABA while the expression of some of the genes/gene isoforms is not induced by ABA.
Sugars Do Not Induce Bilberry Fruit Ripening Processes
Soluble sugars have been indicated during recent years as fruit ripening regulators in some non-climacteric fruits acting as signaling molecules rather than a carbon source. The role of sugars in fruit ripening has been studied in strawberry and grapes where especially sucrose has been shown to accelerate fruit ripening and anthocyanin biosynthesis. In strawberry, the signaling function of sucrose in fruit ripening was proposed when exogenous sucrose injected at 50 mM dramatically accelerated the fruit ripening while glucose had smaller but also obvious role in ripening and anthocyanin accumulation (Jia et al., 2011, 2013b). Exogenous sucrose at 100 mM was shown to accelerate strawberry fruit ripening in both pre- and post-harvest experiments with injected and immersed berries, respectively (Jia et al., 2013a). Similar results have been reported for some grape varieties (Lecourieux et al., 2014). Recently, Jia et al. (2017) showed that treatments with sugars, especially sucrose, induced anthocyanin biosynthesis and fruit softening in detached Fujiminori grapes. Also, Zheng et al. (2009) demonstrated elevated anthocyanin biosynthesis and accumulation in Cabernet Sauvignon grape berry disks after immersion in solutions containing 50–200 mM glucose, sucrose, or fructose. Spraying with sucrose either alone or in combination with ABA has been shown to increase anthocyanin biosynthesis and accumulation in Crimson Seedless grape berries (Ferrara et al., 2015; Olivares et al., 2017).
The role of sugars in bilberry fruit ripening and anthocyanin biosynthesis has not been studied earlier. Based on the results of the present study, immersion of bilberry fruits into 50 mM or 200 mM glucose, fructose, or sucrose solution does not induce anthocyanin biosynthesis and accumulation but slightly elevates the expression of some genes associated with cell wall modification. Furthermore, sucrose in the ABA + Suc treatment did not enhance the ripening response induced by ABA alone. These results indicate that the role of sugars in the regulation of bilberry fruit ripening differentiates from that reported to strawberry and grapes, and sugars seem to have less important regulatory role in bilberry fruit ripening. However, intensity of the response may also vary between different studies based on the application method of the sugar solutions.
A cross-signaling between ABA and sucrose in anthocyanin biosynthesis has been suggested (Loreti et al., 2008). In strawberry fruit, exogenous sucrose has been shown to stimulate ABA accumulation by promoting dramatically the expression of FaNCED (Jia et al., 2011, 2013a,b). Also, silencing of sucrose transporter FaSUT1 in strawberry led to a decrease in both sucrose and ABA content indicating connection between the two signaling routes (Jia et al., 2013b). In fact, a model for interaction of these two signaling molecules as the core mechanism in regulation of strawberry fruit ripening was recently suggested (Cherian et al., 2014; Jia et al., 2016). Jia et al. (2013b) proposed that sucrose functions as a signal upstream of ABA and induces strawberry fruit ripening both through ABA-dependent and ABA-independent pathways. The effect of ABA on sugar metabolism has also been shown in grape fruits (Çakir et al., 2003; Pan et al., 2005).
However, in the current study, sucrose or other sugar treatments had no inducing effect on VmNCED1 expression suggesting that sugars do not induce ABA biosynthesis in bilberry during fruit ripening. We also studied the effect of ABA on sucrose biosynthetic gene expression. These results do not support the assumption that ABA might induce sucrose biosynthesis in bilberry fruit. Sucrose phosphate synthase (SPS), catalyzing sucrose synthesis, and sucrose synthase (SS), catalyzing reversible conversion of sucrose to monosaccharides, are indicated as the key enzymes affecting sucrose accumulation in different fruits (Choudhury et al., 2009; Dai et al., 2011; Feng et al., 2012; Li et al., 2012; Tian et al., 2012; Jia et al., 2016). High rate of sucrose accumulation during strawberry ripening was demonstrated to be accompanied by the high expression level of FaSPS genes and low expression of FaSS gene (Jia et al., 2016). Furthermore, FaSS1 was suggested as important regulator of strawberry fruit ripening which expression was significantly inhibited by ABA and sucrose treatments (Zhao et al., 2017). Based on our studies, ABA does not induce expression of the VmSPS genes in bilberry fruit but increases expression of VmSS that is contrast to the results reported in strawberry (Zhao et al., 2017).
Overall, our results indicate that the role of sugars in bilberry fruit differs from strawberry, the current model of non-climacteric fruit ripening. In bilberry fruit, glucose, fructose, or sucrose seem not to act as major signaling molecules to clearly regulate and induce anthocyanin biosynthesis. Despite that strawberry is not an ovary-derived fruit and, thus, considered as a false fruit deviating from bilberry fruit, the regulatory mechanisms of fruit development and ripening has been considered to be conserved among angiosperms (Daminato et al., 2013; Karlova et al., 2014). In order to further clarify the fruit ripening regulation and signaling in bilberry, the signaling route of ABA-mediated bilberry fruit ripening needs to be studied in more detail in the future.
