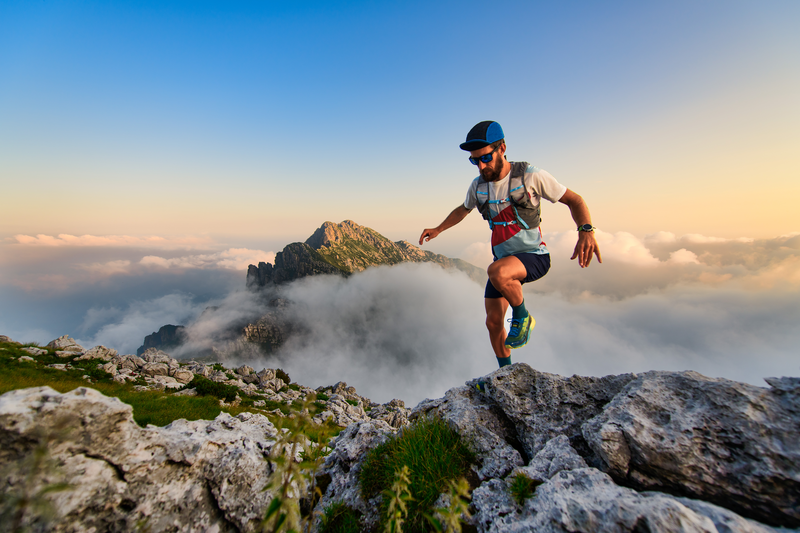
94% of researchers rate our articles as excellent or good
Learn more about the work of our research integrity team to safeguard the quality of each article we publish.
Find out more
MINI REVIEW article
Front. Plant Sci. , 12 April 2017
Sec. Plant Membrane Traffic and Transport
Volume 8 - 2017 | https://doi.org/10.3389/fpls.2017.00556
A correction has been applied to this article in:
Corrigendum: Shoot the Message, Not the Messenger—Combating Pathogenic Virulence in Plants by Inhibiting Quorum Sensing Mediated Signaling Molecules
Immunity, virulence, biofilm formation, and survival in the host environment are regulated by the versatile nature of density dependent microbial cell signaling, also called quorum sensing (QS). The QS molecules can associate with host plant tissues and, at times, cause a change in its gene expression at the downstream level through inter-kingdom cross talking. Progress in controlling QS through fungicide/bactericide in pathogenic microscopic organisms has lead to a rise of antibiotic resistance pathogens. Here, we review the application of selective quorum quenching (QQ) endophytes to control phytopathogens that are shared by most, if not all, terrestrial plant species as well as aquatic plants. Allowing the plants to posses endophytic colonies through biotization will be an additional and a sustainable encompassing methodology resulting in attenuated virulence rather than killing the pathogens. Furthermore, the introduced endophytes could serve as a potential biofertilizer and bioprotection agent, which in turn increases the PAMP- triggered immunity and hormonal systemic acquired resistance (SAR) in plants through SA-JA-ET signaling systems. This paper discusses major challenges imposed by QS and QQ application in biotechnology.
The use of synthetic broad-spectrum fungicides/bactericides in plant disease management results in imbalances within the microbial community and the continuous evolution of multiple bactericide-resistant strains. Microbes with the ability to produce quorum quenching enzymes, which can degrade the wide-spread quorum sensing signals from pathogenic microbes, could be employed in development of sustainable methods of suppressing virulence expression and abolishing bacterial infection. Quorum quenching enzymes produced by endophytes have a more limited selection pressure for microbial survival than biocide treatments (Cirou et al., 2012). Endophytic bacterial growth in plants aids disease control and promotes plant growth (Nowak, 1998; Senthilkumar et al., 2008; Jie et al., 2009; Cirou et al., 2012). Quorum quenching endophytic microbial inocula, primarily bacteria, can be used as propagating priming agents for co-culturing with plant tissues under in vitro conditions. This practice is an emerging trend in biotechnological approaches that harbors unprecedented potential for efficient control over virulent pathogens.
Microbial cell signaling is a precise mechanism involving many factors in play. It is now clear that the transmission of signals from synthesis to sensing depends and varies among organisms and host environments. Virulence-contributing factors like extrapolysaccharide (EPS), degradative exoenzymes, horizontal gene transfer (HGT), (Seitz and Blokesch, 2013), and effectors' secretion are controlled in a cell density-dependent manner in several plant pathogens (Helman and Chernin, 2015). Quorum sensing control of these determinants prevents the early production of factors like EPS, which could interfere with other important processes that govern invasion, such as adhesion (Koutsoudis et al., 2006).
Prokaryotes and eukaryotes have both coexisted and survived for billions of years. During this time period, both were exposed to various signaling molecules produced by each other (Shiner et al., 2005; Hughes and Sperandio, 2008). Although the existence of interkingdom signaling is predictable, the specificity of the ligands and the functions that are regulated are unique to each signaling circuit (Rampioni et al., 2014). Decoding the language taking place between plants and bacteria will be a major challenge for future research due to the numerous and different associations and/or interactions taking place in nature. This article gives a summary of advances in quorum quenching microbial research with a focus on plant-microbe interactions and the impact of QS signal molecules on the cells and tissues of plants.
QS-based microbial cell signaling aids pathogenicity of the most of pathogens (Chevrot et al., 2006; Frederix and Downie, 2011) but also helps in plant growth promotion interaction with plants (Brencic et al., 2005; Soto et al., 2006; Downie, 2010). Acyl homoserine lactone (AHL)-based quorum sensing is present in pathogens as well as many beneficial microbes, such as Methylobacterium (Poonguzhali et al., 2007a,b). Many Gram-negative plant-associated bacterial pathogens have been reported to regulate their virulence by AHL-based QS (Helman and Chernin, 2015). These plant pathogenic bacteria fall within a large number of species among the Pseudomonas and Ralstonia (Mansfield et al., 2012) that cause severe damage to crops.
A major bacterial intercellular signaling system in Gram-negative bacteria is LuxI/R quorum sensing based on the production (via the LuxI-family proteins) and detection (via the LuxR-family proteins) of AHL signaling molecules. Schaefer et al. (2013) screened many genomes in the Proteobacteria taxon for the presence of LuxI and LuxR homologs. Though LuxI and LuxR homolog pairs exist in Alpha-, Beta-, and Gammaproteobacteria, many isolates having LuxI/LuxR were not found to produce AHLs. LuxR proteins that have the same modular structure as LuxRs but are devoid of a cognate LuxI AHL synthase are called solos. LuxR solos have been shown to be responsible to respond to exogenous AHLs and AHLs produced by neighboring cells (Ferluga and Venturi, 2009; Gonzalez and Venturi, 2013). The LuxR-like solo protein OryR transcriptional regulator of Xanthomonas oryzae pv. oryzae interacts with an unknown rice signal molecule (RSM) to activate plant virulence genes (Ferluga and Venturi, 2009). Such LuxR-like solos function as messengers of both interspecies and interkingdom signaling (Gonzalez and Venturi, 2013).
