- State Key Laboratory of Crop Biology and College of Agronomy, Shandong Agricultural University, Taian, China
Maize is one of the most important crops globally that provides food, feed, and bioenergy. However, shading stress threatens maize production. In this study, we investigated the effects of shading on photosynthate accumulation and distribution of summer maize in the field. Zhengdan958 (ZD958) and Denghai 605 (DH605) were used as experimental materials in a field experiment running from 2013 to 2015. Shading treatments were applied over different growth stages: from the tassel stage (VT) to physiological maturity (R6) (S1), from the six-leaf stage (V6) to VT (S2), and from emergence stage (VE) to R6 (S3). The effects of shading on plant photosynthesis, photosynthate accumulation and distribution, and yield were evaluated in comparison to ambient sunlight. Shading significantly decreased the leaf area, SPAD value, net photosynthetic rate, dry matter accumulation, and grain yield. During the 3-year experimental period, grain yields of ZD958 and DH605 were reduced by 83.4%, 34.2%, 53.1% and 79.3%, 24.2%, 57.6% as compared to the CK by treatments S3, S2, and S1, respectively. 13CO2 stable isotope tracing revealed that shading differentially affected the photosynthate transfer rate in different stages; photosynthates were transferred from top to bottom plant parts, in the order control > S2 > S1 > S3. We conclude that shading clearly disrupted photosynthate metabolism, and reduced the photosynthate accumulation in the grain, resulting in a yield reduction.
Introduction
Light provides energy for the generation of plant assimilatory power and acts as a signal for photomorphogenesis (Kumar et al., 2016). Sufficient light is important for high and steady yields, especially in maize (Zea mays L.), which is a typical C4 plant. The high productivity of C4 plants is closely related to the differentiation and development of parenchyma, thus, C4 plants are very sensitive to light restriction (Ubierna et al., 2011; Chandra and Howard, 2014). CO2 has a high anti-diffusion effect in crops only under high light intensity, thereby enhancing CO2 fixation. Changes in light duration and intensity, both of which affect the structure and function of leaf mesophyll cells and vascular bundle sheath cells, also impact the synergistic effect of the C4 and C3 cycles (Evans et al., 2008; Tazoe et al., 2008; Kramer and Evans, 2011). In recent years, the low efficiency of C4 photosynthesis under low light conditions has been a topic of concern (Ubierna et al., 2011; Bellasio and Griffiths, 2014a).
In the North China Plain, under the influence of climatic and environmental conditions (e.g., wet weather, plant density, and altitude), summer maize is often subjected to low-light stress or self-shading in the later stages of growth. Increases in atmospheric aerosol content reduced the sunshine duration and solar radiation in the past 50 years, and the average annual temperature and frequency of extreme weather events are rising currently (Ren et al., 2005). There are more problems in maize production with the changes in climate, including shading, lodging and so on, which have a negative effect on national food security. For example, in the Huanghuaihai region, the rainy weather frequently occurs during the summer maize growing season (June–September), and can result in a 3–6% decrease in China’s total maize grain yield (Cui et al., 2013a). Previous studies indicated that shading decreased carbon fixation and canopy net photosynthetic rate (Hashemi-Dezfouli and Herbert, 1992; Kromdijk et al., 2008; Bellasio and Griffiths, 2014b), and the changes in photosynthetic organs occurred, such as leaf tissue morphology (Kloss and Schütze, 2015), stomatal movement (Kaushal et al., 2015), functional leaf chloroplast morphology and ultrastructure (Sheue et al., 2015), and chlorophyll content. In short, the low-light stress has negative effects on photosynthesis and grain yield of summer maize.
Crop yield is mainly determined by dry matter production and accumulation, which is also limited by the harvest index (Hu, 1995). Dry matter accumulation after anthesis in high-yield maize accounts for more than 60% of the total dry matter mass, and the harvest index is more than 0.53 (Lian et al., 2003). Dry matter formation and distribution in vegetative organs such as stem, leaf, and sheath ultimately determine maize grain yield (Huang Z.H. et al., 2007). The carbon transfer rate depends on the positions of the stem and leaves, which act as carbon sources (Wei et al., 2009). The distribution of photosynthate in the organs varies with the translocation of the growth center. Photosynthates are distributed mainly in the leaves before the nine-leaf vegetative stage (V9), and in stems and leaves at later stages. Assimilates accumulated before reproductive stage R3 (silk stage) contributes the most to grain formation. The carbon in the grain is derived from both photosynthesis and redistribution of carbon from vegetative organs. As photosynthesis gradually weakens after R3, dry matter in the grain is mainly translocated from the stem and leaves in this stage (Liu W. et al., 2011). Promoting the redistribution of carbon during later growth stages allows coordinating carbon metabolism and nitrogen metabolism, which requires carbon skeletons for N sequestration, so as to achieve higher crop yield and quality (Zhang et al., 2016). Based on previous research (Ren et al., 2005; Cui et al., 2013a, 2015), shading decreased dry matter accumulation and grain yields of summer maize. Thus, we hypothesized that under different shading conditions, the distribution of the 13C-photosynthate to the grains was different and shading after anthesis had greatest effects on photosynthate distribution and translocation compared with shading occured before anthesis. We tested this hypothesis by designing a field experiment in which shading occurred at different growth stages: S1, tassel stage (VT) to physiological maturity (R6); S2, six-leaf stage (V6) to VT; and S3, emergence to R6 using 13CO2 stable isotope tracer to explore the effect of shading in different period on 13C-photosynthate accumulation and distribution.
Materials and Methods
Experimental Site
The field experiment was conducted at the Experimental Farm of Shandong Agricultural University (36°09′N, 117°09′E, 158 m a.s.l.) and the State Key Laboratory of Crop Biology, China in the summer maize growing seasons of 2013–2015. The region is characterized by a temperate continental monsoon climate with an average annual temperature of about 12.9°C. The mean total precipitation that occurred during the summer maize growth periods in 2014 and 2015 was 240.0 and 282.6 mm, respectively. The soil type was brown soil, with a pH of 7.12 (Cambisol; FAO, 2003). The contents of organic matter, total N, total P, available N, rapidly available P, and rapidly available K in the 0–20 cm soil layer were 9.34 g kg-1, 0.76 g kg-1, 0.88 g kg-1, 80.61 mg kg-1, 37.19 mg kg-1, and 84.23 mg kg-1, respectively.
Experimental Materials and Design
After harvesting of the winter wheat, two summer maize hybrids, Zhengdan 958 (ZD958) and Denghai 605 (DH605), which are the most popular varieties in China, were used as experimental materials. Maize was sown on June 15 in both years at a plant density of 67,500 plants per hectare. Four treatments were arranged in a split-plot randomized complete-block design with three replicates: ambient sunlight was used as a control (CK) and shading (40% of ambient light intensity) was applied during the following three growth periods: S1, tassel stage (VT) to physiological maturity (R6); S2, six-leaf stage (V6) to VT; and S3, emergence to R6. Each experimental plot was 27 m2 (3 × 9 m) in size and consisted of five rows of maize spaced 0.6 m apart. Shade cloth (Hongda Shade Cloth Company, Shouguang City, China) and scaffold formed a shed. A distance of 2 m between the shade cloth and the top of the maize canopy was maintained to keep the microclimate under the cloth consistent with that of the CK.
Fertilizers were applied at 240 kg N ha-1, 120 kg P2O5 ha-1, and 200 kg K2O ha-1 as urea (46% N), calcium dihydrogen phosphate (17% P2O5), and muriate of potash (60% K2O). Nitrogen fertilizer was sidedressed at V6 and 12-leaf stage (V12) at a ratio of 2:3, while P and K fertilizers were sidedressed at V6. Disease, weeds, and pests were well controlled in each treatment. Atrazine and acetochlor were surface applied before maize germination to control weeds, and phoxim was applied to control corn borer at V12.
Sampling and Measurement
Field Microclimate
Irradiance was measured with a CI-110 plant canopy digital image analyzer (CID Company, Camas, WA, United States) placed 30 cm above the canopy. Canopy CO2 concentration and relative humidity were measured with a GXH-305 portable infrared CO2 instrument (Beijing Analytical Instrument Company, Beijing, China) and canopy air temperature with a thermometer at mid-plant height before VT and at ear height thereafter. Wind speed was determined with an AR816 anemometer (Huier Analytical Instrument Company, Hangzhou, China). Soil temperatures were determined with a geothermometer in the upper 0–5 cm of the soil (Ren et al., 2005; Cui et al., 2015). All parameters were measured at the center of each plot daily at 11:00 am for 7 days at VT. Means of three replications were calculated (Table 9).
