- 1Key Laboratory of Resource Biology and Biotechnology in Western China (Ministry of Education), College of Life Sciences, Northwest University, Xi'an, China
- 2United States Department of Agriculture Forest Service Hardwood Tree Improvement and Regeneration Center, Department of Forestry and Natural Resources, Purdue University, West Lafayette, IN, USA
Juglans L. (walnuts and butternuts) is an economically and ecologically important genus in the family Juglandaceae. All Juglans are important nut and timber trees. Juglans regia (Common walnut), J. sigillata (Iron walnut), J. cathayensis (Chinese walnut), J. hopeiensis (Ma walnut), and J. mandshurica (Manchurian walnut) are native to or naturalized in China. A strongly supported phylogeny of these five species is not available due to a lack of informative molecular markers. We compared complete chloroplast genomes and determined the phylogenetic relationships among the five Chinese Juglans using IIumina sequencing. The plastid genomes ranged from 159,714 to 160,367 bp encoding 128 functional genes, including 88 protein-coding genes and 40 tRNA genes each. A complete map of the variability across the genomes of the five Juglans species was produced that included single nucleotide variants, indels (insertions and deletions), and large structural variants, as well as differences in simple sequence repeats (SSR) and repeat sequences. Molecular phylogeny strongly supported division of the five walnut species into two previously recognized sections (Juglans/Dioscaryon and Cardiocaryon) with a 100% bootstrap (BS) value using the complete cp genomes, protein coding sequences (CDS), and the introns and spacers (IGS) data. The availability of these genomes will provide genetic information for identifying species and hybrids, taxonomy, phylogeny, and evolution in Juglans, and also provide insight into utilization of Juglans plants.
Introduction
The estimate of phylogenetic relationships plays a key role in understanding evolution and has been an essential component of evolutionary biology. In plants, much effort in reconstructing the Tree of Life has focused on the relationships of major clades, and significant advances have been made above the order or family levels (The Angiosperm Phylogeny Group III, 2009; Soltis et al., 2011). Until recently, progress in inferring phylogenetic relationships at lower taxonomic levels and among recently diverged species has been less encouraging, especially for species-rich, morphologically diverse lineages (Waterway et al., 2009). In the past few years, however, important advances have been made in multispecies coalescent approaches for resolving genome-level relationships among closely related species using next generation sequencing to resolve incomplete lineage sorting and inter-lineage hybridization (Huang et al., 2014; Carbonell-Caballero et al., 2015; Daniell et al., 2016).
Walnuts and butternuts (Juglans) are known for their edible nuts and high-quality wood (Manning, 1978; Aradhya et al., 2007). The genus Juglans includes about 21 species distributed in Asia, southern Europe, North America, Central America, western South America, and the West Indies (Manning, 1978; Stanford et al., 2000; Aradhya et al., 2007). Species of Juglans are diploid, with a karyotype of 2n = 2x = 32 (Woodworth, 1930; Komanich, 1982). J. regia (common walnut), J. sigillata (iron walnut), J. cathayensis (Chinese walnut), J. hopeiensis (Ma walnut), and J. mandshurica (Manchurian walnut) grow in China (Manning, 1978; Fjellstrom and Parfitt, 1995; Aradhya et al., 2007). Juglans is taxonomically and phylogenetically challenging. Classical taxonomy divides the genus into four sections (sect. Dioscaryon, sect. Cardiocaryon, sect. Trachycaryon, and sect. Rhysocaryon) mainly based on species' geographical distribution, leaf, flower, and fruit morphology (Dode, 1909; Manning, 1978). Molecular evidence, however, including sequence data from the internal transcribed spacer (ITS), five chloroplast DNA spacer sequences (atpB-rbcL, psbA-trnH, trnS-trnfM, trnT-trnF, and trnV-16S rRNA), a hyper-variable matK, and restriction fragment length polymorphisms (RFLPs), has been interpreted as supporting three or four sections (Fjellstrom and Parfitt, 1995; Stanford et al., 2000; Aradhya et al., 2007).
Chinese Juglans species are divided into two sections (sect. Dioscaryon and sect. Cardiocaryon). Common walnut (J. regia) and Iron walnut (J. sigillata) belong to sect. Dioscaryon, and the other three species (J. cathayensis, J. hopeiensis, and J. mandshurica) belong to sect. Cardiocaryon (Dode, 1909; Fjellstrom and Parfitt, 1995; Stanford et al., 2000; Aradhya et al., 2007). Common walnut (J. regia) is native to the mountainous regions of central Asia (Pollegioni et al., 2015), while Iron walnut (J. sigillata) is indigenous to China, and distributed mainly in southwestern China (Wang et al., 2015). Chinese walnut (J. cathayensis) is widely distributed in southern China (Bai et al., 2014; Dang et al., 2015), while J. mandshurica is mainly distributed in northern China, northeast China, and the Korean Peninsula (Wang et al., 2016). J. hopeiensis is narrowly distributed in northern China in the hilly, mid-elevation area between Hebei province, Beijing, and Tianjin (Hu et al., 2015). A strongly supported phylogeny of these five species is not available due to a lack of informative molecular markers (Fjellstrom and Parfitt, 1995; Stanford et al., 2000; Aradhya et al., 2007). Studies of gene flow and introgression have concluded J. regia and J. sigillata are particularly closely related, and some have questioned whether they are distinct (Wang et al., 2008, 2015). Aradhya et al. (2007) used ITS, RFLP, and cpDNA sequence data to suggest J. regia and J. sigillata are distinct species. J. cathayensis and J. mandshurica were combined into one species in Flora of China (English version) (Lu et al., 1999), which does not consider J. hopeinesis (Kuang and Lu, 1979; Aradhya et al., 2004, 2007) a valid taxon. In addition, some previous phylogenetic studies of Juglans omitted J. hopeiensis and J. sigillata (Fjellstrom and Parfitt, 1995; Stanford et al., 2000; Aradhya et al., 2007). Thus, the phylogeny and systematics of the five Chinese walnut (Juglans) species is uncertain.
In this study, we combined de novo and reference-guided assembly of five Chinese walnut (Juglans) species' whole chloroplast genomes (Cpgs). This is the first comprehensive Cpg analysis of multiple Juglans species. Our aims were: (1) to investigate global structural patterns of whole chloroplast genome of five Juglans species including genome structure, gene order, and gene content; (2) to examine variations of simple sequence repeats (SSRs) and large repeat sequence in the whole Cpgs of Juglans; (3) to identify divergence hotspots as regions potentially under selection pressure; and (4) to construct a chloroplast phylogeny for the five Chinese Juglans species using their whole cp DNA sequences, protein coding sequences, and the introns and spacers.
Materials and Methods
Taxon Sampling, Plant Material, and Deposition of Voucher
Fresh leaves of four Juglans species were collected from different mountains in China, including a J. mandshurica tree growing in the Xiaolongmen National Forest Park, a J. sigillata tree from Lijiang, Yunan, a J. hopeiensis tree growing Laishui, Beijing, and a J. cathayensis tree growing in the Qingling Mountains (Table 1). The leaves were dried in silica gel and stored at −4°C. The leaves of J. regia were collected fresh from a tree growing the orchard of Northwest University, Shaanxi, China. Voucher specimens of each of the sampled trees were deposited at the herbarium of Northwest University, Xi'an, China. All the DNA samples were stored at Evolutionary Botany Lab, Northwest University, Xi'an, China. High-quality genomic DNA was extracted using a modified CTAB method (Zhao and Woeste, 2011). The DNA concentration was quantified using a NanoDrop spectrophotometer (Thermo Scientific, Carlsbad, CA, USA). The final DNA concentration >30 ng μL−1 were chosen for further Illumina sequencing. We sequenced the complete chloroplast genome of J. regia with the Illumina MiSeq sequencing platform (Sangon Biotech, Shanghai, China). We assembled the chloroplast genomes using SPAdes v3.6.2 (Bankevich et al., 2012) (http://bioinf.spbau.ru/spades) and annotated them with CpGAVAS (http://www.biomedcentral.com/1471-2164/13/715) (Liu et al., 2012a; Hu et al., 2016). We sequenced the complete Cpg of four Juglans species using Illumina HiSeq 2500 sequencing technology via a combination of de novo and reference-guided assembly based on the Cpg of J. regia (Hu et al., 2016, NCBI Accession number: KT963008). A paired-end (PE) library with 350-bp insert size was constructed using the Illumina PE DNA library kit according to the manufacturer's instructions and sequenced using an Illumina Hiseq2500 by Novogene (http://www.novogene.com, China).
Chloroplast Genome Sequencing, Assembly, and Gap Filling
Raw reads with sequences shorter than 50 bp or with more than the allowed maximum percentage of ambiguous bases (2%) were removed from the total NGS PE reads using the NGSQC toolkit v2.3.3 (Patel and Jain, 2012) trim tool. After trimming, high-quality PE reads were assembled using MIRA v4.0.2 (Chevreux et al., 2004) assembler. Then, to further assemble the Cpg, some ambiguous regions were picked out for extension with a baiting and iteration method based on MITObim v1.8 (Hahn et al., 2013). A de novo assembly strategy combined with a reference-based assembly allowed us to reconstruct each Cpg. Reads were then remapped to references for each taxon to check for mis-assemblies or rearrangements using Geneious v8.0.2 (http://www.Geneious.com; Kearse et al., 2012) and reads matching the draft reference were assembled de novo, also in Geneious, using suggested settings. Inverted repeat boundaries were determined and verified by remapping reads in Geneious. Lastly, primers were developed with Primer3 (Untergrasser et al., 2012) to close low coverage gaps between contigs (for a few single end datasets). Small gaps in the assemblies were bridged by designing custom primers for PCR (Table S1) based on their flanking sequences, followed by conventional Sanger sequencing. The PCR primers were designed using J. regia sequences when they appeared identical to our original de novo assembly (Hu et al., 2016). Eleven primer pairs were used to validate junctions using PCR based sequencing in each of five Juglans Cpgs. PCR amplification was carried out on a SimpliAmp Thermal Cycler (Applied Biosystem, USA) in 20 μL reaction volumes (10 μL 2 × PCR Master Mix including 0.1 U Taq polymerase/μL; 500 μM each dNTP; 20 mM Tris-HCl (pH 8.3); 100 mM KCl; 3.0 mM MgCl2 (Tiangen, Beijing, China), 0.5 μL each primer, 2 μL BSA, 2 μL of 10 ng/μL DNA). The PCR was programmed for 3 min at 94°C followed by 35 cycles of 15 s at 93°C, 1 min at annealing temperature (60°C), 30 s at 72°C and extension of 10 min at 72°C. After PCR amplification, fragments were sequenced by Sangon Biotech (Shanghai, China). All newly generated sequences were deposited in GenBank (Table S1).
