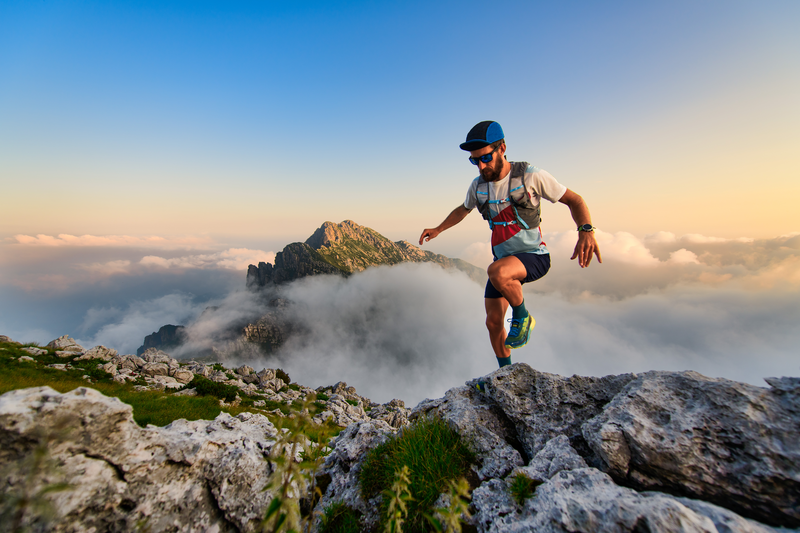
94% of researchers rate our articles as excellent or good
Learn more about the work of our research integrity team to safeguard the quality of each article we publish.
Find out more
ORIGINAL RESEARCH article
Front. Plant Sci. , 04 February 2016
Sec. Plant Pathogen Interactions
Volume 7 - 2016 | https://doi.org/10.3389/fpls.2016.00075
This article is part of the Research Topic Biotechnological potential of plant-microbe interactions in environmental decontamination View all 19 articles
Application of hyperaccumulator-endophyte symbiotic systems is a potential approach to improve phytoremediation efficiency, since some beneficial endophytic bacteria are able to detoxify heavy metals, alter metal solubility in soil, and facilitate plant growth. The objective of this study was to isolate multi-metal resistant and plant beneficial endophytic bacteria and to evaluate their role in enhancing plant growth and metal accumulation/translocation. The metal resistant endophytic bacterial strain E6S was isolated from stems of the Zn/Cd hyperaccumulator plant Sedum plumbizincicola growing in metalliferous mine soils using Dworkin and Foster salts minimal agar medium with 1-aminocyclopropane-1-carboxylate (ACC) as the sole nitrogen source, and identified as homologous to Achromobacter piechaudii based on morphological and biochemical characteristics, partial 16S rDNA sequence and phylogenetic analysis. Strain E6S showed high level of resistance to various metals (Cd, Zn, and Pb). Besides utilizing ACC, strain E6S exhibited plant beneficial traits, such as solubilization of phosphate and production of indole-3-acetic acid. Inoculation with E6S significantly increased the bioavailability of Cd, Zn, and Pb in soil. In addition, bacterial cells bound considerable amounts of metal ions in the following order: Zn > Cd >Pb. Inoculation of E6S significantly stimulated plant biomass, uptake and bioaccumulation of Cd, Zn, and Pb. However, E6S greatly reduced the root to shoot translocation of Cd and Zn, indicating that bacterial inoculation assisted the host plant to uptake and store heavy metals in its root system. Inoculation with the endophytic bacterium E6S homologous to A. piechaudii can improve phytostabilization of metalliferous soils due to its effective ability to enhance in situ metal rhizoaccumulation in plants.
Mining activities produce waste tailings containing high levels of metal pollutants that have significant environmental impacts and can affect human health through the food chain (Moreno et al., 2010). During mineral ore processing mine tailings are generated and mostly left without proper management, therefore leading to metal contamination of surrounding soils, with detrimental impacts on the soil microbial community and consequent reduction in ecosystem functioning (Kossoff et al., 2014).
