- 1Department of Wildlife, Fish, and Environmental Studies, Swedish University of Agricultural Sciences, Umeå, Sweden
- 2Department of Forest Ecology and Management, Swedish University of Agricultural Sciences, Umeå, Sweden
Climate change, as well as a more intensive forestry, is expected to increase the risk of damage by pests and pathogens on trees, which can already be a severe problem in tree plantations. Recent development of biotechnology theoretically allows for resistance enhancement that could help reduce these risks but we still lack a comprehensive understanding of benefits and tradeoffs with pest resistant GM (genetically modified) trees. We synthesized the current knowledge on the effectiveness of GM forest trees with increased resistance to herbivores. There is ample evidence that induction of exogenous Bacillus thuringiensis genes reduce performance of target pests whereas upregulation of endogenous resistance traits e.g., phenolics, generates variable results. Our review identified very few studies estimating the realized benefits in tree growth of GM trees in the field. This is concerning as the realized benefit with insect resistant GM plants seems to be context-dependent and likely manifested only if herbivore pressure is sufficiently high. Future studies of secondary pest species and resistance evolution in pest to GM trees should be prioritized. But most importantly we need more long-term field tests to evaluate the benefits and risks with pest resistant GM trees.
Introduction
There is an urgent need to find alternatives to fossil fuels to reduce our input of CO2 into the atmosphere and thus mitigate climate change. It has been estimated that trees can become a major source of bioenergy and help mitigate the anticipated rise in CO2 over the next 50 years (Smeets and Faaij, 2007). This may require more intense management practices, such as the use of trees as short rotation energy crops. However, intensified forestry is expected to increase the risk of damage by pests and pathogens on trees (Klapwijk et al., 2013). Pests can already be a severe problem in tree plantations (Gruppe et al., 1999) and pest problems are expected to increase due to ongoing climate change (Klapwijk et al., 2013) (Figure 1). Tree pests can severely effect growth and survival of forest trees and thus inflict large economic losses (Ayres and Lombardero, 2000).
In general only a few herbivores on a particular tree species develop into pests. For example, only a small number of Populus-feeding insects are regarded as serious pests in North America. But these insects can severely hamper establishment, reduce growth, and increase mortality in managed stands (Dickmann and Stuart, 1983; Coyle et al., 2002; Nordman et al., 2005). Important pests on conifers in North America include both defoliating insects (e.g., pine sawflies) and cambium consumers (bark beetles) (Dukes et al., 2009). In China, damage to hybrid Populus plantations by the poplar lopper (Apochemia cinerarua) and the gypsy moth (Lymantria dispar) can inflict substantial (up to 40%) stand losses (Hu et al., 2001). Similarly, in Europe Gruppe et al. (1999) reported up to 50% leaf damage on some aspen clones and that a large majority of damage was inflicted by a few but abundant pest species, such as the leaf beetles Chrysomela tremulae and Phratora vitellinae, and the leaf roller Byctiscus populi (Curculionidae: Coleoptera). Coyle et al. (2002) found that leaf beetles damage reduced above ground biomass of aspens by 50–73%. Bark beetles, pine weevils, and pine moths are important pests on conifers in Europe and the impact of pests on forests are expected to increase in a warming climate (Leather et al., 1999; Jacquet et al., 2012; Marini et al., 2012).
The expected increasing impact of pests could uncontrolled cause severe problems in the form of reduced production, lower economic yield, and profoundly reduce our ability to replace fossil fuels with bioenergy. It could also result in increased use of pesticides which could have negative effects on the environment. It is therefore of outmost importance that we find other means to control pests and pathogens on trees. This likely calls for developments in a range of fields including changes to management practices and technology but also improvements in tree characteristics through genetic enhancements (Smeets and Faaij, 2007; Fenning et al., 2008).
With the recent developments in biotechnology, genetic enhancement of trees theoretically allows modification of most individual traits in selected genotypes. As a result, GM (gene modification) technology is much more specific and is not hampered by the constraint of traditional breeding, e.g., late flowering, slow maturation, long reproductive cycles, and complex mating systems (including self-incompatibility and a high degree of heterozygosity) in trees. Difficulties in identifying the best parents (and controlling their mating), maintaining genetic gain with high heterozygosity (Cheliak and Rogers, 1990), and understanding the complex genomes of many tree species causes problems for tree breeders. Consequently, tree breeding is a slow process that generally, in a short time span, only allows for minor improvements of tree resistance (Ye et al., 2011). By contrast, through genetic engineering, it is possible to introduce novel traits as well as regulate native traits and thus change plant expression of various biochemicals, which allow new strategies for breeding (FAO, 2004).
Two principally different strategies are used to modify plants for enhanced resistance. The first is to up-regulate innate resistance traits (endogenous traits) such as phenolics and the second is to introduce new traits (exogenous traits) such as the production of Bacillus thuringiensis (hereafter Bt) toxins. The principal difference between these two approaches is that endogenous traits are something that herbivores have evolved mechanisms to deal with, e.g., some specialists use plant derived chemicals for their own defense (Pasteels et al., 1983). Typically these defenses work as a deterrent or by reducing growth rate of herbivores. Exogenous traits on the other hand are completely novel to the herbivores and their inherent ability for resistance are expected to be restricted (but see for example Genissel et al., 2003) and exogenous traits often cause high mortality in targeted pests. Thus, these two GM approaches can be expected to have different effects on herbivore guilds.
However, even if GM trees with increased resistance to herbivores have been available for more than 25 years, we still lack knowledge on the efficiency of different modifications for resistance in reducing damage by forest pests and thereby enhancing tree growth. Lab studies evaluating resistance are relatively common but field studies are rare (Ye et al., 2011). Still, before a commercial release of GM trees, it is important that benefits and risk are balanced and for that we need a proper understanding of the benefits as well as the risks of GM trees.
