- 1Botany Department, Faculty of Science, South Valley University, Qena, Egypt
- 2Biology Department, College of Applied Medical Science, Taif University, Taif, Saudi Arabia
- 3Plant Abiotic Stress Research Group & Faculty of Applied Sciences, Ton Duc Thang University, Ho Chi Minh City, Vietnam
- 4Signaling Pathway Research Unit, RIKEN Center for Sustainable Resource Science, Yokohama, Japan
Silicon (Si) has been known to augment plant defense against biotic and abiotic pressures. Maize (Zea maize L.) is classified as a Si accumulator and is relatively susceptible to alkaline stress. In this study, seeds of maize were grown in pots and exposed to various concentrations of Na2CO3 (0, 25, 50, and 75 mM) with or without 1.5 mM Si in the form of sodium metasilicate Na2O3Si.5H2O for 25 days. Alkaline-stressed plants showed a decrease in growth parameters, leaf relative water content (LRWC), and the contents of photosynthetic pigments, soluble sugars, total phenols and potassium ion (K+), as well as potassium/sodium ion (K+/Na+) ratio. By contrast, alkaline stress increased the contents of soluble proteins, total free amino acids, proline, Na+ and malondialdehyde (MDA), as well as the activities of superoxide dismutase (SOD), catalase (CAT), and peroxidase (POD) in stressed plants. On the other hand, application of Si by seed-priming improved growth of stressed plants, which was accompanied by the enhancement in LRWC, and levels of photosynthetic pigments, soluble sugars, soluble proteins, total free amino acids and K+, as well as activities of SOD, CAT, and POD enzymes. Furthermore, Si supplement resulted in a decrease in the contents of proline, MDA and Na+, which together with enhanced K+ level led to a favorable adjustment of K+/Na+ ratio, in stressed plants relative to plants treated with alkaline stress alone. Taken together, these results indicate that Si plays a pivotal role in alleviating the negative effects of alkaline stress on maize growth by improving water status, enhancing photosynthetic pigments, accumulating osmoprotectants rather than proline, activating the antioxidant machinery, and maintaining the balance of K+/Na+ ratio. Thus, our findings demonstrate that seed-priming with Si is an efficient strategy that can be used to boost tolerance of maize plants to alkaline stress.
Introduction
Alkaline stress, which is defined as the existence of alkaline salts (Na2CO3 or NaHCO3) in the soil (Paz et al., 2012), is one of the most crucial abiotic stressors which plants encounter in the era of climate change. A number of studies have shown that alkaline stress is more dangerous than saline stress, owing to its additional high pH stress (Campbell and Nishio, 2000; Hartung et al., 2002; Chen et al., 2012; Radi et al., 2012). High pH value may lead to reduction in seed germination, destruction of the root cell structure, change in the nutrient availability and disorder in nutrient uptake, and thus resulting in a significant decrease in the yield of agricultural plants (Peng et al., 2008; Gao et al., 2014). A few studies have been carried out on the effects of alkaline stress on plant growth and productivity. However, only scant information is available about the morphological, physiological, biochemical, and antioxidative responses in plants under alkaline stress. Egyptian soils are in general distinguished by a little alkaline to alkaline pH values (7.5–8.7) which are mainly due to its arid ambience (Abd-Alla et al., 2014).
Priming of seeds with silicon (Si) is one of the major techniques, which can improve abiotic stress tolerance in plants (Hameed et al., 2013). Si is the second most abundant element found in soil, next to oxygen, and the eighth most common element in nature (Sahebi et al., 2015). It is recognized as ‘quasi essential’ element for plants because its deficiency results in various dysfunctions with respect to plant growth, evolution, and proliferation (Hasanuzzaman et al., 2013). Si, as a fertilizer, biostimulant or plant protectant, plays a pivotal role in enhancing the plants growth and productivity, especially in stress regimes (Sawas and Ntatsi, 2015). Over the last two decades, numerous laboratory, greenhouse and field experiments have demonstrated that Si is able to hamper both biotic pressures caused by plant diseases and pest attacks, as well as abiotic pressures, including physical pressures such as drought, waterlogging, freezing, high temperature, and UV, and chemical pressures as salinity, nutrient deficiencies, and metal toxicity (for reviews, see Guntzer et al., 2012; Balakhnina and Borkowska, 2013; Marafon and Endres, 2013; Van Bockhaven et al., 2013; Zhu and Gong, 2014; Rizwan et al., 2015).
Maize (Zea mays L.) is the third most cultivated cereal crop in total world production after wheat and rice (Malčovská et al., 2014a). Maize is one of the important crops in Egypt due to its significance as a feed crop, being used in a number of foods and in oil, starch and pharmaceutical industries, as well as newly emerging as a biofuel crop (Ali et al., 2015). Unluckily, in many regions, especially in the tropics and sub-tropics, the productivity of maize is markedly reduced due to alkalinity. Maize has been known as a Si accumulator (Liang et al., 2006); and thus it is a popular crop for studies on the useful impacts of Si under environmental pressures (Malčovská, 2014b).
Despite application of Si has been investigated in different plants grown under various abiotic stresses, literature has not yet been available regarding the strategies of the Si-mediated protective effects under alkaline stress. Thus, in the present study, we attempted to explain the impacts of Si on growth and tolerance of maize plants subjected to alkaline stress. Therefore, we designed a series of experiments to examine the possible promotion effects of Si pre-treatment on growth traits, the contents of photosynthetic pigments, osmoprotectants, total phenols, sodium ion (Na+), potassium ion (K+), and malondialdehyde (MDA), as well as the activities of antioxidant enzymes of maize seedlings grown in soil irrigated with different concentrations of alkaline salt.
Materials and Methods
Plant Growth and Treatments
This experiment was conducted in the wire-house of the experimental farm of South Valley University, Qena, Egypt located at latitude 26°11′ 25′′ N and longitude 32° 44′ 42′′ E. Plants were grown under natural conditions of temperature, light, and humidity during growing season 2015 (from May 15th to June 8th). Seeds of maize (Zea maize L. cv. single hybrid 10) were surface-sterilized with mercuric chloride (0.1%) for 5 min, and then rinsed three times with distilled water. The sterilized seeds were divided into two groups; the first group was primed with distilled water, and the second group with 1.5 mM of freshly prepared Si (as sodium metasilicate Na2O3Si.5H2O) solution for 12 h, thereafter air-dried. The seeds of both groups were sown in plastic pots (five seeds/pot) filled with 2 kg of dried soil. The pots were arranged in completely randomized design in factorial arrangement with three replications. At the time of sowing, the seeds were irrigated at field capacity with various alkaline salt concentrations of 0 (control), 25, 50, and 75 mM Na2CO3 based on the method of Radi et al. (2012); with each pot receiving 400 ml of a designated saline solution. The Na2CO3 concentrations used were equivalent to 0 (control), 0.528, 1.056, and 1.584 g Na2CO3 kg-1 soil, respectively. Leaching was avoided by maintaining soil water below field capacity at all times. The Si and Na2CO3 concentrations were selected based on our preliminary tests. The pots were then irrigated at field capacity with normal water through the whole experimental period (25 days). After 25 days after sowing the maize plants were harvested for further analyses.
