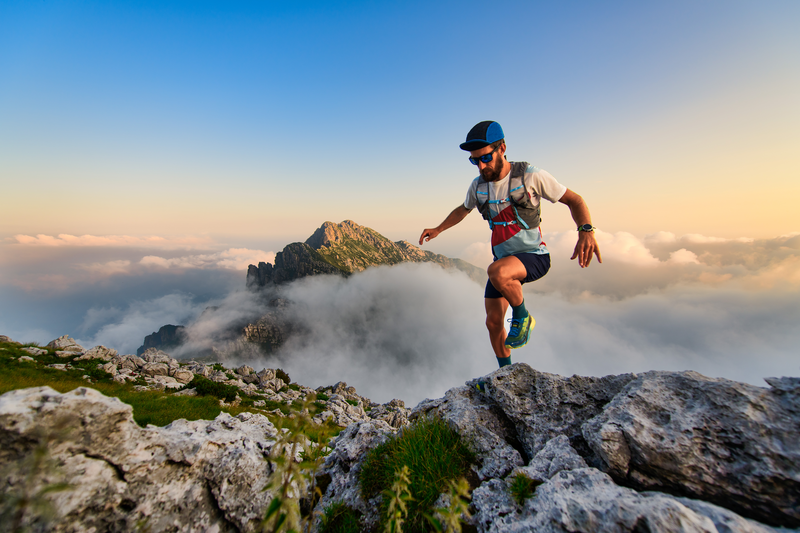
95% of researchers rate our articles as excellent or good
Learn more about the work of our research integrity team to safeguard the quality of each article we publish.
Find out more
REVIEW article
Front. Plant Sci. , 21 October 2016
Sec. Plant Physiology
Volume 7 - 2016 | https://doi.org/10.3389/fpls.2016.01457
This article is part of the Research Topic Roots – the hidden provider View all 17 articles
Grafting is a centuries-old technique used in plants to obtain economic benefits. Grafting increases nutrient uptake and utilization efficiency in a number of plant species, including fruits, vegetables, and ornamentals. Selected rootstocks of the same species or close relatives are utilized in grafting. Rootstocks absorb more water and ions than self-rooted plants and transport these water and ions to the aboveground scion. Ion uptake is regulated by a complex communication mechanism between the scion and rootstock. Sugars, hormones, and miRNAs function as long-distance signaling molecules and regulate ion uptake and ion homeostasis by affecting the activity of ion transporters. This review summarizes available information on the effect of rootstock on nutrient uptake and utilization and the mechanisms involved. Information on specific nutrient-efficient rootstocks for different crops of commercial importance is also provided. Several other important approaches, such as interstocking (during double grafting), inarching, use of plant-growth-promoting rhizobacteria, use of arbuscular mycorrhizal fungi, use of plant growth substances (e.g., auxin and melatonin), and use of genetically engineered rootstocks and scions (transgrafting), are highlighted; these approaches can be combined with grafting to enhance nutrient uptake and utilization in commercially important plant species. Whether the rootstock and scion affect each other's soil microbiota and their effect on the nutrient absorption of rootstocks remain largely unknown. Similarly, the physiological and molecular bases of grafting, crease formation, and incompatibility are not fully identified and require investigation. Grafting in horticultural crops can help reveal the basic biology of grafting, the reasons for incompatibility, sensing, and signaling of nutrients, ion uptake and transport, and the mechanism of heavy metal accumulation and restriction in rootstocks. Ion transporter and miRNA-regulated nutrient studies have focused on model and non-grafted plants, and information on grafted plants is limited. Such information will improve the development of nutrient-efficient rootstocks.
Grafting is a special type of plant propagation, in which a part of a plant (scion) is joined to another plant (rootstock) for the two parts to grow together and form a new plant. Grafting commonly occurs in nature, and the occurrence of natural grafts might have inspired humans to use grafting in the agriculture sector a thousand years ago (Mudge et al., 2009). The rootstocks used for a specific crop are the crop's close relatives or wild selections (mostly within the genera), but natural grafts between different families have also been observed (Warschefsky et al., 2016). Grafting has been practiced in fruit trees for a long time; however, its application in vegetables is relatively new. Various fruit grafting references that appear in the Bibble and ancient Greek and Chinese literature suggest that grafting was utilized in Asia, Europe, and the Middle East in fifth century BC (Melnyk and Meyerowitz, 2015). Similarly, a Chinese book written in first century BC and a Korean book written in seventeenth century AD indicate that grafting was utilized in these periods to produce large gourd fruits (Lee and Oda, 2003). Grafting enhances plant vigor, extends the harvesting period (Lee et al., 2010), improves yield and fruit quality (Huang et al., 2009; Rouphael et al., 2010; Tsaballa et al., 2013), prolongs postharvest life (Zhao et al., 2011), tolerates low and high temperatures (López-Marín et al., 2013; Li et al., 2016b), deals with salinity and heavy metal stress (Santa-Cruz et al., 2002; Estañ et al., 2005; Albacete et al., 2009; Schwarz et al., 2010; Huang et al., 2013a; Wahb-Allah, 2014; Penella et al., 2015, 2016), increases drought and flooding stress endurance (Schwarz et al., 2010; Bhatt et al., 2015), improves water use efficiency (Cantero-Navarro et al., 2016), manages soil-borne and foliar pathogens (Louws et al., 2010; Arwiyanto et al., 2015; Miles et al., 2015; Suchoff et al., 2015), manages root knot nematodes (Lee et al., 2010), controls weeds (Dor et al., 2010; Louws et al., 2010), and produces new plant species (Fuentes et al., 2014). Commercial grafting is currently practiced in a number of plant species, including fruits (citrus, apple, mango, grape, peach, plum, apricot, almond, and cherry), vegetables (watermelon, melon, cucumber, tomato, pepper, eggplant, and bitter gourd), and ornamentals (rose, chrysanthemum, bougainvillea, and bonsai), to obtain economic benefits.
In addition to these commercially important benefits, grafting increases nutrient uptake and utilization efficiency in a number of plant species (Pulgar et al., 2000; Zen et al., 2004; Ahmed et al., 2006, 2007; Albacete et al., 2009, 2015a,b; Lee et al., 2010; Schwarz et al., 2013; Huang et al., 2013b; Esmaeili et al., 2015). The world's nutrient resources are finite (Venema et al., 2011) and thus require justified utilization; moreover, inorganic fertilizers are expensive. Scientists are working to modify the root architecture of cereal crops (monocots) to enhance nutrient uptake and utilization efficiency (Meister et al., 2014; Rogers and Benfey, 2015; Wissuwa et al., 2016). Rootstock grafting is used as an alternative for horticultural crops; appropriate and compatible rootstocks are utilized to improve water and nutrient acquisition and nutrient utilization efficiency (Gregory et al., 2013; Albacete et al., 2015a,b). Nutrient efficiency is a general term that refers to the capacity of a plant to acquire and use nutrients. It is measured by plant dry weight produced per unit of nutrient supplied (g DW/g nutrient supplied). This parameter includes nutrient uptake efficiency and nutrient use efficiency (Gerendás et al., 2008). Although other efficiency indicators, such as nutrient harvest index, nutrient influx rate, and nutrient partitioning, have been proposed (Rengel and Damon, 2008), measurement of plant growth and harvestable yield per unit input of applied fertilizer remains reliable (Venema et al., 2011; Santa-Maria et al., 2015).
Several reviews have been conducted on grafting history, grafting methods, breeding and selection of rootstocks, biotic and abiotic stresses, pathogens, amelioration of heavy metals, and hormonal signaling (Lee, 1994; Lee and Oda, 2003; Davis et al., 2008; Kubota and McClure, 2008; Aloni et al., 2010; Lee et al., 2010; Louws et al., 2010; Pérez-Alfocea et al., 2010; Rouphael et al., 2010; Savvas et al., 2010; Schwarz et al., 2013; Edelstein et al., 2016), but none of these reviews sufficiently elucidated the role of grafting in modifying ion uptake and accumulation. Therefore, the current review summarizes available information on the effects of rootstocks on enhancing nutrient uptake, accumulation, and utilization as well as the mechanism involved. Additionally, other important approaches are presented; these approaches can be combined with grafting to further enhance nutrient acquisition and utilization efficiency.
The use of rootstocks in fruit plants affects tree vigor and size, precocity, fruit quality and taste, harvestable yield, resistance to pests, and tolerance against edaphic and environmental conditions by invigorating the scions and increasing nutrient uptake, transport, and utilization efficiency (Albrigo, 1977; Ahmed et al., 2006, 2007; Castle, 2010; Habran et al., 2016). In a previous study, the grafting of kinnow (Citrus reticulata Blanco) onto nine different rootstocks increased the concentration of nitrogen (4–18%), phosphorous (11–77%), and potassium (3–43%) in the leaves of the grafted plants; the number of fruits per plant increased to 0.12–5.63 times that of poorly performing rootstock (Ahmed et al., 2007). In another study, total nitrogen accumulation and utilization efficiency differed in various citrus rootstocks; rough lemon (Citrus jambhiri Lush.) accumulated a total nitrogen value of 22.1 mg/g DW, whereas Cleopatra mandarin (Citrus reshni hort. ex Tanaka) accumulated only 6.1 mg/g DW (Sorgona et al., 2006). Boron concentration in the leaves, stems, and roots of different citrus rootstocks is significantly affected in normal and boron-deficient conditions (Mei et al., 2011). Citrange rootstock [C. sinensis (L.) Osb. × P. trifoliata (L.) Raf.] is superior to trifoliate orange [Poncirus trifoliata (L.) Raf.] under both low and high levels of boron supply. Although the level of boron in different plant parts grafted onto citrange is lower than that grafted onto trifoliate orange, the ratio of semi-bound boron to free boron is much higher in citrange grafted plants; this higher ratio increases boron use efficiency (Liu et al., 2011, 2013). Evidently, uptake capacity is not the only important factor; the transport and reallocation of cellular boron to cell walls also affect rootstock responses to nutrient deficiency. Wang et al. (2016a) recently applied inarching (a special type of grafting) of Carrizo citrange [C. sinensis (L.) Osb. × P. trifoliate (L.) Raf.] to enhance boron uptake in Newhall navel orange [C. sinensis (L.) Osb. cv. Newhall] budded onto trifoliate orange [P. trifoliata (L.) Raf.]. Inarching significantly increased the levels of boron in the new leaves, new twigs, scion stem, and new rootstock stem under boron-adequate and boron-deficient conditions. This technique can improve nutrient supply in already growing grafted or self-rooted fruit plants under field conditions.
In apples, Cong et al. (2014) found that rootstocks have different potassium uptakes and accumulation efficiencies; these differences become increasingly pronounced under potassium-deficient conditions. Zarrouk et al. (2005) discovered that the concentrations of macro and micronutrients (N, P, K, Ca, Mg, Fe, Mn, Zn, Na, and Cu) in the leaves and flowers of peach are significantly affected by different rootstocks. Similarly, Amiri et al. (2014) reported that apple rootstocks (M9, MM106, and MM111) increase the concentration of N (5–34%), Mg (5–68%), Zn (10–39%), Fe (4–34%), and Mn (11–70%) and reduce the uptake of K (6–19%), and Ca (10–28%). Trees grafted onto M9 are efficient in N, Mn, and Fe uptake but perform poorly in K and Ca uptake. MM106 rootstock possesses the highest efficiency in P uptake and transport. In this study, the fruit yield of plants grafted onto MM111 increased by 44% compared with plants grafted onto M9 rootstock. Mestre et al. (2015) observed that the concentrations of all macro and micronutrients differ significantly in the leaves of Big Top nectarine (Prunus persica var. nectarina) grafted onto 12 Prunus rootstocks. The rootstocks demonstrate a selective behavior for different elements, such as K, Ca, and Cu.
