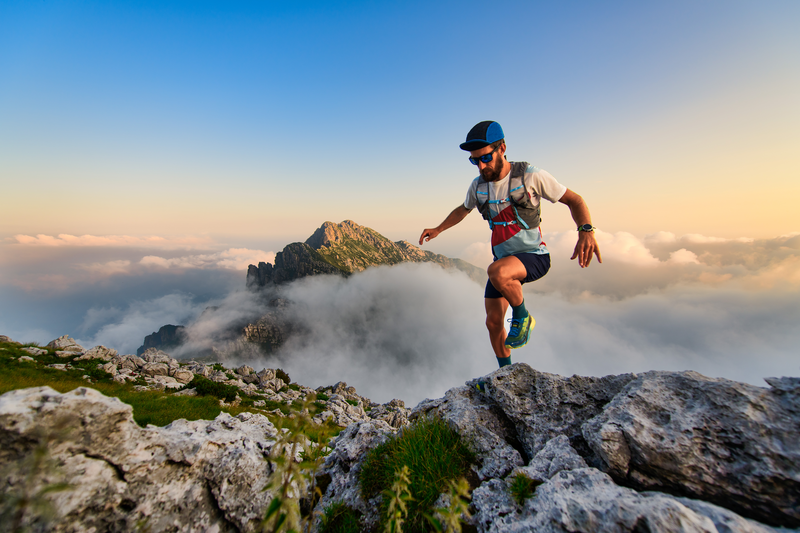
94% of researchers rate our articles as excellent or good
Learn more about the work of our research integrity team to safeguard the quality of each article we publish.
Find out more
MINI REVIEW article
Front. Plant Sci. , 27 February 2018
Sec. Plant Nutrition
Volume 9 - 2018 | https://doi.org/10.3389/fpls.2018.00248
Stemflow, a precipitation and solute supply to soils near tree stems, can play a wide array of roles in ecosystem functioning. However, stemflow’s ecohydrological functions have been primarily studied in forests with voluminous stemflow because resource subsidy is currently considered stemflow’s only impact on near-stem soils. This common assumption ignores controls that stemflow generation may exert via resource limitation (when stemflow < open rainfall and near-stem throughfall is negligible). We reviewed selected literature across numerous forests to evaluate the predominance of stemflow as a potential resource limitation to near-stem soils and characterized the concentrated, but meager, solute flux from low stemflow generators. Global observations of stemflow were highly skewed (skewness = 4.6) and leptokurtic (kurtosis = 28.8), where 69% of observations were ≤2% of rainfall. Stemflow ≤ 2% of rainfall is 10–100 times more chemically enriched than open rainfall, yet low volumes result in negligible solute fluxes (under 1 g m-2 y-1). Reduced stemflow may be the global and regional norm, creating persistently dry near-stem soils that receive infrequent, salty, and paltry precipitation flux if throughfall is also low. Ignoring stemflow because it results in scarcity likely limits our understanding of ecosystem functioning as resource limitations alter the fate of soil nutrients, energy flows, and spatial patterning of biogeochemical processes.
Forest canopies are often the first terrestrial surfaces to store, evaporate and redistribute precipitation, thereby affecting all subsequent hydrological processes (Savenije, 2004) and related energy flows (Davies-Barnard et al., 2014). Precipitation that is redistributed to the forest surface either drips through canopy gaps and from canopy surfaces, as throughfall, or drains along tree stems, as stemflow. This precipitation redistribution produces persistent patterns of wet and dry areas at the forest floor (Keim et al., 2005; Johnson and Lehmann, 2006) that influence soil physicochemical and microbial community characteristics (Chang and Matzner, 2000; Moore et al., 2016; Rosier et al., 2016). Most rainfall is redistributed as throughfall (>70%), generally resulting in <5% of rainfall becoming stemflow (Levia and Frost, 2003). However, stemflow from 5% of rainfall across the area of a mature tree canopy can funnel >20 times more water to near-stem soils than open rainfall on an equivalent area (Rodrigo et al., 2003). This stemflow “funneling” effect has been reviewed extensively (Levia and Frost, 2003; Johnson and Lehmann, 2006; Ikawa, 2007; McKee, 2010; Levia and Germer, 2015) and, in part, inspired stemflow studies around the globe (Figure 1A). Yet, instances where the funneling effect fails (stemflow inputs < throughfall or open rainfall) are rarely discussed, often ignored, and, as a result, the authors know of no study that has placed reduced stemflow in biogeochemical context. Low stemflow generation may produces the opposite extreme of the funneling effect if poorly subsidized by near-stem throughfall—localized resource scarcity—which is a condition with profound impacts on soil biogeochemical players and processes (Manzoni et al., 2012).
FIGURE 1. (A) Locations around the globe (Supplementary Table S1) where stemflow has been measured are well-distributed throughout the Earth’s forests. (B) Histograms of stemflow (% rainfall) from studies reporting on 346 tree species or forest types (Supplementary Table S2). Studies that simply report “negligible” stemflow have been placed in the <1% category in the histogram.
