- College of Resources and Environment, Henan Agricultural University, Zhengzhou, China
Selenium (Se) is a necessary trace element for humans and animals, and Se fertilization is an efficient way to increase Se concentration in the edible parts of crops, thus enhance the beneficiary effects of Se in human and animal health. Due to the similarity of physical and chemical properties between phosphate () and selenite (
), phosphorus (P) supply often significantly impacts the absorption of Se in plants, but little is known about how P supply influences the subcellular distribution and chemical forms of Se. In this study, the effects of P supply on subcellular distribution and chemical forms of Se in winter wheat were investigated in a hydroponic trial with medium Se level (0.1 mg Se L-1). P was applied with three concentrations (0.31, 3.1, and 31 mg P L-1) in the experiment. The results showed that increasing P supply significantly decreased the concentration and accumulation of Se in the roots, stems, and leaves of winter wheat. An increase in P supply significantly inhibited Se accumulation in the root cell wall, but enhanced Se distribution in the organelles and soluble fraction of root cells. These findings suggest that increased P supply inhibited the root-to-shoot transport of Se. An increase in P supply enhanced Se accumulation in the cell wall of plant stems (both apical and axillary stem) and cell organelles of plants leaves, but inhibited Se distribution in the soluble fraction of stems and leaves. This suggests that P supply enhances Se transportation across the cell membrane in shoots of winter wheat. In addition, increased P supply also altered the chemical forms of Se in tissues of winter wheat. These findings will help in understanding of the regulation grain Se accumulation and provide a practical way to enhance Se intake for humans inform Se-enriched grains.
Introduction
Selenium (Se) is a necessary trace element for human, and the critical components of glutathione peroxidase (GSH-Px) and prosthetic group of many enzymes (Awasthi et al., 1975; Rotruck et al., 1980). Se plays important roles in antioxidation, detoxification, and high immunity for human beings. Many studies have shown that a low intake of Se may increase the risk of Keshan disease, Kashin–Beck disease, muscle syndrome, liver disease, and many cancers (Liu et al., 2004; Arinola and Charles-Davies, 2008). To increase the intake of Se for human beings, Se supplements in soil or through foliage spray thus producing Se-enriched food from corps have been a popular subject in recent research (He et al., 1998). Although Se is not an essential element for plants, low concentrations of Se have beneficial effects on plant growth and yield. Se was found to elevate antioxidant activity, stimulate starch accumulation in chloroplasts, and ameliorate the toxic effects of several HMs in plants (Pennanen et al., 2002; Turakainen et al., 2004; Malik et al., 2012). However, higher concentrations of Se supply result in toxicity in plants, and there is a narrow boundary between beneficial and toxic concentrations for Se (Kaur et al., 2014). Therefore, a better understanding of the basic mechanism about Se absorption and transport in plants is important to enhance Se accumulation and Se intake in the human diet while not affecting plant growth.
Selenate () is the main form of Se in alkaline soils, whereas Se exists predominantly as selenite (
) in acid and neutral soils (Elrashidi et al., 1987). Selenite becomes the dominant form in strongly reduced soil (pE + pH < 7.5) (Elrashidi et al., 1987). It has been reported that selenate is similar to sulfate in chemical characteristics, and thus is taken up by plants through sulfate transporters (Anderson, 1993). Furthermore, plants take up Se and phosphorus (P) in the form of anions. Although Se and P are not in the same periodic group, uptake of selenite (
) is promoted by P deficiency in wheat, which indicates that selenite (
) uptake is an active process likely mediated by phosphate transporters (Li H.F. et al., 2008). Earlier studies also pointed out that P could markedly reduce the uptake of Se in plants (Lévesque, 1974; Singh and Singh, 1978). However, conflict results about the effect of P application on the uptake of Se have been reported in plants. Cater et al. (1972) observed that adding P to the soil increased the Se concentrations in alfalfa grown on 6 of 14 soils, and P application increased the Se absorption of berseem when Se supplied from 0 to 16 mg L-1 (Singh and Malhotra, 1976). As yet, there have been no reports that describe the chemical pathways in which P affects Se subcellular distribution and chemical form in plants.
Many researchers have studied the subcellular distribution and chemical form of elements in plants (Li et al., 2006; Pan et al., 2016). However, most of these studies were focused on heavy metals, especially Cd and Zn, because the chemical forms and subcellular distribution of heavy metals are closely related to the detoxification mechanism in plants (Li et al., 2006). Vacuole is the most important compartment contributing to the cell soluble fraction (Ramos et al., 2002; Zhang et al., 2013). Deposition in the cell wall and compartmentation to the vacuole are two important mechanisms for detoxification, tolerance, and hyperaccumulation of some heavy metals and trace elements in plants (Zhang et al., 2014). Du et al. (2010) determined Se subcellular distribution in spring tea leaves according to the method used for heavy metals. In view of the important significance of Se biofortification for humans, some studies have determined the Se chemical forms and speciation in plants (Pickering et al., 2003; Ogra et al., 2004; Zhao et al., 2004; Hart et al., 2011), but these studies focused on the Se metabolism compounds (e.g., selenomethionine and selenocystine). Determination of Se chemical forms in plants using the method of sequential extraction is rare, although this method was used to determine the chemical forms of heavy metals in plants. For example, Liu Y. et al. (2010) analyzed the alcohol-soluble, water-soluble, and salt-soluble Se concentrations in spring tea leaves. The effects of Se on growth and physiological metabolism of plants were contradictorily reported because the results were highly dependent on the concentrations and chemical forms of Se used in the assays (Kassis et al., 2007).
Wheat is the main dietary component and the most important source of both calories and protein in many countries (Cakmak, 2008). In China, the Northern Winter Wheat Region contributes about 70% of the national wheat production (Zhuang, 2003). Applying Se-rich fertilizers to improve grain Se content in winter wheat is an effective strategy to increase Se intake for humans in the winter wheat-planting region (Lyons et al., 2003; Li H.F. et al., 2008). It has been reported that excessive Se dietary intake is harmful for humans and animals, which can result in some selenosis symptoms including dermatitis, cracking of nails, hair loss and respiratory distress, and so on (Rayman, 2012). Above 400 μg Se per day for adults can increase the risk of developing Se toxicity (Institute of Medicine, 2000); therefore, control of grain Se concentration in winter wheat is very important for Se nutrition in humans in the north of China. P fertilization is a common agricultural practice to improve grain yield and quality of winter wheat in the north of China. However, excessive P fertilization in intensive agricultural areas results in severe environmental problems such as eutrophication and heavy metal pollution and has recently received great attention in China (Atafar et al., 2010; Le et al., 2010; Li et al., 2014). Policies related to regulation of P application have been highlighted by the Department of Agriculture of China, which helps to meet the key target of balancing crop production and environmental protection (Zhang et al., 2016). Therefore, the influence of P fertilization on Se absorption and translocation in winter wheat is an important issue to be addressed.
The results are expected to enhance our understanding in the interaction of P and Se in plants and provide practical measures for fertilizers containing P and Se that will maintain appropriate concentration of Se in the grain of winter wheat for human health. The objectives of this study were to: (1) validate the effect of P supply on the Se absorption in winter wheat; (2) explore the cellular mechanism of the effect of P on Se uptake and transport in winter wheat; and (3) investigate the transformation of chemical forms of Se in the different tissues of winter wheat in response to different levels of P supply.