Aim
The aim of this study was to synthesize the current knowledge on the effectiveness of GM forest trees (fruit trees are not included in our study) with increased resistance to herbivores. More specifically we ask how effective different types of enhanced resistance are at reducing damage by pests and how well this translates into increased growth and production in GM trees. In addition, based on the above synthesis we highlight areas of uncertainty and suggest future directions for resistance enhancement in GM trees.
To collect the necessary literature we used the following search string in Web of Science: Tree and [(Transgenic, GMO, GE, or GM) and (resistance) and (herbivores)]. Selected articles included studies on preference and performance of herbivores, and damage on plants and effects on plant performance in terms of growth and biomass production.
Current Knowledge
Populus became the first genera to be genetically transformed and regenerated (Fillatti et al., 1987) and it now serves as the leading model system in forest biotechnology (FAO, 2010; Ye et al., 2011). Ye et al. (2011) reviewed different applications of genetic modifications in Populus and showed that in this single tree taxon, modified traits include; insect- herbicide- and disease-resistance, tolerance toward different abiotic stresses, wood properties, and growth (i.e., growth-rate, rooting, and flowering). In general, field studies with GM forest trees are restricted to a few species, with Populus species clearly dominating (Häggman et al., 2013). Other genera studied in field experiments include Pinus, Liquidambar, and Eucalyptus, and, more recently, Picea, and Betula (Häggman et al., 2013). The majority of field studies address questions related to methods (e.g., gene stability, gene expression) or traits related to herbicide resistance, wood quality, or wood chemistry and very few deal with pest resistance (FAO, 2010; Häggman et al., 2013). Our review on resistance in GM trees revealed only three “true” field studies and one study conducted with potted plants in “semi-natural” environments and all of these addressed pest resistance in Populus sp.
Effects in Target and Non-target Pests
The singly most common transformation for pest resistance involves the introduction of exogenous Bt genes, enabling the plant to produce Cry toxins lethal to certain targeted insect pests. More than 150 different Cry proteins have been identified (Schnepf et al., 1998), with examples including Cry3Aa proteins targeting coleopteran insects and the Cry1 and Cry2 families effective against lepidopteran species (Hu et al., 2001; Hussein et al., 2005). Other exogenous defenses include scorpion neurotoxin (Ye et al., 2011) and tobacco anionic peroxidase (Dowd et al., 1998).
The effectiveness of Bt toxins against specific pest species on trees has been tested and verified both in laboratory (Genissel et al., 2003; Kleiner et al., 2003) and field studies (Hu et al., 2001; Ye et al., 2011). Most studies report significant reduction of consumption and performance of target insect pests on Bt trees. Genissel et al. (2003) found that ingestion of leaves from Bt aspens induced 100% mortality in Chrysomela tremulae within 2–13 days depending on instar, with older instars and adult beetles living the longest. Bt induction in Pinus radiata induced up to 80% mortality in larvae of the painted apple moth, Teia anartoides (Grace et al., 2005). Similar results have been found in other studies of both conifers and deciduous trees (Shin et al., 1994; Kleiner et al., 1995; Harcourt et al., 2000; Tang and Tian, 2003; Lachance et al., 2007; Zhang et al., 2011) (Table 1). Thus, there is ample evidence that Bt have a strong impact on survival and performance of target pests and that even short time Bt exposure can cause long lasting effects (Hjälten et al., 2013). However, Axelsson et al. (2012) found that the density of one species from the targeted order, the leaf rolling beetle Byctiscus populi (Coleoptera), was unaffected on Bt aspens. Furthermore, non-target herbivores belonging to different genera than the target pests seem unaffected by Bt plants (Ye et al., 2011; Zhang et al., 2011) or even prefer them (Axelsson et al., 2011). Induction of exogenous tobacco anionic peroxidase in GM Liquidambar styraciflua had variable effects on the herbivore guild. It resulted in negative effects on the preference/performance of some herbivores but others preferred GM trees (Dowd et al., 1998).