Phytoremediation, application of plants to remove (phytoextraction), stabilize (phytostabilization), or volatilize (phytovolatilization) heavy metals in situ in a more attractive and cost-effective manner than the conventional physicochemical technologies, has received increasing attention over the last decades (Raskin and Ensley, 2000). Particularly, phytostabilization of metal contaminated mine tailings, which uses plants that minimize metal accumulation into aboveground tissues, seems to be most promising for remediating polluted sites when phytoextraction is not a feasible option (Mendez and Maier, 2008). Because mine tailings have low nutrient contents, to overcome the limitations of plant establishment, soil amendments with fresh or composted organic matter (biochemical amendments) can enhance plant colonization and reduce metal toxicity and solubility, thereafter improving phytostabilization efficiency (Lee et al., 2011). However, most of those biochemical amendments, such as cyclonic ashes, steel shots. and superphosphate, are toxic to plants and their associated microbes (Ribeiro Filho et al., 2011). Some beneficial bacteria have been successfully employed for environmental applications (so-called bioaugmentation) due to their ability to: (1) promote plant growth by producing beneficial metabolites [e.g., siderophores, indole-3-acetic acid (IAA) and 1-aminocyclopropane-1-carboxylate (ACC) deaminase] and solubilizing phosphate (P); and/or (2) alter soil metal mobility without disturbing soil ecological structure and function through various mechanisms such as metal biosorption/bioaccumulation, redox reaction, chelation, or complexation (Glick, 2010; Ma et al., 2011a). Amongst all the beneficial features, the production of ACC deaminase is considered as one of the major plant growth promoting traits of bacteria (Ma et al., 2011a). Mesa et al. (2015) reported that the inoculation of autochthonous rhizobacteria stimulated the biomass of native Spartina maritima, and enhanced metal (As, Cu, Pb, and Zn) accumulation in the root of plants (rhizoaccumulation) grown in multi-metal contaminated soils. Since bacterial endophytes have more intimate association with host plants than rhizobacteria, they could be reliable bioinoculants for improving metal phytostabilization.
Although the effects of inoculating endophytic bacterial strains on growth promotion of various host and/or non-host plants have been reported (Ma et al., 2011b; Shin et al., 2012; Visioli et al., 2014), little is known on application of endophytes for enhanced phytoremediation of natural non-sterile polluted soils. Sedum plumbizincicola is known to hyper-accumulate or extract Cd and Zn from soils (Jiang et al., 2010). Despite the potential of S. plumbizincicola to remove various metals from polluted soil, its slow growth is a limitation that needs to be overcome.
The objectives of this study were to: (1) isolate and characterize metal resistant endophytic bacteria that can utilize ACC as the sole nitrogen (N) source; (2) assess plant beneficial activities, metal biosorption and mobilization capacities of a selected endophytic bacterium; (3) examine the effect of the endophytic bacterium on plant growth and metal accumulation/translocation in host S. plumbizincicola in non-sterile multi-metal contaminated soils.
Bacterial strains were isolated from tissues of Zn/Cd hyperaccumulator S. plumbizincicola growing on metalliferous mine soils in Chunan city of Zhejiang, Southeast of China. Plant samples were washed thoroughly with tap water followed by three rinses with deionized water and then separated into roots, stems, and leaves. Plant organs were sterilized by immersion for 1 min in 70% (v/v) ethanol, and then 3% sodium hypochlorite for 3 min and washed three times with sterile water to remove residual chemicals. To confirm the success of the surface sterilization process, plant tissues were plated on Luria–Bertani (LB) agar plate to detect epiphytic bacteria. No contamination was found. The plant tissues (0.5 g) were ground with a mortar and pestle in 5 mL of sterile deionized water. The appropriate dilutions were plated onto sucrose-minimal salts low-phosphate agar medium supplemented with 100 mg L-1 of Cd (CdCl2), Zn (ZnSO4), and Pb [Pb(NO3)2]. After incubating at 26°C for 5 days, colonies were randomly picked based on distinct colony morphology, purified and re-streaked on the same media. To isolate beneficial endophytes, the growth of all metal resistant isolates was evaluated on Dworkin and Foster salts minimal medium (Dworkin and Foster, 1958) containing ACC as the sole N source.
One bacterial isolate which showed fast growth and capability of utilizing ACC as the sole N source was identified and used for further study. Total DNA was extracted from cells of the selected bacterial isolate using the QuickExtractTM bacterial DNA extraction kit. The 16S ribosomal gene was amplified using the primers 27F (5′-AGAGTTTGATCCTGGCTCAG-3′) and 1492R (5′-GGTTACCTTGTTACGACTT-3′) under the reaction conditions described by Branco et al. (2005). The amplification product (5 μL) was separated by agarose gel (1%, w/v) electrophoresis in TAE buffer (0.04 M Tris acetate, 1 mM EDTA) containing 1 μg mL-1 ethidium bromide. Partial nucleotide sequence of the amplified 16S rDNA was determined with automated DNA sequencer and then compared with similar sequences in the GenBank using BLAST.
The bacterial isolate was grown for 5 days at 26°C on LB media supplemented with heavy metal (Cd, Zn, and Pb) at varying concentrations (50–1500 mg L-1). The highest concentration of metal allowing bacterial growth was defined as its resistance level. The antibiotic sensitivity of the isolate was determined by the disk diffusion method (Rajkumar et al., 2008).
The ACC deaminase activity of the isolate was examined by monitoring the concentration of α-ketobutyrate (α-KB) generated through the enzymatic hydrolysis of ACC as described by Honma and Shimomura (1978). The protein content of cell suspensions was determined by the Bradford method (Bradford, 1976). Synthesis of IAA by the strain was assayed as described by Bric et al. (1991) using LB medium with 0.5 mg mL-1 of L-tryptophan. Bacterial siderophore production was detected by chrome azurol S (CAS) agar plate assay (Schwyn and Neilands, 1987). The P solubilization by the isolate was analyzed in modified Pikovskayas medium (Sundara-Rao and Sinha, 1963) and the solubilized P in the culture supernatant was determined as described by Park et al. (2011).