Growth Criteria
The fresh weight of maize seedlings was estimated after harvesting. The harvested seedlings were washed with deionized water, and blotted on paper towels before being weighed. Their dry weight was estimated after oven drying at 80°C to constant weight. The dried tissues were powderized and stored in clean sealed glasses at room temperature for later analysis. Leaf relative water content (LRWC) was determined using the method described in Garíca-Mata and Lamattina (2001), using the equation: RWC (%) = (Fresh weight – Dry weight/Turgid weight – Dry weight) × 100. Leaf area was measured using a Planimeter (SOKKIA PLANIMELER KP-90 UK).
Photosynthetic Pigments
The contents of chlorophyll (Chl) a and b, and carotenoids in fresh leaves were assessed spectrophotometrically according to Lichtenthaler and Wellburn (1983). The 0.05 g of fully expanded young leaves of 25-day-old plants were used for pigment extraction in 80% acetone. The extract of pigments was measured versus a blank of pure 80% acetone at 663, 644, and 452.5 nm for Chl a, Chl b, and carotenoid contents, respectively.
Estimation of Osmoprotectant Contents
Total soluble sugar content in 25-day-old plants was determined by the anthrone sulfuric acid method according to Irigoyen et al. (1992). Total soluble protein content in 25-day-old plants was determined according to Bradford (1976). The contents of total free amino acids of 25-day-old plants tissues were determined according to the method of Lee and Takanashi (1966). The content of proline in 25-day-old plants was measured according to the method described by Bates et al. (1973).
Estimation of Total Phenol Content
Total phenol content in 25-day-old plants was assayed by Folin–Ciocalteu reagent (Skerget et al., 2005). The 2.5 mL of Folin–Ciocalteu reagent (diluted 10 times with water) and 2 mL of sodium carbonate (7.5% w/v) solution were added to 0.5 mL of sample extract (0.25 mg mL-1) in water. After 20 min of incubation at room temperature, the absorbance was measured spectrophotometrically at 760 nm. Total phenols were quantified by calibration curve obtained from measuring the known concentrations of standard gallic acid (0–100 μg mL-1). The phenolic contents of the samples were expressed as mg of GAE (gallic acid equivalent) g-1 dry weight of sample.
Estimation of Na+ and K+ Levels
Dried powder samples (0.1 g) were treated with perchloric acid 80% (2 mL) and concentrated H2SO4 (10 mL) for 12 h. Each digested material was then diluted with distilled water to a definite volume (100 mL). The contents of Na+ and K+ were determined by flame photometry according to the method described by Williams and Twine (1960).
Determination of MDA Content
Malondialdehyde content was determined according to the thiobarbituric acid (TBA) reaction as described by Zhang and Qu (2004). Fresh leaf sample (0.5 g) was homogenized with 5% trichloroacetic acid and centrifuged at 4,000 × g for 10 min. Two milliliter of extract was mixed with 2 mL of 0.6% TBA, and the mixture was placed in a boiling water bath for 10 min. Subsequently, the absorbances were read at 532, 600, and 450 nm. The MDA content was calculated using the formula: 6.45 × (A532 - A600) - 0.56 × A450.
Assays for Antioxidant Enzyme Activities
Extraction of Enzymes
Samples were extracted from the fresh leaves based on the method of Mukherjee and Choudhari (1983). The fresh leaves (0.5 g) were frozen in liquid nitrogen and ground in 10 mL of 100 mM phosphate buffer (KH2PO4/K2HPO4) pH 7.0, containing 0.1 mM Na2EDTA and 0.1 g of polyvinylpyrrolidone (PVP). The homogenate was centrifuged at 15,000 × g at 4°C for 10 min. Subsequently, the supernatant was stored at 4°C until being used for superoxide dismutase (SOD), catalase (CAT), and peroxidase (POD) assays.
Assay for SOD Activity
The activity of SOD (EC 1.15.1.1) was determined using the nitro blue tetrazolium (NBT) method described by Giannopolitis and Ries (1977). One unit of SOD was defined as the amount of enzyme required to cause 50% inhibition of the reduction of NBT as monitored at 560 nm.
Assay for CAT Activity
Catalase (EC 1.11.1.6) activity was measured according to the method previously described (Aebi, 1984). The CAT activity was assayed by the decline of absorbance at 240 nm as a consequence of H2O2 consumption.
Assay for POD Activity
Peroxidase (EC 1.11.1.7) activity was determined using the method of Maehly and Chance (1954) by assessing the oxidation rate of guaiacol in the presence of H2O2 at 470 nm (Klapheck et al., 1990).
Statistical Analysis
All data shown are the mean values. Data were statistically analyzed by the analysis of variance (ANOVA) with SAS software (Version 9.1; SAS Institute, Cary, NC, USA) using Duncan’s multiple range test at the 0.05 level of significance (p < 0.05). Data represented in the Tables and Figures are means ± standard deviation (SD) of three independent replicates of each treatment.
Results
Positive Impacts of Si on Maize Growth Parameters
Data shown in Table 1 indicated that the values of fresh and dry weights, LRWC, and leaf area of the Na2CO3-stressed seedlings showed an appreciable decrease with the rise of Na2CO3 concentration. The maximum reduction in these growth parameters was recorded at the highest alkalinity level (75 mM Na2CO3) relative to untreated control plants (Table 1). Priming seeds with Si relieved the injurious impacts of alkaline stress on all the growth parameters examined, especially at the highest alkalinity level, at which the maximum recover was noted, as compared with stressed alone plants (Table 1). Pre-treatment of seeds with Si alone significantly increased the fresh weight, dry weight and leaf area; however, it did not significantly affect the LRWC compared with untreated control group (Table 1).
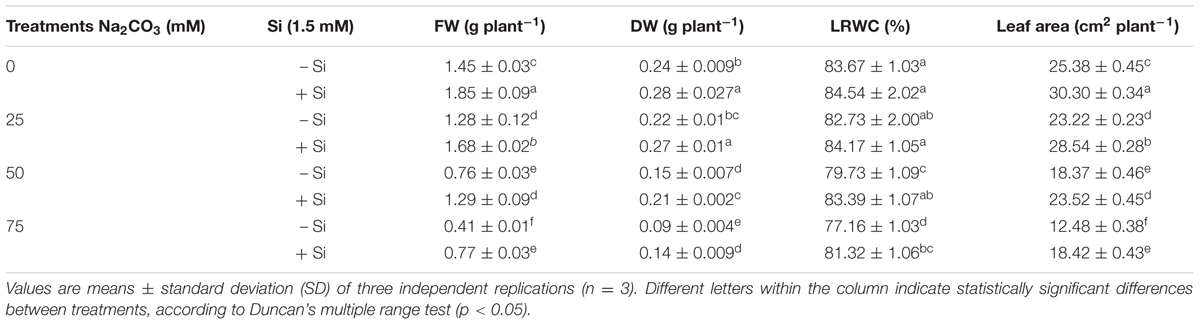
TABLE 1. Effects of different levels of alkaline stress with and without seed-priming with silicon (Si) on fresh weight (FW), dry weight (DW), leaf relative water content (LRWC), and leaf area of 25-day-old maize plants.
