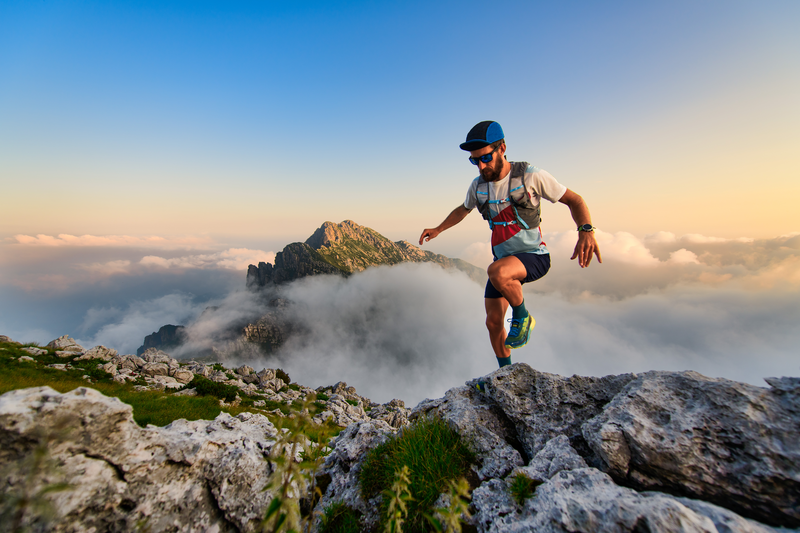
94% of researchers rate our articles as excellent or good
Learn more about the work of our research integrity team to safeguard the quality of each article we publish.
Find out more
REVIEW article
Front. Plant Sci. , 22 August 2018
Sec. Plant Cell Biology
Volume 9 - 2018 | https://doi.org/10.3389/fpls.2018.01232
This article is part of the Research Topic New Insights into Mechanisms of Epigenetic Modifiers in Plant Growth and Development View all 15 articles
In eukaryotes, genetic material is packaged into a dynamic but stable nucleoprotein structure called chromatin. Post-translational modification of chromatin domains affects the expression of underlying genes and subsequently the identity of cells by conveying epigenetic information from mother to daughter cells. SWI/SNF chromatin remodelers are ATP-dependent complexes that modulate core histone protein polypeptides, incorporate variant histone species and modify nucleotides in DNA strands within the nucleosome. The present review discusses the SWI/SNF chromatin remodeler family, its classification and recent advancements. We also address the involvement of SWI/SNF remodelers in regulating vital plant growth and development processes such as meristem establishment and maintenance, cell differentiation, organ initiation, flower morphogenesis and flowering time regulation. Moreover, the role of chromatin remodelers in key phytohormone signaling pathways is also reviewed. The information provided in this review may prompt further debate and investigations aimed at understanding plant-specific epigenetic regulation mediated by chromatin remodeling under continuously varying plant growth conditions and global climate change.
The packaging of genetic material into nucleosomes is a distinctive evolutionary feature of eukaryotic cells. Nucleosomes are repetitive units consisting of 147 bp DNA strands tightly wrapped around a central octamer comprising one histone heterotetramer (H3/H4) and two histone heterodimers (H2A/H2B). Histones are highly conserved proteins found in all eukaryotes as core nucleosome units (Suzuki and Bird, 2008). Together, the histone octamer and DNA resemble “beads” on a string and are referred to as chromatin (Luger et al., 1997). This packaging ensures that long DNA strands are tightly condensed by supercoiling to precisely fit into the nucleus. Such compaction of nucleosomes in chromatin hinders DNA accessibility to important regulatory proteins essential for various nuclear processes. However, cells have evolved in response to this impediment through chromatin remodeling (Groth et al., 2007).
Gene regulatory mechanisms in eukaryotic cells (such as transcription, DNA repair and replication) act upon chromatin structure as a substrate. These activities induce cellular changes and regulate gene expression in a number of biological processes, including genome stability, recombination, developmental reprogramming and response to extracellular signals (Feng et al., 2010; Soria et al., 2012; Zhu et al., 2013). The regulatory precision of these fundamental genome processes relies on the high fidelity of chromatin remodeling mechanisms that permit temporal access to – or blocking of – vital DNA sequences, such as gene promoters. Histone modifications, nucleosome remodeling and DNA methylation have been shown to regulate chromatin structure and gene expression (Henikoff and Shilatifard, 2011).
There are two main players regulating chromatin dynamics: (1) chromatin remodelers that alter DNA-histone interactions by energy harnessed through ATP hydrolysis and (2) nucleosome-modifying enzymes that modulate DNA and histone residues by specifically adding or removing covalent modifications (Jerzmanowski, 2007). Organisms rely on gene expression regulation to achieve normal cell differentiation, organogenesis, growth and development. Moreover, gene expression regulation is temporally and spatially coordinated via crosstalk between chromatin remodeling complexes (CRCs) and the transcription machinery (Deem et al., 2012). In plants, such precise control of gene expression mediated by chromatin modifications in response to endogenous and environmental stimuli is fundamental for proper development and reproductive success (Sarnowski et al., 2005; Bezhani et al., 2007; Han et al., 2012; Archacki et al., 2013; Efroni et al., 2013; Sarnowska et al., 2013; Qin et al., 2014; Vercruyssen et al., 2014).
Plant development can be divided into the embryonic and postembryonic phases. The embryonic development phase includes the establishment of the seedling apical-basal axis, the shoot apical meristem (SAM) and the root apical meristem (RAM). The subsequent establishment of the leaf, stem and flower meristems occurs in the postembryonic phase. In this review, we discuss recent investigations underpinning the involvement of SWI/SNF chromatin remodeling ATPases in regulating key plant growth and development processes, including meristem establishment and maintenance, cell differentiation, organ initiation, flower morphogenesis and flowering time regulation. We also discuss the role of chromatin remodelers in the plant response to key phytohormone signals. This overview may be useful for framing the current knowledge gaps, thereby stimulating further debate and research aimed at a comprehensive understanding of epigenetic regulatory mechanisms in plant development. This in turn may guide molecular-based plant improvement techniques for desirable agronomic traits.
Chromatin remodeling complexes are evolutionarily conserved multi-unit protein complexes that regulate chromatin structure by altering nucleosome composition and interactions (Narlikar et al., 2002). A common feature of all purified CRCs is that they harbor an ATPase/helicase of the SWITCHING DEFECTIVE2/SUCROSE NON-FERMENTING2 (SWI2/SNF2) family as their catalytic core. The SWI/SNF family is part of superfamily2 (SF2), a large family of helicases and translocases, and is named after the first identified CRC (Peterson et al., 1994). SWI/SNF CRCs utilize the energy derived from ATP hydrolysis to control accessibility to vital DNA sequences by influencing nucleosome structure and position and determining the kind of variant histone subspecies to be incorporated (Eisen et al., 1995; Clapier and Cairns, 2009).
A number of Arabidopsis SWI/SNF complex subunits have been identified based on sequence similarity with metazoan subunits. These include four SWI2/SNF2 ATPases (BRAHMA [BRM], SPLAYED [SYD], MINU1/CHR12 and MINU2/CHR23) (Knizewski et al., 2008; Han et al., 2015); four SWI3 proteins (SWI3A to SWI3D); two SWI/SNF ASSOCIATED PROTEINS 73 (SWP73A/CHC2 and SWP73B/CHC1, also called BRAHMA ASSOCIATED FACTOR 60 (BAF60) in humans); two ACTIN RELATED PROTEINS belonging to SWI/SNF complexes (ARP4 and ARP7); and the BUSHY (BSH) protein (Meagher et al., 2005; Jerzmanowski, 2007; Kwon and Wagner, 2007; Sang et al., 2012). Eukaryotic SWI2/SNF2 family chromatin remodelers can be categorized into four classes/subfamilies based on phylogenetic analysis, and all four of these subfamilies are represented in plants (Farrona et al., 2008).
The SWI/SNF subfamily of remodelers initially purified from Saccharomyces cerevisiae is probably the most comprehensively studied. SWI/SNF subfamily CRCs have a well-established role in gene expression regulation. The SWI/SNF subfamily remodelers contain 8–14 subunits, and the catalytic ATPases of most SWI/SNF subfamily remodelers are composed of a helicase-SANT domain, a post-HSA domain and a C-terminal bromodomain. While the fungal SWI/SNF subfamily ATPases consist of a pair of actin-related proteins (ARPs) (Cairns et al., 1998), higher orthologs of SWI/SNF subfamily complexes contain a dimer of an ARP and actin (Lessard et al., 2007). The mode of action of SWI/SNF complexes has been characterized as sliding and/or ejecting nucleosomes at many target loci, but they reportedly have no vital role in the assembly of eukaryotic chromatin structure (Clapier and Cairns, 2009). It was recently reported that the Arabidopsis SWI/SNF complex expedites its role in activating and repressing target gene expression by binding to both promoters and terminators and that it regulates the expression of both promoter-centered genes and non-coding RNAs (Archacki et al., 2017).