Genome Annotation and Analysis
The completed genome sequences were imported into the online program Dual Organellar Genome Annotator (DOGMA, Wyman et al., 2004) for annotation, coupled with manual investigation of the positions of start and stop codons and boundaries between introns and exons. Putative starts, stops, and intron positions were determined by comparison with homologous genes in other chloroplast genomes using MAFFT v7.0.0 (Katoh and Standley, 2013). Genes and open reading frames (ORF) that may not have been annotated were identified with the aid of Geneious. In addition, all tRNA genes were further verified online using tRNAscan-SE search server (Lowe and Eddy, 1997) (http://lowelab.ucsc.edu/tRNAscan-SE/). The circular Juglans regia chloroplast genome map was drawn using Organellar Genome DRAW (Lohse et al., 2013). Genome annotation was performed in Geneious, and the GC-content of protein-coding genes, tRNA genes, introns and intergenic spacers (IGSs) was determined on the basis of their annotation. Cpg comparison among the five Juglans species was performed with VISTA (Frazer et al., 2004). Genome, protein coding gene, intron, and spacer sequence divergences were evaluated using DnaSP v5.10 (Librado and Rozas, 2009) after alignment. For the protein coding gene sequences, introns, and spacers, every gene or fragment was annotated using the software Geneious v8.0.2 (http://www.Geneious.com; Kearse et al., 2012). For purposes of the subsequent phylogenetic analysis and plant identification, the complete Cpg of each Juglans species was compared and diagramed using VISTA to show sequence divergence.
Repeat Sequencing Analysis
The genomic sequences were analyzed to identify potential microsatellites (simple sequence repeats orSSRs, i.e., mono-, di-, tri-, tetra-, penta-, and hexanucleotide repeats) using MISA software (http://pgrc.ipk-gatersleben.de/misa/) with thresholds of ten repeat units for mononucleotide SSRs and five repeat units for di-, tri-, tetra-, penta-, and hexanucleotide SSRs. The web-based software REPuter (Kurtz et al., 2001) (http://bibiserv.techfak.uni-bielefeld.de/reputer/) was used to analyze the repeat sequences, which included forward, reverse, complement, palindromic and tandem repeats with minimal lengths of 30 bp and edit distances of less than 3 bp. The large repeat sequences were analyzed by using the Web-based Tandem Repeats Finder (http://tandem.bu.edu/trf/trf.html). We investigated if the repeated elements identified in the chloroplast of J. regia were also present in other four other Chinese Juglans species by aligning their cp genomes using Geneious v8.0.2 (http://www.Geneious.com; Kearse et al., 2012). Tandem repeat sequences (>10 bp in length) were detected using the online program Tandem Repeats Finder (Benson, 1999), with 2, 7, and 7 set for the alignment parameters match, mismatch, and indel, respectively. The minimum alignments core and maximum period size were 80 and 500, respectively.
Mutation Events Analysis, Substitution Rate Analyses, and Inference of Rate Changes
To identify the microstructural mutations of Juglans, the five aligned sequences were further analyzed using DnaSP v5 (Librado and Rozas, 2009) and MEGA v5.0 (Tamura et al., 2011). Indel and SNP events were counted and positioned in the cp genome using DnaSP v5. Signatures of natural selection were studied for every chloroplast gene located outside of the inverted repeats region. Selective pressures (KA/KS) were computed with the codeml tool from PAML package v4.0 (Yang, 2007) using a YN00 model to test every gene sequence. We used the KaKs_calculator program to check the selective pressures (KA/KS) using same model as YN (Zhang et al., 2006). To avoid potential convergence biases, those genes with few mutations were filtered out from selective pressure analysis.
Phylogenetic Analysis
The Juglans Cpg sequences from the finalized data set were aligned with MAFFT v7.0.0 (Katoh and Standley, 2013). The analyses were carried out based on the following three data sets: (1) the complete cp DNA sequences; (2) protein coding sequences; (3) the introns and spacers. We conducted ML analyses using each of the data sets separately. The phylogenetic analyses were carried out using the Cpgs of all five Juglans species plus eight other species with complete Cpgs (Table S2). The Maximum Likelihood (ML) phylogenetic tree analysis was conducted using RAxML v8.0 (Stamatakis, 2014) under GTRGAMMA model. For ML analysis, difference general time reversible models were performed with all three data sets. For all analyses, 10 independent ML searches were conducted, bootstrap support was estimated with 1000 bootstrap replicates, and bootstrap proportions were drawn on the tree with highest likelihood score from the 10 independent searches. The choice of substitution model for each partition was primarily determined by using Modeltest v3.7 (Posada and Crandall, 1998) with the Akaike information criterion (AIC) (Posada and Buckley, 2004). Maximum Parsimony (MP) phylogenetic analyses were performed in MEGA v5.0 (Tamura et al., 2011) using 1000 bootstrap replicates.BI trees were produced by MrBayes v3.2.6 (Huelsenbeck and Ronquist, 2001; Ronquist and Huelsenbeck, 2003; Altekar et al., 2004) with the setting of 1,000,000 generations and stopval = 0.01, under GTRGAMMA model with one cold and three incrementally heated Markov Chain Monte Carlo (MCMC) run simultaneously (Ronquist and Huelsenbeck, 2003) in two parallel runs sampling every 1000 generations. The first 25% of the trees were discarded as burn-in. The remaining trees were used for generating the consensus tree. The phylogenetic relationships and divergence time between lineages were estimated using Bayesian inference method BEAST v1.8.0 (Drummond et al., 2012). Calibration of the Juglandaceae and Fagaceae split (73.4 ± 0.1 Myr) was based on references in Thomas et al. (2012) and Hedges et al. (2015). The GTRAGMMA nucleotide substitution model was selected using software MODELTEST v3.7 (Posada and Crandall, 1998). A relaxed clock with lognormal distribution of uncorrelated rate variation was specified. A normal prior probability distribution was used to accommodate the uncertainly of prior knowledge. Two independent Markov chains of 10,000,000 generations, sampled every 10,000 th iteration, were generated. An adequate effective sample size (larger than 200) and convergence of the Markov chain Monte Carlo chains were diagnosed in Tracer v1.6 with the first 10% samples discarded as burn-in (Drummond et al., 2012). The phylogenetic trees were then complied into a maximum clade credibility tree using TreeAnnotator v1.8.0 (Drummond et al., 2012) and the program FigTree v1.3.1 (Drummond et al., 2012) to visualize mean node ages and highest posterior density (HPD) intervals at 95% (upper and lower) for each node and to estimate branch lengths and divergence times.
Results
Genome Assembly and PCR-Based Gap Filling
Using the Illumina HiSeq system, five Juglans species were sequenced to produce a total of 10,285,876 to 13,320,133 bp paired-end raw reads from four Juglans species, while Common walnut (J. regia) had 6,321,912 bp raw reads (Table 1). After aligning the paired-end reads with the reference Cpg (common walnut, J. regia), 689,686 to 1,118,104 bp Cpg reads were assembled (Table 1). The four Chinese Juglans Cpgs were deposited in NCBI GenBank (accession numbers, KX671976, KX671977, KX671975, and KT963008).
General Features of the Five Chinese Walnut (Juglans) Chloroplast Genomes
The five Juglans Cpgs ranged from 159,714 bp (J. hopeiensis) to 160,367 bp (J. regia), the average Cpg sequence length was 159,978 bp (Figure 1, Table 1). The coding sequence of the five Juglans Cpg ranged from 80,110 bp (J. cathayensis) to 80,475 bp (J. regia and J. sigillata), while the LSC length and SSC length ranged from 89,316 bp (J. hopeiensis) to 89, 872 bp (J. regia and J. sigillata) and 18,351 bp (J. cathayensis) to 18, 423 bp (J. regia), respectively (Table 1). For all five Cpgs the average GC content was 36.1% (Table 1). There are four introns located in the IR region and 13 introns in the LSC region in each of the Cpgs. There was only one gene (ndhA) located in SSC region (Table 2). All five Cpgs included a large single-copy (LSC) region of 89,316 to 89,872 bp, a small single-copy (SSC) region of 18,351 to 18,406 bp, and the inverted repeats (IR)were 26,023 bp (Figure S1, Table 1). All five walnut Cpgs encoded 128 functional genes, including 88 protein-coding genes, 40 tRNA genes, and 8 ribosomal RNA genes (Table 1). There were 18 intron-containing genes (one class I intron in trn-UAA and 17 class II introns), of which three genes rps12, clpP, and ycf3, contained two introns and the rest had only one intron each (Table 2).In addition, there were two pseudogenes: infA and ycf15, in which several internal stop codons were identified. The ycf15 gene displayed exactly the same structure in all five Chinese Juglans Cpgs. The pseudogene infA contained internal stop codons which differed among the five Juglans Cpg.