Priming with Si Improves the Contents of Photosynthetic Pigments
Results presented in Figure 1 indicated that the increase in Na2CO3 concentration in the soil was associated with progressive fall in photosynthetic pigment biosynthesis in maize leaves relative to untreated control. On the other hand, the lowest level of Na2CO3 (25 mM) increased the biosynthesis of carotenoids compared with the untreated control. Priming maize seeds with Si amended the inhibitory effects of alkaline stress on pigment contents, resulting in significant enhancement of pigment contents in comparison with stressed alone plants (Figure 1). Under non-stressed regimes, the levels of Chl a and Chl b remained relatively unchanged but that of carotenoids increased by 40.74% in plants treated with Si alone versus untreated control (Figure 1).
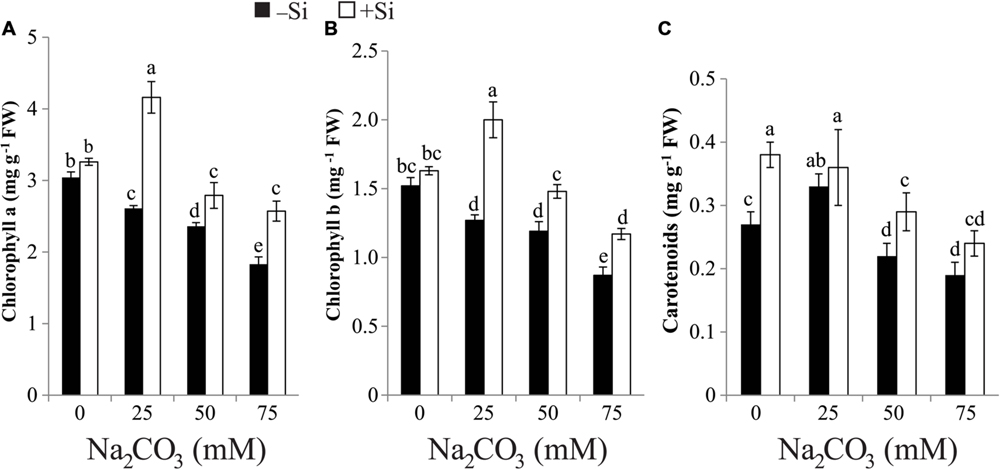
FIGURE 1. Effects of different levels of alkaline stress with and without seed-priming with silicon (Si) on the contents of (A) chlorophyll a, (B) chlorophyll b and (C) carotenoids in leaves of 25-day-old maize plants. Bars represent standard deviation (SD) of the means (n = 3). Different letters indicate significant differences among the treatments at P < 0.05, according to Duncan’s multiple range test. FW, fresh weight.
Si Increases the Levels of Soluble Sugars, Soluble Proteins, Total Phenols, and Total Free Amino Acids, While Restrains Proline Accumulation
The effects of alkaline stress on the contents of osmoprotectants and antioxidants varied. Soluble sugar content in maize plants was not significantly affected by Na2CO3 treatment at 25 mM, while it significantly decreased at 50 and 75 mM Na2CO3, compared with untreated control (Table 2). Although both soluble protein and total phenol contents remained unchanged up to 50 mM of Na2CO3, soluble protein content increased at 75 mM Na2CO3, whereas total phenol content progressively reduced at 75 mM Na2CO3 in comparison with untreated control (Table 2). Total free amino acid content slightly increased at 25 mM of Na2CO3, thereafter a constant increment in this content was recorded at 50 and 75 mM Na2CO3 compared with untreated control (Table 2). Alkaline stress induced a dramatic accumulation of proline, and this accumulation reached the maximum value at 75 mM Na2CO3 versus untreated control (Table 2).
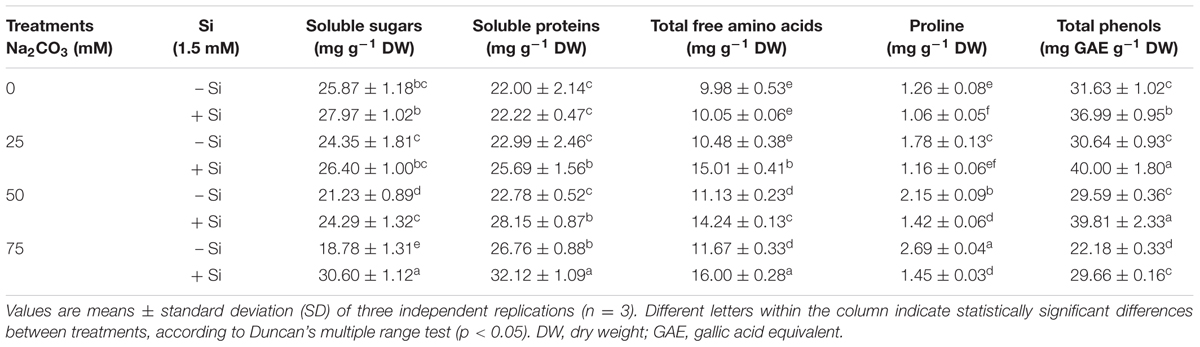
TABLE 2. Effects of different levels of alkaline stress with and without seed-priming with silicon (Si) on the contents of soluble sugars, soluble proteins, total free amino acids, proline, and total phenols in 25-day-old maize plants.
Application of Si alleviated the adverse effects of alkaline stress on soluble sugar accumulation, especially at 75 mM Na2CO3 compared with stressed alone plants (Table 2). Supplementation with Si as seed-priming provoked the accumulation of soluble proteins, total phenols, and total free amino acids. The maximum increase in soluble protein and total phenol contents was noted at 50 mM Na2CO3, while that of total free amino acid content was observed at 25 mM Na2CO3 in maize plants treated with both Na2CO3 and Si, when compared with the stressed alone plants (Table 2). In contrary, application of Si in terms of seed-priming restrained the accumulation of proline in maize plants treated with both Na2CO3 and Si as compared with respective plants treated with Na2CO3 alone (Table 2). It is worth mentioning that under non-stressed conditions, only total phenol content was significantly affected by Si treatment, with an increase of 16.94% in comparison with untreated control (Table 2).
Si Attenuates Na+ Toxicity and Modulates K+ Homeostasis and K+/Na+ Ratio
The concentration of Na+ positively correlated with the increase of alkaline salt and recorded its highest value (twofold) at the severest alkalinity compared with control (Figure 2A). On the other hand, the reverse trend was noticed with K+ content and the highest reduction in K+ content was recorded at the highest level of Na2CO3 relative to that of control (Figure 2B). As a consequence, K+/Na+ ratio was reduced by increasing the concentration of Na2CO3 (Figure 2C). Si supplementation in the form of seed-priming resulted in significant repression in the accumulation of Na+ at all Na2CO3 concentrations. Furthermore, Si application increased the content of K+ and K+/Na+ ratio in stressed maize plants, with the maximum increase being observed at 75 mM Na2CO3 compared with the corresponding stressed alone plants (Figures 2A–C).