ABA Up-Regulates Expression of Potential Bilberry Fruit Ripening Regulators
Several types of TFs belonging to different families have earlier been identified as regulators of fruit ripening and anthocyanin biosynthesis, and some of them are regulated by ABA. Fruit ripening regulation has been studied extensively in tomato and in fruits of Rosaceae family. In the current study, we aimed to identify potential TFs of ABA-regulated bilberry fruit ripening processes by searching the closest homologs of functionally characterized TFs of fruit ripening/anthocyanin biosynthesis from publicly available Vaccinium transcriptome libraries. Some of the TFs showed highly increased expression in bilberry fruits after ABA treatment. Furthermore, we found elevated TF transcript levels in ripening or ripe fruits indicating a potential role in bilberry fruit ripening-related processes.
One of them was VmSCL8, the closest bilberry homolog for FaSCL8 that is similar to AtSCL8 in Arabidopsis, a member of SCARECROW-LIKE gene family, which members are known to have general roles in plant development. FaSCL8 expression has been shown to be induced in strawberry receptacle at fruit ripening increasing further in ripe red fruit indicating a role as a regulator of fruit ripening (Pillet et al., 2015). Furthermore, silencing FaSCL8 in strawberry resulted in lower transcript accumulation of PAL, CHS, CHI, F3H, UFGT, and MYB10 but increased F3′H and ANR transcripts suggesting a role as a general modulator of flavonoid pathway possibly affecting cyanidin-pelargonidin balance by enhancing expression of flavonoid regulating MYB TFs (Pillet et al., 2015). Recently, Medina-Puche et al. (2016) showed that the FaSCL8 expression in strawberry is elevated by ABA. In the current study, VmSCL8 also showed elevated expression after ABA treatment. The expression of VmSCL8 was highest in ripe bilberry fruit similarly to FaSCL8 indicating a possible role in the ABA-regulated bilberry fruit ripening at the late stages of ripening.
Similarly, elevated expression after ABA treatments in bilberry fruits was observed for three MADS-box genes, VmMADS18, VmMADS9, and VmSHP, the bilberry homologs for PyMADS18, FaMADS9, and FaSHP, respectively. MADS-box genes represent highly conserved TF family in plants and have been shown to play important roles in floral and fruit development. While the expression of VmMADS18 was high in this study both in small green and ripe bilberry fruit, the expression of VmMADS9 increased in large green stage and VmSHP in ripening fruit, indicating their differential roles in bilberry fruit. The PyMADS18 has been suggested to be involved in the regulation of anthocyanin biosynthesis in pear (Wu et al., 2013). During the fruit ripening, expression of PyMADS18 was shown to increase at early stages of development, after that decreasing until it was up-regulated again at the end of fruit maturation period (Wu et al., 2013) resembling the expression pattern obtained in this study for VmMADS18.
SEPALLATA (SEP)-like MADS-box TFs have been indicated to play central roles in ripening of both climacteric and non-climacteric fruits, best known example being LeMADS-RIN (Seymour et al., 2011). FaMADS9, a fruit-related SEP1/2-like gene was indicated as a positive regulator of both development and ripening of strawberry fruit with its expression up-regulated at white stage of strawberry fruit development (Seymour et al., 2011). Silencing of the gene led to inhibition of normal development of strawberry fruit (Seymour et al., 2011). The gene was shown to be ABA-inducible later by Daminato et al. (2013). Also FaSHP, a C-type MADS-box gene belonging to a SHATTERPROOF group was indicated as a positive regulator of strawberry fruit ripening with its expression induced by ABA (Daminato et al., 2013; Medina-Puche et al., 2016). The expression of the FaSHP increases in strawberry fruit after large green stage due to ABA control being highest at pink stage (Daminato et al., 2013) similarly to VmSHP shown in our study. Overall, our results indicate that VmMADS18, VmMADS9, and VmSHP have potential roles in the ABA-regulated bilberry fruit development and ripening.
The fourth studied MADS-box gene in the present study was VmTDR4 belonging to a SQUAMOSA group. VmTDR4 was earlier demonstrated to have a role in the anthocyanin accumulation during bilberry fruit ripening with its expression especially associated in flesh of ripening fruit (Jaakola et al., 2010). Silencing of VmTDR4 in bilberry fruit resulted in chimeric berries with decreased expression of CHS, DFR, and ANS but elevated expression of ANR indicating modulation of flavonoid pathway through flavonoid regulating MYB TFs (Jaakola et al., 2010). Also tomato homolog for TDR4 induced anthocyanin biosynthesis when expressed in Arabidopsis siliques (Jaakola et al., 2010). The response of VmTDR4 to ABA has not been studied earlier but the present study demonstrates that VmTDR4 is highly induced by ABA indicating its role in the ABA-regulated fruit ripening associated anthocyanin accumulation.
Also NAC family TFs have been proposed as activators of fruit ripening with some of their expression shown to be increased by ABA (Zhu et al., 2014; Jiang et al., 2017; Moyano et al., 2018). Recently, a PpBL gene (BLOOD) was indicated as a key regulator of anthocyanin biosynthesis in maturing blood-fleshed peach fruit (Zhou et al., 2015). It was shown to act as a heterodimer with another NAC family member, PpNAC1 (Zhou et al., 2015). In our study, the closest bilberry homolog for the peach BL gene, VmBL, showed a significant induction in its expression by ABA treatments as well as increased expression at the onset of bilberry fruit ripening slightly before VmTDR4. This suggests that VmBL could have a role in the regulation of bilberry fruit ripening and/or anthocyanin biosynthesis through ABA-mediated signaling.