In the metal mobilization assay, the physicochemical properties of contaminated agricultural soil collected from Fuyang city of Zhejiang Province, China were: pH (1:1 w/v water) 8.1; organic matter 36.3 g kg-1; cation exchange capacity 11.4 cmol kg-1; Cd 5.9 mg kg-1; Zn 736 mg kg-1; Pb 153 mg kg-1. The soil was air dried, crushed (<2 mm) and then autoclaved at 121°C for 2 h. The bacterial strain was incubated at 27°C with shaking at 200 rpm for 18 h. After adjusting cell density to an OD600 of 1.5, 5 mL of culture were centrifuged, then gently washed twice with 0.05 M phosphate buffer (pH 7.2) and three times with sterile deionized water, recentrifuged and finally resuspended in 5 mL sterile deionized water. One milliliter of washed bacterial culture at an OD600 of 1 (treatment) or sterile deionized water (control), was added to 1 g of sterilized soil in 50 mL sealed centrifuge tubes. All tubes were weighed and kept at 27°C with 200 rpm in the dark. The total weight was kept constant by adding sterile deionized water to compensate for evaporation. After 7 days, 10 mL of sterile deionized water were added to extract water-soluble metal from soil. The suspensions were centrifuged at 7000 rpm for 10 min and filtered. Metal (Cd, Zn, and Pb) contents of bioavailable fraction in each filtrate were determined using a flame atomic absorption spectrophotometer (AAS; Ma et al., 2011b).
The biosorption of Cd, Zn, and Pb by bacterial cells was evaluated as described by Rajkumar et al. (2009). The bacterium was grown to exponential (OD600 = 1) at 27°C in LB medium. Cells were harvested by centrifugation at 6000 rpm for 20 min and washed twice with sterile deionized water. The wet biomass was re-suspended in 150 mg L-1 of Cd, Zn, or Pb. Cells were harvested after incubation at room temperature for 10 h by centrifugation and the residual metal ion in the supernatant was measured by AAS. The amount of metal biosorbed onto bacterial cells was calculated by subtracting the metal concentration in the supernatant from the original concentration.
The selected ACC-utilizing strain was initially assessed for its ability to promote plant growth in phytagar media supplied with or without 10 mg Cd L-1 using Brassica napus as a model plant (Ma et al., 2011b). For pot experiment, the multi-metal contaminated soil described above was used, while agricultural soil from Nanjing, China was used for comparison. The soil was air dried, sieved (2 mm), and stored at 20°C before use. S. plumbizincicola seedlings of equal size were obtained from an old Pb/Zn mine in the Zhejiang province of China. Shoot samples with ca. 5 cm were well cleaned with tap water and grown hydroponically in half-strength Hoagland’s nutrient solution for a week. Before inoculation, bacterial colony marked with antibiotic resistance was obtained after incubating parental strain onto LB agar containing ampicillin (100 mg L-1) and tetracycline (75 mg L-1), simultaneously. Surface sterilized roots of precultured seedlings were soaked for 2 h in the bacterial culture (OD600 of 1.5) or sterile water (controls) and transplanted into 1 L pot filled with 750 g of soil. The seedlings (six plants pot-1) were allowed to grow under greenhouse condition (25 ± 5°C, 16:8 day/night regime). Each treatment was performed in five replicates. After 75 days, plants were carefully removed from the pots and the root surface was cleaned thoroughly with deionized water. Plant root and shoot length, fresh and dry weight were measured. The concentrations of Cd, Zn, and Pb in root and shoot were quantified as described by Ma et al. (2009). The translocation factor (TF) was calculated as the ratio of metal content in the shoots to that in the roots (Malik et al., 2010) and the bioaccumulation factor (BCF) was calculated as the ratio of metal contents in the entire plant to that in the soil (Abdul and Thomas, 2009). The colonization of introduced strain in the rhizosphere and interior tissues of S. plumbizincicola was determined using the intrinsic antibiotic marker combined with the dilution-plate method (Ma et al., 2011b).
Student’s t-test (p < 0.05) or Analysis of Variance (ANOVA) followed by the post hoc Fisher Least Significant Difference test (p < 0.05) were used to compare treatment means. All the statistical analyses were performed using SPSS 17.0.
Although the interaction between endophytic bacteria and their host plants is not completely understood, some endophytic bacteria isolated from heavy metal hyperaccumulators appear to exert beneficial effects on their hosts (Shin et al., 2012), such as amelioration of metal stress, stimulation of plant establishment and growth, and biocontrol of phytopathogens (Ma et al., 2011a). Consequently, such beneficial endophytic bacteria could be isolated and selected for their application in assisting phytoremediation of metal contaminated soils (Rajkumar et al., 2009). In this study, we isolated a metal resistant endophytic bacterial strain from stems of Zn/Cd hyperaccumulator S. plumbizincicola and assessed its effect as a bioinoculant on multi-metal phytoremediation by its host plant. The initial screening was based on the morphological differences of bacterial colonies and resulted in the isolation of 42 metal (100 mg L-1 of Cd, Zn, and Pb) resistant endophytic strains from interior tissues of S. plumbizincicola. Out of 42 strains, isolate E6S was specifically chosen based on its fast growth and capability of utilizing ACC as the sole N source.