Net Photosynthetic Rate (Pn)
Photosynthetic rates in ear leaves were measured at the middle of the uppermost and fully expanded leaves between 10:00 and 12:00 at V6, V12, VT, silk stage (R3), and R6 using a portable infrared gas analyzer (CIRAS II; PP Systems, Hansatech, United Kingdom). Five plants per treatment were randomly selected for measurements. Measurement conditions were kept consistent, the chamber was equipped with a red/blue LED light source. The PAR of CK was set at 1600 μmol m-2 s-1. The PAR of S was set at 500 μmol m-2 s-1.
Leaf Area Index (LAI)
Leaf length (L) and maximum leaf width (W) were measured in 15 representative plants per plot at V6, V12, VT, R3, and R6, and leaf area and LAI were calculated according to the method of Montgomery (1911).
Chlorophyll SPAD Value
The chlorophyll SPAD value was measured at V6, V12, VT, R3, and R6 in 10 randomly selected plants per treatment using a portable chlorophyll meter (SPAD-502, Soil-plant Analysis Development Section, Minolta Camera Co., Osaka, Japan).
Dry Matter Accumulation
Five representative plants were collected at V6, V12, VT, R3, and R6, and separated into leaves and stems (including stem, sheath, tassel, and ear-stalk) for V6, V12, and VT samples, and into leaves, stems, cob, and grain for R3 and R6 samples. The samples were oven-dried to constant weight at 80°C in a force-draft oven (DHG-9420A; Bilon Instruments, Shanghai, China) and then weighed.
13C-Photosynthate Accumulation and Distribution
Ten representative plants in each plot were selected at V12. And the ear leaf of each selected plant was encased in a 0.1-mm-thick Mylar plastic bag, which permits sunlight into the bag at levels up to 95% of natural intensity. Bags were sealed at the base with Sellotape and injected with 50 mL 13CO2. After photosynthesis was allowed to proceed for 60 min, the 13CO2 in each bag was extracted through a KOH washer to absorb the remaining 13CO2, and the plastic bag was removed. This experiment was conducted on clear days between 9:00 AM and 11:00 AM.
The ear leaf and the leaf above and below this leaf were defined as the middle leaf, the leaves above the middle leaf were defined as the top leaf, and the leaves below the middle leaf were defined as the bottom leaf; the ear internode and the internodes immediately above and below were defined as the middle stem, the part above the middle stem was defined as top stem, and the part below the middle stem was defined as bottom stem.
Three plants of each treatment labeled with13C were harvested close to the ground at VT and R6 and were separated into top leaf, middle leaf, bottom leaf, top stem, middle stem, and bottom stem at VT, and into top leaf, middle leaf, bottom leaf, top stem, middle stem, and bottom stem, cob, and grain at R6. The samples were placed in paper bags, deactivated at 105°C for 30 min, dried to constant weight at 80°C, and weighed to record dry matter (g plant-1). All samples were ground into powder and passed through a 200-mesh sieve. 13C enrichment in 4-mg plant samples was determined by using an isotope 100 mass spectrometer (Isoprime, Manchester, United Kingdom), and the 13C allocation rate was calculated using the following equations (Wei et al., 2009):
13C abundance :
RPBD (Carbon isotope ratio) = 0.0112372
Carbon content of each organ: Ci = total organ mass (g) × total carbon content (%) 13C (mg) into each component:
where Ci is the carbon content (g) contained in each component; nl is not labeled.
Net 13C assimilation by maize plants at the end of labeling was calculated by summing the 13C in each component. The percentage distribution of 13C into each component was calculated as:
Grain Yield, Yield Components, and Harvest Index
Thirty ears from the middle three rows of each plot were harvested at R6 using a continuous sampling method and were used to determine yield and yield components (standard moisture content is 14%). The harvest index was calculated by dividing the grain weight (standard moisture content is 14%) by the aboveground dry matter weight at R6.
Statistical Analysis
The data were subjected to three-way analysis of variance (ANOVA). Growing season, blocks, and block interactions were included as random effects. Shading treatment and hybrids were included as fixed effects. In case of significant treatment effects, comparison of means was performed by means of LSD at a significance level of 0.05. LSD was used to compare adjacent means arranged in order of magnitude. ANOVA and the LSD test were conducted using the SPSS17.0 software program (Ver. 17.0, SPSS, Chicago, IL, United States). Figures were produced with Sigma Plot 12.5.
Results
Grain Yield, Yield Components, and Harvest Index
Grain yield, yield components, and harvest index for the two hybrids in 2013 to 2015 are shown in Table 1. There was no significant year × hybrid × treatment interaction effect on grain yield. Grain yields decreased significantly with respect to the CK after shading. In 2015, the grain yields of ZD958 and DH605 in S3, S2, and S1 were 83.2%, 41.4%, and 47.8%, and 74.2%, 33.4%, and 48.6% significantly lower than that of CK, respectively. Thus, the grain yield in S3 was the lowest, and that in S2 the highest. Shading significantly affected yield components: grains per ear and ear number of the two hybrids were reduced under shading as compared to CK, resulting in lower yield. In addition, the harvest indexes of the two hybrids were significantly decreased in S3 and S1 under shading. There were no interactions among year, hybrid and treatment on grain yield and its components. Three-year results are consistent.
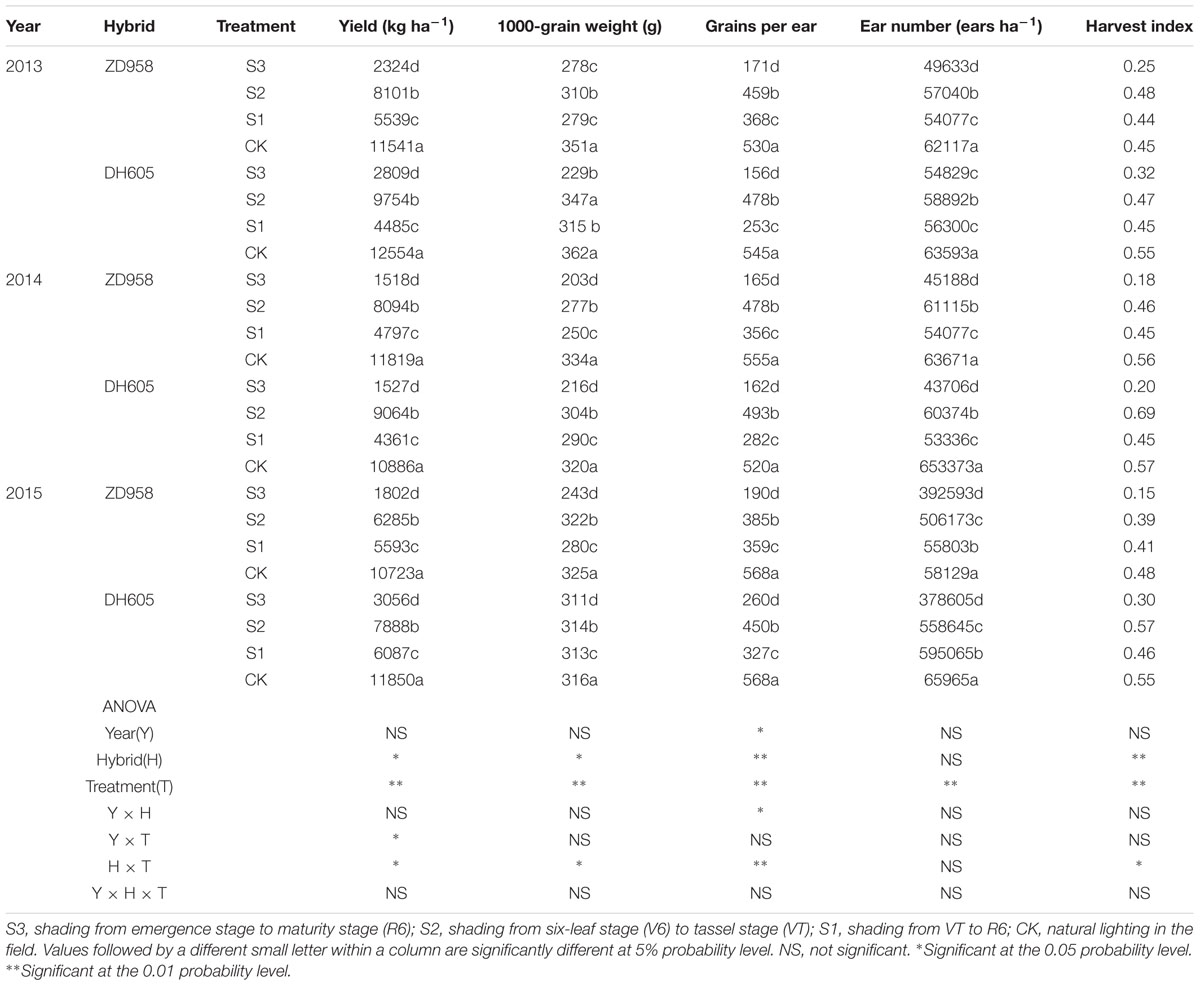
TABLE 1. Grain yield and yield components of summer maize under different light treatments from 2013 to 2015.