Conclusion
This is the first report regarding the role of ABA and sugars on the regulation of bilberry fruit ripening. By using both pre- and post-harvest experiments and a molecular approach, we showed that ABA is an important positive regulator of bilberry fruit ripening processes, inducing anthocyanin biosynthesis and fruit softening. However, based on our results, sugars (glucose, fructose, and sucrose) have minor roles in the regulation of bilberry fruit ripening as sugars failed to induce anthocyanin or ABA biosynthesis in bilberry fruit but could elevate expression of some genes associated with cell wall modification. Moreover, sucrose did not enhance the effect of ABA in ripening responses. Our results suggest that the ripening regulation may be different in bilberry fruit compared to the current model of non-climacteric fruit ripening, strawberry, in which the coordinated regulation by the two signaling molecules, ABA and sucrose, have been proposed to have a key role in fruit ripening.
Author Contributions
KK and LJ designed the experiments. KK was responsible for conducting the experiments and analyses. PT and KK conducted pre-harvest ABA treatments and subsequent gene expression analyses. KK was responsible for the writing of the manuscript with contribution of LJ and HH. All authors read and approved the final manuscript.
Funding
This work was financially supported by the Finnish Cultural Foundation, Niemi Foundation, Otto A. Malm Foundation and Osk. Huttunen Foundation to KK, and Interreg Nord to HH.
Conflict of Interest Statement
The authors declare that the research was conducted in the absence of any commercial or financial relationships that could be construed as a potential conflict of interest.
Acknowledgments
The authors would like to thank Nelli Mikkola for the help in the field experiment in Tromsø.
Supplementary Material
The Supplementary Material for this article can be found online at: https://www.frontiersin.org/articles/10.3389/fpls.2018.01259/full#supplementary-material
References
Buran, T. J., Sandhu, A. K., Azeredo, A. M., Bent, A. H., Williamson, J. G., and Gu, L. (2012). Effects of exogenous abscisic acid on fruit quality, antioxidant capacities, and phytochemical contents of southern high bush blueberries. Food Chem. 132, 1375–1381. doi: 10.1016/j.foodchem.2011.11.124
Bustamante, C. A., Civello, P. M., and Martínez, G. A. (2009). Cloning of the promoter region of β-xylosidase (FaXyl1) gene and effect of plant growth regulators on the expression of FaXyl1 in strawberry fruit. Plant Sci. 177, 49–56. doi: 10.1016/j.plantsci.2009.03.015
Çakir, B., Agasse, A., Gaillard, C., Saumonneau, A., Delrot, S., and Atanassova, R. (2003). A grape ASR protein involved in sugar and abscisic acid signaling. Plant Cell 15, 2165–2180. doi: 10.1105/tpc.013854
Chai, Y., Jia, H., Li, C., Dong, Q., and Shen, Y. (2011). FaPYR1 is involved in strawberry fruit ripening. J. Exp. Bot. 62, 5079–5089. doi: 10.1093/jxb/err207
Chen, J., Mao, L., Lu, W., Ying, T., and Luo, Z. (2016). Transcriptome profiling of postharvest strawberry fruit in response to exogenous auxin and abscisic acid. Planta 243, 183–197. doi: 10.1007/s00425-015-2402-5
Cherian, S., Figueroa, C. R., and Nair, H. (2014). ‘Movers and shakers’ in the regulation of fruit ripening: a cross-dissection of climacteric versus non-climacteric fruit. J. Exp. Bot. 65, 4705–4722. doi: 10.1093/jxb/eru280
Choudhury, S. R., Roy, S., and Sengupta, D. N. (2009). A comparative study of cultivar differences in sucrose phosphate synthase gene expression and sucrose formation during banana fruit ripening. Postharvest Biol. Technol. 54, 15–24. doi: 10.1016/j.postharvbio.2009.05.003
Dai, N., Cohen, S., Portnoy, V., Tzuri, G., Harel-Beja, R., Pompan-Lotan, M., et al. (2011). Metabolism of soluble sugars in developing melon fruit: a global transcriptional view of the metabolic transition to sucrose accumulation. Plant Mol. Biol. 76, 1–18. doi: 10.1007/s11103-011-9757-1
Dai, S., Li, P., Chen, P., Li, Q., Pei, Y., He, S., et al. (2014). Transcriptional regulation of genes encoding ABA metabolism enzymes during the fruit development and dehydration stress of pear ‘Gold Nijisseiki’. Plant Physiol. Biochem. 82, 299–308. doi: 10.1016/j.plaphy.2014.06.013
Daminato, M., Guzzo, F., and Casadoro, G. (2013). A SHATTERPROOF-like gene controls ripening in non-climacteric strawberries, and auxin and abscisic acid antagonistically affect its expression. J. Exp. Bot. 64, 3775–3786. doi: 10.1093/jxb/ert214
Dandena, A., Leimane, I., and Klētnieks, U. (2012). Validation of monomeric anthocianin determination method for bilberry juice and marc extracts. J. Life Sci. 6, 1378–1382.