Plants seem to respond differently to AHL-biomolecules, which points to the existance of different receptors or signaling cascades (Götz-Rösch et al., 2015). However, until now, no specific AHL-receptor has been identified in plants. Perez-Montano et al. (2013) reported the existence of AHL-mimic QS molecules in diverse Oryza sativa (rice) and Phaseolus vulgaris (bean) plant samples. These bimolecular analogs bind to signal receptors of bacteria, but they fail to do the signaling activity of AHLs, resulting in confusing bacterial populations. A thorough analysis using biosensors carrying the lactonase enzyme showed that rice and bean seed extracts contain biomolecules that lack lactones' typical ring of AHLs. Although Götz-Rösch et al. (2015) believe that the bacterial AHL molecule might positively influence plant growth, evidence is lacking. However, plant-influenced gene expression in the rice endophyte Burkholderia kururiensis M130 was reported (Coutinho et al., 2015). Captivatingly, these AHL-mimicking molecules specifically alter the QS-regulated biofilm formation of two plant microbes, Sinorhizobium fredii and Pantoea ananatis, suggesting that plants can enhance or inhibit bacterial QS systems depending on the bacterial strain (Perez-Montano et al., 2013). Further studies would contribute to a better understanding of plant-bacteria relationships at the molecular level.
The problem of increasing pathogens resistance to antibiotics/pesticides has prompted the search for phytometabolites with anti-QS activities (Nazzaro et al., 2013; Tan et al., 2013). However, plants have the capacity to produce secondary metabolites in smaller amounts. The considerable amount of natural anti-microbial molecule production in plants is achieved through various methods like the suspension hairy root culture and concentrations of such produced compounds were found to be sufficient for virulence suppression (Ahmad et al., 2014). Similarly, a monoterpenoid phenol carvacol demonstrated QS inhibition in bacteria, which limited biofilm formation and/or chitinase production (Borges et al., 2013; Kerekes et al., 2013; Burt et al., 2014). Phytohormones change plant-microbe interactions by orchestrating host immune responses and modulating microbial virulence traits (Xu et al., 2015). AHLs have evolved to act as interkingdom signals; many plants have been shown to respond to AHLs, which influence and alter plant gene expression (Schuhegger et al., 2006; Ortiz-Castro et al., 2008; Von Rad et al., 2008; Schikora et al., 2011; Schenk and Schikora, 2015; Schikora, 2016). These AHLs promotes plant growth in part by causing a shift in the hormonal balance between indole acetic acid and cytokinin. Long-chain AHLs that are unsubstituted at position C3 have been implicated in the modulation of root development and/or root hair formation; however, the exact mechanisms involved in recognition of microbial and/or synthetic AHLs by plant receptor proteins needs to be functionally validated. AHLs with long lipid chains that are substituted at position C3 with either a ketone or a hydroxyl have been implicated in the induction of resistance against microbial pathogens. Plants have also evolved the ability to affect bacterial AHL-QS systems given that they produce low molecular weight compounds that interfere by acting as agonists or antagonists (Adonizio et al., 2006; Degrassi et al., 2007).
Innate immunity in plants is triggered by PAMPs (pathogen-associated molecular patterns) in response to microbial infection. PAMPs are common in pathogens, non-pathogens, and saprophytes (Jeworutzki et al., 2010; Thomma et al., 2011; De Freitas and Stadnik, 2012; Vidhyasekaran, 2014). Bacterial PAMPs [e.g., certain proteins in bacterial structures and flagella, lipopolysaccharide components, muropeptides, and sugar backbone structures in peptidoglycans, the elf18 epitope in elongation factor Tu (EF-Tu), the CSP22 cold-shock protein, the Ax21 sulfated protein, rhamnolipids, superoxide dismutase (SOD), bacterial DNA, and NEP1-like proteins]; fungal PAMPs [e.g., chitooligosaccharides, ergosterol, the EIX protein, cerebrosides, and NEP1-like proteins]; and oomycete PAMPs [e.g., PEP-13, elicitins, cell wall glucans, the cell wall glycoprotein CBEL with CBD motifs, and NEP1-like proteins] are non-self-response signaling molecules recognized by plant pattern-recognition receptors (PRRs; Nicaise et al., 2009; Tsuda and Katagiri, 2010). Most PRRs are receptor-like kinase (RLK) proteins with a receptor and a signaling domain in the same molecule. In response to PAMPs, PAMP-triggered immunity (PTI) is activated (Bigeard et al., 2015), except when pathogens deliver effector proteins that interfere with PTI signaling to the host plasma membrane. In turn, plants use unique resistance (R) proteins to sense the presence of these effectors in microbes, which triggers effector-triggered immunity (ETI) (Bigeard et al., 2015).
Other intracellular signaling pathways contribute to plant immunity as well. The calcium ion, a regulator of gene expression in plants, is an intracellular second messenger involved in various defense signaling pathways in plants (Galon et al., 2010). Calcium molecules are mainly recognized by calcium sensors, which transduce calcium-mediated signals into downstream events (Hashimoto et al., 2012; Wang et al., 2012). Guanosine triphosphate (GTP)-binding proteins (G-proteins) act as molecular switches in the signal transduction system (Yalowsky et al., 2010; Zhang et al., 2012). Reactive oxygen species (ROS) and nitric oxide (NO) are highly diffusible second messengers that act in cellular signal transduction pathways. Also mitogen-activated protein kinases (MAPKs) form important signaling cascades, which act as a second line of defense in concert with PAMP. MAPKs modules are major pathways downstream of sensors/receptors that transduce extracellular stimuli into intracellular responses in plants (Hettenhausen et al., 2012). In addition to PAMP and MAPK, plant hormones such as salicylic acid (Mukherjee et al., 2010; Dempsey et al., 2011), jasmonate (Sheard et al., 2010; Bertoni, 2012), ethylene (Nambeesan et al., 2012), abscisic acid (Yazawa et al., 2012), auxin (Fu and Wang, 2011), cytokinin (Choi et al., 2011), gibberellins (Qin et al., 2013), and brassinosteroids (Vleesschauwer et al., 2012) play an important role in defense signaling against various pathogens (Vidhyasekaran, 2014). Although microbes employ various defense mechanisms to counter the pathogen attack, these mechanisms fail when pathogens reach a maximum population size.