Based on morphological and biochemical characteristics (Table 1), comparative analysis of 16S rDNA sequence and phylogenic analysis (Figure 1), strain E6S was identified as being homologous to Achromobacter piechaudii (100% similarity). The sequence obtained (858 bp) was submitted to the NCBI databases under the accession number KC151254. Strain E6S was gram-negative, motile, rod shaped and positive for oxidase and catalase. It was able to produce H2S, utilize citrate and hydrolyze starch (Table 1).
TABLE 1. Morphological, physiological, and biochemical characteristics of endophytic bacterium E6S homologous to Achromobacter piechaudii.
FIGURE 1. Phylogenetic tree showing the relationship of partial 16S rDNA gene sequences from endophytic bacterium E6S homologous to Achromobacter piechaudii with other related sequences from Achromobacter. Escherichia coli was used as the out-group. The value on each branch is the percentage of bootstrap replications supporting the branch.
Under various abiotic stresses (e.g., heavy metals, drought, and salinity), microorganisms face a constant battle for limited resources and try to adapt to unfavorable environmental conditions by acting as stress ameliorators (Hibbing et al., 2010). Hence, strain E6S was tested for the ability to grow on both metal and antibiotic-supplemented agar media. Strain E6S was found to exhibit resistance to heavy metal (Cd, Zn, and Pb) and various antibiotics (Table 2). The order of the toxicity of metals to the isolate was found to be Cd > Zn > Pb. This strain exhibited high tolerance to multiple metals, which could be attributed to the fact that it was isolated from tissues of a Zn/Cd hyperaccumulator plant, likely containing high levels of these bioavailable metal ions (Idris et al., 2004). Among six antibiotics tested, strain E6S showed resistance to ampicillin and tetracycline.
TABLE 2. Heavy metal tolerance, antibiotic resistance, and plant growth promoting traits of endophytic bacterium E6S homologous to Achromobacter piechaudii.
Endophytic bacteria can stimulate plant growth directly by solubilizing unavailable nutrients (e.g., P, N, and potassium), sequestering iron by siderophores and phytohormones (e.g., IAA) or indirectly by inducing a systemic resistance in plants against various types of pathogens (Ma et al., 2011b). These features make them perfect choices for improving phytoremediation. Strain E6S was able to produce ACC deaminase, IAA and solubilize P, whereas no biosynthesis of siderophore was found (Table 2). In general, bacterial IAA at low level has been implicated in promoting primary root elongation through cell division, however, a high IAA level can inhibit primary root growth but stimulate lateral root formation (Gravel et al., 2007). A low level of IAA production by strain E6S (22 mg L-1) suggests close relationship between plant growth promotion activity (Table 3) and IAA production. Strain E6S exhibited high tricalcium phosphate-solubilizing ability (135 mg L-1; Table 2), which may compensate for P deficiency-induced plant growth retardation in metal contaminated soil. ACC-utilizing bacteria have been found to prevent the inhibition of root growth by hydrolyzing the ethylene precursor ACC into ammonia and α-KB and inhibiting ACC synthase activity (Arshad et al., 2007). Although strain E6S showed relatively low ACC deaminase activity (11 μm α-KB mg-1 h-1), it seemed to be efficient in promoting plant growth under metal stress (Table 3). The results suggest that the endophytic bacteria, which can synthesize beneficial metabolites such as IAA, ACC deaminase, and solubilize P should be considered as promising biofertilizers.
TABLE 3. Effects of endophytic bacterium E6S homologous to Achromobacter piechaudii on the growth of Brassica napus in phytagar assay and Sedum plumbizincicola in pot experiment.
Endophytic bacterium E6S homologous to A. piechaudii displayed the potential for biological mobilization of Cd, Zn, and Pb in multi-metal contaminated soil (Figure 2). The inoculation of E6S significantly increased (p < 0.05) water extractable Cd, Zn, and Pb in metal contaminated soils by 3.9-, 5.8- and 6.0-fold, respectively, compared to the controls. The observation indicates that strain E6S facilitated the release of metals from non-soluble phase in the soil matrix, thus improving their bioavailability to plants. This may be attributed to organic acid production and phosphate solubilization mediated reduction in soil pH (Ma et al., 2009, 2015).
FIGURE 2. Effect of inoculation with E6S on the mobilization of Cd, Zn, and Pb in soil. Bars represent standard deviations of triplicates. An asterisk (∗) denotes a value significantly greater than the corresponding control value according to Student’s t-test (p < 0.05).