The ISWI subfamily remodelers have 2–4 subunits. Eukaryotic ISWI remodeler complexes typically have 1 or 2 distinct catalytic subunits and specialized attendant proteins that give rise to various domains. These domains include plant homeodomains, bromodomains, DNA-binding histone fold motifs and additional DNA-binding motifs (Clapier and Cairns, 2009). ISWI complexes were initially purified from Drosophila melanogaster. They include the nucleosome remodeling factor (dNURF), chromatin accessibility complex (dCHRAC) and ATP-utilizing chromatin assembly and remodeling factor (dACF) (Corona and Tamkun, 2004). SANT-like ISWI ATPases contain a SANT domain adjacent to a SLIDE domain in their C-terminus, which acts as a binding site for unmodified histone tails and DNA. The diversity resulting from attendant subunits is exhibited in the modes of action of the ISWI family complexes. Both ACF and CHRAC have been shown to promote chromatin assembly and transcription repression through the optimization of nucleosome spacing, whereas NURF assists RNA polymerase II (RNAPII) activation by randomizing nucleosome spacing (Corona and Tamkun, 2004).
The CHD subfamily remodelers were first purified from Xenopus laevis and comprise 1–10 subunits. The N-terminus of their catalytic subunit consists of two chromodomains arranged tandemly, with the composition varying from monomeric in lower eukaryotes to multimeric in vertebrates (Marfella and Imbalzano, 2007). CHD subfamily remodelers vary in their structure, composition and function, due in part to the diversity of their chromodomains. One of the members of the CHD subfamily in vertebrates, the nucleosome remodeling and deacetylase (Mi-2/NuRD) complex, has repressive roles due to its inclusion of histone deacetylases (HDAC1/2) and methyl CpG-binding domain (MBD) proteins. In contrast, other CHD subfamily remodelers have been reported to promote transcription by either ejecting or sliding nucleosomes along the DNA double strand (Denslow and Wade, 2007).
The INO80 subfamily is the most recently characterized chromatin remodeler subfamily. Many INO80 subunit homologs have been identified in yeast, fruit fly, mammals and plants, making it the most conserved subfamily. The presence of a split ATPase subunit distinguishes INO80 complexes from the SWI/SNF, CHD and ISWI subfamilies (Morrison and Shen, 2009). In S. cerevisiae, the INO80 subfamily is represented by two complexes, INO80 and Swi2/snf2-related 1 (SWR1); in mammals, it is represented by three complexes, INO80, Snf2-related CBP activator protein (SRCAP) and p400. D. melanogaster has INO80 and p400 complexes (Morrison and Shen, 2009; Bao and Shen, 2011). S. cerevisiae INO80 and SWR1 ATPases are approximately 1.2–1.5 MDa in mass and are reported to contain 15 and 14 subunits, respectively (Shen et al., 2000; Krogan et al., 2003; Mizuguchi et al., 2004). In general, the INO80 subfamily has a relatively conserved composition of individual complexes and a high degree of homology in the ATPase subunit. Studies of the S. cerevisiae SWR1 complex indicate the importance of a spacer (split) region in the ATPase subunit, as deletion of this region leads to dissociation of a number of subunits from the complex, including the RuvB-like Rvb1 and Rvb2 subunits (Wu et al., 2005). The INO80 subfamily is responsible for various functions in eukaryotic cells including transcriptional activation and DNA double-strand break (DSB) repair (Ebbert et al., 1999).
Plant development is characterized by the presence of stem cells with the capacity to self-renew and transform into tissue-specific founder cells. The stem cells of the RAM and SAM have been widely studied in plants and are found at the root and shoot tips, respectively (Shen and Xu, 2009). Stem cell homeostasis and identity in both plants and mammals are associated with the activity of SWI/SNF chromatin remodeling ATPases (Ori et al., 2000; Kwon et al., 2005; Ho et al., 2009; Aichinger et al., 2011). Perturbations in the Polycomb group (PcG) repressive complex, histone acetylation or chromatin assembly may lead to improper RAM or SAM development (Phelps-Durr et al., 2005; Schubert et al., 2006; Barrero et al., 2007; Xu and Shen, 2008; Kornet and Scheres, 2009). Individual Arabidopsis SWI/SNF subfamily chromatin remodelers (BRM, SYD, CHR12 and CHR23) (Flaus et al., 2006) are also involved in regulating numerous plant developmental processes (Farrona et al., 2004; Hurtado et al., 2006; Kwon et al., 2006; Bezhani et al., 2007; Tang et al., 2008; Wu et al., 2012).
SYD promotes SAM maintenance by binding to the promoter region of its target gene WUSCHEL (WUS) and activating WUS expression in the organizing center (OC) region of the SAM (Figure 1). Consistent with this function, Arabidopsis syd mutant plants exhibit reduced WUS transcript levels accompanied by abnormal SAM development (Kwon et al., 2005).
FIGURE 1. Chromatin remodelers in shoot and root apical meristem establishment and maintenance. SYD binds to the promoter region of WUS in the OC region of the SAM, activating WUS expression to promote SAM maintenance. CHR12 and CHR23 have redundant roles in plant development regulation, as single mutations in either produce no obvious defects but strong double mutations lead to impaired SAM maintenance. BRM acts in the PLT pathway to regulate the expression of PIN genes, which in turn influence auxin distribution in the QC region to ensure RAM maintenance.
The CHR12 and CHR23 ATPases have redundant roles in plant development processes including meristem initiation and maintenance. Plants with strong double mutant chr12chr23 combinations show embryonic lethality, endosperm defects and failure to initiate stem cell populations in both roots and shoots, whereas plants with weak double mutant chr12chr23 combinations are small viable plants with noticeable defects in RAM and SAM maintenance (Sang et al., 2012).
A study by Wu et al. (2015) demonstrated a role of chromatin in local developmental progressions in the root and shoot. In the SAM, cells with high auxin levels initiate proteolysis of Aux/IAA leading to the dissociation of the co-repressor complex TOPLESS-HISTONE DEACETYLASE 19 (TPL-HDA19). This enables the recruitment of SYD and BRM to direct the acquisition of flower primordium founder cell fate (Wu et al., 2015). In the RAM, a small group of mitotically inactive cells known as the quiescent center (QC) maintains the root stem cells. BRM was found to act in the PLETHORA (PLT) pathway to maintain the root stem cell niche by altering the expression of PIN-FORMED (PIN) genes responsible for auxin distribution in the RAM, as illustrated in Figure 1. brm mutants exhibit faulty root stem cell niche maintenance, reduced meristem activity and retarded root growth (Yang et al., 2015).
To attain continuous plant growth, cells derived from pluripotent stem cells must undergo asymmetric cell division and expansion. This allows for the generation of lateral organs and the maintenance of stem cell populations for additional growth (Jarillo et al., 2009). The role of BRM in ensuring asymmetric cell division and cell fate determination in both plant and animal stem cells was discussed extensively in a recent review (Pillitteri et al., 2016).
Our current knowledge of mechanisms involving chromatin-mediated regulation of plant stem cell initiation and maintenance remains limited to the few Arabidopsis SWI/SNF complexes discussed above. However, emerging evidence suggests complex interconnections among many players including key hormone signaling pathways. This underscores the ability of plants to activate or arrest lateral organ founder cell formation in response to both internal developmental signals and external biotic and abiotic stress signals. These attributes may eventually be harnessed or targeted for crop improvement applications.
Stem cells in the SAM initiate organ founder cells that later differentiate into aboveground organ-specific tissues such as leaves and stems (Sinha, 1999; Laux, 2003). Plant roots are formed from a reservoir of undifferentiated cells in the RAM called the root stem cells. Mature plant organs usually maintain relatively undifferentiated cells as a fallback for hormonal stimuli or mechanical injury, helping to generate new tissues and organs through cellular reprogramming (Ikeuchi et al., 2015). Cellular differentiation results from global changes in gene expression patterns and these changes involve many transcription regulators and are epigenetically mediated by chromatin remodeling (Bruex et al., 2012; Taylor-Teeples et al., 2015).
ANGUSTIFOLIA3 (AN3)/GRF-INTERACTING FACTOR1 (GIF1), a member of the GIF family of transcriptional coactivators, plays a key role in Arabidopsis shoot development (Kim and Kende, 2004), cotyledon identity establishment during embryogenesis (Kanei et al., 2012) and leaf size increase resulting from increased cell number (Horiguchi et al., 2005; Lee et al., 2009). Transcriptional coactivators often work together with DNA-binding transcription factors to promote transcription either by recruiting chromatin remodelers or stimulating general complex formation around RNA polymerase II (Pol II). Genetic interaction between AN3 and the Arabidopsis SWI/SNF chromatin remodeling complex BRM (Figure 2) suggests that AN3 recruits SWI/SNF complexes to promote cell division during leaf development (Vercruyssen et al., 2014). BRM is recruited to its target gene loci via association with the plant-specific H3K27 demethylase RELATIVE OF EARLY FLOWERING 6 (REF6). Through its zinc-finger (ZnF) domains, REF6 recognizes genomic loci harboring a CTCTGYTY motif (Li et al., 2016). A forward genetic screen in Arabidopsis indicated that BRM and SWINGER (SWN) (a key component of Polycomb Group Repressive Complex 2 in plants) antagonistically control vegetative phase change through the temporal expression of miR156 at the nucleosome level. Specifically, the accelerated vegetative phase change of brm mutants was accompanied by reduced miR156 expression and increased levels of H3K27me3 at the MIR156A locus (Xu et al., 2016).