An effective defense strategy is to block cell-signaling pathways in pathogens to arrest their growth in the host environment. The LuxI and AinS families of Acyl-HSL synthase produce AHL signals using SAM and Acyl-Acp as substrates (Gilson et al., 1995; Parsek et al., 1999). SAM analogs and the SAM biosynthesis inhibitor cycloleucine can inhibit AHL production (Hanzelka and Greenberg, 1996; Parsek et al., 1999). Mutations in AHL biosynthesis genes have direct effect on signal synthesis and biofilm formation. Mutant P. aeruginosa lasI, failing to synthesize 3OXOC12-HSL, forms a flat, unstructured biofilm in a flow cell. Likewise, many other mutants (e.g., B. cenocepacia K56-2 cepI, J2315 cepI, and cciI) are defective when grown in biofilms (Huber et al., 2001; Hentzer and Givskov, 2003; Tomlin et al., 2005; McCarthy et al., 2010; Udine et al., 2013).
Rgg-class proteins are transcriptional regulators on the cytoplasmic membrane that act as receptors for intracellular signaling peptides. They are found in low-G+C-content, Gram-positive bacteria (Firmicutes) communication mediated by peptide molecules (Chang et al., 2011). Domain architecture prediction in Rgg proteins has revealed domains similar to that of another family of regulators (RNPP: Rap, NprR, PlcR, and PrgX) that is responsible for peptide interaction (Cook and Federle, 2014). Recently, cyclosporine, a cyclic peptide compound, was found to curb the activity of Rgg peptide receptors (Parashar et al., 2015). Though they remain crucial windows into peptide-based signaling in Gram-positive bacteria, the synthesis, and processing of Rgg peptides have not been well-studied. Further attention to this field may result in the discovery of new, effective quenching molecules against these peptide receptors.
Autoinducer AI-2 is a common signaling molecule used in both intra- and interspecies communication. It is a furanosyl borate diester molecule, unique due to the presence of boron in its structure (Pereira et al., 2013). Quenching of the AI-2 molecule helps in broad-spectrum control of pathogens (Zhu and Li, 2012; Guo et al., 2013; Pereira et al., 2013), as this molecule acts as a universal language for bacterial interaction. Quenching can be accomplished either by inhibiting signal biosynthesis or by inhibiting signal detection by microbes (LaSarre and Federle, 2013). Different bacterial species sense the AI-2 molecule in different forms, so a single inhibitor cannot be used widely; however, targeting the LuxS protein—which is wholly responsible for the synthesis of AI-2—results in defective signaling and is more effective in controlling a wide range of pathogens. 5'-methylthioadenosine nucleosidase (MTAN) inhibitors play dual roles as quorum quenchers in AI-2 and AHL biosynthesis (LaSarre and Federle, 2013). Halogenated furanoes, such as brominated furanoes derived from the red alga Delisea pulchra, have a direct role in inhibiting AI-2 quorum sensing (Lennen, 2007). Increasing the concentration of in vitro-produced AI-2 has a negative impact on biofilm density (Auger et al., 2006). Several diol-containing compounds (including pyrogallol), boronic acids, and sulfones have been shown to be potent antagonists of AI-2-LuxP binding (Lowery et al., 2005, 2009; Frezza et al., 2006, 2007; Ni et al., 2008, 2009; Peng et al., 2009).
Cross-kingdom interaction leads to specific adjustments and physiological adaptations in colonized eukaryotes (Hartmann and Schikora, 2012). The process by which non-native microbes are introduced into a plant environment is termed biotization. Biotization in the rhizosphere region helps plants obtain more transition metals through siderophore production, which in turn increases plant immunity against phytopathogens. Other evidence also shows that plants with hyper-accumulation of metal have increased resistance to pathogens (Fones and Preston, 2013). In order to successfully colonize a host, microbes undergo several modifications. R. solanacearum appears to alter its membrane architecture in complex ways during adaptation to life in the xylem (Poussier et al., 2003; Brown and Allen, 2004). Thus, culturing of beneficial microbes with plant cells in in vitro conditions can be used for endophytic colonization in plants (Senthilkumar et al., 2008). In plants, endophytes have an advantage over epiphytes, in that they are protected from external growth-limiting factors such as temperature, UV radiation, and osmotic potentials.
Various quorum quenching endophytes that have been identified in plants with AHL-ase activity are presented in Table 1. The main advantage of the artificial introduction of quorum quenching bacteria into plants is that introduced bacteria will occupy most of the intercellular spaces without leaving space for later-invading pathogenic bacteria, as shown in Figure 1. Also, biotization prevents soil bacteria entering into plant tissue (Kung and Almeida, 2014). Though a few pathogenic bacteria enter into the plant system, they remain as avirulent strain due to quorum quenching activity. Virulence-expressing factors are suppressed by AHL-degrading enzymes (Figure 1). Absence of endophytes in the tissues of culture-propagated plants may be related to increased disease severity, an idea supported by Jie et al. (2009).
Table 1. Quorum quenching endophytes that have been identified in plants with experimental evidence.
Figure 1. Behavior and interactions of quorum quenching endophytes with pathogens in both biotized and non-biotized plants. (A) The flow chart explains various methods employed by natural, and/or synthetic quorum quenching molecules to disrupt the bacterial cell signaling (left side) and potential benefits to the plants by introducing non-native endophytes (right side). (B) Interactions of pathogens with biotized plant tissue. The phenomenon explains the virulence activity of pathogens, and antagonistic effect of endophytes over pathogens in pre-biotized and non-biotized plants.
So far there are no universal QQ bacterai to be used for all plants. Also, biotization has been shown to be limited by the absence of AHL-based quorum sensing in Gram-positive bacteria, instead inhibition of Rgg pheromone receptors could be employed (Cook and Federle, 2014). Research has shown that there is a chance that bacteria can evolve resistance to QS-disruption-related control methods (Defoirdt et al., 2010). Hence, a deep understanding of plant-microbe interactions in both biotized and non-biotized plants should be the goal of future research. The challenges of in vitro biotization are summarized in Figure 1.
Biotization helps at various physiological and developmental stages in plants. It enhances induced biotic and abiotic stress resistance (Badosa and Montesinos, 2008; Lugtenberg and Kamilova, 2009; Senthilkumar et al., 2011). Endophytes improve plants' health mainly through siderophore production, thereby enabling biological nitrogen fixation (Ngamau et al., 2012), phosphate solubilization (Andrade et al., 2014), and effective transport of iron (Fe) and zinc (Zn) from the rhizosphere region by ZIP transporters (Krithika and Balachandar, 2016).
The quorum quenching mechanism can serve as a potential target for developing new antimicrobials to overcome microbial pathogenesis. Quorum quenching endophytes will attenuate virulence factors rather than kill the microbes, a feature that should hugely reduce the selective pressures associated with bactericidal agents that have led to the rapid emergence of resistance. Before engineering the quorum sensing pathway in native endophytes, it is important to ensure the presence of naturally available beneficial mechanisms in the host environment. The key to improving plant resistance to bacterial diseases in a changing environment may lie in creating biotized plants. Pesticide poisoning has been acknowledged as a serious problem in many agricultural communities of low- and middle-income countries. Efforts to develop a systematic and a sustainable approach to prevent and manage pesticide poisoning remain inadequate. Thus, the novel possibility of exploiting the quorum quenching endophytes may serve as a sustainable tool for plant disease management.
