- 1Environmental Microbiology and Food Safety Laboratory, U.S. Department of Agriculture Agricultural Research Service, Beltsville, MD USA
- 2Department of Food Science and Nutrition, University of Maryland, College Park, MD, USA
- 3Crop Systems and Global Change Laboratory, U.S. Department of Agriculture Agricultural Research Service, Beltsville, MD, USA
The genus Alyssum (Brassicaceae) contains Ni hyperaccumulators (50), many of which can achieve 30 g kg−1 Ni in dry leaf. Some Alyssum hyperaccumulators are viable candidates for commercial Ni phytoremediation and phytomining technologies. It is not known whether these species secrete organic and/or amino acids into the rhizosphere to solubilize Ni, or can make use of such acids within the soil to facilitate uptake. It has been hypothesized that in fields with mixed plant species, mobilization of metals by phytosiderophores secreted by Graminaceae plants could affect Alyssum Ni, Fe, Cu, and Mn uptake. We co-cropped the Ni hyperaccumulator Alyssum murale, non-hyperaccumulator A. montanum and perennial ryegrass in a natural serpentine soil. All treatments had standard inorganic fertilization required for ryegrass growth and one treatment was compost amended. After 4 months A. murale leaves and stems contained 3600 mg kg−1 Ni which did not differ significantly with co-cropping. Overall Ni and Mn concentrations were significantly higher in A. murale than in A. montanum or L. perenne. Copper was not accumulated by either Alyssum species, but L. perenne accumulated up to 10 mg kg−1. A. montanum could not compete with either A. murale or ryegrass, and neither Alyssum species survived in the compost-amended soil. Co-cropping with ryegrass reduced Fe and Mn concentrations in A. murale but not to the extent of either increasing Ni uptake or affecting plant nutrition. The hypothesized Alyssum Ni accumulation in response to phytosiderophores secreted by co-cropped grass did not occur. Our data do not support increased mobilization of Mn by a phytosiderophore mechanism either, but the converse: mobilization of Mn by the Alyssum hyperaccumulator species significantly increased Mn levels in L. perenne. Tilling soil to maximize root penetration, adequate inorganic fertilization and appropriate plant densities are more important for developing efficient phytoremediation and phytomining approaches.
Introduction
More than 400 plant species are known to naturally accumulate high levels of metals such as Cd, Cu, Co, Mn, Ni, and Zn (Baker et al., 2010; Krämer, 2010; van der Ent et al., 2013). The genus Alyssum (Brassicaceae) contains the greatest number of reported Ni hyperaccumulators (50), many of which can achieve 30 g kg−1 Ni in dry leaf biomass (Baker and Brooks, 1989; Reeves and Adigüzel, 2008; van der Ent et al., 2015). Previously we have demonstrated commercially feasible phytoremediation and phytomining technologies that can potentially clean up Ni-contaminated soils and recover high purity Ni metal (Chaney et al., 1999, 2010; Li et al., 2003a,b; Nkrumah et al., 2016). The technology employs the Ni-hyperaccumulating species Alyssum murale and A. corsicum to phytoextract Ni from a range of Ni-rich soil types. A. murale and A. corsicum are endemic to serpentine soils developed from ultramafic rock throughout Mediterranean Southern Europe.
Ni localization patterns have been determined for 10 Alyssum Ni hyperaccumulator species/ecotypes (Krämer et al., 1997; Psaras et al., 2000; Küpper et al., 2001; Kerkeb and Krämer, 2003; Marmiroli et al., 2004; Broadhurst et al., 2004a,b, 2009; McNear et al., 2005; Asemaneh et al., 2006; Tappero et al., 2007). Nickel is stored mainly in the leaves, and is particularly concentrated in in vacuoles of epidermal cells and trichome pedicels. Alyssum hyperaccumulators also accumulate appreciable Mn in the same locations that contain Ni (Broadhurst et al., 2004b, 2009).
Although Ni hyperaccumulation is a constitutive property for these Alyssum species, it is not known whether they secrete organic and/or amino acids into the rhizosphere to solubilize Ni, or can make use of such acids within the soil to greatly facilitate uptake. Other than rhizobiome interactions, there is essentially no evidence for unusual ligand species or highly elevated ligand concentrations associated with Ni in Alyssum (McNear et al., 2010; Centofanti et al., 2013). There is evidence that rhizosphere bacteria endemic to serpentine soils may stimulate Ni uptake and this may be an important factor explaining why field trials and native vegetation consistently outperform pot and hydroponic studies with respect to phytoextraction yields (Abou-Shanab et al., 2003, 2007; Rajkumar et al., 2009, 2013; Cabello-Conejo et al., 2014; Visioli et al., 2015). Two serpentine-endemic bacteria in particular (Microbacterium arabinogalactanolyticum and M. oxydans) were shown to strongly increase Ni accumulation in A. murale (Abou-Shanab et al., 2003, 2007). Similarly, endemic Arthrobacter sp. increased Ni uptake in A. pintodasilvae and A. serpyllifolium (Cabello-Conejo et al., 2014).
Cd/Zn hyperaccumulators have not shown evidence for specialized ligand secretion into the rhizosphere either (Zhao et al., 2001; Whiting et al., 2001a; Sterckeman et al., 2005; Wang et al., 2006). Specifically, root exudates collected from the Cd/Zn hyperaccumulator Noccaea caerulescens F.K. Mey (Brassicaceae) (syn. Thlaspi caerulescens J &C Presl) did not mobilize Cd, Cu, Fe, or Zn (Zhao et al., 2001). Further, Cd/Zn hyperaccumulators may not take advantage of potential phytosiderophore-related improvements in metal solubilization provided by intercropping with Graminaceae.