FIGURE 2. Chromatin remodelers in cell differentiation and organ development regulation. The recruitment of BRM by the transcriptional coactivator AN3 promotes the transcription of genes responsible for leaf development. Direct binding of SWP73B to the promoter regions of KANADI1/3, YABBY2/3/5 and ASYMMETRIC LEAVES1/2 also controls leaf development. Physical interaction between PIF1 and BRM regulates chlorophyll biosynthesis by affecting the expression levels of associated enzymes.
SWP73 subunits of Arabidopsis SWI/SNF CRCs function in various plant development pathways including the regulation of leaf and flower development (Vercruyssen et al., 2014; Sacharowski et al., 2015). For example, SWP73B directly binds to the promoters of the KANADI1/3, YABBY2/3/5 and ASYMMETRIC LEAVES1/2 genes involved in leaf development (Figure 2) (Sacharowski et al., 2015). RNA interference (RNAi) silencing of SWP73B leads to dwarfism in Arabidopsis, evidencing its role in plant development (Crane and Gelvin, 2007).
A study of the chromatin remodeler protein ZmCHB101, which is the core subunit of maize SWI3, revealed its key roles in normal maize growth and development. ZmCHB101 controls the transcriptional reprogramming of a set of genes involved in gene expression regulation, photosynthesis, metabolic regulation and stress response. The RNAi maize lines generated in the study exhibited improper tassel and cob development and abaxially curling leaves caused by increased bulliform cell numbers (Yu et al., 2016).
Chlorophyll biosynthesis is a critical mark of the transition from heterotrophic to autotrophic growth in plants. Physical interaction between BRM and the transcription factor PHYTOCHROME-INTERACTING FACTOR 1 (PIF1) was shown to regulate chlorophyll biosynthesis in Arabidopsis (Figure 2). When exposed to light, dark-grown brm plants exhibit higher greening rates, reduced protochlorophyllide accumulation and lower levels of reactive oxygen species (ROS) compared to wild-type plants; there is also increased expression of NADPH:protochlorophyllide oxidoreductase A (PORA), PORB and PORC, enzymes which accelerate a key step in chlorophyll biosynthesis (Zhang et al., 2017).
The importance of chromatin remodelers for proper plant growth progression and development is exemplified by their involvement in asymmetric cell differentiation and in initiating organ founder cells. Proper plant growth also involves precise developmental phase transitions, and the antagonism between SWI/SNF ATPases and PcG repressive complex components is crucial for these shifts.
The precise regulation of the transition from vegetative growth to flowering is paramount for plant reproductive success. This process is characterized by the transition of the vegetative SAM into an inflorescence meristem (IM) and the initiation of floral meristems (FMs) (Sablowski, 2007; Kaufmann et al., 2010). While SAM maintenance ensures indeterminate plant growth, the determinate nature of FMs determines reproductive success, seed development and the yield of agricultural crops (Liu et al., 2011). Complex regulatory networks of transcription factors and chromatin remodelers guide flowering time and flower development while integrating both internal and external signals (Wils and Kaufmann, 2017). These networks comprise photoperiod, vernalization and thermo-sensory pathways for sensing long days, cold winter and ambient temperature, respectively, together with pathways responsive to internal factors, such as the age pathway and the gibberellins (GA) signaling pathway (He, 2012).
In Arabidopsis, a repressor complex that consists of the two MADS box transcription factors MADS AFFECTING FLOWERING 4/5 (MAF4/5), FLOWERING LOCUS C (FLC) and SHORT VEGETATIVE PHASE (SVP) serves as a negative regulator of flowering time. The components directly repress the expression of the floral pathway integrators FLOWERING LOCUS T (FT) and SUPPRESSOR OF OVEREXPRESSION OF CONSTANS 1 (SOC1) (Lee et al., 2007; Li et al., 2008; He, 2012). FRIGIDA (FRI) promotes higher FLC levels that inhibit flowering (Michaels and Amasino, 2001) through the recruitment of multiple active chromatin modifications at the FLC locus (Choi et al., 2011). Vernalization overrides the FRI-mediated activation of FLC expression and thereby enables flowering (Kim et al., 2009; Crevilleńn and Dean, 2011).
The core subunit components of the Arabidopsis SWR1 chromatin remodeling complex (including PHOTOPERIOD-INDEPENDENT EARLY FLOWERING 1 (PIE1), ACTIN RELATED PROTEIN 6 (ARP6) and SWR1 COMPLEX 6 (SWC6)/SERRATED LEAVES AND EARLY FLOWERING (SEF)) have been shown to play important roles in regulating the proper growth and development of most plant organs. Importantly, SWR1 controls plant development by generating a balance between microRNAs and target mRNAs at the transcriptional level (Choi et al., 2016). It was recently demonstrated that SWR1 regulates gene expression by establishing lowly accessible and highly accessible nucleosome structure at the first nucleosome upstream and downstream of the transcription start site (TSS), respectively (Dai et al., 2017). SWR1 subunit loss-of-function mutants exhibit pleiotropic phenotypes including early flowering, serrated leaves, frequent absence of inflorescence internodes, bushy growth and flowers with altered organ number and size (Choi et al., 2005; Deal et al., 2005; Martin-Trillo et al., 2006; March-Diaz et al., 2007; Qin et al., 2014). H2A.Z deposition around the FLC TSS by SWR1 is necessary for FRI-meditated activation of FLC (Figure 3). One line of evidence is that functional disruption of SWR1 prevents H2A.Z deposition at FLC chromatin, suppressing its expression and leading to early flowering (Choi et al., 2007; Deal et al., 2007). Additionally, PIE1 mutations were earlier shown to substantially reduce FLC transcript levels, with a concomitant conversion of the winter-annual habit to the rapid flowering summer-annual habit (Noh and Amasino, 2003). A recent study revealed an Arabidopsis homolog of the yeast SANT domain protein Swc4/Eaf2 as a novel SWR1-C subunit. SWC4 is a DNA-binding protein that contributes to the recruitment SWR1-C through specific recognition of AT-rich DNA segments in chromatin regions of target genes to deposit H2A.Z. Further, knock-out and knockdown studies showed that SWC4 is essential for both embryo viability and the control of post-embryonic processes, including flowering time, by repressing transcription of a number of genes including the floral integrator FT and key transcription factors (Gomez-Zambrano et al., 2018).
FIGURE 3. Chromatin modulation in flowering regulation. Red lines with perpendicular bars denote repression, blue arrows indicate activation, and colored ovals represent proteins. Floral transition in the SAM involves IM and FM specification and is regulated by a balance between environmental and internal factors. SYD, BRM and SWR1 chromatin remodeling complexes coordinate with other factors to influence floral development by regulating the expression of critical genes, such as AG, FT, FLC and SVP, in response to both external and internal stimuli.
The AtINO80 complex is required for somatic homologous recombination (HR) and expression regulation of FLC and MAF4/5 by facilitating the enrichment of H2A.Z at their ends. Independent Atino80-5 and Atino80-6 mutant alleles display similar pleiotropic phenotypes of small plant size, reduced organ size and late flowering. These observations provide a link between plant responses to environmental and developmental signals and epigenetic mechanisms involved in plant chromatin stability (Zhang et al., 2015).
The SWP73B (BAF60) subunit of the Arabidopsis SWI/SNF complex has also been implicated in flowering time control through its involvement in chromatin loop formation at the FLC locus (Jegu et al., 2014). SWP73A has been shown to be confined to flowering time modulation under short day conditions, with functional overlap between SWP73A and SWP73B during embryogenesis (Sacharowski et al., 2015).
SVP, another key flowering repressor, is highly expressed during the vegetative phase to promote growth (Hartmann et al., 2000) but is down-regulated during the floral transition by the autonomous and GA signaling pathways (Li et al., 2008). FT and SOC1 are subsequently activated to promote flowering (Figure 3). One study proposed that BRM controls flowering time in Arabidopsis by directly activating SVP expression (Li et al., 2015). Genome-wide analysis of H3K27me3, a histone mark associated with gene repression, in brm mutant seedlings revealed increased H3K27me3 deposition at several genes including SVP, indicating an antagonism between BRM and PcG repressor proteins (Li et al., 2015).
Another key flowering time regulator in Arabidopsis activated by CONSTANS (CO) under long days to induce flowering is the FT-encoded florigen protein (FT) (Corbesier et al., 2007). FT moves from the phloem to the SAM and forms a complex with the bZIP transcription factor FD to activate the expression of the FM identity genes LEAFY (LFY) and APETALA1 (AP1). This activation leads to the formation of the floral primordium, which sequentially generates three types of lateral floral organs (sepals, petals and stamens) (Zik and Irish, 2003; Abe et al., 2005; Wigge et al., 2005). LFY recruits SYD and BRM ATPases to induce the expression of the floral homeotic regulator AGAMOUS (AG) by removing H3K27me3 marks on its second intron (Figure 3) (Wu et al., 2012). The SWR1 complex is also involved in FT expression regulation, with functional disruption of SWR1 leading to temperature-insensitive FT activation and early flowering. An ambient temperature increase from 17 to 27°C causes eviction of the H2A.Z nucleosomes, which enables FT transcription by Pol II (Kumar and Wigge, 2010).
