- 1Department of Sustainable Crop Protection, Università Cattolica del Sacro Cuore, Piacenza, Italy
- 2Instituto Agroforestal Mediterráneo, Universitat Politècnica de València, Valencia, Spain
The fungal genus Venturia Sacc. (anamorph Fusicladium Bonord.) includes plant pathogens that cause substantial economic damage to fruit crops worldwide. Although Venturia inaequalis is considered a model species in plant pathology, other Venturia spp. also cause scab on other fruit trees. Relative to the substantial research that has been conducted on V. inaequalis and apple scab, little research has been conducted on Venturia spp. affecting other fruit trees. In this review, the main characteristics of plant-pathogenic species of Venturia are discussed with special attention to V. inaequalis affecting apple, V. pyrina affecting European pear, V. nashicola affecting Asian pear, V. carpophila affecting peach and almond, Fusicladium oleagineum affecting olive, F. effusum affecting pecan, and F. eriobotryae affecting loquat. This review has two main objectives: (i) to identify the main gaps in our knowledge regarding the biology and epidemiology of Venturia spp. affecting fruit trees; and (ii) to identify similarities and differences among these Venturia spp. in order to improve disease management. A thorough review has been conducted of studies regarding the phylogenetic relationships, host ranges, biologies, and epidemiologies of Venturia spp. A multiple correspondence analysis (CA) has also been performed on the main epidemiological components of these Venturia spp. CA separated the Venturia spp. into two main groups, according to their epidemiological behavior: the first group included V. inaequalis, V. pyrina, V. nashicola, and V. carpophila, the second F. oleagineum and F. eriobotryae, with F. effusum having an intermediate position. This review shows that Venturia spp. affecting fruit trees are highly host-specific, and that important gaps in understanding the life cycle exist for some species, including V. pyrina; gaps include pseudothecia formation, ascospore and conidia germination, and mycelial growth. Considering the epidemiological information reviewed, this paper shows that the use of Mills tables to predict infection periods should be avoided for Venturia spp. other than V. inaequalis.
Introduction
The fungal genus Venturia Sacc. (anamorph Fusicladium Bonord.) includes plant pathogens that cause substantial economic damage to fruit crops worldwide (Sivanesan, 1977; Schubert et al., 2003). Although, Venturia inaequalis (Cooke) G. Winter is considered a model species in plant pathology (Machardy, 1996) and it is the causal agent of apple scab, the most important apple disease worldwide, other Venturia spp. also cause scab on other fruit trees. In this review, the main characteristics of plant-pathogenic species of Venturia are discussed with special attention to V. inaequalis affecting apple (Malus spp.); V. pyrina and V. nashicola affecting European pear (Pyrus communis) and Asian pear (P. pyrifolia var. culta and P. ussuriensis), respectively; V. carpophila affecting peach (Prunus domestica) and almond (Prunus dulcis); Fusicladium oleagineum affecting olive (Olea europea); F. effusum affecting pecan (Carya illinoinensis); and F. eriobotryae affecting loquat (Eriobotrya japonica). Aspects of V. asperata affecting apple and V. cerasi affecting cherry (Prunus cerasus) are also discussed (Table 1).
Since the late nineteenth century, apple scab has been extensively investigated, and substantial information—covering all key aspects of the biology and genetics of the fungus and the epidemiology and control of the disease—has been published and reviewed by Machardy (1996) and Bowen et al. (2011). In contrast to the efforts devoted to investigating V. inaequalis, little work has been conducted on Venturia spp. affecting other fruit trees, as shown by the number of papers published for each species (Figure 1A). This difference in research effort and number of publications, however, does not directly reflect the importance of the host crops worldwide (Figure 1B). The difference might be explained by (i) minor investments in these non-apple crops, (ii) less specialized management directed at the non-apple crops, and (iii) the common use of the information developed for V. inaequalis for managing the other fruit scabs. Concerning the last point, researchers generally assume that infection of any scab fungus may occur under environmental conditions similar to those required by V. inaequalis. The Mills and Laplante's (1954) table, which is the most popular system for scheduling fungicides against apple scab, has been broadly recommended for management of pear scab (Sobreiro and Mexia, 2000; Mitcham and Elkins, 2007; Travis et al., 2012; Elkins et al., 2016), cherry scab (Schweizer, 1958), peach scab (Keitt, 1917; Pineau et al., 1991), and loquat scab (Ramos, 2008; GVA, 2014). However, there is no clear evidence that the environmental conditions conducive for infection are similar for all of these Venturia species. In fact, recent studies have revealed important differences concerning the environmental requirements for infection by F. eriobotryae and F. oleagineum vs. V. inaequalis (Viruega et al., 2011; González-Domínguez et al., 2013). In addition, substantial differences exist in the ecophysiologies and the life cycles of their hosts.
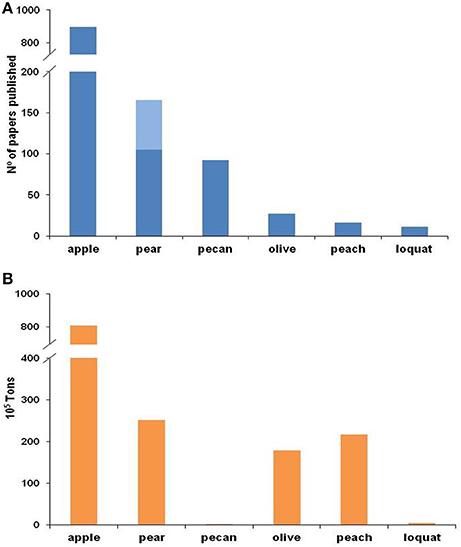
Figure 1. Number of papers published on scab (A) and worldwide production (B) for each fruit crop. For (A), the Web of Science was searched on 30 August 2016 for different combinations of words in the title (for apple: “Venturia inaequalis” or “Fusicladium pomi” or “Spilocaea pomi” or “apple scab”; for pear: “Venturia pyrina” or “Venturia pirina” or “Fusicladium pyrorum” or “pear scab” and in light blue “Venturia nashicola” or “Fusicladium nashicola”; for pecan: “Fusicladium effusum” or “Cladosporium effusum” or “Cladosporium caryigenum” or ”pecan scab”; for olive “Spilocaea oleagina” or “Fusicladium oleagineum” or “olive scab” or “olive leaf spot”; for peach: “Venturia carpophila” or “Fusicladium carpophilum” or “Cladosporium carpophilum” or “peach scab”; and for loquat: “Fusicladium eriobotryae” or “Spilocaea eriobotryae” or “loquat scab”). Worldwide production data for (B) were extracted from FAOSTAT, except in the case of loquat, where data from González-Domínguez (2014) were used.
This review has two main objectives: (i) to identify the main gaps in our knowledge regarding the biology and epidemiology of Venturia spp. affecting fruit trees; and (ii) to identify similarities and differences between these Venturia spp. in order to improve disease management. To accomplish these objectives, the authors have thoroughly reviewed the studies regarding the phylogenetic relationships, host ranges, biologies, and epidemiologies of Venturia spp. Finally, the review discusses the implications of the similarities and differences in the fungi and the diseases for the management of the different scab diseases.
Taxonomy of the Genus Venturia
The genus Venturia belongs to the phylum Ascomycota, class Dothideomycetes (Schoch et al., 2009). Traditionally, this genus has been included in the family Venturiaceae, order Pleosporales, according to its “Pleospora-type centrum and bitunicate asci” (Sivanesan, 1977). However, recent molecular phylogenetic analyses of Dothideomycetes, using both nuclear and mitochondrial gene regions, have indicated that the family Venturiaceae forms a well-supported monophyletic group separate from the Pleosporales (Kodsueb et al., 2006; Kruys et al., 2006; Zhang et al., 2011). Thus, Zhang et al. (2011) recently reordered Venturiaceae into Venturiales ord. nov. (together with Sympoventuriaceae fam. nov.).
The genus Venturia Sacc. (1882) was first noted in 1844 by Notaris, who described, V. dianthi and V. rosae. Cesati & Notaris added new species in 1863 (Sivanesan, 1977). Saccardo reevaluated the genus in 1882, excluding both V. dianthi and V. rosae (Sivanesan, 1977). Sivanesan (1977) listed 52 species of Venturia, which comprised parasitic species with pseudothecia immersed in the host tissue, bitunicate asci, and olive-brown, septate ascospores (Sivanesan, 1977). Currently, 290 species are recognized in the Mycobank dabatase (http://www.mycobank.org/Biolomics.aspx?Table=Mycobankx, March/2017).
The anamorphs of Venturia spp. have been traditionally classified in three genera: Fusicladium Bonord., Pollaccia Baldacci & Cif., and Spilocaea Fr. Assignment to these genera depends on the morphology of the conidiogenous cells. These cells are sympodial in Fusicladium and percurrent in Pollaccia and Spilocaea (Hughes, 1953; Sivanesan, 1977). Recents works that used both morphological and molecular characters concluded that the anamorphic species of Venturia should not be separated into these three genera because (i) most species have both sympodial and percurrent conidiogenous cells, and (ii) molecular phylogenetic analysis clearly shows that Venturia and its anamorphs are monophyletic group (Schubert et al., 2003; Beck et al., 2005). Because most anamorphs of Venturia have been classified as Fusicladium, this name was proposed to designate the asexual stage of Venturia spp. (Braun et al., 2002), and used in the monograph written by Schubert et al. (2003). Very recently, the International Commission on the Taxonomy of Fungi has proposed the use of Venturia instead of Fusicladium for the species with only anamorph stage, following the guidelines of the “Amsterdam Declaration on Fungal Nomenclature” (May, 2017). In this paper we keep the dual nomenclature because it facilitates to distinguish the species with or without sexual phase.
In 2003, Partridge and Morgan-Jones (2003) proposed the new genus Fusicladosporium, including the anamorphs of pecan (F. effusum), peach (F. carpophillum), and maple scab (F. humile; teleomorph V. acerina). The authors considered that significant morphological differences (conidia formed in chains and the prominence of conidial scars on conidiophores) distinguished these anamorphs from those in the genera Cladosporium and Fusicladium. However, this new taxon seems unjustified because two older generic names for Venturia anamorphs with catenate conidia are available (Hormocladium Höhn. and Ramalia Bat) and because phylogenetic analysis of the ITS region demonstrates that the erection of Fusicladosporium results in a polyphyletic genus (Schubert et al., 2003; Beck et al., 2005). Thus, Fusicladosporium is currently considered a synonym of Fusicladium (Schubert et al., 2003; Crous et al., 2007; Scherm et al., 2008; Seyran et al., 2009; Lalancette et al., 2012).
Venturia Species as Fruit Tree Pathogens
Geographical Distribution
Differences exist in the geographical distribution of the Venturia spp. that are pathogens of fruit trees (Figure 2).
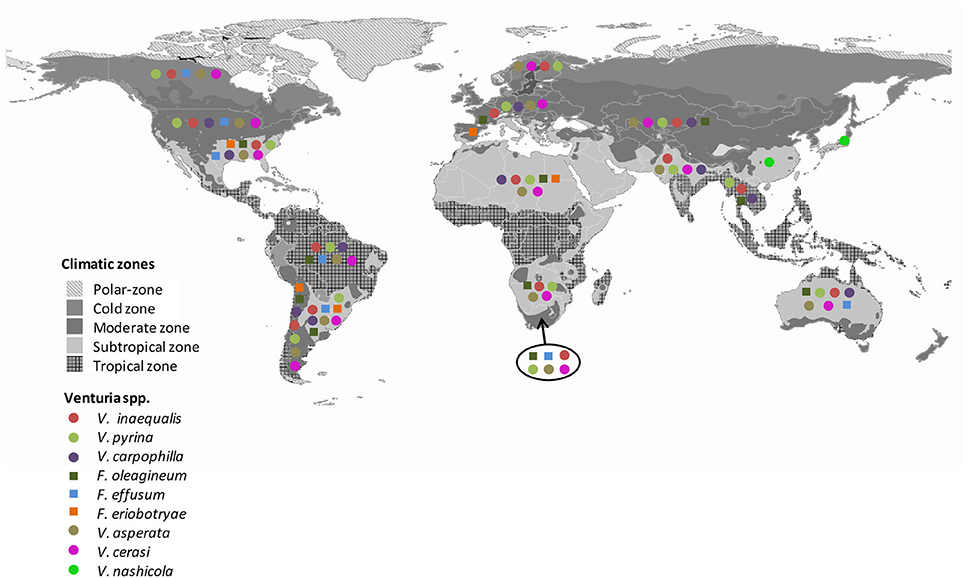
Figure 2. Worldwide distribution of Venturia spp. Gray colors indicate the climate regions proposed by Kottek et al. (2006). Colors and symbols indicate the presence of the different Venturia spp. in each region. Data are from Plantwise and EPPO database.