Feng, C., Chen, M., Xu, C., Bai, L., Yin, X., Li, X., et al. (2012). Transcriptomic analysis of Chinese bayberry (Myrica rubra) fruit development and ripening using RNA-Seq. BMC Genomics 13:19. doi: 10.1186/1471-2164-13-19
Ferrara, G., Mazzeo, A., Matarrese, A. M. S., Pacucci, C., Punzi, R., Faccia, M., et al. (2015). Application of abscisic acid (S-ABA) and sucrose to improve colour, anthocyanin content and antioxidant activity of cv. Crimson Seedless grape berries. Aust. J. Grape Wine Res. 21, 18–29. doi: 10.1111/ajgw.12112
Forney, C. F., Kalt, W., Abrams, S. R., and Owen, S. J. (2009). Effects of postharvest light and ABA treatments on the composition of late-harvested white cranberry fruit. Acta Hort. 810, 799–806. doi: 10.17660/ActaHortic.2009.810.106
Giribaldi, M., Gény, L., Delrot, S., and Schubert, A. (2010). Proteomic analysis of the effects of ABA treatments on ripening Vitis vinifera berries. J. Exp. Bot. 61, 2447–2458. doi: 10.1093/jxb/erq079
Goulao, L. F., and Oliveira, C. M. (2008). Cell wall modifications during fruit ripening: when a fruit is not the fruit. Trends Food Sci. Technol. 19, 4–25. doi: 10.1016/j.tifs.2007.07.002
Huang, H., Xie, S., Xiao, Q., Wei, B., Zheng, L., Wang, Y., et al. (2016). Sucrose and ABA regulate starch biosynthesis in maize through a novel transcription factor, ZmEREB156. Sci. Rep. 6, 27590. doi: 10.1038/srep27590
Jaakola, L., Pirttilä, A. M., Halonen, M., and Hohtola, A. (2001). Isolation of high quality RNA from the bilberry (Vaccinium myrtillus L.) fruit. Mol. Biotechnol. 19, 201–203. doi: 10.1385/MB:19:2:201
Jaakola, L., Pirttilä, A. M., Vuosku, J., and Hohtola, A. (2004). A method based on electrophoresis and gel extraction for obtaining genomic DNA-free cDNA without DNase treatment. BioTechniques 37, 744–748. doi: 10.2144/04375BM06
Jaakola, L., Poole, M., Jones, M. O., Kämäräinen-Karppinen, T., Koskimäki, J. J., Hohtola, A., et al. (2010). A SQUAMOSA MADS box gene involved in the regulation of anthocyanin accumulation in bilberry fruits. Plant Physiol. 153, 1619–1629. doi: 10.1104/pp.110.158279
Ji, K., Kai, W., Zhao, B., Sun, Y., Yuan, B., Dai, S., et al. (2014). SlNCED1 and SlCYP707A2: key genes involved in ABA metabolism during tomato fruit ripening. J. Exp. Bot. 65, 5243–5255. doi: 10.1093/jxb/eru288
Jia, H., Jiu, S., Zhang, C., Wang, C., Tariq, P., Liu, Z., et al. (2016). Abscisic acid and sucrose regulate tomato and strawberry fruit ripening through the abscisic acid-stress-ripening transcription factor. Plant Biotechnol. J. 14, 2045–2065. doi: 10.1111/pbi.12563
Jia, H., Li, C., Chai, Y., Xing, Y., and Shen, Y. (2013a). Sucrose promotes strawberry fruit ripening by stimulation of abscisic acid biosynthesis. Pak. J. Bot. 45, 169–175.