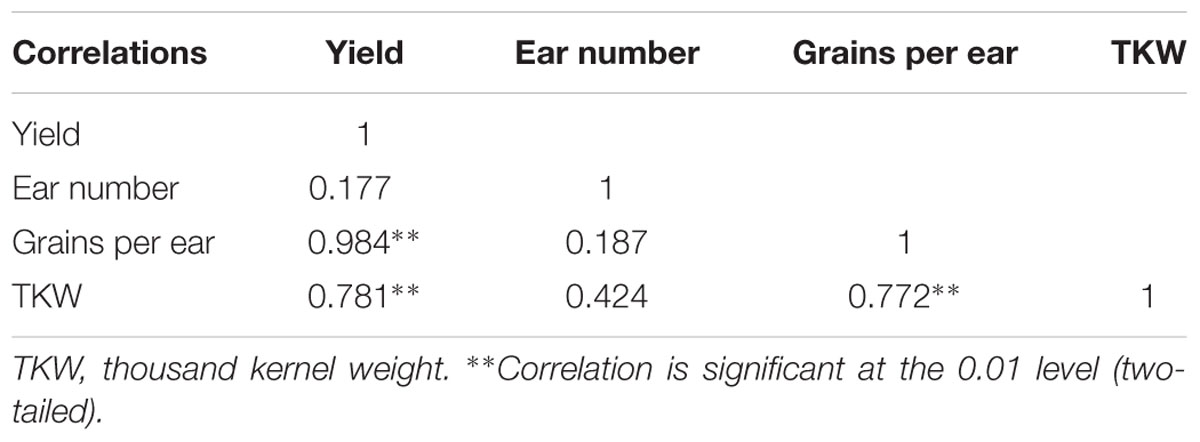
TABLE 2. Correlation of yield, ear number, grains per ear and thousand-kernel weight from 2013 to 2015.
Net Photosynthetic Rate (Pn)
The Pn significantly changed after shading (Table 3). For all shading treatments, the Pn was lower than that of CK at the corresponding growth stages, and the greatest reduction was observed in S3. In 2015, the Pn of ZD958 and DH605 in S3, S2, and S1 was 59.2%, 31.7%, and 48.8%, and 46.7%, 14.0%, and 37.9% significantly lower than that of CK in R3, respectively. Thus, the largest decrease in Pn was observed in S3, followed by S1 and S2. Year and treatment had significant effect on Pn and there were no interactions among year, hybrid and treatment. The trend in 2014 was in accordance with that in 2015.
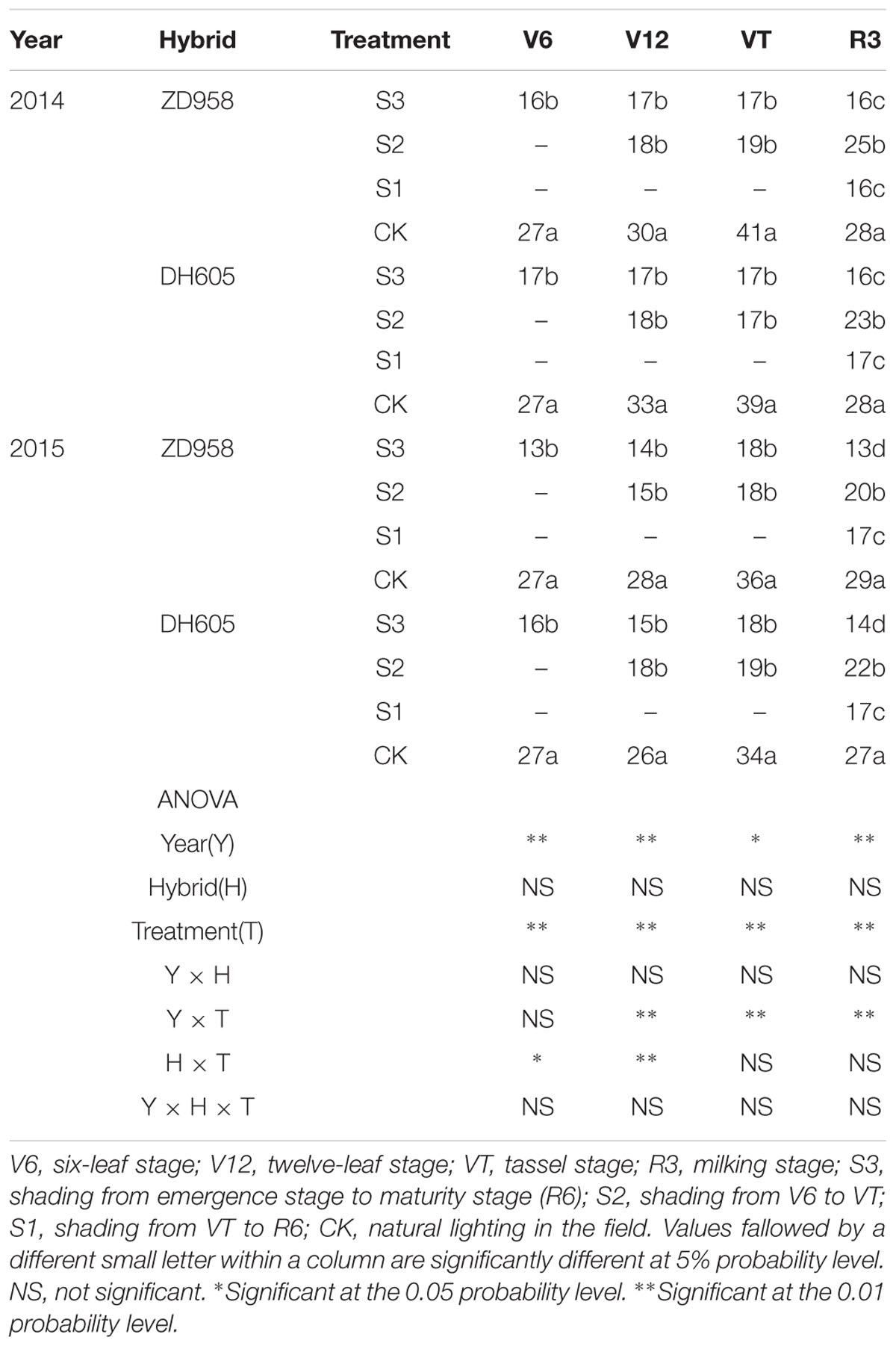
TABLE 3. Net photosynthetic rate in functional leaf of summer maize under different light treatments in 2014 and 2015 (μmol m-2 s-1).
Leaf Area Index (LAI)
The LAI of the two hybrids showed a single-peak curve, and the LAI under shading was lower than that in CK in the same period (Table 4). In 2015, the LAI of ZD958 in S3 was 23.9%, 37.5%, 25.5%, 34.7%, and 57.1% significantly lower than that of CK at each growth stage (V6, V12, VT, R3, and R6), respectively, and that of DH605 was 34.9%, 44.1%, 33.9%, 38.6%, and 36.3% significantly lower than that of CK, respectively. The LAI of ZD958 in S2 was 15.2%, 20.1%, 25.8%, and 9.0% lower than that of CK at each growth stage, respectively, and that of DH605 was 26.6%, 11.5%, 13.9%, and 6.8% lower than that of CK, respectively. Overall, the growth rate slowed down in S2 after the end of shading. The LAI of ZD958 and DH605 in S1 was 14.4%, 15.9%, and 12.1%, 11.1% lower than that of CK, respectively. The greatest LAI reduction occurred at S3. Year, hybrid (except at V6) and treatment had significant effect on LAI. The trend in 2014 was in accordance with that in 2015.