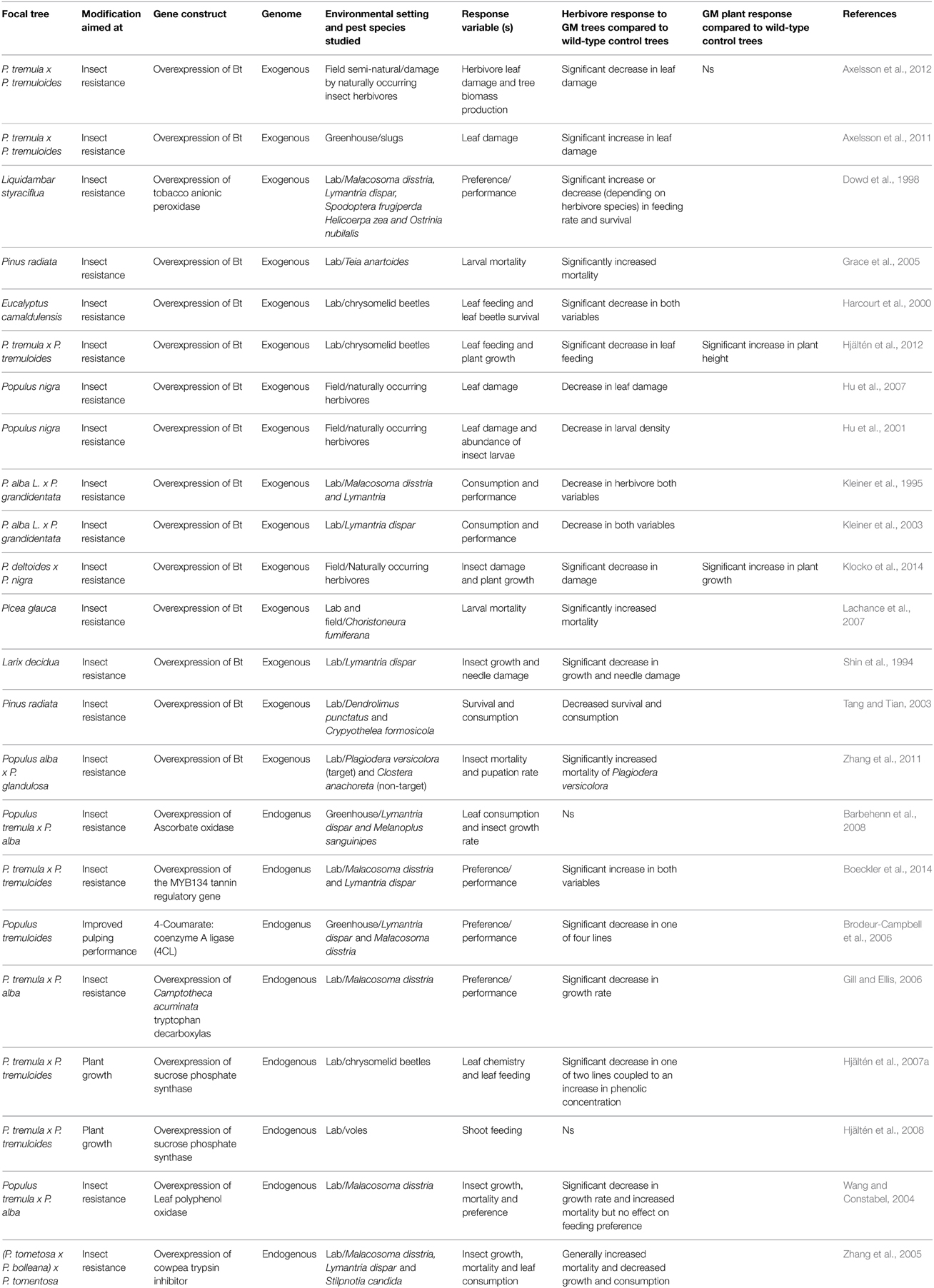
Table 1. Summary of studies addressing herbivore preference/performance on GM trees and/or GM plant performance in the presence of herbivores.
Up-regulation of endogenous defenses in GM trees include e.g., different types of plant phenolics, that are known to act as plant defenses against tree pests (Miranda et al., 2007; Barbehenn and Constabel, 2011). GM trees with overexpression of condensed tannins, proteinase inhibitor (Ye et al., 2011), leaf polyphenol oxidase (Wang and Constabel, 2004) and trypsin inhibitors (Zhang et al., 2005) has therefore been produced. Although up-regulation of endogenous defenses can be effective, e.g., expression of tryptophan decarboxylase gene in aspens reduced the growth rate of Malacosoma disstria (Gill and Ellis, 2006), the effects may not always be consistent. Boeckler et al. (2014) reported that up-regulation of condensed tannins synthesis increased performance and leaf consumption by Malacosoma disstria and Lymantria dispar. This could potentially be explained by reduced levels of phenolic glucosides in GM lines (a consequence of the up-regulation of condensed tannin synthesis) as phenolic glucosides can deter herbivores (Boeckler et al., 2014). Furthermore, increased synthesis of ascorbate oxidase in GM aspen had no significant effect on insect pests (Barbehenn et al., 2008).
Thus, the outcome of induction of exogenous such as Bt on pest resistance seem more consistence than induction of endogenous traits. However, one reason for this pattern could be that modifications of endogenous traits on pest resistance have been much less studied than the effects of e.g., Bt induction. Thus, more studies on enhancement of endogenous defense traits, including indirect defenses (e.g., traits attracting natural enemies to herbivores) are urgently needed. Still, potential drawback with the use of endogenous defenses is the intraspecific variation in insect adaptation to endogenous plant defenses (Pentzold et al., 2014). Also, coevolution between certain herbivores and their host may cause defensive substances previously working deterrent to the herbivore to act as feeding stimulants (Hjältén et al., 2007b). Consequently specialist and generalist herbivores may respond differently to changes in the expression of plant defenses (Hjältén et al., 2007b; Boeckler et al., 2011). Potential drawback with exogenous traits like Bt is evolution of Bt resistance in target pests (Tabashnik et al., 2013). In addition, due to the high specificity of Bt, outbreaks of secondary non-targets pest could become a severe problem (Dorhout and Rice, 2010). There are also indications that Bt and tannins interact antagonistically with respect to herbivore resistance, i.e., that tannins reduced the negative effect of Bt on the performance and survival of spruce budworm (Bauce et al., 2006). However, by contrast (Guan et al., 2009; Delvas et al., 2011) found synergistic effects of tannins and Bt on Helicoverpa armigera, an important pest on cotton. Thus, the combined effect of exogenous and endogenous defense traits is unclear.
Careful examination of indirect metabolic changes in transformed lines is also important for proper assessment of benefits and risks. Inconsistencies in how pests respond to genetic modifications may stem from unintended changes in other traits of importance for plant resistance. Events in the transformation process may cause variability in gene expression or gene silencing and have secondary, unintended effects on plant physiology and innate resistance traits. Transformations intended to increase growth or improve pulping performance can induce unintended effects on tree resistance to pests and pathogens (Brodeur-Campbell et al., 2006; Hjältén et al., 2007a). As unintended effects could have both positive and negative effects on plant resistance, they need to be considered in evaluation of GM trees (Brodeur-Campbell et al., 2006; Hjältén et al., 2007a; Axelsson et al., 2011).