The grass family of plants differs from all other plant families by using a different mechanism of absorbing Fe from soils. All other species use a combination of acidification of the rhizosphere and reduction of ferric to ferrous coupled with absorption of ferrous ion. Instead, Graminaceae use a combination of chelating amino acids, the phytosiderophores of the mugineic acid family of compounds, for specific uptake of intact Fe–phytosiderophore chelates. It is known that phytosiderophores are not highly specific to Fe and can increase mobilization and possibly support uptake of Zn, Mn, Ni, Cu, and Cd as well (Zhang et al., 1991a,b; Marschner and Römheld, 1994; Awad and Römheld, 2000). Intercropping peanut (Arachis hypogaea L.), for example, with maize, oats, barley or wheat significantly increased Fe, Cu, and Zn uptake to the extent that Fe deficiency in peanut could be mitigated (Zuo and Zhang, 2011).
Previous results from co-cropping hyperaccumulators and grasses are mixed. N. caerulescens had no increase in Cd or Zn concentration when grown in the same pot with ryegrass (Lolium perenne L.), but yield was almost doubled in an experiment where plants were grown with sufficient time and soil volume to establish potential rhizosphere interactions with or without root mingling (Jiang et al., 2010). It was determined that the ryegrass did not solubilize Cd and Zn, however Fe was not discussed. The improved yield could be at least partially due to improved Fe availability. Increased P availability from arbuscular mycorrhizal fungi which are known to colonize Graminaceae including ryegrass (Grimold et al., 2005) is another factor which could significantly affect yield since many of the metalliferous soils that hyperaccumulators are native to are P deficient. However, co-planting the Cd/Zn hyperaccumulator Sedum alfredii (Hance) with ryegrass reduced both S. alfredii yield and Cd uptake (Wang et al., 2013). Co-planting with corn improved S. alfredii yield by providing shade but did not increase Cd uptake. Cd and Zn uptake by corn was unaltered by co-cropping and corn did not suffer phytotoxicity (Wu et al., 2007).
Co-cropping barley (Hordeum vulgare L.) and N. caerulescens in multiple metal-rich soils from a biosolids management facility showed little evidence for interaction between plants other than a slight increase in Cd, Cu, Ni, Zn in co-cropped pots with root interaction vs. N. caerulescens alone, but this probably reflected simple depletion of metals in the relatively small soil volume utilized, and not a specific phytosiderophore mechanism (Gove et al., 2002). Again, Fe was not considered in the experiment. Both Gove et al. (2002) and Jiang et al. (2010) observed an increase in ryegrass Cd but not Zn concentrations when grown with N. caerulescens. Similarly, Whiting et al. (2001a) showed no interaction between N. caerulescens and Festuca rubra L. with respect to Zn levels or yield.
Improved growth and reduced Zn uptake by the non-hyperaccumulator Thlaspi arvense L. was reported when T. arvense and T. caerulescens were grown together in pots that allowed root intermingling. Zinc salts were added to the soils at a level that was phytotoxic to T. arvense. Zn hyperaccumulation by T. caerulescens was not affected, however yield increased when root intermingling was allowed, leading to the conclusion that this system could facilitate revegetation of contaminated soils (Whiting et al., 2001b).
Herein we report a co-cropping experiment with Alyssum hyperaccumulator and non-hyperaccumulator species and perennial ryegrass in a natural serpentine soil. The soil is infertile and high in Ni, but is not Ni phytotoxic (Zhang et al., 2007) and supports native vegetation. Soils such as this are candidates for Ni phytomining (Chaney et al., 2010; Nkrumah et al., 2016). We tested whether ryegrass facilitates Ni, Fe, and Mn uptake by Alyssum. whether co-cropping with ryegrass affects Alyssum yield, and whether Alyssum hyperaccumulator and non-hyperaccumulator species can benefit from co-cropping.
Materials and Methods
Horticulture
A. murale (Waldst. et Kit) “Kotodesh,” a Ni-hyperaccumulator, was grown from seed collected from a wild Albanian serpentine population. All Alyssum hyperaccumulator species known have leaves covered with stellate trichomes (Figure 1). Nickel is stored in leaf epidermal cells, particularly in the trichome pedicels. A. montanum L. “Mountain Gold” (a non-hyperaccumulator species that also has leaf trichomes) was grown from commercial seed (Hazzard's Seeds, Deford, MI). Both Alyssum species were started in flats with Promix® potting soil and standard fertilization (half-strength Miracle Grow®). After 40 days healthy Alyssum seedling roots were rinsed to remove potting medium and transplanted to prepared soils in pots. Four weeks after transplant, when seedlings had become established, commercial perennial ryegrass (L. perenne L. “Amazing GS,” Ampac Seed, Tangent, OR) was seeded directly into the pots. Twenty cm polyethylene pots which hold about 3 kg air dry soil were utilized. Plastic mesh was not used across the pot over the drain holes in order to avoid interference with root growth. All watering was with deionized water, and plastic trays were placed under each pot. To avoid overwatering Alyssum. 250–650 ml was added 2 or 3 times per week to ensure that soil dried between waterings. The co-cropped plants were grown for an additional 12 weeks.
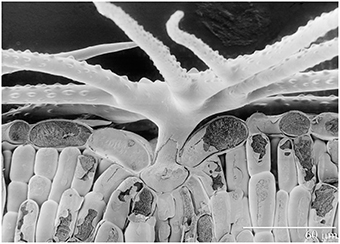
Figure 1. Scanning electron photomicrograph of frozen hydrated Ni-hyperaccumulator Alyssum murale ‘Kotodesh’ and surface of leaf. Note stellate trichome; Ni is very high in the vacuole below the trichome.
We utilized Brockman variant serpentine soil from Josephine Co., Oregon (Typic Xerochrepts), air dried and sieved <4 mm using stainless steel sieves. Standard inorganic fertilization for serpentine soils (75 mg N as NH4NO3, 100 mg P as KH2PO4, 500 mg Ca as 2H2O, and 0.5 mg B as H3BO3 per kg soil) was added. The Brockman soil as collected is pH 6.6, very high in Ni (4710 mg kg−1), more than adequate in Mn and Fe, but deficient in Ca and P (Table 1). One set of treatments had 30 vol% (10%DW) aged dairy manure compost from the USDA Beltsville composting facility mixed into the serpentine soil. Fertilizer rates allowed normal growth of ryegrass on this soil which would not normally support growth of non-serpentinophytes.