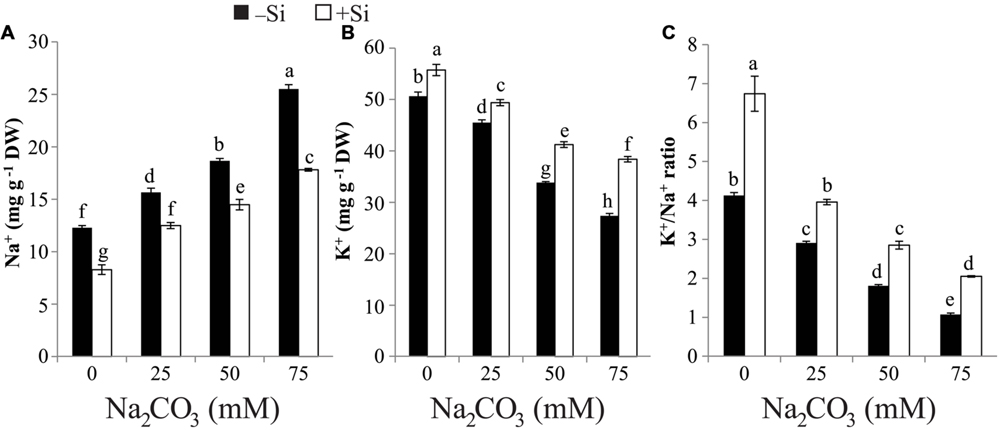
FIGURE 2. Effects of different levels of alkaline stress with and without seed-priming with silicon (Si) on (A) Na+ content, (B) K+ content and (C) K+/Na+ ratio in 25-day-old maize plants. Bars represent standard deviation (SD) of the means (n = 3). Different letters indicate significant differences among the treatments at P < 0.05, according to Duncan’s multiple range test. DW, dry weight.
Si Mitigates Na2CO3-Induced Oxidative Damage
The rise in alkalinity induced a significant accumulation in MDA content in stressed maize plants even at the lowest level of Na2CO3. The maximum accumulation in MDA content was attained at the highest level of alkalinity compared with untreated control plants (Figure 3A). Seed-priming with Si resulted in reduction in MDA content in Si-primed maize plants treated with any level of Na2CO3 relative to respective unprimed plants treated with Na2CO3 alone (Figure 3A).
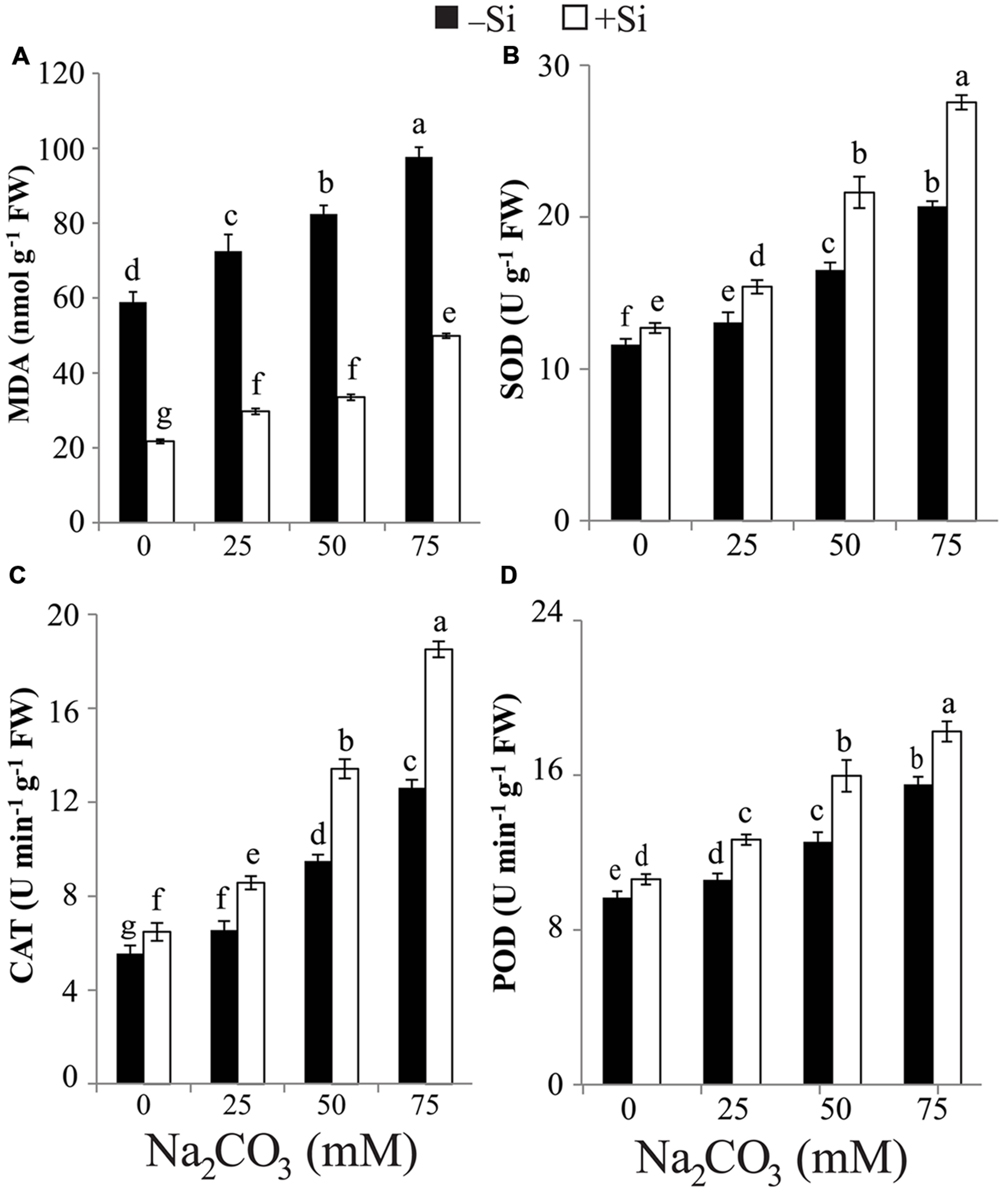
FIGURE 3. Effects of different levels of alkaline stress with and without seed-priming with silicon (Si) on (A) the content of malondialdehyde (MDA) and the activities of (B) superoxide dismutase (SOD), (C) catalase (CAT) and (D) peroxidase (POD) in leaves of 25-day-old maize plants. Bars represent standard deviation (SD) of the means (n = 3). Different letters indicate significant differences among the treatments at P < 0.05, according to Duncan’s multiple range test. FW, fresh weight.