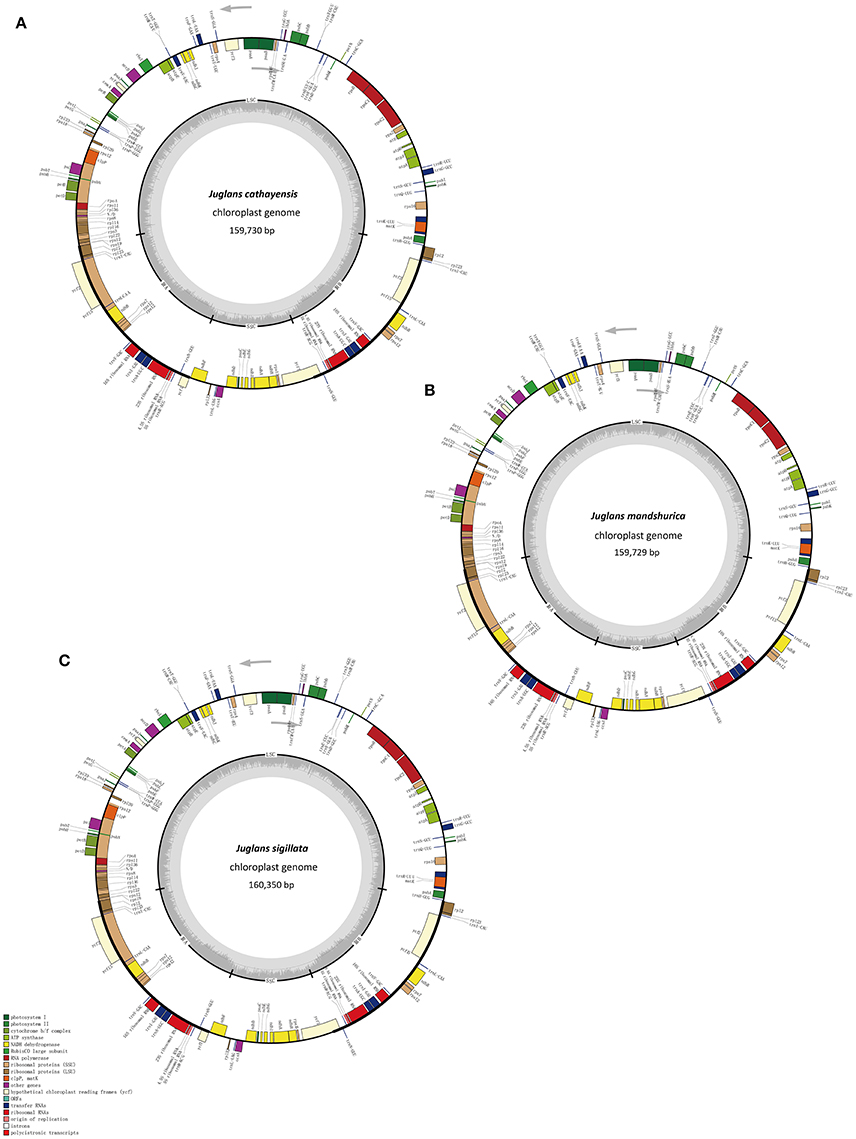
Figure 1. Chloroplast genome maps of three Juglans species. (A) J. cathayensis chloroplast genome, (B) J. mandshurica chloroplast genome, (C) J. sigillata chloroplast genome. Genes drawn outside the outer circle are transcribed clockwise, and those inside are transcribed counter-clockwise. Genes belonging to different functional groups are colorcoded. Thedark gray in the innercircle indicates GC content of the chloroplast genomes.
Conservation within Juglans Cps and Comparison with Fagaceae and Betulaceae
When duplicated genes in IR regions were counted only once, all five Juglans Cpgs harbored 128 functional genes (except eight rRNA and pseudogenes ycf15 and infA) arranged in the same order, including 88 protein-coding genes and 40 tRNAs (Table 2). Fourteen of the protein-coding genes and six of the tRNA genes contained introns, 19 of which contained a single intron, whereas four had two introns (Table 2). The numbers of protein-coding genes in the Cpgs of the five Chinese Juglans was similar to the number of protein-coding genes in the Betulaceae and Fagaceae, two closely related plant families. As described above, ycf15 was a pseudogene in all five Chinese Juglans; it is also non-functional in the Betulaceae, and Fagaceae except in Q. rubra. We identified seven internal stop codons in the ycf15 sequence of Chinese Juglans (Figure 2B). The infA gene was also present as a pseudogene in all five Chinese Juglans Cpgs because of several stop codons. By contrast, infA appears to be a protein-coding gene in Quercus, Castanopsis, and Trigonobalanus. In Castanea, the infA gene contains a long indel (70 bp) rather than an internal stop codon (Figure 2). In this study, we identified nine internal stop codons in the infA sequence of J. regia and J. sigillata (sect. Dioscaryon). By contrast, we found five, five, and two internal stop codons in the infA sequence of J. hopeiensis, J. mandshurica, and J. cathayensis, respectively (Figure 2A).
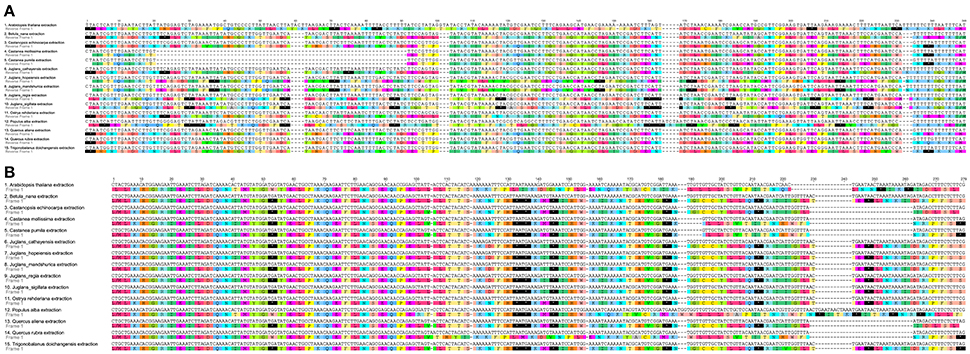
Figure 2. Alignment of two pseudogenes in the five Chinese Juglans species and 10 eudicot outgroups chloroplast genome. (A) infA. (B) ycf15. The black box with an asterisk represents stop codons.
All five Juglans Cpg IR regions were well conserved, including gene number and gene order, but they exhibited obvious differences at the single-copy (SC) boundary regions (Figure S1). The nucleotide sequence length of SSC regions ranged from 18,351 to 18,423 bp (72 bp difference), while the nucleotide sequence length of the IR regions ranged from 26,023 to 26,036 bp (13 bp difference) (Table 1). The nucleotide sequence differences were mainly found between members of the two sections (sect. Dioscaryon, and sect. Cardiocaryon). Within the IR region, the gene ycf2 had two SNPs, and ycf7 had one SNP. There were two polymorphisms (12 bp indel and 6 bp indel) in the ycf2-trnV-GAC spacer region, and one SNP in the rRNA-trnI-GAU 16S interval, one SNP in the intron of trnI-GAU, six in the rRNA 23S, and one in rRNA-trnR-ACG. The trnR-ACG-trnN-GUU spacer region had three SNPs. The gene ycf1 had six SNPs and one indel of 7 bp (Table S3). The gene ycf1 crossed into the SSC region, and the pseudogene fragment ycf1 was located in the IRA region at 1158 to 1162 bp.
The coding regions of the Cpgs were more highly conserved than the non-coding regions, as expected (Figure 3), but there were differences among the five species. The most dissimilar coding regions were ndhA and rpoC2 (Figure 3). Other evolutionary differences among the five cp genomes were inferred from differences in genome size in general and, in particular, differences in the size of the single copy (SC) region (Figure S1).
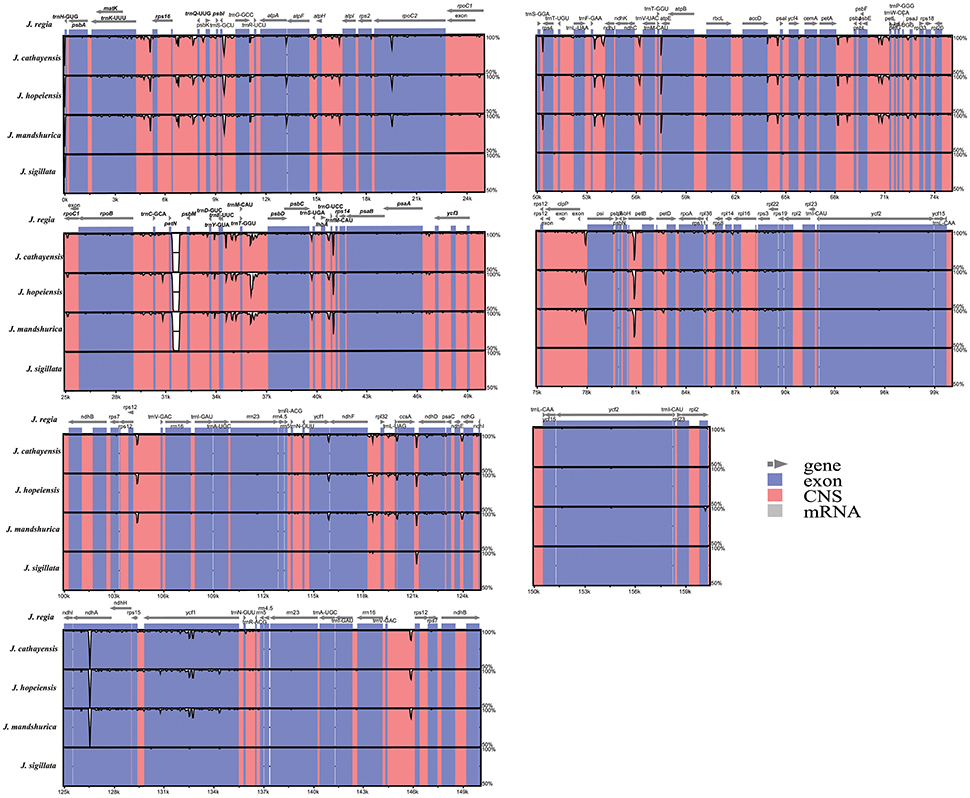
Figure 3. Sequence identity plot comparing the five Juglans chloroplast genomes with J. regia as a reference by using mVISTA. Vertical scale indicates the percentage of identity ranging from 50 to 100%. Coding regions are marked in blue and non-coding regions are marked in red. Gray arrows indicate the position and direction of each gene.
Microsatellite Polymorphims and Repeat Sequences
Each Juglans Cpg contained 66 to 83 SSRs at least 10 bp in length (Table 3, Figure 4A, Table S4). Among these SSRs (about 73 SSRs per Cpg), most were located in noncoding sections of the LSC/SSC region (96.3% of the total occurrences), and about 11 per Cpg were in protein-coding genes (ycf1, rpoC1, ropC2, rpoB, and atpB) (Table 3, Table S4). J. hopeiensis and J. mandshurica included about 17 more SSR loci in their Cpgs than the other three species. Mono-, di-, trin-, tetra-, penta-, and complex nucleotide SSRs were detected in every species, the mononucleotide, complex nucleotide, and dinucleotide SSRs averaged 64.8, 10.4, and 5.6%, of all SSRs, respectively. SSRs in walnut Cpgs are especially rich in AT. Nearly all SSRs (84.0%) were mononucleotide A/T repeats; only one or two C/G mononucleotide SSRs per genome were present. Among dinucleotide SSRs, AT/TA repeats were the most common (typically about seven per Cpg), trinucleotide SSRs (ATT/ATA) repeats were present in a small number of loci (one or three, depending on species), and depending on species, from 8 to 11 loci contained complex nucleotide repeats (Table 3, Figure S2, Table S4). AAAAT/ATTTT SSRs and AAATAT/ATATTT SSRs were only found in J. regia and J. sigillata (section Dioscaryon), and AAGAT/ATCTT repeat units were only found in J. cathayensis, J. hopeiensis and J. mandshurica) (Table 3, Figure S2, Table S4).