Realized Benefits in Tree Growth
Although both field-and lab experiments suggest a high efficiency of GM resistance traits against target herbivores (Hu et al., 2001; Balestrazzi et al., 2006), our review identified very few studies estimating the realized benefits in tree growth (Table 1). Significant reduced leaf damage on Bt trees have been found in both lab and greenhouse studies (Harcourt et al., 2000; Hjältén et al., 2012) as well as in field studies (Hu et al., 2001; Axelsson et al., 2012). This sometimes translates to higher growth in Bt plants (Hjältén et al., 2012; Klocko et al., 2014) but this is not always the case (Axelsson et al., 2012). One likely explanation for the lack of growth response could be that the damage levels are too low to induce reduced growth in wildtype plants and under such circumstances Bt induction provides no growth benefits (Axelsson et al., 2012). Leaf damage levels by insects in aspen plantations range between 3.8% and ca 50% (Gruppe et al., 1999; Coyle et al., 2002; Tomescu and Nef, 2007). The damage level in Axelsson et al. (2012) was only ~3.5%, probably too low to have any significant impact on tree biomass growth. A plants ability to compensate for herbivory damage depends both on the type and timing of damage as well as the nutritional status of plants (Hjältén et al., 1993; Anttonen et al., 2002) and young trees can compensate for 25% leaf loss if well fertilized (Anttonen et al., 2002). Thus, the realized benefit with insect resistant GM plants is context-dependent and is likely to be manifested only if herbivore pressure is sufficiently high (Hjältén et al., 2012). Klocko et al. (2014) reported in excess of 25% leaf damage on wildtype aspen, and as a likely consequence of the high herbivore pressure, they found 14% higher biomass in Bt aspens compared to the wildtype under field conditions.
Knowledge Gaps
From this short review it is clear that we need more field studies and especially long-term studies of growth benefits of pest resistant GM trees. Often the effects in controlled environments such as the lab or greenhouse cannot be extrapolated to natural environments. Also, when time is scaled up, predictability is reduced (Raffa, 2001). Without knowledge of long-term benefits of GM trees under different growing conditions, it is impossible to conduct proper cost-benefit analyses. However, our ability to conduct long term field experiments are often limited by the strict regulation for field trials with GM trees (Häggman et al., 2013). The study by Klocko et al. (2014) is one of the few field studies of realized benefits spanning more than one field season. Given that trees are long lived organisms associated with a myriad of species, the circumstances faced by a tree throughout its growing cycle may reduce our ability to predict the realized effect in the field.
Secondary pest species could reduce the benefits of insect resistant GM trees. Raffa (1989) suggested that the effective prevention of dominant pest species should benefit less dominant natural enemies. Experiences from crop systems support this prediction, Dorhout and Rice (2010) demonstrated enhanced survival of, and a corresponding range shift in Western bean cutworm (Striacosta albicosta) foraging on Bt corn lacking the target pest species European corn borer (Ostrinia nubilalis) and corn earworm (Helicoverpa zea). Similarly, Lu et al. (2010) found progressive increased population sizes of Mirid bugs in cotton and other crops, associated with a regional increase in Bt cotton which was adopted to target cotton bollworm (Helicoverpa armigera). Thus, secondary pest species could develop into a major problem in GM tree systems and strategies to counteract such development should be developed.
Resistance evolution in pests is a topic of debate which may be of special concern when it comes to long lived crops such as trees. Resistance evolution in pests and weeds toward different pesticide treatments are not uncommon (Tong et al., 2013) and the number of examples of pests evolving resistance toward insect resistant GM plants expressing Bt toxins are also growing. Tabashnik et al. (2011) reported reduced efficiency of Bt crops caused by field-evolved resistance in 5 out of 13 examined pest species. For trees, the poplar leaf beetle Chrysomela tremulae rapidly evolved resistance to sprays of Bt toxins in the laboratory and in the field (Augustin et al., 2004), which underscores that resistance development remains a serious concern and stresses the need for resistance management of Bt plants.
Most studies of resistance in GM trees have been conducted on deciduous trees. However, many of the most commercially important forest trees are conifer. Furthermore, many of the pest species on conifers are not leaf-feeders but rather cambium feeders, e.g., bark beetles. It is unclear how they might respond to induction of resistance traits in growing tissue, such as leaves, because other defense mechanisms, such as resin flow, confer resistance against these types of herbivores (Boone et al., 2011). Thus, more focus should be on evaluating the benefits of different types of GM conifers with respect to pest resistance.
Ways Forward
The knowledge gaps identified in this review involves the efficiency of upregulated endogenous defenses on pest species. As some specialist pest species are adapted to deal with some types of plant defenses and even use them as defenses themselves, their response to resistance enhancements might not be the expected (Pentzold et al., 2014). This also has implications for traditional resistance breeding and explains why this method only allows for minor improvements of tree resistance. In that respect, exogenous resistance traits hold better promises of, at least initially, higher efficiency. However, the problem with, e.g., Bt is that it targets specific taxa, and this infers a risk of secondary pest outbreaks. Thus, a combination of induction of both exogenous and endogenous resistant traits in GM trees might be advantageous as it might reduce the risk for outbreaks of secondary pests, as can be inferred from studies in crop systems (Ma et al., 2014). The development of GM technology for increased pest resistance likely need to be tackled on a broad front that includes the merging of several research fields, such as molecular biology, plant physiology, chemical ecology, and community ecology. In the end we will also rely on long-term field experiments for a complete understanding of the long term benefits and potential risk with the use of GM trees. Long-term field experiments in natural settings are essential for our understanding of the risks for secondary pest outbreaks or resistance evolution in pests, and are thus instrumental for developing efficient management practices that deal with these and other potential problems related to the use of GM trees. In addition, long-term field experiments are also essential for ecological safety assessments of GM trees (e.g., potential effects of non-target species or important ecosystem processes) and thus for our ability to balance benefits and risks with GM trees.