Studies of the CHD3 chromatin remodeler PICKLE (PKL) have highlighted its role in plant reproductive development by promoting crosstalk between the sporophytic and gametophytic generations. Loss of PKL in the maternal sporophyte leads to improper development of the Arabidopsis female gametophyte, integument and pollen tube, accompanied by delayed ovule and embryo development (Carter et al., 2016).
It was recently shown that during megasporogenesis, somatic cells cooperatively use SWR1 to restrict female reproductive founder cell specification to a single cell in the ovule primordia by incorporating H2A.Z at a particular WRKY28 nucleosome and promoting expression of the gene (Zhao et al., 2018). The WRKY28 transcription factor is exclusively expressed in hypodermal somatic cells surrounding the megasporocyte and represses those cells from differentiating into functional megasporocytes (Zhao et al., 2018). Other separate findings demonstrated that both BRM and SWR1 are involved in determining inflorescence architecture in response to developmental cues (Zhao et al., 2015; Cai et al., 2017).
The above findings reveal mechanisms involving the recruitment of SWI/SNF ATPases to specific flower development regulatory pathways at an appropriate time. They also emphasize the centrality of SWI/SNF complexes in shaping plant growth and ensuring the continuity of plant viability through generations by precise spatiotemporal control of the relevant developmental processes. Nevertheless, these conclusions about important regulatory mechanisms are generally based on findings from model plants such as Arabidopsis. Thus, the understanding of such regulatory mechanisms in agriculturally important crop species remains inadequate and will require further investigation.
Plant hormones are critical for the proper regulation of plant growth and development processes including seed germination, vegetative and reproductive growth and abiotic stress response. The biosynthesis and degradation of plant hormones are also tightly controlled through the transcriptional regulation of target genes. SWI/SNF ATPases are involved in plant hormone responses through physical interaction with hormone signaling pathway components and transcriptional regulators of genes involved in hormone biosynthesis and perception (Sarnowska et al., 2016).
Abscisic acid (ABA) is an important phytohormone that promotes seed dormancy and arrests growth in post-germination embryos under water stress conditions. In the absence of stress stimuli, BRM was initially implicated in repressing the activity of the ABA pathway, with adult brm mutants exhibiting increased drought tolerance (Han et al., 2012). It was later revealed that important ABA signaling pathway components physically interact with BRM, leading to post-translational phosphorylation/dephosphorylation of BRM. For example, phosphorylation of BRM by SnRK2 kinases leads to its inhibition, while PP2CA-mediated dephosphorylation restores the ability of BRM to repress the ABA response (Figure 4). Moreover, the phosphomimetic BRM mutant shows hypersensitivity to ABA (Peirats-Llobet et al., 2016).
FIGURE 4. Phytohormone signaling response under chromatin remodeling. Blue arrows represent activation/interaction, blue lines with perpendicular bars denote inhibition, red dashed lines indicate the mutated state of an ATPase, and the yellow arrow represents overexpression. Both BRM and PKL positively regulate GA biosynthesis by activating underlying genes. Phosphorylation of BRM and overexpression of CHR12 both activate the ABA response pathway, while dephosphorylation of BRM and interaction of SWI3B with HABI repress the ABA response. SWP73B negatively regulates CK biosynthesis and CK-mediated cell cycle changes. Mutations in BRM and SYD inhibit GA and IAA biosynthesis, while normal SYD positively regulates JA- and ET-dependent gene expression.
BRM has also been shown to play a direct role in the positive regulation of GA biosynthesis by binding to chromatin near the GA3ox1 promoter, thereby activating the gene (Figure 4). Arabidopsis brm null mutants exhibit a significant decrease in active GA levels (Archacki et al., 2013). Furthermore, transcriptional profiling revealed that most genes involved in the GA and auxin signaling pathways are affected in both syd and brm null mutants (Bezhani et al., 2007). Previous studies showed that plants over-expressing CHR12 had enhanced growth arrest when exposed to drought or heat stress (Mlynarova et al., 2007). In addition, SYD was linked to ethylene (ET)- and jasmonic acid (JA)-dependent gene regulation (Walley et al., 2008), and the SWI3B subunit was found to interact with a negative regulator of ABA signaling, the PP2C-type phosphatase HAB1 (Saez et al., 2008).
During development, plants transition from the embryonic stage to the seedling stage. Previous studies have characterized the joint effects of PKL and GA in repressing embryonic traits during plant development transitions (Zhang et al., 2008; Zhang et al., 2012). Specifically, PKL expression represses the embryonic state by controlling a significant number of GA-responsive genes. pkl seedlings exhibit a semi-dwarf phenotype similar to that of GA-response mutants and are able to express embryonic traits under GA-biosynthesis inhibition. In contrast, treatment of pkl mutants with GA greatly decreases their characteristic pickle-root phenotype (Henderson et al., 2004). Both PKL and BRM CRCs have been proposed to act as positive regulators of the GA pathway possibly through distinct mechanisms. Unlike brm mutants, pkl mutant plants have an increased abundance of active GA (Archacki et al., 2013). Recently, PKL was found to positively regulate most GA-mediated developmental processes including promoting vegetative growth (hypocotyl, leaf, and inflorescence stem elongation) and phase transitions (i.e., the juvenile-to-adult and vegetative-to-reproductive transitions) (Park et al., 2017).
Excess cytokinin (CK) production inhibits primary root elongation and weakens lateral root development (Kuderova et al., 2008). SWP73B is one of the accessory subunits of Arabidopsis SWI/SNF complexes shown to positively regulate root development and cell cycle progression in the root meristem by suppressing CK biosynthesis (Figure 4). SWP73B negatively regulates the CK biosynthesis genes ADENOSINE PHOSPHATE-ISOPENTENYLTRANSFERASE (IPT3) and (IPT7) and the CK-regulated cell cycle inhibitor KIP-RELATED PROTEIN7 (KRP7) by hindering the deposition of active histone marks on their gene bodies (Jegu et al., 2015).
In aggregate, these findings indicate that both phytohormone biosynthesis and degradation in response to extracellular and intracellular cues partly depend on SWI/SNF chromatin remodeling ATPases. The findings also suggest the role of SWI/SNF ATPases as a hub in plant perception and response to phytohormone signaling as well as hormone crosstalk through direct physical interactions with hormone signaling pathway components. The outcomes of these interactions influence the plant response to abiotic stress, enabling plant adaptability under changing climates.
Chromatin remodeling has been extensively studied in the context of various regulatory and developmental processes in a number of eukaryotic organisms, including humans, mice, yeast, fruit fly and Arabidopsis. Phylogenetic analysis suggests that the chromatin remodeling machinery and their modes of operation are evolutionarily conserved across eukaryotes. However, SWI/SNF subunit loss-of-function studies have uncovered varied organism-specific phenotypes. These phenotypes also depend on the strength of a given mutation, leading to phenotypic differences among single and double mutants of SWI/SNF subunit genes. The functional redundancy of some SWI/SNF complex subunits also necessitates further in-depth analyses of their interaction patterns in different plant development pathways.
As discussed above, a number of plant regulatory and developmental transitions are controlled by chromatin modifiers through complex pathways in response to both endogenous and environmental factors. These precisely orchestrated mechanisms are heritable, underscoring their importance for plant survival in changing habitats. Moreover, the dynamics of these epigenetic controls go hand in hand with changes in plant growth conditions. Positive attributes that could potentially be integrated into plant breeding schemes are of interest to many plant scientists and breeders insofar as they may yield varieties that can withstand adverse weather conditions, early flowering or various biotic stresses and maximize nutrient utilization for improved yield. High-throughput technologies together with the vast genomic data presently available in public repositories should facilitate comparative and functional studies aimed at plant improvement. Future studies in other species of interest (e.g., rice and other crops) will likely build upon the findings on ATP-dependent CRCs in Arabidopsis and more recently in maize. Plant-specific molecular and genetic approaches should also characterize regulatory differences between developmental stages and under different growth conditions for a more comprehensive understanding of the involvement of SWI/SNF chromatin modifiers in plant growth and development.
The following research questions and topics are of particular interest in terms of how CRCs may be involved in various processes either directly or indirectly related to plant development.
(1) Involvement of SWI/SNF ATPases in flowering regulation in the temperate legume model plant Medicago truncatula, whose flowering is prompted by winter cold and long-day photoperiods despite the absence of an FLC gene.
(2) The role played by chromatin remodelers in oxidative stress remediation processes resulting from various metabolic activities in plant cells other than DNA double-strand break repair.
(3) Besides their known function in the phosphate starvation response, there is a need to ascertain other roles of SWI/SNF remodelers in mediating the uptake of essential micro- and macronutrients from the soil in conjunction with nutrient transporter gene families and rhizosphere microbial activities.
(4) The mechanism by which the chromatin remodeling complex SWR1 mediates chromatin structural dynamics by replacing the core H2A-H2B histone dimer with the H2A.Z-H2B dimer in octamers is well characterized. However, the molecular mechanisms underlying the reverse process remain elusive.