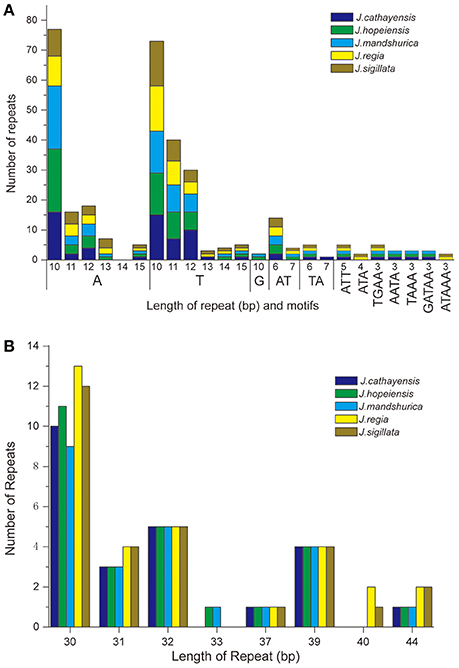
Figure 4. Analysis of repeated sequences in the five Chinese Juglans chloroplast genomes. (A) Frequency of selected motifs of simple sequence repeats (SSRs) >10 bp. (B) Frequency of repeat sequences of length >40 bp.
Long Repeat Analysis
Juglans Cpgs contained numerous forward repeats, palindromic repeats, and reverse repeats of at least 30 bp with a sequence identity ≥ 90% (Figure 4B, Table S5). These “long repeats” ranged from 30 to 44 bp in length and were repeated twice. Protein-coding genes (e.g., rpoC1, psaB, petB, and ycf2) contained a range of five to seven long repeat sequences (across species). Species also varied somewhat for number of long repeat sequences located in the intergenic regions (J. regia n = 24; J. sigillata n = 22; J. hopeiensis n = 21; J. mandshurica, n = 20; J. cathayensis n = 19; Table S5). Depending upon species, we observed 12 or 13 forward repeats, 11 to 16 palindromic repeats, one or two reverse repeats, and one complementary repeat (only seen in J. hopeiensis)(Table 4, Table S5). The longest forward repeat unit was 44 bp; it was located in the psbT-psbN intergenic spacer of the LSC region of J. regia and J. sigillata. A different 44 bp repeat was located in the protein-coding genes psaB-psaA in the LSC of J. cathayensis, J. hopeiensis, and J. mandshurica (Table S5). In the sections Juglans/Dioscaryon, J. sigillata and J. regia each contained 13 forward repeats and two reverse repeats, and 16 (J. regia) or 13 (J. sigillata) palindromic repeats (Table 4, Table S5). In the section Cardiocaryon, J. cathayensis contained 13 forward and 11 palindromic repeats, J. hopeiensis contained 13 forward, 11 palindromic, onereverse, and one complementary repeat, and J. mandshurica contained 12 forward, 12 palindromic, and 1 reverse repeat (Table 4, Table S5). Tandem repeats of more than 20 bp and 100% sequence identity were identified in the intergenic spacers of trnK-UUU-rps16 (one repeat each in J. hopeiensis, J. mandshurica, and J. cathayensis); trnE-UUC-trnT-GGU (J. regia, 1; J. sigillata, 1; J. hopeiensis, 2; J. mandshurica, 1; J. cathayensis, 1); trnT-GGU-psbD (J. regia, 1; J. sigillata, 1; J. hopeiensis, 1; J. mandshurica, 2; J. cathayensis, 1); lhbA-trnG-UCC (J. hopeiensis, 1; J. mandshurica, 1; J. cathayensis, 1); ndhC-trnV-UAC (every Juglans species had one repeat); trnF-GAA-ndhJ (J. regia, 1; J. sigillata, 1); and trnG-UCC-trnfM-CAU (J. regia, 1; J. sigillata, 1). Two identical tandem repeats were found in the protein-coding regions of all five Juglans Cpgs (Table S6).
Divergence Hotspots
The coding genes, non-coding regions, and introns were compared among the five Chinese Juglans species for divergence hotspots. The level of sequence divergence among all five species was estimated as the nucleotide variability value (Pi = 0.00219).The number of parsimony informative sites incoding genes, non-coding regions, and the complete Cpg was 192, 342, and 534, respectively (Table S7). The protein-coding CDS region was much more conserved than the IGS regions (i.e., LSC and SSC is much more conserved than the IR region). Within the CDS region, the ten genes with the greatest variability were rps3, psbL, petD, rpl22, psaJ, ndhD, rps19, rpoA, rpl32, and ndhA (Figure 5A), and the twelve least variable genes in CDS were petA, psbC, atpB, psbD, ndhG, ndhK, rps2, psbA, rbcL, psi, psaB, rrn23, and ycf2 (Figure 5A). Some IGS were quite conserved; rpl12-trnH-GUG, atpA-atpF, trnL-UAG-ccsA, psbC-trnS-UGA, ndhE-ndhG, rps19-rpl2, rpl14-rpl16, psi-psbT, ihbA-trnG-UCC, trnG-GCC-trnR-UCU, trnT-GGU/trnM-CAU-psbD, and trnP-UGG/trnP-GGG-psaJ showed lower levels of variation than genes located in the CDS region (Figure 5B). Across all five species, the regions with greatest sequence divergence were rps16-trnQ-UUG, trnE-UUC-trnT-GGU, trnT-GGU-psbD, petN-psbM, petB intron, rpoC2, ndhA, and ycf1. These intergenic regions were also generally rich in SSRs; rps16-trnQ-UUGhad four SSRs [(T)10, (A)10, (T)11, and (A)11]; trnE-UUC-trnT-GGU had three SSRs [(T)10, (A)11, and (AT)7]; trnT-GGU-psbD had one SSR [(AT)6]; petN-psbM, one SSR [(T)10]; petB intron, two SSRs[(A)10 and (A)10]; rpoC2, three SSRs [(T)11, (T)11, (T)11]; ndhA intron, four SSRs [(A)15, (T)13aattg…(T)11, (AT)6]; and ycf1 had six SSRs [(T)11, (T)10, (T)12, (A)10, and (T)12. Within section Juglans/Dioscaryon, rps4-trnT-UGU (1 SNP), ndhC-trnV-UAC (1 SNP), ycf1 (1 SNP; IRa), ccsA-ndhD, ycf1 (3 SNP; IRb) were variable. Within section Cardiocaryon, trnC-GCA-petN, trnE-UUC-trnT-GGU, trnT-GGU-psbD, and trnF-GAA-ndhJ were most variable (Figure 3). In total, we identified 610 SNPs or indels that were distinct between Juglans/Dioscaryon and Cardiocaryon.
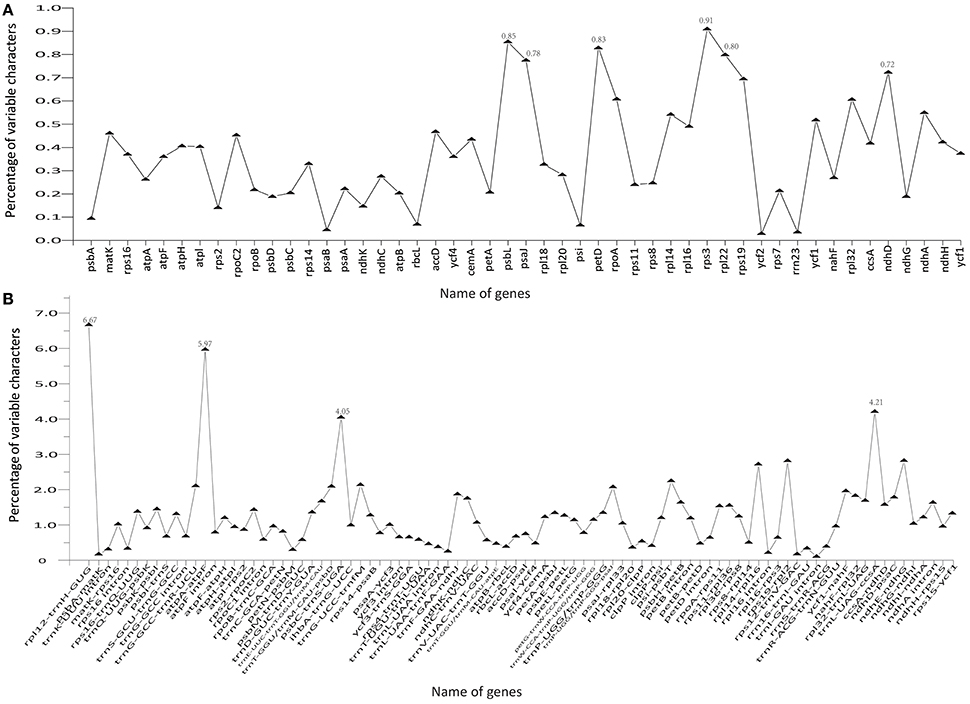
Figure 5. Comparison of percentage of variable characters (SNPs, indels, and mutations) in five aligned Juglans chloroplast genomes. (A) Protein coding sequences (CDS); (B) The introns and spacers (IGS).
Selective Pressures in the Evolution of Juglans
A total of 79 protein-coding genes were used to analyze synonymous and nonsynonymous change rates in Juglans. We identified five genes (matK, ycf1, accD, rps3, and rpoA) under positive selection (KA/KS ratio >1; Figure S3; Table S8). The KA/KS ratio for accD for all five species was 1.23. The KA/KS ratio for matK for all five species was 1.34, for rpoA it was 1.17, and for rps3 it was 1.38 (Table S8). Interestingly, these five genes were previously found to present above average SNV and indel densities in exons (Table S8). All five genes were under positive pressure exclusively between sect. Cardiocaryon and sect. Dioscaryon; none of these five genes showed evidence of positive selection within either section (Figure S3; Table S8).
