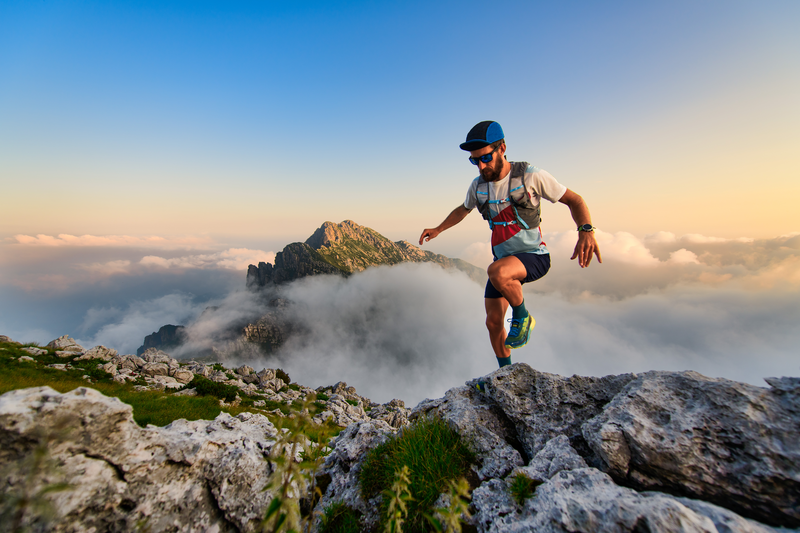
95% of researchers rate our articles as excellent or good
Learn more about the work of our research integrity team to safeguard the quality of each article we publish.
Find out more
REVIEW article
Front. Plant Sci. , 16 March 2015
Sec. Plant Biotechnology
Volume 6 - 2015 | https://doi.org/10.3389/fpls.2015.00163
Osmotin is a stress responsive antifungal protein belonging to the pathogenesis-related (PR)-5 family that confers tolerance to both biotic and abiotic stresses in plants. Protective efforts of osmotin in plants range from high temperature to cold and salt to drought. It lyses the plasma membrane of the pathogens. It is widely distributed in fruits and vegetables. It is a differentially expressed and developmentally regulated protein that protects the cells from osmotic stress and invading pathogens as well, by structural or metabolic alterations. During stress conditions, osmotin helps in the accumulation of the osmolyte proline, which quenches reactive oxygen species and free radicals. Osmotin expression results in the accumulation of storage reserves and increases the shelf-life of fruits. It binds to a seven-transmembrane-domain receptor-like protein and induces programmed cell death in Saccharomyces cerevisiae through RAS2/cAMP signaling pathway. Adiponectin, produced in adipose tissues of mammals, is an insulin-sensitizing hormone. Strangely, osmotin acts like the mammalian hormone adiponectin in various in vitro and in vivo models. Adiponectin and osmotin, the two receptor binding proteins do not share sequence similarity at the amino acid level, but interestingly they have a similar structural and functional properties. In experimental mice, adiponectin inhibits endothelial cell proliferation and migration, primary tumor growth, and reduces atherosclerosis. This retrospective work examines the vital role of osmotin in plant defense and as a potential targeted therapeutic drug for humans.