Conflict of Interest Statement
The authors declare that the research was conducted in the absence of any commercial or financial relationships that could be construed as a potential conflict of interest.
Acknowledgments
We thank the research council FORMAS for economic support.
References
Anttonen, S., Piispanen, R., Ovaska, J., Mutikainen, P., Saranpaa, P., and Vapaavuori, E. (2002). Effects of defoliation on growth, biomass allocation, and wood properties of Betula pendula clones grown at different nutrient levels. Can. J. For. Res. Rev. 32, 498–508. doi: 10.1139/x01-217
Augustin, S., Courtin, C., Rejasse, A., Lorme, P., Genissel, A., and Bourguet, D. (2004). Genetics of resistance to transgenic Bacillus thuringiensis poplars in Chrysomela tremulae (Coleoptera: Chrysomelidae). J. Econ. Entomol. 97, 1058–1064. doi: 10.1093/jee/97.3.1058
PubMed Abstract | Full Text | CrossRef Full Text | Google Scholar
Axelsson, E. P., Hjältén, J., and Leroy, C. J. (2012). Performance of insect-resistant Bacillus thuringiensis (Bt)-expressing aspens under semi-natural field conditions including natural herbivory in Sweden. For. Ecol. Manag. 264, 167–171. doi: 10.1016/j.foreco.2011.10.006
Axelsson, E. P., Hjältén, J., Whitham, T. G., Julkunen-Tiitto, R., Pilate, G., and Wennström, A. (2011). Leaf ontogeny interacts with Bt modification to affect innate resistance in GM aspens. Chemoecology 21, 161–169. doi: 10.1007/s00049-011-0080-8
Ayres, M. P., and Lombardero, M. J. (2000). Assessing the consequences of global change for forest disturbance from herbivores and pathogens. Sci. Total Environ. 262, 263–286. doi: 10.1016/S0048-9697(00)00528-3
PubMed Abstract | Full Text | CrossRef Full Text | Google Scholar
Balestrazzi, A., Allegro, G., and Confalonieri, M. (2006). “Genetically modified trees expressing genes for insect pest resistance,” in Tree Transgenesis: Recent Developments, eds M. Fladung and D. Ewald (Berlin: Springer), 253–273.
Barbehenn, R. V., and Constabel, C. P. (2011). Tannins in plant-herbivore interactions. Phytochemistry 72, 1551–1565. doi: 10.1016/j.phytochem.2011.01.040
PubMed Abstract | Full Text | CrossRef Full Text | Google Scholar
Barbehenn, R. V., Jaros, A., Yip, L., Tran, L., Kanellis, A. K., and Constabel, C. P. (2008). Evaluating ascorbate oxidase as a plant defense against leaf-chewing insects using transgenic poplar. J. Chem. Ecol. 34, 1331–1340. doi: 10.1007/s10886-008-9539-7
PubMed Abstract | Full Text | CrossRef Full Text | Google Scholar
Bauce, E., Kumbasli, M., van Frankenhuyzen, K., and Carisey, N. (2006). Interactions among white spruce tannins, Bacillus thuringiensis subsp kurstaki, and spruce budworm (Lepidoptera: Tortricidae), on larval survival, growth, and development. J. Econ. Entomol. 99, 2038–2047. doi: 10.1093/jee/99.6.2038
PubMed Abstract | Full Text | CrossRef Full Text | Google Scholar
Boeckler, G. A., Gershenzon, J., and Unsicker, S. B. (2011). Phenolic glycosides of the Salicaceae and their role as anti-herbivore defenses. Phytochemistry 72, 1497–1509. doi: 10.1016/j.phytochem.2011.01.038
PubMed Abstract | Full Text | CrossRef Full Text | Google Scholar
Boeckler, G. A., Towns, M., Unsicker, S. B., Mellway, R. D., Yip, L., Hilke, I., et al. (2014). Transgenic upregulation of the condensed tannin pathway in poplar leads to a dramatic shift in leaf palatability for two tree-feeding Lepidoptera. J. Chem. Ecol. 40, 150–158. doi: 10.1007/s10886-014-0383-7
PubMed Abstract | Full Text | CrossRef Full Text | Google Scholar
Boone, C. K., Aukema, B. H., Bohlmann, J., Carroll, A. L., and Raffa, K. F. (2011). Efficacy of tree defense physiology varies with bark beetle population density: a basis for positive feedback in eruptive species. Can. J. For. Res. Rev. 41, 1174–1188. doi: 10.1139/x11-041
Brodeur-Campbell, S. E., Vucetich, J. A., Richter, D. L., Waite, T. A., Rosemier, J. N., and Tsai, C. J. (2006). Insect herbivory on low-lignin transgenic aspen. Environ. Entomol. 35, 1696–1701. doi: 10.1093/ee/35.6.1696
Cheliak, W. M., and Rogers, D. L. (1990). Integrating biotechnology into tree improvement programs. Can. J. For. Res. Rev. 20, 452–463. doi: 10.1139/x90-062
Coyle, D. R., McMillin, J. D., Hall, R. B., and Hart, E. R. (2002). Cottonwood leaf beetle (Coleoptera; Chrysomelidae) defoliation impact on Populus growth and above-ground volume in a short-rotation woody crop plantation. Agric. For. Entomol. 4, 293–300. doi: 10.1046/j.1461-9563.2002.00149.x
Delvas, N., Bauce, E., Labbe, C., Ollevier, T., and Belanger, R. (2011). Phenolic compounds that confer resistance to spruce budworm. Entomol. Exp. Appl. 141, 35–44. doi: 10.1111/j.1570-7458.2011.01161.x
Dickmann, D. I., and Stuart, K. W. (1983). The Culture of Poplars in Eastern North America. East Lansing, MI: Department of Forestry, Michigan State University.