GA, KA, and MM initiated the project. All the authors have made a substantial, direct, intellectual contribution to the work, and reviewed the final version of the manuscript.
The authors declare that the research was conducted in the absence of any commercial or financial relationships that could be construed as a potential conflict of interest.
Adonizio, A. L., Downum, K., Bennett, B. C., and Mathee, K. (2006). Anti-quorum sensing activity of medicinal plants in southern Florida. J. Ethnopharmacol. 105, 427–435. doi: 10.1016/j.jep.2005.11.025
Ahmad, A., Viljoen, A. M., and Chenia, H. Y. (2014). The impact of plant volatiles on bacterial quorum sensing. Lett. Appl. Microbiol. 60, 8–19. doi: 10.1111/lam.12343
Andrade, L. F., Larisse, G., Dorasio, O., Nietsche, S., Xavier, A. A., Costa, M. R., et al. (2014). Analysis of the abilities of endophytic bacteria associated with banana tree roots to promote plant growth. J. Microbiol. 52, 27–34. doi: 10.1007/s12275-014-3019-2
Auger, S., Krin, E., Aymerich, S., and Gohar, M. (2006). Autoinducer 2 affects biofilm formation by Bacillus cereus. Appl. Environ. Microbiol. 72, 937–941. doi: 10.1128/AEM.72.1.937
Badosa, E., and Montesinos, E. (2008). Growth promotion and biological control of root-knot nematodes in micropropagated banana during the nursery stage by treatment with specific bacterial strains. J. Microbiol. 152, 41–48. doi: 10.1111/j.1744-7348.2007.00189.x
Bertini, E. V., Ana, C. L., and Nieto, P. C. G. (2014). “Endophytic yeasts from sugarcane exhibit quorun quenching activity,” in Congress; X Argentine Congress SAMIGE General Microbiology (Argentina).
Bertoni, I. N. (2012). Oxylipins and plant palatability. Plant Cell 24, 9–10. doi: 10.1105/tpc.112.240412
Bigeard, J., Colcombet, J., and Hirt, H. (2015)., Signaling mechanisms in pattern-triggered immunity (PTI). Mol. Plant 8, 521–539. doi: 10.1016/j.molp.2014.12.022
Borges, A., Serra, S., Cristina Abreu, A., Saavedra, M. J., Salgado, A., and Simões, M. (2013). Evaluation of the effects of selected phytochemicals on quorum sensing inhibition and in vitro cytotoxicity. Biofouling 7014, 1–14. doi: 10.1080/08927014.2013.852542
Brencic, A., Winans, S. C., and Colonization, P. (2005). Detection of and response to signals involved in host-microbe interactions by plant-associated bacteria. Microbiol. Mol. Biol. Rev. 69, 155–194. doi: 10.1128/MMBR.69.1.155
Brown, D. G., and Allen, C. (2004). Ralstonia solanacearum genes induced during growth in tomato: an inside view of bacterial wilt. Mol. Microbiol. 53, 1641–1660. doi: 10.1111/j.1365-2958.2004.04237.x
Burt, S. A., Ojo-Fakunle, V. T. A., Woertman, J., and Veldhuizen, E. J. A. (2014). The natural antimicrobial carvacrol inhibits quorum sensing in chromobacterium violaceum and reduces bacterial biofilm formation at sub-lethal concentrations. PLoS ONE 9:e93414. doi: 10.1371/journal.pone.0093414
Chang, J. C., LaSarre, B., Jimenez, J. C., Aggarwal, C., and Federle, M. J. (2011). Two group a streptococcal peptide pheromones act through opposing rgg regulators to control biofilm development. PLoS Pathog. 7:e1002190. doi: 10.1371/journal.ppat.1002190
Chankhamhaengdecha, S., Hongvijit, S., Srichaisupakit, A., Charnchai, P., and Panbangred, W. (2013). Endophytic actinomycetes : a novel source of potential acyl homoserine lactone degrading enzymes. Biomed. Res. Int. 2013:782847. doi: 10.1155/2013/782847
Chevrot, R., Rosen, R., Haudecoeur, E., Cirou, A., Shelp, B. J., Ron, E., et al. (2006). GABA controls the level of quorum-sensing signal in Agrobacterium tumefaciens. Proc. Natl. Acad. Sci. U.S.A. 103, 7460–7464. doi: 10.1073/pnas.0600313103
Cho, H., Park, S., Ryu, C., Kim, J. F., Kim, J., and Park, S. (2007). Interference of quorum sensing and virulence of the rice pathogen Burkholderia glumae by an engineered endophytic bacterium. FEMS Microb. Ecol. 60, 14–23. doi: 10.1111/j.1574-6941.2007.00280.x
Choi, J., Choi, D., Lee, S., Ryu, C., and Hwang, I. (2011). Cytokinins and plant immunity : old foes or new friends ? Trends Plant Sci. 16, 388–394. doi: 10.1016/j.tplants.2011.03.003
Chu, Y. Y., Nega, M., Wölfle, M., Plener, L., Grond, S., Jung, K., et al. (2013). A new class of quorum quenching molecules from Staphylococcus species affects communication and growth of gram-negative bacteria. PLoS Pathog. 9:e1003654. doi: 10.1371/journal.ppat.1003654
Cirou, A., Mondy, S., An, S., Charrier, A., Sarrazin, A., Thoison, O., et al. (2012). Efficient biostimulation of native and introduced quorum-quenching Rhodococcus erythropolis populations is revealed by a combination of analytical chemistry, microbiology, and pyrosequencing. Appl. Environ. Microbiol. 78, 481–492. doi: 10.1128/AEM.06159-11
Cook, L. C., and Federle, M. J. (2014). Peptide pheromone signaling in Streptococcus and Enterococcus. FEMS Microbiol. Rev. 38, 473–492. doi: 10.1111/1574-6976.12046
Coutinho, B. G., Licastro, D., Mendonça-Previato, L., Cámara, M., and Venturi, V. (2015). Plant-influenced gene expression in the rice endophyte Burkholderia kururiensis M130. Mol. Plant Microbe Interact. 28, 10–21. doi: 10.1094/MPMI-07-14-0225-R
De Freitas, M. B., and Stadnik, M. J. (2012). Physiological and molecular plant pathology race-speci fi c and ulvan-induced defense responses in bean (Phaseolus vulgaris) against Colletotrichum lindemuthianum. Physiol. Mol. Plant Pathol. 78, 8–13. doi: 10.1016/j.pmpp.2011.12.004
Defoirdt, T., Boon, N., and Bossier, P. (2010). Can bacteria evolve resistance to quorum sensing disruption? PLoS Pathog. 6:e1000989. doi: 10.1371/journal.ppat.1000989
Degrassi, G., Devescovi, G., Solis, R., Steindler, L., and Venturi, V. (2007). Oryza sativa rice plants contain molecules that activate different quorum-sensing N-acyl homoserine lactone biosensors and are sensitive to the specific AiiA lactonase. FEMS Microbiol. Lett. 269, 213–220. doi: 10.1111/j.1574-6968.2006.00624.x
Dempsey, D. A., Vlot, A. C., Wildermuth, M. C., and Klessig, D. F. (2011). Salicylic acid biosynthesis and metabolism. Arabidopsis Book 9:e0156. doi: 10.1199/tab.0156
Downie, J. A. (2010). The roles of extracellular proteins, polysaccharides and signals in the interactions of rhizobia with legume roots. FEMS Microbiol. Rev. 34, 150–170. doi: 10.1111/j.1574-6976.2009.00205.x