Jia, H., Wang, Y., Sun, M., Li, B., Han, Y., Zhao, Y., et al. (2013b). Sucrose functions as a signal involved in the regulation of strawberry fruit development and ripening. New Phytol. 198, 453–465. doi: 10.1111/nph.12176
Jia, H., Xie, Z., Wang, C., Shangguan, L., Qian, N., Cui, M., et al. (2017). Abscisic acid, sucrose, and auxin coordinately regulate berry ripening process of the Fujiminori grape. Funct. Integr. Genomics 17, 441–457. doi: 10.1007/s10142-017-0546-z
Jia, H.-F., Chai, Y.-M., Li, C.-L., Lu, D., Luo, J.-J., Qin, L., et al. (2011). Abscisic acid plays an important role in the regulation of strawberry fruit ripening. Plant Physiol. 157, 188–199. doi: 10.1104/pp.111.177311
Jiang, G., Yan, H., Wu, F., Zhang, D., Zeng, W., Qu, H., et al. (2017). Litchi fruit LcNAC1 is a target of LcMYC2 and regulator of fruit senescence through its interaction with LcWRKY1. Plant Cell Physiol. 58, 1075–1089. doi: 10.1093/pcp/pcx054
Jimenez-Garcia, S. N., Guevara-Gonzalez, R. G., Miranda-Lopez, R., Feregrino-Perez, A. A., Torres-Pacheco, I., and Vazquez-Cruz, M. A. (2013). Functional properties and quality characteristics of bioactive compounds in berries: Biochemistry, biotechnology, and genomics. Food Res. Int 54, 1195–1207. doi: 10.1016/j.foodres.2012.11.004
Ju, Y.-L., Liu, M., Zhao, H., Meng, J.-F., and Fang, Y.-L. (2016). Effect of exogenous abscisic acid and methyl jasmonate on anthocyanin composition, fatty acids, and volatile compounds of Cabernet Sauvignon (Vitis vinifera L.) grape berries. Molecules 21, 1354. doi: 10.3390/molecules21101354
Kadomura-Ishikawa, Y., Miyawaki, K., Takahashi, A., Masuda, T., and Noji, S. (2015). Light and abscisic acid independently regulated FaMYB10 in Fragaria × ananassa fruit. Planta 241, 953–965. doi: 10.1007/s00425-014-2228-6
Karlova, R., Chapman, N., David, K., Angenent, G. C., Seymour, G. B., and de Maagd, R. A. (2014). Transcriptional control of fleshy fruit development and ripening. J. Exp. Bot. 65, 4527–4541. doi: 10.1093/jxb/eru316
Karppinen, K., Hirvelä, E., Nevala, T., Sipari, N., Suokas, M., and Jaakola, L. (2013). Changes in the abscisic acid levels and related gene expression during fruit development and ripening in bilberry (Vaccinium myrtillus L.). Phytochemistry 95, 127–134. doi: 10.1016/j.phytochem.2013.06.023
Karppinen, K., Zoratti, L., Sarala, M., Carvalho, E., Hirsimäki, J., Mentula, H., et al. (2016). Carotenoid metabolism during bilberry (Vaccinium myrtillus L.) fruit development under different light conditions is regulated by biosynthesis and degradation. BMC Plant Biol. 16:95. doi: 10.1186/s12870-016-0785-5
Kolehmainen, M., Mykkänen, O., Kirjavainen, P. V., Leppänen, T., Moilanen, E., Adriaens, M., et al. (2012). Bilberries reduce low-grade inflammation in individuals with features of metabolic syndrome. Mol. Nutr. Food Res. 56, 1501–1510. doi: 10.1002/mnfr.201200195
Koyama, K., Sadamatsu, K., and Goto-Yamamoto, N. (2010). Abscisic acid stimulated ripening and gene expression in berry skins of the Cabernet Sauvignon grape. Funct. Integr. Genomics 10, 367–381. doi: 10.1007/s10142-009-0145-8
Lecourieux, F., Kappel, C., Lecourieux, D., Serrano, A., Torres, E., Arce-Johnson, P., et al. (2014). An update on sugar transport and signalling in grapevine. J. Exp. Bot. 65, 821–832. doi: 10.1093/jxb/ert394
Lee, J., Durst, R. W., and Wrolstad, R. E. (2005). Determination of total monomeric anthocyanin pigment content of fruit juices, beverages, natural colorants, and wines by the pH differential method: collaborative study. J. AOAC Int. 88, 1269–1278.
Lee, J., Rennaker, C., and Wrolstad, R. E. (2008). Correlation of two anthocyanin quantification methods: HPLC and spectrophotometric methods. Food Chem. 110, 782–786. doi: 10.1016/j.foodchem.2008.03.010
Leng, P., Yuan, B., and Guo, Y. (2014). The role of abscisic acid in fruit ripening and responses to abiotic stress. J. Exp. Bot. 65, 4577–4588. doi: 10.1093/jxb/eru204
Li, C., Jia, H., Chai, Y., and Shen, Y. (2011). Abscisic acid perception and signaling transduction in strawberry: A model for non-climacteric fruit ripening. Plant Signal. Behav. 6, 1950–1953. doi: 10.4161/psb.6.12.18024
Li, D., Li, L., Luo, Z., Mou, W., Mao, L., and Ying, T. (2015). Comparative transcriptome analysis reveals the influence of abscisic acid on the metabolism of pigments, ascorbic acid and folic acid during strawberry fruit ripening. PLoS ONE 10:e0130037. doi: 10.1371/journal.pone.0130037
Li, D., Luo, Z., Mou, W., Wang, Y., Ying, T., and Mao, L. (2014). ABA and UV-C effects on quality, antioxidant capacity and anthocyanin contents of strawberry fruit (Fragaria ananassa Duch.). Postharvest Biol. Technol. 90, 56–62. doi: 10.1016/j.postharvbio.2013.12.006
Li, M., Feng, F., and Cheng, L. (2012). Expression patterns of genes involved in sugar metabolism and accumulation during apple fruit development. PLoS ONE 7:e33055. doi: 10.1371/journal.pone.0033055