Dorhout, D. L., and Rice, M. E. (2010). Intraguild competition and enhanced survival of western bean cutworm (Lepidoptera: Noctuidae) on transgenic Cry1Ab (MON810) Bacillus thuringiensis corn. J. Econ. Entomol. 103, 54–62. doi: 10.1603/EC09247
PubMed Abstract | Full Text | CrossRef Full Text | Google Scholar
Dowd, P. F., Lagrimini, L. M., and Herms, D. A. (1998). Differential leaf resistance to insects of transgenic sweetgum (Liquidambar styraciflua) expressing tobacco anionic peroxidase. Cell. Mol. Life Sci. 54, 712–720. doi: 10.1007/s000180050198
PubMed Abstract | Full Text | CrossRef Full Text | Google Scholar
Dukes, J. S., Pontius, J., Orwig, D., Garnas, J. R., Rodgers, V. L., Brazee, N., et al. (2009). Responses of insect pests, pathogens, and invasive plant species to climate change in the forests of northeastern North America: what can we predict? Can. J. For. Res. Rev. 39, 231–248. doi: 10.1139/X08-171
FAO. (2004). Preliminary Review of Biotechnology in Forestry, including Genetic Modification. Forest Genetic Resources Working Paper FGR/59E. Rome: Forest Resources Development Service, Forest Resources Division.
Fenning, T. M., Walter, C., and Gartland, K. M. A. (2008). Forest biotech and climate change. Nat. Biotechnol. 26, 615–617. doi: 10.1038/nbt0608-615
PubMed Abstract | Full Text | CrossRef Full Text | Google Scholar
Fillatti, J. J., Sellmer, J., McCown, B., Haissig, B., and Comai, L. (1987). Agrobacterium mediated transformation and regeneration of Populus. Mol. Gen. Genet. 206, 192–199. doi: 10.1007/BF00333574
Genissel, A., Leple, J. C., Millet, N., Augustin, S., Jouanin, L., and Pilate, G. (2003). High tolerance against Chrysomela tremulae of transgenic poplar plants expressing a synthetic cry3Aa gene from Bacillus thuringiensis ssp tenebrionis. Mol. Breed. 11, 103–110. doi: 10.1023/A:1022453220496
Gill, R. I. S., and Ellis, B. E. (2006). Over-expression of tryptophan decarboxylase gene in poplar and its possible role in resistance against Malacosoma disstria. New For. 31, 195–209. doi: 10.1007/s11056-005-2719-1
Grace, L. J., Charity, J. A., Gresham, B., Kay, N., and Walter, C. (2005). Insect-resistant transgenic Pinus radiata. Plant Cell Rep. 24, 103–111. doi: 10.1007/s00299-004-0912-x
PubMed Abstract | Full Text | CrossRef Full Text | Google Scholar
Gruppe, A., Fusseder, M., and Schopf, R. (1999). Short rotation plantations of aspen and balsam poplar on former arable land in Germany: defoliating insects and leaf constituents. For. Ecol. Manag. 121, 113–122. doi: 10.1016/S0378-1127(98)00560-X
Guan, X., Liu, X., Lu, Z., Zhao, Z., Zhang, Q., and Xia, J. (2009). Influence of tannic acid and Cry1Ac toxin of Bacillus thuringiensis on larval survival, growth, and development of Helicoverpa armigera. Entomol. Exp. Appl. 132, 50–58. doi: 10.1111/j.1570-7458.2009.00866.x
Häggman, H., Raybould, A., Borem, A., Fox, T., Handley, L., Hertzberg, M., et al. (2013). Genetically engineered trees for plantation forests: key considerations for environmental risk assessment. Plant Biotechnol. J. 11, 785–798. doi: 10.1111/pbi.12100
PubMed Abstract | Full Text | CrossRef Full Text | Google Scholar
Harcourt, R. L., Kyozuka, J., Floyd, R. B., Bateman, K. S., Tanaka, H., Decroocq, V., et al. (2000). Insect- and herbicide-resistant transgenic eucalypts. Mol. Breed. 6, 307–315. doi: 10.1023/A:1009676214328
Hjälten, J., Axelsson, E. P., Julkunen-Tiitto, R., Wennstrom, A., and Pilate, G. (2013). Innate and introduced resistance traits in genetically modified aspen trees and their effect on leaf beetle feeding. PLoS ONE 8:73819. doi: 10.1371/journal.pone.0073819
PubMed Abstract | Full Text | CrossRef Full Text | Google Scholar
Hjältén, J., Axelsson, E. P., Whitham, T. G., Leroy, C. J., Julkunen-Tiitto, R., Wennström, A., et al. (2012). Increased resistance of Bt aspens to Phratora vitellinae (Coleoptera) leads to increased plant growth under experimental conditions. PLoS ONE 7:30640. doi: 10.1371/journal.pone.0030640
PubMed Abstract | Full Text | CrossRef Full Text | Google Scholar
Hjältén, J., Danell, K., and Ericson, L. (1993). Effects of simulated herbivory and intraspecific competiotion on the compensatory ability of birches. Ecology 74, 1136–1142. doi: 10.2307/1940483
Hjältén, J., Lindau, A., Wennström, A., Blomberg, P., Witzell, J., Hurry, V., et al. (2007a). Unintentional changes of defence traits in GM trees can influence plant-herbivore interactions. Basic Appl. Ecol. 8, 434–443. doi: 10.1016/j.baae.2006.09.001
PubMed Abstract | Full Text | CrossRef Full Text | Google Scholar
Hjältén, J., Lindau, A., Wennstrom, A., Blomberg, P., Witzell, J., Hurry, V., et al. (2008). Vole response to unintentional changes in the chemistry of GM poplars. Chemoecology 18, 227–231. doi: 10.1007/s00049-008-0409-0
Hjältén, J., Niemi, L., Wennström, A., Ericson, L., Roininen, H., and Julkunen-Tiitto, R. (2007b). Variable responses of natural enemies to Salix triandra phenotypes with different secondary chemistry. Oikos 116, 751–758. doi: 10.1111/j.0030-1299.2007.15365.x
Hu, J. J., Tian, Y. C., Han, Y. F., Li, L., and Zhang, B. E. (2001). Field evaluation of insect-resistant transgenic Populus nigra trees. Euphytica 121, 123–127. doi: 10.1023/A:1012015709363
Hu, J., Li, S., Lu, M., Li, J., Li, K., Sun, X., et al. (2007). Stability of insect-resistance of Bt transformed Populus nigra plantation and its effects on the natural enemies of insects. Forest Res. 20, 656–659.