The experiment was conducted in the USDA Beltsville greenhouse under controlled temperature and light conditions and ambient humidity. Photoperiod was 15/9 h day/night. During this time supplemental high-intensity sodium and incandescent lights capable of supplying 400 μmol m−2s−1 supplemented sunlight if necessary. Daytime temperature was 24°C with cooling initiated at 27°C. Nighttime temperature was 18°C with cooling initiated at 21°C. During the final 3 weeks of growth in late May and June supplemental lighting was turned off to avoid overheating.
Experimental Treatments
Two types of soil and six planting schemes made up 12 treatments, with three replicates per treatment. Alyssum plants that died soon after transplanting were replaced for the first 2 weeks of growth. Overall Alyssum grew 4 months after transplant, and rye grass grew 3 months after seeding. At harvest plant roots filled the pot and intermingled but plants were not pot-bound.
Soil A: Natural Brockman variant serpentine soil.
Soil B: Natural Brockman variant serpentine soil with 10 wt% compost.
Planting Scheme:
1. A. murale, four plants per pot spread radially and evenly.
2. A. montanum, four plants per pot spread radially and evenly.
3. L. perenne four groups of approximately 20 seedlings spread radially and evenly.
4. A. murale two plants, and A. montanum, 2 plants with the same plant spread as #1, alternating species.
5. A. murale two plants, and L. perenne two groups of approximately 20 seedlings, alternating species.
6. A. montanum two plants, and L. perenne two groups of approximately 20 seedlings alternating species.
Plant Material Metals Analysis
All clean, healthy aerial plant material was harvested. Material that was stained from the red serpentine soil or unhealthy was discarded. Harvested plant material was washed in a dilute detergent bath and rinsed in deionized water to remove adhering soil particles. Plants were dried for 72 h at 60°C, weighed, and ashed in a 480°C oven for 16 h. After cooling, the ash was digested with 2 ml concentrated HNO3, mixed well and then heated to dryness. The sample was then dissolved in 10 ml 3 N HCl, filtered through Whatman #40 filter paper and brought to volume in a 25 ml volumetric flask using 0.1 N HCl. Concentrations of Ca, Cd, Cu, Fe, K, Mg, Ni, Mn, P, and Zn were determined by inductively-coupled plasma atomic emission spectrometry using 40 mg L−1 yttrium as an internal standard in all samples and standard solutions.
Soil Analysis
Total soil metals were measured by atomic absorption spectrometry after digestion with boiling HNO3. Exchangeable Ca, Mg, and Ni were obtained by extracting 5 g air-dried soil with 50 mL 1.0 M ammonium acetate at pH 7, soil texture by pipette method, and organic matter by combustion. The Bray-2 method was used to estimate phytoavailable P. The DTPA-extraction used 5 g soil per 50 mL standard DTPA extractant rather than the usual 10 g per 20 mL because of the high metals levels in this and other Ni-rich soils studied in our laboratory.
Results
Yields and dry weight metal concentrations are reported in Table 2, and examples of co-cropped healthy plants in the serpentine soil (A) are given in Figures 2, 3. All results were statistically analyzed by ANOVA with SAS. None of the Alyssum transplants survived in compost-amended soil B. The compost evidently contained pathogen(s) that both species were susceptible to, and it also kept the soil damp longer between waterings. Normally this is desirable in pot studies, however A. murale in particular is adapted to semi-arid conditions, and once established survives with watering once per week or less. The symptoms exhibited were consistent with fungal infection. None of the plants in soil A or seedlings in Promix were affected.
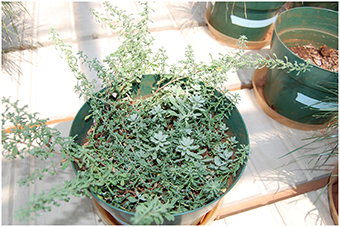
Figure 2. A. murale (most of the plant material in pot, with long stems, and darker oblong leaves) and A. montanum (a few plants in center with larger, lighter leaves in a rosette pattern) co-cropped in the fertilized serpentine soil. A. montanum grew less vigorously than A. murale in the fertilized serpentine soil, and was outcompeted and shaded after 4–5 weeks.
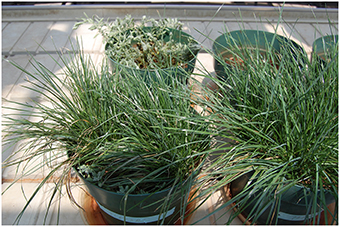
Figure 3. Lolium perenne and A. montanum co-cropped in the fertilized serpentine soil. The nonhyperacumulator Alyssum species was not able to compete with the grass.
In soil A, A. murale shoots contained approximately 3600 mg kg−1 Ni which did not differ significantly with co-cropping (Figure 4). Overall Ni and Mn concentrations were significantly higher in A. murale than A. montanum or L. perenne (Figures 4, 5). However, A. murale Fe concentrations were significantly reduced (p < 0.05) by co-cropping with ryegrass, and Mn was somewhat reduced (p < 0.4) but half-pot yield was equivalent. In general Fe concentrations were unreliable in A. montanum due to contamination with Fe3+ oxide staining deep within the leaves, coupled with only a small amount of plant material available for analysis, but this does not affect the relationship between Ni and Mn in A. murale vs. A. montanum. Although Fe is much higher in A. montanum than A. murale, both Ni and Mn are significantly lower (p < 0.01), a result that cannot be due to soil contamination.