Biosorption capacity of bacteria plays an important role in reducing metal phytotoxicity by limiting the entry of metal ions into plant cells and may contribute for enhanced plant growth in metal contaminated soils (Ma et al., 2011a). At 150 mg L-1 initial metal concentrations, strain E6S was able to remove significant amounts of Cd, Zn, or Pb within 10 h incubation (Figure 3). After 8 h incubation, maximum biosorption by E6S was reached, thereafter remaining constant. This was probably due to the achievement of specific equilibrium for metal concentrations. The highest content of metal biosorption was observed with Zn (10.9 mg g-1 of cell dry weight), while the lowest was seen with Pb (3.2 mg g-1 of cell dry weight). This was probably due to ionic radius of each metal ion (Karakagh et al., 2012), since Zn (0.88 Å) with smaller ionic radius may be more rapidly complexed by bacterial cell wall/membrane compared with Cd (0.97 Å) and Pb (1.2 Å).
FIGURE 3. Biosorption of Cd, Zn, and Pb on E6S cells. Bars represent standard deviations of triplicates.
In phytagar assay, inoculation of E6S induced significant increases in fresh and dry weight, ratio of root/shoot dry weight of B. napus in both unpolluted and polluted (10 mg L-1 Cd) phytagar media (Table 3). For instance, E6S induced an increase in fresh and dry weight, and ratio of root/shoot dry weight in Cd polluted media by 65, 47, and 94%, respectively, compared to non-inoculated control. Bacterial inoculation greatly enhanced the biomass production of B. napus under non-stressed and metal-stressed conditions. Plants inoculated with strain E6S presented a significantly higher root/shoot ratio than the respective controls, indicating that with the help of endophytic bacterium E6S, plants can acquire nutrients more efficiently from soils for plant biomass production, especially for roots. Moreover, the relative elongation rate (RER) of root and shoot in unpolluted media (1.62 and 1.15) were lower than that in polluted treatment (2.78 and 1.54). A possible explanation might be that the endophytic bacteria could exert more efficient functions that help plants to cope with adverse environmental stress (Rajkumar et al., 2009).
In pot experiment, inoculation of E6S significantly improved fresh and dry weight of S. plumbizincicola in both pristine and multi-metal polluted soils under non-sterile conditions (Table 3). For example, inoculation of E6S considerably increased plant fresh and dry weight by 35 and 28%, respectively, in metal polluted soils. The beneficial effects of E6S on growth of host S. plumbizincicola were also observed on growth of non-host B. napus in phytagar assay. These effects may be attributed to beneficial metabolites produced by strain E6S, such as IAA and ACC deaminase, which alleviated metal phytotoxicity and thereafter stimulated plant development. Additionally, inoculation of E6S did not significantly influence ratio of root/shoot dry weight in neither pristine nor polluted soils. The presence of metals declined ratio of root/shoot dry weight, compared with non-polluted control. These results concur with the earlier observations of Wan et al. (2012) that elevated metal concentrations mainly impaired root growth, in spite of S. plumbizincicola being qualified as a Zn/Cd hyperaccumulator. It is therefore concluded that bioinoculant E6S could serve as biofertilizer for phytoremediation purposes. Interestingly, the fresh and dry weights of S. plumbizincicola grown in metal contaminated soil were higher than in pristine soil (Table 3). A possible explanation could be that the current levels of heavy metals in soil are not toxic to the plant and can stimulate its growth. This may be attributed to both a beneficial effect of improved metal nutrition and/or the activation of stress scavenging mechanisms, which can help the plant to cope with environmental stresses (Dalcorso et al., 2013).
The changes in metal solubility in polluted soils caused by biological amendments can contribute to facilitate metal accumulation in plants (Ma et al., 2009). Therefore, the effects of metal mobilizing bacterium E6S on metal uptake and translocation by S. plumbizincicola were evaluated. In general, inoculation of strain E6S significantly improved plant uptake of Cd, Zn, and Pb (Table 4). For instance, strain E6S increased Cd, Zn, and Pb concentration in S. plumbizincicola by 32, 37, and 89%, respectively, which is in accordance with significant improvements of BCF of metal (Cd, Zn, and Pb) induced by E6S. This corroborates the data shown in Figure 2 for bacterial metal mobilization, indicating that inoculation of E6S facilitated metal (Cd, Zn, and Pb) bioavailability in soils and thereby their uptake by plants. However, the bacterial inoculation significantly decreased TF of Cd and Zn (p < 0.05; Table 4) and metal accumulation in shoots (data not shown). The TF was 2.7 and 1.5 (>1) for Cd and Zn in non-inoculated plants, but it decreased to 0.5 and 0.7 (<1) after inoculation with strain E6S. S. plumbizincicola has been reported to be a hyperaccumulator for Cd and Zn phytoextraction due to their TF > 1, however, our data showed that the inoculation of strain E6S inhibited the plant-self translocation of metal from roots to shoots and helped plants store metals in their roots, which is desirable for phytostabilization purposes. The present observations indicate that strain E6S effectively increased the bioavailability of metal (Cd, Zn, and Pb) in the rhizosphere soils and also promoted the growth of host S. plumbizincicola plants, consequently increasing the total plant metal uptake, while diminishing the translocation of metals from roots to shoots. Results suggest that endophytic bacterium E6S can not only protect the plants against the inhibitory effects of multiple metals, but also effectively improve rhizoaccumulation of toxic metals. Therefore, it can be used to assist phytostabilization of heavy metals in the plant root system. Previously, Srivastava et al. (2013) also reported that Staphylococcus arlettae NBRIEAG-6 enhanced As accumulation in roots of B. juncea and helped in As phytostabilization. Recently, Sura-de Jong et al. (2015) reported that selenium hyperaccumulators harbor a diverse endophytic bacterial community. It is becoming apparent that some endophytic bacteria play an important role in metal hyperaccumulation and translocation processes, contributing to phytoextraction, while others seem to be effective in improving rhizoaccumulation, contributing to phytostabilization. Future studies are, therefore, needed to access the structure and diversity of bacterial endophytes of S. plumbizincicola and their influence in phytoremediation processes.