Rootstocks significantly alter the concentration of nutrients in sweet cherry leaves. The GiSelA 6 rootstock (Prunus cerasus × Prunus canescens, Gi 148/1) performs well in N, P, K, Zn, B, and Mn uptake and transport, but trees grafted onto it tend to develop Ca, Mg, and Cu deficiencies. Similarly, the Prunus mahaleb rootstock performs efficiently in N, P, K, Ca, Mg, Fe, and Cu uptake and transport but performs poorly in Zn, B, and Mn absorption and translocation in plants. The leaves of plants grafted onto Prunus fruticosa Prob. show deficiencies in several nutrients (N, P, Ca, Mg, and Cu) and are thus unfit for use (Hrotko et al., 2014). Slowik et al. (1979) studied the avocado (Persea americana Mill.) cultivar Hass by using Duke and Topa Topa (avocado rootstocks of Mexican origin) and concluded that only the concentrations of N, P, Mg, Cu, Mn, and Fe are affected by the rootstocks; no significant differences were observed for K, Ca, Na, Cl, and Zn. Sherafati et al. (2011) found that in pistachio (Pistacia vera L.), the K, Zn, and Fe contents of the leaves are affected by rootstock and scion combinations. Akbari scion (Pistacia vera L.) budded onto Badami rootstock (P. vera L.) efficiently absorbed K and Zn (1.56% and 11.05 ppm, respectively), whereas minimal amounts of K and Zn (0.80% and 7.33 ppm, respectively) were found in Akbari scion budded onto Daneshmandi rootstock (P. vera L.). Barg-seyah scion (P. vera L.) budded onto Kalle-ghouchi rootstock (P. vera L.) absorbed the largest amount of Fe (241 ppm) and Cu (12.15 ppm) among all rootstock and scion combinations.
Ibacache and Sierra (2009) demonstrated that rootstocks significantly affect grapevine nutrition. They studied the effect of 10 rootstocks on grapevines and found significant differences in nutrient concentration in the petioles of four scion cultivars. The petiole P levels doubled in all scion cultivars when Salt Creek rootstock (Vitis vinifera L.) was used. Potassium was also increased by up to 5–155% by rootstocks in all scion cultivars. Similarly, N levels increased to 67% in Flame Seedless (V. vinifera L.), 77% in Red Globe (V. vinifera L.), 33% in Thompson Seedless (V. vinifera L.), and 8.5% in Superior Seedless (V. vinifera L.) scions compared with self-rooted vines. Bavaresco et al. (2003) utilized two rootstocks for grapevines and observed that the hybrid rootstock 41 B (V. vinifera × V. berlandieri) significantly increased the nutrient uptake and whole plant dry mass (24%) compared with 3309 C (V. riparia × V. rupestris). The total N, P, K, Ca, and Mg contents of the plant increased by 38, 21.5, 18, 35, and 12%, respectively. Additionally, the concentrations of micronutrients (Zn, Mn, and B) increased by 16, 94, and 18%, respectively, whereas Cu slightly decreased (1%). Lecourt et al. (2015) observed that rootstocks significantly affect the plant growth (dry matter) and concentration of macro and micronutrients in the leaves and root tissues of grapevine (V. vinifera L.). Cabernet Sauvignon (V. vinifera L.) grafted onto Riparia Gloire de Montpellier (V. riparia L.) increased the leaf N (3%), K (44%), S (70%), Ca (27%), Mg (58%), Fe (65%), Zn (57%), B (93%), Mn (83%), and Al (46%) concentrations compared with Cabernet Sauvignon grafted onto 1103 Paulsen (V. rupestris × V. berlandieri) irrigated with 2.45 mmol N supply. Habran et al. (2016) investigated the effect of N and rootstock on N uptake and their effect on secondary metabolism in grapes. Cabernet Sauvignon (V. vinifera L.) grafted onto 110 Richter increased the leaf and petiole N concentration by up to 14% and 1%, and 9% and 30% under low and optimum levels of N supply, respectively, compared with Cabernet Sauvignon grafted onto Riparia Gloire de Montpellier (V. riparia). Rootstocks also affected vine growth (14–16%), berry weight, and composition at the time of harvest. Zamboni et al. (2016) recently declared M1 rootstock [106-8 (V. riparia × V. cordifolia × V. rupestris) × Resseguier n.4 (V. berlandieri)] as superior over M3 [R 27 (V. berlandieri × V. riparia) × Teleki 5C (V. berlandieri × V. riparia)], 1103P (V. berlandieri × V. rupestris), and 101–14 (V. riparia × V. rupestris) rootstocks because of its balanced leaf nutrient profile, nitrogen use efficiency, and improved vine growth (30%). Various rootstocks behave differently in terms of yield, physiological responses, and severity of Fe deficiency; proper rootstock selection can mitigate nutrient deficiency symptoms and responses in grapevines (Covarrubias et al., 2016).
Grafting in vegetable crops is a relatively new procedure that has gained popularity in the last few decades (Lee et al., 2010). Similar to fruit crops, melon (Cucumis melo L.) cultivars Yuma and Gallicum grafted onto three Cucurbita maxima (Shintoza, RS-841, and Kamel) rootstocks show a significant variation in macronutrients in leaf tissues. Grafting increases the yield (88%–121%) and concentration of P and N in the leaves by up to 6–58% and 6–81%, respectively, compared with self-rooted melon plants (Ruiz et al., 1997). Zhang et al. (2012) found that P uptake and utilization in grafted watermelons are better than those in self-rooted watermelons under low P supply. Various rootstocks perform differently; watermelon grafted onto pumpkin (Cucurbita moschata Duch.) performs better than watermelon grafted onto bottle gourd (Lagenaria siceraria Standl.). Huang et al. (2013b) found that grafting of watermelon onto Hongdun (Citrullus lanatus sp.) and Jingxinzhen No.4 (C. moschata Duch.) increases K uptake and whole plant dry mass (1.82 fold) and induces low K tolerance in the watermelon scion. Uygur and Yetisir (2009) worked on watermelons and concluded that the watermelon [C. lanatus (Thunb.) Matsum and Nakai] cultivar Crimson Tide grafted onto gourd rootstock (L. siceraria Standl.) landraces significantly increases N and K uptake from the soil, especially under salt stress conditions. The concentration of P in the shoots of grafted plants is almost twice that in non-grafted watermelon plants. Therefore, bottle gourds, especially the Lagenaria type, can be utilized as a rootstock for watermelon under saline conditions.
Comprehensive mineral nutrient analysis has shown that the concentration of macro and micronutrients in the leaves, fruit rind, fruit flesh, and seeds of grafted watermelon is altered by gourd rootstocks [Ferro and RS841 (C. maxima × C. moschata) and Argentario and Macis (Lagenaria hybrid)]. In leaves, rootstocks increase the N, K, Ca, and Mg concentrations by up to 12–28, 8–36, 13–81, and 12–300%, respectively, and reduce the concentrations of P, Fe, Zn, Mn, and Cu. In fruit rind, flesh, and seeds, rootstocks increase the concentration of all nutrients, thereby proving the superiority of specific graft combinations over self-rooted plants (Yetisir et al., 2013). Watermelon cultivar Zaojia 8424 [C. lanatus (Thunb.) Matsum and Nakai] grafted onto bottle gourd cv. Jingxinzhen No.1 (L. siceraria Standl.) and pumpkin cv. Qingyanzhen No.1 (C. maxima × C. moschata) increases the total uptake (mg/plant) and concentration [mg g−1 dry weight (DW)] of N, K, Ca, Fe, Mg, and Mn in the roots, stems, leaves, fruit rind, and flesh of grafted plants. The total nutrient uptake of plants grafted onto bottle gourd and pumpkin increases by 30.41 and 49.14%, respectively, at the fruit development stage and by 21.33 and 47.46%, respectively, at the fruit maturation stage compared with non-grafted watermelon plants (Huang et al., 2016a). Another experiment showed that pumpkin rootstock (C. moschata Duch.) increases the Mg2+ uptake (39%) and whole plant dry mass (1.71 fold) of watermelon plants compared with self-grafted plants under low Mg conditions (Huang et al., 2016b). The C. maxima variety Dulce Maravilla clearly increases Fe uptake and translocation to watermelon scions (Rivero et al., 2004).
Grafting is useful in manipulating plant growth and is used in multiple ways. Zhang et al. (2010) reported that when cucumber plants (Cucumis sativus L. cv. Xintaimici) are grafted onto Cucurbita ficifolia, the uptake of Cu from the soil under Cu stress conditions is significantly reduced. The concentration of Cu in the stem and leaves of the scion is lower in grafted plants than in self-rooted plants; moreover, the concentration of Cu in the roots of grafted plants is higher. This result suggests that the C. ficifolia rootstock acts as a Cu accumulator and reduces Cu transport to the scion. However, its sensing, signaling, regulation, and withholding mechanisms still require further explanation. Grafting increases the uptake of K and limits the transport of Na and Cl ions to the scion compared with self-rooted plants under salt stress conditions in cucumber. Grafting also enhances nutrient utilization efficiency as manifested by improved shoot biomass production, yield, and fruit quality (Rouphael et al., 2008; Zhu et al., 2008; Albacete et al., 2009, 2015a,b; Huang et al., 2009; Colla et al., 2012, 2013; Farhadi and Malek, 2015; Gao et al., 2015).
Grafting of tomato (Solanum lycopersicum L.) onto Maxifort rootstock (S. lycopersicum L. × Solanum habrochaites) improves scion growth (11%) and concentration of macro and micronutrients (Table 1). However, different forms of nitrogen (, ) exert a significant effect on the uptake of other elements; for example, increased availability of reduces the uptake of Ca and Mg in plant tissues (Borgognone et al., 2013). Savvas et al. (2009) reported that grafting tomato onto He-Man rootstock (S. lycopersicum × S. habrochaites; Syngenta Seeds, Basel, Switzerland) improves fruit yield (11.5%) and increases the concentrations of Ca, K, and Zn by 18, 8, and 11%, respectively, in tomato leaves and reduces the uptake of Mg (27%) and Fe (27%), especially under low levels of Mn. In pepper and eggplant, rootstocks also show a positive response by modifying ion uptake and acquisition of macro and micronutrients and by providing protection from heavy metals (Leonardi and Giuffrida, 2006; Arao et al., 2008; Penella et al., 2015).
Rootstocks play a pivotal role in fertilizer management because they can ensure the justified utilization of available or applied fertilizers in the soil. Harvestable yield is an important criterion of nutrient utilization efficiency. An overview of reported percentage growth and yield increments obtained through grafting among various vegetables is presented in Table 1.
Previous studies on ornamental crops have elucidated the effects of rootstock on the nutrient uptake of such crops. Gammon and McFadden (1979) grafted rose onto seven different rootstocks and determined that rootstock combinations significantly increase nutrient uptake and accumulation. Rosa fortuniana Lindl. triggered Mn accumulation five times more than Rosa odorata; however, R. odorata was superior to R. fortuniana in terms of K accumulation. Niu and Rodriguez (2008) evaluated the performance of four rose rootstocks [Dr. Huey (Rosa hybrida L.), R. fortuniana Lindl., Rosa multiflora Thunb., and R. odorata (Andr.) Sweet] under chloride-or sulfate-dominated salinity and observed that rootstocks behave differentially in terms of Ca, Mg, K, Na, and Cl uptake. R. multiflora accumulates more Na than R. odorata, and R. fortuniana accumulates the least amount. However, R. multiflora retains most of Na in the roots, whereas R. odorata transports 57% of the absorbed Na to the shoots. According to another study, commercial rose varieties grafted onto different rootstocks significantly differ in macro (P, K, and Mg) and micro (Cl, Mn, Fe, B, Zn, and Na) nutrient concentrations (Cabrera, 2002). The N use efficiency (NUE) in tobacco can be improved by grafting it onto Nicotiana tabacum H-20 rootstock (Ruiz et al., 2006). Several details on increased nutrient uptake through grafting are summarized in Table 1. These pieces of evidence demand the careful selection of the rootstock to enhance nutrient use efficiency in ornamental crops. Grafting is also utilized to produce bonsai plants (an important category of ornamentals); in bougainvillea and several other ornamentals, it is also used to produce plants bearing flowers of different colors or multicolored flowers on the same plant to enhance anesthetics (Relf, 2015). In a recent report, Zhang et al. (2013) observed increased productivity and rooting ability of cuttings obtained from grafted chrysanthemum. In the near future, an increase in the use of grafting is expected in ornamental crops. However, serious efforts are required for the selection of compatible rootstocks in ornamental plants.