Loreti, E., Povero, G., Novi, G., Solfanelli, C., Alpi, A., and Perata, P. (2008). Gibberellins, jasmonate and abscisic acid modulate the sucrose-induced expression of anthocyanin biosynthetic genes in Arabidopsis. New Phytol. 179, 1004–1016. doi: 10.1111/j.1469-8137.2008.02511.x
Luo, H., Dai, S., Ren, J., Zhang, C., Ding, Y., Li, Z., et al. (2014). The role of ABA in the maturation and postharvest life of a nonclimacteric sweet cherry fruit. J. Plant Growth Regul. 33, 373–383. doi: 10.1007/s00344-013-9388-7
Medina-Puche, L., Blanco-Portales, R., Molina-Hidalgo, F. J., Cumplido-Laso, G., García-Caparrós, N., Moyano-Cañete, E., et al. (2016). Extensive transcriptomic studies on the roles played by abscisic acid and auxins in the development and ripening of strawberry fruits. Funct. Integr. Genomics 16, 671–692. doi: 10.1007/s10142-016-0510-3
Medina-Puche, L., Cumplido-Laso, G., Amil-Ruiz, F., Hoffmann, T., Ring, L., Rodríguez-Franco, A., et al. (2014). MYB10 plays a major role in the regulation of flavonoid/phenylpropanoid metabolism during ripening of Fragaria × ananassa fruits. J. Exp. Bot. 65, 401–417. doi: 10.1093/jxb/ert377
Molina-Hidalgo, F. J., Franco, A. R., Villatoro, C., Medina-Puche, L., Mercado, J. A., Hidalgo, M. A., et al. (2013). The strawberry (Fragaria × ananassa) fruit-specific rhamnogalacturonate lyase 1 (FaRGLyase1) gene encodes an enzyme involved in the degradation of cell-wall middle lamellae. J. Exp. Bot. 64, 1471–1483. doi: 10.1093/jxb/ers386
Mou, W., Li, D., Luo, Z., Mao, L., and Ying, T. (2015). Transcriptomic analysis reveals possible influences of ABA on secondary metabolism of pigments, flavonoids and antioxidants in tomato fruit during ripening. PLoS ONE 10:e0129598. doi: 10.1371/journal.pone.0129598
Moyano, E., Martínez-Rivas, F. J., Blanco-Portales, R., Molina-Hidalgo, F. J., Ric-Varas, P., Matas-Arroyo, A. J., et al. (2018). Genome-wide analysis of the NAC transcription factor family and their expression during the development and ripening of the Fragaria × ananassa fruits. PLoS ONE 13:e0196953. doi: 10.1371/journal.pone.0196953
Nicolas, P., Lecourieux, D., Kappel, C., Cluzet, S., Cramer, G., Delrot, S., et al. (2014). The basic leucine zipper transcription factor ABSCISIC ACID RESPONSE ELEMENT-BINDING FACTOR2 is an important transcriptional regulator of abscisic acid-dependent grape berry ripening processes. Plant Physiol. 164, 365–383. doi: 10.1104/pp.113.231977
Oh, H. D., Yu, D. J., Chung, S. W., Chea, S., and Lee, H. J. (2018). Abscisic acid stimulates anthocyanin accumulation in ‘Jersey’ highbush blueberry fruits during ripening. Food Chem. 244, 403–407. doi: 10.1016/j.foodchem.2017.10.051
Olivares, D., Contreras, C., Muñoz, V., Rivera, S., González-Agüero, M., Retamales, J., et al. (2017). Relationship among color development, anthocyanin and pigment-related gene expression in ‘Crimson Seedless’ grapes treated with abscisic acid and sucrose. Plant Physiol. Biochem. 115, 286–297. doi: 10.1016/j.plaphy.2017.04.007
Opazo, M. C., Lizana, R., Pimentel, P., Herrera, R., and Moya-León, M. A. (2013). Changes in the mRNA abundance of FcXTH1 and FcXTH2 promoted by hormonal treatments of Fragaria chiloensis fruit. Postharvest Biol. Technol. 77, 28–34. doi: 10.1016/j.postharvbio.2012.11.007
Osorio, S., Scossa, F., and Fernie, A. R. (2013). Molecular regulation of fruit ripening. Front. Plant Sci. 4:198. doi: 10.3389/fpls.2013.00198
Pan, Q.-H., Li, M.-J., Peng, C.-C., Zhang, N., Zou, X., Zou, K.-Q., et al. (2005). Abscisic acid activates acid invertases in developing grape berry. Physiol. Plantarum 125, 157–170. doi: 10.1111/j.1399-3054.2005.00552.x
Paniagua, C., Blanco-Portales, R., Barceló-Muñoz, M., García-Gago, J. A., Waldron, K. W., Quesada, M. A., et al. (2016). Antisense down-regulation of the strawberry β-galactosidase gene FaβGal4 increases cell wall galactose levels and reduces fruit softening. J. Exp. Bot. 67, 619–631. doi: 10.1093/jxb/erv462
Payasi, A., Mishra, N. N., Chaves, A. L. S., and Singh, R. (2009). Biochemistry of fruit softening: an overview. Physiol. Mol. Biol. Plants 15, 103–113. doi: 10.1007/s12298-009-0012-z
Percival, D., and MacKenzie, J. L. (2007). Use of plant growth regulators to increase polyphenolic compounds in the wild blueberry. Can. J. Plant. Sci. 87, 333–336. doi: 10.4141/P06-120
Pillet, J., Yu, H.-W., Chambers, A. H., Whitaker, V. M., and Folta, K. M. (2015). Identification of candidate flavonoid pathway genes using transcriptome correlation network analysis in ripe strawberry (Fragaria × ananassa) fruits. J. Exp. Bot. 66, 4455–4467. doi: 10.1093/jxb/erv205
Rattanakon, S., Ghan, R., Gambetta, G. A., Deluc, L. G., Schlauch, K. A., and Cramer, G. R. (2016). Abscisic acid transcriptomic signaling varies with grapevine organ. BMC Plant Biol. 16:72. doi: 10.1186/s12870-016-0763-y
Roubelakis-Angelakis, K. A., and Kliewer, W. M. (1986). Effects of exogenous factors on phenylalanine ammonia-lyase activity and accumulation of anthocyanins and total phenolics in grape berries. Am. J. Enol. Vitic. 37, 275–280.