Si Increases the Activities of SOD, CAT, and POD to Mitigate Oxidative Stress
In comparison with control, the activities of SOD, CAT, and POD significantly increased in stressed maize plants with increasing levels of alkaline salt (Figures 3B–D). The highest increment in SOD, CAT, and POD activities was recorded in stressed alone maize plants at 75 mM Na2CO3 compared with untreated control plants. Si application induced further increase in the activities of SOD, CAT, and POD in Si-primed and stressed maize plants relative to stressed alone plants (Figures 3B–D). The maximum increase in the activities of SOD and CAT in Si + Na2CO3-treated plants was observed at 75 mM Na2CO3 (Figures 3B,C), while that of POD was recorded at 50 mM Na2CO3 (Figure 3D). Under non-stressed conditions, Si treatment also enhanced the activities of SOD, CAT, and POD in Si-treated plants versus untreated control (Figures 3B–D).
Discussion
In this study, the impacts of Si as an agriculturally effective fertilizer element on mitigation of alkalinity pressure were studied in maize. The detrimental influences caused by alkaline salt on different growth parameters of maize plants could occur due to the raise in pH, reduction in cell enlargement and cell division, metabolic disorders, nutritional damage, and ion imbalance (Table 1) (Radi et al., 2012; Abd-Alla et al., 2014; Mohsenian and Roosta, 2015). The improvement of LRWC in stressed maize plants observed under Si treatment was perhaps due to the deposition of Si as silicate crystals in epidermal tissues, which composes a barrier to water transpiration through the cuticles and stomata (Habibi, 2015; Karmollachaab and Gharineh, 2015), resulting in higher leaf area of maize plants as recorded in Si-primed plants under non-stressed and alkaline stress conditions (Table 1). Furthermore, Si pre-treatment as seed-priming improved other growth parameters of alkaline-stressed maize seedlings (Table 1), strongly demonstrating the ameliorating action of Si in minimizing alkaline stress. Thus, the results of this study and previously published reports collectively indicate the protecting role of Si against a wide range of environmental pressures (Farooq et al., 2013; Habibi and Hajiboland, 2013; Tripathi et al., 2013; Shen et al., 2014).
The depression in photosynthetic pigments of maize leaves during Na2CO3 treatment found in this study (Figure 1) was supported by the findings of Radi et al. (2012). The decrease in Chl contents under alkaline stress might be due to (i) Mg2+ precipitation that results in the degradation of green pigments (Shi and Zhao, 1997), (ii) enhanced oxidative stress that causes injury to chloroplast structure, and (iii) increase in the activity of chlorophyllase enzyme that is responsible for the Chl degradation (Kariola et al., 2005; Abdel Latef and Chaoxing, 2011; Abdel Latef et al., 2014; Mostofa et al., 2015). Additionally, alkaline stress decreased the content of carotenoids in stressed maize plants (Figure 1C), which act as effective scavengers of free radicals provoked by reactive oxygen species (ROS; Gururani et al., 2015). It is worth noting that, the increase of carotenoid content observed by 25 mM Na2CO3 treatment in stressed maize plants could improve the amplitudes of this compound to diminish the damage caused by ROS under low level of alkaline salt. Si augmented the Chl and carotenoid contents in maize plants exposed to alkaline stress, which could be resulted from the increase in leaf area, leading to the increase in green pigments per unit area and safeguarding of Chl pigments from ROS by reinforcing the level of carotenoids (Figure 1; Table 1).
Importantly, Si-induced restoration of the levels of LRWC and photosynthetic pigments in the alkaline-stressed maize seedlings demonstrated an osmoprotective and membrane-protecting role of Si for maize seedlings subjected to alkaline stress (Figure 1; Table 1). The decrease in both soluble sugar and Chl contents under alkaline stress provides a reason to believe that low Chl content causes a relevant reduction of light absorption by leaves, and subsequently reduces the soluble sugar content (Figure 1; Table 2) (Hamdia et al., 2004; Hamdia and Shaddad, 2010). The amelioration in osmoregulation potential in terms of increasing soluble sugar content by Si could be highly associated with enhanced photosynthetic activity and better growth under alkaline conditions (Figure 1; Tables 1 and 2). This finding suggests that pre-treatment of maize seeds with Si might enhance the catabolism of starch to soluble sugars under alkaline stress.
The accumulation of soluble proteins in maize plants under high alkaline pressure may supply a storage form of nitrogen that is reutilized when stress is over (Abdel Latef, 2010; Abdel Latef and Chaoxing, 2014; Zhang et al., 2014). The increase in soluble protein content of maize under the highest alkaline level applied was accompanied by a marked reduction in growth (Tables 1 and 2). This finding suggests that under alkaline stress, maize plants divert most of the synthesized proteins from being used for growth to being used for osmoregulation to survive stress. It is noteworthy that the high soluble protein content observed at 75 mM Na2CO3 was not resulted from reduction of total free amino acid and proline contents (Table 2). Thus, the strategy that maize plants use to cope with alkaline stress could be the enhancement of nitrogen metabolism. The increase in soluble protein content in response to Si application may be due to the facts that (i) Si has a pivotal role in binding amino acids to form specific proteins (Soundararajan et al., 2014), and (ii) Si is actively engaged in formation of DNA and functioning of mRNA (Abbas et al., 2015). In addition, seed-priming with Si reduced the accumulation of proline content in the alkaline-stressed seedlings, which is associated with improved growth of maize plants under alkaline stress (Tables 1 and 2). This result suggested that seed-priming with Si could provide protection to cells by keeping the accumulation of proline to an optimum level, and Si probably employed other osmoprotectants for stress mitigation, in which high level of proline accumulation was not required.
In the present study, increase of alkaline stress provoked an increment in the Na+ content and a depression in the K+ content, resulting in reduction in K+/Na+ ratio (Figures 2A–C). The decrease in K+ uptake under alkaline stress may be due to the repressive influence of the stress on the absorption of this cation and competition of Na+ ion with K+ ion for binding sites needful for various cellular functions (Azooz et al., 2015). Si-primed maize plants significantly decreased Na+ concentration, while improved the K+ concentration, and thus increasing the K+/Na+ ratio (Figure 2). This reduction in Na+ accumulation might be due to the deposition of silicate in the exodermis and endodermis of maize roots, which leads to an obstruction of the apoplastic Na+ absorption by roots under alkaline stress (Wang et al., 2015). The possible reason for the increased K+ uptake could be the promotion effect of Si on the plasma membrane H+-ATPases in the roots, as found in wheat under water deficit (Karmollachaab and Gharineh, 2015). The high K+/Na+ ratio is a good sign on the balancing impacts of Si on K+ and Na+ uptakes under alkaline stress, encouraging the application of Si as seed-priming for plants grown on alkaline soils.