Rootstocks modify the ion uptake in grafted plants. The final concentration of nutrients is a result of uptake, transport, recirculation, and growth. The absorption of nutrients from the soil is affected by numerous factors, including soil properties (pH, cation exchange capacity, and concentration of the nutrients) and root characteristics (root architecture, organic acids and metabolites exudation capacity, and transport ability). This article focuses on root-related mechanisms.
In cereal crops, modification of the root architecture is a popular means to enhance ion uptake, accumulation, and utilization efficiency, and this means has been discussed in several reviews (Meister et al., 2014; Rogers and Benfey, 2015; Wissuwa et al., 2016). Nutrient uptake and utilization in horticultural crops are enhanced by selecting appropriate rootstocks. Rootstocks play a vital role in manipulating the nutrient status of the scions by directly affecting ion uptake and transport (Amiri et al., 2014). The ion concentration in the roots and shoots of grafted grapevines depends on rootstock genotype (Lecourt et al., 2015). The selected rootstocks have a vigorous root system, i.e., large main roots, many lateral roots and root hair, large total root length, and root surface area. These roots absorb a large amount of water and nutrients by exploring wide and deep soil volumes (Pérez-Alfocea, 2015). According to a report, the root dry weight of watermelon grafted onto Jingxinzhen No. 4 pumpkin (C. moschata Duch.) is 2.24 times that of self-rooted plants (Huang et al., 2013b), and the K uptake efficiency of grafted plants is 2.02 times that of self-rooted plants. Similarly, the root dry weight of a rose cultivar (Rosa “BAIore”) is thrice that of a poorly performing cultivar Rosa “Frontenac” under stress conditions (Harp et al., 2015). Therefore, this vigorous root system of rootstock can capture and transport a large amount of nutrients to the above ground scion. Vigorous rootstocks have high levels of sugars, amino acids, and enzymes and secrete organic acids in the soil, which are important in nutrient mobilization and affect nutrient availability and uptake (Jaitz et al., 2011; Khorassani et al., 2011; Dam and Bouwmeester, 2016). Jiménez et al. (2011) reported that high concentrations of root sucrose, total organic and amino acids, and phosphoenolpyruvate carboxylase activity in the roots of Prunus rootstocks subjected to iron deficiency promote root growth and trigger iron uptake. Similarly, under iron-deficient conditions, the roots of Malus species secrete acids, reduce the pH of rhizosphere, and significantly increase the activities of root ferric chelate reductase (FCR), thereby leading to increased ferrous uptake (Zha et al., 2014).
Rootstocks modify ion uptake and transport to the scion by affecting the activities of ion transporters. Transporters are involved in ion uptake, root compartmentation, and translocation of these absorbed ions to aboveground plant parts. Transporters are highly specific under different environmental conditions and in the concentrations of specific nutrients present in the soil. For example, in two citrus rootstocks [Carrizo citrange (C. sinensis [L.] Osb. × P. trifoliata [L.] Raf.) and Cleopatra mandarin (C. reshni Hort. ex Tanaka)], eight different K transporters have been identified (Caballero et al., 2013). Grafting affects the activity of ion transporters. The expression levels of Mg transporter genes MGT1, MGT3, MGT4, MGT5, and MGT7 are much higher in the roots of Jingxinzhen No.4 pumpkin (C. moschata Duch.) used as rootstock compared with the roots of self-rooted watermelon cultivar Zaojia 8424 [C. lanatus (Thunb.) Matsum and Nakai], especially under low Mg conditions. These higher expression levels enhance the Mg2+ uptake of watermelon grafted onto Jingxinzhen No.4 pumpkin rootstock (Huang et al., 2016b). However, the signaling mechanism in the activation of these genes still requires further investigation. Similarly, the activities of ferric-related uptake and transport genes (NAS1, FRD3, and NRMAP3) are significantly increased under iron-deficient conditions in apple rootstock Malus xiaojinensis, thereby increasing the ferrous uptake (Zha et al., 2014). Han et al. (2009) reported that a genetically engineered bottle gourd (L. siceraria Standl.) rootstock having a modified Arabidopsis Ca2+/H+ exchanger sCAX2B improves the biomass and quality of watermelon fruits by enhancing nutrient transport to the scion. This transporter provides enhanced Ca2+ transporter substrate specificity and decreases Mn transport capability. Gonzalo et al. (2011) studied the response mechanism of two Prunus rootstocks [Myrobalan plum (P 2175) and peach–almond hybrid (Felinem)]. Felinem showed an activated expression of FCR and the iron transporter gene. The local signal induced a quick response of the transporter, whereas the FCR gene was expressed later. By contrast, in P 2175, the response appeared later, and long-distance signals appeared to be involved. However, in both situations, signaling molecules were not identified; this mechanism thus requires further investigation.
Grape rootstocks [SO4 and K5BB (VITIS Rauscedo, Società Cooperativa Agricola, Rauscedo, Italy) favor nitrate uptake by affecting the activities of low-affinity nitrate transporter (VvNRT1.3like) and high-affinity nitrate transporter (VvNRT2.4like) genes in grapevines (Tomasi et al., 2015). In pear rootstock (Pyrus betulaefolia), six transporter genes (LjAMT;1, LjAMT;2, LjAMT;3, LeAMT;3, PbAMT1;3, and PbAMT1;5) have been reported (Mota et al., 2008; Li et al., 2016d); however, the roles of these and other transporters in grafted plants remain unclear. In two citrus rootstocks [Cleopatra mandarin (C. reshni hort. ex Tanaka) and Troyer citrange (C sinensis × P trifoliata)], low-and high-affinity transport systems work depending on the availability of nitrate ions in the external medium (Cerezo et al., 2007). However, the sensing and signaling mechanisms (how plants sense the internal and external concentrations of nitrate and respond accordingly) have not been explained in grafted plants. Non-grafted plants utilize a dual-affinity nitrate transporter (CHL1) and protein kinases (CIPK28 and CIPK8) to sense a wide range of nitrate concentration changes in the soil and to alter their own transport properties (Ho et al., 2009). Another recent study revealed that small peptides are produced in nitrogen-starved roots and then transported to the shoot; this root-to-shoot signaling helps plants adopt under prevailing conditions (Tabata et al., 2014). These pieces of evidence are missing in grafted plants and need further study.
Hormones and miRNAs are the key players that affect nutrient absorption and transport by affecting the root growth and activity of ion transporters. Grafting onto rootstocks changes hormonal levels (cytokinin and auxin) in roots and shoots, triggers growth, delays leaf senescence, and helps tolerate environmental stress (Albacete et al., 2009; Van-Hooijdonk et al., 2010; Ghanem et al., 2011). In a previous study, grafting a transient root IPT induction (HSP70::IPT) and wild type (WT) tomato (S. lycopersicum L. cv. UC82-B) scions onto root localized IPT-expressing tomato rootstock (35S::IPT) grown under salt stress conditions (75, 100 mM NaCl) increased root, xylem sap, and leaf bioactive cytokinin by 2–3 times, increased leaf K concentration (20%), and reduced Na concentration (30%) in the transient root IPT induction scion compared with plants grafted onto non-transformed plants. Similarly, IPT-expressing tomato rootstock (35S::IPT) increased fruit trans-zeatin concentrations 1.5–2 fold and fruit yield (30%) in WT tomato scion compared with WT tomato plants grafted onto non-transformed rootstock (Ghanem et al., 2011). Roots of rootstock-grafted apple with more branching (MB) mutant scion show elevated cytokinin and auxin contents and reduced expressions of MrPIN1, MrARF, MrAHP, most MrCRE1 genes, and cell growth-related genes MrGH3, MrSAUR, and MrTCH4. Auxin accumulation and transcription of MrPIN3, MrALF1, and MrALF4 induce lateral root formation in MB-grafted rootstock, and these new roots contribute to nutrient absorption (Li et al., 2016a). miRNAs regulate the expression of transporters involved in nutrient uptake and mobilization (Fujii et al., 2005; Chiou et al., 2006; Sunkar et al., 2007; Valdés-Lopez et al., 2010; Paul et al., 2015). A recent report (Li et al., 2016c) showed that under N or P deficiency, the expression levels of 19 miRNA target genes in the roots of pumpkin (C. moschata Duch.)-grafted cucumber (C. sativus L.) are higher than those in self-grafted cucumber. Many studies have revealed the role of miRNA 399 in P absorption, transport, metabolism, and homeostasis in plants. P deprivation is rapidly transmitted to the shoots where miRNA 399 is produced, conjugates, changes the activity of PHO2 (an essential transporter of phosphate mobilization), and regulates phosphate homeostasis (Pant et al., 2008). The expressions of miRNA 399 and csa-miR-n08 are higher in pumpkin (Cucurbita moschata Duch.)-grafted plants. These affect the expressions of PHR1 and E3 (ubiquitin–protein ligase), which are essential for P absorption and metabolism. The PHR1 transcription factor binds to the promoter region of many phosphorous-deficient responsive genes, including those encoding phosphate transporters and protein kinases (Rubio et al., 2001; Todd et al., 2004). Under phosphorous starvation, the activity of miR2111 doubles within 3 h, whereas under N deficiency, the opposite occurs (Pant et al., 2008; Xu et al., 2013). Therefore, the relationship among the expression levels of different miRNAs still requires explanation. Several recently published articles have summarized the role of miRNAs (Liu and Vance, 2010; Zeng et al., 2014; Kulcheski et al., 2015; Paul et al., 2015) and ion transporters (Conte and Walker, 2011; Finazzi et al., 2015; Pinto and Ferreira, 2015; Gu et al., 2016) in nutrient acquisition, transport, and homeostasis. However, information on grafted plants is limited and requires the attention of plant biologists because grafted plants are complex in nature, and their responses are influenced by the genetic makeup of the scion and the rootstock and their interaction.
In conclusion, rootstocks modify nutrient availability to the scion. A vigorous root system, increased secretion of root exudates (organic acids and primary and secondary metabolites), enhanced expression of transport-related genes, increased absorption and transport, improved ion homeostasis, and remobilization capacity of the rootstocks ensure improved nutrient supply to the scion. These nutrients enable scions to increase light energy transformation, CO2 conductivity, dark reaction activity, and rate of photosynthesis, thereby improving nutrient utilization (Weng, 2000; Sun et al., 2002; Qi et al., 2006; Wei et al., 2006; Huang et al., 2011).
Rootstocks improve the acquisition of essential elements and reduce the uptake and transport of salts (e.g., Na and Cl) and heavy metals (e.g., Cr, Ni, Cd, Sr, and Ti) through ion exclusion or retention. Reciprocal grafting experiments on cucumber (C. sativus L.), pumpkin (C. moschata Duch.), and melon (C. melo L.) rootstocks revealed that pumpkin is salt tolerant. Pumpkin excludes 74% of the available Na, whereas no Na is excluded by melon. Similarly, Na retention in pumpkin rootstock reduces the level of Na in leaves by 46.9%, whereas no decrease is observed in melon rootstock (Edelstein et al., 2011). Estañ et al. (2005) found that grafting a commercial tomato cultivar Jaguar (S. lycopersicum L.) onto tomato rootstocks (Radja, Pera, and the hybrid Volgogradskij × Pera) increases fruit yield by up to 80% by regulating saline ions (Na and Cl). Pumpkin-grafted cucumber shows an increased expression of plasma membrane H+-ATPases (PMA) and plasma membrane Na+/H+ antiporter (SOS1) under NaCl stress conditions compared with self-grafted cucumber. The increased activities of PMA and SOS1 enable the root to pump Na+ from the cytosol of root cells to the external medium (Lei et al., 2014). Citrus rootstock responds differently to Cl stress, and a number of uncharacterized membrane transporter genes, such as NRT1-2, are differentially expressed in poor Cl excluder Carrizo citrange (C. sinensis × P. trifoliata) and efficient Cl excluder Cleopatra mandarin (C. reshni hort. ex Tanaka) rootstocks (Brumós et al., 2009). The strong repression of the IClngene in Cleopatra mandarin regulates Cl uptake and transport to the shoot. It reduces the net Cl loading to the root xylem and thus enhances the Cl tolerance of Cleopatra mandarin (C. reshni hort. ex Tanaka) (Brumós et al., 2010). Transporters also help enhance salt stress tolerance. Overexpression of a Malus Na+/H+ anti-porter gene improves the salt tolerance of M26 dwarfed apple rootstock (Li et al., 2013). Rootstocks reduce Na and Cl loading and transport to the scion while increasing the uptake of K, Ca, and Mg ions and allow small osmotic potentials with a low energy cost. Therefore, rootstocks increase the tolerance of scion species to salt and toxic elements.