Ferluga, S., and Venturi, V. (2009). OryR is a LuxR-family protein involved in interkingdom signaling between pathogenic Xanthomonas otyzae pv. oryzae and Rice. J. Bacteriol. 191, 890–897. doi: 10.1128/JB.01507-08
Fones, H., and Preston, G. M. (2013). The impact of transition metals on bacterial plant disease. FEMS Microbiol. Rev. 37, 495–519. doi: 10.1111/1574-6976.12004
Frederix, M., and Downie, A. J. (2011). Quorum sensing: regulating the regulators. Adv. Microb. Physiol. 58, 23–80. doi: 10.1016/B978-0-12-381043-4.00002-7
Frezza, M., Castang, S., Estephane, J., Soulère, L., Deshayes, C., Chantegrel, B., et al. (2006). Synthesis and biological evaluation of homoserine lactone derived ureas as antagonists of bacterial quorum sensing. Bioorg. Med. Chem. 14, 4781–4791. doi: 10.1016/j.bmc.2006.03.017
Frezza, M., Soulère, L., Balestrino, D., Gohar, M., Deshayes, C., Queneau, Y., et al. (2007). Ac2-DPD, the bis-(O)-acetylated derivative of 4,5-dihydroxy-2,3-pentanedione (DPD) is a convenient stable precursor of bacterial quorum sensing autoinducer AI-2. Biorg. Med. Chem. Lett 17, 1428–1431. doi: 10.1016/j.bmcl.2006.11.076
Fu, J., and Wang, S. (2011). Insights into auxin signaling in plant – pathogen interactions. Front. Plant Sci. 2:74. doi: 10.3389/fpls.2011.00074
Galon, Y., Aloni, R., Nachmias, D., Snir, O., and Bouche, N. (2010). Calmodulin-binding transcription activator 1 mediates auxin signaling and responds to stresses in Arabidopsis. Planta 232, 165–178. doi: 10.1007/s00425-010-1153-6
Gilson, L., Kuo, A., and Dunlap, P. V. (1995). AinS and a new family of autoinducer synthesis proteins. J. Bacteriol. 177, 6946–6951.
Gonzalez, J. F., and Venturi, V. (2013). A novel widespread interkingdom signaling circuit. Trends Plant Sci. 18, 167–174. doi: 10.1016/j.tplants.2012.09.007
Götz-Rösch, C., Sieper, T., Fekete, A., Schmitt-Kopplin, P., Hartmann, A., and Schröder, P. (2015). Influence of bacterial N-acyl-homoserine lactones on growth parameters, pigments, antioxidative capacities and the xenobiotic phase II detoxification enzymes in barley and yam bean. Front. Plant Sci. 6:205. doi: 10.3389/fpls.2015.00205
Guo, M., Gamby, S., Zheng, Y., and Sintim, H. O. (2013). Small molecule inhibitors of AI-2 signaling in bacteria: state-of-the-art and future perspectives for anti-quorum sensing agents. Int. J. Mol. Sci. 14, 17694–17728. doi: 10.3390/ijms140917694
Hanzelka, B. L., and Greenberg, E. P. (1996). Quorum sensing in Vibrio fischeri : evidence that S-adenosylmethionine is the amino acid substrate for autoinducer synthesis. Quorum Sensing in Vibrio fischeri : evidence that S -adenosylmethionine is the amino acid substrate for autoinducer synthesis. J. Bacteriol. 178, 5291–5294.
Hartmann, A., and Schikora, A. (2012). Quorum sensing of bacteria and trans-kingdom interactions of N-acyl homoserine lactones with eukaryotes. J. Chem. Ecol. 38, 704–713. doi: 10.1007/s10886-012-0141-7
Hashimoto, K., Eckert, C., Anschu, U., Scholz, M., Held, K., Waadt, R., et al. (2012). Phosphorylation of Calcineurin B-like (CBL) Calcium sensor proteins by their CBL-interacting Protein Kinases (CIPKs) is required for full activity of CBL-CIPK complexes toward their target proteins. J. Biol. Chem. 287, 7956–7968. doi: 10.1074/jbc.M111.279331
Helman, Y., and Chernin, L. (2015). Silencing the mob: disrupting quorum sensing as a means to fight plant disease. Mol. Plant Pathol. 16, 316–329. doi: 10.1111/mpp.12180
Hentzer, M., and Givskov, M. (2003). Pharmacological inhibition of quorum sensing for the treatment of chronic bacterial infections. J. Clin. Invest. 112, 1300–1307. doi: 10.1172/JCI200320074
Hettenhausen, C., Baldwin, I. T., and Wu, J. (2012). Silencing MPK4 in Nicotiana attenuata enhances photosynthesis and seed production but compromises abscisic acid-induced stomatal closure and guard cell-mediated resistance to Pseudomonas syringae. Plant Physiol. 158, 759–776. doi: 10.1104/pp.111.190074
Huber, B., Riedel, K., Hentzer, M., Heydorn, A., Gotschlich, A., Givskov, M., et al. (2001). The cep quorum-sensing system of Burkholderia cepacia H111 controls biofilm formation and swarming motility. Microbiology 147, 2517–2528. doi: 10.1099/00221287-147-9-2517
Hughes, D. T., and Sperandio, V. (2008). Inter-kingdom signalling: communication between bacteria and their hosts. Nat. Rev. Microbiol. 6, 111–120. doi: 10.1038/nrmicro1836
Jeworutzki, E., Roelfsema, M. R. G., Anschu, U., Krol, E., Elzenga, J. T. M., Felix, G., et al. (2010). Early signaling through the Arabidopsis pattern recognition receptors FLS2 and EFR involves Ca 2 + -associated opening of plasma membrane anion channels. Plant J. 62, 367–378. doi: 10.1111/j.1365-313X.2010.04155.x
Jie, L., Zifeng, W., Lixiang, C., Hongming, T., Patrik, I., Zide, J., et al. (2009). Artificial inoculation of banana tissue culture plantlets with indigenous endophytes originally derived from native banana plants. Biol. Control 51, 427–434. doi: 10.1016/j.biocontrol.2009.08.002
Kerekes, E. B., Deák, É., Takó, M., Tserennadmid, R., Petkovits, T., Vágvölgyi, C., et al. (2013). Anti-biofilm forming and anti-quorum sensing activity of selected essential oils and their main components on food-related micro-organisms. J. Appl. Microbiol. 115, 933–942. doi: 10.1111/jam.12289
Koutsoudis, M. D., Tsaltas, D., Minogue, T. D., and Von Bodman, S. B. (2006). Quorum sensing regulation governs bacterial adhesion, biofilm development, and host colonization in Pantoea stewartii subspecies stewartii. Proc. Natl. Acad. Sci. U.S.A. 103, 5983–5988. doi: 10.1073/pnas.0509860103
Krithika, S., and Balachandar, D. (2016). Expression of zinc transporter genes in rice as influenced by zinc-solubilizing Enterobacter cloacae strain ZSB14. Front. Plant Sci. 7:446. doi: 10.3389/fpls.2016.00446
Kung, S. H., and Almeida, R. P. P. (2014). Biological and genetic factors regulating natural competence in a bacterial plant pathogen. Microbiology 160, 37–46. doi: 10.1099/mic.0.070581-0
Kusari, P., Kusari, S., and Lamshöft, M. (2014). Quorum quenching is an antivirulence strategy employed by endophytic bacteria. Appl. Microbiol. Biotechnol. 98, 7173–7183. doi: 10.1007/s00253-014-5807-3
LaSarre, B., and Federle, M. J. (2013). Exploiting quorum sensing to confuse bacterial pathogens. Microbiol. Mol. Biol. Rev. 77, 73–111. doi: 10.1128/MMBR.00046-12
Lennen, R. (2007). Inhibitors of Autoinducer-2 Quorum Sensing and their Effect on Bacterial Biofilm Formation. Michigan: ProQuest.