Chlorophyll SPAD Values
The different shading treatments significantly decreased the chlorophyll SPAD values with respect to the CK, with the most significant reduction noted in S3 (Table 5). Taking the results of 2015 as an example, the SPAD of ZD958 in S3 was 5.1%, 18.0%, 22.1%, 37.8%, and 43.7% significantly lower than that of CK at each growth stage, respectively, and that of DH605 was 10.1%, 18.2%, 20.9%, 38.3%, and 48.5% significantly lower than that of CK, respectively. The SPAD of ZD958 in S2 was 9.2%, 15.2%, 8.7%, and 32.5% significantly lower than that of CK at each growth stage, respectively, and that of DH605 was 8.3%, 13.7%, 14.7%, and 39.7% significantly lower than that of CK, respectively. This indicates that the growth rate slowed down in S2 after the end of shading. The SPAD of ZD958 and DH605 in S1 was 20.8%, 40.7% and 22.2%, 23.2% significantly lower than that of CK, respectively. The hybrid and treatment had significant effects on SPAD values. Results in 2014 showed a similar trend.
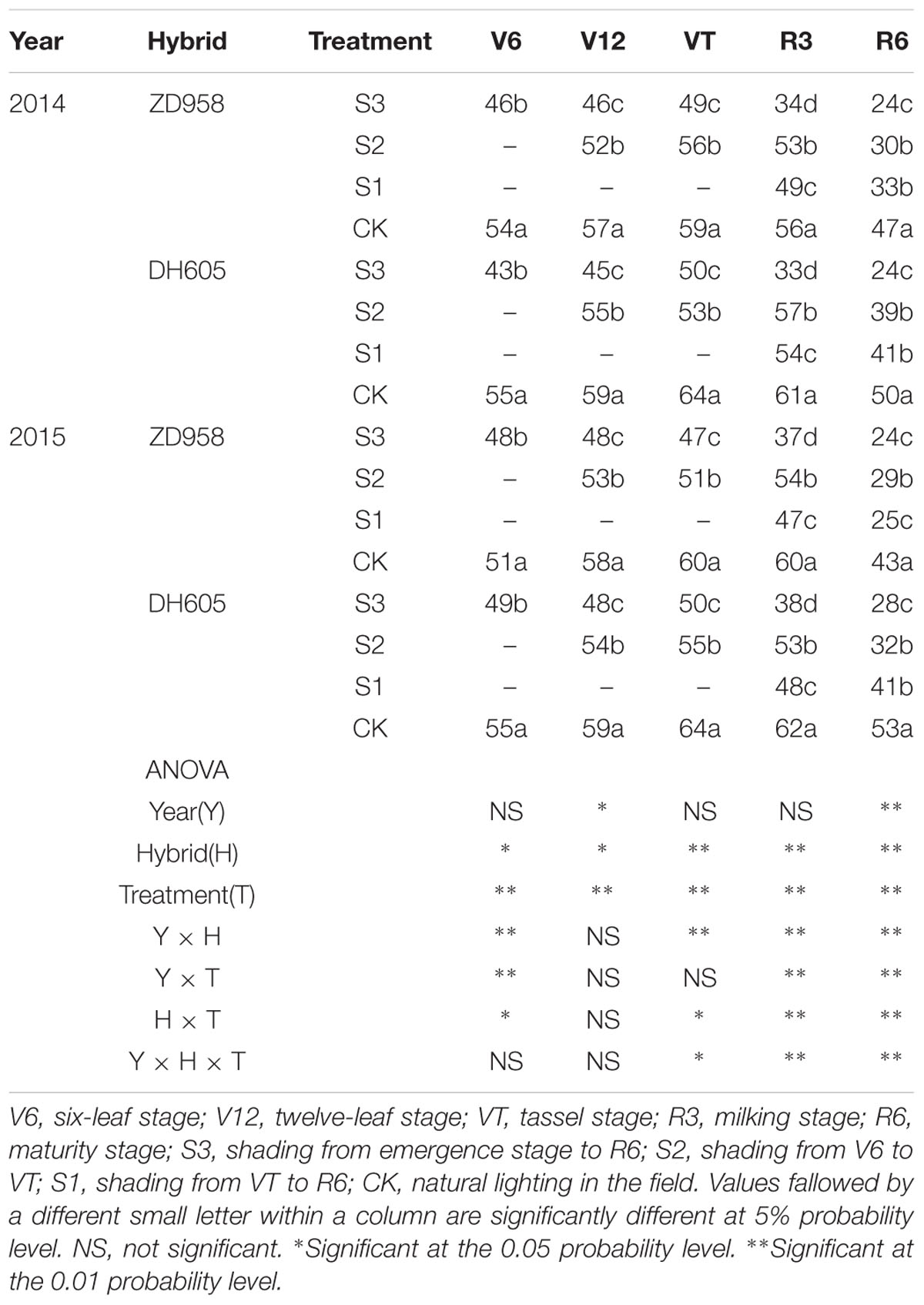
TABLE 5. SPAD value in functional leaves of summer maize under different light treatments in 2014 and 2015.
Dry Matter
Figure 1 shows that the dry matter accumulation in summer maize showed an “S-type” curve under the shading treatments at different stages. It increased slowly before VT, showed a steep increase at R3, and reached a maximum at R6. Taking the results of 2015 as an example, the dry matter accumulation of ZD958 in S3 significantly decreased by 56.5%, 52.9%, 55.9%, 56.3%, and 46.8% compared with CK at each growth stage, respectively, and that of DH605 significantly decreased by 60.7%, 43.7%, 57.0%, 48.6%, and 52.0% compared with CK, respectively. The dry matter accumulation of ZD958 in S2 significantly decreased by 42.4%, 50.9%, 31.5%, and 26.7% compared with CK at each growth stage, respectively, and that of DH605 significantly decreased by 32.8%, 37.2%, 26.6%, and 29.1% compared with CK, respectively. The dry matter accumulation of ZD958 and DH605 in S3 significantly decreased by 46.7% and 44.4%, and 35.7% and 45.8% compared with CK at each growth stage, respectively. Thus, shading not only reduces the amount of dry matter accumulated but also affects the proportion of dry matter accumulation and the distribution in the organs in different growth stages (Figure 2).
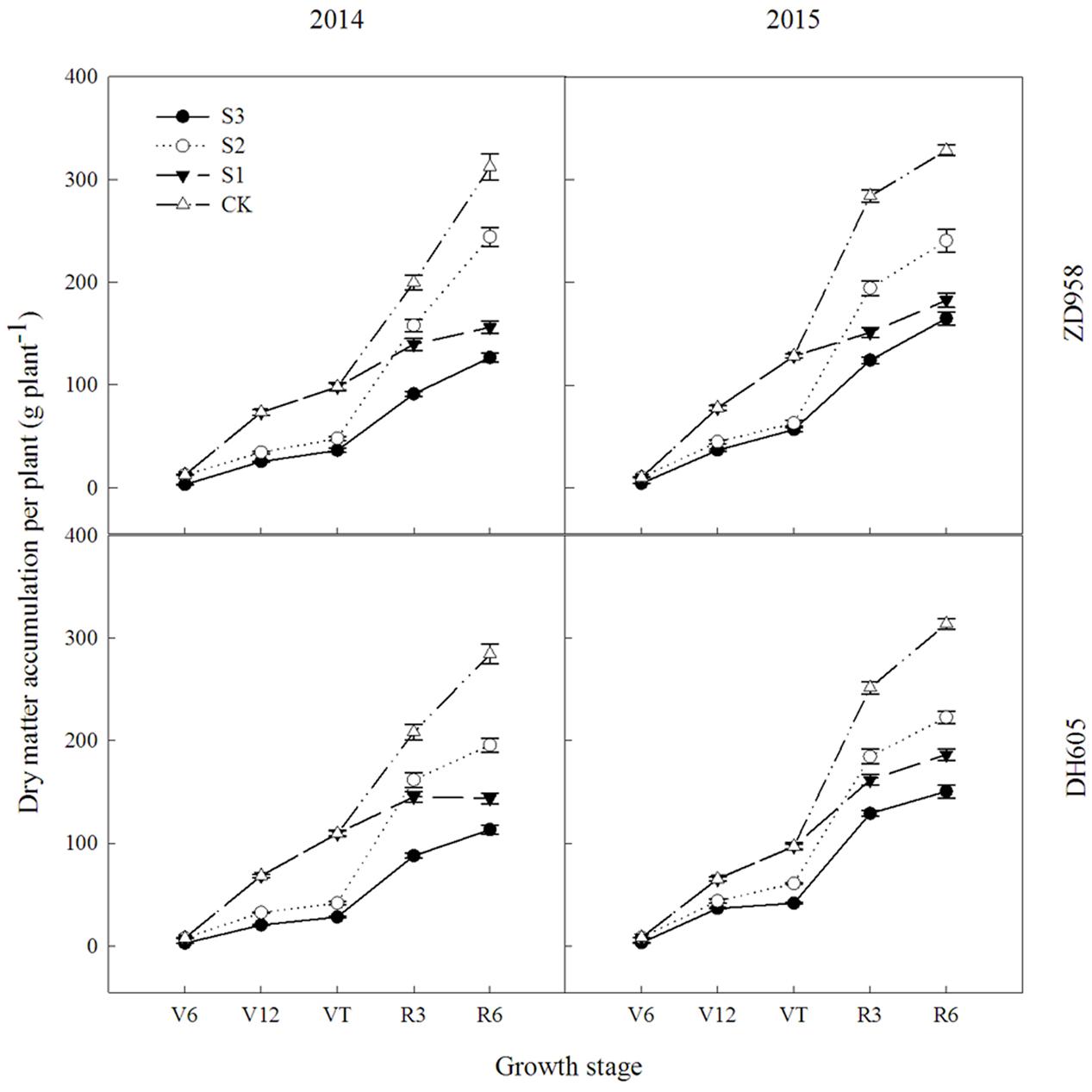
FIGURE 1. Dynamic changes in dry matter accumulation of summer maize under different treatments in 2014 and 2015 (g per plant). Means and standard errors based on three replicates are shown. S3, shading from emergence stage to maturity stage (R6); S2, shading from six-leaf stage (V6) to tassel stage (VT); S1, shading from VT to R6; CK, natural lighting in the field.