Plants are subjected to various kinds of biotic (Lodge et al., 1993; Friedrich et al., 2000; Selitrennikoff, 2001; Kessler and Baldwin, 2002; Poupard et al., 2003; Anssour and Baldwin, 2010) and abiotic stresses (Brune et al., 1995; Apse and Blumwald, 2002; Rossel et al., 2002; Kaplan et al., 2004; Yamaguchi-Shinozaki and Shinozaki, 2006; Parent et al., 2008; Sanchez et al., 2008; Gill and Tuteja, 2010) during different developmental phases. These stresses impair many cellular activities, resulting in reduced growth and huge yield losses (Boyer, 1982; Wang et al., 2003; Rodriguez et al., 2005; Oerke, 2006). But, plants can sense and respond to these different stresses that are complex and integrative. Consequently, an array of cascade interactions evolve in the plants (Chisholm et al., 2006; Jones and Dangl, 2006; Atkinson and Urwin, 2012). Biotic stresses activate many intracellular defense signals leading to the production of antimicrobials and pathogenesis-related (PR) proteins (Vigers et al., 1991; Yun et al., 1997a; Veronese et al., 2003). PR proteins act as first line of plant defense and are induced in response to not only biotic but also to abiotic stresses (Bol et al., 1990; Linthotst and Van Loon, 1991; Stintzi et al., 1993; Van Loon, 1997). They were first observed in tobacco infected with tobacco mosaic virus and high levels of PR-5 proteins were detected in young leaves when exposed to salt stress (Van Loon and Kammen, 1970; Singh et al., 1989). Based on isoelectric point (pI), PR-5 proteins are divided into three groups: acidic (PR-S), basic (osmotin), and neutral (osmotin like proteins-OLPs; Koiwa et al., 1994; Van Loon and Van Strien, 1999). The counterparts of osmotin from tobacco PR-R and PR-S are acidic (Skriver and Mundy, 1990). But, Glycine max OLP (GmOLP) is an acidic protein (Onishi et al., 2006). PR-5 proteins are also called thaumatin-like proteins (TLPs) since they show structural homology with thaumatin, a protein isolated from Thaumatococcus danielli (Vander and Loeve, 1972; Edens et al., 1982; Cornelissen et al., 1986; Velazhahan et al., 1999). In spite of their high sequence similarity, even a small change in the amino acids of these proteins leads to diverse functions. Thaumatin tastes sweet but does not exhibit antifungal activity (Ogata et al., 1992; Zemanek and Wasserman, 1995). Contrarily, zeamatin does not taste sweet but exhibits high antifungal activity (Malehorn et al., 1994). Osmotin and OLPs accumulate in response to both biotic and abiotic stresses which facilitate the compartmentation of ions or solutes and exhibit antifungal activities. Adiponectin, the insulin sensitizing mammalian hormone is secreted in adipose tissues and exerts its function by binding to the plasma membrane receptors called as adiponectin receptors (AdipoRs). Adiponectin deficiency results in diabetes, fatty liver diseases, and cardiovascular disorders (Kadowaki and Yamauchi, 2005; Tang et al., 2005; Holland and Scherer, 2013). Adiponectin is a structural and functional homolog of osmotin (Narasimhan et al., 2005; Miele et al., 2011; Naseer et al., 2014). Husaini and Rafiqi (2012) and Viktorova et al. (2012) reviewed the importance of osmotin. But, the present paper deals with the review of progress made about the multiple activities of osmotin and identify the gaps in our understanding of osmotin protein, counterfeit for adiponectin.
Osmotin, a multifunctional stress responsive PR-5 protein is named on the basis of its induction by osmotic stress to low water potential (Singh et al., 1985). Osmotin and its homolog proteins are ubiquitous in most fruits and vegetables. Osmotin and OLPs confer stress tolerance to plants and their expression was induced by NaCl (LaRosa et al., 1987, 1989, 1992; Singh et al., 1987a; Bol et al., 1990; Raghothama et al., 1993, 1997; Zhu et al., 1993, 1995a; Koyama et al., 2001; Sokhansanj et al., 2006; Qureshi et al., 2007), abscisic acid (ABA; LaRosa et al., 1987, 1992; Singh et al., 1989; Raghothama et al., 1993, 1997; Zhu et al., 1993, 1995b), ethylene (LaRosa et al., 1992; Raghothama et al., 1993, 1997; Sato et