There are numerous reports about the worldwide distribution of apple scab (Machardy, 1996; Schnabel et al., 1999; Rossi et al., 2007; Gladieux et al., 2008, 2010b; Xu et al., 2009; Bowen et al., 2011; Li et al., 2011; Padder et al., 2013). Apple scab occurs in every country where apple (Malus x domestica) is cultivated (Machardy, 1996; Gladieux et al., 2008), with the exception of West Australia, where the disease was eradicated (McKirdy et al., 2001). Venturia inaequalis probably emerged in Central Asia, the center of apple origin (Tenzer and Gessler, 1999; Gladieux et al., 2008, 2010b; Xu et al., 2008, 2012), and followed its host's expansion into Europe and, more recently, into regions with the expansion of apple cultivation (Gladieux et al., 2008). Venturia inaequalis infecting apple in Europe and Central Asia consists of three distinct populations: (i) a large European population infecting the domesticated apple and the wild Malus sylvestris; (ii) a large Central Asian population infecting the domesticated apple and populations of Malus sieversii; and (iii) a more geographically restricted population associated with M. sieversii in areas where M. domestica is absent (Gladieux et al., 2010b). Xu et al. (2008, 2012) found a higher variability in a population of V. inaequalis from the same orchard in the UK than in populations from different cultivars or regions in China. Overall, V. inaequalis appears to be a model invasive plant pathogen with a broad geographic distribution and well-established populations (Gladieux et al., 2010b).
Like V. inaequalis, V. pyrina has a worldwide distribution that is closely associated with the distribution of its host, the European pear (P. communis; Ogawa and English, 1991; Figure 2). V. nashicola, in contrast, is restricted to China, Japan, South Korea, and Taiwan, where Japanese and Chinese pears are widely cultivated (www.plantwise.org; EPPO, 2016). V. nashicola is currently considered a quarantine organism in the EU, USA, Israel, and Turkey (EPPO, 2016).
Venturia carpophila affecting Prunus spp., F. oleagineum affecting olive, and V. cerasi affecting cherries have relatively restricted distributions, which again reflects the distributions of their hosts (www.plantwise.org) (Figure 2). Venturia cerasi has been reported in Canada, Brazil, New Zealand, Iran, and northern Europe, where cherry is widely cultivated (www.plantwise.org; www.fao.org). Fusicladium oleagineum is widespread in the Mediterranean basin as well as in other temperate and subtropical areas of the world (Graniti, 1993). However, its presence has not been noted in other areas where olives are grown, such as North America, Northern Europe, and South Asia (i.e., India, Nepal, Thailand, and Vietnam; Figure 2). The distribution of V. carpophila is similar to that of F. oleagineum, although the former species has been reported in South Asia but not in South Africa (www.plantwise.org).
Fusicladium effusum and F. eriobotryae apparently have relatively restricted distributions. F. effusum has been detected in South Africa and Australia (Figure 2) and is widely distributed in the Americas, where pecan is an important crop. F. eriobotryae has mainly been reported in the Mediterranean basin, but it was also reported in the USA and Chile (Raabe and Gardner, 1972; Acuña, 2010). As far as known, F. eriobotryae is not present in China, the center of origin of loquat. Moreover, no references were found confirming the presence of F. eriobtryae in Japan or Taiwan, where loquat is widely cultivated.
Phylogenetic Relationships
Several molecular studies have evaluated the phylogenetic relationships among the Venturia species affecting fruit trees (Schnabel et al., 1999; Stehmann et al., 2001; González-Lamothe et al., 2002; Le Cam et al., 2002; Beck et al., 2005; Sánchez-Torres et al., 2009; Gladieux et al., 2010a; Zhao G.-J. et al., 2011; Zhao P. et al., 2011). In these studies, Venturia species and their anamorphs formed a monophyletic clade composed of several small sub-clades. The sub-clades mainly contain strains of the same species, although in some cases strains from different species form a unique sub-clade, as occurs with V. cerasi and V. asperata (Schnabel et al., 1999; Stehmann et al., 2001; González-Lamothe et al., 2002; Beck et al., 2005), V. pyrina and V. nashicola (Schnabel et al., 1999; Stehmann et al., 2001; González-Lamothe et al., 2002; Beck et al., 2005), and V. inaequalis, F. eriobotryae, and F. pyracanthae (Le Cam et al., 2002). All of these analyses concerned the ITS region, which is the most widely used region for species identification in mycology. However, results from this region cannot be considered conclusive for distinguishing closely related fungal species (Kiss, 2012; Schoch et al., 2012). When other nuclear loci have been used, such as the elongation factor or the G3PD genes, differences between closely related Venturia spp. have been observed (Sánchez-Torres et al., 2009; Gladieux et al., 2010b; Zhao P. et al., 2011).
V. inaequalis, F. eriobotryae, and F. pyracanthae exhibit a high sequence similarity in their ITS and other DNA regions (Gladieux et al., 2010a). In general, the topology of the phylogram for Venturia species aligns closely with that of the host genera, demonstrating a close co-evolutionary relationship between the pathogenic Venturia spp. and their respective fruit tree hosts (Ishii and Yanase, 2000; Beck et al., 2005; Bowen et al., 2011). Because of these high similarity and based on the criterion of concordance between multiple gene genealogies (Taylor et al., 2000), Le Cam et al. (2002) and Gladieux et al. (2010a) considered F. eriobotryae and F. pyracanthae as formae speciales of V. inaequalis. Sánchez-Torres et al. (2009) performed further molecular analyses (a phylogenetic analysis of the G3PD gene, a microsatellite-primed PCR analysis, and RAPD fingerprinting) and pathogenicity tests for F. eriobotryae and V. inaequalis; these authors concluded that the loquat scab fungus is a distinct species from V. inaequalis.
Similarly, researchers were able to distinguish between V. pyrina and V. nashicola based on a further phylogenetic analysis of the elongation factor gene (Zhao P. et al., 2011), whereas an earlier study was unable to do so based on the ITS region (Beck et al., 2005). Until the 1960s, V. pyrina was considered the causal agent of scab on Japanese and Chinese pear (Pyrus pyrifolia var. culta and P. ussuriensis, respectively). However, further studies indicated that the causal agent of scab on Asian pears differed from V. pyrina (Tanaka and Yamamoto, 1964; Ishii and Yanase, 2000; Abe et al., 2008; Zhao P. et al., 2011).
The results described in the previous paragraphs show that Venturia spp. have a monophyletic evolutionary origin and a close co-evolutionary development with their hosts. In addition, species delimitations become clearer when the phylogenetic analyses are performed in DNA regions in addition to the ITS region.
Host Range
Venturia spp. are confined to six host families: Acaraceae, Betulaceae, Cornaceae, Oleaceae, Rosaceae, and Salicaceae (Sivanesan, 1977). Venturia spp. also seem to be highly host specific in that each species is usually confined to one host genus or at least to allied host genera in one host family (Schubert et al., 2003).
Some controversy exists regarding the host range of the Venturia spp. on fruit trees. In the monograph of Venturia published in 1977, Sivanesan listed 14 species of Rosaceae as hosts of V. inaequalis, and these hosts belonged to the genera Cotoneaster, Malus, Pyracantha, Pyrus, and Sorbus. Schubert et al. (2003) increased the hosts of V. inaequalis to including 12 genera by adding Amelanchier, Aronia, Docynia, Eriobotrya, Heteromeles, Kageneckia, and Prunus. The latter authors, however, did not cite specific studies regarding the ability of V. inaequalis to infect these hosts, and some of these host-pathogen interactions have been refuted (Menon, 1956; Raabe and Gardner, 1972; Ishii and Yanase, 2000; Stehmann et al., 2001; Le Cam et al., 2002; Chevalier et al., 2004; Sánchez-Torres et al., 2007a, 2009; Abe et al., 2008).
Both Schubert et al. (2003) and Sivanesan (1977) considered P. communis to be a host of V. inaequalis; Schubert et al. (2003) also considered Malus domestica to be a host of V. pyrina. Other studies, however, have failed to obtain infection of pear by V. inaequalis or infection of apple by V. pyrina (Menon, 1956; Stehmann et al., 2001; Chevalier et al., 2004) (Table 2). Menon (1956) inoculated apple and pear plants with three kinds of V. inaequalis and V. pyrina inocula (mycelium plugs, conidial suspensions, and ascospore suspensions) and observed clear scab symptoms on apple only with V. inaequalis and on pear only with V. pyrina and in both cases only with conidia or ascospores (Table 2).
Stehmann et al. (2001) found pseudothecia of both V. inaequalis and V. pyrina in dead apple leaves but cross inoculations with the conidial suspensions of these isolates on detached apple and pear leaves resulted in infection only when apple was inoculated with V. inaequalis and when pear was inoculated with V. pyrina (Table 2). Although the conidia of V. inaequalis and V. pyrina germinated on both hosts, and although the germ tubes produced appressoria and runner hyphae on both hosts, a dense subcuticular network of stroma was produced only in the compatible host (Stehmann et al., 2001; Chevalier et al., 2004).
Pyracantha (Pyracantha spp.) and loquat (E. japonica) have also been considered hosts of V. inaequalis (Sivanesan, 1977; Jones and Aldwinckle, 1990; Machardy, 1996; Schubert et al., 2003; Jha et al., 2009; Bowen et al., 2011). However, Le Cam et al. (2002) were unable to obtain infection of pyracantha by V. inaequalis or infection of apple by F. pyracanthae (Table 2). Similarly, inoculations of loquat with V. inaequalis, V. pyrina, or V. carpophila did not cause infection (Sánchez-Torres et al., 2007b, 2009; Table 2). Loquat plants inoculated with V. pyrina showed symptoms on leaves, but the symptoms differed from those caused by the loquat scab fungus F. eriobotryae (Sánchez-Torres et al., 2007b). Raabe and Gardner (1972) successfully infected loquat plants with F. pyracanthae. Based on this information, Gladieux et al. (2010a) considered F. pyracanthae and F. eriobotryae as unique species, but the results of Raabe and Gardner (1972) have never been confirmed.
The designation of V. nashicola and V. pyrina as distinct species was confirmed by the unsuccessful inoculation of European pear (P. communis) by V. nashicola and of Japanese and Chinese pear (P. pyrifolia and P. ussuriensis, respectively) by V. pyrina (Ishii and Yanase, 2000; Park et al., 2000; Abe et al., 2008). Like V. inaequalis and V. pyrina on non-hosts, V. nashicola germinated and formed appressoria on European pears but the hyphae collapsed after the host was penetrated (Abe et al., 2008).
Overall, results from cross inoculations of Venturia spp. on different Rosaceous hosts (Table 2) show that these pathogens are host specific, and the information contained in the monographs of Schubert et al. (2003) and Sivanesan (1977) cannot be reproduced. Host specificity requires further study for F. pyracanthae and F. eriobotryae.
Life Cycle
A main characteristic distinguishing the life cycles of some Venturia spp. from others is the presence/absence of the sexual stage (Table 1). Venturia inaequalis, V. pyrina, V. nashicola, and V. cerasi form pseudothecia in detached leaves on the orchard ground (Schweizer, 1958; Latorre et al., 1985; Umemoto, 1990b; Spotts and Cervantes, 1994; Machardy, 1996; Rossi et al., 2001; Eguchi and Yamagishi, 2007; Lian et al., 2007). Pseudothecia of V. carpophila were also observed in affected detached leaves of apricot (Fisher, 1961), but their epidemiological role is unknown, and the conidia of V. carpophila overwintering in affected twigs are commonly considered the primary inoculum (Lan and Scherm, 2003; Lalancette et al., 2012). Ascocarps have never been found in nature for F. effusum, F. oleagineum, F. eriobotryae, or F. pyracanthae; conidia are considered the only form of primary inoculum for these species (Raabe and Gardner, 1972; Gottwald and Bertrand, 1982; Graniti, 1993; González-Domínguez et al., 2014b).
Interestingly, pseudothecia are found in those Venturia spp. affecting deciduous fruit trees such as apple, cherry, nectarine, or European and Asian pears, but not in those species affecting evergreen trees, including pyracantha, loquat, and olive (Table 1).
The pseudothecia-forming species survive winter mainly as pseudothecia in detached leaves on the surface of the orchard floor. In spring, these pseudothecia repeatedly discharge ascospores, which constitute the primary inoculum for infection. Species that lack pseudothecia overwinter (or oversummer) as mycelium and conidia in lesions on twigs and leaves and in the mummified fruits remaining in the tree after harvest (Figure 3).
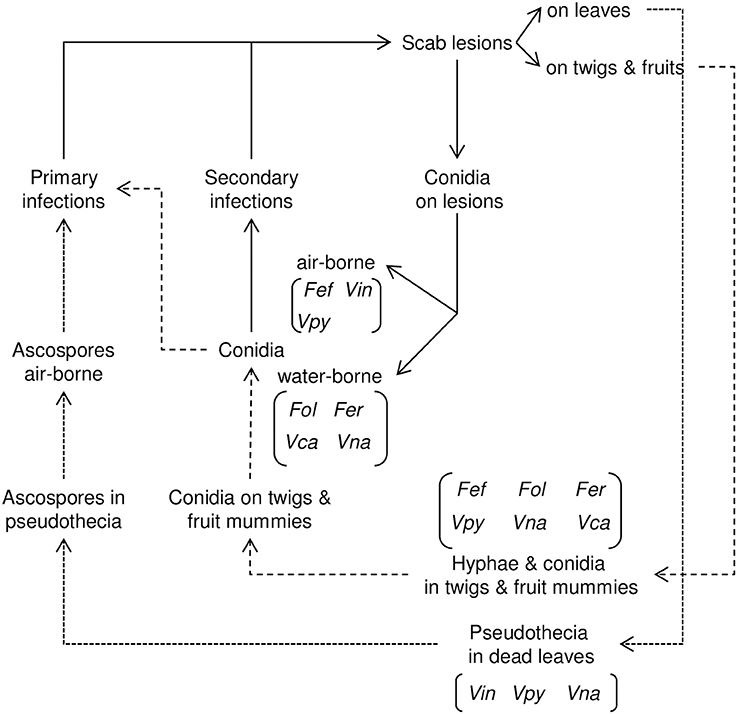
Figure 3. Relational diagram of the life cycle of Venturia spp. Dotted lines (…) indicate the sexual phase of the life cycle; dashed lines (-----) indicate the asexual phase of the life cycle. The species of Venturia in which the different stages occur are indicated in brackets. Fef, Fusicladium effusum; Fer, Fusicladium eriobotryae; Fol, Fusicladium oleagineum; Vca, Venturia carpophila; Vin, Venturia inaequalis; Vna, Venturia nashicola; Vpy, Venturia pyrina.