(5) The specific roles of accessory subunits of Arabidopsis SWI/SNF CRCs in regulating plant development are only beginning to be understood. The latest example is SWP73 involvement in the cell cycle, leaf development, flowering time and embryogenesis. Much remains to be uncovered in this regard.
SO and SC conceived the idea, wrote the manuscript, and drafted the figures. SP designed and organized figures and revised the manuscript. WL, MY, MA, and HZ revised the manuscript and organized the figures. YQ conceived the idea and revised and organized the manuscript.
This work was supported by NSFC (31761130074, U1605212, and 31470284) and a Newton Advanced Fellowship to YQ.
The authors declare that the research was conducted in the absence of any commercial or financial relationships that could be construed as a potential conflict of interest.
We extend our apologies to all our colleagues whose work could not be reviewed here owing to space limitations. We are indebted to Fujian Agriculture and Forestry University for its financial support and to members of the Qin Lab (Haixia Institute of Science and Technology, Centre for Genomics and Biotechnology) for their motivation and suggestions for this manuscript.
Abe, M., Kobayashi, Y., Yamamoto, S., Daimon, Y., Yamaguchi, A., Ikeda, Y., et al. (2005). FD, a bZIP protein mediating signals from the floral pathway integrator FT at the shoot apex. Science 309, 1052–1056. doi: 10.1126/science.1115983
Aichinger, E., Villar, C. B., Di Mambro, R., Sabatini, S., and Kohler, C. (2011). The CHD3 chromatin remodeler pickle and polycomb group proteins antagonistically regulate meristem activity in the Arabidopsis root. Plant Cell 23, 1047–1060. doi: 10.1105/tpc.111.083352
Archacki, R., Buszewicz, D., Sarnowski, T. J., Sarnowska, E., Rolicka, A. T., Tohge, T., et al. (2013). Brahma ATPase of the SWI/SNF chromatin remodeling complex acts as a positive regulator of gibberellin-mediated responses in Arabidopsis. PLoS One 8:e58588. doi: 10.1371/journal.pone.0058588
Archacki, R., Yatusevich, R., Buszewicz, D., Krzyczmonik, K., Patryn, J., Iwanicka-Nowicka, R., et al. (2017). Arabidopsis SWI/SNF chromatin remodeling complex binds both promoters and terminators to regulate gene expression. Nucleic Acids Res. 45, 3116–3129.
Bao, Y., and Shen, X. (2011). Snapshot: chromatin remodeling: INO80 and SWR1. Cell 144, 158–158.e2. doi: 10.1016/j.cell.2010.12.024
Barrero, J. M., Gonzalez-Bayon, R., del Pozo, J. C., Ponce, M. R., and Micol, J. L. (2007). INCURVATA2 encodes the catalytic subunit of DNA polymerase alpha and interacts with genes involved in chromatin-mediated cellular memory in Arabidopsis thaliana. Plant Cell 19, 2822–2838. doi: 10.1105/tpc.107.054130
Bezhani, S., Winter, C., Hershman, S., Wagner, J. D., Kennedy, J. F., Kwon, C. S., et al. (2007). Unique, shared, and redundant roles for the Arabidopsis SWI/SNF chromatin remodeling ATPases BRAHMA and SPLAYED. Plant Cell 19, 403–416. doi: 10.1105/tpc.106.048272
Bruex, A., Kainkaryam, R. M., Wieckowski, Y., Kang, Y. H., Bernhardt, C., Xia, Y., et al. (2012). A gene regulatory network for root epidermis cell differentiation in Arabidopsis. PLoS Genet. 8:e1002446. doi: 10.1371/journal.pgen.1002446
Cai, H., Zhao, L., Wang, L., Zhang, M., Su, Z., Cheng, Y., et al. (2017). ERECTA signaling controls Arabidopsis inflorescence architecture through chromatin-mediated activation of PRE1 expression. New Phytol. 214, 1579–1596. doi: 10.1111/nph.14521
Cairns, B. R., Erdjument-Bromage, H., Tempst, P., Winston, F., and Kornberg, R. D. (1998). Two actin-related proteins are shared functional components of the chromatin-remodeling complexes RSC and SWI/SNF. Mol. Cell 2, 639–651. doi: 10.1016/S1097-2765(00)80162-8
Carter, B., Henderson, J. T., Svedin, E., Fiers, M., McCarthy, K., Smith, A., et al. (2016). Cross-talk between sporophyte and gametophyte generations is promoted by chd3 chromatin remodelers in Arabidopsis thaliana. Genetics 203, 817–829. doi: 10.1534/genetics.115.180141
Choi, K., Kim, J., Hwang, H. J., Kim, S., Park, C., Kim, S. Y., et al. (2011). The FRIGIDA complex activates transcription of FLC, a strong flowering repressor in Arabidopsis, by recruiting chromatin modification factors. Plant Cell 23, 289–303. doi: 10.1105/tpc.110.075911
Choi, K., Kim, J., Muller, S. Y., Oh, M., Underwood, C., Henderson, I., et al. (2016). Regulation of microRNA-mediated developmental changes by the SWR1 chromatin remodeling complex. Plant Physiol. 171, 1128–1143.
Choi, K., Kim, S., Kim, S. Y., Kim, M., Hyun, Y., Lee, H., et al. (2005). Suppressor of Frigida3 encodes a nuclear actin-related protein6 required for floral repression in Arabidopsis. Plant Cell 17, 2647–2660. doi: 10.1105/tpc.105.035485
Choi, K., Park, C., Lee, J., Oh, M., Noh, B., and Lee, I. (2007). Arabidopsis homologs of components of the SWR1 complex regulate flowering and plant development. Development 134, 1931–1941. doi: 10.1242/dev.001891
Clapier, C. R., and Cairns, B. R. (2009). The biology of chromatin remodeling complexes. Annu. Rev. Biochem. 78, 273–304. doi: 10.1146/annurev.biochem.77.062706.153223
Corbesier, L., Vincent, C., Jang, S., Fornara, F., Fan, Q., Searle, I., et al. (2007). FT protein movement contributes to long-distance signaling in floral induction of Arabidopsis. Science 316, 1030–1033. doi: 10.1126/science.1141752
Corona, D. F., and Tamkun, J. W. (2004). Multiple roles for ISWI in transcription, chromosome organization and DNA replication. Biochim. Biophys. Acta 1677, 113–119. doi: 10.1016/j.bbaexp.2003.09.018
Crane, Y. M., and Gelvin, S. B. (2007). RNAi-mediated gene silencing reveals involvement of Arabidopsis chromatin-related genes in Agrobacterium-mediated root transformation. Proc. Natl. Acad. Sci. U.S.A. 104, 15156–15161. doi: 10.1073/pnas.0706986104
Crevilleńn, P., and Dean, C. (2011). Regulation of the floral repressor gene FLC: the complexity ofntranscription in a chromatin context. Curr. Opin. Plant Biol. 14, 38–44. doi: 10.1016/j.pbi.2010.08.015
Dai, X., Bai, Y., Zhao, L., Dou, X., Liu, Y., Wang, L., et al. (2017). H2A.Z represses gene expression by modulating promoter nucleosome structure and enhancer histone modifications in Arabidopsis. Mol. Plant 10, 1274–1292. doi: 10.1016/j.molp.2017.09.007
Deal, R. B., Kandasamy, M. K., McKinney, E. C., and Meagher, R. B. (2005). The nuclear actin-related protein ARP6 is a pleiotropic developmental regulator required for the maintenance of flowering locus C expression and repression of flowering in Arabidopsis. Plant Cell 17, 2633–2646. doi: 10.1105/tpc.105.035196
Deal, R. B., Topp, C. N., McKinney, E. C., and Meagher, R. B. (2007). Repression of flowering in Arabidopsis requires activation of flowering locus C expression by the histone variant H2A.Z. Plant Cell 19, 74–83. doi: 10.1105/tpc.106.048447
Deem, A. K., Li, X., and Tyler, J. K. (2012). Epigenetic regulation of genomic integrity. Chromosoma 121, 131–151. doi: 10.1007/s00412-011-0358-1
Denslow, S. A., and Wade, P. A. (2007). The human Mi-2/NuRD complex and gene regulation. Oncogene 26, 5433–5438. doi: 10.1038/sj.onc.1210611
Ebbert, R., Birkmann, A., and Schu¨ller, H. J. (1999). The product of the SNF2/SWI2 paralogue INO80 of Saccharomyces cerevisiae required for efficient expression of various yeast structural genes is part of a high-molecular-weight protein comple. Mol. Microbiol. 32, 741–751. doi: 10.1046/j.1365-2958.1999.01390.x
Efroni, I., Han, S. K., Kim, H. J., Wu, M. F., Steiner, E., Birnbaum, K. D., et al. (2013). Regulation of leaf maturation by chromatin-mediated modulation of cytokinin responses. Dev. Cell 24, 438–445. doi: 10.1016/j.devcel.2013.01.019