Hussein, H. M., Habustova, O., and Sehnal, F. (2005). Beetle-specific Bacillus thuringiensis Cry3Aa toxin reduces larval growth and curbs reproduction in Spodoptera littoralis (Boisd.). Pest Manag. Sci. 61, 1186–1192. doi: 10.1002/ps.1112
PubMed Abstract | Full Text | CrossRef Full Text | Google Scholar
Jacquet, J.-S., Orazio, C., and Jactel, H. (2012). Defoliation by processionary moth significantly reduces tree growth: a quantitative review. Ann. For. Sci. 69, 857–866. doi: 10.1007/s13595-012-0209-0
Klapwijk, M. J., Csoka, G., Hirka, A., and Bjorkman, C. (2013). Forest insects and climate change: long-term trends in herbivore damage. Ecol. Evol. 3, 4183–4196. doi: 10.1002/ece3.717
PubMed Abstract | Full Text | CrossRef Full Text | Google Scholar
Kleiner, K. W., Ellis, D. D., McCown, B. H., and Raffa, K. F. (1995). Field-evaluation of transgenic poplar expressing a Bacillus-thuringiensis Cry1a(A) D-endotoxin gene against forest tent caterpillar (Lepidoptera, Lasiocampidae) and gypsy-moth (Lepidoptera, Lymantriidae) following winter dormancy. Environ. Entomol. 24, 1358–1364. doi: 10.1093/ee/24.5.1358
Kleiner, K. W., Ellis, D. D., McCown, B. H., and Raffa, K. F. (2003). Leaf ontogeny influences leaf phenolics and the efficacy of genetically expressed Bacillus thuringiensis cry1A(a) d-endotoxin in hybrid poplar against gypsy moth. J. Chem. Ecol. 29, 2585–2602. doi: 10.1023/A:1026370220613
PubMed Abstract | Full Text | CrossRef Full Text | Google Scholar
Klocko, A. L., Meilan, R., James, R. R., Viswanath, V., Ma, C., Payne, P., et al. (2014). Bt-Cry3Aa transgene expression reduces insect damage and improves growth in field-grown hybrid poplar. Can. J. For. Res. Rev. 44, 28–35. doi: 10.1139/cjfr-2013-0270
Lachance, D., Hamel, L. P., Pelletier, F., Valero, J., Bernier-Cardou, M., Chapman, K., et al. (2007). Expression of a Bacillus thuringiensis cry1Ab gene in transgenic white spruce and its efficacy against the spruce budworm (Choristoneura fumiferana). Tree Genet. Genomes 3, 153–167. doi: 10.1007/s11295-006-0072-y
Leather, S. R., Day, K. R., and Salisbury, A. N. (1999). The biology and ecology of the large pine weevil, Hylobius abietis (Coleoptera: Curculionidae): a problem of dispersal? Bull. Entomol. Res. 89, 3–16. doi: 10.1017/S0007485399000024
Lu, Y., Wu, K., Jiang, Y., Xia, B., Li, P., Feng, H., et al. (2010). Mirid bug outbreaks in multiple crops correlated with wide-scale adoption of Bt cotton in China. Science 328, 1151–1154. doi: 10.1126/science.1187881
PubMed Abstract | Full Text | CrossRef Full Text | Google Scholar
Ma, H., Zhao, M., Wang, H., Wang, Z., Wang, Q., and Dong, H. (2014). Comparative incidence of cotton spider mites on transgenic Bt versus conventional cotton in relation to contents of secondary metabolites. Arthropod. Plant Interact. 8, 1–7. doi: 10.1007/s11829-014-9291-6
Marini, L., Ayres, M. P., Battisti, A., and Faccoli, M. (2012). Climate affects severity and altitudinal distribution of outbreaks in an eruptive bark beetle. Clim. Change 115, 327–341. doi: 10.1007/s10584-012-0463-z
Miranda, M., Ralph, S. G., Mellway, R., White, R., Heath, M. C., Bohlmann, J., et al. (2007). The transcriptional response of hybrid poplar (Populus trichocarpa x P-deltoides) to infection by Melampsora medusae leaf rust involves induction of flavonoid pathway genes leading to the accumulation of proanthocyanidins. Mol. Plant Microbe Interact. 20, 816–831. doi: 10.1094/MPMI-20-7-0816
PubMed Abstract | Full Text | CrossRef Full Text | Google Scholar
Nordman, E. E., Robison, D. J., Abrahamson, L. P., and Volk, T. A. (2005). Relative resistance of willow and poplar biomass production clones across a continuum of herbivorous insect specialization: univariate and multivariate approaches. Forest Ecol. Manag. 217, 307–318. doi: 10.1016/j.foreco.2005.06.009
Pasteels, J. M., Rowel-Rahier, M., Braekman, J. C., and Dupont, A. (1983). Salicin from host plants as a precursor of salicylaldehyd in defensive secretion of Chrysomeline larvae. Physiol. Entomol. 8, 307–314. doi: 10.1111/j.1365-3032.1983.tb00362.x
Pentzold, S., Zagrobelny, M., Rook, F., and Bak, S. (2014). How insects overcome two-component plant chemical defence: plant beta-glucosidases as the main target for herbivore adaptation. Biol. Rev. 89, 531–551. doi: 10.1111/brv.12066
PubMed Abstract | Full Text | CrossRef Full Text | Google Scholar
Raffa, K. F. (1989). Genetic-engineering of trees to enhance resistance to insects - evaluating the risks of biotype evolution and secondary pest outbreak. Bioscience 39, 524–534. doi: 10.2307/1310975
Raffa, K. F. (2001). “Use of transgenic resistance in plantation trees: efficacy, risk, and integration with other pest management tactics,” in Proceedings of the First International Symposium on Ecological and Societal Aspects of Transgenic Plantations, eds S. H. Strauss and H. D. Bradshaw (Corvallis, OR: College of Forestry, Oregon State University), 138–147.