For example, soils surrounding the stems of Amazonian Eschweilera trees receive 0.07% of the annual rainfall, with the remaining rainfall becoming throughfall or returned to the atmosphere via interception (Schroth et al., 2001). This results in 127 L of rain each year reaching an infiltration area around individual Eschweilera stems ≥0.8 m2 (Schroth et al., 2001). Consequently, if throughfall is absent or low near the stem, the stemflow process surprisingly classifies tropical rainforest soils near Eschweilera stems as “highly arid” per the De Martonne Aridity Index (4.4 mm °C-1) based on the reported mean annual temperature (26°C). Near-stem soils of other tropical rainforest tree species studied by Schroth et al. (2001) also classify as arid-to-semiarid based on low stemflow supply: Bixa orellana (4.6 mm °C-1) and Theobroma grandiflorum (12.0 mm °C-1). Furthermore, the classical definition of “true deserts” (mean annual precipitation < 200 mm y-1: Ward, 2015) classifies the ∼160 mm y-1 of stemflow precipitation reaching soils near the stems of these tropical rainforest tree species as arid. The juxtaposition of two extreme stemflow conditions (stemflow funneling versus scarcity) raises questions not addressed by previous reviews: what is the frequency of low versus high stemflow observations in forests across the globe? Moreover, what is the shape of the distribution of global stemflow observations in forests? Previous work has reported mean and median stemflow production values across climates (Levia and Frost, 2003; McKee, 2010), but past stemflow statistics were not controlled for repetitive sampling of forests/species in areas with greater research output (e.g., European and northeastern United States hardwoods), nor does previous work report how well a median or mean represented the data distribution (i.e., was the data skewed and, if so, to what degree?).
How stemflow production varies across forest systems has important biogeochemical implications beyond water abundance or scarcity for near-stem soils. The concentration and flux of solutes, particulates, microbial cells, and fungal spores transported by stemflow to the surface are strongly linked to a canopy’s ability to efficiently drain rainfall to the stem (Levia and Germer, 2015). In this way, stemflow quantity is linked to soil structure (Li et al., 2009), soil chemistry (Aboal et al., 2015), soil solution chemistry (Chang and Matzner, 2000), and soil microbial community structure (Rosier et al., 2016). This mini-review evaluated select literature that represents unique forest type-climate combinations around the globe to test the primary hypothesis that stemflow, globally and across major climate zones, may be more often a resource limitation than a resource subsidy to forests’ near-stem soils. Next, we review the chemical concentration, flux, and edaphic effects of low stemflow generation to describe the unique near-stem soil conditions it engenders. The review and contextualization of a major canopy influence on near-stem soil areas in forests strengthens our understanding of canopy-soil interchanges, many of which are linked to global biogeochemical processes of societal significance (Bonan, 2016).
To date, stemflow has been sampled in most forest ecosystems (Figure 1A, data provided in Supplementary Table S1), from Nothofagus pumilio at the southern-most tip of Patagonia (54°S: Frangi et al., 2005) to Picea glauca in the Northwest Territories (69° N: Gill, 1975). Stemflow has also been measured in several remote ecosystems, including the Galapagos Islands (Pryet et al., 2012), Canary Islands (Aboal et al., 2015), Central Pacific atolls of the Republic of Kiribati (White et al., 2007), and the Namibian Desert (Roth-Nebelsick et al., 2012) (Figure 1A). An important data gap remains in the Congo tropical rainforest (Figure 1A), a biodiversity hotspot that plays important roles in global biogeochemical cycles. Stemflow research in the boreal forests has been sparse, particularly in Russia (Figure 1A). In contrast, stemflow of northern temperate forests has been extensively studied (Figure 1A).
A survey of 346 observations selected to represent the range of combinations in tree species-climate conditions (Supplementary Table S2) indicates that approximately two-thirds (69%) of stemflow observations fall below 2% of rainfall (Figure 1B). Drainage of less than 2% of rainfall across a tree canopy can have a range of outcomes regarding precipitation supply to the near-stem soils dependent, in large part, on the ratio of the canopy area to near-stem infiltration area. For stemflow produced from 2% of rainfall to equal open rainwater supply, for example, the ratio of canopy area (for capturing and draining water to the stem) to a conservative 1 m2 infiltration area around the stem base (this area is unlikely to be smaller for most mature trees) would have to be 50:1. Thus, forests draining ≤2% of rainfall as stemflow are likely depriving near-stem soils of water relative to the open if near-stem throughfall is negligible. Despite this, most attention regarding stemflow and its biogeochemical impacts is focused on the rarer instances where stemflow is voluminous (Levia and Germer, 2015). Of course, stemflow percentage widely ranged across species, with a minimum of 0.003% for Larix cajanderi in Siberia (Toba and Ohta, 2005) and maximum of 33.9% for Psidium cattleyanum invading Hawaiian forests (Safeeq and Fares, 2012).