Lowery, C. A., Abe, T., Park, J., Eubanks, L. M., Sawada, D., Kaufmann, G. F., et al. (2009). Revisiting AI-2 quorum sensing inhibitors: direct comparison of alkyl-DPD analogues and a natural product fimbrolide. J. Am. Chem. Soc. 131, 15584–15585. doi: 10.1021/ja9066783
Lowery, C. A., McKenzie, K. M., Qi, L., Meijler, M. M., and Janda, K. D. (2005). Quorum sensing in Vibrio harveyi: probing the specificity of the LuxP binding site. Bioorg. Med. Chem. Lett. 15, 2395–2398. doi: 10.1016/j.bmcl.2005.02.069
Lugtenberg, B., and Kamilova, F. (2009). Plant-growth-promoting rhizobacteria. Annu. Rev. Microbiol. 63, 541–556. doi: 10.1146/annurev.micro.62.081307.162918
Ma, A., Lv, D., Zhuang, X., and Zhuang, G. (2013). Quorum quenching in culturable phyllosphere bacteria from tobacco. Int. J. Mol. Sci. 14, 14607–14619. doi: 10.3390/ijms140714607
Mansfield, J., Genin, S., Magori, S., Citovsky, V., Sriariyanum, M., Ronald, P., et al. (2012). Top 10 plant pathogenic bacteria in molecular plant pathology. Mol. Plant Pathol. 13, 614–629. doi: 10.1111/j.1364-3703.2012.00804.x
Martin-Rodriguez, A. J., Reyes, F., Martín, J., Pérez-Yépez, J., León-Barrios, M., Couttolenc, A., et al. (2014). Inhibition of bacterial quorum sensing by extracts from aquatic fungi: first report from marine endophytes. Mar. Drugs 12, 5503–5526. doi: 10.3390/md12115503
McCarthy, Y., Yang, L., Twomey, K. B., Sass, A., Tolker-Nielsen, T., Mahenthiralingam, E., et al. (2010). A sensor kinase recognizing the cell-cell signal BDSF (cis-2-dodecenoic acid) regulates virulence in Burkholderia cenocepacia. Mol. Microbiol. 77, 1220–1236. doi: 10.1111/j.1365-2958.2010.07285.x
Mukherjee, M., Larrimore, K. E., Ahmed, N. J., Bedick, T. S., Barghouthi, N. T., Traw, M. B., et al. (2010). Ascorbic acid deficiency in Arabidopsis induces constitutive priming that is dependent on hydrogen peroxide, salicylic acid, and the NPR1 gene. Mol. Plant Microbe. Interact. 23, 340–351. doi: 10.1094/MPMI-23-3-0340
Nambeesan, S., Abuqamar, S., Laluk, K., Mattoo, A. K., Mickelbart, M. V., Ferruzzi, M. G., et al. (2012). Polyamines attenuate ethylene-mediated defense responses to abrogate resistance to Botrytis cinerea in tomato. Plant Physiol. 158, 1034–1045. doi: 10.1104/pp.111.188698
Nazzaro, F., Fratianni, F., De Martino, L., Coppola, R., and De Feo, V. (2013). Effect of essential oils on pathogenic bacteria. Pharmaceuticals 6, 1451–1474. doi: 10.3390/ph6121451
Newman, K. L., Chatterjee, S., Ho, K. A., and Lindow, S. E. (2008). Virulence of plant pathogenic bacteria attenuated by degradation of fatty acid cell-to-cell signaling factors. Mol. Plant Microbe Interact. 21, 326–334. doi: 10.1094/MPMI-21-3-0326
Ngamau, C. N., Matiru, V. N., Tani, A., and Wangari, C. (2012). Isolation and identification of endophytic bacteria of bananas (Musa spp.) in Kenya and their potential as biofertilizers for sustainable banana production. Afr. J. Microbiol. Res. 6, 6414–6422. doi: 10.5897/AJMR12.1170
Ni, N., Choudhary, G., Li, M., and Wang, B. (2008). Pyrogallol and its analogs can antagonize bacterial quorum sensing in Vibrio harveyi. Bioorg. Med. Chem. Lett. 18, 1567–1572. doi: 10.1016/j.bmcl.2008.01.081
Ni, N., Li, M., Wang, J., and Wang, B. (2009). Inhibitors and antagonists of bacterial quorum sensing. Med. Res. Rev. 29, 65–124. doi: 10.1002/med.20145
Nicaise, V., Roux, M., and Zipfel, C. (2009). Recent advances in PAMP-Triggered immunity against bacteria : pattern recognition receptors watch over and raise the alarm. Plant Physiol. 150, 1638–1647. doi: 10.1104/pp.109.139709
Nowak, J. (1998). Benefits of in vitro “biotization” of plant tissue cultures with microbial inoculants. In Vitro Cell. Dev. Biol. Plant 34, 122–130. doi: 10.1007/BF02822776
Ortiz-Castro, R., Martinez-Trujillo, M., and Lopez-Bucio, J. (2008). N-acyl-L-homoserine lactones: a class of bacterial quorum-sensing signals alter post-embryonic root development in Arabidopsis thaliana. Plant Cell Environ. 31, 1497–1509. doi: 10.1111/j.1365-3040.2008.01863.x
Parashar, V., Aggarwal, C., Federle, M. J., and Neiditch, M. B. (2015). Rgg protein structure-function and inhibition by cyclic peptide compounds. Proc. Natl. Acad. Sci. U. S.A. 112, 5177–5182. doi: 10.1073/pnas.1500357112
Parsek, M. R., Val, D. L., Hanzelka, B. L., Cronan, J. E., and Greenberg, E. P. (1999). Acyl homoserine-lactone quorum-sensing signal generation. Proc. Natl. Acad. Sci. U.S.A. 96, 4360–4365. doi: 10.1073/pnas.96.8.4360
Peng, H., Cheng, Y., Ni, N., Li, M., Choudhary, G., Chou, H. T., et al. (2009). Synthesis and evaluation of new antagonists of bacterial quorum sensing in Vibrio harveyi. Chem. Med. Chem. 4, 1457–1468. doi: 10.1002/cmdc.200900180