Materials and Methods
Greenhouse Conditions
According to the method of Nie et al. (2017), a hydroponic experiment was conducted in a greenhouse with controlled environmental condition at about 14/10 h in light/dark regime, 22/18°C in air temperatures, approximate 500 μmol m-2 s-1 in photon flux density, and 65% in relative humidity.
Solution Culture
Winter wheat (Triticum aestivum cv. Wenmai 8) seeds, obtained from Henan Qiule Seeds Company (Zhengzhou, China), were germinated in deionized water at 25°C for 5 d, after sterilized in 0.5% NaClO solution for 15 min. Then, 20 seedlings were transferred to 4 L plastic containers with Hoagland–Arnon nutrition solutions (Arnon and Hoagland, 1940), which was consisted of 945 mg L-1 Ca(NO3)2⋅4H2O, 607 mg L-1 KNO3, 493 mg L-1 MgSO4⋅7H2O, 20 mg L-1 EDTA-Fe, 2.86 mg L-1 H3BO3, 1.81 mg L-1 MnCl2⋅4H2O, 0.22 mg L-1 ZnSO4⋅7H2O, 0.08 mg L-1 CuSO4⋅5H2O, and 0.02 mg L-1 (NH4)6Mo7O24⋅4H2O. The final pH of the solution was adjusted to 6.0. P was added to the solutions as NaH2PO4 at three rates: 0.31, 3.1, and 31 mg P L-1 and Se was added as Na2SeO3 at 0.1 mg Se L-1 (Liu et al., 2004; Liu H. et al., 2010). Three times was replicated in each treatment. Seedlings were supplied full-strength nutrient solutions until sampled, except for the quarter and half strength solutions supplied at the first and second weeks, respectively. The solutions were replaced every 3 days to ensure enough nutrients. Solution of 5% HCl was used to dip all vessels for 1 week, and then deionized water was used to wash those vessels more than three times. After cultivation for 21 d, the roots, stems, and leaves of 14 seedlings were separately sampled, dried at 70 ± 5°C, and analyzed for dry weights and concentrations of P and Se. The leaves, stems, and roots of other six seedlings were immediately frozen in liquid nitrogen, and then stored at -20°C for further subcellular fractions and chemical forms analysis. All the procedures about preparation and renewal of nutrient solution and the harvest of plants samples were conducted according to the method of Nie et al. (2017).
Subcellular Fractions Analysis
The subcellular fractions of Se were analyzed according to the method of Weigel and Jäger (1980). About 1 g of frozen samples (leaf, stem, or root) was placed into 50 mL polypropylene centrifuge tubes and was homogenized in 20 mL extraction buffer containing 50 mM Tris–HCl (pH 7.5), 250 mM sucrose, and 1.0 mM dithioerythritol. The cell wall fraction was obtained in the residue after centrifuging the homogenate at 300 × g for 30 s. The cell organelle fraction was in retained pellet after centrifuging the supernatant at 10,000 × g for 30 min. The resultant supernatant solution was regarded as soluble fraction. All steps were performed at 4°C.
Chemical Forms Analysis
The chemical forms of Se in the different plant issues were determined according to the method of Liu Y. et al. (2010). The extractive solutions were in the following order: (1) 80% ethanol, extracting monosaccharides, two sugars, and small molecules organic Se dissolved in ethanol soluble. (2) Deionized water (d-H2O), extracting water soluble protein and sugar-integrated Se, and inorganic Se. (3) 1 M NaCl, extracting salt soluble protein-integrated Se and exchangeable Se substance with salt. (4) 2% Acetic acid (HAc), extracting Se–phosphate complexes. (5) 0.6 M HCl, extracting Se oxalate. (6) Residual Se. A 0.5 g portion of frozen leaf, stem, or root samples were homogenized in the above extractive solutions with a diluted ratio at 1:100 (w/v), and then shaken at 25°C for 22 h. After that, the first supernatant solution was obtained after centrifuging the homogenate at 5000 × g for 10 min, and placed into a conical flask. The sedimentation was re-suspended by the same extractive solution for twice and repeated the centrifuge steps for each extraction solutions. The supernatant of the three suspending was then pooled.
Mineral Analysis
Selenium concentration was analyzed according to the method of Chinese Standard (GB/T 21729, 2008). The ground dry samples were digested with 5 mL of HNO3: HClO4 (4:1, v/v) until white smoke appeared. And then, 10 mL 6 mol L-1 HCl was added into the digestive system until the white smoke appeared again. The residual was then diluted with 25 mL deionized water. The cell wall, cell organelle, and soluble fractions of tissues, the extraction solutions of five chemical forms, and residual were transferred to conical flasks to dry by distillation, and digested as described above. The concentrations of Se in digested solutions were determined using Atomic Fluorescence Spectrometer (AFS8220, Beijing Jitian Instruments Company, China). The details for Se subcellular fractions and chemical forms analysis were: limits of detection 0.01 μg L-1, recovery rate 85.4–98.5%.
The P concentration was determined according to the method of Bao (2002). Ground samples were digested with 10 mL of H2SO4 and several drops of H2O2, and then diluted with deionized water. The sample solution was determined using the vanadomolybdo-phosphoric yellow colorimetric method using a FOSS FIAstar 5000 Autoanalyzer.
Calculations and Statistical Analysis
The Se accumulation was calculated with the Se concentration, multiplied by the dry weights.
The proportion (%) of subcellular fractions (or chemical form) of Se was calculated as the percentage of Se concentrations in each fraction (forms) to all fractions (forms), according to the method of Nie et al. (2017).
According to the method of Erenoglu et al. (2011), all data were statistically analyzed by one-way ANOVA with LSD multiple comparison at a 5% level (P < 0.05) using SPSS 18.0 software.
Results
Dry Weights, Se Concentrations, and Accumulation
According to the results of one-way ANOVA, P application exerted significant effects (P < 0.05) on dry weights, Se concentrations, and accumulation in tissues of winter wheat (Supplementary Table S1). At three concentrations of P supply, P0.31, P3.1, and P31, the dry weight of roots significantly increased comparing P3.1 with P0.31, while the root dry weight decreased comparing P31 to P0.31 (P < 0.05). The dry weights of stems and leaves remarkably increased with P3.1 comparing to P0.31 (P < 0.05), but no significant differences between the treatments of P3.1 and P31 (Table 1).