TABLE 4. Effects of endophytic bacterium E6S homologous to Achromobacter piechaudii on metal uptake, translocation factor and bioaccumulation factor by Sedum plumbizincicola.
After 75 days of inoculation onto plants, strain E6S exhibited high-density colonization of the rhizosphere [3.9 × 105 colony-forming units (CFU) g-1], and fresh roots, stems and leaves (5.4, 3.7, and 0.8 × 103 CFU g-1, respectively) of S. plumbizincicola, indicating the great potential of this strain to establish, survive, and develop inside plant tissues and in the root zone. Since beneficial bacteria have great potential to contribute to sustainable plant growth promotion and metal rhizoaccumulation, the colonization and survival properties of introduced beneficial strains are crucial features to evaluate the capacity to assist their host plants in coping with metal stress and therefore phytostabilization efficiency in contaminated sites (Ma et al., 2011a).
Endophytic bacterium E6S homologous to A. piechaudii isolated from metal hyperaccumulator S. plumbizincicola stems was able to facilitate plant growth through the production of IAA and ACC deaminase and solubilization of P. The characterization studies showed that the isolate resisted high concentrations of Cd, Zn, and Pb, via extracellular biosorption in metal containing liquid media and increased water extractable metal concentration in metal contaminated soils. The inoculation of E6S significantly enhanced plant growth and rhizoaccumulation of Cd, Zn, and Pb by host S. plumbizincicola, however, the TFs of Cd and Zn remarkably declined in the presence of bacterial inoculation. Effective metal biosorption and mobilizing capacities as well as the potential to adapt to/survive multi-metal stress conditions along with various plant beneficial traits are clear indications of the advantages of employing this microorganism as a bioinoculant for ameliorating metal phytotoxicity and thus enhancing phytostabilization efficiency. Further work will address the mechanism of the selected bacterial strain contributing to reduce metal translocation from roots to shoots and its effect on the plant biomass yield and metal phytostabilization in field experiments.
YM wrote the manuscript and carried out experiments; CZ and RO analyzed experimental results; HF was the project sponsor; YL designed experiments.
The authors declare that the research was conducted in the absence of any commercial or financial relationships that could be construed as a potential conflict of interest.
YM and RO wish to acknowledge the support of Fundação para a Ciência e a Tecnologia (FCT) through the research grants SFRH/BPD/76028/2011 and SFRH/BPD/85008/2012 and Fundo Social Europeu. This work is financed by National Funds through the FCT within the Project UID/BIA/04004/2013.
Abdul, H. B. O., and Thomas, B. V. (2009). Translocation and bioaccumulation of trace metals in desert plants of Kuwait Governorates. Res. J. Environ. Sci. 3, 581–587. doi: 10.3923/rjes.2009.581.587
Arshad, M., Saleem, M., and Hussain, S. (2007). Perspectives of bacterial ACC deaminase in phytoremediation. Trends Biotechnol. 25, 356–362. doi: 10.1016/j.tibtech.2007.05.005
Bradford, M. M. (1976). A rapid and sensitive method for the quantitation of microgram quantities of protein utilizing the principle of protein dye binding. Anal. Biochem. 72, 248–254. doi: 10.1016/0003-2697(76)90527-3
Branco, R., Chung, A. P., Verıssimo, A., and Morais, P. V. (2005). Impact of chromium contaminated wastewaters on the microbial community of a river. FEMS Microbiol. Ecol. 54, 35–46. doi: 10.1016/j.femsec.2005.02.014
Bric, J. M., Bostock, R. M., and Silversone, S. E. (1991). Rapid in situ assay for indole acetic acid production by bacteria immobilization on a nitrocellulose membrane. Appl. Environ. Microbiol. 57, 535–538.
Dalcorso, G., Fasani, E., and Furini, A. (2013). Recent advances in the analysis of metal hyperaccumulation and hypertolerance in plants using proteomics. Front. Plant Sci. 4:280. doi: 10.3389/fpls.2013.00280
Dworkin, M., and Foster, J. (1958). Experiments with some microorganisms which utilize ethane and hydrogen. J. Bacteriol. 75, 592–601.