Pereira, C. S., Thompson, J. A., and Xavier, K. B. (2013). AI-2-mediated signalling in bacteria. FEMS Microbiol. Rev. 37, 156–181. doi: 10.1111/j.1574-6976.2012.00345.x
Perez-Montano, F., Jimenez-Guerrero, I., Contreras Sanchez-Matamoros, R., Lopez-Baena, F. J., Ollero, F. J., Rodriguez-Carvajal, M. A., et al. (2013). Rice and bean AHL-mimic quorum-sensing signals specifically interfere with the capacity to form biofilms by plant-associated bacteria. Res. Microbiol. 164, 749–760. doi: 10.1016/j.resmic.2013.04.001
Polkade, A. V., Mantri, S. S., Patwekar, U. J., and Jangid, K. (2016). Quorum sensing: an under explored phenomenon in the Phylum Actinobacteria. Front. Microbiol. 7:131. doi: 10.3389/fmicb.2016.0013
Poonguzhali, S., Madhaiyan, M., and Sa, T. (2007a). Production of acyl-homoserine lactone quorum-sensing signals is wide-spread in gram-negative Methylobacterium. J. Microbiol. Biotechnol. 17:226.
Poonguzhali, S., Madhaiyan, M., and Sa, T. (2007b). Quorum-sensing signals produced by plant-growth promoting Burkholderia strains under in vitro and in planta conditions. Res. Microbiol. 158, 287–294. doi: 10.1016/j.resmic.2006.11.013
Poussier, S., Thoquet, P., Trigalet-Demery, D., Barthet, S., Meyer, D., Arlat, M., et al. (2003). Host plant-dependent phenotypic reversion of Ralstonia solanacearum from non-pathogenic to pathogenic forms via alterations in the phcA gene. Mol. Microbiol. 49, 991–1003. doi: 10.1046/j.1365-2958.2003.03605.x
Qin, X., Liu, J. H., Zhao, W. S., Chen, X. J., Guo, Z. J., and Peng, Y. L. (2013). Gibberellin 20-oxidase gene OsGA20ox3 regulates plant stature and disease development in rice. Mol. Plant Microbe Interact. 26, 227–239. doi: 10.1094/MPMI-05-12-0138-R
Rajesh, P. S., and Rai, V. R. (2013). Biocatalysis and agricultural biotechnology hydrolytic enzymes and quorum sensing inhibitors from endophytic fungi of Ventilago madraspatana Gaertn. Biocatal. Agric. Biotechnol. 2, 120–124. doi: 10.1016/j.bcab.2013.01.002
Rajesh, P. S., and Rai, V. R. (2014). Quorum quenching activity in cell-free lysate of endophytic bacteria isolated from Pterocarpus santalinus Linn., and its effect on quorum sensing regulated biofilm in Pseudomonas aeruginosa PAO1. Microbiol. Res. 169, 561–569. doi: 10.1016/j.micres.2013.10.005
Rajesh, P. S., and Rai, V. R. (2016). Inhibition of QS-regulated virulence factors in Pseudomonas aeruginosa PAO1 and Pectobacterium carotovorum by AHL-lactonase of endophytic bacterium Bacillus cereus VT96. Biocatal. Agric. Biotechnol. 7, 154–163. doi: 10.1016/j.bcab.2016.06.003
Rampioni, G., Leoni, L., and Williams, P. (2014). The art of antibacterial warfare: deception through interference with quorum sensing-mediated communication. Bioorg. Chem. 55, 60–68. doi: 10.1016/j.bioorg.2014.04.005
Schaefer, A. L., Lappala, C. R., Morlen, R. P., Pelletier, D. A., Lu, T.-Y. S., Lankford, P. K., et al. (2013). LuxR- and LuxI-Type quorum-sensing circuits are prevalent in members of the populus deltoides microbiome. Appl. Environ. Microbiol. 79, 5745–5752. doi: 10.1128/aem.01417-13
Schenk, S. T., and Schikora, A. (2015). AHL-priming functions via oxylipin and salicylic acid. Front. Plant Sci. 5:784. doi: 10.3389/fpls.2014.00784
Schikora, A. (2016). Beneficial effects of bacteria-plant communication based on quorum sensing molecules of the N -acyl homoserine lactone group. Plant Mol. Biol. 90, 605–612. doi: 10.1007/s11103-016-0457-8
Schikora, A., Schenk, S., Stein, E., Molitor, A., Zuccaro, A., and Kogel, K.-H. (2011). N-acyl-homoserine lactone confers resistance toward biotrophic and hemibiotrophic pathogens via altered activation of AtMPK6. Plant Physiol. 157, 1407–1418. doi: 10.1104/pp.111.180604
Schuhegger, R., Ihring, A., Gantner, S., Bahnweg, G., Knappe, C., Vogg, G., et al. (2006). Induction of systemic resistance in tomato by N-acyl-L-homoserine lactone-producing rhizosphere bacteria. Plant Cell Environ. 29, 909–918. doi: 10.1111/j.1365-3040.2005.01471.x
Seitz, P., and Blokesch, M. (2013). Cues and regulatory pathways involved in natural competence and transformation in pathogenic and environmental Gram-negative bacteria. FEMS Microbiol. Rev. 37, 336–363. doi: 10.1111/j.1574-6976.2012.00353.x
Senthilkumar, M., Anandham, R., Madhaiyan, M., Venkateswaran, V., and Sa, T. (2011). “Endophytic bacteria: perspectives and applications in agricultural crop production,” in Bacteria in Agrobiology: Crop Ecosystems, ed D. K. Maheshwari (Dordrecht; Heidelberg; New York, NY; London: Springer), 61–96.