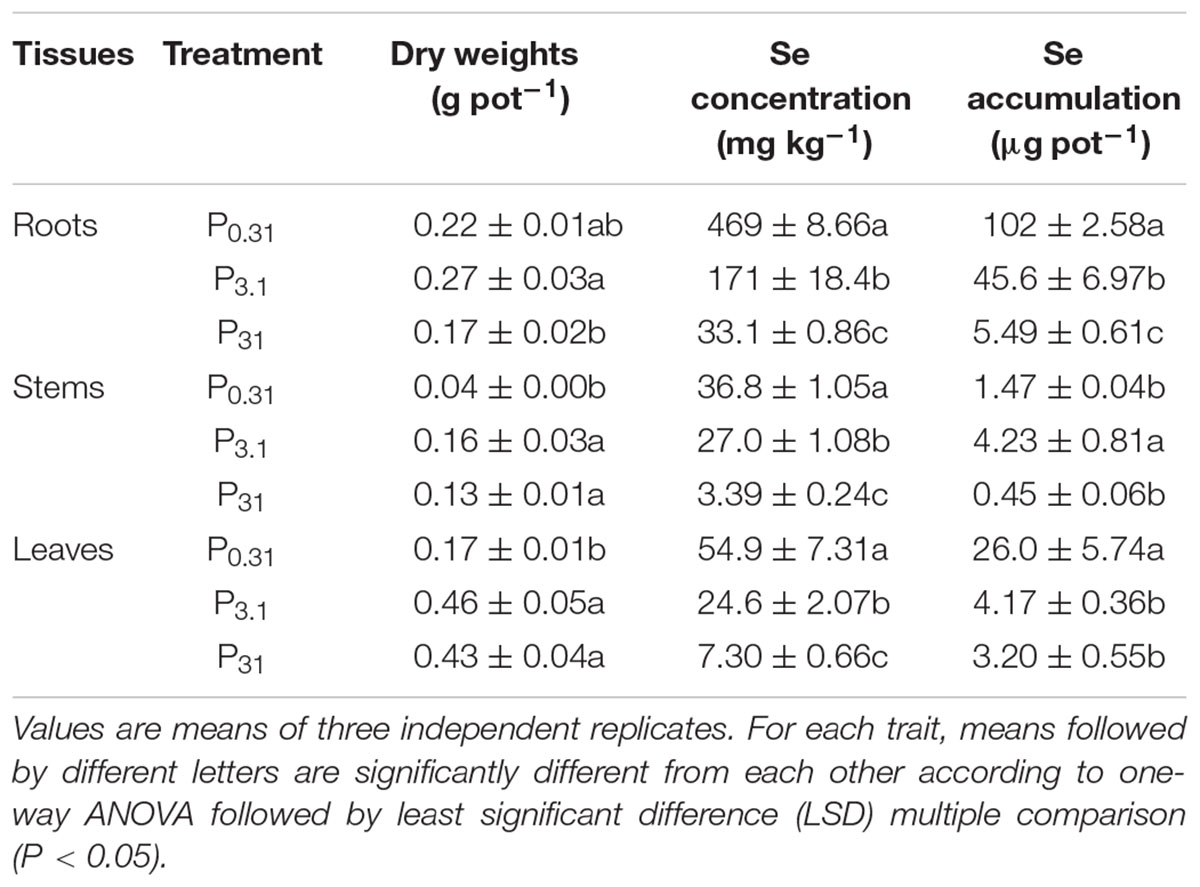
TABLE 1. Dry weights, Se concentration, and accumulation in tissues of winter wheat (Triticum aestivum cv. Wenmai 8) seedlings, pre-cultured with 0.31, 3.1, and 31 mg P L-1 in a nutrient solution for 21 d.
Selenium concentrations in roots, stems, and leaves were sharply decreased (P < 0.05) with increased P supply. Increasing P supply also significantly decreased (P < 0.05) Se accumulation in roots and leaves. Se accumulation in stems in the treatment of P3.1 was higher than that in the treatment of P0.31 and P31.
Se Subcellular Fraction and Distribution
One-way ANOVA results revealed significant effect of P application on subcellular fractions of Se in tissues of winter wheat (Supplementary Table S2; P < 0.05). According to Table 2, most of Se accumulated in the cell wall and cell organelle in stems and leaves, with less Se present in the soluble fraction. However, Se accumulation in each fraction in roots depended on the P supply levels. In roots, most of Se accumulated in the cell wall in the treatment of P0.31, while Se accumulated in the soluble fraction in the treatment of P31.
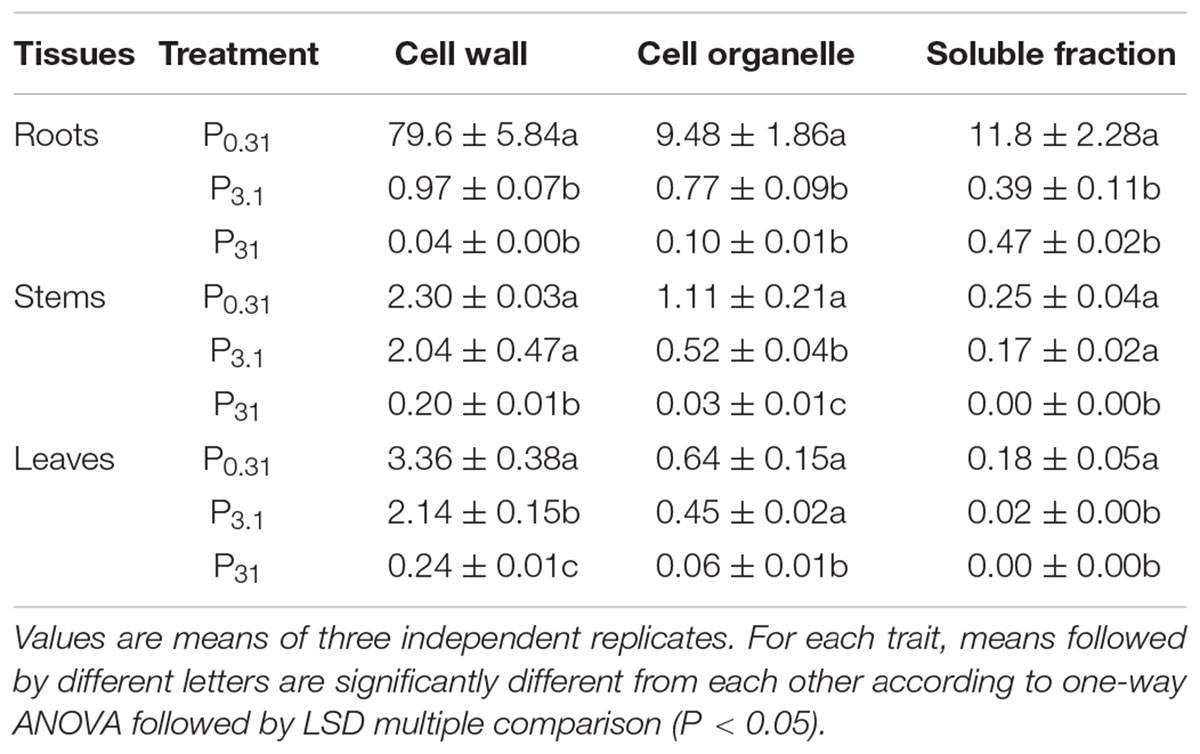
TABLE 2. Subcellular fractions of Se in tissues of winter wheat (T. aestivum cv. Wenmai 8) seedlings, pre-cultured with 0.31, 3.1, and 31 mg P L-1 in a nutrient solution for 21 d (Unit: mg kg-1 FW).
Selenium concentration in each fraction of three tissues all showed a strong decrease (P < 0.05) with increasing P supply levels, with the lowest Se concentration in the treatment of P31 (Table 2). However, there were no obvious differences of Se concentration in each fraction of roots, and in the soluble fractions of leaves between the treatment of P3.1 and P31. No pronounced differences were observed in Se concentrations in the cell wall and soluble fractions of stems, and in cell organelles of leaves between the treatment of P0.31 and P3.1.
The proportion of Se in the cell wall was higher than the cell organelles or soluble fraction, except for the major portion of soluble fractions in roots at P31 treatment (Figure 1). Se proportion in the cell wall of roots was strongly decreased by increasing P supply; however, an increase in Se in the cell organelle and soluble fraction was observed in roots (Figure 1A). On the contrary, increasing P supply levels significantly increased Se proportion in the cell wall fractions of stems, but decreased Se proportion in cell organelle and soluble fractions of stems (Figure 1B). The Se proportion in the cell organelle of leaves in the treatment of P0.31 was lower than that in the treatment of P3.1 or P31; however, Se proportion in the soluble fraction of leaves in the treatment of P0.31 was higher than that in the treatment of P3.1 or P31 (Figure 1C). The P supply levels had no effect on Se proportion in the cell wall fraction of leaves.