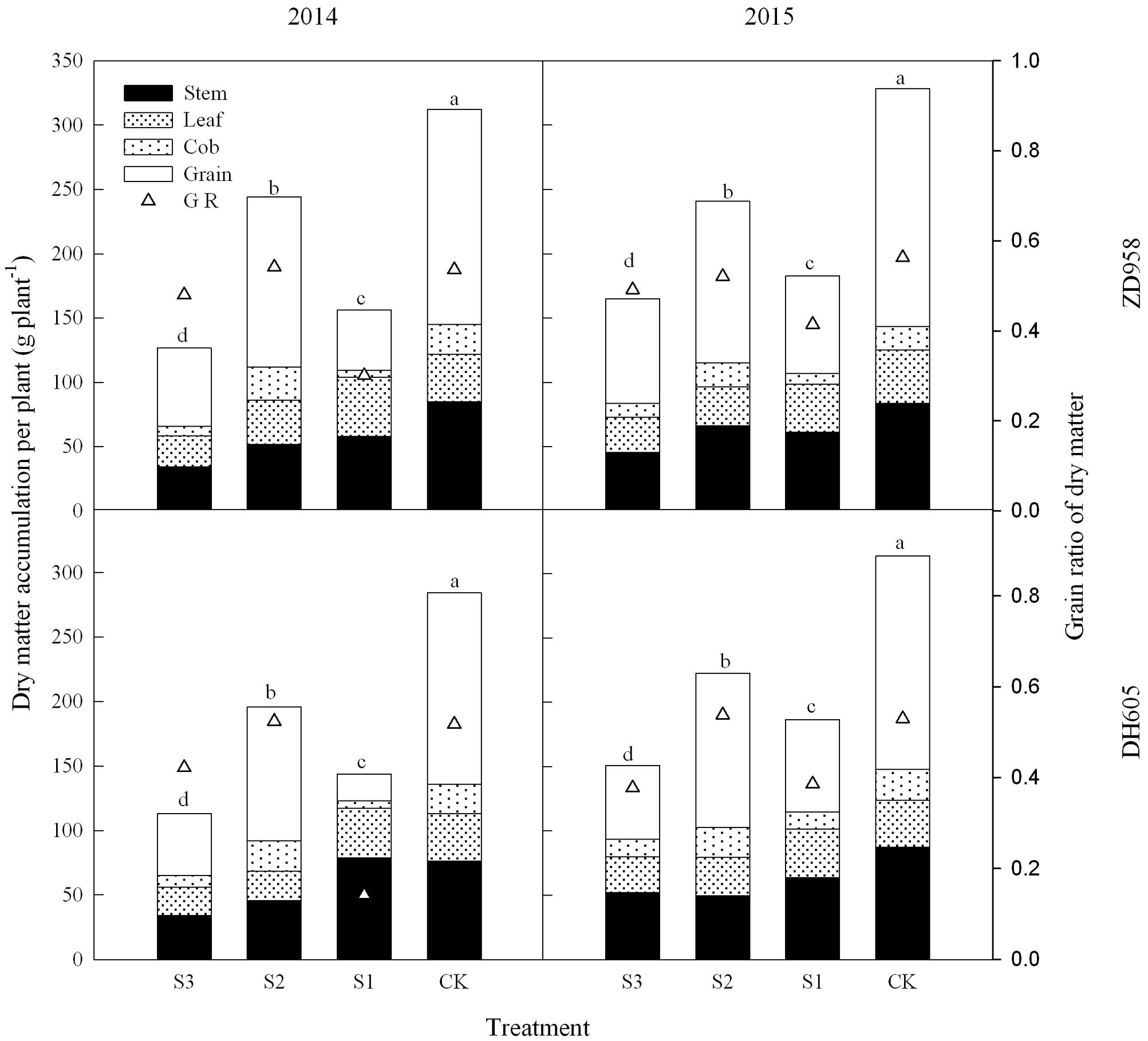
FIGURE 2. Histograms of dry matter accumulation and distribution of summer maize under different treatments at maturity stage (g per plant). The different small letters are significantly different at 5% probability level. S3, shading from emergence stage to maturity stage (R6); S2, shading from six-leaf stage (V6) to tassel stage (VT); S1, shading from VT to R6; CK, natural lighting in the field.
Carbon Distribution
The largest effect of shading on the plant carbon content was observed in S3, while the smallest effect was found in S2 (Table 6). The stem carbon contents of ZD958 and DH605 showed similar trends: bottom stem > middle stem > top stem. The carbon distribution rates for ZD958 in top stem, middle stem, and bottom stem were 5.4%, 12.9%, 21.7% in S3; 3.1%, 10.0%, 16.6% in S2; 4.3%, 9.0%, 18.4% in S1; and 3.2%, 7.8%, 14.3% in CK, and those for DH605 were 5.9%, 13.4%, 20.4% in S3; 2.8%, 9.1%, 14.7% in S2; 4.6%, 14.5%, 17.2% in S1 and 3.3%, 9.0%, 11.7% in CK. The leaf carbon contents varied along the stem. The leaf carbon contents of ZD958 in S3, S1, and CK showed the same trend: bottom leaf > middle leaf > top leaf. The distribution rates for ZD958 in top leaf, middle leaf, and bottom leaf were 4.6%, 7.0%, 8.4% in S3; 2.9%, 4.0%, 3.5% in S2; 4.5%, 6.2%, 9.5% in S1; and3.2%, 3.5%, 5.3% in CK, respectively. Those for DH605 were 7.0%, 7.6%, 5.5% in S3; 3.0%, 4.0%, 3.6% in S2; 7.1%, 7.0%, 6.8% in S1 and 3.7%, 4.3%, 3.9% in CK, respectively. Grain carbon content showed the same trend in both hybrids: CK > S2 > S1 > S3.
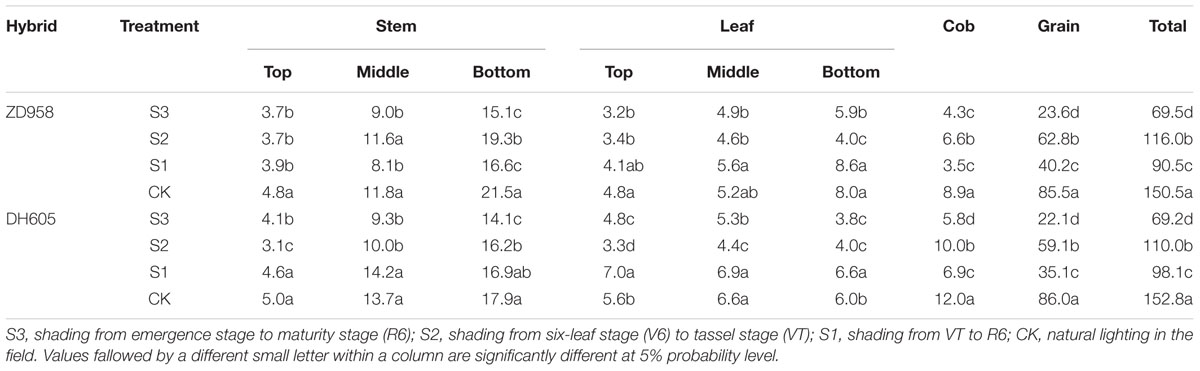
TABLE 6. Plant carbon distribution of summer maize under different light treatments at maturity stage (g per plant).