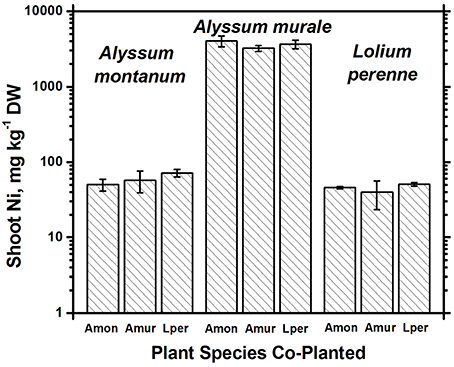
Figure 4. Ni concentrations for species in monoculture and co-cropped. Nickel levels were significantly greater in the hyperacccumulator Alyssum murale (p < 0.001) but did not differ significantly within a given species as a function of co-cropping. Error bars means ± Standard Error.
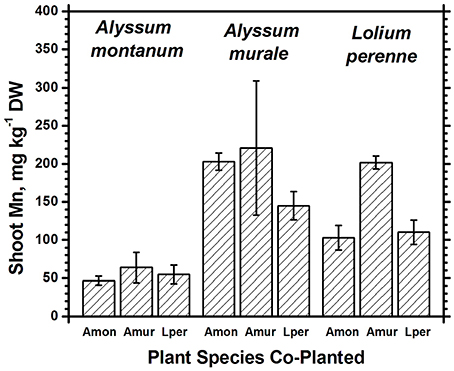
Figure 5. Mn concentrations for species in monoculture and co-cropped. Mn levels were significantly greater in A. murale than A. montanum. Mn was increased in L. perenne with A. murale co-cropping. Error bars means ± Standard Error.
Nickel concentrations in A. montanum and L. perenne remained relatively constant and below 75 mg kg−1 in for A2 through A6. However, there was increased variability of the Ni concentration in both A. murale or L. perenne with A. murale intercropping, and intercropping with A. murale significantly increased Mn in L. perenne (Figure 5). Calcium concentrations were three to six times greater in Alyssum species as compared to L. perenne due to the high Ca in Alyssum leaf trichomes (Table 2; Figure 1). However, Cu concentrations were consistently greater in L. perenne than Alyssum (Figure 6).
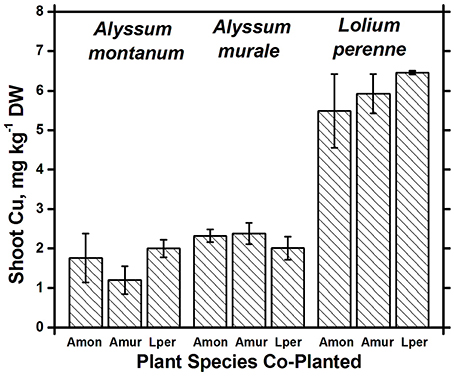
Figure 6. Cu concentrations for species in monoculture and co-cropped. Copper levels were significantly greater in Lolium perenne compared to both Alyssum species (p < 0.001). With the exception of a slight Cu reduction in A. montanum co-cropped with A. murale, Cu concentrations did not differ significantly within a given species as a function of co-cropping. Error bars means ± Standard Error.
In soil A, A. montanum could not compete with either A. murale or ryegrass and was nearly killed by co-cropping, with 10-fold yield reductions (Figures 3, 4). Due to its poor growth, A. montanum did not significantly affect the growth or metal concentrations of A. murale or L. perenne. However, there was a significant ryegrass yield reduction with A. murale co-cropping. Ryegrass yield was increased when co-cropped with A. montanum because it thoroughly out-competed A. montanum with only half the plants.
Because Alyssum did not survive in the treatments with compost, all three ryegrass planting schemes grew ryegrass only. Essentially there were nine replicates for B3, all of which had Ni concentrations that did not differ significantly from one another, but did differ from A3 and A5, as expected due to the high levels of Ni in the serpentine soil (Figure 4). There was a trend for increased yield with compost but it was not significant. Calcium, Fe, Mg, and Ni concentrations in ryegrass were reduced with compost and Cu and Zn concentrations were increased. Ni concentrations in ryegrass were about twice as high in treatment A compared to B, while the reverse was true for Cu and Zn.
Discussion
Our results indicate there is no value with respect to phytomining or phytoextraction in co-cropping A. murale with L. perenne. Neither yield nor Ni uptake was improved; the ryegrass shoots only interfered with the growth of A. murale. The average full-pot yield for A. murale grown alone was 15.0 ± 6.3 g, therefore phytoextraction could be doubled just with Alyssum monoculture. Further, given larger pot sizes or field growth, A. murale and A. corsicum develop extensive root systems than can increase shoot Ni concentration up to five times that achieved in 1 kg pots (Baklanov et al., 2015; Bani et al., 2015a). Therefore, root growth interference from the equally extensive L. perenne root system is almost certainly a negative factor with respect to maximizing Ni phytoextraction. Co-cropping Lupinus albus and A. murale in natural serpentine soils showed similar results in a study investigating whether co-cropping with a nitrogen-fixing plant could improve overall A. murale Ni phytoextraction (Jiang et al., 2015). Without supplemental P fertilization, 90% of the biomass in the pots was L. albus. With P fertilization A. murale increased to 39%, however Ni accumulation in the shoots was significantly reduced compared to monocropping. Overall Ni phytoextraction was maximized in the monocrop with P fertilization.
Co-cropping with ryegrass somewhat reduced Fe and Mn concentrations in A. murale but not to the extent of either increasing Ni uptake or affecting plant nutrition, so the result, while interesting, has a neutral effect on phytoextraction in this soil. The increased variability of the Ni concentration in both A. murale and L. perenne, and increased Mn in L. perenne with A. murale co-cropping may reflect increased rhizosphere mobilization of Ni and Mn by A. murale but in this experiment it did not translate to any tangible benefit. The hypothesized increase in Ni accumulation in response to phytosiderophores secreted by co-cropped grasses clearly did not occur. Our data do not support increased mobilization of Mn by a phytosiderophore mechanism either, but the converse: mobilization of Mn by the Alyssum hyperaccumulator species significantly increased Mn levels in the grass.