The asexual cycle is similar for all Venturia species. Conidia produced on lesions cause secondary infections during the entire tree-growing season as long as the environmental conditions permit conidial production, dispersal, germination, infection, and lesion growth (Figure 3).
Main Epidemiological Components
Primary Inoculum Sources
Venturia inaequalis survives in winter mainly as pseudothecia on dead, scabbed leaves on the ground (Table 3; Machardy, 1996). Under some environmental conditions, the fungus can survive in winter as conidia, and when this occurs, these conidia contribute to the primary inoculum. Studies by Holb et al. (2004a) in The Netherlands, Hill (1975) in Germany, and Becker et al. (1992) in New York found that, although large numbers of conidia are present on the surface of shoots or outer bud tissues, conidia are able to overwinter (i.e., are viable in early spring) only on the inner of bud tissues. Becker et al. (1992) and Holb et al. (2004a) reported that this conidia that overwinter in buds are able to infect young green tissues. Recently, Passey et al. (2017) suggested that conidia may contribute 20–50% of the primary inoculum in early spring.
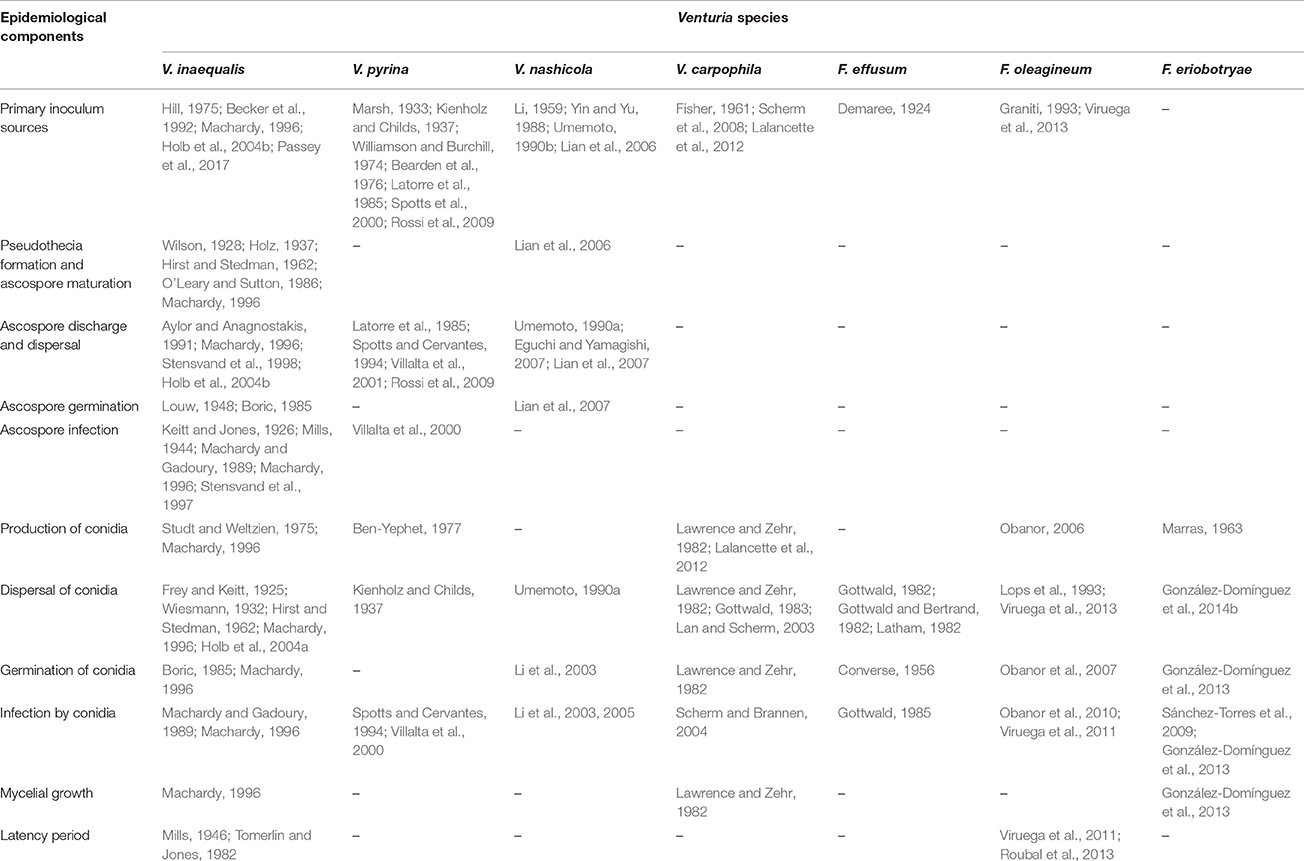
Table 3. Publications reporting specific experiments concerning the epidemiological components of Venturia spp.
Venturia pyrina overwinters as both pseudothecia in affected leaves on the orchard floor and as conidia in twig lesions (Marsh, 1933; Kienholz and Childs, 1937; Williamson and Burchill, 1974; Bearden et al., 1976; Latorre et al., 1985; Spotts et al., 2000; Rossi et al., 2009). Based on conidia and ascospore trappings in England and Oregon, respectively, Marsh (1933) and Kienholz and Childs (1937) considered that primary infections by V. pyrina resulted largely from conidia derived from the previous seasons. Other reports have indicated that ascospores from pseudothecia are the main inoculum source (Bearden et al., 1976; Latorre et al., 1985; Spotts et al., 2000; Rossi et al., 2009) and that infection from conidia overwintering on twig lesions only occur on severely affected pear trees.
Conidia from dormant mycelia in buds of pear trees have been considered the main source of primary inoculum for V. nashicola (Li, 1959; Yin and Yu, 1988). Ascospores of V. nashicola have also been considered an important source of primary inoculum in China (Lian et al., 2006) and Japan (Umemoto, 1990b).
Venturia carpophila overwinters as mycelia in lesions on fruit-bearing 1-year-old twigs (Scherm et al., 2008; Lalancette et al., 2012), and the conidia produced on these lesions act as the primary inoculum. Pseudothecia of V. carpophila were found in overwintering apricot leaves only in a severely affected orchard in Australia (Fisher, 1961). However, the epidemiological role of the teleomorph in the V. carpophila disease cycle remains unknown (Lalancette et al., 2012).
On pecan trees, F. effusum overwinters mainly on the surface of twigs and nuts as stromata, which sporulate profusely in early spring (Demaree, 1924). The most important inoculum sources of F. oleagineum are the infected leaves remaining in the olive canopy (Graniti, 1993; Viruega et al., 2013), because the fungus does not produce conidia on fallen, scabbed leaves (Viruega et al., 2013).
Little is known about the inoculum sources of F. eriobotryae. The fungus probably oversummers (its host, loquat, blooms in autumn, develops fruit in winter, and ripens in early spring) in lesions on branches and leaves and in mummified fruits (González-Domínguez et al., 2014a). However, the ability of the fungus to sporulate on these potential inoculum sources and their epidemiological role have never been studied.
Pseudothecia Formation and Ascospore Maturation
For V. inaequalis and V. nashicola, light enhances pseudothecial production; pseudothecia formation is significantly reduced when the leaves overwinter in darkness (Table 3; Hirst and Stedman, 1962; Lian et al., 2006). Leaves overwintering in soil developed abnormal V. inaequalis pseudothecia, whereas those exposed weekly to 20 min of light produced normal ones (Holz, 1937). For both pathogens, moisture plays a key role. For V. inaequalis, rain is necessary for the growth of mycelium into the leaf lamina and for initiaion of the ascigerous stage (Machardy, 1996). Pseudothecia developed at low rates during dry periods and matured rapidly during rain periods (Wilson, 1928); however, continous wetness delayed ascospore maturation. Similarly, continuous wetness prevented pseudothecial development by V. nashicola (Lian et al., 2006). Pseudothecia formation and ascospore maturation occurred under a wide range of temperatures for both pathogens (O'Leary and Sutton, 1986; Lian et al., 2006).
Ascospore Discharge and Dispersal
Environmental requirements for ascospore discharge have been frequently studied for V. inaequalis (Table 3). For V. inaequalis, V. pyrina, and V. nashicola, ascospores are mainly discharged during or following rain events (Latorre et al., 1985; Umemoto, 1990a; Spotts and Cervantes, 1994; Machardy, 1996; Villalta et al., 2001; Eguchi and Yamagishi, 2007; Lian et al., 2007; Rossi et al., 2009). Villalta et al. (2001) captured 90% of V. pyrina ascospores during rain events, and Rossi et al. (2009) observed that at least 1.2 mm of rain was neccesary for ascopore ejection.
For V. nashicola, Lian et al. (2007) observed that 10 s of wetness was sufficient for ascospore discharge. For V. inaequalis, 0.0025 mm of rain may result in ascospore ejection, but >0.2 mm of rain is usually necessary to capture ascospores in traps (Machardy, 1996). Although some papers have reported the trapping of a few ascospore in periods without rain (Machardy, 1996), the trapping of many ascospores in the early morning following heavy dew at night has been reported only in Norway (Stensvand et al., 1998).
In general, a daily periodicity of ascospore discharge has been observed for V. pyrina (Latorre et al., 1985; Villalta et al., 2001), V. nashicola (Eguchi and Yamagishi, 2007), and V. inaequalis (Machardy, 1996). In all of these cases, most of the ascospores were trapped between 6:00 and 18:00 h.
Pseudothecia are able to eject ascospore to a height of 8 mm for V. nashicola (Umemoto, 1990a) and 5–13 mm for V. inaequalis (Aylor and Anagnostakis, 1991). After ejection, ascospores of both pathogens are dispersed by wind. Umemoto (1990a) was able to sample air-borne ascospores of V. nashicola to a distance of 10 m from the inoculum source. In the case of V. inaequalis, ascospores have been captures as far as 45 m from the inoculum source (Holb et al., 2004b).
Ascospore Germination and Infection
Ascospore germination has been studied for V. inaequalis and V. nashicola (Table 3), and had similar temperature requirements for the two species. The ascospores germinate between 5 and 30°C (germination of V. inaequalis has been observed at 0.5°C), with the optimum at 15–25°C (Louw, 1948; Boric, 1985; Lian et al., 2007). Ascospores begin to germinate after 2–3 h when the temperature is optimal; at 10°C, V. inaequalis germinated after 3 h of wetness and V. nashicola after 6 h of wetness (Boric, 1985; Lian et al., 2007).
Keitt and Jones (1926) were the first to conduct a controlled-environment experiment concerning the minimum number of hours of wetness required for infection by V. inaequalis ascospores. This information was then used by Mills (1944) to develop a chart representing the minimum hours of wetness for light, moderate, and severe infection. Although this publication is considered a milestone in plant pathology, many reports (reviewed by Machardy and Gadoury, 1989) have found that the chart requires modification. Infection by ascospores requires approximately 3 h less than the minimum proposed by Mills, i.e., it requires 5 h of wetness at 20°C and 8 h of wetness at 12° or 25°C. Therefore, Machardy and Gadoury (1989) proposed a new curve that describes the minimum hours of wetness necessary for infection at any temperature. Stensvand et al. (1997) subsequently modified the infection curve for temperatures between 2 and 8°C.
Villalta et al. (2000) reported that infection by V. pyrina ascospores was similar to that reported for V. inaequalis (Machardy and Gadoury, 1989) at temperatures below 10°C and at 25°C. In the optimal temperature range of 20–25°C, however, infection by V. pyrina ascospores required 9 h of wetness rather than the 5 h required by V. inaequalis.
Production of Conidia
Venturia inaequalis, V. nashicola and Fusicladium oleagineum are able to sporulate at temperatures from 5 to 25°C (Table 3). V. pyrina can sporulate at temperatures between 5 and 28°C, which are the only temperatures tested for this species (Ben-Yephet, 1977), whereas V. carpophila can also sporulate at 30°C (Lalancette et al., 2012). The highest sporulation rate occurred from 15 to 20°C for all species, except for F. eriobotryae, which produced the most conidia at 5–10°C (Marras, 1963).