Schnepf, E., Crickmore, N., van Rie, J., Lereclus, D., Baum, J., Feitelson, J., et al. (1998). Bacillus thuringiensis and its pesticidal crystal proteins. Microbiol. Mol. Biol. Rev. 62, 775–806.
Shin, D. I., Podila, G. K., Huang, Y. H., and Karnosky, D. F. (1994). Transgenic larch expressing genes for herbicide and insect resistance. Can. J. For. Res. Rev. 24, 2059–2067. doi: 10.1139/x94-264
Smeets, E. M. W., and Faaij, A. P. C. (2007). Bioenergy potentials from forestry in 2050–An assessment of the drivers that determine the potentials. Clim. Change 81, 353–390. doi: 10.1007/s10584-006-9163-x
Tabashnik, B. E., Brevault, T., and Carriere, Y. (2013). Insect resistance to Bt crops: lessons from the first billion acres. Nat. Biotechnol. 31, 510–521. doi: 10.1038/nbt.2597
PubMed Abstract | Full Text | CrossRef Full Text | Google Scholar
Tabashnik, B. E., Huang, F., Ghimire, M. N., Leonard, B. R., Siegfried, B. D., Rangasamy, M., et al. (2011). Efficacy of genetically modified Bt toxins against insects with different genetic mechanisms of resistance. Nat. Biotechnol. 29, U1128–U1198. doi: 10.1038/nbt.1988
PubMed Abstract | Full Text | CrossRef Full Text | Google Scholar
Tang, W., and Tian, Y. C. (2003). Transgenic loblolly pine (Pinus taeda L.) plants expressing a modified delta-endotoxin gene of Bacillus thuringiensis with enhanced resistance to Dendrolimus punctatus Walker and Crypyothelea formosicola Staud. J. Exp. Bot. 54, 835–844. doi: 10.1093/jxb/erg071
PubMed Abstract | Full Text | CrossRef Full Text | Google Scholar
Tomescu, R., and Nef, L. (2007). Leaf eating insect damage on different poplar clones and sites. Ann. For. Sci. 64, 99–108. doi: 10.1051/forest:2006093
Tong, H., Su, Q., Zhou, X., and Bai, L. (2013). Field resistance of Spodoptera litura (Lepidoptera: Noctuidae) to organophosphates, pyrethroids, carbamates and four newer chemistry insecticides in Hunan, China. J. Pest Sci. (2004) 86, 599–609. doi: 10.1007/s10340-013-0505-y
PubMed Abstract | Full Text | CrossRef Full Text | Google Scholar
Wang, J. H., and Constabel, C. P. (2004). Polyphenol oxidase overexpression in transgenic Populus enhances resistance to herbivory by forest tent caterpillar (Malacosoma disstria). Planta 220, 87–96. doi: 10.1007/s00425-004-1327-1
PubMed Abstract | Full Text | CrossRef Full Text | Google Scholar
Ye, X., Busov, V., Zhao, N., Meilan, R., McDonnell, L. M., Coleman, H. D., et al. (2011). Transgenic populus trees for forest products, bioenergy, and functional genomics. CRC. Crit. Rev. Plant Sci. 30, 415–434. doi: 10.1080/07352689.2011.605737
Zhang, B., Chen, M., Zhang, X., Luan, H., Tian, Y., and Su, X. (2011). Expression of Bt-Cry3A in transgenic Populus alba x P. glandulosa and its effects on target and non-target pests and the arthropod community. Transgenic Res. 20, 523–532. doi: 10.1007/s11248-010-9434-1
PubMed Abstract | Full Text | CrossRef Full Text | Google Scholar
Keywords: GM trees, herbivore resistance, traits efficiency, tree growth, leaf damage
Citation: Hjältén J and Axelsson E P (2015) GM trees with increased resistance to herbivores: trait efficiency and their potential to promote tree growth. Front. Plant Sci. 6:279. doi: 10.3389/fpls.2015.00279
Received: 02 February 2015; Accepted: 07 April 2015;
Published: 01 May 2015.
Edited by:
Andrea Polle, Georg-August Universität Göttingen, GermanyReviewed by:
Gong-yin Ye, Zhejiang University, ChinaMaaria Rosenkranz, Helmholtz Zentrum München, Germany
Copyright © 2015 Hjältén and Axelsson. This is an open-access article distributed under the terms of the Creative Commons Attribution License (CC BY). The use, distribution or reproduction in other forums is permitted, provided the original author(s) or licensor are credited and that the original publication in this journal is cited, in accordance with accepted academic practice. No use, distribution or reproduction is permitted which does not comply with these terms.
*Correspondence: Joakim Hjältén, Department of Wildlife, Fish, and Environmental Studies, Swedish University of Agricultural Sciences, Skogsmarksgränd 2, Umeå SE-90183, Sweden,am9ha2ltLmhqYWx0ZW5Ac2x1LnNl