Eisen, J. A., Sweder, K. S., and Hanawalt, P. C. (1995). Evolution of the SNF2 family of proteins: subfamilies with distinct sequences and functions. Nucleic Acids Res. 23, 2715–2723. doi: 10.1093/nar/23.14.2715
Farrona, S., Coupland, G., and Turck, F. (2008). The impact of chromatin regulation on the floral transition. Semin. Cell Dev. Biol. 19, 560–573. doi: 10.1016/j.semcdb.2008.07.015
Farrona, S., Hurtado, L., Bowman, J. L., and Reyes, J. C. (2004). The Arabidopsis thaliana SNF2 homolog AtBRM controls shoot development and flowering. Development 131, 4965–4975. doi: 10.1242/dev.01363
Feng, S., Jacobsen, S. E., and Reik, W. (2010). Epigenetic reprogramming in plant and animal development. Science 330, 622–627. doi: 10.1126/science.1190614
Flaus, A., Martin, D. M., Barton, G. J., and Owen-Hughes, T. (2006). Identification of multiple distinct Snf2 subfamilies with conserved structural motifs. Nucleic Acids Res. 34, 2887–2905. doi: 10.1093/nar/gkl295
Gomez-Zambrano, A., Crevillen, P., Franco-Zorrilla, J. M., Lopez, J. A., Moreno-Romero, J., Roszak, P., et al. (2018). Arabidopsis SWC4 binds DNA and recruits the SWR1 complex to modulate histone H2A.Z deposition at key regulatory genes. Mol. Plant 11, 815–832. doi: 10.1016/j.molp.2018.03.014
Groth, A., Rocha, W., Verreault, A., and Almouzni, G. (2007). Chromatin challenges during DNA replication and repair. Cell 128, 721–733. doi: 10.1016/j.cell.2007.01.030
Han, S. K., Sang, Y., Rodrigues, A., Biol, F., Wu, M. F., Rodriguez, P. L., et al. (2012). The SWI2/SNF2 chromatin remodeling ATPase BRAHMA represses abscisic acid responses in the absence of the stress stimulus in Arabidopsis. Plant Cell 24, 4892–4906. doi: 10.1105/tpc.112.105114
Han, S. K., Wu, M. F., Cui, S., and Wagner, D. (2015). Roles and activities of chromatin remodeling ATPases in plants. Plant J. 83, 62–77. doi: 10.1111/tpj.12877
Hartmann, U., Höhmann, S., Nettesheim, K., Wisman, E., Saedler, H., and Huijser, P. (2000). Molecular cloning of SVP: a negative regulator of the floral transition in Arabidopsis. Plant J. 21, 351–360. doi: 10.1046/j.1365-313x.2000.00682.x
He, Y. (2012). Chromatin regulation of flowering. Trends Plant Sci. 17, 556–562. doi: 10.1016/j.tplants.2012.05.001
Henderson, J. T., Li, H. C., Rider, S. D., Mordhorst, A. P., Romero-Severson, J., Cheng, J. C., et al. (2004). Pickle acts throughout the plant to repress expression of embryonic traits and may play a role in gibberellin-dependent responses. Plant Physiol. 134, 995–1005. doi: 10.1104/pp.103.030148
Henikoff, S., and Shilatifard, A. (2011). Histone modification: cause or cog? Trends Genet. 27, 389–396. doi: 10.1016/j.tig.2011.06.006
Ho, L., Jothi, R., Ronan, J. L., Cui, K., Zhao, K., and Crabtree, G. R. (2009). An embryonic stem cell chromatin remodeling complex, esBAF, is an essential component of the core pluripotency transcriptional network. Proc. Natl. Acad. Sci. U.S.A. 106, 5187–5191. doi: 10.1073/pnas.0812888106
Horiguchi, G., Kim, G. T., and Tsukaya, H. (2005). The transcription factor AtGRF5 and the transcription coactivator AN3 regulate cell proliferation in leaf primordia of Arabidopsis thaliana. Plant J. 43, 68–78. doi: 10.1111/j.1365-313X.2005.02429.x
Hurtado, L., Farrona, S., and Reyes, J. C. (2006). The putative SWI/SNF complex subunit BRAHMA activates flower homeotic genes in Arabidopsis thaliana. Plant Mol. Biol. 62, 291–304. doi: 10.1007/s11103-006-9021-2
Ikeuchi, M., Iwase, A., and Sugimoto, K. (2015). Control of plant cell differentiation by histone modification and DNA methylation. Curr. Opin. Plant Biol. 28, 60–67. doi: 10.1016/j.pbi.2015.09.004
Jarillo, J. A., Pineiro, M., Cubas, P., and Martinez-Zapater, J. M. (2009). Chromatin remodeling in plant development. Int. J. Dev. Biol. 53, 1581–1596. doi: 10.1387/ijdb.072460jj
Jegu, T., Domenichini, S., Blein, T., Ariel, F., Christ, A., Kim, S. K., et al. (2015). A SWI/SNF chromatin remodelling protein controls cytokinin production through the regulation of chromatin architecture. PLoS One 10:e0138276. doi: 10.1371/journal.pone.0138276
Jegu, T., Latrasse, D., Delarue, M., Hirt, H., Domenichini, S., Ariel, F., et al. (2014). The BAF60 subunit of the SWI/SNF chromatin-remodeling complex directly controls the formation of a gene loop at flowering locus C in Arabidopsis. Plant Cell 26, 538–551. doi: 10.1105/tpc.113.114454
Jerzmanowski, A. (2007). SWI/SNF chromatin remodeling and linker histones in plants. Biochim. Biophys. Acta 1769, 330–345. doi: 10.1016/j.bbaexp.2006.12.003
Kanei, M., Horiguchi, G., and Tsukaya, H. (2012). Stable establishment of cotyledon identity during embryogenesis in Arabidopsis by ANGUSTIFOLIA3 and HANABA TARANU. Development 139, 2436–2446. doi: 10.1242/dev.081547
Kaufmann, K., Pajoro, A., and Angenent, G. C. (2010). Regulation of transcription in plants: mechanisms controlling developmental switches. Nat. Rev. Genet. 11, 830–842. doi: 10.1038/nrg2885
Kim, D. H., Doyle, M. R., Sung, S., and Amasino, R. M. (2009). Vernalization: winter and the timing of flowering in plants. Annu. Rev. Cell Dev. Biol. 25, 277–299. doi: 10.1146/annurev.cellbio.042308.113411
Kim, J. H., and Kende, H. (2004). A transcriptional coactivator, AtGIF1, is involved in regulating leaf growth and morphology in Arabidopsis. Proc. Natl. Acad. Sci. U.S.A. 101, 13374–13379. doi: 10.1073/pnas.0405450101
Knizewski, L., Ginalski, K., and Jerzmanowski, A. (2008). Snf2 proteins in plants: gene silencing and beyond. Trends Plant Sci. 13, 557–565. doi: 10.1016/j.tplants.2008.08.004
Kornet, N., and Scheres, B. (2009). Members of the GCN5 histone acetyltransferase complex regulate PLETHORA-mediated root stem cell niche maintenance and transit amplifying cell proliferation in Arabidopsis. Plant Cell 21, 1070–1079. doi: 10.1105/tpc.108.065300
Krogan, N. J., Keogh, M. C., Datta, N., Sawa, C., Ryan, O. W., Ding, H., et al. (2003). A Snf2 Family ATPase complex required for recruitment of the histone H2A variant Htz1. Mol. Cell 12, 1565–1576. doi: 10.1016/S1097-2765(03)00497-0
Kuderova, A., Urbankova, I., Valkova, M., Malbeck, J., Brzobohaty, B., Nemethova, D., et al. (2008). Effects of conditional IPT-dependent cytokinin overproduction on root architecture of Arabidopsis seedlings. Plant Cell Physiol. 49, 1001–1001. doi: 10.1093/pcp/pcn073
Kumar, S. V., and Wigge, P. A. (2010). H2A.Z-containing nucleosomes mediate the thermosensory response in Arabidopsis. Cell 140, 136–147. doi: 10.1016/j.cell.2009.11.006
Kwon, C. S., Chen, C., and Wagner, D. (2005). WUSCHEL is a primary target for transcriptional regulation by SPLAYED in dynamic control of stem cell fate in Arabidopsis. Genome Res. 12, 47–56. doi: 10.1101/gad.1276305
Kwon, C. S., Hibara, K., Pfluger, J., Bezhani, S., Metha, H., Aida, M., et al. (2006). A role for chromatin remodeling in regulation of CUC gene expression in the Arabidopsis cotyledon boundary. Development 133, 3223–3230. doi: 10.1242/dev.02508
Kwon, C. S., and Wagner, D. (2007). Unwinding chromatin for development and growth: a few genes at a time. Trends Genet. 23, 403–412. doi: 10.1016/j.tig.2007.05.010
Laux, T. (2003). The stem cell concept in plants: a matter of debate. Cell 113, 281–283. doi: 10.1016/S0092-8674(03)00312-X
Lee, B. H., Ko, J. H., Lee, S., Lee, Y., Pak, J. H., and Kim, J. H. (2009). The Arabidopsis GRF-Interacting Factor gene family performs an overlapping function in determining organ size as well as multiple developmental properties. Plant Physiol. 151, 655–668. doi: 10.1104/pp.109.141838