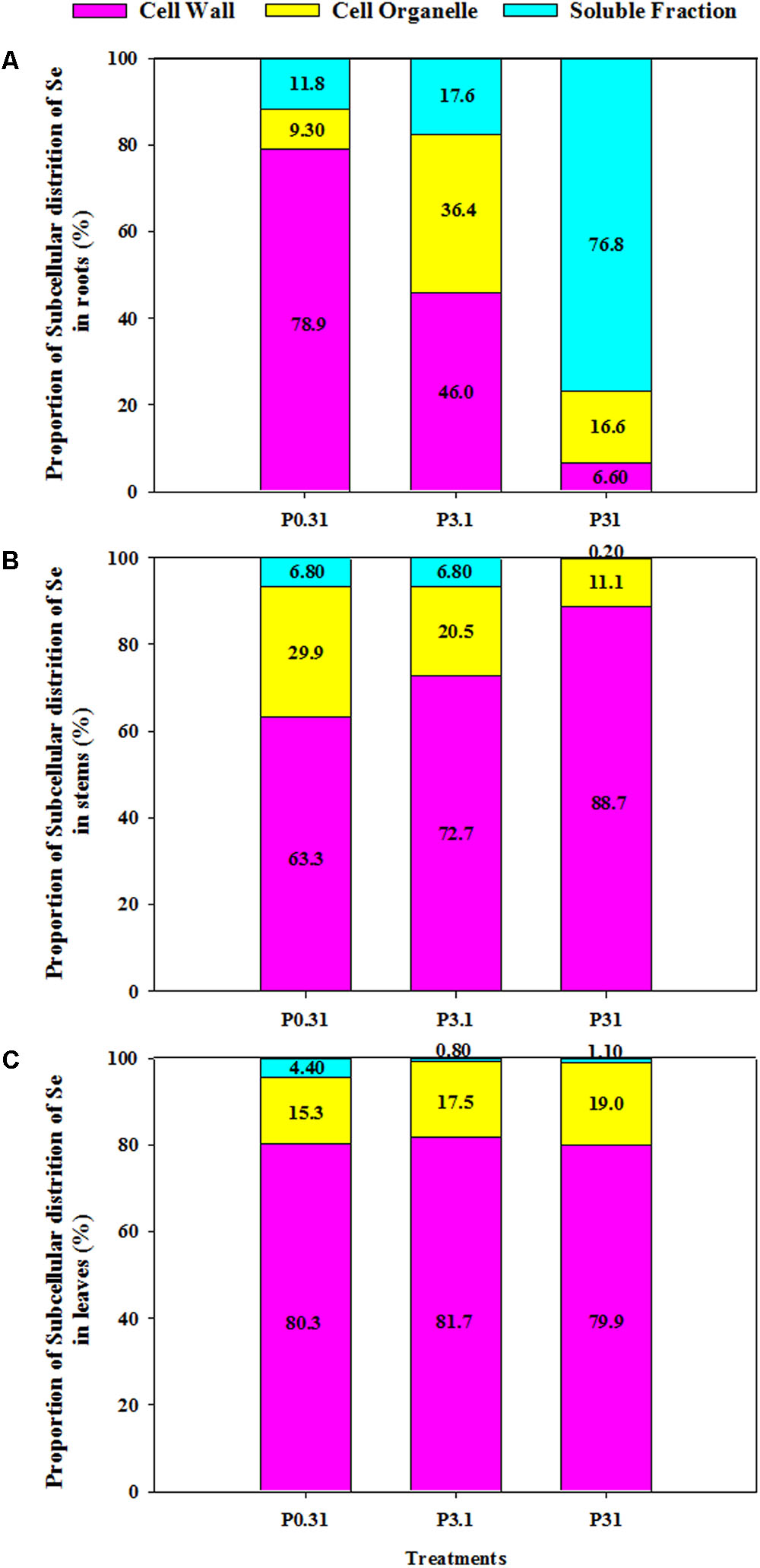
FIGURE 1. Proportion of subcellular distribution of Se in roots (A), stems (B), and leaves (C) of winter wheat (Triticum aestivum cv. Wenmai 8) seedlings, grown at 0.31, 3.1, or 31 mg P L-1 in a nutrient solution for 21 d. The proportion of subcellular distribution of Se (%) was calculated as the percentage of Se concentrations in each fraction to all fractions in each tissue. Values are means of three independent replicates.
Se Chemical Forms and Distribution
According to the results of one-way ANOVA, P application had significant effects (P < 0.05) on chemical forms of Se in tissues of winter wheat (Supplementary Table S3). Residual, 80% ethanol, and deionized water extractive forms of Se were dominant in each treatment in winter wheat (Table 3 and Figure 2).
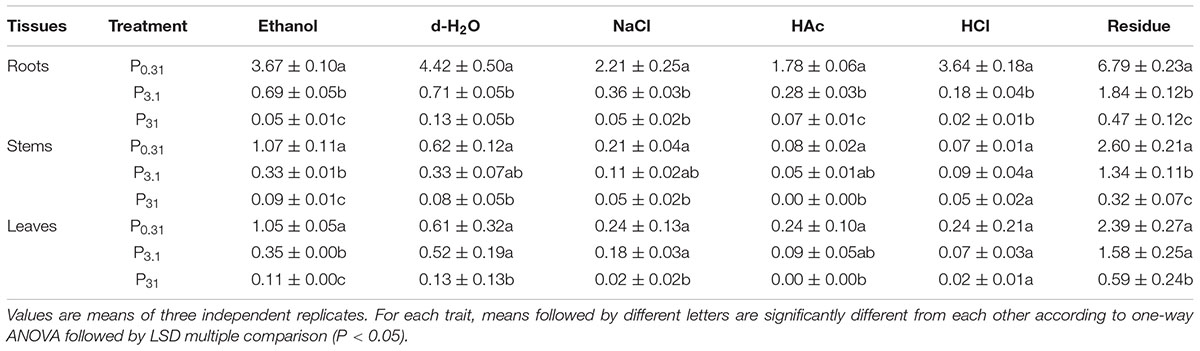
TABLE 3. Chemical forms of Se in tissues of winter wheat (T. aestivum cv. Wenmai 8) seedlings, pre-cultured with 0.31, 3.1, and 31 mg P L-1 in a nutrient solution for 21 d (Unit: mg kg-1 FW).