Under alkaline regimes, MDA content accumulated in maize plants (Figure 3A), clearly suggesting ROS burst and prospective oxidative damage to plant cells. This result is supported by the study of Ahmad et al. (2014), who found an increase in MDA content in two mulberry (Morus alba L.) cultivars with increase in exogenous NaHCO3 level. On the other hand, treatment with Si significantly hampered MDA accumulation in stressed maize plants compared with that of control and Na2CO3- treated alone plants (Figure 3A), suggesting that Si triggers mechanisms to mitigate oxidative damage in stressed plants. Indeed, Si pre-treatment significantly increased the content of antioxidant phenols in maize plants under alkaline stress (Table 2). Furthermore, seed-priming with Si also resulted in a significant increase in SOD, CAT, and POD activities in stressed maize plants relative to plants treated with Na2CO3 alone (Figures 3B–D). These results indicate that Si enhances antioxidant system to protect plants against alkalinity-induced oxidative damage, as evidenced by the observed reduced MDA level (Figure 3A).
In view of all the above observations, a schematic diagram was delineated in Figure 4, which represents the possible physiological and biochemical strategies involving alkalinity-induced stress and the Si-mediated alkaline stress tolerance in maize plants. To our knowledge, this is the first report dealing with the effects of Si on growth parameters, antioxidant activities and selected physiological and biochemical characteristics of maize plants under alkaline stress. Our results demonstrate the potential application of Si in dealing with alkaline stress. Further efforts are required to gain a full understanding of how Si amends the alkaline stress responses in plants.
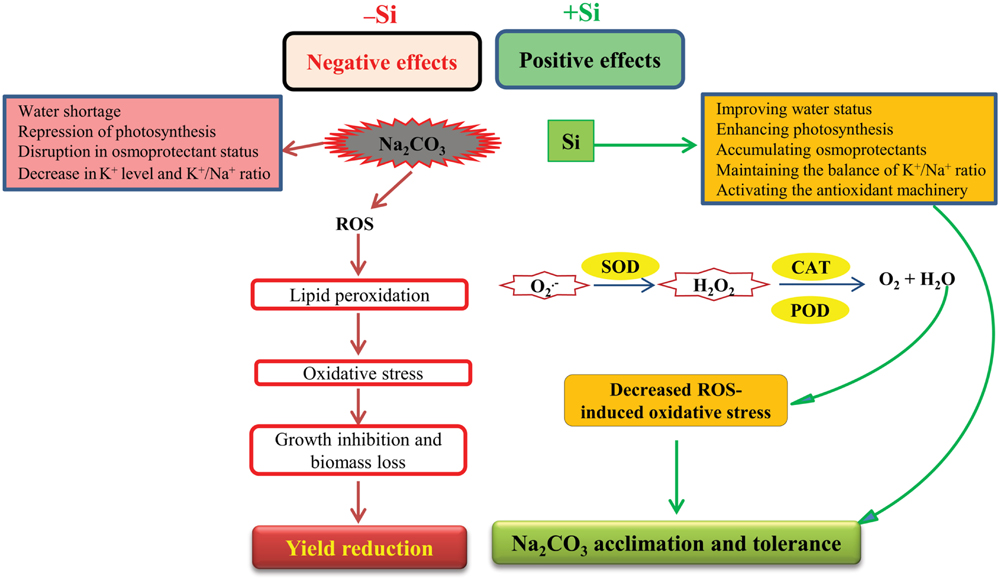
FIGURE 4. A schematic diagram representing effects of alkaline stress (e.g., Na2CO3 stress) and defensive system induced by seed-priming with Si, underlying maize alkaline stress tolerance. Alkaline stress causes negative impacts by repressing photosynthesis, disrupting osmoprotectant status and negatively influencing water status and ionic balance. Excessive alkalinity can promote production of reactive oxygen species (ROS). ROS contribute to lipid peroxidation, resulting in oxidative stress that causes growth inhibition, biomass loss, and subsequently extreme yield reduction. Conversely, seed-priming with Si shows protective mechanism against alkaline stress by improving water balance and photosynthesis, accumulating osmoprotectants and maintaining ionic balance. Si also enhances the detoxification of ROS by stimulating the activities of antioxidant enzymes, such as SOD, CAT, and POD, leading to oxidative stress mitigation. As a result, Si improves alkaline stress tolerance by retaining better growth of alkaline-stressed plants. , superoxide; H2O2, hydrogen peroxide.
Author Contributions
AA conceived, designed, conducted the experiments, collected and analyzed the data. AA and L-ST wrote and revised the manuscript.
Conflict of Interest Statement
The authors declare that the research was conducted in the absence of any commercial or financial relationships that could be construed as a potential conflict of interest.
Acknowledgment
We would like to thank Dr. Mona Fawzy Abu Alhmad for excellent technical support.
References
Abbas, T., Balal, R. M., Shahid, M. A., Pervez, M. A., Ayyub, C. M., Aqueel, M. A., et al. (2015). Silicon-induced alleviation of NaCl toxicity in okra (Abelmoschus esculentus) is associated with enhanced photosynthesis, osmoprotectants and antioxidant metabolism. Acta Physiol. Plant 37, 1–15. doi: 10.1007/s11738-014-1768-5
Abd-Alla, M. H., El-Enany, A. W., Nafady, N. A., Khalaf, D. M., and Morsy, F. M. (2014). Synergistic interaction of Rhizobium leguminosarum bv. viciae and arbuscular mycorrhizal fungi as a plant growth promoting biofertilizers for faba bean (Vicia faba L.) in alkaline soil. Microbiol. Res. 169, 49–58. doi: 10.1016/j.micres.2013.07.007
Abdel Latef, A. A. (2010). Changes of antioxidative enzymes in salinity tolerance among different wheat cultivars. Cereal Res. Commun. 38, 43–55. doi: 10.1556/CRC.38.2010.1.5
Abdel Latef, A. A., Abu Alhmad, M. F., and Sallam, M. M. (2014). Comparative study of the physiological response of two wheat cultivars to salinity stress. Assiut Univ. J. Bot. 43, 55–69.
Abdel Latef, A. A., and Chaoxing, H. (2011). Effect of arbuscular mycorrhizal fungi on growth, mineral nutrition, antioxidant enzymes activity and fruit yield of tomato grown under salinity stress. Sci. Hortic. 127, 228–233. doi: 10.1016/j.scienta.2010.09.020
Abdel Latef, A. A., and Chaoxing, H. (2014). Does the inoculation with Glomus mosseae improve salt tolerance in pepper plants? J. Plant Growth Regulat. 33, 644–653. doi: 10.1007/s00344-014-9414-4
Ahmad, P., Ozturk, M., Sharma, S., and Gucel, S. (2014). Effect of sodium carbonate induced salinity–alkalinity on some key osmoprotectants, protein profile, antioxidant enzymes, and lipid peroxidation in two mulberry (Morus alba L.) cultivars. J. Plant Inter. 9, 460–467.
Ali, Z. A., Hussein, M. M., and El-Taher, A. M. (2015). Effect of antioxidants on some morphological and anatomical features of maize grown under salinity conditions. Int. J. Chem. Technol. Res. 8, 389–400.