Senthilkumar, M., Madhaiyan, M., Sundaram, S. P., Sangeetha, H., and Kannaiyan, S. (2008). Induction of endophytic colonization in rice (Oryza sativa L.) tissue culture plants by Azorhizobium caulinodans. Biotechnol. Lett. 30, 1477–1487. doi: 10.1007/s10529-008-9693-6
Sheard, L. B., Tan, X., Mao, H., Withers, J., Ben-nissan, G., Hinds, T. R., et al. (2010). Jasmonate perception by. Nature 468, 400–405. doi: 10.1038/nature09430
Shiner, E. K., Rumbaugh, K. P., and Williams, S. C. (2005). Interkingdom signaling: deciphering the language of acyl homoserine lactones. FEMS Microbiol. Rev. 29, 935–947. doi: 10.1016/j.femsre.2005.03.001
Soto, M. J., Sanjuán, J., and Olivares, J. (2006). Rhizobia and plant-pathogenic bacteria: common infection weapons. Microbiology 152, 3167–3174. doi: 10.1099/mic.0.29112-0
Tan, L. Y., Yin, W. F., and Chan, K. G. (2013). Piper nigrum, Piper betle and Gnetum gnemon–natural food sources with anti-quorum sensing properties. Sensors 13, 3975–3985. doi: 10.3390/s130303975
Thomma, B. P. H. J., Nu, T., and Joosten, M. H. A. J. (2011). Of PAMPs and effectors : the blurred PTI-ETI dichotomy. Plant Cell 23, 4–15. doi: 10.1105/tpc.110.082602
Tomlin, K. L., Malott, R. J., Ramage, G., Storey, D. G., Sokol, P. A., and Ceri, H. (2005). Quorum-sensing mutations affect attachment and stability of Burkholderia cenocepacia biofilms quorum-sensing mutations affect attachment and stability of Burkholderia cenocepacia biofilms. Appl. Environ. Microbiol. 71, 5208–5218. doi: 10.1128/AEM.71.9.5208
Tsuda, K., and Katagiri, F. (2010). Comparing signaling mechanisms engaged in pattern-triggered and effector-triggered immunity. Curr. Opin. Plant Biol. 13, 459–465. doi: 10.1016/j.pbi.2010.04.006
Udine, C., Brackman, G., Bazzini, S., Buroni, S., van Acker, H., Pasca, M. R., et al. (2013). Phenotypic and genotypic characterisation of Burkholderia cenocepacia J2315 mutants affected in homoserine lactone and diffusible signal factor-based quorum sensing systems suggests interplay between both types of systems. PLoS ONE 8:e55112. doi: 10.1371/journal.pone.0055112
Uroz, S., and Heinonsalo, J. (2008). Degradation of N-acyl homoserine lactone quorum sensing signal molecules by forest root-associated fungi. FEMS Microbiol. Ecol. 65, 271–278. doi: 10.1111/j.1574-6941.2008.00477.x
Vidhyasekaran, P. (2014). “PAMP signals in plant innate immunity: signal perception and transduction,” in Signaling and Communication in Plants, Vol. 21, ed F. Baluška (Dordrecht; Heidelberg; New York, NY; London: Springer), 17–161.
Vleesschauwer, D., Van Buyten, E., Satoh, K., Balidion, J., Mauleon, R., Choi, I. R., et al. (2012). Brassinosteroids antagonize gibberellin-and salicylate- mediated root immunity in rice. Plant Physiol. 1581. 1833–1846. doi: 10.1104/pp.112.193672
Von Rad, U., Klein, I., Dobrev, P. I., Kottova, J., Zazimalova, E., Fekete, A., et al. (2008). Response of Arabidopsis thaliana to N-hexanoyl-DL-homoserine-lactone, a bacterial quorum sensing molecule produced in the rhizosphere. Planta 229, 73–85. doi: 10.1007/s00425-008-0811-4
Wang, Z., Tan, X., Zhang, Z., Gu, S., Li, G., and Shi, H. (2012). Plant science defense to Sclerotinia sclerotiorum in oilseed rape is associated with the sequential activations of salicylic acid signaling and jasmonic acid signaling. Plant Sci. 184, 75–82. doi: 10.1016/j.plantsci.2011.12.013
Xu, J., Zhou, L., Venturi, V., He, Y.-W., Kojima, M., Sakakibari, H., et al. (2015). Phytohormone-mediated interkingdom signaling shapes the outcome of rice-Xanthomonas oryzae pv. oryzae interactions. BMC Plant Biol. 15:10. doi: 10.1186/s12870-014-0411-3
Yalowsky, S., Baluska, F., and Jones, A. (2010). Integrated G proteins Signaling in Plants. Heidelberg: Springer.
Yazawa, K., Jiang, C., Kojima, M., Sakakibara, H., and Takatsuji, H. (2012). Physiological and molecular plant pathology reduction of abscisic acid levels or inhibition of abscisic acid signaling in rice during the early phase of Magnaporthe oryzae infection decreases its susceptibility to the fungus. Physiol. Mol. Plant Pathol. 78, 1–7. doi: 10.1016/j.pmpp.2011.12.003
Zhang, H., Gao, Z., Zheng, X., and Zhang, Z. (2012). The role of G-proteins in plant immunity. Plant Signal. Behav. 7, 1284–1288. doi: 10.4161/psb.21431
Keywords: biotization, Quorum sensing, pesticide poisoning, endophytes, Quorum quenching
Citation: Alagarasan G, Aswathy KS and Madhaiyan M (2017) Shoot the Message, Not the Messenger—Combating Pathogenic Virulence in Plants by Inhibiting Quorum Sensing Mediated Signaling Molecules. Front. Plant Sci. 8:556. doi: 10.3389/fpls.2017.00556
Received: 10 January 2017; Accepted: 28 March 2017;
Published: 12 April 2017.
Edited by:
Gerald Alan Berkowitz, University of Connecticut, USAReviewed by:
Tamara Pecenkova, Institute of Experimental Botany (ASCR), CzechiaCopyright © 2017 Alagarasan, Aswathy and Madhaiyan. This is an open-access article distributed under the terms of the Creative Commons Attribution License (CC BY). The use, distribution or reproduction in other forums is permitted, provided the original author(s) or licensor are credited and that the original publication in this journal is cited, in accordance with accepted academic practice. No use, distribution or reproduction is permitted which does not comply with these terms.
*Correspondence: Ganesh Alagarasan, YWxhZ2FyYXNhbi5nYW5lc2hAaG90bWFpbC5jb20=
Disclaimer: All claims expressed in this article are solely those of the authors and do not necessarily represent those of their affiliated organizations, or those of the publisher, the editors and the reviewers. Any product that may be evaluated in this article or claim that may be made by its manufacturer is not guaranteed or endorsed by the publisher.
Research integrity at Frontiers
Learn more about the work of our research integrity team to safeguard the quality of each article we publish.