13C-Photosynthate Accumulation and Distribution
The 13C isotope was concentrated in the middle and bottom parts of the stem and leaves in the flowering period, and started to be transferred to the grain after anthesis (Table 7). The middle stem of ZD958 and DH605 showed the highest transfer rates in S3, S1, and CK, with 13.0%, 66.7%, 51.6%, and 9.4%, 50.0%, 51.4%, respectively. In the stem of ZD958 in S2, 13C-photosynthate increased as compared to the CK. The bottom leaves of DH605 showed the highest transfer rates in S3, S2, S1, and CK, of 31.0%, 33.3%, 15.0%, and 22.5%, respectively. The top leaves of ZD958 had the highest rates in S3 and S1, with rates of 5.9%, and 19.2%, while the bottom leaves of ZD958 had the highest rates in S2 and CK, with values of 34.4%, and 12.8%. The grain transfer rates of ZD958 and DH605 showed similar trends: CK > S2 > S1 > S3.
Discussion
Effects of Shading on Field Microclimate and Fertility
No significant difference on microclimatic indexes, except light intensity, was observed. The same results were reported by Du et al. (2011). Previous studies suggested that under shading, when the plant obtains less energy, fertility is significantly delayed, and the degree of retardation is related to the degree (Ubierna et al., 2011), duration (Chandra and Howard, 2014), and period of shading (Evans et al., 2008). According previous researches, the sensitivity of maize to shading is different significantly. In this study, the greatest impact of shading on the fertility process of summer maize was observed in treatment S3 resulting from shading prevent pollen from scattering (Table 10).
Effects of Shading on Photosynthetic Properties
Leaf photosynthetic performance and yield are closely related, and increasing the leaf photosynthetic performance is one of the key measures for obtaining high yields in summer maize (Liu et al., 2015; Ren et al., 2016). Theoretically, photosynthetic area, capacity, and time are important parameters determining photosynthetic performance. As leaves catch light energy, the sum of their sizes to a certain extent reflects the light interception and energy conversion capacity of the canopy (Li et al., 2010). The length of the functional period of maize leaves from flowering to silk ripening and the amount of dry matter accumulation after silking directly determine the yield (Tollenaar and Daynard, 1982; Sen et al., 2016). Previous studies have shown that under low-light stress, maize leaves lose chlorophyll and show early failure, resulting in a decrease in photosynthetic leaf area, and consequently, in yield loss (Jia et al., 2010; Cui et al., 2013b; Kong et al., 2012). Our results corroborated that the source such as photosynthetic leaf area and photosynthetic performance decreased under shading.
A previous study suggested that leaf chlorophyll content and photosynthetic rate in theory determine the potential grain yield of summer maize (Dong et al., 1997; Zhong et al., 2014). Higher rate and longer functional period of photosynthesis at the late growth stage benefit maize grain yield. In this study, it was found that after shading, the SPAD value and net photosynthetic rate decreased, photosynthetic function decayed rapidly, and dry matter quality and yield decreased. After the light was restored in S2, the photosynthetic function of the leaf was restored, but did not entirely remove the effects of shading, which remained significant, perhaps because shading might have damaged the leaf photoprotection mechanisms.
Effects of Shading on Dry Matter Accumulation and Distribution
Photosynthesis produces more than 90% of the plant dry matter. Especially, the dry matter distribution and transport characteristics in the late growth stage determines the final yield in summer maize (Huang Z.X. et al., 2007; Chen et al., 2008). The movement of photosynthates from different plant parts to the reproductive organs is determined by the sink strength of the latter (Wei et al., 2009). Sink activity and carbon demand by the sink are considered the main drivers of carbon allocation in plants (Roland et al., 2015). Shading reduces carbon uptake and impairs photosynthetic performance in maize plants (Cui et al., 2013a). Our results showed that dry matter accumulation was consistently and significantly reduced under the shading regimens, which was in accordance with previous observations (Cui et al., 2015). Shading impaired sink strength including decreasing grains per ear and delaying development of endosperm cell (Gao et al., 2017), which decreased kernel number per ear and 1000-kernel weight. Before flowering, shading affected the reproductive growth of the plant, and dry matter accumulation decreased. After flowering, upon restoration of light in S2, plant growth was rapidly restored and dry matter accumulation increased. Shading in S2 affected the dry matter accumulation before VT, resulting in decreases in source and total dry matter. Shading in S1 affected grain filling, weakened the sink, and decreased grain number and grain weight, eventually leading to decreases in total dry matter accumulation and yield.
Effects of Shading on 13C-Photosynthate Accumulation and Distribution
As dry matter accumulation and transportation are important factors determining maize yield, it is important to understand the contribution of dry matter from different parts to grain yield, to allow the development of improved summer maize varieties (Song and Tong, 2010). The dry matter accumulation pre-anthesis is mainly used for plant formation, such as the formation and growth of roots, stem, leaves, spikes, and other organs. The dry matter accumulated after anthesis is used mainly for grain formation; accordingly, high dry matter productivity after anthesis is one of the high-yield characteristics of summer maize (Liu et al., 2010; Zhang et al., 2011). Although the total 13C fixed was underestimated because the amount of 13C lost to the soil was not measured (Wei et al., 2009), assessing the amount of 13CO2 fixed in maize plants and translocated to each component provided an efficient way to assess the contribution of each part to the grain under shading stress. The 13C isotope tracer experiment showed that the distribution and transportation of photosynthate were different in each treatment. Shading significantly reduced the dry matter accumulation in each organ, and moreover, the proportion of dry matter allocated to the stem and leaves were increased, while that allocated to the grain was reduced. Photosynthates of top, middle, and bottom stem and leaves contributed to grain yield, which is consistent with the report of Li et al. (2012). In the early stages of maize grain sink formation, most of the 13C assimilates were distributed in the stalks, and then distributed to the leaves, and the distribution ratio in the stems was approximately 70% (Table 7). After flowering, 13C photosynthates in S3 were used for plant rather than grain formation, although the contribution to grain yield could not be ignored. After return to ambient light in S2, after anthesis, plant formation and grain enrichment took place simultaneously, and the transfer rates in stem, leaves, and other vegetative organs increased. However, dry matter accumulation in the stem, leaves, and “temporary” storage organs was low, and thus, grain could not get sufficient carbon from the vegetative organs and yield could not be restored to the normal level. Photosynthesis and thus, photosynthate, in S1 decreased after anthesis, and plants senesced prematurely. This had a negative effect on grain filling, reducing sink activity. The unbalanced source-to-sink ratio led to more 13C photosynthate in the stalk, thus decreasing yield (Table 8).
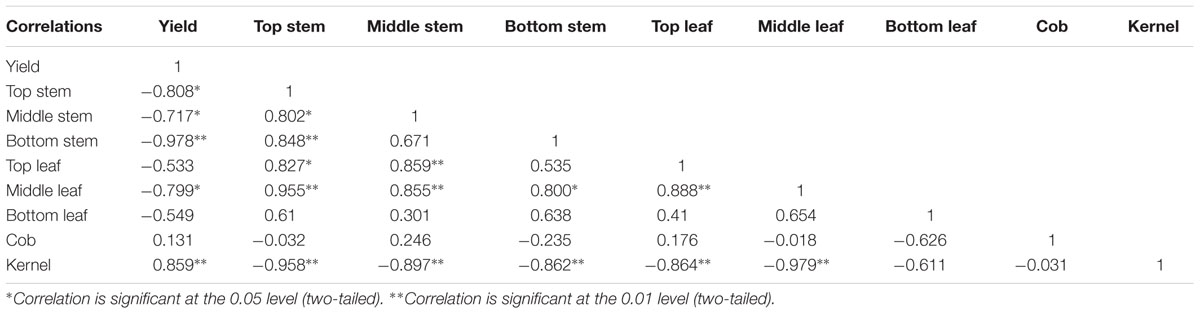
TABLE 8. Correlation of yield and distribution of 13C-assimilate from functional leaf of summer maize (%).