A. montanum could not compete with either A. murale or ryegrass and was nearly killed by co-cropping. In field growth it would be unlikely to survive. In contrast to results with Noccaea (Whiting et al., 2001b), there would be no value in utilizing an Alyssum hyperaccumulator to improve the growth of a non-hyperaccumulator; A. montanum yield was strongly reduced by co-cropping, yet it grew well alone in the fertilized serpentine soil.
A murale and A. montanum accumulated about 13 and 24 g Ca kg−1 respectively, consistent with all previous observations in which CaCO3 nodules cover the surface of the trichomes (Krämer et al., 1997; Psaras et al., 2000; Küpper et al., 2001; Kerkeb and Krämer, 2003; Marmiroli et al., 2004; Broadhurst et al., 2004a,b; Broadhurst et al., 2009; McNear et al., 2005; Asemaneh et al., 2006; Tappero et al., 2007). Calcium fertilization was necessary for L. perenne growth in this experiment, however Alyssum hyperaccumulator species native to typically low Ca, low Ca:Mg ratio serpentine soils nonetheless accumulate Ca in the absence of fertilization. A. montanum is not a Ni hyperaccumulator but had twice the Ca of A. murale. but only half the Mn.
Both Ni and Mn concentrations were significantly higher in A. murale than A. montanum or L. perenne. The high variability in the A. murale Mn concentration is typical of the species, which in natural serpentine soils is observed to hyperaccumulate Mn only in some leaves on a given plant. If Mn soil levels are exceedingly high without addition of Ni, Mn is not hyperaccumulated throughout the plant and instead becomes phytotoxic (Broadhurst et al., 2004b, 2009; Tappero et al., 2007). Ni hyperaccumulators are very specific to Ni and to a lesser extent Mn and Co, and do not non-selectively accumulate/hyperaccumulate other transition metals such as Fe, Cr, or Cu. In the case of Cu, despite 3600 mg kg−1 Ni accumulation, A. murale Cu concentrations were only 2 mg kg−1, far below L. perenne, which accumulated typical foliar Cu levels for ryegrass. These observations support a specific relationship between Mn accumulation and Ni hyperaccumulation (Broadhurst et al., 2009; Ghaderian et al., 2015) rather than a general situation for Alyssum species where Mn uptake and storage is related to enhanced Ca uptake to synthesize the unique trichome tissues (McNear and Kupper, 2013).
Although the compost utilized was a standard, mature aged product from USDA Beltsville it was very detrimental to Alyssum growth, most likely due to pathogenic fungi. We have repeatedly observed fungal infections in Alyssum species grown in humid summer greenhouse conditions. Although we grew the plants in the late winter/spring season and in a majority of their native soil, they were nonetheless unable to survive transplant to the manure compost amended soil. Seeding in the pot was tried but the germination rate of A. montanum was below 40% and seedlings that did come up were very weak. Several plants were transplanted to soil B and grown outdoors but also succumbed with the same disease pattern. However, both Alyssum species grew very well alone in the fertilized serpentine soil with no evidence of disease or phytotoxicity.
In a similar recent study, Álvarez-López et al. (2016) grew the hyperaccumulators A. serpyllifolium ssp. lusitanicum, A. serpyllifolium ssp. malacitanum, A. pintodasilvae. and A. bertolonii. in their native serpentine soil with 2.5, 5, and 10 wt% commercial municipal solid waste compost added. These species grow slowly so did not achieve the large shrub size that A. murale can in one season. The lower levels of compost addition significantly increased yield but no further benefits were achieved with 10%. All levels of compost addition reduced extractable Ni; at the 10% level the reduction was 11-fold. Overall yield was lower without compost but with inorganic NPK fertilization, however NPK addition did not affect Ni accumulation. In an Albanian field trial with ultramafic Vertisols, Bani et al. (2015b) found A. murale yield was increased 10-fold with 120 kg NPK and 77 kg Ca ha−1 plus monocot herbicide to control Graminaceae—as opposed to encouraging co-cropping. These agronomic practices increased Ni phytoextraction yield from 2.0 to 29.5 kg ha−1. Thus, in a long-term field Ni phytoextraction or phytomining situation, standard inorganic fertilization may be both adequate and preferable. If a compost source is utilized, it should be tested with every species/ecotype used in the field program prior to application. Another factor to consider is a possible negative effect of compost biota on serpentine-endemic rhizobacteria which can act to facilitate Ni uptake. The two bacteria shown to strongly increase Ni accumulation in A. murale (M. arabinogalactanolyticum and M. oxydans) were isolated from the Oregon soil that we utilized in this study (Abou-Shanab et al., 2003, 2007), thus were potentially present in each pot. They may not have thrived in the compost-amended soil just as the Alyssum species did not, however rhizobiome interactions cannot explain our results.
Ryegrass growth was not negatively affected by the compost and the Ni concentration was significantly reduced without inducing Fe or Mn deficiency. With fertilization and adequate water ryegrass grew reasonably well on the serpentine soil; adding compost would be a significant benefit to retain soil moisture and improve root growth. Ryegrass yield may have increased in treatment B if grass was cut one or two times during the experiment. This was not done because in a field intercropping situation A. murale would need to grow as long as the season permits in order to maximize Ni phytoextraction, and it would not be practicable to selectively cut the ryegrass. Similarly, regular light irrigation and cool, relatively humid conditions to maximize L. perenne yield would not be practicable since A. murale grows better with infrequent but thorough waterings and relatively hot, sunny, low humidity conditions. In commercial phytomining of Ni, weed control to prevent grasses would normally be practiced to limit competition for water and nutrients (Bani et al., 2015b).