The data distributions for global and, when enough observations were available, individual climates were strongly skewed (Figure 1B). Shape of the data distributions were analyzed for skewness (asymmetry) and kurtosis (tail structure). A common rule for kurtosis is that normal distributions are <3 (platykurtic) while long, “heavy” tailed distributions are >3 (leptokurtic). Skewness of global stemflow data was double the common normality threshold (4.6 ± 0.1 SE versus 2.0) and it was strongly leptokurtic (28.8 ± 0.3 SE). Therefore, mean (and even median) stemflow percentage does not represent the data’s central tendency well. Mean and median stemflow values have been reported, ranging 3.7–5.0%, 3.5–11.3%, 1.6–4.0% for boreal, temperate, and tropical forests, respectively (Levia and Frost, 2003; McKee, 2010), but our literature synthesis reveals that a majority of stemflow observations in boreal, temperate, and tropical forests fall below these ranges: 94% (n = 31/33), 77% (n = 103/133), and 66% (n = 55/83), respectively (Figure 1B). Thus, to date, stemflow in most forests appears to act as a water resource limitation to near-stem soils, unless subsidized by near-stem throughfall.
Stemflow is mostly measured by installing collars to capture stem drainage at, or near, breast height: 1.4 m above the surface—see photographs by Levia and Germer (2015). As a result, prior to entering the soil, stemflow must first pass through the various covers at the stem base and forest floor – all of which can store substantial amounts of water. Vascular and non-vascular epiphytic vegetation attach to stem bark surfaces and are ubiquitous across forest ecosystems (Zotz and Bader, 2009). Although the global distribution of cryptogamic covers is poorly understood, they inhabit all studied forest ecosystems (Elbert et al., 2012). Lichens commonly fix themselves to stem bark and can become dense near the stem base, where, for example, over one-third of lichen species have been found on the bottom 2 m of the tree stem (Marmor et al., 2013). Bryophytes (mosses, liverworts, and hornworts) are also commonly found at the stem bottom. Should the stem lack epiphytes, stemflow will still likely encounter lichen, bryophyte, or vascular vegetation in the soils immediately surrounding the trunk. The significant water storage capacity of these vegetation covers (up to 1000% of dry weight: Van Stan and Pypker, 2015), when present, can further diminish stemflow water supply to soils. When on- and near-stem vegetation cover is absent, there will be an absorbent litter layer capable of storing more water than the ≤2% of rainfall that is drained as stemflow by most forests (1–3 mm storm-1: Gerrits and Savenije, 2011). Gerrits and Savenije (2011) also found that forests generally lose 10–60% of net rainfall when both evaporation and storage of rainfall by the forest floor are considered. The amount of rainfall lost to the forest floor is therefore often an order of magnitude larger than that provided by stemflow generated from ≤2% of rainfall.
Volumetrically small stemflow in most forests is unlikely to bypass major water storage reservoirs in the forest floor (as has been observed for prodigious stemflow producers: Schwärzel et al., 2012). It also unlikely that rainwater traveling down the stem is sheltered significantly from evaporative drivers, as recent work found stem evaporative losses can be as high as those at the canopy edge (Van Stan et al., 2017a). These hydrometeorological conditions and water storage demands of elements at the stem base and forest floor, in conjunction with inefficient stemflow generation, can require substantial rainfall to initiate stemflow generation in most forests. This equates to fewer storms contributing stemflow to near-stem soils. Ultimately, the near-stem soils of most forests will almost certainly access only a fraction of the rainfall drained as stemflow. Even if near-stem soils receive water from lateral flow or superficial runoff, which can occur for large storms in humid forests, they still must compensate for a persistent and profound shortage of stemflow supply. Substantial throughfall has been observed near stems of significant stemflow generating forests (Bialkowski and Buttle, 2015), but the few studies on throughfall contributions near low stemflow generating stems find negligible throughfall near the stem (Guswa and Spence, 2012; Fathizadeh et al., 2014) and, although there is no consensus on throughfall pattern controls (Levia and Frost, 2006), many studies find throughfall decreases with increasing proximity to the stem—particularly in low stemflow generating stands (e.g., Johnson, 1990; Beier et al., 1993; Whelan and Anderson, 1996; Ito et al., 2008). Increased branch biomass and bark surfaces near the stem, due to major branch confluences, enhance the likelihood that throughfall is a negligible near-stem subsidy in low-stemflow generating stands due to the substantial water storage not often satisfied by negligible stem drainage (Levia and Herwitz, 2005). Whether and to what extent throughfall can supplement reduced stemflow inputs is a current knowledge gap. However, this is a stark contrast to the preferential moisture recharge (Durocher, 1990), bypass flow along roots (Martinez-Meza and Whitford, 1996), and infiltration-excess overland flow (Herwitz, 1986) that has long been associated with stemflow.