Grafting cucumber (C. sativus L.) onto C. maxima × C. moschata rootstocks reduces the absorption and transport of Cd and Ni to the scion. The absorption of Cd depends on rootstock genotype (Savvas et al., 2013). Heavy metals also affect the availability of essential nutrients; however, the negative effect can be minimized by grafting onto appropriate rootstocks. Root structure (genotype) and transporter activities appear to be involved in the reduction of hazardous ion absorption and transport. In a reciprocal grafting experiment, Xin et al. (2013) found that spinach shoot Cd concentration is unrelated to root Cd absorption; it depends on Cd transport from the root to the shoot. The thick phellem (outer zone of periderm) and outer cortex cell walls of the water spinach (Ipomoea aquatic Forsk.) rootstock QLQ retain more Cd in the root compared with water spinach rootstock T308. Apple rootstock (Malus Baccata Borkh.) reduces the influx of Cd and shows the least amount of Cd in leaf petioles compared with other apple rootstocks (Malus hupehensis Rehd., M. micromalus “qingzhoulinqin,” and M. robusta Rehd.). M. Baccata has a low transcript level for Cd uptake and transport genes (HA7, FRO2-like, NRAMP1, and NRAMP3) and a strong transcript level for detoxification genes (NAS1 and MT2). The reduced expression of Cd transport genes results in reduced uptake and transport of Cd (Zhou et al., 2016). Repression of Cd uptake and transport genes occurs through a local signal or as a result of long-distance signaling, although this issue remains unclear. Thus, investigations about the sensing and signaling mechanisms of heavy metals in grafted plants are required.
Although grafting is a centuries-old phenomenon currently practiced at the commercial scale, the physiological and molecular bases of grafting remain unclear. With Arabidopsis as a model plant, the basic scientific mechanism of grafting is being investigated. Rootstocks and scions affect each other; their interaction mechanism also requires further attention. Grafting compatibility between the rootstock and scion is important for uninterrupted flow of water, nutrients, and carbohydrates. Grafting incompatibility occurs when either of the plant parts (rootstock or scion) completely or partially fails to grow after successful grafting and in several latter stages of plant growth. Overgrowth of the scion over the rootstock, overgrowth of the rootstock over the scion, and bud union crease are common symptoms of incompatibility. Double grafting can resolve compatibility issues, but it complicates the process of grafted seedling production and increases the production cost. Grafting incompatibility may be related to defense responses. Cookson et al. (2014) observed a coordinated upregulation of stress response-related genes in hetero-grafts compared with self-grafted grapevines (Vitis spp.). In a recent study, Melnyk et al. (2015) revealed that auxin response genes IAA18, IAA28, and ALF4 are responsible for vascular reconnection at the graft junction. Although authors have not linked these genes with incompatibility, the roles of these genes in incompatibility cannot be overlooked and require further investigation. Crease formation affects water and nutrient transport; however, to what extent this effect impairs the availability of nutrients to the scion remains unclear. Similarly, different methods of grafting are utilized for fruits and vegetables, but their effects on the quality of the vascular connection, crease development, and nutrient supply have not been considered to date. Detailed histochemical investigations are required to study the cell and vascular tissue structures at graft junctions and their relationship with water and nutrient transport. The rootstock and scion are important, but the rootstock is considered critical. No failure caused by scion variety has been reported in the fruit industry in any country, but a number of examples show failure caused by inappropriate rootstock (Castle, 2010). Therefore, in perennial fruit plants, the choice of proper/compatible rootstock is highly significant because once an orchard is established; it remains productive for a long time. Similarly, selecting compatible and appropriate rootstocks for important vegetables and ornamental crops need consideration. Although large numbers of rootstocks are available and used for different crops, improved rootstocks with improved characteristics, such as multi-disease resistance and enhanced nutrient absorption and utilization efficiencies, are still lacking.
Rootstocks enable increased water and nutrient absorption through efficient uptake systems and/or their capacity to explore wide and deep soil volumes. However, these properties should be transferred to increase yield or improve efficiency. This transfer requires well-designed studies on yield responses vs. a gradient of nutrient/water application to the plant. The accumulation of a given nutrient in the leaves during vegetative growth is not an indicator of nutrient use efficiency and should be considered in relation to the total nutrients absorbed by the plant and the harvestable yield produced (Pérez-Alfocea, 2015). Moreover, the response of rootstocks under different growing conditions changes considerably (Cong et al., 2014). Rootstocks are also inherently selective in the absorption of different nutrients. Fernández-García et al. (2002) observed an increase in the uptake of P and Ca and a decrease in the uptake of N, K, S, and Mg by grafting Fanny and Goldmar (S. lycopersicum L.) onto AR-9704 (S. lycopersicum L.). Nutrient-specific rootstock breeding is currently eliciting the attention of scientists (Venema et al., 2011). Macronutrients, such as P and K, are finite in nature and costly, so they are of particular interest. With the passage of time, linkage map locations for quantitative trait loci (QTLs) have become available for nutrient uptake and transport. For example, several QTLs controlling the concentration of nutrients in the leaves of tomato and apple rootstocks have been found (Fazio et al., 2013; Asins et al., 2015). These QTLs allow scientists to alter the root architecture of plants as needed. Several transgenic rootstocks have been reported to control fan leaf virus in grapes (Gambino et al., 2010), fungal diseases in citrus (Mitani et al., 2006), pathogen damage in tomato (Haroldsen et al., 2012), and nutrient absorption in tomato and watermelon (Han et al., 2009; Ghanem et al., 2011). Thus, nutrient-efficient transgenic rootstocks are expected to become commercially available for different horticultural crops in the near future. The use of genetically engineered nutrient-efficient rootstocks (transgrafting) and scions will extend the utility of grafting by combining an ancient technique with molecular strategies of the modern era (Goldschmidt, 2014), leading to improved nutrient use efficiency. However, the acceptability of genetically modified crops by the public is a question that needs to be addressed.
Remarkable achievements have been made in the field of ion transporter in Arabidopsis, rice, wheat, and other crops. However, a limited number of studies related to the uptake and transport of essential nutrients have been performed on grafted plants. Thus, further study is needed to thoroughly understand the sensing, signaling, uptake, and transport mechanisms in grafted plants. This information will be helpful for breeding programs to develop nutrient-efficient rootstocks. Grafting in horticultural crops can also help understand the basic biology of the grafting mechanism, the reasons for incompatibility, sensing and signaling of nutrients, ion uptake and transport, the mechanism involved in the accumulation of heavy metals in the rootstock, and the restriction of heavy metal transport to the scions. Moreover, vegetable grafting studies can be performed quickly, and the results can be compared with those on self-rooted or self-grafted plants. Imperative conclusions can be obtained for commercial adoption.
Several approaches, such as the use of plant-growth-promoting rhizobacteria (PGPR), can be combined with grafting to further enhance nutrient uptake and utilization efficiency. These PGPR act in diverse ways, including the production or degradation of important plant growth hormones, which in turn controls root growth and affects nutrient absorption (Dodd et al., 2010; Wang et al., 2016b). The use of arbuscular mycorrhizal fungi enhances the acquisition of P, N, Mg, and Ca, maintains the K:Na ratio, affects nodulation and nitrogen fixation, and alters gene expression (PIP, Na/H antiporters, Lsnced, Lslea, and LsP5CS), thereby improving plant growth (Parniske, 2008; Evelin et al., 2009; Kumar et al., 2015; Miceli et al., 2016; Wang et al., 2016b). Single- and double-root grafting of cucumber increase the population of soil actinomycetes (bacteria) and reduce the population of fungi (Fusarium oxysporum) (Xie et al., 2012). However, for other crops, whether the rootstock and scion affect each other's soil microbiota and their effect on the nutrient absorption of rootstocks remain largely unknown and thus need further study. The use of natural (humic acid, root exudates, phytosiderophores) and synthetic chelators [ethylenediaminetetraacetic acid (EDTA), [(ethylenediamine-N, N'-bis (2-hydroxyphenylacetic acid) (EDDHA)], diethylenetriamine pentaacetic acid (DTPA)] is reported to enhance nutrient (Fe, Zn, Cu, Mn, etc.) mobilization and phyto-availability; however, the suitability and efficacy of these chelators in grafted plants need investigation (Treeby et al., 1989; Bocanegra et al., 2006). Exogenous application of plant growth regulators (e.g., auxin) and several other novel substances (e.g., melatonin) is currently being considered by scientists to alter root architecture and enhance nutrient uptake (Nawaz et al., 2016). However, further studies are required to standardize the concentration and method of application of these substances for grafted plants.
MN, MI, QK, and FC wrote the manuscript, YH, WA, and ZB revised, and finally approved the manuscript for submission. All authors declare no competing financial interests.
The authors declare that the research was conducted in the absence of any commercial or financial relationships that could be construed as a potential conflict of interest.
We are thankful to Prof. Sergey Shabala from University of Tasmania, Australia for providing comments and helping in revision of this manuscript. This work was supported by National Natural Science Foundation of China (31201660, 31471919, and 31410303012), The International Science and Technology Cooperation Program of China (2015DFG32310), China Agriculture Research System (CARS-26-16), and The Fundamental Research Funds for the Central Universities (2013PY086).
Ahmed, W., Nawaz, M. A., Iqbal, M. A., and Khan, M. M. (2007). Effect of different rootstocks on plant nutrient Status and yield in Kinnow mandarin (Citrus reticulata Blanco). Pak. J. Bot. 39, 1779–1786. Available online at: http://www.pakbs.org/pjbot/PDFs/39(5)/PJB39(5)1779.pdf
Ahmed, W., Pervez, M. A., Amjad, M., Khalid, M., Ayyub, C. M., and Nawaz, M. A. (2006). Effect of stionic combination on the growth and yield of Kinnow mandarin (Citrus reticulata Blanco.). Pak. J. Bot. 38, 603–612. Available online at: http://www.pakbs.org/pjbot/PDFs/38(3)/PJB38(3)603.pdf
Albacete, A., Martínez-Andújar, C., Ghanem, M. E., Acosta, M., Sánchez-Bravo, J., Asins, M. J., et al. (2009). Rootstock-mediated changes in xylem ionic and hormonal status are correlated with delayed leaf senescence and increased leaf area and crop productivity in salinized tomato. Plant Cell Environ. 32, 928–938. doi: 10.1111/j.1365-3040.2009.01973.x
Albacete, A., Martínez-Andújar, C., Pérez-Alfocea, F., Ruiz-Lozano, J. M., and Asins, M. J. (2015b). “Rootstock-mediated variation in tomato vegetative growth under low potassium or phosphorous supplies,” in Proceeding of the First International Symposium on Vegetable Grafting. Acta Horticulturae, Vol. 1086, eds Z. Bie Y. Huang, and M. A. Nawaz (Wuhan), 147–152.
Albacete, A., Martinez-Anujar, C., Marinez-Pérez, A., Thompson, A. J., Dodd, I. C., and Pérez-Alfocea, F. (2015a). Unravelling rootstock×scion interactions to improve food Security. J. Exp. Bot. 66, 2211–2226. doi: 10.1093/jxb/erv027
Albrigo, L. G. (1977). Rootstocks affect ‘Valencia’ orange fruit quality and water balance. Proc. Int. Soc. Citriculture 1, 62–65.