Venturia inaequalis was able to sporulate between 60 and 100% RH, with the optimum at 90% RH (Studt and Weltzien, 1975). V. carpophila and Fusicladium oleagineum sporulated at <70% RH (Lawrence and Zehr, 1982; Obanor, 2006). For F. oleaginum, sporulation at 70% RH was <50% of the maximum observed under continuous wetness. Under optimal conditions, V. carpophila sporulation was highest after 72 h of incubation, whereas F. oleaginum sporulation still increased after 14 days of incubation at 100% RH (Lawrence and Zehr, 1982; Obanor, 2006; Lalancette et al., 2012; Figure 4).
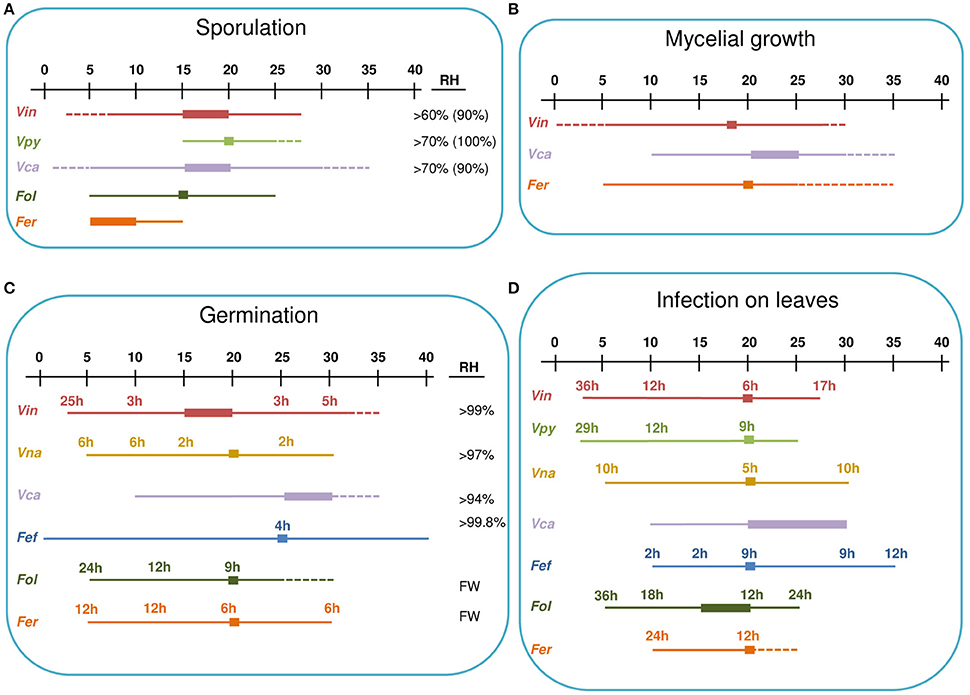
Figure 4. Environmental requirements of Venturia spp. for sporulation (A), mycelial growth (B), germinacion (C), and leaves infection (D). A temperature scale from 0 to 40°C is indicated at the top of each panel. Thin lines indicate the temperature at which the different processes occur for each species. Thick lines indicate optimal temperatures. Dotted lines indicate temperatures that are known not to support the process based on experimental evidence. Numbers indicate the hours of wetness necessary at each temperature. For sporulation (A), the RH range in which the process can occur is indicated, with the optimal RH in brackets. For conidial germination (C), the RH range in which the process can occur is indicated; FW indicates that free water is required for germination. Fef, Fusicladium effusum; Fer, Fusicladium eriobotryae; Fol, Fusicladium oleagineum; Vca, Venturia carpophila; Vin, Venturia inaequalis; Vna, Venturia nashicola; Vpy, Venturia pyrina.
Dispersal of Conidia
Spore sampling studies have been conducted for several species (Table 3). These studies consistently show that conidia of Venturia spp. are mainly collected during or soon after rain events.
Venturia conidiophores (which are short and blunt) do not favor the removal of conidia by wind. Conidia of V. inaequalis were dislodged from dry, sporulating lesions by strong air currents only; the addition of a drop of water, however, caused conidiophores to swell and conidia to dislodge almost immediately (Frey and Keitt, 1925). In several spore-sampling studies in orchards, conidia were trapped from the air, frequently in low numbers, only during rainy weather; in only a few cases were high numbers of V. inaequalis conidia trapped during dry weather (Keitt and Jones, 1926; Gupta and Lele, 1980), with a diurnal periodicity and a peak in the afternoon (Hirst and Stedman, 1961). Machardy (1996) hypothesized that the release of conidia on dry days was triggered by the moisture provided by dew. A steep dispersal gradient was observed for V. inaequalis conidia, with few conidia sampled at >10 m from the inoculum source (Wiesmann, 1932).
For V. pyrina, conidia were also sampled from the air in periods with rain (Kienholz and Childs, 1937). The steep dispersal gradient for V. nashicola conidia (maximum dispersal distance = 8 m) suggests that these conidia are splash dispersed (Umemoto, 1990a).
Venturia carpophila and F. effusum conidia were traditionally considered both wind and splash dispersed (Gottwald, 1982, 1983; Gottwald and Bertrand, 1982; Latham, 1982; Lawrence and Zehr, 1982) because they were sampled from the air during several dry periods without rain (Gottwald and Bertrand, 1982; Latham, 1982; Lawrence and Zehr, 1982). In controlled-environment experiments, V. carpophila and F. effusum conidia were mainly dispersed in periods with a rapid decrease of RH and light (Gottwald and Bertrand, 1982; Gottwald, 1983). Latham (1982) observed a marked diurnal periodicity for F. effusum conidial dispersal, with a peak at 12:00 h, concomitant with decreasing humidity.
The role of rain dispersal for V. carpophila was re-evaluated by Lan and Scherm (2003). In a 4-year study, air-borne conidia contributed little to fruit scab in comparison to water-borne conidia; exclusion of splashing conidia decreased disease severity by >90%, and runoff of water from the twig to the fruit via the peduncle also contributed to scab development. Bock et al. (2011) showed that V. carpophila lesions are not uniformly distributed on the peach fruit surface, i.e., most lesions develop near the peduncle.
Conidia of F. oleagineum were mainly dispersed by rain, with a low degree of wind dissemination in the absence of rain when RH was high (Lops et al., 1993). Fusicladium oleagineum conidia were dispersed near the inoculum source (<10 m) with a linear and positive relationship between rainfall and numbers of conidia dispersed (Viruega et al., 2013). De Marzo et al. (1993) observed that the psocopteran Ectopsocus briggsi helps spread F. oleagineum conidia by carrying them on its body surface or by allowing them to pass without damage through its alimentary canal.
Dispersal of F. eriobotryae conidia was also closely associated with rain. More than 90% of the conidia were collected during rainy periods, and 0.2 mm of rain was sufficient to trigger a dispersal event (González-Domínguez et al., 2014b). A strong aggregation of loquat scab lesions between and within loquat trees also confirmed that F. eriobotryae conidia were mainly splash dispersed (Madden, 1992; González-Domínguez et al., 2014b).
Germination of Conidia
Environmental effects on conidial germination have been studied in vitro for several species (Table 3 and Figure 4). Conidia of all of these species are able to germinate at temperatures between 10 and 30°C, with the exception of F. oleagineum, whose conidia did not germinate at temperatures >25°C (Obanor et al., 2007). Germination at 5°C occurs in all of the species in which it has been tested, i.e., V. inaequalis, V. nashicola, F. effusum, F. oleagineum, and F. eriobotryae (Figure 4). Temperatures >30°C have been tested only for V. inaequalis and F. effusum, whose conidia were able to germinate at 32 and 40°C, respectively (Converse, 1956; Boric, 1985). Optimal germination temperatures are close to 20°C for all of the species, except for V. carpophila and F. effusum, for which the optimum was 25°C.
Conidia of V. inaequalis, V. nashicola, V. carpophila, and F. effusum germinate at 94–99% RH, but germination was higher in free water (Converse, 1956; Lawrence and Zehr, 1982; Machardy, 1996; Li et al., 2003). Conidia of F. oleagineum and F. eriobotryae germinated only in free water (Obanor et al., 2007; González-Domínguez et al., 2013). In free water and at optimal temperatures, V. inaequalis, V. nashicola, and F. effusum began to germinate after 3, 2, and 4 h, respectively, whereas F. oleagineum and F. eriobotryae required 9 and 6 h, respectively. At 10°C in free water, V. inaequalis and V. nashicola began to germinate after 3 and 6 h, respectively, whereas F. oleagineum and F. eriobotryae required at least 12 h (Figure 4; Machardy, 1996; Li et al., 2003; Obanor et al., 2007; González-Domínguez et al., 2013).
Infection by Conidia
The effect of environment on conidial infection has been studied for most of the Venturia spp. considered in this review (Table 3 and Figure 4). For V. carpophila, laboratory experiments have not been performed, and only general requirements were mentioned by Scherm and Brannen (2004). Mills and Laplante (1954) stated that V. inaequalis conidia were able to cause infection in two-thirds of the time required by ascospores. Subsequent laboratory and field studies have been reviewed by Machardy and Gadoury (1989). For conidial infection, the latter authors used the results of Schwabe (1980) to develop curves for minimum requirements of temperature and wetness duration for infection. The curve developed with data from laboratory studies was similar in shape to the Mill's curve but the time required to infect was greater for the Machardy and Gadoury curves than for the Mills curves.
All Venturia spp. are able to infect leaves at temperatures from 10 to 25°C, except for F. eriobotryae, which was unable to infect loquat plants at 25°C (Figure 4). Infection at 5°C was documented for V. inaequalis, V. pyrina, V. nashicola, and F. oleagineum. V. nashicola, V. carpophila, and F. effusum caused infection at 30°C, and F. effusum caused infection at 35°C (Figure 4).
For all of the species considered in this review, the optimal temperature for infection is 20°C, but there are differences in the minimum number of hours with high humidity or wetness required for infection (Figure 4). At 20°C and under continuous wetness, V. nashicola and V. inaequalis are able to infect within 5 and 6 h after inoculation, respectively; V. pyrina and F. effusum require 9 h, and F. oleagineum and F. eriobotryae only cause infection after 12 h of continuous wetness. At 10°C, the number of hours of continuous wetness required for infection ranged from 10 to 12 h for V. inaequalis, V. pyrina, and V. nashicola, 18 for F. oleagineum, and 24 for F. eriobotryae. F. effusum had similar wetness requirements (>2 h) at 10–35°C (Gottwald, 1985). The equation of Magarey et al. (2005) showed a similar behavior for V. inaequalis, V. pyrina, V. nashicola, and F. effusum, in that all four species were able to cause infection with only a few hours of wetness under a wide temperature range; F. eriobotryae and F. oleagineum, in contrast, had stricter requirements for both wetness duration and temperature (Figure 5).
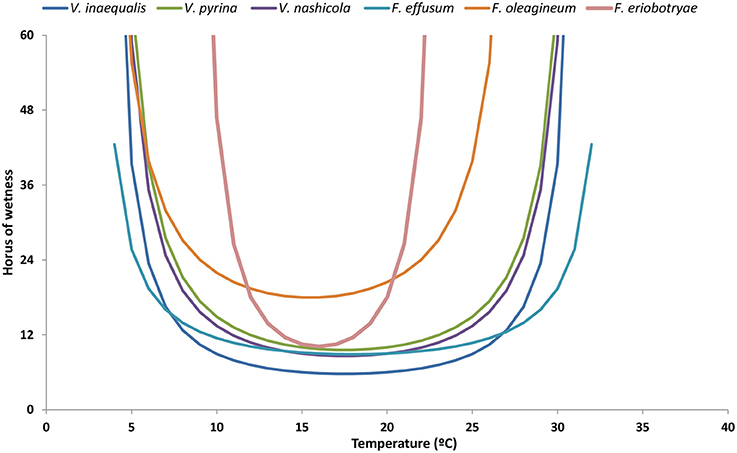
Figure 5. Minimum requirements of temperature and hours of wetness for conidial infection by Venturia spp. The requirements have been modeled by using the equation of Magarey et al. (2005). For each species, the maximum, minimum, and optimal temperatures for infection, and the minimum hours of wetness required are those indicated in Table 4.
Mycelial Growth
Venturia inaequalis, V. carpophila, and F. eriobotryae grow at 10–25°C (Lawrence and Zehr, 1982; Machardy, 1996; González-Domínguez et al., 2013). Venturia inaequalis and F. eriobotryae also grow at 5°C, but this temperature has not been tested for V. carpophila. V. carpophila is able to grow at 30°C (Lawrence and Zehr, 1982). No additional information exists in the literature (Table 3 and Figure 4).
Latency Period
Specific experiments on the effect of environmental conditions on the length of latency period (i.e., the time from infection until the occurrence of sporulating lesions) have been conducted only for V. inaequalis and F. oleagineum (Table 3). This period is shorter for V. inaequalis (ranging from 8 days at 18.6°C to 17 days at 9°C) than for F. oleagineum (60 days at 6°C, 16 days at 16°C and >120 days at 25°C; Mills, 1946; Roubal et al., 2013). For V. inaequalis, incubation at low RH (60–70%) for >9 days lengthened the latency period and lesions did not develop on plants incubated at low RH (Tomerlin and Jones, 1982). For F. oleagineum, leaf age affects the length of the latency period in laboratory experiments; latency ranged from 31 days in young leaves to 64 days in old leaves (Viruega et al., 2011).