Lee, J. H., Yoo, S. J., Park, S. H., Hwang, I., Lee, J. S., and Ahn, J. H. (2007). Role of SVP in the control of flowering time by ambient temperature in Arabidopsis. Genes Dev. 21, 397–402. doi: 10.1101/gad.1518407
Lessard, J., Wu, J. I., Ranish, J. A., Wan, M., Winslow, M. M., Staahl, B. T., et al. (2007). An essential switch in subunit composition of a chromatin remodeling complex during neural development. Neuron 55, 201–215. doi: 10.1016/j.neuron.2007.06.019
Li, C., Chen, C., Gao, L., Yang, S., Nguyen, V., Shi, X., et al. (2015). The Arabidopsis SWI2/SNF2 chromatin Remodeler BRAHMA regulates polycomb function during vegetative development and directly activates the flowering repressor gene SVP. PLoS Genet. 11:e1004944. doi: 10.1371/journal.pgen.1004944
Li, C., Gu, L., Gao, L., Chen, C., Wei, C. Q., Qiu, Q., et al. (2016). Concerted genomic targeting of H3K27 demethylase REF6 and chromatin-remodeling ATPase BRM in Arabidopsis. Nat. Genet. 48, 687–693. doi: 10.1038/ng.3555
Li, D., Liu, C., Shen, L., Wu, Y., Chen, H., Robertson, M., et al. (2008). A repressor complex governs the integration of flowering signals in Arabidopsis. Dev. Cell 15, 110–120. doi: 10.1016/j.devcel.2008.05.002
Liu, X., Kim, Y. J., Müller, R., Yumul, R. E., Liu, C., Pan, Y., et al. (2011). AGAMOUS terminates floral stem cell maintenance in Arabidopsis by directly repressing WUSCHEL through recruitment of polycomb group proteins. Plant Cell 23, 3654–3670. doi: 10.1105/tpc.111.091538
Luger, K., Rechsteiner, T. J., Flaus, A. J., Waye, M. M. Y., and Richmond, T. J. (1997). Characterization of nucleosome core particles containing histone proteins made in bacteria. J. Mol. Biol. 272, 301–311. doi: 10.1006/jmbi.1997.1235
March-Diaz, R., Garcia-Dominguez, M., Florencio, F. J., and Reyes, J. C. (2007). SEF, a new protein required for flowering repression in Arabidopsis, interacts with PIE1 and ARP6. Plant Physiol. 143, 893–901. doi: 10.1104/pp.106.092270
Marfella, G. A. C., and Imbalzano, N. A. (2007). The chd family of chromatin remodelers. Mutat. Res. 618, 30–40. doi: 10.1016/j.mrfmmm.2006.07.012
Martin-Trillo, M., Lazaro, A., Poethig, R. S., Gomez-Mena, C., Pineiro, M. A., Martinez-Zapater, J. M., et al. (2006). Early in Short Days 1 (ESD1) encodes Actin-Related Protein 6 (AtARP6), a putative component of chromatin remodelling complexes that positively regulates FLC accumulation in Arabidopsis. Development 133, 1241–1252. doi: 10.1242/dev.02301
Meagher, R. B., Deal, R. B., Kandasamy, M. K., and McKinney, E. C. (2005). Nuclear actin-related proteins as epigenetic regulators of development. Plant Physiol. 139, 1576–1585. doi: 10.1104/pp.105.072447
Michaels, S. D., and Amasino, R. M. (2001). Loss of flowering locus C Activity eliminates the late-flowering phenotype of FRIGIDA and autonomous pathway mutations but not responsiveness to vernalization. Plant Cell 13, 935–941. doi: 10.1105/tpc.13.4.935
Mizuguchi, G., Xuetong, S., Joe, L., Wei-Hua, W., Subhojit, S., and Carl, W. (2004). ATP-driven exchange of histone H2AZ variant catalyzed by SWR1 chromatin remodeling complex. Science 303, 343–348. doi: 10.1126/science.1090701
Mlynarova, L., Nap, J. P., and Bisseling, T. (2007). The SWI/SNF chromatin-remodeling gene AtCHR12 mediates temporary growth arrest in Arabidopsis thaliana upon perceiving environmental stress. Plant J. 51, 874–885. doi: 10.1111/j.1365-313X.2007.03185.x
Morrison, A. J., and Shen, X. (2009). Chromatin remodelling beyond transcription: the INO80 and SWR1 complexes. Nat. Rev. Mol. Cell Biol. 10, 373–384. doi: 10.1038/nrm2693
Narlikar, G. J., Fan, H.-Y., and Kingston, R. E. (2002). Cooperation between complexes that regulate chromatin structure and transcription. Cell 108, 475–487. doi: 10.1016/S0092-8674(02)00654-2
Noh, Y. S., and Amasino, R. M. (2003). PIE1, an ISWI family gene, is required for FLC activation and floral repression in Arabidopsis. Plant Cell 15, 1671–1682. doi: 10.1105/tpc.012161
Ori, N., Eshed, Y., Chuck, G., Bowman, J. L., and Hake, S. (2000). Mechanisms that control knox gene expression in the Arabidopsis shoot. 127, 5523–5532.
Park, J., Oh, D. H., Dassanayake, M., Nguyen, K. T., Ogas, J., Choi, G., et al. (2017). Gibberellin signaling requires chromatin remodeler PICKLE to promote vegetative growth and phase transitions. Plant Physiol. 173, 1463–1474. doi: 10.1104/pp.16.01471
Peirats-Llobet, M., Han, S. K., Gonzalez-Guzman, M., Jeong, C. W., Rodriguez, L., Belda-Palazon, B., et al. (2016). A direct link between abscisic acid sensing and the chromatin-remodeling ATPase BRAHMA via core ABA signaling pathway components. Mol. Plant 9, 136–147. doi: 10.1016/j.molp.2015.10.003
Peterson, C. L., Dingwall, A., and Scott, M. P. (1994). Five SWI/SNF gene products are components of a large multisubunit complex required for transcriptional enhancement. Proc. Natl. Acad. Sci. U.S.A. 91, 2905–2908. doi: 10.1073/pnas.91.8.2905
Phelps-Durr, T. L., Thomas, J., Vahab, P., and Timmermans, M. C. (2005). Maize rough sheath2 and its Arabidopsis orthologue Asymmetric Leaves1 interact with HIRA, a predicted histone chaperone, to maintain knox gene silencing and determinacy during organogenesis. Plant Cell 17, 2886–2898. doi: 10.1105/tpc.105.035477
Pillitteri, L. J., Guo, X., and Dong, J. (2016). Asymmetric cell division in plants: mechanisms of symmetry breaking and cell fate determination. Cell. Mol. Life Sci. 73, 4213–4229. doi: 10.1007/s00018-016-2290-2
Qin, Y., Zhao, L., Skaggs, M. I., Andreuzza, S., Tsukamoto, T., Panoli, A., et al. (2014). Actin-related protein6 regulates female meiosis by modulating meiotic gene expression in Arabidopsis. Plant Cell 26, 1612–1628. doi: 10.1105/tpc.113.120576
Sablowski, R. (2007). Flowering and determinacy in Arabidopsis. J. Exp. Bot. 58, 899–907. doi: 10.1093/jxb/erm002
Sacharowski, S. P., Gratkowska, D. M., Sarnowska, E. A., Kondrak, P., Jancewicz, I., Porri, A., et al. (2015). SWP73 subunits of Arabidopsis SWI/SNF chromatin remodeling complexes play distinct roles in leaf and flower development. Plant Cell 27, 1889–1906. doi: 10.1105/tpc.15.00233
Saez, A., Rodrigues, A., Santiago, J., Rubio, S., and Rodriguez, P. L. (2008). HAB1-SWI3B interaction reveals a link between abscisic acid signaling and putative SWI/SNF chromatin-remodeling complexes in Arabidopsis. Plant Cell 20, 2972–2988. doi: 10.1105/tpc.107.056705
Sang, Y., Silva-Ortega, C. O., Wu, S., Yamaguchi, N., Wu, M. F., Pfluger, J., et al. (2012). Mutations in two non-canonical Arabidopsis SWI2/SNF2 chromatin remodeling ATPases cause embryogenesis and stem cell maintenance defects. Plant J. 72, 1000–1014. doi: 10.1111/tpj.12009
Sarnowska, E., Gratkowska, D. M., Sacharowski, S. P., Cwiek, P., Tohge, T., Fernie, A. R., et al. (2016). The role of SWI/SNF chromatin remodeling complexes in hormone crosstalk. Trends Plant Sci. 21, 594–608. doi: 10.1016/j.tplants.2016.01.017
Sarnowska, E. A., Rolicka, A. T., Bucior, E., Cwiek, P., Tohge, T., Fernie, A. R., et al. (2013). DELLA-interacting SWI3C core subunit of switch/sucrose nonfermenting chromatin remodeling complex modulates gibberellin responses and hormonal cross talk in Arabidopsis. Plant Physiol. 163, 305–317. doi: 10.1104/pp.113.223933
Sarnowski, T. J., Rios, G., Jasik, J., Swiezewski, S., Kaczanowski, S., Li, Y., et al. (2005). SWI3 subunits of putative SWI/SNF chromatin-remodeling complexes play distinct roles during Arabidopsis development. Plant Cell 17, 2454–2472. doi: 10.1105/tpc.105.031203