Seymour, G. B., Ryder, C. D., Cevik, V., Hammond, J. P., Popovich, A., King, G. J., et al. (2011). A SEPALLATA gene is involved in the development and ripening of strawberry (Fragaria × ananassa Duch.) fruit, a non-climacteric tissue. J. Exp. Bot. 62, 1179–1188. doi: 10.1093/jxb/erq360
Shen, X., Zhao, K., Liu, L., Zhang, K., Yuan, H., Liao, X., et al. (2014). A role for PacMYBA in ABA-regulated anthocyanin biosynthesis in red-colored sweet cherry cv. Hong Deng (Prunus avium L.). Plant Cell Physiol. 55, 862–880. doi: 10.1093/pcp/pcu013
Singh, S. P., Saini, M. K., Singh, J., Pongener, A., and Sidhu, G. S. (2014). Preharvest application of abscisic acid promotes anthocyanins accumulation in pericarp of litchi fruit without adversely affecting postharvest quality. Postharvest Biol. Technol. 96, 14–22. doi: 10.1016/j.postharvbio.2014.05.005
Solfanelli, C., Poggi, A., Loreti, E., Alpi, A., and Perata, P. (2006). Sucrose-specific induction of the anthocyanin biosynthetic pathway in Arabidopsis. Plant Physiol. 140, 637–646. doi: 10.1104/pp.105.072579
Soto, A., Ruiz, K. B., Ravaglia, D., Costa, G., and Torrigiani, P. (2013). ABA may promote or delay peach fruit ripening through modulation of ripening- and hormone-related gene expression depending on the developmental stage. Plant Physiol. Biochem. 64, 11–24. doi: 10.1016/j.plaphy.2012.12.011
Sun, L., Sun, Y., Zhang, M., Wang, L., Ren, J., Cui, M., et al. (2012). Suppression of 9-cis-epoxycarotenoid dioxygenase, which encodes a key enzyme in abscisic acid biosynthesis, alters fruit texture in transgenic tomato. Plant Physiol. 158, 283–298. doi: 10.1104/pp.111.186866
Sun, Y., Chen, P., Duan, C., Tao, P., Wang, Y., Ji, K., et al. (2013). Transcriptional regulation of genes encoding key enzymes of abscisic acid metabolism during melon (Cucumis melo L.) fruit development and ripening. J. Plant Growth Regul. 32, 233–244. doi: 10.1007/s00344-012-9293-5
Tian, L., Jia, H. F., Li, C. L., Fan, P. G., Xing, Y., and Shen, Y. Y. (2012). Sucrose accumulation during grape berry and strawberry fruit ripening is controlled predominantly by sucrose synthase activity. J. Hortic. Sci. Biotechnol. 87, 661–667. doi: 10.1080/14620316.2012.11512927
Törrönen, R., Kolehmainen, M., Sarkkinen, E., Poutanen, K., Mykkänen, H., and Niskanen, L. (2013). Berries reduce postprandial insulin responses to wheat and rye breads in healthy women. J. Nutr. 143, 430–436. doi: 10.3945/jn.112.169771
Van den Ende, W., and El-Esawe, S. K. (2014). Sucrose signaling pathways leading to fructan and anthocyanin accumulation: A dual function in abiotic and biotic stress responses? Environ. Exp. Bot. 108, 4–13. doi: 10.1016/j.envexpbot.2013.09.017
Villalobos-González, L., Peña-Neira, A., Ibáñez, F., and Pastenes, C. (2016). Long-term effects of abscisic acid (ABA) on the grape berry phenylpropanoid pathway: Gene expression and metabolite content. Plant Physiol. Biochem. 105, 213–223. doi: 10.1016/j.plaphy.2016.04.012
Vishwakarma, K., Upadhyay, N., Kumar, N., Yadav, G., Singh, J., Mishra, R. K., et al. (2017). Abscisic acid signaling and abiotic stress tolerance in plants: A review on current knowledge and future prospects. Front. Plant Sci. 8:161. doi: 10.3389/fpls.2017.00161
Wang, Y., Wang, Y., Ji, K., Dai, S., Hu, Y., Sun, L., et al. (2013). The role of abscisic acid in regulating cucumber fruit development and ripening and its transcriptional regulation. Plant Physiol. Biochem. 64, 70–79. doi: 10.1016/j.plaphy.2012.12.015
Wei, Y.-Z., Hu, F.-C., Hu, G.-B., Li, X.-J., Huang, X.-M., and Wang, H.-C. (2011). Differential expression of anthocyanin biosynthetic genes in relation to anthocyanin accumulation in the pericarp of Litchi chinensis Sonn. PLoS ONE 6:e19455. doi: 10.1371/journal.pone.0019455