Aloni, B., Cohen, R., Karni, L., Aktas, H., and Edelstein, M. (2010). Hormonal signaling in rootstock–scion interactions. Sci. Hortic. 127, 119–126. doi: 10.1016/j.scienta.2010.09.003
Amiri, M. E., Fallahi, E., and Safi-Songhorabad, M. (2014). Influence of rootstock on mineral uptake and scion growth of ‘golden delicious’ and ‘Royal Gala’ apples. J. Plant Nutr. 37, 16–29. doi: 10.1080/01904167.2013.792838
Arao, T., Takeda, H., and Nishihara, E. (2008). Reduction of cadmium translocation from roots to shoots in eggplant (Solanum melongena) by grafting onto Solanum torvum rootstock. Soil Sci. Plant Nutr. 54, 555–559. doi: 10.1111/j.1747-0765.2008.00269.x
Arwiyanto, T., Lwin, K., Maryudani, Y., and Purwantoro, A. (2015). “Evaluation of local Solanum torvum as a rootstock to control of Ralstonia solanacearum in Indonesia,” in Proceeding of the First International Symposium on Vegetable Grafting. Acta Horticulturae, Vol. 1086, eds Z. Bie Y. Huang, and M. A. Nawaz (Wuhan), 101–106.
Asins, M. J., Raga, V., Roca, D., Belver, A., and Carbonell, E. A. (2015). Genetic dissection of tomato rootstock effects on scion traits under moderate salinity. Theor. Appl. Genet. 128, 667–679. doi: 10.1007/s00122-015-2462-8
Bautista, A. S., Calatayud, A., Nebauer, S. G., Pascual, B., Maroto, J. V., and Lopez-Galarza, S. (2011). Effects of simple and double grafting melon plants on mineral absorption, photosynthesis, biomass and yield. Sci. Hortic. 130, 575–580. doi: 10.1016/j.scienta.2011.08.009
Bavaresco, L., Giachino, E., and Pezzutto, S. (2003). Grapevine rootstock effects on lime-induced chlorosis, nutrient uptake, and source–sink relationships. J. Plant Nutr. 26, 1451–1465. doi: 10.1081/PLN-120021054
Bhatt, R. M., Upreti, K. K., Divya, M. H., Bhat, S., Pavithra, C. B., and Sadashiva, A. T. (2015). Interspecific grafting to enhance physiological resilience to flooding stress in tomato (Solanum lycopersicum L.). Sci. Hortic. 182, 8–17. doi: 10.1016/j.scienta.2014.10.043
Bocanegra, M. P., Lobartini, J. C., and Orioli, G. A. (2006). Plant uptake of iron chelated by humic acids of different molecular weights. Commun. Soil Sci. Plant Anal. 37, 239–248. doi: 10.1080/00103620500408779
Borgognone, D., Colla, G., Rouphael, Y., Cardarelli, M., Reac, E., and Schwarzd, D. (2013). Effect of nitrogen form and nutrient solution pH on growth and mineral composition of self-grafted and grafted tomatoes. Sci. Hortic. 149, 61–69. doi: 10.1016/j.scienta.2012.02.012
Brumós, J., Colmenero-Flores, J. M., Conesa, A., Izquierdo, P., Sánchez, G., Iglesias, D. J., et al. (2009). Membrane transporters and carbon metabolism implicated in chloride homeostasis differentiate salt stress responses in tolerant and sensitive Citrus rootstocks. Funct. Integr. Genomics 9, 293–309. doi: 10.1007/s10142-008-0107-6
Brumós, J., Talón, M., Bouhlal, R., and Colmenero-Flores, J. M. (2010). Cl- homeostasis in includer and excluder citrus rootstocks: san transport mechanisms and identification of candidate genes. Plant Cell Environ. 33, 2012–2027. doi: 10.1111/j.1365-3040.2010.02202.x
Caballero, F., García-Sánchez, F., Gimeno, V., Syvertsen, J. P., Martínez, V., and Rubio, F. (2013). High-affinity potassium uptake in seedlings of two citrus rootstocks Carrizo citrange (Citrus sinensis [L.] Osb. × Poncirus trifoliata [L.] Raf.) and Cleopatra mandarin (Citrus reshni Hort. ex Tanaka). Aust. J. Crop Sci. 7, 538–542. Available online at: http://www.crec.ifas.ufl.edu/academics/faculty/syvertsen/PDF/2013_Caballero_et_al.pdf
Cabrera, R. I. (2002). Rose yield, dry matter partitioning and nutrient status responses to rootstock selection. Sci. Hortic. 95, 75–83. doi: 10.1016/S0304-4238(02)00020-1
Cantero-Navarro, E., Romero-Aranda, R., Fernández-Muñoz, R., Martínez-Andújar, C., Pérez Alfocea, F., and Albacete, A. (2016). Improving agronomic water use efficiency in tomato byrootstock-mediated hormonal regulation of leaf biomass. Plant Sci. 251, 90–100. doi: 10.1016/j.plantsci.2016.03.001
Castle, W. S. (2010). A career perspective on citrus rootstocks, their development, and commercialization. HortScience 45, 11–15. Available online at: http://hortsci.ashspublications.org/content/45/1/11.full.pdf+html
Cerezo, M., Camañes, G., Flors, V., Primo-Millo, E., and García-Agustín, P. (2007). Regulation of nitrate transport in citrus rootstocks depending of nitrogen availability. Plant Signal. Behav. 2, 337–342. doi: 10.4161/psb.2.5.4578
Chiou, T. J., Aung, K., Lin, S. I., Wu, C. C., Chiang, S. F., and Su, C. L. (2006). Regulation of phosphate homeostasis by microRNA in Arabidopsis. Plant Cell 18, 412–21. doi: 10.1105/tpc.105.038943
Colla, G., Rouphael, Y., Jawad, R., Kumar, P., Rea, E., and Cardarelli, M. (2013). The effectiveness of grafting to improve NaCl and CaCl2 tolerance in cucumber. Sci. Hortic. 164, 380–391. doi: 10.1016/j.scienta.2013.09.023
Colla, G., Rouphael, Y., Rea, E., and Cardarelli, M. (2012). Grafting cucumber plants enhance tolerance to sodium chloride and sulfate salinization. Sci. Hortic. 135, 177–185. doi: 10.1016/j.scienta.2011.11.023
Cong, C., Chao, L., Cui-ying, L., Xiao-yu, K., Yang-jun, Z., and Feng-wang, M. (2014). Differences in the efficiency of potassium (K) uptake and use in five apple rootstock genotypes. J. Integr. Agric. 13, 1934–1942. doi: 10.1016/S2095-3119(14)60839-X
Conte, S. S., and Walker, E. L. (2011). Transporters contributing to iron trafficking in plants. Mol. Plant. 4, 464–476. doi: 10.1093/mp/ssr015
Cookson, S. J., Moreno, M. J. C., Hevin, C., Mendome, L. Z. N., Delrot, S., Magnin, N., et al. (2014). Heterografting with nonself rootstocks induces genes involved in stress responses at the graft interface when compared with autografted controls. J. Exp. Bot. 65, 2473–2481. doi: 10.1093/jxb/eru145
Covarrubias, J. I., Retamales, C., Donnini, S., Rombolà, A. D., and Pastenes, C. (2016). Contrasting physiological responses to iron deficiency in Cabernet Sauvignon grapevines grafted on two rootstocks. Sci. Hortic. 199, 1–8. doi: 10.1016/j.scienta.2015.12.013
Dam, N. M. V., and Bouwmeester, H. J. (2016). Metabolomics in the rhizosphere: tapping into belowground chemical communication. Trends Plant Sci. 21, 256–265. doi: 10.1016/j.tplants.2016.01.008
Davis, A. R., Perkins-Veazie, P., Sakata, Y., López-Galarza, S., Maroto, J. V., Lee, S. G., et al. (2008). Cucurbit grafting. Crit. Rev. Plant Sci. 27, 50–74. doi: 10.1080/07352680802053940
Dodd, I. C., Zinovkina, N. Y., Safronova, V. I., and Belimov, A. A. (2010). Rhizobacterial mediation of plant hormone status. Ann. Appl. Biol. 157, 361–379. doi: 10.1111/j.1744-7348.2010.00439.x
Dor, E., Alperin, B., Wininger, S., Ben-Dor, B., Somvanshi, V. S., Koltai, H., et al. (2010). Characterization of a novel tomato mutant resistant to the weedy parasites Orobanche and Phelipanche spp. Euphytica 171, 371–380. doi: 10.1007/s10681-009-0041-2
Edelstein, M., Ben-Hur, M., Cohen, R., Burger, Y., and Ravina, I. (2005). Boron and salinity effects on grafted and non-grafted melon plants. Plant Soil 269, 273–284. doi: 10.1007/s11104-004-0598-4
Edelstein, M., Cohen, R., Baumkoler, F., and Ben-Hur, M. (2016). Using grafted vegetables to increase tolerance to salt and toxic elements. Israel J. Plant Sci. 2016, 1–18. doi: 10.1080/07929978.2016.1151285
Edelstein, M., Plaut, Z., and Ben-Hur, M. (2011). Sodium and chloride exclusion and retention by non-grafted and grafted melon and Cucurbita plants. J. Exp. Bot. 62, 177–184. doi: 10.1093/jxb/erq255
Esmaeili, M., Salehi, R., Taheri, M. R., Babalar, M., and Mohammadi, H. (2015). “Effect of different nitrogen rates on fruit yield and quality of grafted and non-grafted muskmelon,” in Proceeding of the First International Symposium on Vegetable Grafting. Acta Horticulturae, Vol. 1086, eds Z. Bie Y. Huang, and M. A. Nawaz (Wuhan), 255–260.
Estañ, M. T., Martinez-Rodriguez, M. M., Pérez-Alfocea, F., Flowers, T. J., and Bolarin, M. C. (2005). Grafting raises the salt tolerance of tomato through limiting the concentration of sodium and chloride to the shoot. J. Exp. Bot. 56, 703–712. doi: 10.1093/jxb/eri027
Evelin, H., Kapoor, R., and Giri, B. (2009). Arbuscularmycorrhizal fungi in alleviation of salt stress: a review. Ann. Bot. 104, 1263–1280. doi: 10.1093/aob/mcp251
Farhadi, A., and Malek, S. (2015). “Evaluation of graft compatibility and organoleptic traits of greenhouse cucumber seedlings grafted on different rootstocks,” in Proceeding of the First International Symposium on Vegetable Grafting. Acta Horticulturae, Vol. 1086, eds Z. Bie Y. Huang, and M. A. Nawaz (Wuhan), 219–224. Available online at: https://www.cabdirect.org/cabdirect/abstract/20143073666
Fazio, G., Kviklys, D., Grusak, M. A., and Robinson, T. L. (2013). Phenotypic diversity and QTL mapping of absorption and translocation of nutrients by apple rootstocks. Aspects Appl. Biol. 119, 37–50.