Schubert, D., Primavesi, L., Bishopp, A., Roberts, G., Doonan, J., Jenuwein, T., et al. (2006). Silencing by plant polycomb-group genes requires dispersed trimethylation of histone H3 at lysine 27. Eur. Mol. Biol. Organ. 25, 4638–4649. doi: 10.1038/sj.emboj.7601311
Shen, W. H., and Xu, L. (2009). Chromatin remodeling in stem cell maintenance in Arabidopsis thaliana. Mol. Plant 2, 600–609. doi: 10.1093/mp/ssp022
Shen, X., Mizuguchi, G., Hamiche, A., and Wu, C. (2000). A chromatin remodelling complex involved in transcription and DNA processing. Nature 406, 541–544. doi: 10.1038/35020123
Sinha, N. (1999). Leaf development in angiosperms. Annu. Rev. Plant Physiol. Plant Mol. Biol. 50, 419–446. doi: 10.1146/annurev.arplant.50.1.419
Soria, G., Polo, S. E., and Almouzni, G. (2012). Prime, repair, restore: the active role of chromatin in the DNA damage response. Mol. Cell 46, 722–734. doi: 10.1016/j.molcel.2012.06.002
Suzuki, M. M., and Bird, A. (2008). DNA methylation landscapes: provocative insights from epigenomics. Nat. Rev. Genet. 9, 465–476. doi: 10.1038/nrg2341
Tang, X., Hou, A., Babu, M., Nguyen, V., Hurtado, L., Lu, Q., et al. (2008). The Arabidopsis BRAHMA chromatin-remodeling ATPase is involved in repression of seed maturation genes in leaves. Plant Physiol. 147, 1143–1157. doi: 10.1104/pp.108.121996
Taylor-Teeples, M., Lin, L., de Lucas, M., Turco, G., Toal, T. W., Gaudinier, A., et al. (2015). An Arabidopsis gene regulatory network for secondary cell wall synthesis. Nature 517, 571–575. doi: 10.1038/nature14099
Vercruyssen, L., Verkest, A., Gonzalez, N., Heyndrickx, K. S., Eeckhout, D., Han, S. K., et al. (2014). Angustifolia3 binds to SWI/SNF chromatin remodeling complexes to regulate transcription during Arabidopsis leaf development. Plant Cell 26, 210–229. doi: 10.1105/tpc.113.115907
Walley, J. W., Rowe, H. C., Xiao, Y., Chehab, E. W., Kliebenstein, D. J., Wagner, D., et al. (2008). The chromatin remodeler SPLAYED regulates specific stress signaling pathways. PLoS Pathog. 4:e1000237. doi: 10.1371/journal.ppat.1000237
Wigge, P. A., Kim, M. C., Jaeger, K. E., Busch, W., Schmid, M., Lohmann, J. U., et al. (2005). Integration of spatial and temporal information during floral induction in Arabidopsis. Science 309, 1056–1059. doi: 10.1126/science.1114358
Wils, C. R., and Kaufmann, K. (2017). Gene-regulatory networks controlling inflorescence and flower development in Arabidopsis thaliana. Biochim. Biophys. Acta 1860, 95–105. doi: 10.1016/j.bbagrm.2016.07.014
Wu, M. F., Sang, Y., Bezhani, S., Yamaguchi, N., Han, S. K., Li, Z., et al. (2012). SWI2/SNF2 chromatin remodeling ATPases overcome polycomb repression and control floral organ identity with the Leafy and Sepallata3 transcription factors. PNAS 109, 3576–3581. doi: 10.1073/pnas.1113409109
Wu, M.-F., Yamaguchi, N., Xiao, J., Bargmann, B., Estelle, M., Sang, Y., et al. (2015). Auxin-regulated chromatin switch directs acquisition of flower primordium founder fate. eLife 4:e09269. doi: 10.7554/eLife.09269
Wu, W. H., Alami, S., Luk, E., Wu, C. H., Sen, S., Mizuguchi, G., et al. (2005). Swc2 is a widely conserved H2AZ-binding module essential for ATP-dependent histone exchange. Nat. Struct. Mol. Biol. 12, 1064–1071. doi: 10.1038/nsmb1023
Xu, L., and Shen, W. H. (2008). Polycomb silencing of KNOX genes confines shoot stem cell niches in Arabidopsis. Curr. Biol. 18, 1966–1971. doi: 10.1016/j.cub.2008.11.019
Xu, Y., Guo, C., Zhou, B., Li, C., Wang, H., Zheng, B., et al. (2016). Regulation of vegetative phase change by SWI2/SNF2 chromatin remodeling ATPase BRAHMA. Plant Physiol. 172, 2416–2428. doi: 10.1104/pp.16.01588
Yang, S., Li, C., Zhao, L., Gao, S., Lu, J., Zhao, M., et al. (2015). The Arabidopsis SWI2/SNF2 chromatin remodeling ATPase BRAHMA targets directly to PINs and is required for root stem cell niche maintenance. Plant Cell 27, 1670–1680. doi: 10.1105/tpc.15.00091
Yu, X., Jiang, L., Wu, R., Meng, X., Zhang, A., Li, N., et al. (2016). The core subunit of a chromatin-remodeling complex, ZmCHB101, plays essential roles in maize growth and development. Sci. Rep. 6:38504. doi: 10.1038/srep38504
Zhang, C., Cao, L., Rong, L., An, Z., Zhou, W., Ma, J., et al. (2015). The chromatin-remodeling factor AtINO80 plays crucial roles in genome stability maintenance and in plant development. Plant J. 82, 655–668. doi: 10.1111/tpj.12840
Zhang, D., Li, Y., Zhang, X., Zha, P., and Lin, R. (2017). The SWI2/SNF2 chromatin-remodeling ATPase BRAHMA regulates chlorophyll biosynthesis in Arabidopsis. Mol. Plant 10, 155–167. doi: 10.1016/j.molp.2016.11.003
Zhang, H., Bishop, B., Ringenberg, W., Muir, W. M., and Ogas, J. (2012). The CHD3 remodeler pickle associates with genes enriched for trimethylation of histone H3 lysine 27. Plant Physiol. 159, 418–432. doi: 10.1104/pp.112.194878
Zhang, H., Rider, S. D. Jr., Henderson, J. T., Fountain, M., Chuang, K., et al. (2008). The CHD3 remodeler PICKLE promotes trimethylation of histone H3 lysine 27. J. Biol. Chem. 283, 22637–22648. doi: 10.1074/jbc.M802129200
Zhao, L., Cai, H., Su, Z., Wang, L., Huang, X., Zhang, M., et al. (2018). KLU suppresses megasporocyte cell fate through SWR1-mediated activation of WRKY28 expression in Arabidopsis. Proc. Natl. Acad. Sci. U.S.A. 115, E526–E535. doi: 10.1073/pnas.1716054115
Zhao, M., Yang, S., Chen, C. Y., Li, C., Shan, W., Lu, W., et al. (2015). Arabidopsis brevipedicellus interacts with the SWI2/SNF2 chromatin remodeling ATPase BRAHMA to regulate KNAT2 and KNAT6 expression in control of inflorescence architecture. PLoS Genet. 11:e1005125. doi: 10.1371/journal.pgen.1005125
Zhu, J., Adli, M., Zou, J. Y., Verstappen, G., Coyne, M., Zhang, X., et al. (2013). Genome-wide chromatin state transitions associated with developmental and environmental cues. Cell 152, 642–654. doi: 10.1016/j.cell.2012.12.033
Keywords: chromatin remodeling, SWI/SNF complexes, histones, gene regulation, meristem, hormone signaling, plant development, flowering
Citation: Ojolo SP, Cao S, Priyadarshani SVGN, Li W, Yan M, Aslam M, Zhao H and Qin Y (2018) Regulation of Plant Growth and Development: A Review From a Chromatin Remodeling Perspective. Front. Plant Sci. 9:1232. doi: 10.3389/fpls.2018.01232
Received: 11 March 2018; Accepted: 03 August 2018;
Published: 22 August 2018.
Edited by:
Ming Luo, South China Botanical Garden (CAS), ChinaReviewed by:
Chenlong Li, Agriculture and Agri-Food Canada (AAFC), CanadaCopyright © 2018 Ojolo, Cao, Priyadarshani, Li, Yan, Aslam, Zhao and Qin. This is an open-access article distributed under the terms of the Creative Commons Attribution License (CC BY). The use, distribution or reproduction in other forums is permitted, provided the original author(s) and the copyright owner(s) are credited and that the original publication in this journal is cited, in accordance with accepted academic practice. No use, distribution or reproduction is permitted which does not comply with these terms.
*Correspondence: Yuan Qin, eXVhbnFpbkBmYWZ1LmVkdS5jbg==; eXVhbnFpbjAwMUBmb3htYWlsLmNvbQ==
†These authors have contributed equally to this work
Disclaimer: All claims expressed in this article are solely those of the authors and do not necessarily represent those of their affiliated organizations, or those of the publisher, the editors and the reviewers. Any product that may be evaluated in this article or claim that may be made by its manufacturer is not guaranteed or endorsed by the publisher.
Research integrity at Frontiers
Learn more about the work of our research integrity team to safeguard the quality of each article we publish.