Overall, tilling soil to maximize root penetration, adequate inorganic fertilization and appropriate plant densities are more important for developing efficient phytoremediation and phytomining approaches with Alyssum Ni hyperaccumulator species than organic soil amendments or co-cropping.
Author Contributions
CB Principal Experimentalist, analyzed data, and wrote ms. RC Research Group Leader, involved in all experimentation in the laboratory. Co-designed experiment and co-wrote ms.
Funding
RC is a Federal Employee, US Department of Agriculture Agricultural Research Service.
CB is posted at US Department of Agriculture Agricultural Research Service and is a State of Maryland Employee.
Conflict of Interest Statement
The authors declare that the research was conducted in the absence of any commercial or financial relationships that could be construed as a potential conflict of interest.
References
Abou-Shanab, R. A., Angle, J. S., Delorme, T. A., Chaney, R. L., van Berkum, P., Moawad, H., et al. (2003). Rhizobacterial effects on nickel extraction from soil and uptake by Alyssum murale. New Phytol. 158, 219–224. doi: 10.1046/j.1469-8137.2003.00721.x
Abou-Shanab, R. A., van Berkum, P., and Angle, J. S. (2007). Heavy metal resistance and genotypic analysis of metal resistance genes in gram-positive and gram-negative bacteria present in Ni-rich serpentine soil and in the rhizosphere of Alyssum murale. Chemosphere 68, 360–367. doi: 10.1016/j.chemosphere.2006.12.051
Álvarez-López, A., Prieto-Fernández, Á., Cabello-Conejo, M. I., and Kidd, P. S. (2016). Organic amendments for improving biomass production and metal yield of Ni-hyperaccumulating plants. Sci. Total Environ. 548, 370–379. doi: 10.1016/j.scitotenv.2015.12.147
Asemaneh, T., Ghaderian, S. M., Crawford, S. A., Marshall, A. T., and Baker, A. J. M. (2006). Cellular and subcellular compartmentation of Ni in the Eurasian serpentine plants Alyssum bracteatum, Alyssum murale (Brassicaceae) and Cleome heratensis (Capparaceae). Planta 225, 193–202. doi: 10.1007/s00425-006-0340-y
Awad, F., and Römheld, V. (2000). Mobilization of heavy metals from contaminated calcareous soils by plant-born, microbial and synthetic chelators and their uptake by wheat plants. J. Plant Nutr. 23, 1847–1855. doi: 10.1080/01904160009382147
Baker, A. J. M., and Brooks, R. R. (1989). Terrestrial higher plants which hyperaccumulate metallic elements: a review of their distribution, ecology and phytochemistry. Biorecovery 1, 81–126.
Baker, A. J. M., Ernst, W. H. O., Van Der Ent, A., Malaisse, F., and Ginocchio, R. (2010). Metallophytes: the unique biological resource its ecology, and conservational status in Europe, central Africa and Latin America, in Ecology of Industrial Pollution, eds L. C. Batty and K. B. Hallberg (Cambridge, UK: University Press), 7–40. doi: 10.1017/cbo9780511805561.003
Baklanov, I., Ryan, T. C., Chaney, R. L., and Davis, A. P. (2015). Effect of soil volume on yield of and Ni hyperaccumulation by Alyssum corsicum. in Abstracts International Phytotechnologies Society Conference (Manhattan, KS).
Bani, A., Echevarria, G., Sulçe, S., and Morel, J. L. (2015b). Improving the agronomy of Alyssum murale for extensive phytomining: a five-year field study. Int. J. Phytorem. 17, 117–127. doi: 10.1080/15226514.2013.862204
Bani, A., Echevarria, G., Zhang, X., Benizri, E., Laubie, B., Morel, J. L., et al. (2015a). The effect of plant density in nickel-phytomining field experiments with Alyssum murale in Albania. Aust. J. Bot. 63, 72–77. doi: 10.1071/bt14285
Broadhurst, C. L., Chaney, R. L., Angle, J. A., Erbe, E. F., and Maugel, T. K. (2004a). Nickel localization and response to increasing Ni soil levels in leaves of the Ni hyperaccumulator Alyssum murale. Plant Soil 265, 225–242. doi: 10.1007/s11104-005-0974-8
Broadhurst, C. L., Chaney, R. L., Angle, J. A., Maugel, T. K., Erbe, E. F., and Murphy, C. A. (2004b). Simultaneous hyperaccumulation of nickel, manganese and calcium in Alyssum leaf trichomes. Environ. Sci. Technol. 38, 5797–5802. doi: 10.1021/es0493796
Broadhurst, C. L., Tappero, R. V., Maugel, T. K., Erbe, E. F., Sparks, D. L., and Chaney, R. L. (2009). Interaction of nickel and manganese in accumulation and localization in leaves of the Ni hyperaccumulators Alyssum murale and Alyssum corsicum. Plant Soil 314, 35–48. doi: 10.1007/s11104-008-9703-4
Cabello-Conejo, M. I., Becerra-Castro, C., Prieto-Fernández, A., Monterroso, C., Saavedra-Ferro, A., Mench, M., et al. (2014). Rhizobacterial inoculants can improve nickel phytoextraction by the hyperaccumulator Alyssum pintodasilvae. Plant Soil 379, 35–50. doi: 10.1007/s11104-014-2043-7
Centofanti, T., Sayers, Z., Cabello-Conejo, M. I., Kidd, P., Nishizawa, N. K., Kakei, Y., et al. (2013). Xylem exudate composition and root-to-shoot nickel translocation in Alyssum species. Plant Soil 373, 59–75. doi: 10.1007/s11104-013-1782-1
Chaney, R. L., Angle, J. S., Baker, A. J. M., and Li, Y.-M. (1999). Method for Phytomining of Nickel, Cobalt and Other Metals from Soil. US Patent No. 5,944,872 issued Aug. 31, 1999. (Continuation-in-Part of US Patent 5,711,784 issued Jan. 27, 1998).