Fernández-García, N., Martínez, V., Cerdá, A., and Carvajal, M. (2002). Water and nutrient uptake of grafted tomato plants grown under saline Conditions. J. Plant Physiol. 159, 899–905. doi: 10.1078/0176-1617-00652
Finazzi, G., Petroutsos, D., Tomizioli, M., Flori, S., Sautron, E., Villanova, V., et al. (2015). Ions channels/transporters and chloroplast regulation. Cell Calcium 58, 86–97. doi: 10.1016/j.ceca.2014.10.002
Fuentes, I., Stegemann, S., Golczyk, H., Karcher, D., and Bock, R. (2014). Horizontal genome transfer as an asexual path to the formation of new species. Nature 511, 232–235. doi: 10.1038/nature13291
Fujii, H., Chiou, T. J., Lin, S. I., Aung, K., and Zhu, J. K. (2005). A miRNA involved in phosphate starvation responses in Arabidopsis. Curr. Biol. 15, 2038–2043. doi: 10.1016/j.cub.2005.10.016
Gambino, G., Perrone, I., Carra, A., Chitarra, W., Boccacci, P., Marinoni, D. T., et al. (2010). Transgene silencing in grapevines transformed with GFLV resistance genes: analysis of variable expression of transgene, siRNAs production and cytosine methylation. Transgenic Res. 19, 17–27. doi: 10.1007/s11248-009-9289-5
Gammon, N. Jr., and McFadden, S. E. Jr. (1979). Effect of rootstocks on greenhouse rose flower yield and leaf nutrient levels. Comm. Soil Sci. Plant Anal. 10, 1171–1184. doi: 10.1080/00103627909366970
Gao, Y., Tian, Y., Liang, X., and Gao, L. (2015). Effects of single-root-grafting, double-root grafting and compost application on microbial properties of rhizosphere soils in Chinese protected cucumber (Cucumis sativus L.) production systems. Sci. Hortic. 186, 190–200. doi: 10.1016/j.scienta.2015.02.026
Gerendás, J., Abbadi, J., and Sattelmacher, B. (2008). Potassium efficiency of safflower (Carthamus tinctorius L.) and sunflower (Helianthus annuus L.). J. Plant Nutr. Soil Sci. 171, 431–439. doi: 10.1002/jpln.200720218
Ghanem, M. E., Albacete, A., Smigocki, A. C., Frebort, I., Pospisilova, H., Martinez-Andujar, C., et al. (2011). Root-synthesised cytokinins improve shoot growth and fruit yield in salinised tomato (Solanum lycopersicum L.). J. Exp. Bot. 62, 125–140. doi: 10.1093/jxb/erq266
Goldschmidt, E. E. (2014). Plant grafting: new mechanisms, evolutionary implications. Front. Plant Sci. 5:727. doi: 10.3389/fpls.2014.00727
Gonzalo, M. J., Moreno, M. A., and Gogorcena, Y. (2011). Physiological responses and differential gene expression in Prunus rootstocks under iron deficiency conditions. J. Plant Physiol. 168, 887–893. doi: 10.1016/j.jplph.2010.11.017
Gregory, P. J., Atkinson, C. J., Bengough, A. G., Else, M. A., Fernandez-Fernandez,. F., Harrison, R. J., et al. (2013). Contributions of roots and rootstocks to sustainable, intensified crop production. J. Exp. Bot. 64, 1209–1222. doi: 10.1093/jxb/ers385
Gu, M., Chen, A., Sun, S., and Xu, G. (2016). Complex regulation of plant phosphate transporters and the gap between molecular mechanisms and practical application: what is missing? Mol. Plant. 9, 396–416. doi: 10.1016/j.molp.2015.12.012
Habran, A., Commisso, M., Helwi, P., Hilbert, G., Negri, S., Ollat, N., et al. (2016). Roostocks/scion/nitrogen Interactions affect secondary metabolism in the grape berry. Front. Plant Sci. 7:1134. doi: 10.3389/fpls.2016.01134
Han, J. S., Park, S., Shigaki, T., Hirschi, K. D., and Kim, C. K. (2009). Improved watermelon quality using bottle gourd rootstock expressing a Ca2+/H+ antiporter. Mol. Breeding 24, 201–211. doi: 10.1007/s11032-009-9284-9
Haroldsen, V. M., Chi-Ham, C. L., and Bennett, A. B. (2012). Transgene mobilization and regulatory uncertainty for non-GE fruit products of transgenic rootstocks. J. Biotechnol. 161, 349–353. doi: 10.1016/j.jbiotec.2012.06.017
Harp, D. A., Kay, K., Zlesak, C. D., and George, S. (2015). The effect of rose root size on drought stress tolerance and landscape plant performance. Texas J. Agric. Nat. Resour. 28, 82–88. Available online at: http://txjanr.agintexas.org/index.php/txjanr/article/view/13/8
Ho, C. H., Lin, S. H., Hu, H. C., and Tsay, Y. F. (2009). CHL1 functions as a nitrate sensor in plants. Cell 138, 1184–1194. doi: 10.1016/j.cell.2009.07.004
Hrotko, K., Magyar, L., Borsos, G., and Gyeviki, M. (2014). Rootstock effect on nutrient concentration of sweet cherry leaves. J. Plant Nutr. 37, 395–1409. doi: 10.1080/01904167.2014.911317
Huang, Y., Bie, Z. L., Liu, Z. X., Zhen, A., and Jiao, X. R. (2011). Improving cucumber photosynthetic capacity under NaCl stress by grafting onto two salt-tolerant pumpkin rootstocks. Biol. Plant. 55, 285–290. doi: 10.1007/s10535-011-0040-8
Huang, Y., Bie, Z., Liu, P., Niu, M., Zhen, A., Liu, Z., et al. (2013a). Reciprocal grafting between cucumber and pumpkin demonstrates the roles of the rootstock in the determination of cucumber salt tolerance and sodium accumulation. Sci. Hortic. 149, 47–54. doi: 10.1016/j.scienta.2012.04.018
Huang, Y., Jiao, Y., Nawaz, M. A., Chen, C., Liu, L., Lu, Z., et al. (2016b). Improving magnesium uptake, photosynthesis and antioxidant enzyme activities of watermelon by grafting onto pumpkin rootstock under low magnesium. Plant Soil 1–18. doi: 10.1007/s11104-016-2965-3. Available online at: http://link.springer.com/article/10.1007/s11104-016-2965-3/fulltext.html
Huang, Y., Li, J., Hua, B., Liu, Z., Fan, M., and Bie, Z. (2013b). Grafting onto different rootstocks as a means to improve watermelon tolerance to low potassium stress. Sci. Hortic. 149, 80–85. doi: 10.1016/j.scienta.2012.02.009
Huang, Y., Tang, R., Cao, Q., and Bie, Z. (2009). Improving the fruit yield and quality of cucumber by grafting onto the salt tolerant rootstock under NaCl stress. Sci. Hortic. 122, 26–31. doi: 10.1016/j.scienta.2009.04.004
Huang, Y., Zhao, L. Q., Kong, Q. S., Cheng, F., Niu, M. L., Xie, J. J., et al. (2016a). Comprehensive mineral nutrition analysis of watermelon grafted onto two different rootstocks. Hortic. Plant J. 2, 105–113. doi: 10.1016/j.hpj.2016.06.003
Ibacache, G. A., and Sierra, B. C. (2009). Influence of rootstocks on nitrogen, phosphorus and potassium content in petioles of four table grape varieties. Chilean J. Agric. Res. 69, 503–508. doi: 10.4067/S0718-58392009000400004
Jaitz, L., Mueller, B., Koellensperger, G., Huber, D., Oburger, E., Puschenreiter, M., et al. (2011). LC-MS analysis of low molecular weight organic acids derived from root exudation. Anal. Bioanal. Chem. 400, 2587–2596. doi: 10.1007/s00216-010-4090-0
Jiménez, S., Ollat, N., Deborde, C., Maucourt, M., Rellan-Alvarez, R., Moreno, M. A., et al. (2011). Metabolic response in roots of Prunus rootstocks submitted to iron chlorosis. J. Plant Physiol. 168, 415–423. doi: 10.1016/j.jplph.2010.08.010
Khorassani, R., Hettwer, U., Ratzinger, A., Steingrobe, B., Karlovsky, P., and Claassen, N. (2011). Citramalic acid and salicylic acid in sugar beet root exudates solubilize soil phosphorus. BMC Plant Biol. 11, 121. doi: 10.1186/1471-2229-11-121
Kubota, C., and McClure, M. A. (2008). Vegetable grafting: history, use, and current technology status in North America. HortScience 43, 1664–1669. Available online at: http://hortsci.ashspublications.org/content/43/6/1664.full.pdf+html
Kulcheski, F. R., Côrrea, R., Gomes, I. G., Lima, J. C., and Margis, R. (2015). NPK macronutrients and microRNA homeostasis. Front. Plant Sci. 6:451. doi: 10.3389/fpls.2015.00451
Kumar, P., Lucini, L., Rouphael, Y., Cardarelli, M., Kalunke, R. M., and Colla,. G. (2015). Insight into the role of grafting and arbuscular mycorrhiza on cadmium stress tolerance in tomato. Front. Plant Sci. 6:477. doi: 10.3389/fpls.2015.00477
Lecourt, J., Lauvergeat, V., Ollat, N., Vivin, P., and Cookson, S. J. (2015). Shoot and root ionome responses to nitrate supply in grafted grapevines are rootstock genotype dependent. Aust. J. Grape Wine Res. 21, 311–318. doi: 10.1111/ajgw.12136
Lee, J. M. (1994). Cultivation of grafted vegetables I. Current status, grafting methods, and benefits. HortScience 29, 235–239.
Lee, J. M., and Oda, M. (2003). “Grafting of herbaceous vegetable and ornamental crops,” in: Horticultural Reviews, Vol. 28, ed J. Janick (New York, NY: John Wiley and Sons), 61–124.
Lee, J. M., Kubota, C., Tsao, S. J., Bie, Z., Echevarria, P. H., Morra, L., et al. (2010). Current status of vegetable grafting: diffusion, grafting techniques, automation. Sci. Hortic. 127, 93–105. doi: 10.1016/j.scienta.2010.08.003
Lei, B., Huang, Y., Xie, J. J., Liu, Z. X., Zhen, A., Fan, M. L., et al. (2014). Increased cucumber salt tolerance by grafting on pumpkin rootstockand after application of calcium. Biol. Plant. 58, 179–184. doi: 10.1007/s10535-013-0349-6
Leonardi, C., and Giuffrida, F. (2006). Variation of plant growth and macronutrient uptake in grafted tomatoes and eggplants on three different rootstocks. Eur. J. Hortic. Sci. 71, 97–101. Available online at: https://www.researchgate.net/publication/235799269_Variation_of_plant_growth_and_macronutrient_uptake_in_grafted_tomatoes_and_eggplants_on_three_different_rootstocks
Li, C., Wei, Z., Liang, D., Zhou, S., Li, Y., Liu, C., et al. (2013). Enhanced salt resistance in apple plants overexpressing a Malus vacuolar Na/H antiporter gene is associated with differences in stomatal behavior and photosynthesis. Plant Physiol. Biochem. 70, 164–173. doi: 10.1016/j.plaphy.2013.05.005
Li, C., Yu, X., Bai, L., He, C., and Yansu, L. (2016c). Responses of miRNAs and their target genes to nitrogen- or phosphorus-deficiency in grafted cucumber seedlings. Hortic. Environ. Biotechnol. 57, 97–112. doi: 10.1007/s13580-016-0092-y
Li, G., Ma, J., Tan, M., Mao, J., An, N., Sha, G., et al. (2016a). Transcriptome analysis reveals the effects of sugar metabolism and auxin and cytokinin signaling pathways on root growth and development of grafted apple. BMC Genomics 17:150. doi: 10.1186/s12864-016-2484-x
Li, H., Han, J. L., Chang, Y. H., Lin, J., and Yang, Q. S. (2016d). Gene characterization and transcription analysis of two new ammonium transporters in pear rootstock (Pyrus betulaefolia). J. Plant Res. 129, 737–748. doi: 10.1007/s10265-016-0799-y
Li, H., Wang, Y., Wang, Z., Guo, X., Wang, F., Xia, X. J., et al. (2016b). Microarray and genetic analysis reveals that csa-miR159b plays a critical role in abscisic acid-mediated heat tolerance in grafted cucumber plants. Plant Cell Environ. 39, 1790–1804. doi: 10.1111/pce.12745
Liu, G. D., Jiang, C. C., and Wang, Y. H. (2011). Distribution of boron and its forms in young “Newhall” navel orange (Citrus sinensis Osb.) plants grafted on two rootstocks in response to deficient and excessive boron. Soil Sci. Plant Nutr. 57, 93–104. doi: 10.1080/00380768.2010.551299
Liu, G. D., Wang, R. D., Liu, L. C., Wu, L. S., and Jiang, C. C. (2013). Cellular boron allocation and pectin composition in two citrus rootstock seedlings differing in boron-deficiency response. Plant Soil 370, 555–565. doi: 10.1007/s11104-013-1659-3
Liu, J., and Vance, C. P. (2010). Crucial roles of sucrose and microRNA399 in systemic signaling of P deficiency. A tale of two team players? Plant Signal. Behav. 5, 1–5. doi: 10.4161/psb.5.12.13293
López-Marín, J., González, A., Pérez-Alfocea, F., Egea-Gilabert, C., and Fernández, J. A. (2013). Grafting is an efficient alternative to shading screens to alleviate thermal stress in greenhouse grown sweet pepper. Sci. Hortic. 149, 39–46. doi: 10.1016/j.scienta.2012.02.034
Louws, F. J., Rivard, C. L., and Kubota, C. (2010). Grafting fruiting vegetables to manage soil borne pathogens, foliar pathogens, arthropods and weeds. Sci. Hortic. 127, 127–146. doi: 10.1016/j.scienta.2010.09.023
Mei, L., Sheng, O., Peng, S., Zhou, G., Wei, Q., and Li, Q. (2011). Growth, root morphology and boron uptake by citrus rootstock seedlings differing in boron-deficiency responses. Sci. Hortic. 129, 426–432. doi: 10.1016/j.scienta.2011.04.012
Meister, R., Rajani, M. S., Ruzicka, D., and Schachtman, D. P. (2014). Challenges of modifying root traits in crops for agriculture. Trends Plant Sci. 19, 779–788. doi: 10.1016/j.tplants.2014.08.005
Melnyk, C. W., and Meyerowitz, E. M. (2015). Plant grafting. Curr. Biol. 25, R183–R188. doi: 10.1016/j.cub.2015.01.029
Melnyk, C. W., Schuster, C., Leyser, O., and Meyerowitz, E. M. (2015). A developmental framework for graft formation and vascular reconnection in Arabidopsis thaliana. Current Biol. 25, 1306–1318. doi: 10.1016/j.cub.2015.03.032
Mestre, L., Reig, G., Betrán, J. A., Pinochet, J., and Moreno, M. A. (2015). Influence of peach-almond hybrids and plum-based rootstocks on mineral nutrition and yield characteristics of ‘Big Top’ nectarine in replant and heavy-calcareous soil conditions. Sci. Hortic. 192, 475–481. doi: 10.1016/j.scienta.2015.05.020
Miceli, A. C., Moncada, R. A., Piazza, G., Torta, L., D'Anna, F., and Vetrano, F. (2016). Yield and quality of mini-watermelon as affected by grafting and mycorrhizal inoculum. J. Agric. Sci. Technol. 18, 505–516. Available online at: http://jast.modares.ac.ir/article_14314_8201b9c9312c76336d62b1329c4c91f1.pdf
Miles, C., Wimer, J., and Inglis, D. (2015). “Grafting eggplant and tomato for Verticillium wilt resistance,” in Proceeding of the First International Symposium on Vegetable Grafting. Acta Horticulturae, Vol. 1086, eds Z. Bie Y. Huang, and M. A. Nawaz (Wuhan), 113–118.