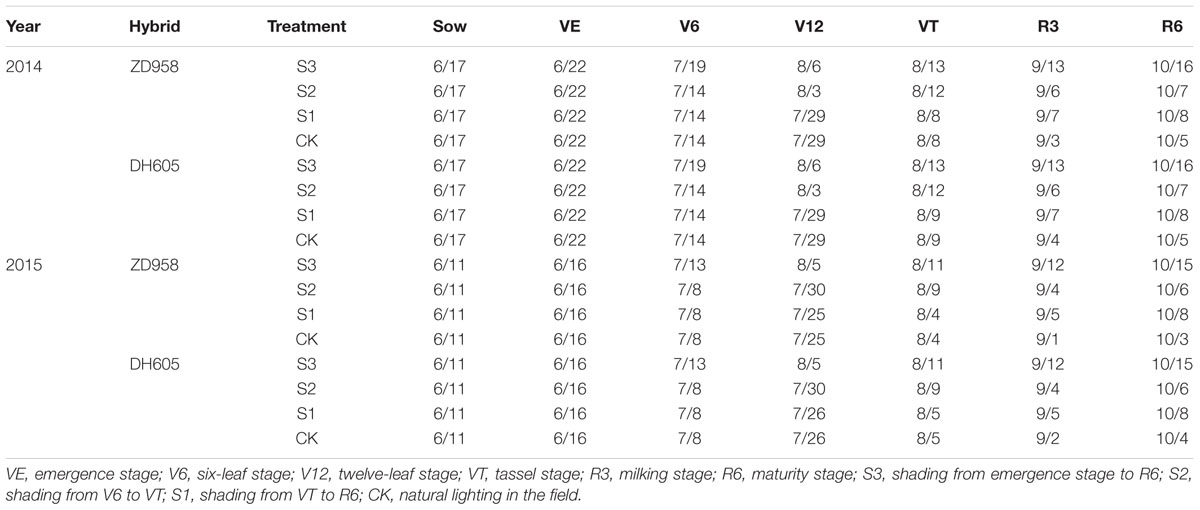
TABLE 10. Developmental progress of summer maize under different light treatments in 2014 and 2015 (M/D).
Effects of Shading on Grain Yield and Yield Components
Appropriate light intensity and photoperiod are important for high and stable yields (Du et al., 2011; Liu Z.F. et al., 2011). Weak light in the later stages of maize growth results in smaller endosperm cells and lower grain weight at maturity and thus, directly affects yield (Li and Cui, 1989; Setter and Flannigan, 1989). Cui et al. (2015) pointed out that female spikelet differentiation is very sensitive to light; spike differentiation was retarded under shading, which decreased grains per ear and yield. The current study showed that shading reduced the maize grain yield, in the order S3 > S1 > S2, which was consistent with our previous findings (Li et al., 2005; Cui et al., 2013b). The correlations between yield and these parameters (Table 2) revealed that increasing the grains per ear and thousand-kernel weight with constant ear number might be an effective way to obtain high yield under shading.
Conclusion
Shading significantly decreased the photosynthetic leaf area, SPAD value, net photosynthetic rate, and dry matter accumulation, while changing the distribution of dry matter in various organs and reducing the dry matter quality in the grain, thus lowering the grain yield. Therefore, this study suggests that the sowing date should be adjusted to avoid rainy weather in the late growing period, to ensure appropriate light conditions for the transport of photosynthates to safeguard yield. Reasonable fertilizer and water management should ensure that the photosynthates are efficiently transported to the grain. Thus, future research should focus on improving cultivation techniques, increasing photosynthetic efficiency, prolonging the photosynthetic function period, and promoting nutrient translocation to the grain under shading for harmonizing production and the environment.
Author Contributions
JG carried out the measurements, data analysis, and drafted the manuscript. JZ designed the experiment. BZ, SD, PL, BR, and JZ made substantial contributions to conception, and critically revised the manuscript.
Funding
This study was funded by National Natural Science Funds (31671629), National Modern Agricultural Technology and Industry System (CARS-02-20), Shandong “Double Tops” Program (SYL2017YSTD02), and State Key Research and Development Program “Food Production Ehhancement and Efficiency Innovation” Key Special Project (SQ2017YFNC050063).
Conflict of Interest Statement
The authors declare that the research was conducted in the absence of any commercial or financial relationships that could be construed as a potential conflict of interest.
The reviewer EN and handling Editor declared their shared affiliation.
Acknowledgment
The authors are grateful to the reviewers and editors for their constructive review and suggestions for this paper.
References
Bellasio, C., and Griffiths, H. (2014a). Acclimation to low light by C4 maize: implications for bundle sheath leakiness. Plant Cell Environ. 37, 1046–1058. doi: 10.1111/pce.12194
Bellasio, C., and Griffiths, H. (2014b). The operation of two decarboxylases, transamination, and partitioning of C4 metabolic processes between mesophyll and bundle sheath cells allows light capture to be balanced for the maize C4 pathway. Physiol. Plant. 164, 466–480. doi: 10.1104/pp.113.228221
Chandra, B., and Howard, G. (2014). Acclimation of C4 metabolism to low light in mature maize leaves could limit energetic losses during progressive shading in a crop canopy. J. Exp. Bot. 65, 3725–3736. doi: 10.1093/jxb/eru052
Chen, G. P., Yang, G. H., Zhao, M., Wang, L. C., Wang, Y. D., Xue, J. Q., et al. (2008). Studies on maize small area super-high yield trails and cultivation technique. J. Maize Sci. 16, 1–4.
Cui, H. Y., James, J. C., Jin, L. B., and Zhang, J. W. (2015). Effects of shading on spike differentiation and grain yield formation of summer maize in the field. Int. J. Biometeorol. 09, 1189–1200. doi: 10.1007/s00484-014-0930-5
Cui, H. Y., Jin, L. B., Li, B., Dong, S. T., Liu, P., Zhao, B., et al. (2013a). Effects of shading on dry matter accumulation and nutrient absorption of summer maize. Chin. J. Appl. Ecol. 11, 3099–3105.
Cui, H. Y., Jin, L. B., Li, B., Zhao, B., Dong, S. T., Liu, P., et al. (2013b). Effects of shading on photosynthetic characteristics and xanthophyll cycle of summer maize in the field. Acta Agron. Sin. 3, 478–485. doi: 10.3724/SP.J.1006.2013.00478
Dong, S. T., Gao, R. Q., Hu, C. H., Wang, Q. Y., and Wang, K. J. (1997). Study of canopy photosynthesis property and high yield potential after anthesis in maize. Acta Agron. Sin. 23, 318–325.
Du, C. F., Li, C. H., Liu, T. X., and Zhao, Y. L. (2011). Response of anatomical structure and photosynthetic characteristics to low light stress in leaves of different maize genotypes. Acta Ecol. Sin. 31, 6633–6640.
Evans, J. R., Vogelmann, T. C., and von Caemmerer, S. (2008). “Balancing light capture with distributed metabolic demand during C4 photosynthesis,” in Charting New Pathways to C4 Rice, eds J. E. Sheehy, P. L. Mitchell, and B. Hardy (Singapore: World Scientific Publishing), 127–143.
FAO (2003). European Communities, International Soil Reference and Information Centre (FAO, EC, ISRIC). WRB Map of World Soil Resources, 1:25 000 000. Rome: FAO.
Gao, J., Shi, J. G., Dong, S. T., Liu, P., Zhao, B., and Zhang, J. W. (2017). Response of endosperm cell proliferation and grain yield of summer maize to different light conditions. Acta Agron. Sin. 43, 1548–1558.
Hashemi-Dezfouli, A., and Herbert, S. J. (1992). Intensifying plant density response of corn with artificial shade. Agron. J. 84, 547–551. doi: 10.2134/agronj1992.00021962008400040001x
Huang, Z. H., Shen, L., Sun, G., Cao, Y., Bao, Y., Liang, X. H., et al. (2007). Study on leaf area and dry matter accumulation and distribution in super high-yield maize. J. Anhui Agric. Sci. 35, 2227–2228. doi: 10.13989/j.cnki.0517-6611.2007.08.014
Huang, Z. X., Wang, Y. J., Wang, K. J., Li, D. H., Zhao, M., Liu, J. G., et al. (2007). Photosynthetic characteristics during grain filling stage of summer maize hybrids with high yield potential of 15 000 kg ha-1. Sci. Agric. Sin. 40, 1898–1906.
Jia, S. F., Dong, S. T., Wang, K. J., Zhang, J. W., and Liu, P. (2010). Physiological mechanism of shading stress on photosynthetic efficiency in summer maize (Zea mays L.). Chin. J. Plant Ecol. 34, 1439–1447. doi: 10.3773/j.issn.1005-264x.2010.12.010
Kaushal, S., Saini, J. P., and Sankhyan, N. K. (2015). Performance of maize (Zea mays)-based intercropping systems and their residual effect on wheat (Triticum aestivum) + lentil (Lens culinaris) intercropping system under organic conditions. Indian J. Agron. 60, 224–229.
Kloss, S., and Schütze, N. (2015). Investigation of water productivity for maize with focus on the difference in global radiation between a greenhouse and a field site. Eur. Geosci. Union Gen. Assem. 60, 224–229.