Multivariate Analysis of the Epidemiological Components
As part of the current review, a multiple correspondence analysis (CA) was performed on epidemiological components of seven Venturia spp. CA is a multivariate statistical method that makes it possible to represent contingency tables in a pictorial form (Savary et al., 1995). CA is based on a raw data matrix, in which the rows are the objects and the columns are the variables. In this case, the objects are the seven Venturia spp.—V. carpophila, V. inaequalis, V. pyrina, V. nashicola, F. effusum, F. eriobotryae, and F. oleagineum—for which epidemiological information exists for seven qualitative variables and eight quantitative variables (Table 4).
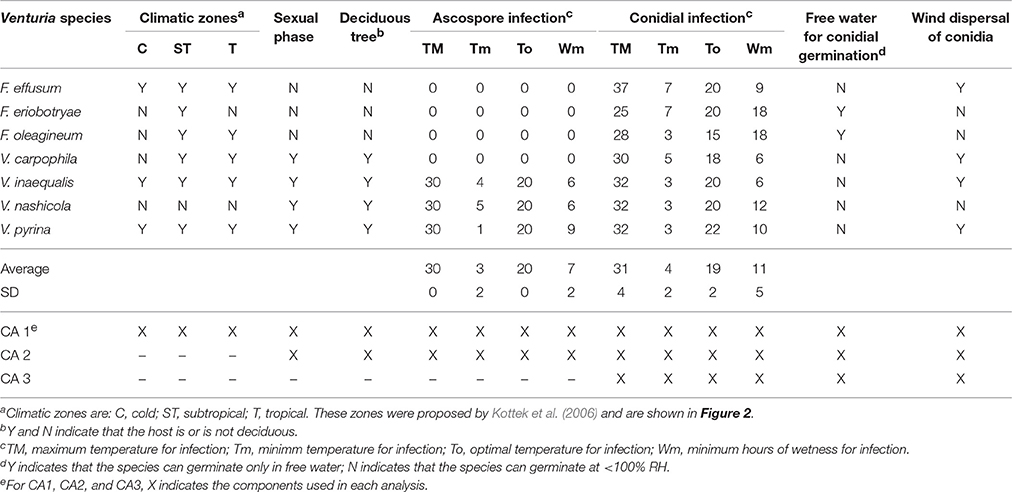
Table 4. Epidemiological components of seven Venturia spp. used for three kinds of correspondence analysis (CA1, CA2, and CA3).
In our case, qualitative variables (e.g., the known presence of the sexual stage in nature) were classified using Yes or No; quantitative variables (e.g., optimal temperature for conidial infection) were ranked as high, medium, or low based on the average ± standard deviation (SD) of each data set. For example, the maximum temperature for conidial infection (TM) of the seven Venturia spp. ranged from 25 to 37°C, with an average of 31°C and a SD of 4. Thus, Venturia spp. in which the maximum temperature for infection was TM ≤ 27°C (i.e., 31–4) were classified as low, those with 27 > TM < 35°C were classified as medium, and those with TM ≥ 35°C were classified as high (Table 4).
The data matrix of Table 4 was used to perform CA with two dimensions (D1 and D2) using the multiple correspondence analysis procedure of SPSS (ver. 23; SPSS Inc.). Three analyses were performed: (i) with all components (CA1); (ii) with all components except those concerning the distribution in different climate types (CA2); and (iii) with only those components concerning the asexual stage (CA3). These analyses accounted for 80.0, 88.5, and 98.2% of data variance, respectively (Table 5).
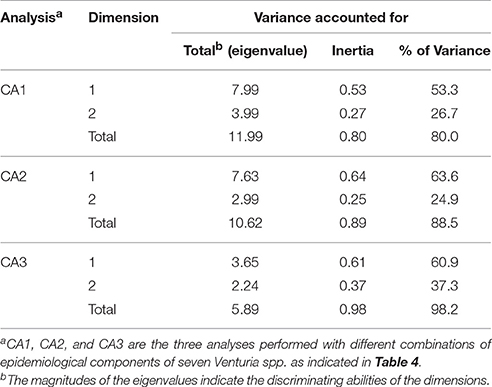
Table 5. Statistics of three correspondence analyses (CA1, CA2, and CA3) performed for seven Venturia species with the data in Table 4.
Overall, these analyses separated the Venturia spp. into two main groups when the seven species were plotted on the D1-D2 space (Figure 6). The first group contained V. inaequalis, V. pyrina, V. nashicola, and V. carpophila, and the second group contained F. oleagineum and F. eriobotryae, with F. effusum having an intermediate position depending on the CA analysis.
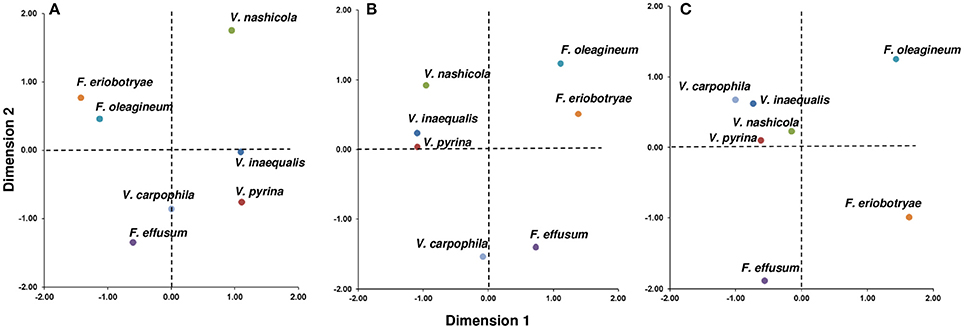
Figure 6. Distribution of seven Venturia spp. based on the environmental requirements of the pathogens and the biological characteristics of the pathogens and their hosts. (A) Joint plot of the correspondence analysis performed with 15 components related to climate and epidemiological variables of the sexual and asexual phase (CA1 in Table 4); (B) Joint plot of the correspondence analysis performed with 12 components related to epidemiological variables of the sexual and asexual phase (CA2 in Table 4); (C) Joint plot of the correspondence analysis performed with six components related to epidemiological variables of the asexual phase (CA3 in Table 4).
In CA1 (Figure 6A), grouping of the species was mainly based on the presence/absence of the sexual stage, infection of deciduous vs. non-deciduous trees, and the thermal and moisture requirements for ascosporic infection. These variables had the most influence (i.e., had high discrimination values, DVs) in D1, which accounted for 53.3% of the variance in the dataset (Table 5); the DV was >0.829 for these variables (Table 6). In CA1, V. nashicola was far from the other species in D2 (Figure 6A), mainly because of its different distribution among climate types (presence/absence of the species in tropical and subtropical climates had DVs = 0.514 and 0.637, respectively; Table 6); the presence/absence of the species in tropical and subtropical climates together with wind dispersal of conidia were the most influential variables in D2 (Table 6). This result may be biased by the distribution of V. nashicola being restricted to China, Japan, South Korea, and Taiwan, a restriction that may be caused more by quarantine measures by other countries than by differences in environmental requirements. This inference is supported by CA2, in which the pathogen distribution among climates was not considered. In CA2 (Figure 6B), the species were grouped mainly based on the presence/absence of the sexual stage and environmental requirements for ascosporic infection in D1 (Table 6), which accounted for 63.6% of the variance (Table 5). In CA3 (Figure 6C), the grouping was mainly determined by moisture conditions for conidial germination, infection, and dispersal in D1 (Table 6), which accounted for 60.9% of the variance (Table 5). The conidia of V. inaequalis, V. pyrina, V. nashicola, and V. carphophila are similar in that they require only a few hours of wetness (6–12 h) to infect and are capable of being dispersed by wind if dislodged by rain or dew. Fusicladium oleagineum and F. eriobotryae require longer periods of wetness to infect (>18 h), have lower maximum temperatures for infection (25–28°C), and have conidia that germinate only in the presence of free water. In CA3, F. oleagineum and F. eriobotryae had similar scores in D1, but they were far apart in D2 (Figure 6C) because of different temperature requirements for conidial infection (Tables 4, 6). Both F. effusum and the Venturia group can infect with <12 h of wetness and have wind-dispersed conidia that do not require free water to germinate; however, the temperature requirements for conidial infection differ between F. effusum and the Venturia group.
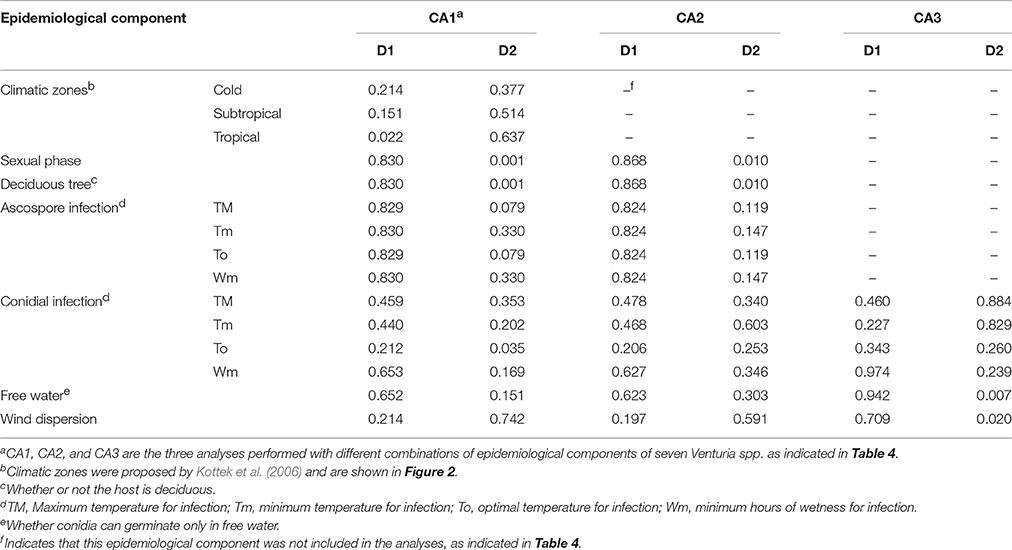
Table 6. Discrimination values (DVs) of the epidemiological components used in different correspondence analyses (CA1, CA2, and CA3) and dimensions (D1 and D2).
The presence of two main groups of species probably reflects pathogen adaptation to host ecophysiology. This hypothesis is supported by the monophyly of the genus Venturia (Ishii and Yanase, 2000; Beck et al., 2005; Gladieux et al., 2010a; Bowen et al., 2011). F. eriobotryae and F. oleagineum are both pathogens of Mediterranean plants (loquat and olive, respectively). They are adapted to a warm and dry climate in which the low annual rainfall is distributed mainly in autumn and spring (Csa climate class; Graniti, 1990; Kottek et al., 2006). The absence of the teleomorph in nature may be related to the mild winter temperatures, which can be survived without a quiescent stage. In these fungi, dispersal of conidia occurs only during rain events, perhaps because conidia dispersed in water have a higher probability of germinating and causing infection. The requirement of free water for conidial germination and long periods of wetness for conidial infection may also be adaptations to a dry climate. These requirements would prevent the initiation of an infection cycle in the driest periods of the year. F. eriobotryae and F. oleagineum also have low mycelium growth rates and long latent periods. The evergreen habitus of their hosts means that these species do not require short infection cycles, because the trees are susceptible throughout the year and because the inoculum may survive on the tree during the season in which environmental conditions are not suitable for sporulation and infection.
Venturia spp. that attack deciduous trees, in contrast, require a sexual stage to survive the winter, when there is no host tissue to be infected and temperatures are low. Their conidia can germinate in the absence of free water, and infection requires fewer hours of wetness, especially in the case of V. inaequalis and V. nashicola. Together, these factors result in relatively short infection cycles, with a higher probability of occurrence. In this case, the deciduous habitus of the host makes the occurrence of infection obligatory, because the main inoculum source for the next season will be the fallen, scabbed leaves, even though overwintering in twig lesions and/or buds is possible.
Conclusions and Implications for Scab Management
This review has considered several important aspects of the phylogeny, host range, and life cycle of Venturia spp. affecting fruit trees. These species are responsible for some of the most important diseases of their hosts.
Venturia spp. affecting fruit trees are highly host-specific, as indicated by the general failure to obtain infection by cross-inoculation (Menon, 1956; Raabe and Gardner, 1972; Ishii and Yanase, 2000; Stehmann et al., 2001; Le Cam et al., 2002; Chevalier et al., 2004; Sánchez-Torres et al., 2007a, 2009; Abe et al., 2008). This conclusion partially contradicts some previous reviews of the genus Venturia (Sivanesan, 1977) and Fusicladium (Schubert et al., 2003), and has implications for the management of scab diseases in areas where different host species are grown, as is the case, for instance, in the Emilia-Romagna region of North Italy where apple, pear, cherries, and peaches are grown in close proximity. The inoculum produced in one crop cannot infect another crop, and management of a species of Venturia in one orchard has no effect on neighboring crops of other host species.
Although Venturia is one of the most famous and studied genera of plant pathogens, important gaps in understanding the life cycle still remain for some species. This is particularly unexpected for V. pyrina because of the worldwide importance of the crop and because the gaps involve key aspects of the life cycle, including pseudothecia formation, ascospore and conidia germination, and mycelial growth. The only work regarding dispersal of V. pyrina conidia was published in 1937 (Kienholz and Childs, 1937). In most cases, researchers have assumed that V. pyrina requirements are similar to those of V. inaequalis. This assumption, however, is not valid for ascosporic infection because ascospores of V. pyrina require more wet hours to infect (Figure 4). In the case of V. carpophila, specific experiments on the requirements for infection have never been performed, and this limits our ability to correctly manage the disease.