Chaney, R. L., Broadhurst, C. L., and Centofanti, T. (2010). Phytoremediation of soil trace elements, in Trace Elements in Soil, ed P. S. Hooda ( Chichester, UK: Wiley). doi: 10.1002/9781444319477.ch14
Ghaderian, S. M., Ghasemi, R., and Hajihashemi, F. (2015). Interaction of nickel and manganese in uptake, translocation and accumulation by the nickel-hyperaccumulator plant, Alyssum bracteatum (Brassicaceae). Aust. J. Bot. 63, 47–55. doi: 10.1071/bt14210
Gove, B., Hutchinson, J. J., Young, S. D., Craigon, J., and McGrath, S. P. (2002). Uptake of metals by plants sharing a rhizosphere with the hyperaccumulator Thlaspi caerulescens. Int. J. Phytoremed. 4, 267–281. doi: 10.1080/15226510208500087
Grimold, A. A., Kavanova, M., Lattanzi, F. A., and Schnyder, H. (2005). Phosphorus nutrition-mediated effects of arbuscular mycorrhiza on leaf morphology and carbon allocation in perennial ryegrass. New Phytol. 168, 435–444. doi: 10.1111/j.1469-8137.2005.01500.x
Jiang, C.-A., Wu, Q.-T., Goudon, R., Echevarria, G., and Morel, J.-L. (2015). Biomass and metal yield of co-cropped Alyssum murale and Lupinus albus. Aust. J. Bot. 63, 159–166. doi: 10.1071/bt14261
Jiang, C.-A., Wu, Q.-T., Sterckeman, T., Schwartz, C., Sirguey, C., Ouvrard, S., et al. (2010). Co-planting can phytoextract similar amounts of cadmium and zinc to mono-cropping from contaminated soils. Ecol. Eng. 36, 391–395. doi: 10.1016/j.ecoleng.2009.11.005
Kerkeb, J., and Krämer, U. (2003). The role of free histidine in xylem loading of nickel in Alyssum lesbiacum and Brassica juncea. Plant Physiol. 131, 716–724. doi: 10.1104/pp102.010686
Krämer, U. (2010). Metal hyperaccumulation in plants. Ann. Rev. Plant Biol. 61, 517–534. doi: 10.1146/annurev-arplant-042809-112156
Krämer, U., Grime, G. W., Smith, J. A. C., Hawes, C. R., and Baker, A. J. M. (1997). Micro-PIXE as a technique for studying nickel localization in leaves of the hyperaccumulator Alyssum lesbiacum. Nuclear Inst. Methods Phys. Res. B 130, 346–350. doi: 10.1016/S0168-583X(97)00368-6
Küpper, H., Lombi, E., Zhao, F.-J., Wieshammer, G., and McGrath, S. P. (2001). Cellular compartmentation of nickel in the hyperaccumulators Alyssum lesbiacum, Alyssum bertolonii and Thlaspi goesingense. J. Exp. Bot. 52, 2291–2300. doi: 10.1093/jexbot/52.365.2291
Li, Y.-M., Chaney, R., Brewer, E., Rosenberg, R., Angle, J. S., Baker, A., Reeves, R., et al. (2003b). Development of a technology for commercial phytoextraction of nickel: economic and technical considerations. Plant Soil 249, 107–115. doi: 10.1023/A:1022527330401
Li, Y.-M., Chaney, R. L., Brewer, E., Angle, J. S., and Nelkin, J. (2003a). Phytoextraction of nickel and cobalt by hyperaccumulator Alyssum species grown on nickel-contaminated soils. Environ. Sci. Technol. 37, 1463–1468. doi: 10.1021/es0208963
Marmiroli, M., Gonelli, C., Maestri, E., Gabrielli, R., and Marmiroli, N. (2004). Localisation of nickel and mineral nutrients Ca, K, Fe, Mg by scanning electron microscopy microanalysis in tissues of the nickel-hyperaccumulator Alyssum montanum L. Plant Biosyst. 138, 231–243. doi: 10.1080/11263500400011126
Marschner, H., and Römheld, V. (1994). Strategies of plants for acquisition of iron. Plant Soil 165:261–274. doi: 10.1007/BF00008069
McNear, D. H., Chaney, R. L., and Sparks, D. L. (2010). The hyperaccumulator Alyssum murale uses complexation with nitrogen and oxygen donor ligands for Ni transport and storage. Phytochemistry 71, 188–200. doi: 10.1016/j.phytochem.2009.10.023
McNear, D. H., and Kupper, J. V. (2013). Mechanisms of trichome-specific Mn accumulation and toxicity in the Ni hyperaccumulator Alyssum murale. Planta 227, 1363–1375. doi: 10.1007/s11104-013-2003-7
McNear, D. Jr., Peltier, E., Everhart, J., Chaney, R. L., Newville, M., Rivers, M., et al. (2005). Application of quantitative fluorescence and absorption-edge computed microtomography to image metal compartmentalization in Alyssum murale. Environ. Sci. Technol. 39, 2210–2218. doi: 10.1021/es0492034
Nkrumah, P. N., Baker, A. J. M., Chaney, R. L., Erskine, P. D., Guillame, E., Morel, J.-L., et al. (2016). Current status and challenges in developing nickel phytomining: an agronomic perspective. Environ. Pollut. doi: 10.1007/s11104-016-2859-4
Psaras, G. K., Constantinidis, T. H., Cotsopoulos, B., and Maneta, Y. (2000). Relative abundance of nickel in the leaf epidermis of eight hyperaccumulators: evidence that the metal is excluded from both guard cells and trichomes. Ann. Bot. 86, 73–78. doi: 10.1006/anbo.2000.1161
Rajkumar, M., Ma, Y., and Freitas, H. (2013). Improvement of Ni phytostabilization by inoculation of Ni resistant Bacillus megaterium SR28C. J. Environ. Manage. 128, 973–980. doi: 10.1016/j.jenvman.2013.07.001
Rajkumar, M., Vara Prasad, M. N., Freitas, H., and Ae, N. (2009). Biotechnological applications of serpentine soil bacteria for phytoremediation of trace metals. Crit. Rev. Biotechnol. 29, 120–130. doi: 10.1080/07388550902913772
Reeves, R. J., and Adigüzel, N. (2008). The nickel hyperaccumulating plants of the serpentines of Turkey and adjacent areas: a review with new data. Turk. J. Biol. 32, 143–153.