Stemflow is arguably the longest path a rain drop can travel to reach the soil surface, requiring lengthy interaction between rainfall and canopy (mostly bark) surfaces. As a result of the many mechanisms that can exchange solutes and particulates between the canopy and rainfall, stemflow can potentially be more chemically enriched than most flow paths through the canopy (Levia and Germer, 2015). The canopy solute reservoir available to stemflow, however, is limited and intrastorm observations of efficient stemflow generators reveal that solute concentrations drop precipitously after several liters of stemflow over 0.5–1 h (Levia et al., 2011). No studies known to the authors have reported on intrastorm stemflow solute concentration dynamics for tree species that divert ≤2% of rainfall to their stems. Nevertheless, it is unlikely that low stemflow generators will deplete the canopy solute reservoir (e.g., Bade et al., 2015). In fact, stemflow dissolved organic carbon concentrations from Quercus virginiana (<0.2% stemflow) did not dilute with greater rainfall; rather, concentrations linearly increased with increasing storm size up to tropical storm magnitudes and intensities (Van Stan et al., 2017b). Total stemflow production from <1% of rainfall over several months across Pinus resinosa canopies, for example, was over six times more concentrated in Na+ than voluminous stemflow (nearly 6% of rainfall) from Acer rubrum (Mahendrappa, 1974). In fact, a selection of 31 studies spanning the full range of long-term (several months to annual mean) stemflow percentages shows that the ratio of total dissolved solids (per electrical conductivity) between stemflow and rainfall exponentially decay from 13 times greater than open rainfall (Picea abies: Mosello et al., 2002) to a ∼1:1 ratio when >2% of rainfall drains to the stem (Figure 2). An outlier study not shown in Figure 2, McColl (1970) found the electrical conductivity in stemflow from a Costa Rican Inga species (<1% of rainfall) was over 100 times greater than open rainfall. Since most studied forests generate very low stemflow volumes (Figure 1), the paltry water supply to soils near most tree stems may have several to 100 times the solute concentrations of open precipitation (Figure 2). Theoretical and observational work indicates that a combination of stemflow-induced drought conditions alongside the osmotic stresses caused by infrequent, but highly enriched, water supplies may alter microbial community structure and its traits regulating drought responses (Yuan et al., 2007; Manzoni et al., 2014).
FIGURE 2. The ratio of stemflow (SF) to rainfall (R) electrical conductivity for selected studies that represent a wide range of SF values as a percentage of rainfall amount. Studies associated with each data point are provided in Supplementary Table S3.
Well-researched generous stemflow producers, like Fagus sylvatica in Europe (a Web of Science search for this species, “stemflow,” and “Europe” returned > 80 studies), despite their low solute concentrations, <5 mg l-1 (Staelens, 2006), produce large solute fluxes that can concentrate up to 20% of per ha fluxes for some nutrients (i.e., K+ and Mg2+) to 10–50 m2 ha-1 of basal area, depending on the stand (Berger et al., 2009). In contrast, Larix species that inhabit three continents’ boreal forests generate <1% of stemflow over 20–40 m2 ha-1 of basal area, depending on the stand (Ovington, 1954; Cape et al., 1991; Toba and Ohta, 2005), yet a Web of Science search for the entire genus “Larix” and “stemflow” returns only 25 publications. Larix and the many other inefficient stemflow-generating genera globally—Abies (Negi et al., 1998), Pinus (Santa Regina and Tarazona, 2000), Quercus (Moreno et al., 2001), Tsuga (Ovington, 1954) etc.—can limit soil nutrient flux just as impressively as efficient stemflow generators can augment it. Stemflow from Picea species in the subarctic, for example, supplied <4 and <1% of the annual rainfall K+ and NO3- flux, respectively, to near-stem soils (Moore, 1980). In multiple tropical rainforest sites, total cation and anion solute fluxes from stemflow to near-stem soils were also low, 0.1–0.6 g m-2 y-1 (Tobón et al., 2004). Stemflow in a subtropical Lithocarpus–Castenopsis forest supplied <0.1 g-N m-2 y-1 and <0.01 g-P m-2 y-1 to near-stem soils—an order of magnitude less than precipitation flux despite the removal of epiphytes (Liu et al., 2002). Near-stem soils in temperate hardwood stands containing voluminous stemflow producers, like the aforementioned Fagus sylvatica, alongside low stemflow generators (Fraxinus excelsior, Carpinus betulus, and others) can receive strikingly low solute fluxes at the stand scale: being just 3.7% of throughfall flux (Talkner et al., 2010). Thus, soils near low stemflow generating trees may not only experience severe water limitations (Figure 1) and elevated stemflow solute concentrations (Figure 2), but limited solute flux.
Low stemflow conditions may affect the soil environment and its biogeochemical processes, both individually and in combination. For example, rainfall reductions to forest soils alone have been linked to increased methane uptake (Fest et al., 2017) and shifts in drying-rewetting regimes can alter soil aggregation and aggregate stability (Kaiser et al., 2015). Coupled water-solute flux limitation has been linked to shifts in soil microbial community composition (Rosier et al., 2015) and N-functioning genes (Moore et al., 2016). Limited soil environments, especially those occasionally doused with salty precipitation, also tend to select for lichens and bryophytes due to their high tolerances for desiccation and poor nutrient availability (Proctor et al., 2007; Kranner et al., 2008). These example impacts on the soil environment contrast with those observed and hypothesized for soils receiving substantial stemflow (see discussion of impacts in Introduction).
This mini-review finds that, for most soils near tree stems, the stemflow story is not one of hydrological abundance and the associated biogeochemical processes often described in reviews. Soils near the stems of inefficient stemflow generators may experience little water and nutrient supply and, when stemflow does arrive, it can be 10–100 times more chemically enriched than rainfall or throughfall. This has implications for near-stem soil ecological processes, yet very few studies known to the authors have investigated the effects of stemflow limitation on edaphic conditions and functions (Koch and Matzner, 1993). Stemflow limitation in forests, therefore, merits the attention of scientists working at the intersection of plant ecological and hydrological processes. Questions on this topic abound, including: can throughfall mitigate stemflow-related resource limitations? How do drying-rewetting cycles and near-stem edaphic conditions compare between soils beside high and low stemflow generators? Are near-stem soil microbial communities and/or their functions influenced by low stemflow generation? Does stemflow scarcity versus abundance vary with stand age or management?