Phylogenetic Analysis
We used three datasets (whole complete Cpg, protein-coding exons, and non-coding region) to analyze the phylogenetic relationships among members of two sections of Juglans and closely related species in the Betulaceae and Fagaceae. Arabidopsis thaliana and Populus alba were used as outgroups. Among the three datasets, complete Cpgs contained the greatest number of parsimony informative characters (531, 0.33%), followed by no-coding region (342, 0.42%) and protein-coding exons (192, 0.24%). The reconstructed phylogeny divided into four clades (Figure 6; Figures S4, S5, with members of the Betulaceae (Ostrya rehderiana and Betula nana) joined to the five Juglans species and distinct from the other Fagaceae, irrespective of dataset. Within Juglans, the five Chinese species were divided into two clades corresponding to the two sections (Juglans/Dioscaryon and Cardiocaryon) with 100 % bootstrap (BS)support based on Maximum Likelihood (ML) and Maximum parsimony (MP) analysis (Figure 6A; Figures S4A,B). Analysis of the whole cp genomes of the five Chinese walnut species and 10 eudicot outgroups using Bayesian inference (BI) resulted in cladograms with topology similar to ML and MP, and strongly supported phylognetic trees based on each of three datasets (whole cp genome sequences, protein coding sequences, and the introns and spacers) (Figure 6B; Figures S4C,D). In section Juglans/Dioscaryon, J. regia and J. sigillata were split with a 100% BS, while the Cardiocaryon clade (J. cathayensis and J. hopeiensis, J. mandshurica) diverged from sect. Juglans with 100% BS value (Figure 6; Figures S4, S5). J. hopeiensis was closer to J. mandshurica than to J. cathayensis (Figure 6; Figures S4, S5. We constructed the divergence time tree among five Chinese walnut species based on whole chloroplast genome sequences. The results showed that the divergence time between two sections was 7.91Myr, while J. regia and J. sigillata diverged much more recently (0.05 Myr), and J. cathayensis diverged from J. mandshurica and J. hopeiensis before 3.51Myr (Figure S5).
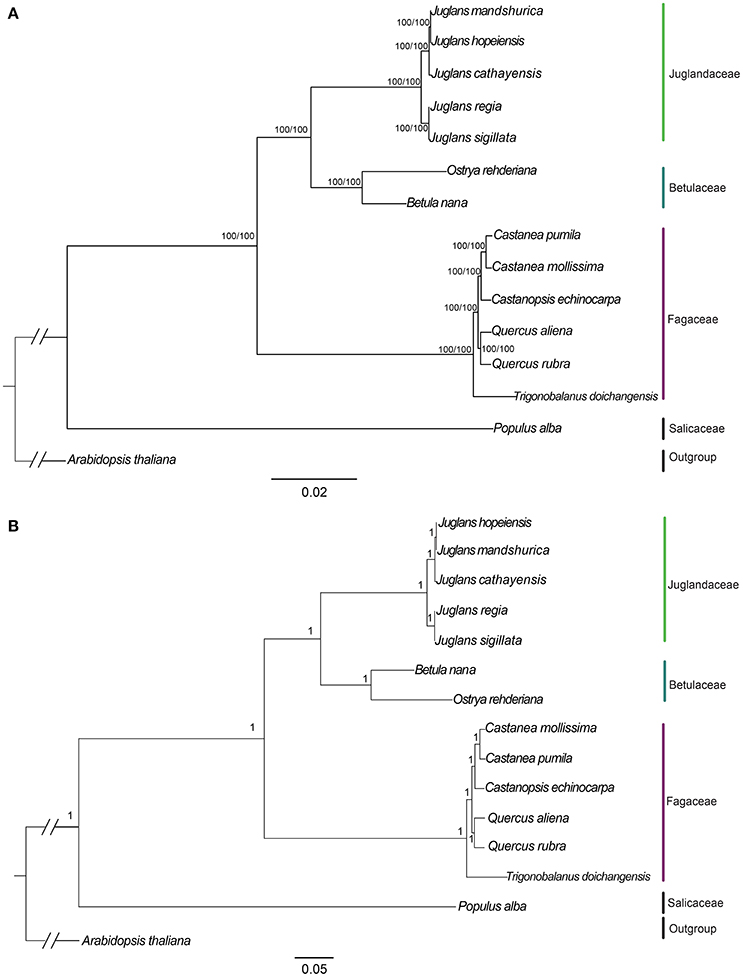
Figure 6. Phylogeny of five Juglans species plus 8 taxa using (A) Maximum Likelihood (ML) and (B) Bayesian inference (BI) based on whole cp genome sequences. Diagonal hash marks nested inside Arabidopsis thaliana represent a branch length truncation of 3/4. Numbers above branches are bootstrap support values.
Discussion
Chloroplast Sequence Variation and Evolution
In the present study, we sequenced the chloroplast genomes of five Juglans species, annotated the chloroplast genomes, identified SSR and tandem repeats within the genomes, and carried out a phylogenetic analysis comparing them to ten other chloroplast genomes. Our results have laid the foundation for future studies on the evolution of chloroplast genomes of walnuts and butternuts, as well as the molecular identification of Juglans species.
Most angiosperm chloroplasts contain 74 protein-coding genes, while an additional five are present in few species (Millen et al., 2001). The five Juglans Cpg we sequenced revealed 88 protein-coding genes (79 unigenes were protein-coding), 40 tRNA genes, and 8 rRNA genes, which is similar to Quercus (Du et al., 2015; Lu et al., 2016; Yang et al., 2016). The number of tRNA genes and rRNA genes in Juglans was the same as in five Quercus species (Yang et al., 2016). Moreover, the total number of introns in the Juglans Cpg was the same as Quercus rubra (Alexander and Woeste, 2014), Ampelopsis (Raman and Park, 2016), and Saxifragales (Dong et al., 2013). Several lineages of angiosperms have independently lost introns from the ribosomal protein genes rps16, rps12, and rpl16 (Downie et al., 1991; Downie and Palmer, 1992), including Geraniaceae and Caryophyllales (Logacheva et al., 2008). The five Chinese Juglans species have not lost introns in any of these genes, however, a characteristic they have in common with the woody plant family Vitaceae (Raman and Park, 2016).
The gene infA encodes translation initiation factor 1. It has been lost completely in some angiosperms (Millen et al., 2001; Steane, 2005), is present as a pseudogenein the majority of angiosperm (Millen et al., 2001; Steane, 2005), and is present and presumed functional in Quercus robur and Quercusrubra (Alexander and Woeste, 2014). In this study, we identified nine internal stop codons in Juglans/Dioscaryon versus five, five, and three internal stop codons in the infA sequence of J. hopeienis, J. mandshuria, and J. cathayensis Cpgs, respectively. Thus, although infA is a pseudogene in all Juglans/Dioscaryon and Cardiocaryon for which there are data, there are inter-sectional differences that deserve additional study (Figure 2A), and infA may reveal important phylogenetic information concerning section Rhysocaryon. We also observed that the hypothetical gene ycf15 was truncated in Dioscaryon species and Cardiocaryon species by five and three internal stop codons, respectively (Figure 2B). A similar truncation was seen in Quercus aliena (Lu et al., 2016, ycf15) and Quercus spinosa (Du et al., 2015) of Fagaceae, in Liliales (Liu et al., 2012b), Kiwi fruit (Actinidia chinensis var. chinensis) (Yao and Huang, 2016), and Vaccinium macrocarpon (Fajardo et al., 2013). ycf15 is a pseudogene in all families of Saxifragales (Dong et al., 2013), but may be a functional protein coding gene in Thalictrum coreanum (Ranunculaceae, Park et al., 2015). The role of ycf15 as a protein coding gene remains unclear and requires further study.
Variability in copy number of simple sequence repeats (SSRs) in the chloroplast makes them important molecular markers for distinguishing lower taxonomic levels (Yang et al., 2011; Xue et al., 2012). Cp SSRs have been used widely in plant population genetics (Doorduin et al., 2011; He et al., 2012), polymorphism investigations (Xue et al., 2012), and ecological and evolutionary studies (Roullier et al., 2011; Wang et al., 2013). The SSRs in the five Juglans Cp genomes we investigated were AT rich. Poly (A)/(T) SSRs are more common than poly (G)/(C) in many plant families (Melotto-Passarin et al., 2011; Nie et al., 2012; Martin et al., 2013). The cpSSRs of the five Juglans we studied are expected to be useful for assays detecting polymorphisms at population-level as well as comparing more distantly phylogenetic relationships among Juglans species.
Large and complex repeat sequences may play an important role chloroplast genome arrangement and sequence divergence (Timme et al., 2007; Guisinger et al., 2011; Weng et al., 2013). We found numerous repeated sequences in the Cpgs of Juglans, particularly in the intergenic spacer regions, similar to those reported in other angiosperm lineages (Yang et al., 2016). We found that repeats in petB, psaA, and ycf2 differed between species in different sections of Juglans, and the same was true of repeats in the gene junctions (trnK-UUU-rps16, trnV-GAC-rps7, trnT-GGU-psbD, and trnT-GGU-psbD) (Table S5). These divergence hotspots within Juglans Cpg sequences are potentially important resources for developing molecular markers for phylogenetic analyses and identification of Juglans species (Stanford et al., 2000; Aradhya et al., 2007).
Phylogenetic Analysis
The classical taxonomy of Juglans based on non-coding regions of the Cpg supported the separation of J. regia and J. sigillata into Sec. Juglans/Dioscaryon and other three Juglans species (J. cathayensis, J. hopeiensis, J. mandshurica) into Sec. Cardiocaryon (Stanford et al., 2000; Aradhya et al., 2007). Whether J. regia and J. sigillata are legitimately distinct taxa in China has been controversial; Iron walnut (J. sigillata) could be an independent species based on RAPD and EST-SSR data (Wu et al., 2000; Qi et al., 2011) and based on RFLP and Cp DNA fragments(92% bootstrap value) (Aradhya et al., 2007). Our data support their maintenance as distinct taxa.