Azooz, M. M., Metwally, A., and Abou-Elhamd, M. F. (2015). Jasmonate-induced tolerance of Hassawi okra seedlings to salinity in brackish water. Acta Physiol. Plant 37, 1–13. doi: 10.1007/s11738-015-1828-5
Balakhnina, T., and Borkowska, A. (2013). Effects of silicon on plant resistance to environmental stresses: review. Int. Agrophys. 27, 225–232. doi: 10.2478/v10247-012-0089-4
Bates, L. S., Wladren, P. R., and Tear, D. T. (1973). Rapid determination of free proline for water-stress studies. Plant Soil 39, 205–207. doi: 10.1016/j.dental.2010.07.006
Bradford, M. M. (1976). A rapid and sensitive method for the quantitation of microgram quantities of protein utilizing the principle of protein binding. Anal. Biochem. 72, 248–254. doi: 10.1016/0003-2697(76)90527-3
Campbell, S. A., and Nishio, J. N. (2000). Iron deficiency studies of sugar beet using an improved sodium bicarbonate buffered hydroponics growth system. J. Plant Nutr. 23, 741–757. doi: 10.1080/01904160009382056
Chen, S., Xing, J., and Lan, H. (2012). Comparative effects of neutral salt and alkaline salt stress on seed germination, early seedling growth and physiological response of a halophyte species Chenopodium glaucum. Afr. J. Biotechnol. 11, 9572–9581.
Farooq, M. A., Ali, S., Hameed, A., Ishaque, W., Mahmood, K., and Iqbal, Z. (2013). Alleviation of cadmium toxicity by silicon is related to elevated photosynthesis, antioxidant enzymes; suppressed cadmium uptake and oxidative stress in cotton. Econ. Environ. Saf. 96, 242–249. doi: 10.1016/j.ecoenv.2013.07.006
Gao, Z., Han, J., Mu, C., and Lin, J. (2014). Effects of saline and alkaline stresses on growth and physiological changes in oat (Avena sativa L.) seedlings. Not. Bot. Horti Agrobot. 42, 357–362.
Garíca-Mata, C., and Lamattina, L. (2001). Nitric oxide induces stomatal closure and enhances the adaptive plant responses against drought stress. Plant Physiol. 126, 1196–1204. doi: 10.1104/pp.126.3.1196
Giannopolitis, C. N., and Ries, S. K. (1977). Superoxide dismutases. I. Occurrence in higher plants. Plant Physiol. 59, 309–314. doi: 10.1104/pp.59.2.309
Guntzer, F., Keller, C., and Meunier, J. D. (2012). Benefits of plant silicon for crops: a review. Agron. Sustain. Dev. 32, 201–213. doi: 10.1007/s13593-011-0039-8
Gururani, M. A., Venkatesh, J., and Tran, L. S. (2015). Regulation of photosynthesis during abiotic stress-induced photoinhibition. Mol. Plant 8, 1304–1320. doi: 10.1016/j.molp.2015.05.005
Habibi, G. (2015). Exogenous silicon leads to increased antioxidant capacity in freezing-stressed pistachio leaves. Acta Agric. Slovenica 105, 43–52. doi: 10.14720/aas.2015.105.1.05
Habibi, G., and Hajiboland, R. (2013). Alleviation of drought stress by silicon supplementation in pistachio (Pistacia vera L.) plants. Folia Hort. 25, 21–29.
Hamdia, M. A., and Shaddad, M. A. K. (2010). Salt tolerance of crop plants. J. Stress Physiol. Biochem. 6, 64–90.
Hamdia, M. A., Shaddad, M. A. K., and Doaa, M. M. (2004). Mechanisms of salt tolerance and interactive effects of Azospirillum brasilense inoculation on maize cultivars grown under salt stress conditions. Plant Growth Regulat. 44, 165–174. doi: 10.1023/B:GROW.0000049414.03099.9b
Hameed, A., Sheikh, M. A., Jamil, A., and Basra, S. M. A. (2013). Seed priming with sodium silicate enhances seed germination and seedling growth in wheat (Triticum aestivum L.) under water deficit stress induced by polyethylene glycol. Pak. J. Life Soc. Sci. 11, 19–24.
Hartung, W., Leport, L., Ratcliffe, R. G., Sauter, A., Duda, R., and Turner, N. C. (2002). Abscisic acid concentration, root pH and anatomy do not explain growth differences of chickpea (Cicer arietinum L.) and lupin (Lupinus angustifolius L.) on acid and alkaline soils. Plant Soil 240, 191–199. doi: 10.1023/A:1015831610452
Hasanuzzaman, M., Nahar, K., and Fujita, M. (2013). “Plant response to salt stress and role of exogenous protectants to mitigate salt-induced damages,” in Ecophysiology and Responses of Plants under Salt Stress, eds P. Ahmad, M. M. Azooz, and M. N. V. Prasad (New York, NY: Springer), 25–87.
Irigoyen, J. J., Emerich, D. W., and Sanchez-DIaz, M. (1992). Water stress induced changes in concentrations of proline and total soluble sugar in nodulated alfalfa (Medicago sativa) plants. Physiol. Plant. 84, 55–60. doi: 10.1111/j.1399-3054.1992.tb08764.x
Kariola, T., Brader, G., Li, J., and Palva, T. E. (2005). Chlorophyllase 1, a damage control enzyme, affects the balance between defense pathways in plants. Plant Cell 17, 282–294. doi: 10.1105/tpc.104.025817
Karmollachaab, A., and Gharineh, M. H. (2015). Effect of silicon application on wheat seedlings growth under water-deficit stress induced by polyethylene glycol. Iran Agric. Res. 34, 31–38.
Klapheck, S., Zimmer, I., and Cosse, H. (1990). Scavenging of hydrogen peroxide in the endosperm of Ricinus communis by ascorbate peroxidase. Plant Cell Physiol. 31, 1005–1013.
Lee, Y. P., and Takanashi, T. (1966). An improved colorimetric determination of amino acids with the use of ninhydrin. Anal. Biochem. 14, 71–77. doi: 10.1016/0003-2697(66)90057-1
Liang, Y., Hua, H., Zhu, Y. G., Zhang, J., Cheng, C., and Römheld, V. (2006). Importance of plant species and external silicon concentration to active silicon uptake and transport. New Phytol. 172, 63–72. doi: 10.1111/j.1469-8137.2006.01797.x
Lichtenthaler, H. K., and Wellburn, R. R. (1983). Determination of total carotenoids and chlorophylls a and b of leaf extracts in different solvents. Biochem. Soc. Trans. 11, 591–592. doi: 10.1042/bst0110591
Maehly, A. C., and Chance, B. (1954). “The assay of catalase and peroxidase,” in Methods of Biochemical Analysis, ed. D. Glick (Hoboken, NJ: John Wiley & Sons, Inc), 357–425.
Malčovská, S. M., Dučaiová, Z., and Bačkor, M. (2014a). Impact of silicon on maize seedlings exposed to short-term UV-B irradiation. Biologia 69, 1349–1355.