All data are available in the supporting information or cited publications.
JVS conceived the study and supervised the literature synthesis and analysis. DG assembled data from the literature and assisted in data analysis. Both authors contributed critically to manuscript preparation.
The authors declare that the research was conducted in the absence of any commercial or financial relationships that could be construed as a potential conflict of interest.
The authors would like to thank the reviewers. Student support was provided by the National Science Foundation (EAR-1518726).
The Supplementary Material for this article can be found online at: https://www.frontiersin.org/articles/10.3389/fpls.2018.00248/full#supplementary-material
Aboal, J., Saavedra, S., and Hernández-Moreno, J. M. (2015). Edaphic heterogeneity related to below-canopy water and solute fluxes in a Canarian laurel forest. Plant Soil 387, 177–188. doi: 10.1007/s11104-014-2285-4
Bade, C., Jacob, M., Leuschner, C., and Hauck, M. (2015). Chemical properties of decaying wood in an old-growth spruce forest and effects on soil chemistry. Biogeochemistry 122, 1–13. doi: 10.1007/s10533-014-0015-x
Beier, C., Hansen, K., and Gunderson, P. (1993). Spatial variability of throughfall fluxes in a spruce forest. Environ. Pollut. 81, 257–267. doi: 10.1016/0269-7491(93)90208-6
Berger, T. W., Untersteiner, H., Toplitzer, M., and Neubauer, C. (2009). Nutrient fluxes in pure and mixed stands of spruce (Picea abies) and beech (Fagus sylvatica). Plant Soil 322, 317–342. doi: 10.1007/s11104-009-9918-z
Bialkowski, R., and Buttle, J. M. (2015). Stemflow and throughfall contributions to soil water recharge under trees with differing branch architectures. Hydrol. Process. 29, 4068–4082. doi: 10.1002/hyp.10463
Bonan, G. B. (2016). Forests, climate, and public policy: A 500-year interdisciplinary odyssey. Annu. Rev. Ecol. Evol. Syst. 47, 97–121. doi: 10.1146/annurev-ecolsys-121415-032359
Cape, J. N., Brown, A. H. F., Robertson, S. M. C., Howson, G., and Paterson, I. S. (1991). Interspecies comparisons of throughfall and stemflow at three sites in northern Britain. For. Ecol. Manage. 46, 165–177. doi: 10.1016/0378-1127(91)90229-O
Chang, S.-C., and Matzner, E. (2000). The effect of beech stemflow on spatial patterns of soil solution chemistry and seepage fluxes in a mixed beech/oak stand. Hydrol. Process. 14, 135–144. doi: 10.1002/(SICI)1099-1085(200001)14:1<135::AID-HYP915>3.0.CO;2-R
Davies-Barnard, T., Valdes, P. J., Jones, C. D., and Singarayer, J. S. (2014). Sensitivity of a coupled climate model to canopy interception capacity. Clim. Dyn. 42, 1715–1732. doi: 10.1007/s00382-014-2100-1
Durocher, M. G. (1990). Monitoring spatial variability of forest interception. Hydrol. Process. 4, 215–229. doi: 10.1002/hyp.3360040303
Elbert, W., Weber, B., Burrows, S., Steinkamp, J., Buedel, B., Andeae, M. O., et al. (2012). Contribution of cryptogamic covers to the global cycles of carbon and nitrogen. Nat. Geosci. 5, 459–462. doi: 10.1038/ngeo1486
Fathizadeh, O., Attarod, P., Keim, R. F., Stein, A., Amiri, G. Z., and Darvishsefat, A. A. (2014). Spatial heterogeneity and temporal stability of throughfall under individual Quercus brantii trees. Hydrol. Process. 28, 1124–1136. doi: 10.1002/hyp.9638
Fest, B., Hinko-Najera, N., von Fischer, J. C., Livesley, S. J., and Arndt, S. K. (2017). Soil methane uptake increases under continuous throughfall reduction in a temperate evergreen, broadleaved Eucalypt forest. Ecosystems 20, 368–379. doi: 10.1007/s10021-016-0030-y
Frangi, J. L., Barrera, M. D., Puigdefábregas, J., Yapura, P. F., Arambarri, A. M., and Richter, L. L. (2005). “Ecologia de los bosques de Tierra Fuego,” in Ecologia y Manejo de los Bosques de Argentina, eds J. F. Goya, J. L. Frangi, and M. F. Arturi (Buenos Aires: Editorial de la Universidad Nacional de La Plata), 1–88.