Venturia spp. can infect several parts of the host trees, but the main damage usually results from fruit infection, except for F. oleagineum affecting olive leaves, which can result in important economic losses. For this reason, fruit growers are generally risk-adverse to scab diseases and schedule a high number of fungicide applications to achieve a very high level of disease control. Machardy (1996) reported that USA apple growers schedule fungicide applications to achieve <1% of scabbed fruit at harvest. Similar thresholds are usually assumed for loquat, a high value fruit crop in Europe (E. Soler, personal communication). Current trends in disease management aim to avoid this high number of treatments, which involve risks to human health and the environment, and encourage the use of decision support systems (DSSs) (Rossi et al., 2012).
The Mills tables represent one of the first and better known DSSs and have been widely accepted by growers and advisors (Machardy, 1996). However, the use of these tables should be avoided for Venturia spp. other than V. inaequalis. For V. pyrina, F. oleagineum, F. effusum, and F. eriobotryae the Mills tables over-predict the number of scab infections because temperature and wetness requirements of these fungi are different from those of V. inaequalis (Figure 6; Gottwald, 1985; Villalta et al., 2000; Viruega et al., 2011; González-Domínguez et al., 2013). Moreover, the Mills tables can over-predict the number of infections even for apple scab (Machardy and Gadoury, 1989).
For some of the diseases considered in this review, epidemiological models have been developed to predict disease development (Table 7). Most of these models are simple and consider only one component of the pathogen life cycle, mainly ascospore maturation or infection. A main constraint of these models is that they have never been validated against independent data, i.e., model output (the prediction) has not been compared with a data set of real-world observations different from that used for model development (Rossi et al., 2010). Before these models are used in practical disease control, a robust validation with real data should be performed; the validation data should be obtained from different areas with different epidemiological conditions and for several years (Rossi et al., 2010).
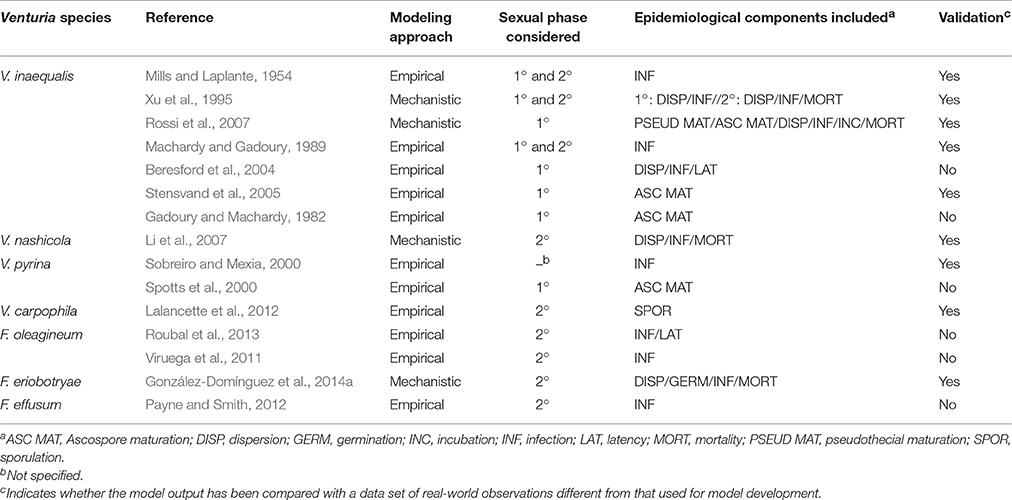
Table 7. Characteristics of the epidemiological models developed for the Venturia spp. considered in this review.
In the case of V. inaequalis, mechanistic weather driven models have been developed for primary infections (Rossi et al., 2007) and for the whole life cycle (Xu et al., 1995). A mechanistic approach has also been used to develop epidemiological models for V. nashicola and F. eriobotryae, and these models consider most of the components of the life cycle (Li et al., 2007; González-Domínguez et al., 2014a). Several advantages have been previously reported for mechanistic vs. empirical models (Caffi et al., 2007; De Wolf and Isard, 2007; Rossi et al., 2010). Mechanistic models attempt to capture the full complexity of the pathogen life cycle and are generally considered to have greater explanatory ability than purely empirical models (De Wolf and Isard, 2007). A main advantage of the mechanistic models is that they can easily incorporate information from previous experiments regarding pathogen biology and epidemiology. Thus, this review should help researchers develop mechanistic models for those scab diseases that currently lack such models. Until such mechanistic models are available, however, the Magarey curves, developed in this review and which identify the requirements for infection, could be used as a starting point to predict infection risk, especially for V. pyrina and F. effusum.
Author Contributions
All the authors contributed to the writing of the manuscript. EG and VR performed the data analyses.
Conflict of Interest Statement
The authors declare that the research was conducted in the absence of any commercial or financial relationships that could be construed as a potential conflict of interest.
Acknowledgments
This work was supported by “Instituto Nacional de Investigaciones Agrarias (INIA)” grant number RTA2013-00004-C03-03, and FEDER Funds.
References
Abe, K., Saito, T., Terai, O., Sato, Y., and Kotobuki, K. (2008). Genotypic difference for the susceptibility of Japanese, Chinese and European pears to Venturia nashicola, the cause of scab on Asian pears. Plant Breed. 127, 407–412. doi: 10.1111/j.1439-0523.2007.01482.x
Acuña, R. P. (2010). Compendio de Bacterias y Hongos de Frutales y Vides en Chile. Santiago de Chile: Servicio Agrícola y Ganadero.
Aylor, D. E., and Anagnostakis, S. L. (1991). Active discharge of ascospores of Venturia inaequalis. Phytopathology 81, 548–551. doi: 10.1094/Phyto-81-548
Beck, A., Ritschel, A., Schubert, K., Braun, U., and Triebel, D. (2005). Phylogenetic relationships of the anamorphic genus Fusicladium s.lat. as inferred by ITS nrDNA data. Mycol. Prog. 4, 111–116. doi: 10.1007/s11557-006-0114-8
Becker, C. M., Burr, T. J., and Smith, C. A. (1992). Overwintering conidia of Venturia inaequalis in apple buds in New York Orchards. Plant Dis. 76, 121–126. doi: 10.1094/PD-76-0121
Ben-Yephet, Y. (1977). Sporulation of Venturia pirina on growth media. Phytoparasitica 5, 109–113. doi: 10.1007/BF02981149
Beresford, R. M., Henshall, W. R., and Palmer, J. W. (2004). A new apple scab risk model that integrates ascospore release, infection risk and susceptible leaf area. New Zeal. Plant Prot. 57, 20–24.
Bock, C. H., Hotchkiss, M. W., Okie, W. R., and Wood, B. W. (2011). The distribution of peach scab lesions on the surface of diseased peaches. Eur. J. Plant Pathol. 130, 393–402. doi: 10.1007/s10658-011-9761-y
Boric, B. (1985). Influence of temperature on germability of spores of Venturia inaequalis (Cooke) Winter, and their viability as affected by age. Zast Bilja 36, 295–302.
Bowen, J. K., Mesarich, C. H., Bus, V. G. M., Beresford, R. M., Plummer, K. I. M. M., and Templeton, M. D. (2011). Venturia inaequalis : the causal agent of apple scab. Mol. Plant Pathol. 12, 105–122. doi: 10.1111/j.1364-3703.2010.00656.x
Braun, U., Ritschel, A., and Schubert, K. (2002). Proposal to conserve the generic name Fusicladium against Spilocaea (Hyphomycetes). Taxon 51:557. doi: 10.2307/1554874
Caffi, T., Rossi, V., Cossu, A., and Fronteddu, F. (2007). Empirical vs. mechanistic models for primary infections of Plasmopara viticola. EPPO Bull. 37, 261–271. doi: 10.1111/j.1365-2338.2007.01120.x
Chevalier, M., Bernard, C., Tellier, M., Audrain, C., and Durel, C. E. (2004). Host and non-host interaction of Venturia inaequalis and Venturia pirina on Pyrus communis and Malus x domestica. Acta Hortic. 663, 205–208. doi: 10.17660/ActaHortic.2004.663.30
Converse, R. H. (1956). The production and germination of Cladosporium effusum in the laboratory. Phytopathology 46:9.
Crous, P. W., Schubert, K., Braun, U., de Hoog, G. S., Hocking, A. D., Shin, H. D., et al. (2007). Opportunistic, human-pathogenic species in the Herpotrichiellaceae are phenotypically similar to saprobic or phytopathogenic species in the Venturiaceae. Stud. Mycol. 58, 185–217. doi: 10.3114/sim.2007.58.07
De Marzo, L., Frisullo, S., Lops, F., and Rossi, V. (1993). Possible dissemination of Spilocaea oleagina conidia by insects (Ectopsocus briggsi). EPPO Bull. 23, 389–391. doi: 10.1111/j.1365-2338.1993.tb01341.x
De Wolf, E. D., and Isard, S. A. (2007). Disease cycle approach to plant disease prediction. Annu. Rev. Phytopathol. 45, 203–220. doi: 10.1146/annurev.phyto.44.070505.143329
Demaree, J. (1924). Pecan scab with special reference to sources of the early spring infections. J. Agric. Res. 28, 321–330.
Eguchi, N., and Yamagishi, N. (2007). Ascospores of the Japanese pear scab fungus (Venturia nashicola Tanaka and Yamamoto) are discharged during the day. J. Gen. Plant Pathol. 74, 41–45. doi: 10.1007/s10327-007-0064-2
Elkins, B., Gubler, W. D., and Adaskaveg, J. E. (2016). UC IPM Pest Management Guidelines: Pear. UC ANR Publication 3455. Available online at: http://ipm.ucanr.edu/PMG/r603100311.html
EPPO (2016). PQR-EPPO Database on Quarantine Pests (database version 5.3.5 of 2015-09-28). Available online at: http://www.eppo.int
Fisher, E. (1961). Venturia carpophila sp. nov., the ascigerous state of the apricot freckle fungus. Trans. Br. mycol. Soc. 44, 337–342. doi: 10.1016/S0007-1536(61)80026-0
Frey, C. N., and Keitt, G. W. (1925). Studies of spore dissemination of Venturia inaequalis in relation to seasonal development of apple scab. Jour. Agric. Res. 30, 529–540.
Gadoury, D. M., and Machardy, W. E. (1982). A model to estimate the maturity of ascospores of Venturia inaequalis. Phytopathology 72, 901–904. doi: 10.1094/Phyto-72-901
Gladieux, P., Caffier, V., Devaux, M., and Le Cam, B. (2010a). Host-specific differentiation among populations of Venturia inaequalis causing scab on apple, pyracantha and loquat. Fungal Genet. Biol. 47, 511–521. doi: 10.1016/j.fgb.2009.12.007
Gladieux, P., Zhang, X. G., Afoufa-Bastien, D., Valdebenito Sanhueza, R. M., Sbaghi, M., and Le Cam, B. (2008). On the origin and spread of the scab disease of apple: out of central Asia. PLoS ONE 3:e1455. doi: 10.1371/journal.pone.0001455
Gladieux, P., Zhang, X. G., Róldan-Ruiz, I., Caffier, V., Leroy, T., Devaux, M., et al. (2010b). Evolution of the population structure of Venturia inaequalis, the apple scab fungus, associated with the domestication of its host. Mol. Ecol. 19, 658–674. doi: 10.1111/j.1365-294X.2009.04498.x
González-Domínguez, E. (2014). Biology, Epidemiology and Control of Fusicladium Eriobotryae, Causal Agent of Loquat Scab. PhD Thesis, Universitat Politècnica de Valencia.
González-Domínguez, E., Armengol, J., and Rossi, V. (2014a). Development and validation of a weather-based model for predicting infection of loquat fruit by Fusicladium eriobotryae. PLoS ONE 9:e107547. doi: 10.1371/journal.pone.0107547
González-Domínguez, E., Rossi, V., Armengol, J., and García-Jiménez, J. (2013). Effect of environmental factors on mycelial growth and conidial germination of Fusicladium eriobotryae, and the infection of loquat leaves. Plant Dis. 97, 1331–1338. doi: 10.1094/PDIS-02-13-0131-RE
González-Domínguez, E., Rossi, V., Michereff, S. J., García-Jiménez, J., and Armengol, J. (2014b). Dispersal of conidia of Fusicladium eriobotryae and spatial patterns of scab in loquat orchards in Spain. Eur. J. Plant Pathol. 139, 849–861. doi: 10.1007/s10658-014-0439-0
González-Lamothe, R., Segura, R., Trapero, A., Baldoni, L., Botella, M., and Valpuesta, V. (2002). Phylogeny of the fungus Spilocaea oleagina, the causal agent of peacock leaf spot in olive. FEMS Microbiol. Lett. 210, 149–155. doi: 10.1111/j.1574-6968.2002.tb11174.x
Gottwald, T. R. (1982). Spore discharge by the pecan scab pathogen, Cladosporium carygenum. Phytopathology 72, 1193–1197. doi: 10.1094/Phyto-72-1193
Gottwald, T. R. (1983). Factors affecting spore liberation by Cladosporium carpophilum. Phytopathology 73, 1500–1505. doi: 10.1094/Phyto-73-1500
Gottwald, T. R. (1985). Influence of temperature, leaf wetness period, leaf age, and spore concentration on infection of pecan leaves by conidia of Cladosporium caryigenum. Phytopathology 75, 190–194. doi: 10.1094/Phyto-75-190
Gottwald, T. R., and Bertrand, P. F. (1982). Patterns of diurnal and seasonal airborne spore concentration of Fusicladium effusum and its impact on a pecan scab epidemic. Phytopathology 72, 330–335.