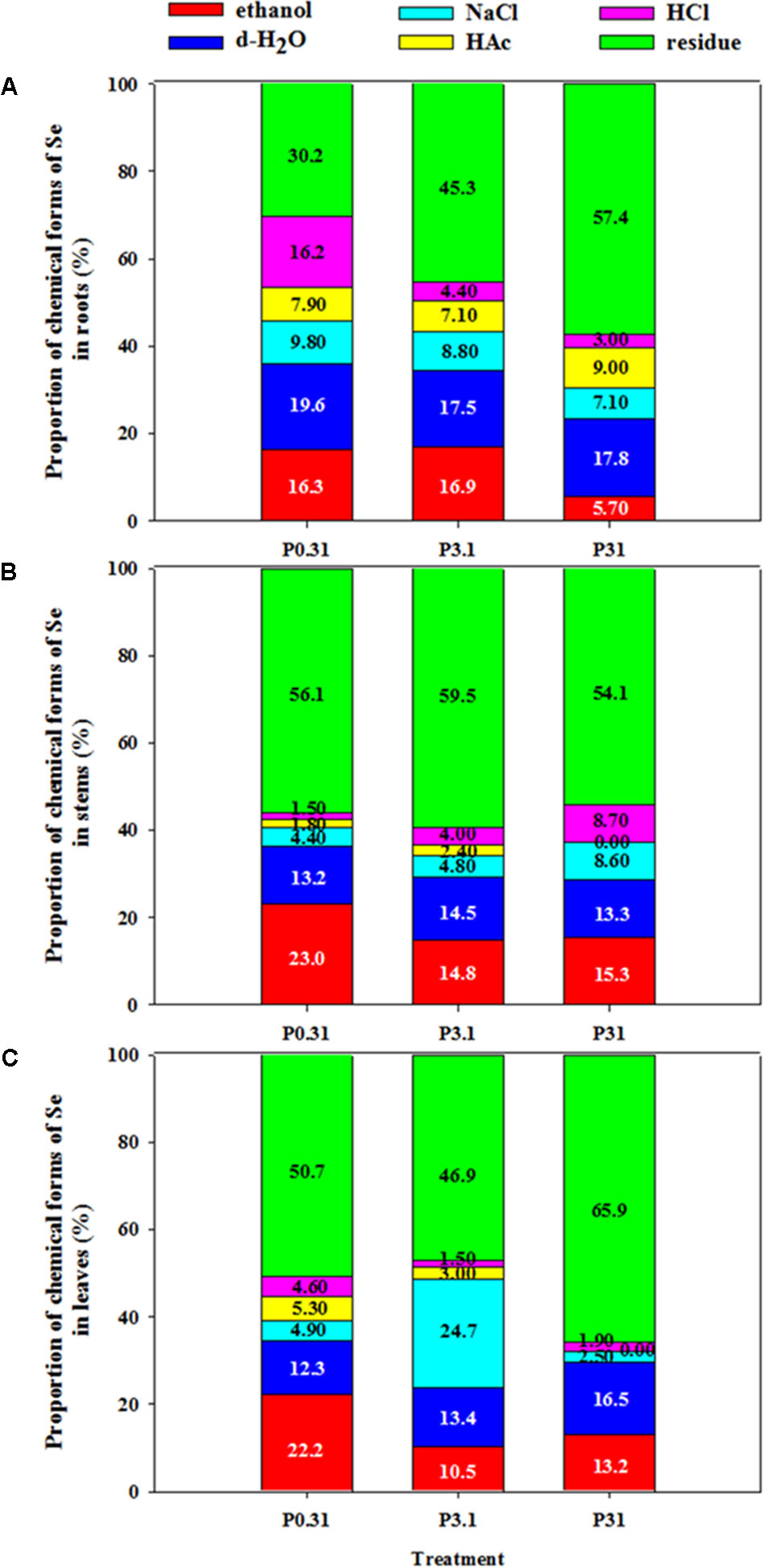
FIGURE 2. Proportion of chemical forms of Se in roots (A), stems (B), and leaves (C) of winter wheat (T. aestivum cv Wenmai 8) seedlings, grown at 0.31, 3.1, or 31 mg P L-1 in a nutrient solution for 21 d. The proportion of chemical forms of Se (%) was calculated as the percentage of Se concentrations in each form to all forms in each tissue. Values are means of three independent replicates.
Selenium forms extracted by 80% ethanol in each tissue, Se forms extracted by deionized water and 2% HAc in roots, and residual Se in roots and stems decrease (P < 0.05) significantly but gradually with increasing P supply levels, with the lowest Se in the treatment of P31 (Table 3). Meanwhile, P0.31 had higher Se forms extracted by 1 M NaCl in roots as well as 6 M HCl in roots and leaves than the treatment of P3.1 and P31. Se forms extracted by deionized water, 1 M NaCl, and 2% HAc in stems and leaves in the treatment of P0.31 were also higher than that in the treatment of P31. Compared to the treatment of P0.31, residual Se in leaves of the treatment of P31 significantly decreased (P < 0.05).
The higher proportion of residual Se than other forms was found in each tissue of winter wheat (Figure 2). In roots, increasing P supply levels significantly increased Se proportion in residue, but decreased Se proportion in 80% ethanol and 6 M HCl (Figure 2A). In stems, Se proportion in 80% ethanol showed a strong decrease, but that in 1 M NaCl and 6 M HCl showed a strong increase with an increase in P supply levels (Figure 2B). In leaves, increasing P supply also significantly decreased Se proportion in 80% ethanol, 2% HAc, and 6 M HCl, but increased Se proportion in deionized water and residue (Figure 2C). In addition, P3.1 had the higher Se proportion in residue of stems and in 1 M NaCl of leaves, but lower Se proportion in residue of leaves than the treatment of P0.31 and P31.
Discussion
Earlier reports showed that Se concentration and accumulation were dependent on the status of P nutrition in plants (Cater et al., 1972; Lévesque, 1974; Singh and Malhotra, 1976; Singh and Singh, 1978; Altansuvd et al., 2014; Zhang et al., 2017). As expected, the current study results clearly indicated that increasing P supply levels greatly decreased Se concentration and accumulation in roots, stems, and leaves of winter wheat (Table 1).
There are contradictory opinions on the effect of P application on the concentration and accumulation of Se in plants. Zhang et al. (2017) suggested that P combined with selenite application declined the Se accumulation coefficient, Se translocation coefficient, and Se concentration in tissues of wheat, but when P was applied with selenate (), the Se content, Se accumulation coefficient, and Se translocation coefficient in wheat all increased. This indicates that the effect of P application on Se concentration and accumulation depended on the type of Se fertilizer. The current study, using selenite (SeO32) as the Se fertilizer, is consistent with the results of Zhang et al. (2017). In contrast, Altansuvd et al. (2014) pointed out that the Se content of potato, wheat, and barley grown in high available P soil was higher than those in low available P soil, and total soil Se was significantly correlated with P availability in soil. During previous studies (Mora et al., 2008; Sanghun et al., 2011), it was observed that moderate P supply combined with Se fertilization could increase grain yield while P application had a negative effect on increasing grain Se concentration, thus avoiding excessive P application in agricultural practice is critical for the balance of obtaining higher grain yield and appropriate grain Se concentration in plants. It was also observed that the interaction of P and Se in plants often focused on Se absorption and translocation, but less attention has been paid to the Se subcellular distribution and chemical form transformation (Mora et al., 2008; Sanghun et al., 2011).
The subcellular distribution pattern of elements correlates to their changes during growth and their biochemical or structural roles in plants (Rathore et al., 1972). Under toxic concentrations, plants roots inhibit the translocation of these elements to shoots as a barrier (Weigel and Jäger, 1980). A general hypothesis is that the cell walls and vacuoles in root are the major sites for sequestrating elements, and distribution of elements in subcellular fractions plays an important role in plant tolerance to the toxic levels of elements (Hall, 2002; Gallego et al., 2012). In the current study, the majority of Se in roots was accumulated in the cell wall fraction (78.9 and 46.0%) at low (P0.31) and medium P (P3.1) application, whereas a minor fraction was accumulated in the cell wall (6.58%) when P supplied at 31 mg L-1. This suggests that the subcellular distribution pattern of Se in roots may be dependent on P concentrations in the medium.