Members of the Cardiocaryon are morphologically distinct from other Juglans in that they have red stigmas, number of leaflets per leaf, and in the number of fruits typically found in a cluster, but the phylogenetic relationships within sect. Cardiocaryon are unsettled. J. hopeiensis is sympatric with J. mandshurica, and based on data from AFLPs and isozymes, some have concluded that J. hopeiensis is a hybrid species between J. regia and J. mandshurica (Wenheng, 1987; Zhang et al., 2009), consistent with the interpretation of floral evolution in the genus by Xi (1987). All phylogenetic trees based on our data indicate that J. hopeiensis is closer to J. mandshurica than J. cathayensis, and that the latter two species are distinct, in contrast to the Flora of China (1999), which relies exclusively on morphological data. The relationship between J. hopeiensis and J. ailantifolia, the only other Asian member of the Cardiocaryon, is now an important question. These results showed that the Stanford et al. (2000) and Aradhya et al. (2007) taxonomy of Juglans is reasonable on the whole. In this study, J. regia and J. sigillata were divided from each other with a 100% BS, while J. cathayensis, J. hopeiensis, and J. mandshurica diverged from sect. Juglans with 100% BS value (Figure 6. Each of the five species is supported as independent species based on whole chloroplast genome sequences.
In this study, the five Chinese walnut species and 10 eudicot outgroups were represented with well-supported cladograms with highly similar topology and strongly supported phylogenetic trees using Maximum Likelihood (ML), Bayesian inference (BI), and Maximum parsimony (MP) analysis. Analysis using whole Cpg sequences, protein coding sequences, and the introns and spacers resulted in consistent and strongly supported results (Figure 6; Figure S4). Our results confirmed that the phylogenetic relationships among the five Chinese Juglans based on chloroplast sequences only are in congruence with those reported by Stanford et al. (2000) and Aradhya et al. (2007). Each of the two sections was confirmed to be monophyletic (Dode, 1909; Manning, 1978). Within sect. Dioscaryon, division of the two species was highly supported, as suggested by Aradhya et al. (2007). With the exception of section Cardiocaryon (Dode, 1909; Manning, 1978), relationships among three Chinese walnuts were fully resolved and statistically supported (P = 0.95; BS = 100%). Stanford et al. (2000) and Aradhya et al. (2007) recovered an unsupported sister relationship between J. mandshurica and J. cathayensis because J. hopeiensis was not included in those analyses (Stanford et al., 2000). Previously suggested relationships among members of section Cardiocaryon were confirmed by our data with even higher support than in Stanford et al. (2000) and Aradhya et al. (2007), although our analysis did not include Japanese walnut (J. ailantifolia), the final member of Cardiocaryon. The chloroplast-based phylogeny presented in this work and by others is not a complete understanding of the evolutionary relationships among these five Chinese Juglans because events we did not consider, including incomplete lineage sorting, chloroplast capture, horizontal transfer, and local fixation of cpG haplotypes can all influence phylogeny (Stegemann et al., 2012; Mariac et al., 2014; Novikova et al., 2016).
The divergence time between the two Asian Juglans sections was estimated at 7.91Myr, although several Juglans species diverged quite recently within each section (Figure 6; Figure S4). The deep evolutionary relationships and divisions within the two Asian sections needs further investigation. The molecular phylogeny of the entire genus (Juglans) and its relationship to other genera in the Juglandaceae also awaits more evidence. These Cpg sequences will provide genetic information necessary to understand the evolution of plastid genomes via phylogenomics.
Data Archiving Statement
The chloroplast genome sequences of Chinese walnut (Juglans) species were submitted on the National Center for Biotechnology Information (NCBI), the accession numbers were: KT820730, KT820731, and KT820732, KT820733.
Ethics Statement
This article does not contain any studies with human participants performed by any of the authors.
Author Contributions
PZ, YH, and KW designed and performed the experiment as well as drafted the manuscript. YH and PZ collected the samples. YH and PZ completed the sequence assembly and analyzed the data. KW and PZ conceived the study and revised the manuscript. All the authors have read and approved the final manuscript.
Conflict of Interest Statement
The authors declare that the research was conducted in the absence of any commercial or financial relationships that could be construed as a potential conflict of interest.
Acknowledgments
This work was supported by the National Natural Science Foundation of China (No. 41471038; No. 31200500; No. J1210063), the Program for Excellent Young Academic Backbones funding by Northwest University, the Northwest University Training Programs of Innovation and Entrepreneurship for Graduates (No. YZZ15062), Changjiang Scholars and Innovative Research Team in University (No. IRT1174). Mention of a trademark, proprietary product, or vendor does not constitute a guarantee or warranty of the product by the U.S. Department of Agriculture and does not imply its approval to the exclusion of other products or vendors that also may be suitable.
Supplementary Material
The Supplementary Material for this article can be found online at: http://journal.frontiersin.org/article/10.3389/fpls.2016.01955/full#supplementary-material
Figure S1. Comparisons of LSC, SSC, and IR region borders among the five Chinese Juglans chloroplast genomes.
Figure S2. Frequency distribution of major SSRs based on main motif type in the five Chinese Juglans cp genomes. Jh, Juglans hopeiensis; Jc, J. cathayensis; Jm, J. mandshurica; Jr, J. regia; Js, J. sigillata.
Figure S3. Gene-specific KA/KS values between the chloroplast genomes of two Juglansspecies (J. regia and J. cathayensis) representing section Juglans/Dioscaryon and section Cardiocaryon, respectively. Five genes (matK, ycf1, accD, rps3, and rpoA) returned KA/KS values greater than 0.8, whereas the KA/KS values of the other genes were below 0.8.
Figure S4. Phylogenetic tree construction of five Juglans species plus eight other taxa. (A) Maximum Likelihood (ML) tree and Maximum parsimony (MP) tree based on protein coding sequences, (B) Maximum Likelihood (ML) tree and Maximum parsimony (MP)tree based on the introns and spacers, (C) Bayesian inference (BI)tree based on protein coding sequences, (D) Bayesian inference (BI) treebased on the introns and spacers. Numbers above branch indicate the bootstrap (BS) support value.
Figure S5. Phylogenetic timetree construction of five Chinese Juglans species plus eight other taxa based on whole cp genome sequences. Blue bars and the numbers at the nodes indicate 95% highest posterior densities (HPDs) of time estimates (million years ago, Myr).
Table S1. Primers used for genome sequence validation.
Table S2. The information of a total of 15 species used for phylogenetic analysis.
Table S3. Indels and single nucleotide polymorphisms (SNP) in the five Chinese Juglans chloroplast genomes.
Table S4. Simple sequence repeats in each of five Chinese Juglans species.
Table S5. The information of the function nucleic acid repeats of five Chinese Juglans species.
Table S6. The length of tandem repeats distribution in five Chinese Juglans species.
Table S7. The number of variable sites in five Chinese Juglans species.
Table S8. KA/KS ratio for protein coding sequences for five Chinese Juglans species. Jh, Juglans hopeiensis; Jc, J. cathayensis; Jm, J. mandshurica; Jr, J. regia; Js, J. sigillata.
References
Alexander, L. W., and Woeste, K. E. (2014). Pyrosequencing of the northern red oak (Quercus rubra L.) chloroplast genome reveals high quality polymorphisms for population management. Tree Genet. Genomes 10, 803–812. doi: 10.1007/s11295-013-0681-1
Aradhya, M. K., Potter, D., and Simon, C. J. (2004). Origin, evolution, and biogeography of Juglans: a phylogenetic perspective. V Int. Walnut Symp. 705, 85–94. doi: 10.17660/ActaHortic.2005.705.8
Altekar, G., Dwarkadas, S., Huelsenbeck, J. P., and Ronquist, F. (2004). Parallel metropolis coupled markov chain monte carlo for bayesian phylogenetic inference. Bioinformatics 20, 407–415. doi: 10.1093/bioiwnformatics/btg427
Aradhya, M. K., Potter, D., Gao, F., and Simon, C. J. (2007). Molecular phylogeny of Juglans (Juglandaceae): a biogeographic perspective. Tree Genet. Genomes 3, 363–378. doi: 10.1007/s11295-006-0078-5
Bai, W. N., Wang, W. T., and Zhang, D. Y. (2014). Contrasts between the phylogeographic patterns of chloroplast and nuclear DNA highlight a role for pollen-mediated gene flow in preventing population divergence in an East Asian temperate tree. Mol. Phylogenet. Evol. 81, 37–48. doi: 10.1016/j.ympev.2014.08.024
Bankevich, A., Nurk, S., Antipov, D., Gurevich, A. A., Dvorkin, M., Kulikov, A. S., et al. (2012). SPAdes: a new genome assembly algorithm and its applications to single-cell sequencing. J. Comput. Biol. 19, 455–477. doi: 10.1089/cmb.2012.0021
Benson, G. (1999). Tandem repeats finder: a program to analyze DNA sequences. Nucleic Acids Res. 27:573. doi: 10.1093/nar/27.2.573
Carbonell-Caballero, J., Alonso, R., Ibañez, V., Terol, J., Talon, M., and Dopazo, J. (2015). A phylogenetic analysis of 34 chloroplast genomes elucidates the relationships between wild and domestic species within the genus Citrus. Mol. Biol. Evol. 32, 2015–2035. doi: 10.1093/molbev/msv082
Chevreux, B., Pfisterer, T., Drescher, B., Driesel, A. J., Müller, W. E., Wetter, T., et al. (2004). Using the miraEST assembler for reliable and automated mRNA transcript assembly and SNP detection in sequenced ESTs. Genome Res. 14, 1147–1159. doi: 10.1101/gr.1917404
Dang, M., Liu, Z. X., Chen, X., Zhang, T., Zhou, H. J., Hu, Y. H., et al. (2015). Identification, development, and application of 12 polymorphic EST-SSR markers for an endemic Chinese walnut (Juglans cathayensis L.) using next-generation sequencing technology. Biochem. Syst. Ecol. 60, 74–80. doi: 10.1016/j.bse.2015.04.004
Daniell, H., Lin, C. S., Yu, M., and Chang, W. J. (2016). Chloroplast genomes: diversity, evolution, and applications in genetic engineering. Genome Biol. 17:134. doi: 10.1186/s13059-016-1004-2
Dode, L. A. (1909). Contribution to the study of the genus Juglans (English translation by Cuendett, R. E). Bull. Soc. Dendrol. France 11, 22–90.