Gerrits, A. M. J., and Savenije, H. H. G. (2011). “Forest floor interception,” in Forest Hydrology and Biogeochemistry, eds D. F. Levia, D. Carlyle-Moses, and T. Tanaka (Heidelberg: Springer-Verlag), 445–454. doi: 10.1007/978-94-007-1363-5_22
Gill, D. (1975). Influence of white spruce trees on permafrost-table microtopography, Mackenzie River Delta. Can. J. Earth Sci. 12, 263–272. doi: 10.1139/e75-023
Guswa, A. J., and Spence, C. M. (2012). Effect of throughfall variability on recharge: application to hemlock and deciduous forests in western Massachusetts. Ecohydrology 5, 563–574. doi: 10.1002/eco.281
Herwitz, S. R. (1986). Infiltration-excess caused by stemflow in a cyclone-prone tropical rainforest. Earth Surf. Process. Landforms 11, 401–412. doi: 10.1002/esp.3290110406
Ikawa, R. (2007). Literature review of stemflow generation and chemical characteristics in Japanese forests. J. Jpn. Assoc. Hydrol. Sci. 37, 187–200. doi: 10.4145/jahs.37.187
Ito, A., Onda, Y., Nanko, K., Fukuyama, T., and Moriwaki, H. (2008). Experimental study on spatial distribution of throughfall under a Japanese cypress tree. J. Jpn. Soc. Hydrol. Water Resour. 21, 273–284. doi: 10.3178/jjshwr.21.273
Johnson, M. S., and Lehmann, J. (2006). Double-funneling of trees: stemflow and root-induced preferential flow. Ecoscience 13, 324–333. doi: 10.2980/i1195-6860-13-3-324.1
Johnson, R. C. (1990). The interception, throughfall and stemflow in a forest in highland Scotland and the comparison with other upland forests in the UK. J. Hydrol. 118, 281–287. doi: 10.1016/0022-1694(90)90263-W
Kaiser, M., Kleber, M., and Berhe, A. A. (2015). How air-drying and rewetting modify soil organic matter characteristics: an assessment to improve data interpretation and inference. Soil Biol. Biochem. 80, 324–340. doi: 10.1016/j.soilbio.2014.10.018
Keim, R. F., Skaugset, A. E., and Weiler, M. (2005). Temporal persistence of spatial patterns in throughfall. J. Hydrol. 314, 263–274. doi: 10.1016/j.jhydrol.2005.03.021
Koch, A. S., and Matzner, E. (1993). Heterogeneity of soil and soil solution chemistry under Norway spruce (Picea abies Karst.) and European beech (Fagus silvatica L.) as influenced by distance from the stem basis. Plant Soil 151, 227–237. doi: 10.1007/BF00016288
Kranner, I., Beckett, R., Hochman, A., and Nash, T. H. (2008). Desiccation-tolerance in lichens: a review. Bryologist 111, 576–593. doi: 10.1639/0007-2745-111.4.576
Levia, D. F., and Frost, E. E. (2003). A review and evaluation of stemflow literature in the hydrologic and biogeochemical cycles of forested and agricultural ecosystems. J. Hydrol. 274, 1–29. doi: 10.1016/S0022-1694(02)00399-2
Levia, D. F., and Frost, E. E. (2006). Variation of throughfall volume and solute inputs in wooded ecosystems. Prog. Phys. Geogr. 30, 605–632. doi: 10.1177/0309133306071145
Levia, D. F., and Germer, S. (2015). A review of stemflow generation dynamics and stemflow-environment interactions in forests and shrublands. Rev. Geophys. 53, 673–714. doi: 10.1002/2015RG000479
Levia, D. F., and Herwitz, S. R. (2005). Interspecific variation of bark water storage capacity of three deciduous tree species in relation to stemflow yield and solute flux to forest soils. Catena 64, 117–137. doi: 10.1016/j.catena.2005.08.001
Levia, D. F., Van Stan, J. T., Siegert, C. M., Inamdar, S. P., Mitchell, M. J., Mage, S. M., et al. (2011). Atmospheric deposition and corresponding variability of stemflow chemistry across temporal scales in a mid-Atlantic broadleaved deciduous forest. Atmos. Environ. 45, 3046–3054. doi: 10.1016/j.atmosenv.2011.03.022
Li, X.-Y., Yang, Z.-P., Li, Y.-T., and Lin, H. (2009). Connecting ecohydrology and hydropedology in desert shrubs: stemflow as a source of preferential flow in soils. Hydrol. Earth Syst. Sci. 13, 1133–1144. doi: 10.5194/hess-13-1133-2009
Liu, W., Fox, J. E. D., and Xu, Z. (2002). Nutrient fluxes in bulk precipitation, throughfall, and stemflow in montane subtropical moist forest on Ailao Mountains in Yunnan, south-west China. J. Trop. Ecol. 18, 527–548. doi: 10.1017/S0266467402002353
Mahendrappa, M. K. (1974). Chemical composition of stemflow from some Eastern Canadian tree species. Can. J. For. Res. 4, 1–7. doi: 10.1139/x74-001
Manzoni, S., Schaeffer, S. M., Katule, G., Porporato, A., and Schimel, J. P. (2014). A theoretical analysis of microbial eco-physiological and diffusion limitations to carbon cycling in drying soils. Soil Biol. Biochem. 73, 69–83. doi: 10.1016/j.soilbio.2014.02.008
Manzoni, S., Schimael, J. P., and Porporato, A. (2012). Responses of soil microbial communities to water stress: results from a meta-analysis. Ecology 93, 930–938. doi: 10.1890/11-0026.1
Marmor, L., Tõrra, T., Saag, L., Leppik, E., and Randlane, T. (2013). Lichens on Picea abies and Pinus sylvestris – from tree bottom to the top. Lichenologist 45, 51–63. doi: 10.1017/S0024282912000564
Martinez-Meza, E., and Whitford, W. G. (1996). Stemflow, throughfall and channelization of stemflow by roots in three Chihuahuan desert shrubs. J. Arid Environ. 32, 271–287. doi: 10.1006/jare.1996.0023
McColl, J. G. (1970). Properties of some natural waters in a tropical wet forest of Costa Rica. BioScience 20, 1096–1100. doi: 10.2307/1295415
McKee, A. J. (2010). The Quantitative Importance of Stemflow: An Evaluation of Past Research and Results from a Study in Lodgepole Pine (Pinus contorta var. Latifolia) Stands in Southern British Columbia. Ph.D. thesis, Thompson Rivers University, Kamloops.