Mitani, N., Kobayashi, S., Nishizawa, Y., Kuniga, T., and Matsumoto, R. (2006). Transformation of trifoliate orange with rice chitinase gene and the use of the transformed plant as a rootstock. Sci. Hortic. 108, 439–441. doi: 10.1016/j.scienta.2006.02.006
Mota, M., Neto, C., and Oliveira, C. M. (2008). Identification of gene coding for transporters in ‘Rocha’ Pear/BA29. Acta Hort. (ISHS) 800, 365–372. doi: 10.17660/ActaHortic.2008.800.46
Mudge, K., Janick, J., Scofield, S., and Goldschmidt, E. E. (2009). “A history of grafting,” in Horticultural Reviews, Vol. 35, ed J. Janick (New Jersey: John Wiley and Sons Inc), 437–493.
Nawaz, M. A., Huang, Y., Bie, Z., Ahmed, W., Reiter, R. J., Niu, M., et al. (2016). Melatonin: current status and future perspectives in plant science. Front. Plant Sci. 6:1230. doi: 10.3389/fpls.2015.01230
Niu, G., and Rodriguez, D. S. (2008). Responses of growth and ion uptake of four rose rootstocks to chloride- or sulfate-dominated salinity. J. Am. Soc. Hortic. Sci. 133, 663–669. Available online at: http://journal.ashspublications.org/content/133/5/663.full.pdf
Pant, B. D., Buhtz, A., Kehr, J., and Scheible, W. R. (2008). MicroRNA399 is a long- distance signal for the regulation of plant phosphate homeostasis. Plant J. 53, 731–738. doi: 10.1111/j.1365-313X.2007.03363.x
Parniske, M. (2008). Arbuscular mycorrhiza: the mother of plant root endosymbioses. Nat. Rev. Microbiol. 6, 763–775. doi: 10.1038/nrmicro1987
Paul, S., Datta, S. K., and Datta, K. (2015). miRNA regulation of nutrient homeostasis in plants. Front. Plant Sci. 6:232. doi: 10.3389/fpls.2015.00232
Penella, C., Landi, M., Guidi, L., Nebauer, S. G., Pellegrini, E., Bautista, A. S., et al. (2016). Salt-tolerant rootstock increases yield of pepper under salinity through maintenance of photosynthetic performance and sinks strength. J. Plant Physiol. 193, 1–11. doi: 10.1016/j.jplph.2016.02.007
Penella, C., Nebauer, S. G., Quinones, A., Bautista, A. S., López-Galarza, S., and Calatayud, A. (2015). Some rootstocks improve pepper tolerance to mild salinity through ionic regulation. Plant Sci. 230, 12–22. doi: 10.1016/j.plantsci.2014.10.007
Pérez-Alfocea, F. (2015). “Why should we investigate vegetable grafting?,” in Proceeding of the First International Symposium on Vegetable Grafting. Acta Horticulturae, Vol. 1086, eds Z. Bie Y. Huang, and M. A. Nawaz (Wuhan), 21–30.
Pérez-Alfocea, F., Albacete, A., Ghanem, M. E., and Dodd, I. C. (2010). Hormonal regulation of source-sink relations to maintain crop productivity under salinity: a case study of root-to-shoot signalling in tomato. Funct. Plant Biol. 37, 592–603. doi: 10.1071/FP10012
Pinto, E., and Ferreira, I. M. P. L. V. O. (2015). Cation transporters/channels in plants: tools for nutrient biofortification. J. Plant Physiol. 179, 64–82. doi: 10.1016/j.jplph.2015.02.010
Pulgar, G., Villora, G., Moreno, D. A., and Romero, L. (2000). Improving the mineral nutrition in grafted watermelon plants: nitrogen metabolism. Biol. Plant. 43, 607–609. doi: 10.1023/A:1002856117053
Qi, H. Y., Li, T. L., Liu, Y. F., and Li, D. (2006). Effects of grafting on photosynthesis characteristics, yield, and sugar content in melon. J. Shenyang Agric. Univ. 37, 155–158. Available online at: http://en.cnki.com.cn/Article_en/CJFDTOTAL-SYNY200602006.htm
Relf, D. (2015). The Art of Bonsai. Blacksburg, VA: Communications and marketing, College of Agriculture and Life Sciences, Virginia Polytechnic Institute and State University. Publication No. 426 601, 1–6.
Rengel, Z., and Damon, P. M. (2008). Crops and genotypes differ in efficiency of potassium uptake and use. Physiol. Plant. 133, 624–636. doi: 10.1111/j.1399-3054.2008.01079.x
Rivero, R. M., Ruiz, J. M., and Romero, L. (2004). Iron metabolism in tomato and watermelon plants: influence of grafting. J. Plant Nutr. 27, 2221–2234. doi: 10.1081/PLN-200034708
Rogers, E. D., and Benfey, P. N. (2015). Regulation of plant root system architecture: implications for crop advancement. Curr. Opin. Biotechnol. 32, 93–98. doi: 10.1016/j.copbio.2014.11.015
Rouphael, Y., Cardarelli, M., Rea, E., and Colla, G. (2008). Grafting of cucumber as a means to minimize copper toxicity. Environ. Exp. Bot. 63, 49–58. doi: 10.1016/j.envexpbot.2007.10.015
Rouphael, Y., Schwarz, D., Krumbein, A., and Colla, G. (2010). Impact of grafting on product quality of fruit vegetables. Sci. Hortic. 127, 172–179. doi: 10.1016/j.scienta.2010.09.001
Rubio, V., Linhares, F., Solano, R., Martin, A. C., Iglesias, J., Leyva, A., et al. (2001). A conserved MYB transcription factor involved in phosphate starvation signaling both in vascular plants and in unicellular algae. Genes Dev. 15, 2122–2133. doi: 10.1101/gad.204401
Ruiz, J. M., Belakbir, A., Lhpez-Cantarero, I., and Romero, L. (1997). Leaf-macronutrient content and yield in grafted melon plants. A model to evaluate the influence of rootstock genotype. Sci. Hortic. 71, 227–234. doi: 10.1016/S0304-4238(97)00106-4
Ruiz, J. M., Rivero, R. M., Cervilla, L. M., Castellano, R., and Romero, L. (2006). Grafting to improve nitrogen-use efficiency traits in tobacco plants. J. Sci. Food Agric. 86, 1014–1021. doi: 10.1002/jsfa.2450
Salehi, R., Kashi, A., Lee, J. M., and Javanpour, R. (2014). Mineral concentration, sugar content and yield of Iranian ‘Khatooni’ melon affected by grafting, pruning and thinning. J. Plant Nutr. 37, 1255–1268. doi: 10.1080/01904167.2014.888740
Santa-Cruz, A., Martinez-Rodriguez, M. M., Perez-Alfocea, F., Romero-Aranda, R., and Bolarin, M. C. (2002). The rootstock effect on the tomato salinity response depends on the shoot genotype. Plant Sci. 162, 825–831. doi: 10.1016/S0168-9452(02)00030-4
Santa-Maria, G. E., Moriconi, J. I., and Oliferuk, S. (2015). Internal efficiency of nutrient utilization: what is it and how to measure it during vegetative plant growth? J. Exp. Bot. 66, 3011–3018. doi: 10.1093/jxb/erv162
Savvas, D., Colla, G., Rouphael, Y., and Schwarz, D. (2010). Amelioration of heavy metal and nutrient stress in fruit vegetables by grafting. Sci. Hortic. 127, 156–161. doi: 10.1016/j.scienta.2010.09.011
Savvas, D., Ntatsi, G., and Barouchas, P. (2013). Impact of grafting and rootstock genotype on cation uptake by cucumber (Cucumis sativus L.) exposed to Cd or Ni stress. Sci. Hortic. 149, 86–96. doi: 10.1016/j.scienta.2012.06.030
Savvas, D., Papastavrou, D., Ntatsi, G., Ropokis, A., and Olympios, C., Hartmann, H., et al. (2009). Interactive effects of grafting and manganese supply on growth, yield, and nutrient uptake by tomato. HortScience 44, 1978–1982. Available online at: http://hortsci.ashspublications.org/content/44/7/1978.full.pdf
Schwarz, D., Öztekin, G. B., Tüzel, Y., Brückner, B., and Krumbein, A. (2013). Rootstocks can enhance tomato growth and quality characteristics at low potassium supply. Sci. Hortic. 149, 70–79. doi: 10.1016/j.scienta.2012.06.013
Schwarz, D., Rouphael, Y., Colla, G., and Venema, J. H. (2010). Grafting as a tool to improve tolerance of vegetables to abiotic stresses: thermal stress, water stress and organic pollutants. Sci. Hortic. 127, 162–171. doi: 10.1016/j.scienta.2010.09.016
Sherafati, A., Hokmabadi, H., and Taheri, M. (2011). Effects of some rootstocks on mineral nutrient uptake in two pistachio cultivars (Pistacia vera L.). Acta Hortic. 912, 97–201. doi: 10.17660/actahortic.2011.912.28
Slowik, K., Labanauskas, C. K., Stolzy, L. H., and Zentmyer, G. A. (1979). Influence of rootstocks, soil oxygen, and soil moisture on the uptake and translocation of nutrients in young avocado. J. Am. Soc. Hortic. Sci. 104, 172–175.
Sorgona, A., Abenavoli, M. A., Gringeri, P. G., and Cacco, G. (2006). A comparison of nitrogen use efficiency definitions in citrus rootstocks. Sci. Hortic. 109, 389–393. doi: 10.1016/j.scienta.2006.06.001
Suchoff, D., Gunter, C., Schulthesis, J., and Louws, F. J. (2015). “On farm grafted tomato trial to manage bacterial wilt,” in Proceeding of the First International Symposium on Vegetable Grafting. Acta Horticulturae, Vol. 1086, eds Z. Bie Y. Huang, and M. A. Nawaz (Wuhan), 119–127.