Glick, B. R. (2010). Using soil bacteria to facilitate phytoremediation. Biotechnol. Adv. 28, 367–374. doi: 10.1016/j.biotechadv.2010.02.001
Gravel, V., Antoun, H., and Tweddell, R. J. (2007). Growth stimulation and fruit yield improvement of greenhouse tomato plants by inoculation with Pseudomonas putida or Trichoderma atroviride: possible role of indole acetic acid, (IAA). Soil Biol. Biochem. 39, 1968–1977. doi: 10.1016/j.soilbio.2007.02.015
Hibbing, M. E., Faqua, C., Parsek, M. R., and Peterson, S. B. (2010). Bacterial competition: surviving and thriving in the microbial jungle. Nat. Rev. Microbiol. 8, 15–25. doi: 10.1038/nrmicro2259
Honma, M., and Shimomura, T. (1978). Metabolism of 1-aminocyclopropane-1-carboxylic acid. Agric. Biol. Chem. Tokyo 42, 1825–1831. doi: 10.1007/978-94-011-4453-7_7
Idris, R., Trifonova, R., Puschenreiter, M., Wenzel, W. W., and Sessitsch, A. (2004). Bacterial communities associated with flowering plants of the Ni hyperaccumulator Thlaspi goesingense. Appl. Environ. Microbiol. 70, 2667–2677. doi: 10.1128/AEM.70.5.2667-2677.2004
Jiang, J. P., Wu, L. H., Li, N., Luo, Y. M., Liu, L., Zhao, Q. G., et al. (2010). Effects of multiple heavy metal contamination and repeated phytoextraction by Sedum plumbizincicola on soil microbial properties. Eur. J. Soil Biol. 46, 18–26. doi: 10.1016/j.ejsobi.2009.10.001
Karakagh, R. M., Chorom, M., Motamedi, H., Kalkhajeh, Y. K., and Oustan, S. (2012). Biosorption of Cd and Ni by inactivated bacteria isolated from agricultural soil treated with sewage sludge. Ecohydrol. Hydrobiol. 12, 191–198. doi: 10.1016/S1642-3593(12)70203-3
Kossoff, D., Dubbin, W. E., Alfredsson, M., Edwards, S. J., Macklin, M. G., and Hudson-Edwards, K. A. (2014). Mine tailings dams: characteristics, failure, environmental impacts, and remediation. Appl. Geochem. 51, 229–245. doi: 10.1016/j.apgeochem.2014.09.010
Lee, S. H., Park, H., Koo, N., Hyun, S., and Hwang, A. (2011). Evaluation of the effectiveness of various amendments on trace metals stabilization by chemical and biological methods. J. Hazard. Mater. 188, 44–51. doi: 10.1016/j.jhazmat.2011.01.046
Ma, Y., Prasad, M. N. V., Rajkumar, M., and Freitas, H. (2011a). Plant growth promoting rhizobacteria and endophytes accelerate phytoremediation of metalliferous soils. Biotechnol. Adv. 29, 248–258. doi: 10.1016/j.biotechadv.2010.12.001
Ma, Y., Rajkumar, M., Luo, Y. M., and Freitas, H. (2011b). Inoculation of endophytic bacteria on host and non-host plants – effects on plant growth and Ni uptake. J. Hazard. Mater. 196, 230–237. doi: 10.1016/j.jhazmat.2011.08.034
Ma, Y., Rajkumar, M., and Freitas, H. (2009). Isolation and characterization of Ni mobilizing PGPB from serpentine soils and their potential in promoting plant growth and Ni accumulation by Brassica spp. Chemosphere 75, 719–725. doi: 10.1016/j.chemosphere.2009.01.056
Ma, Y., Rajkumar, M., Rocha, I., Oliveira, R. S., and Freitas, H. (2015). Serpentine bacteria influence metal translocation and bioconcentration of Brassica juncea and Ricinus communis grown in multi-metal polluted soils. Front. Plant Sci. 5:757. doi: 10.3389/fpls.2014.00757
Malik, R. N., Husain, S. Z., and Nazir, I. (2010). Heavy metal contamination and accumulation in soil and wild plant species from industrial area of Islamabad, Pakistan. Pak. J. Bot. 42, 123–127.