Moore, L. D., Van Stan, J. T., Gay, T. E., Rosier, C., and Wu, T. (2016). Alteration of soil chitinolytic bacterial and ammonia oxidizing archaeal community diversity by rainwater redistribution in an epiphyte-laden Quercus virginiana canopy. Soil Biol. Biochem. 100, 33–41. doi: 10.1016/j.soilbio.2016.05.016
Moore, T. R. (1980). The nutrient status of subarctic woodland soils. Arctic Alp. Res. 12, 147–160. doi: 10.2307/1550512
Moreno, G., Gallardo, J. F., and Bussotti, F. (2001). Canopy modification of atmospheric deposition in oligotrophic Quercus pyrenaica forests of an unpolluted region (central-western Spain). For. Ecol. Manage. 149, 47–60. doi: 10.1016/S0378-1127(00)00544-2
Mosello, R., Brizzio, M. C., Kotzias, D., Marchetto, A., Rembges, D., and Tartari, G. (2002). The chemistry of atmospheric deposition in Italy in the framework of the national programme for forest ecosystems control (CONECOFOR). J. Limnol. 61, 77–92. doi: 10.4081/jlimnol.2002.s1.77
Negi, G. C. S., Rikhari, H. C., and Garkoti, S. C. (1998). The hydrology of three high-altitude forests in Central Himalaya. India: a reconnaissance study. Hydrol. Process. 12, 343–350. doi: 10.1002/(SICI)1099-1085(199802)12:2<343::AID-HYP578>3.0.CO;2-L
Ovington, J. D. (1954). A comparison of rainfall in different woodlands. Forestry 27, 41–53. doi: 10.1093/forestry/27.1.41
Proctor, M. C. F., Oliver, M. J., Wood, A. J., Alpert, P., Stark, L. R., Cleavitt, N. L., et al. (2007). Desiccation-tolerance in bryophytes: a review. Bryologist 110, 595–621. doi: 10.1093/jxb/erw458
Pryet, A., Domínguez, C., Tomai, P. F., Chaumont, C., d’Ozouville, N., Villacís, M., et al. (2012). Quantification of cloud water interception along the windward slope of Santa Cruz Island, Galapagos (Ecuador). Agric. For. Meteorol. 161, 94–106. doi: 10.1016/j.agrformet.2012.03.018
Rodrigo, A., Avila, A., and Rodá, F. (2003). The chemistry of precipitation, throughfall and stemflow in two holm oak (Quercus ilex L.) forests under a contrasted pollution environment in NE Spain. Science of the Total Environment 305, 195–205. doi: 10.1016/S0048-9697(02)00470-9
Rosier, C. L., Levia, D. F., Van Stan, J. T., Aufdenkampe, A., and Kan, J. (2016). Seasonal dynamics of the soil microbial community structure within the proximal area of tree boles: Possible influence of stemflow. Eur. J. Soil Biol. 73, 108–118. doi: 10.1016/j.ejsobi.2016.02.003
Rosier, C. L., Van Stan, J. T., Moore, L. D., Schrom, J. O. S., Wu, T., Reichard, J., et al. (2015). Forest canopy structural controls over throughfall affect soil microbial community structure in an epiphyte-laden maritime oak stand. Ecohydrology 8, 1459–1470. doi: 10.1002/eco.1595
Roth-Nebelsick, A., Ebner, M., Miranda, T., Gottschalk, V., Voigt, D., Gorb, S., et al. (2012). Leaf surface structures enable the endemic Namib desert grass Stipagrostis sabulicola to irrigate itself with fog water. J. R. Soc. Interface 9, 1965–1974. doi: 10.1098/rsif.2011.0847
Safeeq, M., and Fares, A. (2012). Interception losses in three non-native Hawaiian forest stands. Hydrol. Process. 28, 237–254. doi: 10.1002/hyp.9557
Santa Regina, I., and Tarazona, T. (2000). Nutrient return to the soil through litterfall and throughfall under beech and pine stands of Sierra de la Demanda. Spain. Arid Soil Res. Rehabil. 14, 239–252. doi: 10.1080/089030600406653
Savenije, H. H. G. (2004). The importance of interception and why we should delete the term evapotranspiration from our vocabulary. Hydrol. Process. 18, 1507–1511. doi: 10.1002/hyp.5563
Schroth, G., Elias, M. E. A., Uguen, K., Seixas, R., and Zech, W. (2001). Nutrient fluxes in rainfall, throughfall and stemflow in tree-based land use systems and spontaneous tree vegetation of central Amazonia. Agric. Ecosyst. Environ. 87, 37–49. doi: 10.1016/S0167-8809(00)00294-2
Schwärzel, K., Ebermann, S., and Schalling, N. (2012). Evidence of double-funneling effect of beech trees by visualization of flow pathways using dye tracer. J. Hydrol. 47, 184–192. doi: 10.1016/j.jhydrol.2012.08.048
Staelens, J. (2006). Spatio-Temporal Patterns of Throughfall Water and Ion Deposition Under a Dominant Beech Tree (Fagus sylvatica l.) in Relationship to Canopy Structure. Ph.D. thesis, Ghent University, Ghent.