As the first cellular barrier, the cell wall prevents the entrance of ions into cells through ions binding and retention, due to its negative charge (Gallego et al., 2012). The current study results also showed that an increase in P supply levels significantly decreased Se concentration and distribution in the cell wall of roots (Table 2 and Figure 1A). It indicated that P supply resulted in cell wall bind less Se ions and enhanced Se transportation across the cell membrane (Weigel and Jäger, 1980). Enhanced accumulation of heavy metal ions (such as Cd2+) in shoot was contributed by heavy metal ions distributing in the cell wall (Su et al., 2014), thus inhibited Se distribution in cell wall by an increase in P supply might be one of the reasons for decreased Se accumulation in tissues of winter wheat by an increase in P supply (Table 1). On the other hand, most of heavy metal ions enter into the vacuoles of root cells to be compartmentalized, which are considered as a detoxification mechanism of heavy metals due to the reduced heavy metal ions concentration in the cytoplasm (Su et al., 2014). The present study found that an increase in P supply decreased Se concentration in the cell organelle and soluble fraction of roots, but enhanced Se distribution in the cell organelle and soluble fractions (Figure 1A). The large proportion of Se distributing in the vacuoles means that Se entrance into the xylem from the root cells, and thus translocation to shoots was limited (Nocito et al., 2011). In contrast, an increase in P supply enhanced Se distribution in the cell wall of stems, but inhibited Se distribution in the cell organelle and soluble fraction of stems (Figure 1B). The current results also suggested that P supply enhanced Se transportation across the cell membrane and transported to the leaves. In leaves, an increase in P supply decreased Se distribution in the soluble fraction, but increased in the cell organelle fraction (Figure 1C). These results suggest that the increasing P supply may enhance metabolism related to Se by increasing distribution of Se in the cell organelle (Winkel et al., 2015).
Plant Se metabolism and transformation are important for Se nutrition of humans, because human dietary Se are mainly from plants. In addition, the beneficial or toxic effect of Se on plant growth and physiological metabolism is closely related to the chemical forms of Se in plants (Beilstein et al., 1991). Root-to-shoot or stem-to-leaf translocation of Se and the bioavailability of Se in plants are also dependent on its chemical forms in plant tissues (Beilstein et al., 1991; Kassis et al., 2007; Su et al., 2014). The present study found that most of Se in roots, stems, and leaves of winter wheat is in the form of residual Se (30.2–65.9%), followed by the monosaccharides, two sugars, and small molecules organic Se (5.70–23.0%, extracted by 80% ethanol), water-soluble protein and sugar integrated Se, as well as inorganic Se (12.3–19.6%, extracted by deionized-H2O), soluble protein integrated Se, and exchangeable Se substance with salt (4.44–24.75%, extracted by 1 M NaCl) (Table 3 and Figure 2). Liu Y. et al. (2010) and Li F. et al. (2008) also reported that Se chemical forms extracted by 80% ethanol, deionized water, and 1 M NaCl predominated in plant issues of Se-enriched tea. Increasing P supply obviously decreased Se concentration in each chemical forms in roots, stems, and leaves of winter wheat.
An increase in P supply improved Se proportion in the form of residue, but resulted in a decrease in the Se form extracted by 80% ethanol in all tissues (Table 3 and Figure 2), which suggests that the increase of P supply can inhibit Se bioavailability in winter wheat. With P supply levels increased, Se form extracted by 6 M HCl in roots and leaves decreased. The 6 M HCl-extractable Se consisted of Se-oxalate complexes in plant issues, which are the inert metabolism substances in plants (Libert and Franceschi, 1987). Under the condition of lower P supply levels, increasing the proportion of the 6 M HCl-extractable Se may result in decrease in Se phyto-availability, and have a role in alleviating the Se toxicity in plants issues. In addition, with an increase in P supply levels, the Se form extracted by 1 M NaCl in roots decreased, but that in stems and leaves increased. The Se form extracted by 1 M NaCl contains salt soluble protein-integrated Se and exchangeable Se substance with salt (Liu Y. et al., 2010). The Se is assimilated into Se–amino acids complexes to perform its normal physiological function after absorbing by plants (Kaur et al., 2014). These results suggest that the Se forms extracted by 1 M NaCl in stems and leaves due to an increase in P supply levels may play an positive role in Se physiological metabolism in shoots of winter wheat. Moreover, the increasing P supply had different influences on the other forms of Se in various tissues. These differences may be due to the different role of P and Se in diverse plant tissues.
Selenium biofortifications are often through the edible parts of crops to supplement Se nutrition for humans. In the previous study (Sanghun et al., 2011), it was found that moderate application of P fertilizer combined with Se application had a positive effect on grain yield, but excessive P fertilization had a negative effect on Se absorption and grain Se concentration in winter wheat. The current study results imply that avoiding excessive P fertilization is conducive to maintain the balance of obtaining greater grain yield and grain Se concentration in winter wheat in agricultural practices. The current study only investigated the Se uptake and chemical transformation in the vegetative parts of winter wheat in response to the levels of P supply.
Conclusion
The current study would provide better understandings on the mechanism of P application on Se absorption and transformation in winter wheat, and could assist in agricultural practices for increasing grain Se concentration and grain yield of winter wheat to supplement Se nutrition safely for humans and animals. The current study demonstrated that an increase in P supply inhibited Se accumulation in winter wheat that increasing P supply significantly decreased Se concentration and accumulation in roots, stems, and leaves in winter wheat. An increase in P supply significantly inhibited Se distribution in root cell wall and shoots (including stems and leaves) soluble fraction, but enhanced Se distribution in root cell organelle, root soluble fraction, stem cell wall, and leaf cell organelle. Therefore, the current study results suggest that increasing P supply has negative effect on the transport of Se from root to shoot, and enhanced Se transportation across the cell membrane. Increased P supply alters chemical forms of Se in tissues of winter wheat, indicating that P supply influences the bioavailability of Se differently in various tissues.
Author Contributions
ZN conceived and designed the experiments. ZS and JL performed the experiments. PZ and SQ analyzed the data. HL wrote the paper.
Funding
This research was financially supported by the National Natural Science Foundation of China (Program Nos. 41201286 and 41501311) and the Young Core Instructor Funding Scheme of Henan Province, China (Program No. 2013-045).
Conflict of Interest Statement
The authors declare that the research was conducted in the absence of any commercial or financial relationships that could be construed as a potential conflict of interest.
Supplementary Material
The Supplementary Material for this article can be found online at: https://www.frontiersin.org/articles/10.3389/fpls.2018.00373/full#supplementary-material
References
Altansuvd, J., Nakamaru, Y. M., Kasajima, S., Ito, H., and Yoshida, H. (2014). Effect of long-term phosphorus fertilization on soil Se and transfer of soil Se to crops in northern Japan. Chemosphere 107, 7–12. doi: 10.1016/j.chemosphere.2014.02.056
Anderson, J. (1993). “Selenium interactions in sulfur metabolism,” in Sulfur Nutrition and Assimilation in Higher Plants: Regulatory Agricultural and Environmental Aspects, ed. I. S. De Kok (Amsterdam: SPB Academic), 49–60.