Graniti, A. (1990). Plant diseases in the Mediterranean region. Phytoparasitica 18, 57–65. doi: 10.1007/BF02980827
Graniti, A. (1993). Olive scab: a review. EPPO Bull. 23, 377–384. doi: 10.1111/j.1365-2338.1993.tb01339.x
Gupta, G. K., and Lele, V. C. (1980). Morphology, physiology and epidemiology of the apple-scab fungus, Venturia inaequalis (Cke.) Wint., in Kashmir Valley. Indian J. Agric. Sci. 50, 51–60.
Hill, S. (1975). The importance of wood scab caused by Venturia inaequalis (Cke.) Wint. as a source of infection for apple leaves in the spring. J. Phytopathol. 82, 216–223.
Hirst, J. M., and Stedman, J. (1961). The epidemiology of apple scab (Venturia inaequalis (Cke.) Wint.). Ann. appl. Biol. 49, 290–305.
Hirst, J. M., and Stedman, O. J. (1962). The epidemiology of apple scab (Venturia inaequalis (Cke.) Wint.) II. Observations on the liberation of ascospores. Ann. Appl. Biol. 50, 525–550. doi: 10.1111/j.1744-7348.1962.tb06047.x
Holb, I. J., Heijne, B., and Jeger, M. J. (2004a). Overwintering of conidia of Venturia inaequalis and the contribution to early epidemics of apple scab. Plant Dis. 88, 751–757. doi: 10.1094/PDIS.2004.88.7.751
Holb, I. J., Heijne, B., Withagen, J. C. M., and Jeger, M. J. (2004b). Dispersal of Venturia inaequalis ascospores and disease gradients from a defined inoculum source. J. Phytopathol. 152, 639–646. doi: 10.1111/j.1439-0434.2004.00910.x
Holz, W. (1937). Enflub des lichtes auf die Perithezienbildung von Venturia inaequalis Aderhold. Zentralbl. Bakt. 95, 469–471.
Ishii, H., and Yanase, H. (2000). Venturia nashicola, the scab fungus of Japanese and Chinese pears: a species distinct from Venturia pirina. Mycol. Res. 104, 755–759. doi: 10.1017/S0953756299001720
Jha, G., Thakur, K., and Thakur, P. (2009). The Venturia apple pathosystem: pathogenicity mechanisms and plant defense responses. J. Biomed. Biotechnol. 2009:680160. doi: 10.1155/2009/680160
Jones, A., and Aldwinckle, H. (1990). Compendium of Apple and Pear Diseases. Saint Paul, MN: APS Press.
Keitt, G. (1917). Peach scab and its control. Bull. Dep. Agric. U.S.A. 395, 1–64. doi: 10.5962/bhl.title.108350
Keitt, G. W., and Jones, I. K. (1926). Studies of the epidemiology and control of apple scab. Wis. Agric. Exp. Stn. Bull. 73, 1–104.
Kienholz, J., and Childs, L. (1937). Twig lesions as a source of early spring infection by the pear scab organism. J. Agric. Res. 55, 667–682.
Kiss, L. (2012). Limits of nuclear ribosomal DNA internal transcribed spacer (ITS) sequences as species barcodes for Fungi. Proc. Natl. Acad. Sci. U.S.A. 109, E1811: author reply E1812. doi: 10.1073/pnas.1207143109
Kodsueb, R., Jeewon, R., Vijaykrishna, D., McKenzie, E., Lumyong, P., Lumyong, S., et al. (2006). Systematic revision of Tubeufiaceae based on morphological and molecular data. Fungal Divers. 21, 105–130.
Kottek, M., Grieser, J., Beck, C., Rudolf, B., and Rubel, F. (2006). World map of the Köppen-Geiger climate classification updated. Meteorol. Zeitschrift 15, 259–263. doi: 10.1127/0941-2948/2006/0130
Kruys, A., Eriksson, O., and Wedin, M. (2006). Phylogenetic relationships of coprophilous Pleosporales (Dothideomycetes, Ascomycota), and the classification of some bitunicate taxa of unknown position. Mycol. Res. 110, 527–536. doi: 10.1016/j.mycres.2006.03.002
Lalancette, N., McFarland, K., and Burnett, L. (2012). Modeling sporulation of Fusicladium carpophilum on nectarine twig lesions: relative humidity and temperature effects. Phytopathology 102, 421–428. doi: 10.1094/PHYTO-08-10-0222
Lan, Z., and Scherm, H. (2003). Moisture sources in relation to conidial dissemination and infection by Cladosporium carpophilum within peach canopies. Phytopathology 93, 1581–1586. doi: 10.1094/PHYTO.2003.93.12.1581
Latham, A. J. (1982). Effect of some weather factors and Fusicladium effusum conidium dispersal on pecan scab occurrence. Phytopathology 72, 1339–1345. doi: 10.1094/Phyto-72-1339
Latorre, B. A., Yañez, P., and Rauld, E. (1985). Factors affectiong release of ascospores by the pear scab fungus (Venturia pirina). Plant Dis. 69, 213–216.
Lawrence, E., and Zehr, E. (1982). Enviromental effects on the development and dissemination of Cladosporium carpophilum on peach. Phytopathology 72, 773–776. doi: 10.1094/Phyto-72-773
Le Cam, B., Parisi, L., and Arene, L. (2002). Evidence of two formae speciales in Venturia inaequalis, responsible for apple and pyracantha scab. Phytopathology 92, 314–320. doi: 10.1094/PHYTO.2002.92.3.314
Li, B. H., Xu, X. M., Li, J. T., and Li, B. D. (2005). Effects of temperature and continuous and interrupted wetness on the infection of pear leaves by conidia of Venturia nashicola. Plant Pathol. 54, 357–363. doi: 10.1111/j.1365-3059.2005.01207.x
Li, B., Zhao, H., and Xu, X. M. (2003). Effects of temperature, relative humidity and duration of wetness period on germination and infection by conidia of the pear scab pathogen (Venturia nashicola). Plant Pathol. 52, 546–552. doi: 10.1046/j.1365-3059.2003.00887.x
Li, B. H., Yang, J. R., Dong, X. L., Li, B. D., and Xu, X. M. (2007). A dynamic model forecasting infection of pear leaves by conidia of Venturia nashicola and its evaluation in unsprayed orchards. Eur. J. Plant Pathol. 118, 227–238. doi: 10.1007/s10658-007-9138-4
Li, G., Zhang, Z., and Lin, S. (2011). Origin and evolution of Eriobotrya. Acta Hortic. 887, 33–37. doi: 10.17660/ActaHortic.2011.887.2
Lian, S., Li, B. H., and Xu, X. M. (2006). Formation and development of pseudothecia of Venturia nashicola. J. Phytopathol. 154, 119–124. doi: 10.1111/j.1439-0434.2006.01072.x
Lian, S., Li, B. H., Dong, X. L., and Xu, X. M. (2007). Effects of environmental factors on discharge and germination of ascospores of Venturia nashicola. Plant Pathol. 56, 402–411. doi: 10.1111/j.1365-3059.2007.01568.x
Lops, F., Frisullo, S., and Rossi, V. (1993). Studies on the spread of the olive scab pathogen, Spilocaea oleagina. EPPO Bull. 23, 385–387. doi: 10.1111/j.1365-2338.1993.tb01340.x
Louw, A. J. (1948). The germination and longevity of spores of the apple-scab fungus, Venturia inaequalis (Cke.) Wint. Union S. Afric. Sci. Bull. 285:19
Machardy, W. E., and Gadoury, D. M. (1989). A revisions of Mills's criteria for predicting apple scab infection periods. Phytopathology 79, 304–310. doi: 10.1094/Phyto-79-304
Magarey, R. D., Sutton, T. B., and Thayer, C. L. (2005). A simple generic infection model for foliar fungal plant pathogens. Phytopathology 95, 92–100. doi: 10.1094/PHYTO-95-0092
Marras, F. (1963). Studio comparativo di quattro ceppi di Fusicladium eriobotryae Cav. di provenienza diversa. Studi. Sassar. 11, 1–37.
Marsh, R. (1933). Observations on pear scab. J. Pomol. Hortic. Sci. 11, 101–112. doi: 10.1080/03683621.1933.11513412
McKirdy, S. J., Mackie, E., and Kumar, S. (2001). Apple scab successfully eradicated in Western Australia. Australas. Plant Pathol. 30, 371. doi: 10.1071/AP01057
Mills, W. (1944). Efficient use of sulfur dusts and sprays during rain to control apple scab. Cornell Ext. Bull. 630, 1–4.
Mills, W. (1946). Effect of temperature on the incubation period of apple scab. N.Y. State Coll. Agric. Weekly News Lett. Insect Pests Plant Dis. 24–25.
Mitcham, E., and Elkins, R. (2007). Pear Production and Handling Manual. California, CA: University of California Publications.
O'Leary, A., and Sutton, T. B. (1986). The influence of temperature and moisture on the quantitative production of pseudothecia of Venturia inaequalis. Phytopathology 76, 199–204.
Obanor, F. O., Walter, M., Jones, E. E., and Jaspers, M. V. (2007). Effect of temperature, relative humidity, leaf wetness and leaf age on Spilocaea oleagina conidium germination on olive leaves. Eur. J. Plant Pathol. 120, 211–222. doi: 10.1007/s10658-007-9209-6
Obanor, F. O., Walter, M., Jones, E. E., and Jaspers, M. V. (2010). Effects of temperature, inoculum concentration, leaf age, and continuous and interrupted wetness on infection of olive plants by Spilocaea oleagina. Plant Pathol. 60, 190–199. doi: 10.1111/j.1365-3059.2010.02370.x
Ogawa, J. M., and English, H. (1991). Diseases of Temperate Zone. Tree Fruit and Nut Crops. California, CA: University of California Publications.
Padder, B. A., Sofi, T. A., Ahmad, M., Shah, M. U. D., Hamid, A., Saleem, S., et al. (2013). Virulence and molecular diversity of Venturia inaequalis in commercial apple growing regions in Kashmir. J. Phytopathol. 161, 271–279. doi: 10.1111/jph.12061
Park, P., Ishii, H., Adachi, Y., Kanematsu, S., Ieki, H., and Umemoto, S. (2000). Infection behavior of Venturia nashicola, the cause of scab on Asian pears. Phytopathology 90, 1209–1216. doi: 10.1094/PHYTO.2000.90.11.1209
Partridge, E. C., and Morgan-Jones, G. (2003). Notes on Hyphomycetes. Fusicladosporium, a new genus or Cladosporium-like anamorphs of Venturia, and the pecan scab-inducing fungus. Mycotaxon 85, 357–370.
Passey, T. A. J., Robinson, J. D., Shaw, M. W., and Xu, X. M. (2017). The relative importance of conidia and ascospores as primary inoculum of Venturia inaequalis in a southeast England orchard. Plant Pathol. doi: 10.1111/ppa.12686. [Epub ahead of print].
Payne, A. F., and Smith, D. L. (2012). Development and evaluation of two pecan scab prediction models. Plant Dis. 96, 1358–1364. doi: 10.1094/PDIS-03-11-0202
Pineau, R., Raymondaud, H., and Schiavon, M. (1991). Elaboration d'un modèle de prévision des risques d'infection du mirabellier (Prunus domestica L var insititia) par l'agent de la tavelure (Cladosporium carpophilum Thumen). Agron. EDP Sci. 11, 561–570. doi: 10.1094/PDIS-03-11-0202-RE
Raabe, R., and Gardner, M. W. (1972). Scab of pyracantha, loquat, toyon and kageneckia. Phytopathology 62, 914–916. doi: 10.1094/Phyto-62-914
Ramos, N. (2008). Relatório de Aplicação de Modelos de Previsão de Ocorrencia de Inimigos das Culturas. Caso do Modelo Para a Cochonilha de S. José – Resultados preliminares. Algarve (Portugal): Direcção Regional de Agricultura e Pescas do Algarve, Patacão. Julho.
Rossi, V., Giosuè, S., and Bugiani, R. (2007). A - scab (Apple-scab), a simulation model for estimating risk of Venturia inaequalis primary infections. EPPO Bull. 37, 300–308. doi: 10.1111/j.1365-2338.2007.01125.x
Rossi, V., Caffi, T., and Salinari, F. (2012). Helping farmers face the increasing complexity of decision-making for crop protection. Phytopathol. Mediterr. 51, 457–479. doi: 10.14601/Phytopathol_Mediterr-11038
Rossi, V., Giosuè, S., and Caffi, T. (2010). “Modelling Plant Diseases for Decision Making in Crop Protection,” in Precision Crop Protection - the Challenge and Use of Heterogeneity, eds E. C. Oerke, R. Gerhards, G. Menz, and R. A. Sikora, (Dordrecht: Springer Netherlands), 241–258.