Talkner, U., Krämer, I., Hölscher, D., and Beese, F. O. (2010). Deposition and canopy exchange processes in central-German beech forests differing in tree species diversity. Plant Soil 336, 405–420. doi: 10.1007/s11104-010-0491-2
Toba, T., and Ohta, T. (2005). An observational study of the factors that influence interception loss in boreal and temperate forests. J. Hydrol. 313, 208–220. doi: 10.1016/j.jhydrol.2005.03.003
Tobón, C., Sevink, J., and Verstraten, J. M. (2004). Solute fluxes in throughfall and stemflow in four forest ecosystems in northwest Amazonia. Biogeochemistry 70, 1–25. doi: 10.1023/B:BIOG.0000049334.10381.f8
Van Stan, J. T., Norman, Z., Meghoo, A., Friesen, J., Hildebrandt, A., Côté, J.-F., et al. (2017a). Edge-to-stem variability in wet-canopy evaporation from an urban tree row. Boundary Layer Meteorol. 165, 295–310. doi: 10.1007/s10546-017-0277-7
Van Stan, J. T., and Pypker, T. G. (2015). A review and evaluation of forest canopy epiphyte roles in the partitioning and chemical alteration of precipitation. Sci. Total Environ. 536, 813–824. doi: 10.1016/j.scitotenv.2015.07.134
Van Stan, J. T., Wagner, S., Guillemette, F., Whitetree, A., Lewis, J., Silva, L., et al. (2017b). Temporal dynamics in the concentration, flux, and optical properties of tree-derived dissolved organic matter (tree-DOM) in an epiphyte-laden oak-cedar forest. J. Geophys. Res. Biogeosci. 122, 2982–2997. doi: 10.1002/2017JG004111
Ward, D. (2015). “Desert biome,” in Oxford Bibliographies in Ecology, ed. D. Gibson (New York, NY: Oxford University Press).
Whelan, M. J., and Anderson, J. M. (1996). Modeling spatial patterns of throughfall and interception loss in a Norway spruce (Picea abies) plantation at the plot scale. J. Hydrol. 186, 335–354. doi: 10.1016/S0022-1694(96)03020-X
White, I., Falkland, T., Metutera, T., Metai, E., Overmars, M., Perez, P., et al. (2007). Climatic and human influences on groundwater in low atolls. Vadose Zone J. 6, 581–590. doi: 10.2136/vzj2006.0092
Yuan, B.-C., Li, Z.-Z., Liu, H., Gao, M., and Zhang, Y.-Y. (2007). Microbial biomass and activity in salt affected soils under arid conditions. Appl. Soil Ecol. 35, 319–328. doi: 10.1016/j.apsoil.2006.07.004
Keywords: ecohydrology, stemflow, forest ecology, plant–soil interactions, arid environment
Citation: Van Stan JT and Gordon DA (2018) Mini-Review: Stemflow as a Resource Limitation to Near-Stem Soils. Front. Plant Sci. 9:248. doi: 10.3389/fpls.2018.00248
Received: 18 September 2017; Accepted: 12 February 2018;
Published: 27 February 2018.
Edited by:
James Stangoulis, Flinders University, AustraliaReviewed by:
Jeff Wolt, Iowa State University, United StatesCopyright © 2018 Van Stan and Gordon. This is an open-access article distributed under the terms of the Creative Commons Attribution License (CC BY). The use, distribution or reproduction in other forums is permitted, provided the original author(s) and the copyright owner are credited and that the original publication in this journal is cited, in accordance with accepted academic practice. No use, distribution or reproduction is permitted which does not comply with these terms.
*Correspondence: John T. Van Stan, anZhbnN0YW5AZ2VvcmdpYXNvdXRoZXJuLmVkdQ==
Disclaimer: All claims expressed in this article are solely those of the authors and do not necessarily represent those of their affiliated organizations, or those of the publisher, the editors and the reviewers. Any product that may be evaluated in this article or claim that may be made by its manufacturer is not guaranteed or endorsed by the publisher.
Research integrity at Frontiers
Learn more about the work of our research integrity team to safeguard the quality of each article we publish.