Sterckeman, T., Duquene, L., Perriguey, J., and Morel, J.-L. (2005). Quantifying the effect of rhizosphere processes on the availability of soil cadmium and zinc. Plant Soil 276, 335–345. doi: 10.1007/s11104-005-5087-x
Tappero, R., Peltier, E., Grafe, M., Heidel, K., Ginder-Vogel, M., Livi, K. J. T., et al. (2007). Hyperaccumulator Alyssum murale relies on a different metal storage mechanism for cobalt than for nickel. New Phytol. 175, 641–654. doi: 10.1111/j.1469-8137.2007.02134.x
van der Ent, A., Baker, A. J. M., Reeves, R. D., and Chaney, R. L. (2015). Agromining: farming for metals in the future? Environ. Sci. Technol. 49, 4773–4780. doi: 10.1021/es506031u
van der Ent, A., Baker, A. J. M., Reeves, R. D., Pollard, A. J., and Schat, H. (2013). Hyperaccumulators of metal and metalloidtrace elements: facts and fiction. Plant Soil 362, 319–334. doi: 10.1007/s11104-012-1287-3
Visioli, G., D'Egidio, S., and Sanangelantoni, A. M. (2015). The bacterial rhizobiome of hyperaccumulators: future perspectives based on omics analysis and advanced microscopy. Front. Plant Sci. 5:752. doi: 10.3389/fpls.2014.00752
Wang, A. S., Angle, J. S., Chaney, R. L., and Delorme, T. A. (2006). Soil pH effects on uptake of Cd and Zn by Thlaspi caerulescens. Plant Soil 281, 325–337. doi: 10.1007/s11104-005-4642-9
Wang, K., Huang, H., Zhu, Z., Li, T., He, Z., Yang, X., et al. (2013). Phytoextraction of metals and rhizoremediation of PAHs in co-contaminated soil by co-planting of Sedum alfredii with ryegrass (Lolium perenne) or castor (Ricinus communis). Int. J. Phytorem. 15, 283–298. doi: 10.1080/15226514.2012.694501
Whiting, S. N., Leake, J. R., McGrath, S. P., and Baker, A. J. M. (2001a). Assessment of Zn mobilization in the rhizosphere of Thlaspi caerulescens by bioassay with non-accumulator plants and soil extraction. Plant Soil 237, 147–156. doi: 10.1023/A:1013365617841
Whiting, S. N., Leake, J. R., McGrath, S. P., and Baker, A. J. M. (2001b). Hyperaccumulation of Zn by Thlaspi caerulescens can ameliorate Zn toxicity in the rhizosphere of cocropped Thlaspi arvense. Environ. Sci. Technol. 35, 3237–3241. doi: 10.1021/es010644m
Wu, Q. T., Wei, Z. B., and Ouyang, Y. (2007). Phytoextraction of metal-contaminated soil by Sedum alfredii H: effects of chelator and co-planting. Water Air Soil Pollut. 180, 131–139. doi: 10.1007/s11270-006-9256-1
Zhang, F.-S., Römheld, V., and Marschner, H. (1991a). Diurnal rhythm of release of phytosiderophores and uptake rate of zinc in iron-deficient wheat. Soil Sci. Plant Nutr. 37, 671–678. doi: 10.1080/00380768.1991.10416935
Zhang, F.-S., Treeby, M., Römheld, V., and Marschner, H. (1991b). Mobilization of iron by phytosiderophores as affected by other nutrients. Plant Soil 130, 173–178. doi: 10.1007/BF00011872
Zhang, L., Angle, J. S., and Chaney, R. L. (2007). Do high-nickel leaves shed by the Ni-hyperaccumulator Alyssum murale inhibit seed germination of competing plants? New Phytol. 173, 509–516. doi: 10.1111/j.1469-8137.2006.01952.x
Zhao, F. J., Harmon, R. E., and McLaughlin, M. J. (2001). Root exudates of the hyperaccumulator Thlaspi caerulescens do not enhance metal mobilization. New Phytol. 151, 613–620. doi: 10.1046/j.0028-646x.2001.00213.x
Keywords: Alyssum murale, Lolium perenne, nickel hyperaccumulators, ryegrass, co-cropping, phytoremediation, phytomining
Citation: Broadhurst CL and Chaney RL (2016) Growth and Metal Accumulation of an Alyssum murale Nickel Hyperaccumulator Ecotype Co-cropped with Alyssum montanum and Perennial Ryegrass in Serpentine Soil. Front. Plant Sci. 7:451. doi: 10.3389/fpls.2016.00451
Received: 21 December 2015; Accepted: 22 March 2016;
Published: 08 April 2016.
Edited by:
Sarvajeet Singh Gill, Maharshi Dayanand University, IndiaReviewed by:
Mirza Hasanuzzaman, Sher-e-Bangla Agricultural University, BangladeshNarsingh Chauhan, Maharshi Dayanand University, India
Copyright © 2016 Broadhurst and Chaney. This is an open-access article distributed under the terms of the Creative Commons Attribution License (CC BY). The use, distribution or reproduction in other forums is permitted, provided the original author(s) or licensor are credited and that the original publication in this journal is cited, in accordance with accepted academic practice. No use, distribution or reproduction is permitted which does not comply with these terms.
*Correspondence: Catherine L. Broadhurst, bGVpZ2guYnJvYWRodXJzdEBhcnMudXNkYS5nb3Y=