Kong, X. H., Bai, X. H., and Wang, T. Q. (2012). Effects of plant density on summer maize leaf area and grain yield under cloudy & rainy conditions. J. Maize Sci. 4, 64–68.
Kramer, D. M., and Evans, J. R. (2011). The importance of energy balance in improving photosynthetic productivity. Physiol. Plant. 155, 70–78. doi: 10.1104/pp.110.166652
Kromdijk, J., Schepers, H. E., Albanito, F., Fitton, N., Carroll, F., Jones, M. B., et al. (2008). Bundle sheath leakiness and light limitation during C4 leaf and canopy CO2 uptake. Plant Physiol. 148, 2144–2155. doi: 10.1104/pp.108.129890
Kumar, I., Swaminathan, K., Hudson, K., and Hudson, M. (2016). Evolutionary divergence of phytochrome protein function in Zea mays PIF3 signaling. J. Exp. Bot. 67, 4231–4240. doi: 10.1093/jxb/erw217
Li, B. H., and Cui, Y. H. (1989). Studies on the relationship between endosperm cell development and kernel weight of summer–maize (Zea mays L.). J. Agric. Univ. Hebei 12, 39–45.
Li, C. H., Zhao, Y. L., Wang, Q., Luan, L. M., and Li, N. (2005). Effects of shading on the senescence of leaves and yield of different genotype maize. J. Maize Sci. 13, 70–73. doi: 10.13597/j.cnki.maize.science.2005.04.020
Li, L. L., Zhang, J. W., Dong, S. T., Liu, P., Zhao, B., Yang, J.-S., et al. (2012). Characteristics of accumulation, transition and distribution of assimilate in summer maize varieties with different plant height. Acta Agron. Sin. 38, 1080–1087. doi: 10.3724/SP.J.1006.2012.01080
Li, Y. D., Tang, L., Zhang, Y. P., Zhu, X. C., Cao, W. X., and Zhu, Y. (2010). Relationship of PAR interception of canopy to leaf area and yield in rice. Sci. Agric. Sin. 16, 3296–3305. doi: 10.3864/j.issn.0578-1752.2010.16.004
Lian, Y. X., Li, C. H., and Zhou, S. M. (2003). Maize dry matter yield production and distribution characteristic. J. Henan Agric. Sci. 7, 7–9. doi: 10.15933/j.cnki.1004-3268.2003.07.002
Liu, T. N., Gu, L. M., Dong, S. T., Zhang, J. W., Liu, P., and Zhao, B. (2015). Optimum leaf removal increases canopy apparent photosynthesis, 13C-photosynthate distribution and grain yield of maize crops grown at high density. Field Crop Res. 170, 32–39. doi: 10.1016/j.fcr.2014.09.015
Liu, W., Lü, P., Su, K., Yang, J. S., Zhang, J. W., Dong, S. T., et al. (2010). Effects of planting density on the grain yield and source-sink characteristics of summer maize. Chin. J. Appl. Ecol. 21, 1737–1743. doi: 10.13287/j.1001-9332.2010.0258
Liu, W., Zhang, J. W., Lu, P., Yang, J. S., Liu, P., Dong, S. T., et al. (2011). Effect of plant density on grain yield dry matter accumulation and partitioning in summer maize cultivar Denghai 661. Acta Agron. Sin. 7, 1301–1307. doi: 10.3724/SP.J.1006.2011.01301
Liu, Z. F., Gou, L., Zhao, M., and Zhang, B. J. (2011). Effects of shading on stalk morphological characteristics, rind penetration strength and lodging-resistance of maize. Acta Agric. Boreali Sin. 26, 91–96. doi: 10.7668/hbnxb.2011.04.016
Montgomery, E. G. (1911). Correlation studies in corn. Nebraska Agric. Exp. Stn. Annu. Rep. 24, 108–159.
Ren, B. Z., Cui, H. Y., Camberato, J. J., Dong, S. T., Liu, P., Zhao, B., et al. (2016). Effects of shading on the photosynthetic characteristics and mesophyll cell ultrastructure of summer maize. Sci. Nat. 103:67. doi: 10.1007/s00114-016-1392-x
Ren, G. Y., Guo, J., Xu, M. Z., Chu, Z. Y., Zhang, L., Zou, X. K., et al. (2005). Climate changes of Chinese mainland over the past half century. Acta Meteorol. Sin. 63, 942–956.
Roland, H., Lucia, F., Andreas, R., and Michael, B. (2015). Summer drought alters carbon allocation to roots and root respiration in mountain grassland. New Phytol. 205, 1117–1127. doi: 10.1111/nph.13146
Sen, S., Smith, M. E., and Setter, T. (2016). Effects of low nitrogen on chlorophyll content and dry matter accumulation in maize. Afr. J. Agr. Res. 11, 1001–1007. doi: 10.5897/AJAR2015.9673
Setter, T. L., and Flannigan, B. A. (1989). Relationship between photosynthate supply and endosperm development in maize. Ann. Bot. 64, 481–487.
Sheue, C. R., Liu, J. W., and Ho, J. F. (2015). A variation on chloroplast development: the bizonoplast and photosynthetic efficiency in the deep-shade plant Selaginella erythropus. Am. J. Bot. 102, 500–511. doi: 10.3732/ajb.1400485
Song, F. B., and Tong, S. Y. (2010). Characteristics of dry matter accumulation, distribution and translocation in maize with different plant types. Jiangsu J. Agric. Sci. 04, 700–705.
Tazoe, Y., Hanba, Y. T., Furumoto, T., Noguchi, K., and Terashima, I. (2008). Relationships between quantum yield for CO2 assimilation, activity of key enzymes and CO2 leakiness in Amaranthus cruentus, a C4 dicot, grown in high or low light. Plant Cell Physiol. 49, 19–29. doi: 10.1093/pcp/pcm160
Tollenaar, M., and Daynard, T. B. (1982). Effect of source-sink ratio on dry matter accumulation and leaf senescence of maize. Can. J. Plant Sci. 62, 855–860. doi: 10.4141/cjps82-128
Ubierna, N., Wei, S., and Cousins, A. B. (2011). The efficiency of C4 photosynthesis under low light conditions: assumptions and calculations with CO2 isotope discrimination. J. Exp. Bot. 62, 3119–3134. doi: 10.1093/jxb/err073
Wei, X. W., Wei, L., Hao, H. L., Ying, X. C., Medha, D., and Janice, T. (2009). Use of 13C labeling to assess carbon partitioning in transgenic and nontransgenic (parental) rice and their rhizosphere soil microbial communities. FEMS Microbiol. Ecol. 67, 93–102. doi: 10.1111/j.1574-6941.2008.00599.x
Zhang, R. F., Yang, H. S., Bi, W. B., Yang, S. H., Liu, J., and Gao, Q. (2011). Effects of planting patterns and irrigation on chlorophyll fluorescence and yield in winter wheat. Crops 1, 41–44. doi: 10.16035/j.issn.1001-7283.2011.01.015
Zhang, X. L., Zhang, Z. H., Song, H. X., Yu, J.-L., and Guan, C.-Y. (2016). Differences in carbon accumulation and transport in Brassica napus with different nitrogen use efficiency and its effects on oil formation. Sci. Agric. Sin. 49, 3542–3550. doi: 10.3864/j.issn.0578-1752.2016.18.008
Keywords: summer maize, photosynthetic characteristics, shading in the field, 13C photosynthate distribution, grain yield
Citation: Gao J, Zhao B, Dong S, Liu P, Ren B and Zhang J (2017) Response of Summer Maize Photosynthate Accumulation and Distribution to Shading Stress Assessed by Using 13CO2 Stable Isotope Tracer in the Field. Front. Plant Sci. 8:1821. doi: 10.3389/fpls.2017.01821
Received: 24 April 2017; Accepted: 06 October 2017;
Published: 26 October 2017.
Edited by:
Ruth Grene, Virginia Tech, United StatesReviewed by:
Mirza Hasanuzzaman, Sher-e-Bangla Agricultural University, BangladeshErik T. Nilsen, Virginia Tech, United States
Copyright © 2017 Gao, Zhao, Dong, Liu, Ren and Zhang. This is an open-access article distributed under the terms of the Creative Commons Attribution License (CC BY). The use, distribution or reproduction in other forums is permitted, provided the original author(s) or licensor are credited and that the original publication in this journal is cited, in accordance with accepted academic practice. No use, distribution or reproduction is permitted which does not comply with these terms.
*Correspondence: Jiwang Zhang, jwzhang@sdau.edu.cn