Wheeler, S., Loveys, B., Ford, C., and Davies, C. (2009). The relationship between the expression of abscisic acid biosynthesis genes, accumulation of abscisic acid and the promotion of Vitis vinifera L. berry ripening by abscisic acid. Aust. J. Grape Wine Res. 15, 195–204. doi: 10.1111/j.1755-0238.2008.00045.x
Wu, J., Zhao, G., Yang, Y.-N., Le, W.-Q., Khan, M. A., Zhang, S.-L., et al. (2013). Identification of differentially expressed genes related to coloration in red/green mutant pear (Pyrus communis L.). Tree Genet. Genom. 9, 75–83. doi: 10.1007/s11295-012-0534-3
Zaharah, S. S., Singh, Z., Symons, G. M., and Reid, J. B. (2013). Mode of action of abscisic acid in triggering ethylene biosynthesis and softening during ripening in mango fruit. Posharvest Biol. Technol. 75, 37–44. doi: 10.1016/j.postharvbio.2012.07.009
Zhang, M., Yuan, B., and Leng, P. (2009). The role of ABA in triggering ethylene biosynthesis and ripening of tomato fruit. J. Exp. Bot. 60, 1579–1588. doi: 10.1093/jxb/erp026
Zhang, Y.-J., Wang, X.-J., Wu, J.-X., Chen, S.-Y., Chen, H., Chai, L.-J., et al. (2014). Comparative transcriptome analyses between a spontaneous late-ripening sweet orange mutant and its wild type suggest the functions of ABA, sucrose and JA during citrus fruit ripening. PLoS ONE 9:e116056. doi: 10.1371/journal.pone.0116056
Zhao, C., Hua, L.-N., Liu, X.-F., Li, Y.-Z., Shen, Y.-Y., and Guo, J.-X. (2017). Sucrose synthase FaSS1 plays an important role in the regulation of strawberry fruit ripening. Plant Growth Regul. 81, 175–181. doi: 10.1007/s10725-016-0189-4
Zheng, Y., Tian, L., Liu, H., Pan, Q., Zhan, J., and Huang, W. (2009). Sugars induce anthocyanin accumulation and flavanone 3-hydroxylase expression in grape berries. Plant Growth Regul. 58, 251–260. doi: 10.1007/s10725-009-9373-0
Zhou, H., Lin-Wang, K., Wang, H., Gu, C., Dare, A. P., Espley, R. V., et al. (2015). Molecular genetics of blood-fleshed peach reveals activation of anthocyanin biosynthesis by NAC transcription factors. Plant J. 82, 105–121. doi: 10.1111/tpj.12792
Zhu, M., Chen, G., Zhou, S., Tu, Y., Wang, Y., Dong, T., et al. (2014). A new tomato NAC (NAM/ATAF1/2/CUC2) transcription factor, SlNAC2, functions as a positive regulator of fruit ripening and carotenoid accumulation. Plant Cell Physiol. 55, 119–135. doi: 10.1093/pcp/pct162
Zifkin, M., Jin, A., Ozga, J. A., Zaharia, L. I., Schernthaner, J. P., Gesell, A., et al. (2012). Gene expression and metabolite profiling of developing highbush blueberry fruit indicates transcriptional regulation of flavonoid metabolism and activation of abscisic acid metabolism. Plant Physiol. 158, 200–224. doi: 10.1104/pp.111.180950
Keywords: Vaccinium, non-climacteric fruit, berry ripening, hormonal regulation, signaling molecules, anthocyanins, abscisic acid, sucrose
Citation: Karppinen K, Tegelberg P, Häggman H and Jaakola L (2018) Abscisic Acid Regulates Anthocyanin Biosynthesis and Gene Expression Associated With Cell Wall Modification in Ripening Bilberry (Vaccinium myrtillus L.) Fruits. Front. Plant Sci. 9:1259. doi: 10.3389/fpls.2018.01259
Received: 09 June 2018; Accepted: 09 August 2018;
Published: 29 August 2018.
Edited by:
Francisco J. Corpas, Consejo Superior de Investigaciones Científicas (CSIC), SpainReviewed by:
Chiara Pastore, Università degli Studi di Bologna, ItalyRichard Victor Espley, The New Zealand Institute for Plant & Food Research Ltd., New Zealand
Copyright © 2018 Karppinen, Tegelberg, Häggman and Jaakola. This is an open-access article distributed under the terms of the Creative Commons Attribution License (CC BY). The use, distribution or reproduction in other forums is permitted, provided the original author(s) and the copyright owner(s) are credited and that the original publication in this journal is cited, in accordance with accepted academic practice. No use, distribution or reproduction is permitted which does not comply with these terms.
*Correspondence: Laura Jaakola, bGF1cmEuamFha29sYUB1aXQubm8=