Dong, W., Xu, C., Cheng, T., and Zhou, S. (2013). Complete chloroplast genome of Sedum sarmentosum and chloroplast genome evolution in Saxifragales. PLoS ONE 8:e77965. doi: 10.1371/journal.pone.0077965
Doorduin, L., Gravendeel, B., Lammers, Y., Ariyurek, Y., Chin-A-Woeng, T., and Vrieling, K. (2011). The complete chloroplast genome of 17 individuals of pest species Jacobaea vulgaris: SNPs, microsatellites and barcoding markers for population and phylogenetic studies. DNA Res. 18, 93–105. doi: 10.1093/dnares/dsr002
Downie, S. R., Olmstead, R. G., Zurawski, G., Soltis, D. E., Soltis, P. S., Watson, J. C., et al. (1991). Six independent losses of the chloroplast DNA rpl2 intron in dicotyledons: molecular andphylogenetic implications. Evolution 45, 1245–1259. doi: 10.2307/2409731
Downie, S. R., and Palmer, J. D. (1992). “Use of chloroplast DNA rearrangements in reconstructing plant phylogeny” in Molecular Systematics of Plants, eds P. S. Soltis, D. E. Soltis, and J. J. Doyle (New York, NY; London: Chapman & Hall), 14–35.
Drummond, A. J., Suchard, M. A., Xie, D., and Rambaut, A. (2012). Bayesian phylogenetics with BEAUti and the BEAST 1.7. Mol. Biol. Evol. 29, 1969–1973. doi: 10.1093/molbev/mss075
Du, F. K., Lang, T., Lu, S., Wang, Y., Li, J., and Yin, K. (2015). An improved method for chloroplast genome sequencing in non-model forest tree species. Tree Genet. Genomes 11, 1–14. doi: 10.1007/s11295-015-0942-2
Fajardo, D., Senalik, D., Ames, M., Zhu, H., Steffan, S. A., Harbut, R., et al. (2013). Complete plastid genome sequence of Vaccinium macrocarpon: structure, gene content, and rearrangements revealed by next generation sequencing. Tree Genet. Genomes 9, 489–498. doi: 10.1007/s11295-012-0573-9
Fjellstrom, R. G., and Parfitt, D. E. (1995). Phylogenetic analysis and evolution of the genus Juglans (Juglandaceae) as determined from nuclear genome RFLPs. Plant Syst. Evol. 197, 19–32. doi: 10.1007/BF00984629
Frazer, K. A., Pachter, L., Poliakov, A., Rubin, E. M., and Dubchak, I. (2004). VISTA: computational tools for comparative genomics. Nucleic Acids Res. 32, W273–W279. doi: 10.1093/nar/gkh458
Guisinger, M. M., Kuehl, J. V., Boore, J. L., and Jansen, R. K. (2011). Extreme reconfiguration ofplastid genomes in the angiosperm family Geraniaceae: rearrangements, repeats, and codon usage. Mol. Biol. Evol. 28, 583–600. doi: 10.1093/molbev/msq229
Hahn, C., Bachmann, L., and Chevreux, B. (2013). Reconstructing mitochondrial genomes directly from genomic next-generation sequencing reads—a baiting and iterative mapping approach. Nucleic Acids Res. 41:e129. doi: 10.1093/nar/gkt371
He, S., Wang, Y., Volis, S., Li, D., and Yi, T. (2012). Genetic diversity and population structure: implications for conservation of wild soybean (Glycine soja Sieb. etZucc) based on nuclear and chloroplast microsatellite variation. Int. J. Mol. Sci. 13, 12608–12628. doi: 10.3390/ijms131012608
Hedges, S. B., Marin, J., Suleski, M., Paymer, M., and Kumar, S. (2015). Tree of life reveals clock-like speciation and diversification. Mol. Biol. Evol. 32, 835–845. doi: 10.1093/molbev/msv037
Hu, Y. H., Zhao, P., Zhang, Q., Wang, Y., Gao, X. X., Zhang, T., et al. (2015). De novo assembly and characterization of transcriptome using Illumina sequencing and development of twenty five microsatellite markers for an endemic tree Juglans hopeiensis Hu in China. Biochem. Syst. Ecol. 63, 201–211. doi: 10.1016/j.bse.2015.10.011
Hu, Y., Woeste, K. E., Dang, M., Zhou, T., Feng, X., Zhao, G., et al. (2016). The complete chloroplast genome of common walnut (Juglans regia). Mitochondrial DNA B. 1, 189–190. doi: 10.1080/23802359.2015.1137804
Huang, D. I., Hefer, C. A., Kolosova, N., Douglas, C. J., and Cronk, Q. C. (2014). Whole plastome sequencing reveals deep plastid divergence and cytonuclear discordance between closely related balsam poplars, Populus balsamifera and P. trichocarpa (Salicaceae). New Phytol. 204, 693–703. doi: 10.1111/nph.12956
Huelsenbeck, J. P., and Ronquist, F. (2001). MRBAYES: bayesian inference of phylogenetic trees. Bioinformatics 17, 754–755. doi: 10.1093/bioinformatics/17.8.754
Katoh, K., and Standley, D. M. (2013). MAFFT multiple sequence alignment software version 7: improvements in performance and usability. Mol. Biol. Evol. 30, 772–780. doi: 10.1093/molbev/mst010
Kearse, M., Moir, R., Wilson, A., Stones-Havas, S., Cheung, M., Sturrock, S., et al. (2012). Geneious Basic: an integrated and extendable desktop software platform for the organization and analysis of sequence data. Bioinformatics 28, 1647–1649. doi: 10.1093/bioinformatics/bts199
Komanich, I. G. (1982). Kariologicheskoe issledovanie vidov roda Juglans, L. Byull. Glavn. Bot. Sada (Moscow). 125, 73–79.
Kurtz, S., Choudhuri, J. V., Ohlebusch, E., Schleiermacher, C., Stoye, J., and Giegerich, R. (2001). REPuter: the manifold applications of repeat analysis on a genomic scale. Nucleic Acids Res. 29, 4633–4642. doi: 10.1093/nar/29.22.4633
Librado, P., and Rozas, J. (2009). DnaSP v5: a software for comprehensive analysis of DNA polymorphism data. Bioinformatics 25, 1451–1452. doi: 10.1093/bioinformatics/btp187
Liu, C., Shi, L., Zhu, Y., Chen, H., Zhang, J., Lin, X., et al. (2012a). CpGAVAS, an integrated web server for the annotation, visualization, analysis, and GenBank submission of completely sequenced chloroplast genome sequences. BMC Genomics 13:715. doi: 10.1186/1471-2164-13-715
Liu, J., Qi, Z. C., Zhao, Y. P., Fu, C. X., and Xiang, Q. Y. (2012b). Complete cpDNA genome sequence of Smilax china and phylogenetic placement of Liliales–Influences of gene partitions and taxon sampling. Mol. Phylogenet. Evol. 64, 545–562. doi: 10.1016/j.ympev.2012.05.010
Logacheva, M. D., Samigullin, T. H., Dhingra, A., and Penin, A. A. (2008). Comparative chloroplastgenomics and phylogenetics of Fagopyrum esculentum ssp. ancestral a wild ancestor of cultivatedbuckwheat. BMC Plant Biol. 8:59. doi: 10.1186/1471-2229-8-59
Lohse, M., Drechsel, O., Kahlau, S., and Bock, R. (2013). OrganellarGenomeDRAW—a suite of tools for generating physical maps of plastid and mitochondrial genomes and visualizing expression data sets. Nucleic Acids Res. 41, W575–W581. doi: 10.1093/nar/gkt289
Lowe, T. M., and Eddy, S. R. (1997). tRNAscan-SE: a program for improved detection of transfer RNA genes in genomic sequence. Nucleic Acids Res. 25, 955–964. doi: 10.1093/nar/25.5.0955
Lu, S., Hou, M., Du, F. K., Li, J., and Yin, K. (2016). Complete chloroplast genome of the Oriental white oak: Quercus aliena Blume. Mitochondrial DNA A 27, 2802–2804. doi: 10.3109/19401736.2015.1053074
Manning, W. E. (1978). The classification within the Juglandaceae. Ann. Mo. Bot. Gard. 65, 1058–1087.
Mariac, C., Scarcelli, N., Pouzadou, J., Barnaud, A., Billot, C., Faye, A., et al. (2014). Cost-effective enrichment hybridization capture of chloroplast genomes at deep multiplexing levels for population genetics and phylogeography studies. Mol. Ecol. Resour. 14, 1103–1113. doi: 10.1111/1755-0998.12258
Martin, G., Baurens, F. C., Cardi, C., Aury, J. M., and D'Hont, A. (2013). The complete chloroplast genome of banana (Musa acuminata, Zingiberales): insight into plastid monocotyledon evolution. PLoS ONE 8:e67350. doi: 10.1371/journal.pone.0067350
Melotto-Passarin, D. M., Tambarussi, E. V., Dressano, K., De Martin, V. F., and Carrer, H. (2011). Characterization of chloroplast DNA microsatellites from Saccharum spp and related species. Genet Mol. Res. 10, 2024–2033. doi: 10.4238/vol10-3gmr1019
Millen, R. S., Olmstead, R. G., Adams, K. L., Palmer, J. D., Lao, N. T., Heggie, L., et al. (2001). Many parallel losses of infA from chloroplast DNA during angiosperm evolution with multiple independent transfers to the nucleus. Plant Cell 13, 645–658. doi: 10.1105/tpc.13.3.645
Nie, X., Lv, S., Zhang, Y., Du, X., Wang, L., Biradar, S. S., et al. (2012). Complete chloroplast genome sequence of a major invasive species, crofton weed (Ageratinaadenophora). PLoS ONE 7:e36869. doi: 10.1371/journal.pone.0036869
Novikova, P. Y., Hohmann, N., Nizhynska, V., Tsuchimatsu, T., Ali, J., Muir, G., et al. (2016). Sequencing of the genus Arabidopsis identifies a complex history of nonbifurcating speciation and abundant trans-specific polymorphism. Nat. Genet. 48, 1077–1082. doi: 10.1038/ng.3617
Park, S., Jansen, R. K., and Park, S. (2015). Complete plastome sequence of Thalictrum coreanum (Ranunculaceae) and transfer of the rpl32 gene to the nucleus in the ancestor of the subfamily Thalictroideae. BMC Plant Biol. 15:1. doi: 10.1186/s12870-015-0432-6
Patel, R. K., and Jain, M. (2012). NGS QC Toolkit: a toolkit for quality control of next generation sequencing data. PLoS ONE 7:e30619. doi: 10.1371/journal.pone.0030619
Pollegioni, P., Woeste, K. E., Chiocchini, F., Del Lungo, S., Olimpieri, I., Tortolano, V., et al. (2015). Ancient humans influenced the current spatial genetic structure of common walnut populations in Asia. PLoS ONE 10:e0135980. doi: 10.1371/journal.pone.0135980
Posada, D., and Buckley, T. R. (2004). Model selection and model averaging in phylogenetics: advantages of Akaike information criterion and Bayesian approaches over likelihood ratio tests. Syst. Biol. 53, 793–808. doi: 10.1080/10635150490522304
Posada, D., and Crandall, K. A. (1998). Modeltest: testing the model of DNA substitution. Bioinformatics 14, 817–818. doi: 10.1093/bioinformatics/14.9.817
Qi, J., Hao, Y., Zhu, Y., Wu, C., Wang, W., and Leng, P. (2011). Studies on Germplasm of Juglans by EST-SSR Markers. Acta Hortic. Sinica 38, 441–448.