Mendez, M. O., and Maier, R. M. (2008). Phytostabilization of mine tailings in arid and semiarid environments – an emerging remediation technology. Environ. Health Perspect. 116, 278–283. doi: 10.1289/ehp.10608
Mesa, J., Rodríguez-Llorente, I. D., Pajuelo, E., Piedras, J. M., Caviedes, M. A., Redondo-Gómez, S., et al. (2015). Moving closer towards restoration of contaminated estuaries: bioaugmentation with autochthonous rhizobacteria improves metal rhizoaccumulation in native Spartina maritima. J. Hazard. Mater. 300, 263–271. doi: 10.1016/j.jhazmat.2015.07.006
Moreno, M. E., Acosta-Saavedra, L. C., Meza-Figueroa, D., Vera, E., Cebrian, M. E., Ostrosky-Wegman, P., et al. (2010). Biomonitoring of metal in children living in a mine tailings zone in Southern Mexico: a pilot study. Int. J. Hyg. Environ. Health 213, 252–258. doi: 10.1016/j.ijheh.2010.03.005
Park, J. H., Bolan, N., Mallavarapu, M., and Naidu, R. (2011). Isolation of phosphate solubilizing bacteria and their potential for lead immobilization in soil. J. Hazard. Mater. 185, 829–836. doi: 10.1016/j.jhazmat.2010.09.095
Rajkumar, M., Ae, N., and Freitas, H. (2009). Endophytic bacteria and their potential to enhance heavy metal phytoextraction. Chemosphere 77, 153–160. doi: 10.1016/j.chemosphere.2009.06.047
Rajkumar, M., Ma, Y., and Freitas, H. (2008). Characterization of metal-resistant plant growth promoting Bacillus weihenstephanensis isolated from serpentine soil in Portugal. J. Basic Microbiol. 48, 1–9. doi: 10.1002/jobm.200800073
Raskin, I., and Ensley, B. D. (2000). Phytoremediation of Toxic Metals: Using Plants to Clean Up the Environment. New York, NY: John Wiley and Sons, 303.
Ribeiro Filho, M. R., Siqueira, J. O., Vangronsveld, J., Soares, C. R., and Curi, N. (2011). Inorganic materials as ameliorants for soil remediation of metal toxicity to wild mustard (Sinapis arvensis L.). Int. J. Phytoremediation 13, 498–512. doi: 10.1080/15226511003753938
Schwyn, B., and Neilands, J. B. (1987). Universal chemical assay for the detection and determination of siderophores. Anal. Biochem. 160, 47–56. doi: 10.1016/0003-2697(87)90612-9
Shin, M., Shim, J., You, Y., Myung, H., Bang, K. S., Cho, M., et al. (2012). Characterization of lead resistant endophytic Bacillus sp. MN3-4 and its potential for promoting lead accumulation in metal hyperaccumulator Alnus firma. J. Hazard. Mater. 19, 314–320. doi: 10.1016/j.jhazmat.2011.11.010
Srivastava, S., Verma, P. C., Chaudhry, V., Singh, N., Abhilash, P. C., Kumar, K. V., et al. (2013). Influence of inoculation of arsenic-resistant Staphylococcus arlettae on growth and arsenic uptake in Brassica juncea (L.) Czern. Var. R-46. J. Hazard. Mater. 262, 1039–1047. doi: 10.1016/j.jhazmat.2012.08.019
Sundara-Rao, W. V. B., and Sinha, M. K. (1963). Phosphate dissolving microorganisms in the soil and rhizosphere. Indian J. Agr. Sci. 33, 272–278.
Sura-de Jong, M., Reynolds, R. J. B., Richterova, K., Musilova, L., Staicu, L. C., Chocholata, I., et al. (2015). Selenium hyperaccumulators harbor a diverse endophytic bacterial community characterized by high selenium resistance and plant growth promoting properties. Front. Plant Sci. 6:113. doi: 10.3389/fpls.2015.00113
Visioli, G., DEgidio, S., Vamerali, T., Mattarozzi, M., and Sanangelantoni, A. M. (2014). Culturable endophytic bacteria enhance Ni translocation in the hyperaccumulator Noccaea caerulescens. Chemosphere 117, 538–544. doi: 10.1016/j.chemosphere.2014.09.014
Keywords: endophytic bacterium, rhizoaccumulation, multi-metal contamination, phytostabilization, Sedum plumbizincicola
Citation: Ma Y, Zhang C, Oliveira RS, Freitas H and Luo Y (2016) Bioaugmentation with Endophytic Bacterium E6S Homologous to Achromobacter piechaudii Enhances Metal Rhizoaccumulation in Host Sedum plumbizincicola. Front. Plant Sci. 7:75. doi: 10.3389/fpls.2016.00075
Received: 25 November 2015; Accepted: 16 January 2016;
Published: 04 February 2016.
Edited by:
Vincenzo Lionetti, Sapienza – Universitá di Roma, ItalyReviewed by:
Vijai Kumar Gupta, National University of Ireland Galway, IrelandCopyright © 2016 Ma, Zhang, Oliveira, Freitas and Luo. This is an open-access article distributed under the terms of the Creative Commons Attribution License (CC BY). The use, distribution or reproduction in other forums is permitted, provided the original author(s) or licensor are credited and that the original publication in this journal is cited, in accordance with accepted academic practice. No use, distribution or reproduction is permitted which does not comply with these terms.
*Correspondence: Ying Ma, Y2F0aHltYXlpbmdAZ21haWwuY29t
Disclaimer: All claims expressed in this article are solely those of the authors and do not necessarily represent those of their affiliated organizations, or those of the publisher, the editors and the reviewers. Any product that may be evaluated in this article or claim that may be made by its manufacturer is not guaranteed or endorsed by the publisher.
Research integrity at Frontiers
Learn more about the work of our research integrity team to safeguard the quality of each article we publish.