Malčovská, S. M., Dučaiová, Z., Maslaóáková, I., and Bačkor, M. (2014b). Effect of silicon on growth, photosynthesis, oxidative status and phenolic compounds of maize (Zea mays L.) grown in cadmium excess. Water Air Soil Pollut. 225, 1–11.
Marafon, A. C., and Endres, L. (2013). Silicon: fertilization and nutrition in higher plants. Rev. Cienc. Agrar. 6, 80–88.
Mohsenian, Y., and Roosta, H. R. (2015). Effects of grafting on alkali stress in tomato plants: datura rootstock improve alkalinity tolerance of tomato plants. J. Plant Nutr. 38, 51–72. doi: 10.1080/01904167.2014.920370
Mostofa, M. G., Rahman, A., Ansary, M. M. U., Watanabe, A., Fujita, M., and Tran, L. S. P. (2015). Hydrogen sulfide modulates cadmium-induced physiological and biochemical responses to alleviate cadmium toxicity in rice. Sci. Rep. 5:14078. doi: 10.1038/srep14078
Mukherjee, S. P., and Choudhari, M. A. (1983). Implications of water stress induced changes in the levels of endogenous ascorbic acid and hydrogen peroxide in Vigna seedlings. Physiol. Plant. 58, 116–170. doi: 10.1111/j.1399-3054.1983.tb04162.x
Paz, R. C., Rocco, R. A., Reinoso, H., Menéndez, A. B., Pieckenstain, F. L., and Ruiz, O. A. (2012). Comparative study of alkaline, saline, and mixed saline–alkaline stresses with regard to their effects on growth, nutrient accumulation, and root morphology of Lotus tenuis. J. Plant Growth Regulat. 31, 448–459. doi: 10.1007/s00344-011-9254-4
Peng, Y. L., Gao, Z. W., Gao, Y., Liu, G. F., Sheng, L. X., and Wang, D. L. (2008). Eco-physiological characteristics of alfalfa seedlings in response to various mixed salt-alkaline stresses. J. Integr. Plant Biol. 50, 29–39. doi: 10.1111/j.1744-7909.2007.00607.x
Radi, A. A., Abdel-Wahab, D. A., and Hamada, A. M. (2012). Evaluation of some bean lines tolerance to alkaline soil. J. Biol. Earth Sci. 2, B18–B27.
Rizwan, M., Ali, S., Ibrahim, M., Farid, M., Adrees, M., Bharwana, S. A., et al. (2015). Mechanisms of silicon-mediated alleviation of drought and salt stress in plants: a review. Environ. Sci. Pollut. Res. 22, 15416–15431. doi: 10.1007/s11356-015-5305-x
Sahebi, M., Hanafi, M. M., Akmar, A. S., Rafii, M. Y., Azizi, P., Tengoua, F. F., et al. (2015). Importance of silicon and mechanisms of biosilica formation in plants. BioMed Res. Int. 2015, 1–16. doi: 10.1155/2015/396010
Sawas, D., and Ntatsi, G. (2015). Biostimulant activity of silicon in horticulture. Sci. Hortic. 196, 66–81. doi: 10.1016/j.scienta.2015.09.010
Shen, X., Xiao, X., Dong, Z., and Chen, Y. (2014). Silicon effects on antioxidative enzymes and lipid peroxidation in leaves and roots of peanut under aluminum stress. Acta Physiol. Plant 36, 3063–3069. doi: 10.1007/s11738-014-1676-8
Shi, D. C., and Zhao, K. F. (1997). Effects of NaCl and Na2CO3 on growth of Puccinellia tenuiflora and on present state of mineral elements in nutrient solution. Acta Pratacult. Sin. 6, 51–61.
Skerget, M., Kotnik, P., Hadolin, M., Hras, A., Simonic, M., and Knez, Z. (2005). Phenols, proanthocyanidins, flavones and flavonols in some plant materials and their antioxidant activities. Food Chem. 89, 191–198. doi: 10.1016/j.foodchem.2004.02.025
Soundararajan, P., Sivanesan, I., Jana, S., and Jeong, B. R. (2014). Influence of silicon supplementation on the growth and tolerance to high temperature in Salvia splendens. Hort. Environ. Biotechnol. 55, 271–279. doi: 10.1007/s13580-014-0023-8
Tripathi, P., Tripathi, R. D., Singh, R. P., Dwivedi, S., Goutam, D., Shri, M., et al. (2013). Silicon mediates arsenic tolerance in rice (Oryza sativa L.) through lowering of arsenic uptake and improved antioxidant defence system. Ecol. Eng. 52, 96–103. doi: 10.1016/j.ecoleng.2012.12.057
Van Bockhaven, J., De Vleesschauwer, D., and Höfte, M. (2013). Towards establishing broad-spectrum disease resistance in plants: silicon leads the way. J. Exp. Bot. 64, 1281–1293. doi: 10.1093/jxb/ers329
Wang, S., Liu, P., Chen, D., Yin, L., Li, H., and Deng, X. (2015). Silicon enhanced salt tolerance by improving the root water uptake and decreasing the ion toxicity in cucumber. Front. Plant Sci. 6:759. doi: 10.3389/fpls.2015.00759
Williams, V., and Twine, S. (1960). “Flame photometric method for sodium, potassium and calcium,” in Modern Methods of Plant Analysis, eds K. Peach and M. V. Tracey (Berlin: Springer-Verlag), 3–5.
Zhang, Y., Zhang, L., and Hu, X. H. (2014). Exogenous spermidine-induced changes at physiological biochemical parameters levels in tomato seedling grown in saline-alkaline condition. Bot. Stud. 55, 1–8. doi: 10.1186/s40529-014-0058-2
Zhang, Z. L., and Qu, W. (2004). Experimental Guidance of Plant Physiology. Beijing: High Education.
Keywords: alkaline stress, antioxidant enzymes, leaf pigments, maize, osmoprotectants, seed-priming with Si, stress mitigation
Citation: Abdel Latef AA and Tran L-SP (2016) Impacts of Priming with Silicon on the Growth and Tolerance of Maize Plants to Alkaline Stress. Front. Plant Sci. 7:243. doi: 10.3389/fpls.2016.00243
Received: 19 December 2015; Accepted: 13 February 2016;
Published: 10 March 2016.
Edited by:
Mohammad Anwar Hossain, Bangladesh Agricultural University, BangladeshReviewed by:
Chaoxing He, Chinese Academy of Agricultural Sciences, ChinaRoghieh Hajiboland, University of Tabriz, Iran
Copyright © 2016 Abdel Latef and Tran. This is an open-access article distributed under the terms of the Creative Commons Attribution License (CC BY). The use, distribution or reproduction in other forums is permitted, provided the original author(s) or licensor are credited and that the original publication in this journal is cited, in accordance with accepted academic practice. No use, distribution or reproduction is permitted which does not comply with these terms.
*Correspondence: Arafat A. Abdel Latef, arafat.moawad@sci.svu.edu.eg, moawad76@gmail.com; Lam-Son P. Tran, sontran@tdt.edu.vn, son.tran@riken.jp