Raman, G., and Park, S. (2016). The complete chloroplast genome sequence of Ampelopsis: gene organization, comparative analysis, and phylogenetic relationships to other angiosperms. Front. Plant Sci. 7:341. doi: 10.3389/fpls.2016.00341
Ronquist, F., and Huelsenbeck, J. P. (2003). MrBayes 3: bayesian phylogenetic inference under mixed models. Bioinformatics 19, 1572–1574. doi: 10.1093/bioinformatics/btg180
Roullier, C., Rossel, G., Tay, D., McKey, D., and Lebot, V. (2011). Combining chloroplast and nuclear microsatellites to investigate origin and dispersal of new world sweet potato landraces. Mol. Ecol. 20, 3963–3977. doi: 10.1111/j.1365-294X.2011.05229.x
Soltis, D. E., Smith, S. A., Cellinese, N., Wurdack, K. J., Tank, D. C., Brockington, S. F., et al. (2011). Angiosperm phylogeny: 17 genes, 640 taxa. Am. J. Bot. 98, 704–730. doi: 10.3732/ajb.1000404
Stamatakis, A. (2014). RAxML version 8: a tool for phylogenetic analysis and post-analysis of large phylogenies. Bioinformatics 30, 1312–1313. doi: 10.1093/bioinformatics/btu033
Stanford, A. M., Harden, R., and Parks, C. R. (2000). Phylogeny and biogeography of Juglans (Juglandaceae) based on matK and ITS sequence data. Am. J. Bot. 87, 872–882. doi: 10.2307/2656895
Steane, D. A. (2005). Complete nucleotide sequence of the chloroplast genome from the Tasmanian blue gum, Eucalyptus globulus (Myrtaceae). DNA Res. 12, 215–220. doi: 10.1093/dnares/dsi006
Stegemann, S., Keuthe, M., Greiner, S., and Bock, R. (2012). Horizontal transfer of chloroplast genomes between plant species. Proc. Natl. Acad. Sci. U.S.A. 109, 2434–2438. doi: 10.1073/pnas.1114076109
Tamura, K., Peterson, D., Peterson, N., Stecher, G., Nei, M., and Kumar, S. (2011). MEGA5: molecular evolutionary genetics analysis using maximum likelihood, evolutionary distance, and maximum parsimony methods. Mol. Biol. Evol. 28, 2731–2739. doi: 10.1093/molbev/msr121
The Angio sperm Phylogeny Group III (2009). An update of the Angiosperm Phylogeny Group classification for the orders and families of flowering plants: APG III. Bot. J. Linn. Soc. 161, 105–121. doi: 10.1111/j.1095-8339.2009.00996.x
Thomas, D. C., Hughes, M., Phutthai, T., Ardi, W. H., Rajbhandary, S., Rubite, R., et al. (2012). West to east dispersal and subsequent rapid diversification of the mega-diverse genus Begonia (Begoniaceae) in the Malesian archipelago. J. Biogeogr. 39, 98–113. doi: 10.1111/j.1365-2699.2011.02596.x
Timme, R. E., Kuehl, J. V., Boore, J. L., and Jansen, R. K. (2007). A comparative analysis of the Lactuca and Helianthus (Asteraceae) plastidgenomes: identification of divergent regions and categorization of shared repeats. Am. J. Bot. 94, 302–312. doi: 10.3732/ajb.94.3.302
Untergrasser, A., Cutcutache, I., Koressaar, T., Ye, J., Faircloth, B. C., Remm, M., et al. (2012). Primer3-new capabilities and interfaces. Nucleic Acids Res. 40:e115. doi: 10.1093/nar/gks596
Wang, H., Pan, G., Ma, Q., Zhang, J., and Pei, D. (2015). The genetic diversity and introgression of Juglans regia and Juglans sigillata in Tibet as revealed by SSR markers. Tree Genet. Genomes 11, 1–11. doi: 10.1007/s11295-014-0804-3
Wang, H., Pei, D., Gu, R. S., and Wang, B. Q. (2008). Genetic diversity and structure of walnut populations in central and southwestern China revealed by microsatellite markers. J. Am. Soc. Hortic. Sci. 133, 197–203.
Wang, S., Shi, C., and Gao, L. Z. (2013). Plastid genome sequence of a wild woody oil species, Prinsepia utilis, provides insights into evolutionary and mutational patterns of rosaceae chloroplast genomes. PLoS ONE 8:e73946. doi: 10.1371/journal.pone.0073946
Wang, W. T., Xu, B., Zhang, D. Y., and Bai, W. N. (2016). Phylogeography of postglacial range expansion in Juglans mandshurica (Juglandaceae) reveals no evidence of bottleneck, loss of genetic diversity, or isolation by distance in the leading-edge populations. Mol. Phylogenet. Evol. 102, 255–264. doi: 10.1016/j.ympev.2016.06.005
Waterway, M. J., Hoshino, T., and Masaki, T. (2009). Phylogeny, species richness, and ecological specialization in Cyperaceae tribe Cariceae. Bot. Rev. 75, 138–159. doi: 10.1007/s12229-008-9024-6
Weng, M. L., Blazier, J. C., Govindu, M., and Jansen, R. K. (2013). Reconstruction of the ancestral plastid genome in Geraniaceae reveals a correlation between genome rearrangements, repeats and nucleotide substitution rates. Mol. Biol. Evol. 31, 645–659. doi: 10.1093/molbev/mst257
Wenheng, C. S. Y. (1987). Taxonomic studies of ten species of the genus Juglans based on isozymic zymograms. Acta Hortic. Sinica 2, 002.
Woodworth, R. H. (1930). Meiosis of microsporogenesis in the Juglandaceae. Am. J. Bot. 17, 863–869. doi: 10.2307/2435868
Wu, Y., Pei, D., Xi, S., and Li, R. (2000). Study on the genetic relationships among species of walnut by using RAPD. Acta Hortic. Sinica 27, 17–22.
Wyman, S. K., Jansen, R. K., and Boore, J. L. (2004). Automatic annotation of organellar genomes with DOGMA. Bioinformatics 20, 3252–3255. doi: 10.1093/bioinformatics/bth352
Xi, S. (1987). Gene resources of Julgans and genetic improvement of Julgans reiga in China. Scientia Silvae Sinicae 23, 342–349.
Xue, J., Wang, S., and Zhou, S. L. (2012). Polymorphic chloroplast microsatellite loci in Nelumbo (Nelumbonaceae). Am. J. Bot. 99, e240–244. doi: 10.3732/ajb.1100547
Yang, A. H., Zhang, J. J., Yao, X. H., and Huang, H. W. (2011). Chloroplast microsatellite markers in Liriodendron tulipifera (Magnoliaceae) and cross-species amplification in L. chinense. Am. J. Bot. 98, e123–e126. doi: 10.3732/ajb.1000532
Yang, Y., Zhou, T., Duan, D., Yang, J., Feng, L., and Zhao, G. (2016). Comparative analysis of the complete chloroplast genomes of five Quercus species. Front. Plant Sci. 7:959. doi: 10.3389/fpls.2016.00959
Yang, Z. (2007). PAML 4: phylogenetic analysis by maximum likelihood. Mol. Biol. Evol. 24, 1586–1591. doi: 10.1093/molbev/msm088
Yao, X., and Huang, H. (2016). “Cytoplasmic DNA in Actinidia,” in The Kiwifruit Genome, eds R. Testolin, H.-W. Huang, and A. R. Ferguson (Udine; Auckland; Guangzhou: Springer International Publishing), 43–54.
Zhang, Z., Gao, Y., and Zhao, Y. (2009). Genetic relationship and diversity of eight Juglans species in China estimated through AFLP analysis. Int. Walnut Symp. 861, 143–150. doi: 10.17660/ActaHortic.2010.861.18
Zhang, Z., Li, J., Zhao, X. Q., Wang, J., Wong, G. K., and Yu, J. (2006). KaKs_Calculator: calculating Ka and Ks through model selection and model averaging. Genomics Proteomics Bioinformatics 4, 259–263. doi: 10.1016/S1672-0229(07)60007-2
Keywords: persian walnut, ma walnut, iron walnut, chinese walnut, manchurian walnut, phylogeny, China, butternut
Citation: Hu Y, Woeste KE and Zhao P (2017) Completion of the Chloroplast Genomes of Five Chinese Juglans and Their Contribution to Chloroplast Phylogeny. Front. Plant Sci. 7:1955. doi: 10.3389/fpls.2016.01955
Received: 20 September 2016; Accepted: 09 December 2016;
Published: 06 January 2017.
Edited by:
Jill Christine Preston, University of Vermont, USAReviewed by:
Rob DeSalle, American Museum of Natrual History, USAThomas Marcussen, Norwegian University of Life Sciences, Norway
Guo Jian Zhang, Chinese Academy of Forestry, China
Copyright © 2017 Hu, Woeste and Zhao. This is an open-access article distributed under the terms of the Creative Commons Attribution License (CC BY). The use, distribution or reproduction in other forums is permitted, provided the original author(s) or licensor are credited and that the original publication in this journal is cited, in accordance with accepted academic practice. No use, distribution or reproduction is permitted which does not comply with these terms.
*Correspondence: Peng Zhao, cGVuZ3poYW9Abnd1LmVkdS5jbg==