Sun, Y., Huang, W., Tian, X. H., Wu, Y., Zhou, C. T., and Ding, Q. (2002). Study on growth situation, photosynthetic characteristics and nutrient absorption of grafted cucumber seedlings. J. Plant Nutr. Fert. Sci. 8, 81–185, 209. Available online at: http://www.plantnutrifert.org/EN/abstract/abstract2236.shtml
Sunkar, R., Chinnusamy, V., and Zhu, J. K. (2007). Small RNAs as big players in plant abiotic stress responses and nutrient deprivation. Trends Plant Sci. 12, 301–309. doi: 10.1016/j.tplants.2007.05.001
Tabata, R., Sumida, K., Yoshii, T., Ohyama, K., Shinohara, H., and Matsubayashi, Y. (2014). Perception of root-derived peptides by shoot LRR-RKs mediates systemic N-demand signaling. Science 346, 343–346. doi: 10.1126/science.1257800
Todd, C. D., Zeng, P., Huete, A. M. R., Hoyos, M. E., and Polacco, J. C. (2004). Transcripts of MYB-like genes respond to phosphorous and nitrogen deprivation in Arabidopsis. Planta 219, 1003–1009. doi: 10.1007/s00425-004-1305-7
Tomasi, N., Monte, R., Varanini, Z., Cesco, S., and Pinton, R. (2015). Induction of nitrate uptake in Sauvignon Blanc and Chardonnay grapevines depends on the scion and is affected by the rootstock. Aust. J. Grape Wine Res. 21, 331–338. doi: 10.1111/ajgw.12137
Treeby, M., Marschner, H., and Romheld, V. (1989). Mobilization of iron and other micronutrients cations from a calcerous soil by plant-borne, microbial, and synthetic metal chelators. Plant Soil 114, 217–226. doi: 10.1007/BF02220801
Tsaballa, A., Athanasiadis, C., Pasentsis, K., Ganopoulos, I., Nianiou-Obeidat, I., and Tsaftaris, A. (2013). Molecular studies of inheritable grafting induced changes in pepper (Capsicum annuum) fruit shape. Sci. Hortic. 149, 2–8. doi: 10.1016/j.scienta.2012.06.018
Uygur, V., and Yetisir, H. (2009). Effects of rootstocks on some growth parameters, phosphorous and nitrogen uptake by watermelon under salt stress. J. Plant Nutr. 32, 629–643. doi: 10.1080/01904160802715448
Valdés-Lopez, O., Yang, S. S., Aparicio-Fabre, R., Graham, P., Reyes, J. L., Vance, C. P., et al. (2010). MicroRNA expression profile in common bean (Phaseolus vulgaris) under nutrient deficiency stresses and manganese toxicity. New Phytol. 187, 805–818. doi: 10.1111/j.1469-8137.2010.03320.x
Van-Hooijdonk, B. M., Woolley, D. J., Warrington, I. J., and Tustin, D. S. (2010). Initial alteration of scion architecture by dwarfing apple rootstocks may involve shoot–root–shoot signalling by auxin, gibberellin, and cytokinin. J. Hortic. Sci. Biotechnol. 85, 59–65. doi: 10.1080/14620316.2010.11512631
Venema, J. H., Elzenga, J. T. M., and Bouwmeester, H. J. (2011). “Selection and breeding of robust rootstocks as a tool to improve nutrient-use efficiency and abiotic stress tolerance in tomato,” in Proceeding First International Conference on Organic Greenhouse Horticulture. Acta Horticulture, Vol. 915, eds M. Dorais, and S. D. Bishop (Bleiswijk), 109–116.
Wahb-Allah, M. A. (2014). Effectiveness of grafting for the improvement of salinity and drought tolerance in tomato (Solanum lycopersicon L.). Asian J. Crop Sci. 6, 112–122. doi: 10.3923/ajcs.2014.112.122
Wang, N., Wei, Q., Yan, T., Pan, Z., Liu, Y., and Peng, S. (2016a). Improving the boron uptake of boron-deficient navel orange plants under low boron conditions by inarching boron-efficient rootstock. Sci. Hortic. 199, 49–55. doi: 10.1016/j.scienta.2015.12.014
Wang, P., Wu, S. H., Wen, M. X., Wang, Y., and Wu, Q. S. (2016b). Effects of combined inoculation with Rhizophagus intraradices and Paenibacillus mucilaginosus on plant growth, root morphology, and physiological status of trifoliate orange (Poncirus trifoliata L. Raf.) seedlings under different levels of phosphorus. Sci. Hortic. 205, 97–105. doi: 10.1016/j.scienta.2016.04.023
Warschefsky, E. J., Klein, L. L., Frank, M. H., Chitwood, D. H., Londo, J. P., Eric, J. B., et al. (2016). Rootstocks: diversity, domestication, and impacts on shoot phenotypes. Trends Plant Sci. 21, 418–437. doi: 10.1016/j.tplants.2015.11.008
Wei, S., Wu, Y. Z., and Huang, J. (2006). Effects of rootstocks on growth and photosynthetic properties of grafted plants of netted melon. Acta Agric. Shanghai 22, 114–117.
Weng, J. H. (2000). The role of active and passive water uptake in maintaining leaf water status and photosynthesis in tomato under water deficit. Plant Prod. Sci. 3, 296–298. doi: 10.1626/pps.3.296
Wissuwa, M., Kretzschmar, T., and Rose, T. J. (2016). From promise to application: root traits for enhanced nutrient capture in rice breeding. J. Exp. Bot. doi: 10.1093/jxb/erw061. Available online at: http://jxb.oxfordjournals.org/content/early/2016/03/23/jxb.erw061.full.pdf+html
Xie, Y. F., Tian, Y. Q., Li, S., Yao, K. Q., Ma, Z. M., Yang, Y. T., et al. (2012). Effects of single-root-grafting and double-root-grafting on cucumber rhizosphere soil microorganism and enzyme activity. China Vegetables 24, 62–68. Available online at: http://xueshu.baidu.com/s?wd=paperuri%3A%28455933d794c8eadae778d00930553aa3%29&filter=sc_long_sign&tn=SE_xueshusource_2kduw22v&sc_vurl=http%3A%2F%2Fen.cnki.com.cn%2FArticle_en%2FCJFDTotal-ZGSC201224016.htm&ie=utf-8&sc_us=4674814618225490452
Xin, J., Huang, B., Yang, J., Yang, Z., Yuan, J., and Mu, Y. (2013). Role of roots in cadmium accumulation of two water spinach cultivars: reciprocal grafting and histochemical experiments. Plant. Soil 366, 425–432. doi: 10.1007/s11104-012-1439-5
Xu, F., Liu, Q., Chen, L., Kuang, J., Walk, T., Wang, J., et al. (2013). Genome-wide identification of soybean microRNAs and their targets reveals their organ- specificity and responses to phosphate starvation. BMC Genomics 14:66. doi: 10.1186/1471-2164-14-66
Yetisir, H., Özdemir, A. E., Aras, V., Candır, E., and Aslan, O. (2013). Rootstocks effect on plant nutrition concentration in different organ of grafted watermelon. Agric. Sci. 4, 230–237. doi: 10.4236/as.2013.45033
Zamboni, M., Garavani, A., Gatti, M., Vercesi, A., Parisi, M. G., Bavaresco, L., et al. (2016). Vegetative, physiological and nutritional behavior of new grapevine rootstocks in response to different nitrogen supply. Sci. Hortic. 202, 99–106. doi: 10.1016/j.scienta.2016.02.032
Zarrouk, O., Gogorcena, Y., Gomez-Aparisi, J., Betran, J. A., and Moreno, M. A. (2005). Influence of almond peach hybrids rootstocks on flower and leaf mineral concentration, yield and vigour of two peach cultivars. Sci. Hortic. 106, 502–514. doi: 10.1016/j.scienta.2005.04.011
Zen, Y. A., Zhu, Y. L., Huang, B. J., and Yang, L. F. (2004). Effects of Cucurbita ficifolia as rootstock on growth, fruit setting, disease resistance and leaf nutrient element contents in Cucumis sativus, J. Plant Resour. Environ. 13, 15–19. Available online at: http://en.cnki.com.cn/Article_en/CJFDTOTAL-ZWZY200404004.htm
Zeng, H., Wang, G., Hu, X., Wang, H., Du, L., and Zhu, Y. (2014). Role of microRNAs in plant responses to nutrient stress. Plant Soil 374, 1005–1021. doi: 10.1007/s11104-013-1907-6
Zha, Q., Wang, Y., Zhang, X. Z., and Han, Z. H. (2014). Both immanently high active iron contents and increased root ferrous uptake in response to low iron stress contribute to the iron deficiency tolerance in Malus xiaojinensis. Plant Sci. 214, 47–56. doi: 10.1016/j.plantsci.2013.10.002
Zhang, J., Chen, S., Liu, R., Jiang, J., Chen, F., and Fang, W. (2013). Chrysanthemum cutting productivity and rooting ability are improved by grafting. Sci. World J. 2013:286328. doi: 10.1155/2013/286328
Zhang, L., Meng, X. X., Liu, N., Yang, J. H., and Zhang, M. F. (2012). Effects of grafting on phosphorus uptake and utilization of watermelon at early stage under low phosphorus stress. J. Fruit Sci. 29, 120–124. doi: 10.13925/j.cnki.gsxb.2012.01.024
Zhang, Z. K., Li, H., Zhang, Y., Huang, Z. J., Chen, K., and Liu, S. Q. (2010). Grafting enhances copper tolerance of cucumber through regulating nutrient uptake and antioxidative system. Agric. Sci. China. 9, 1758–1770. doi: 10.1016/S1671-2927(09)60274-1
Zhao, X., Guo, Y., Huber, D. J., and Lee, J. (2011). Grafting effects on postharvest ripening and quality of 1-methylcyclopropene-treated muskmelon fruit. Sci. Hortic. 130, 581–587. doi: 10.1016/j.scienta.2011.08.010
Zhou, J., Wan, H., Qin, S., He, J., Lyu, D., and Li, H. (2016). Net cadmium flux and gene expression in relation to differences in cadmium accumulation and translocation in four apple rootstocks. Environ. Exp. Bot. 130, 95–105. doi: 10.1016/j.envexpbot.2016.05.012
Keywords: grafting, rootstock, scion, root growth, ion uptake, miRNAs, utilization efficiency, transgrafting
Citation: Nawaz MA, Imtiaz M, Kong Q, Cheng F, Ahmed W, Huang Y and Bie Z (2016) Grafting: A Technique to Modify Ion Accumulation in Horticultural Crops. Front. Plant Sci. 7:1457. doi: 10.3389/fpls.2016.01457
Received: 24 July 2016; Accepted: 12 September 2016;
Published: 21 October 2016.
Edited by:
Janin Riedelsberger, University of Talca, ChileReviewed by:
Serge Delrot, University of Bordeaux, FranceCopyright © 2016 Nawaz, Imtiaz, Kong, Cheng, Ahmed, Huang and Bie. This is an open-access article distributed under the terms of the Creative Commons Attribution License (CC BY). The use, distribution or reproduction in other forums is permitted, provided the original author(s) or licensor are credited and that the original publication in this journal is cited, in accordance with accepted academic practice. No use, distribution or reproduction is permitted which does not comply with these terms.
*Correspondence: Yuan Huang, aHVhbmd5dWFuQG1haWwuaHphdS5lZHUuY24=
Zhilong Bie, YmllemhpbG9uZ0Bob3RtYWlsLmNvbQ==
Disclaimer: All claims expressed in this article are solely those of the authors and do not necessarily represent those of their affiliated organizations, or those of the publisher, the editors and the reviewers. Any product that may be evaluated in this article or claim that may be made by its manufacturer is not guaranteed or endorsed by the publisher.
Research integrity at Frontiers
Learn more about the work of our research integrity team to safeguard the quality of each article we publish.