Arinola, O. G., and Charles-Davies, M. A. (2008). Micronutrient levels in the plasma of Nigerian females with breast cancer. Afr. J. Biotechnol. 7, 1620–1623. doi: 10.5897/AJB08.115
Arnon, D. I., and Hoagland, D. R. (1940). Crop production in artificial culture solutions and in soils with special reference to factors influencing yields and absorption of inorganic nutrients. Soil Sci. 50, 463–485.
Atafar, Z., Mesdaghinia, A., Nouri, J., Homaee, M., Yunesian, M., Ahmadimoghaddam, M., et al. (2010). Effect of fertilizer application on soil heavy metal concentration. Environ. Monit. Assess. 160, 83–89. doi: 10.1007/s10661-008-0659-x
Awasthi, Y. C., Beutler, E., and Srivastava, S. K. (1975). Purification and properties of human erythrocyte glutathione peroxidase. J. Biol. Chem. 250, 5144–5149.
Beilstein, M., Whanger, P., and Yang, G. (1991). Chemical forms of selenium in corn and rice grown in a high selenium area of China. Biomed. Environ. Sci. 4, 392–398.
Cakmak, I. (2008). Enrichment of cereal grains with zinc: agronomic or genetic biofortification? Plant Soil 302, 1–17. doi: 10.1007/s11104-007-9466-3
Cater, D., Robbins, C., and Brown, M. (1972). Effect of phosphorus fertilization on the selenium concentration in alfalfa (Medicago sativa). Soil Sci. Soc. Am. J. 36, 624–628. doi: 10.2136/sssaj1972.03615995003600040035x
Du, Q., Wang, C. Q., Li, B., Li, H. X., and Liu, Y. (2010). Effects of Se, Zn and their interaction on the subcellular distribution of selenium in spring tea leaves. Acta Hortic. Sin. 37, 794–800.
Elrashidi, M., Adriano, D., Workman, S., and Lindsay, W. (1987). Chemical equilibria of selenium in soils: a theoretical development. Soil Sci. 144, 141–152. doi: 10.1097/00010694-198708000-00008
Erenoglu, E. B., Kutman, U. B., Ceylan, Y., Yildiz, B., and Cakmak, I. (2011). Improved nitrogen nutrition enhances root uptake, root-to-shoot translocation and remobilization of zinc (65Zn) in wheat. New Phytol. 189, 438–448. doi: 10.1111/j.1469-8137.2010.03488.x
Gallego, S. M., Pena, L. B., Barcia, R. A., Azpilicueta, C. E., Iannone, M. F., Rosales, E. P., et al. (2012). Unravelling cadmium toxicity and tolerance in plants: insight into regulatory mechanisms. Environ. Exp. Bot. 83, 33–46. doi: 10.1016/j.envexpbot.2012.04.006
Hall, J. L. (2002). Cellular mechanisms for heavy metal detoxification and tolerance. J. Exp. Bot. 53, 1–11. doi: 10.1093/jexbot/53.366.1
Hart, D. J., Fairweather-Tait, S. J., Broadley, M. R., Dickinson, S. J., Foot, I., Knott, P., et al. (2011). Selenium concentration and speciation in biofortified flour and bread: retention of selenium during grain biofortification, processing and production of Se-enriched food. Food Chem. 126, 1771–1778. doi: 10.1016/j.foodchem.2010.12.079
He, Z., Zhou, Q., and Xie, Z. (1998). Soil-Chemical Balances of Pollution and Beneficial Elements. Beijing: China Environmental Science Press.
Institute of Medicine (2000). Dietary Reference Intakes for Vitamin C, Vitamin E, Selenium, and Carotenoids. Washington, DC: National Academies Press.
Kassis, E., Cathala, N., Rouached, H., Fourcroy, P., Berthomieu, P., Terry, N., et al. (2007). Characterization of a selenate-resistant Arabidopsis mutant. Root growth as a potential target for selenate toxicity. Plant Physiol. 143, 1231–1241. doi: 10.1104/pp.106.091462
Kaur, N., Sharma, S., Kaur, S., and Nayyar, H. (2014). Selenium in agriculture: a nutrient or contaminant for crops? Arch. Agron. Soil Sci. 60, 1593–1624.
Le, C., Zha, Y., Li, Y., Sun, D., Lu, H., and Yin, B. (2010). Eutrophication of lake waters in China: cost, causes, and control. Environ. Manage. 45, 662–668. doi: 10.1007/s00267-010-9440-3
Lévesque, M. (1974). Some aspects of selenium relationships in eastern Canadian soils and plants. Can. J. Soil Sci. 54, 205–214. doi: 10.4141/cjss74-027
Li, F., Wang, F., Yu, F., Fang, Y., Xin, Z., Yang, F., et al. (2008). In vitro antioxidant and anticancer activities of ethanolic extract of selenium-enriched green tea. Food Chem. 111, 165–170. doi: 10.1016/j.foodchem.2008.03.057
Li, H. F., McGrath, S. P., and Zhao, F. J. (2008). Selenium uptake, translocation and speciation in wheat supplied with selenate or selenite. New Phytol. 178, 92–102. doi: 10.1111/j.1469-8137.2007.02343.x
Li, M., Sheng, G.-P., Wu, Y.-J., Yu, Z.-L., Bañuelos, G. S., and Yu, H.-Q. (2014). Enhancement of nitrogen and phosphorus removal from eutrophic water by economic plant annual ryegrass (Lolium multiflorum) with ion implantation. Environ. Sci. Pollut. Res. Int. 21, 9617–9625. doi: 10.1007/s11356-014-2987-4
Li, T. Q., Yang, X. E., Yang, J. Y., and He, Z. L. (2006). Zn accumulation and subcellular distribution in the Zn hyperaccumulator Sedum alfredii Hance. Pedosphere 16, 616–623. doi: 10.1016/S1002-0160(06)60095-7
Libert, B., and Franceschi, V. R. (1987). Oxalate in crop plants. J. Agric. Food Chem. 35, 926–938. doi: 10.1021/jf00078a019
Liu, H., Hu, C., Hu, X., Nie, Z., Sun, X., Tan, Q., et al. (2010). Interaction of molybdenum and phosphorus supply on uptake and translocation of phosphorus and molybdenum by Brassica napus. J. Plant Nutr. 33, 1751–1760. doi: 10.1080/01904167.2010.503778
Liu, Q., Wang, D. J., Jiang, X. J., and Cao, Z. H. (2004). Effects of the interactions between selenium and phosphorus on the growth and selenium accumulation in rice (Oryza sativa). Environ. Geochem. Health 26, 325–330. doi: 10.1023/B:EGAH.0000039597.75201.57
Liu, Y., Wang, C., Li, B., Li, H., and Zhang, W. (2010). Effect of selenium-zinc interaction on the chemical form of selenium of tea leaves in spring season. J. Tea Sci. 30, 343–349.