Rossi, V., Ponti, I., Marinelli, M., Giosuè, S., and Bugiani, R. (2001). Environmental factors influencing the dispersal of Venturia inaequalis ascospores in the orchard air. J. Phytopathol. 149, 11–19. doi: 10.1046/j.1439-0434.2001.00551.x
Rossi, V., Salinari, F., Pattori, E., Giosuè, S., and Bugiani, R. (2009). Predicting the dynamics of ascospore maturation of Venturia pirina based on environmental factors. Phytopathology 99, 453–461. doi: 10.1094/PHYTO-99-4-0453
Roubal, C., Regis, S., and Nicot, P. C. (2013). Field models for the prediction of leaf infection and latent period of Fusicladium oleagineum on olive based on rain, temperature and relative humidity. Plant Pathol. 62, 657–666. doi: 10.1111/j.1365-3059.2012.02666.x
Sánchez-Torres, P., Hinarejos, R., and Tuset, J. J. (2007a). Fusicladium eriobotryae: hongo causante del moteado del níspero en el Mediterráneo español. Bol. San. Veg. Plagas 33, 89–98.
Sánchez-Torres, P., Hinarejos, R., and Tuset, J. J. (2007b). Identification and characterization of Fusicladium eriobotryae: fungal pathogen causing mediterranean loquat scab. Acta Hortic. 33, 343–347. doi: 10.17660/ActaHortic.2007.750.54
Sánchez-Torres, P., Hinarejos, R., and Tuset, J. J. (2009). Characterization and pathogenicity of Fusicladium eriobotryae, the fungal pathogen responsible for loquat scab. Plant Dis. 93, 1151–1157. doi: 10.1094/PDIS-93-11-1151
Savary, S., Madden, L. V., Zadoks, J. C., and Klein-Gebbinck, H. W. (1995). Use of categorical information and correspondence analysis in plant disease epidemiology. Adv. Bot. Res. 21, 214–238. doi: 10.1016/S0065-2296(08)60014-2
Scherm, H., and Brannen, P. M. (2004). “Peach scab,” in Southeastern Peach Grower's Handbook, eds. D. Horton, and D. Johnson (Athens: University of Georgia, College of Agricultural and Environmental Sciences), 134–136.
Scherm, H., Savelle, A. T., Boozer, R. T., and Foshee, W. G. (2008). Seasonal dynamics of conidial production potential of Fusicladium carpophilum on twig lesions in southeastern peach orchards. Plant Dis. 92, 47–50. doi: 10.1094/PDIS-92-1-0047
Schnabel, G., Schnabel, E. L., and Jones, L. (1999). Characterization of ribosomal DNA from Venturia inaequalis and its phylogenetic relationship to rDNA from other tree-fruit Venturia species. Phytopathology 89, 100–108. doi: 10.1094/PHYTO.1999.89.1.100
Schoch, C. L., Seifert, K., Huhndorf, S., Robert, V., Spouge, J. L., Levesque, C. A., et al. (2012). Nuclear ribosomal internal transcribed spacer (ITS) region as a universal DNA barcode marker for Fungi. Proc. Natl. Acad. Sci. U.S.A. 109, 6241–6246. doi: 10.1073/pnas.1117018109
Schoch, C. L., Sung, G. H., López-Giráldez, F., Townsend, J. P., Miadlikowska, J., Hofstetter, V., et al. (2009). The Ascomycota tree of life : a phylum-wide phylogeny clarifies the origin and evolution of fundamental reproductive and ecological traits. Syst. Biol. 58, 224–239. doi: 10.1093/sysbio/syp020
Schubert, K. S., Ritschel, A. R., and Braun, U. B. (2003). A monograph of Fusicladium s. lat. (Hyphomycetes). Schlechtendalia 9, 1–132.
Schwabe, W. F. S. (1980). Wetting and temperature requirements for apple leaf infection by Venturia inaequalis in South Africa. Phytophylactica 12, 69–80.
Schweizer, H. (1958). Contributions to the biology of the pathogen of cherry and peach scab (Fusicladium cerasi (Rabh.) Sacc. Venturia cerasi Ad. and Cladosporium carpophilum v. Thüm). J. Phytopathol. 33, 55–98. doi: 10.1111/j.1439-0434.1958.tb01788.x
Seyran, M., Nischwitz, C., Lewis, K. J., Gitaitis, R. D., Brenneman, T. B., and Stevenson, K. L. (2009). Phylogeny of the pecan scab fungus Fusicladium effusum G. Winter based on the cytochrome b gene sequence. Mycol. Prog. 9, 305–308. doi: 10.1007/s11557-009-0638-9
Sivanesan, A. (1977). The Taxonomy and Pathology of Venturia Species. Bibliotheca Mycologica. Vaduz: Lubrecht and Cramer Ltd.
Sobreiro, J., and Mexia, A. (2000). The simulation of pear scab (Venturia pirina) infection periods and epidemics under field conditions. Acta Hortic. 525, 153–160. doi: 10.17660/ActaHortic.2000.525.18
Spotts, R. A., and Cervantes, L. A. (1994). Factors affecting maturation and release of ascospores of Venturia pirina in Oregon. Phytopathology 84, 260–264. doi: 10.1094/Phyto-84-260
Spotts, R., Cervantes, L., and Niederholzer, F. J. (2000). Pear Scab: components of potential ascospore dose and validation of an ascospore maturity model. Plant Dis. 84, 681–683. doi: 10.1094/PDIS.2000.84.6.681
Stehmann, C., Pennycook, S., and Plummer, K. M. (2001). Molecular identification of a sexual interloper: the pear pathogen, Venturia pirina, has sex on apple. Phytopathology 91, 633–641. doi: 10.1094/PHYTO.2001.91.7.633
Stensvand, A., Amundsen, T., Semb, L., Gadoury, D. M., and Seem, R. C. (1998). Discharge and dissemination of ascospores by Venturia inaequalis during dew. Plant Dis. 82, 761–764. doi: 10.1094/PDIS.1998.82.7.761
Stensvand, A., Eikemo, H., Gadoury, D. M., Seem, R. C., and Norwegian Crop Research Institute, and Plant Protection Centre (2005). Use of a rainfall frequency threshold to adjust a degree-day model of ascospore maturity of Venturia inaequalis. Plant Dis. 89, 198–202. doi: 10.1094/PD-89-0198
Stensvand, A., Gadoury, D. M., Amundsen, T., Semb, L., and Seem, R. C. (1997). Ascospore release and infection of apple leaves by conidia and ascospores of Venturia inaequalis at low temperatures. Phytopathology 87, 1046–1053. doi: 10.1094/PHYTO.1997.87.10.1046
Studt, H. G., and Weltzien, H. C. (1975). Der einfluss der die Konidienbildung beim apfelschorf, Venturia inaequalis (Cooke) Winter. Phytopathol. Z. 84, 115–130. doi: 10.1111/j.1439-0434.1975.tb03553.x
Tanaka, S., and Yamamoto, S. (1964). Studies on pear scab. II. Taxonomy of the causal fungus of Japanese pear scab. Ann. Phytopathol. Soc. Jpn. 29, 128–136. doi: 10.3186/jjphytopath.29.128
Taylor, J. W., Jacobson, D. J., Kroken, S., Kasuga, T., Geiser, D. M., Hibbett, D. S., et al. (2000). Phylogenetic species recognition and species concepts in fungi. Fungal Genet. Biol. 31, 21–32. doi: 10.1006/fgbi.2000.1228
Tenzer, I., and Gessler, C. (1999). Genetic diversity of Venturia inaequalis across Europe. Eur. J. Plant Pathol. 105, 545–552. doi: 10.1023/A:1008775900736
Tomerlin, J. R., and Jones, A. L. (1982). Effect of temperature and relative humidity on the latent period of Venturia inaequalis in apple leaves. Phytopathology 73, 51–54.
Travis, J. W., Rytter, J., and Yoder, K. (2012). Pear Scab (Venturia pirina). Extension Service; West Virginia University.
Umemoto, S. (1990a). Dispersion of ascospores and conidia of causal fungus of japanese pear scab, Venturia nashicola. Ann. Phytopathol. Jpn. Soc. 56, 468–473.
Umemoto, S. (1990b). Infection sources in Japanese pear scab (Venturia nashicola) and their significance in the primary infection. Ann. Phytopathol. Jpn. Soc. 56, 658–664.
Villalta, O., Washington, W. S., Rimmington, G. M., and Machardy, W. E. (2001). Environmental factors influencing maturation and release of ascospores of Venturia pirina in Victoria. Aust. J. Agric. Res. 52, 825–837. doi: 10.1071/AR00093
Villalta, O., Washington, W. S., Rimmington, G. M., and Taylor, P. A. (2000). Effects of temperature and leaf wetness duration on infection of pear leaves by Venturia pirina. Aust. J. Agric. Res. 51, 97–106. doi: 10.1071/AR99068
Viruega, J. R., Moral, J., Roca, L. F., Navarro, N., and Trapero, A. (2013). Spilocaea oleagina in olive groves of southern Spain: survival, inoculum production, and dispersal. Plant Dis. 97, 1549–1556. doi: 10.1094/PDIS-12-12-1206-RE
Viruega, J. R., Roca, L. F., Moral, J., and Trapero, A. (2011). Factors affecting infection and disease development on olive leaves inoculated with Fusicladium oleagineum. Plant Dis. 95, 1139–1146. doi: 10.1094/PDIS-02-11-0126
Wiesmann, R. (1932). Ueberwinterung des Apfelschorfpilzes Fusicladium dendriticum im toten Blatt sowie die Ausbreitung der Sommersporen des Apfelschorfpilzes. Landwirtschaftl Jahrbuch der Schweiz 46, 616–679.
Williamson, C., and Burchill, R. (1974). The perennation and control of pear scab (Venturia pirina Aderh.). Plant Pathol. 23, 67–73. doi: 10.1111/j.1365-3059.1974.tb01828.x
Wilson, E. E. (1928). Studies of the ascigerous stage of Venturia inaequalis (Cke.) Wint. in relation to certain factors environment. Phytopathology 18, 375–417.
Xu, X., Butt, D. J., and Santen, V. A. N. (1995). A dynamic model simulating infection of apple leaves by Venturia inaequalis. Plant Pathol. 44, 865–876. doi: 10.1111/j.1365-3059.1995.tb02746.x
Xu, X., Harvey, N., Roberts, A., and Barbara, D. (2012). Population variation of apple scab (Venturia inaequalis) within mixed orchards in the UK. Eur. J. Plant Pathol. 135, 97–104. doi: 10.1007/s10658-012-0068-4
Xu, X., Roberts, T., Barbara, D., Harvey, N. G., Gao, L., and Sargent, D. J. (2009). A genetic linkage map of Venturia inaequalis, the causal agent of apple scab. BMC Res. Notes 2:163. doi: 10.1186/1756-0500-2-163
Xu, X., Yang, J., Thakur, V., Roberts, A., and Barbara, D. J. (2008). Population variation of apple scab (Venturia inaequalis) isolates from Asia and Europe. Plant Dis. 92, 247–252. doi: 10.1094/PDIS-92-2-0247
Zhang, Y., Crous, P. W., Schoch, C. L., Bahkali, A. H., Guo, L. D., and Hyde, K. D. (2011). A molecular, morphological and ecological re- appraisal of Venturiales-a new order of Dothideomycetes. Fungal Divers. 51, 249–277. doi: 10.1007/s13225-011-0141-x
Zhao, G.-J., Yang, Z.-Q., Chen, X.-P., and Guo, Y.-H. (2011). Genetic relationships among loquat cultivars and some wild species of the genus Eriobotrya based on the internal transcribed spacer (ITS) sequences. Sci. Hortic. 130, 913–918. doi: 10.1016/j.scienta.2011.09.007
Keywords: Fusicladium spp., Spilocaea spp., fruit scab, integrated pest management, multiple correspondence analysis
Citation: González-Domínguez E, Armengol J and Rossi V (2017) Biology and Epidemiology of Venturia Species Affecting Fruit Crops: A Review. Front. Plant Sci. 8:1496. doi: 10.3389/fpls.2017.01496
Received: 18 May 2017; Accepted: 14 August 2017;
Published: 19 September 2017.
Edited by:
Luis Rallo, University of Córdoba, SpainReviewed by:
Carolina Leoni, Instituto Nacional de Investigación Agropecuaria (INIA) Uruguay, UruguayRadu E. Sestras, University of Agricultural Sciences and Veterinary Medicine of Cluj-Napoca, Romania
Copyright © 2017 González-Domínguez, Armengol and Rossi. This is an open-access article distributed under the terms of the Creative Commons Attribution License (CC BY). The use, distribution or reproduction in other forums is permitted, provided the original author(s) or licensor are credited and that the original publication in this journal is cited, in accordance with accepted academic practice. No use, distribution or reproduction is permitted which does not comply with these terms.
*Correspondence: Elisa González-Domínguez, RWxpc2EuR29uemFsZXpEb21pbmd1ZXpAdW5pY2F0dC5pdA==