Lyons, G., Stangoulis, J., and Graham, R. (2003). High-selenium wheat: biofortification for better health. Nutr. Res. Rev. 16, 45–60. doi: 10.1079/NRR200255
Malik, J. A., Goel, S., Kaur, N., Sharma, S., Singh, I., and Nayyar, H. (2012). Selenium antagonises the toxic effects of arsenic on mungbean (Phaseolus aureus Roxb.) plants by restricting its uptake and enhancing the antioxidative and detoxification mechanisms. Environ. Exp. Bot. 77, 242–248. doi: 10.1016/j.envexpbot.2011.12.001
Mora, M. L., Pinilla, L., Rosas, A., and Cartes, P. (2008). Selenium uptake and its influence on the antioxidative system of white clover as affected by lime and phosphorus fertilization. Plant Soil 303, 139–149. doi: 10.1007/s11104-007-9494-z
Nie, Z. J., Zhao, P., Wang, J., Li, J. F., and Liu, H. E. (2017). Absorption kinetics and subcellular fractionation of zinc in winter wheat in response to nitrogen supply. Front. Plant Sci. 8:1435. doi: 10.3389/fpls.2017.01435
Nocito, F. F., Lancilli, C., Dendena, B., Lucchini, G., and Sacchi, G. A. (2011). Cadmium retention in rice roots is influenced by cadmium availability, chelation and translocation. Plant Cell Environ. 34, 994–1008. doi: 10.1111/j.1365-3040.2011.02299.x
Ogra, Y., Ishiwata, K., Ruiz Encinar, J., Łobiński, R., and Suzuki, K. T. (2004). Speciation of selenium in selenium-enriched shiitake mushroom, Lentinula edodes. Anal. Bioanal. Chem. 379, 861–866. doi: 10.1007/s00216-004-2670-6
Pan, X., Chen, G., Shi, C., Chai, M., Liu, J., Cheng, S., et al. (2016). Effects of Zn stress on growth, Zn accumulation, translocation, and subcellular distribution of Spartina alterniflora Loisel. Clean Soil Air Water 44, 579–585. doi: 10.1002/clen.201400288
Pennanen, A., Tailin, X., and Hartikainen, H. (2002). Protective role of selenium in plant subjected to severe UV irradiation stress. J. Appl. Bot. 76, 66–76.
Pickering, I. J., Wright, C., Bubner, B., Ellis, D., Persans, M. W., Yu, E. Y., et al. (2003). Chemical form and distribution of selenium and sulfur in the selenium hyperaccumulator Astragalus bisulcatus. Plant Physiol. 131, 1460–1467. doi: 10.1104/pp.014787
Ramos, I., Esteban, E., Lucena, J. J., and Gárate, A. N. (2002). Cadmium uptake and subcellular distribution in plants of Lactuca sp. Cd–Mn interaction. Plant Sci. 162, 761–767. doi: 10.1016/S0168-9452(02)00017-1
Rathore, V., Bajaj, Y., and Wittwer, S. (1972). Subcellular localization of zinc and calcium in bean (Phaseolus vulgaris L.) tissues. Plant Physiol. 49, 207–211. doi: 10.1104/pp.49.2.207
Rayman, M. P. (2012). Selenium and human health. Lancet 379, 1256–1268. doi: 10.1016/S0140-6736(11)61452-9
Rotruck, J. T., Pope, A. L., Ganther, H. E., Swanson, A. B., Hafeman, D. G., and Hoekstra, W. G. (1980). Selenium: biochemical role as a component of glutathione peroxidasec. Nutr. Rev. 38, 280–283. doi: 10.1111/j.1753-4887.1980.tb05961.x
Sanghun, L., Howard, J. W., and James, J. D. (2011). Effect of phosphate and sulfate fertilizers on selenium uptake by wheat (Triticum aestivum). Soil Sci. Plant Nutr. 57, 696–704. doi: 10.1080/00380768.2011.623282
Singh, M., and Malhotra, P. (1976). Selenium availability in berseem (Trifolium alexandrinum) as affected by selenium and phosphorus application. Plant Soil 44, 261–266. doi: 10.1007/BF00016977
Singh, M., and Singh, N. (1978). Selenium toxicity in plants and its detoxication by phosphorus. Soil Sci. 126, 255–262. doi: 10.1097/00010694-197811000-00001
Su, Y., Liu, J., Lu, Z., Wang, X., Zhang, Z., and Shi, G. (2014). Effects of iron deficiency on subcellular distribution and chemical forms of cadmium in peanut roots in relation to its translocation. Environ. Exp. Bot. 97, 40–48. doi: 10.1016/j.envexpbot.2013.10.001
Turakainen, M., Hartikainen, H., and Seppänen, M. M. (2004). Effects of selenium treatments on potato (Solanum tuberosum L.) growth and concentrations of soluble sugars and starch. J. Agric. Food Chem. 52, 5378–5382. doi: 10.1021/jf040077x
Weigel, H. J., and Jäger, H. J. (1980). Subcellular distribution and chemical form of cadmium in bean plants. Plant Physiol. 65, 480–482. doi: 10.1104/pp.65.3.480
Winkel, L. H. E., Vriens, B., Jones, G. D., Schneider, L. S., Pilon-Smits, E., and Bañuelos, G. S. (2015). Selenium cycling across soil-plant-atmosphere interfaces: a critical review. Nutrients 7, 4199–4239. doi: 10.3390/nu7064199
Zhang, C., Zhang, P., Mo, C., Yang, W., Li, Q., Pan, L., et al. (2013). Cadmium uptake, chemical forms, subcellular distribution, and accumulation in Echinodorus osiris Rataj. Environ. Sci. Proc. Impacts 15, 1459–1465. doi: 10.1039/C3EM00002H
Zhang, C. Q., Wang, L., Hua, C. L., Jin, S. T., and Liu, P. T. (2016). Potentialities of fertilizer reduction for grain produce and effects on carbon emissions. Resour. Sci. 38, 790–797.
Zhang, D., Dong, T., Ye, J., and Hou, Z. (2017). Selenium accumulation in wheat (Triticum aestivum L) as affected by coapplication of either selenite or selenate with phosphorus. Soil Sci. Plant Nutr. 63, 37–44. doi: 10.1080/00380768.2017.1280377
Zhang, W., Lin, K., Zhou, J., Zhang, W., Liu, L., and Zhang, Q. (2014). Cadmium accumulation, sub-cellular distribution and chemical forms in rice seedling in the presence of sulfur. Environ. Toxicol. Pharmacol. 37, 348–353. doi: 10.1016/j.etap.2013.12.006
Zhao, L., Zhao, G., Zhao, Z., Chen, P., Tong, J., and Hu, X. (2004). Selenium distribution in a Se-enriched mushroom species of the genus Ganoderma. J. Agric. Food Chem. 52, 3954–3959. doi: 10.1021/jf049965i
Keywords: selenium, chemical forms, phosphorus, subcellular distribution, winter wheat
Citation: Liu H, Shi Z, Li J, Zhao P, Qin S and Nie Z (2018) The Impact of Phosphorus Supply on Selenium Uptake During Hydroponics Experiment of Winter Wheat (Triticum aestivum) in China. Front. Plant Sci. 9:373. doi: 10.3389/fpls.2018.00373
Received: 06 October 2017; Accepted: 06 March 2018;
Published: 20 March 2018.
Edited by:
Stefano Cesco, Free University of Bozen-Bolzano, ItalyReviewed by:
Avinash Chandra Pandey, Syngenta India, IndiaPrzemyslaw Niedzielski, Adam Mickiewicz University in Poznań, Poland
Fernando Carlos Gómez-Merino, Inicio COLPOS, Mexico
Copyright © 2018 Liu, Shi, Li, Zhao, Qin and Nie. This is an open-access article distributed under the terms of the Creative Commons Attribution License (CC BY). The use, distribution or reproduction in other forums is permitted, provided the original author(s) and the copyright owner are credited and that the original publication in this journal is cited, in accordance with accepted academic practice. No use, distribution or reproduction is permitted which does not comply with these terms.
*Correspondence: Zhaojun Nie, bnpqMDUxMUAxMjYuY29t