al., 1996; Kitajima et al., 1998), dessication (Pla et al., 1998), cold (Newton and Duman, 2000; D’Angeli and Altamura, 2007), drought (Parkhi et al., 2009), salicyclic acid (Kim et al., 2002), wounding (LaRosa et al., 1992; Zhu et al., 1995a), bacterial (Choi et al., 2013), viral (Cornelissen et al., 1986; Stintzi et al., 1991; LaRosa et al., 1992; Elvira et al., 2008; Choi et al., 2013), and fungal stresses (Woloshuk et al., 1991; Vigers et al., 1992; Raghothama et al., 1993; Liu et al., 1994; Zhu et al., 1995a, 1996; Abad et al., 1996; Zuker et al., 2001; Tzou et al., 2011). However, the signaling pathways associated with the induction of osmotin by these different stresses are not known. Transgenic and native expression of osmotin and OLPs was observed in various plants when treated with different biotic and abiotic stresses (Tables 1 and 2). Osmotin is induced in Petunia when treated with Penicillium funiculosum, Erwinia stewartii, Pseudomonas syringae, aspirin, wounding, and salicylic acid (Kim et al., 2002). When treated with salt, Osmotin34 was induced in Bruguiera gymnorhiza transgenics expressing ankyrin repeat protein 1 (BgARP1; Miyama and Tada, 2011). It is also induced in genetic tumors of tobacco plants (Fujita et al., 1994) and at high atmospheric CO2 concentration in potatoes (Plessl et al., 2007) and in leaves and trichomes of tobacco by cadmium metal stress (Harada et al., 2010) implying that it plays a vital role in this diverse array of stresses. Osmotin gene transferred to somatic embryos of tea plants showed an increase in seed storage reserves and desiccation tolerance in recalcitrant embryos (Bhattacharya et al., 2006). Osmotin levels are reduced in virus induced gene silencing of CaOXR1 (Capsicum annum oxidoreductase1) and CaOXR1/CaRAV1 (Capsicum annum related to ABI3/VP1) in pepper leaves when treated with NaCl or mannitol (Lee et al., 2010b). Expression of osmotin in transgenics showed enhanced fruit shelf-life in strawberries (Chen, 2012). It is also induced with fungicide acibenzolar-S-methyl treatment which may act as an elicitor (Whan et al., 2009). Thus, it appears osmotin is expressed differentially by many stresses and has multifaceted roles to perform in plants.
Osmotin and OLPs show spatial and temporal regulation during various stages of development in roots, pollen, pistils, and fruits (Kononowicz et al., 1992; Kim et al., 2002). While osmotin is secreted in an intracellular compartment, its counterpart lacking 20 C-terminal amino acids is secreted into extracellular matrix (Liu et al., 1996; Parkhi et al., 2009). The lack of the C-terminal vacuolar sorting motif in GmOLPa results in secretion of GmOLPa into extracellular space instead of the vacuole while GmOLPb with C-terminal elongation is secreted into the vacuole (Onishi et al., 2006; Tachi et al., 2009).
Osmotin shows tissue-specific activity with ABA, ethylene, and NaCl treatments (Raghothama et al., 1997). Osmotin expression was observed in flowers of tobacco (Neale et al., 1990), somatic embryos (Bhattacharya et al., 2006), leaves, and trichomes (Harada et al., 2010), skin of grapes (Margaria and Palmano, 2011), seed coat and endosperm in olive (D’Angeli et al., 2013).
Osmotin like proteinss also show tissue-specific expression in many plants. Under oxidative stress conditions, OLPs are expressed in the quiescent region of root apex and meristematic region of shoot apex (Pla et al., 1998). Activity of OLPs was also observed in flowers and fruits of tomato (Chen et al., 1996), roots, stems, leaves, and flowers of Arabidopsis (Capelli et al., 1997), root and shoot apices of Quercus suber (Pla et al., 1998), ovary of grapes (Salzman et al., 1998), root, stem, leaves, and flowers of Solanum nigrum (Jami et al., 2007).
Abscisic acid transcriptionally regulates osmotin and osmotin promoter in tobacco (LaRosa et al., 1992; Nelson et al., 1992). In ABA-deficient mutants of tomato, osmotin transcripts are not induced by salt and water stresses suggesting the endogenous levels of ABA are required for the transcript accumulation (Grillo et al., 1995). Along with cis-elements, ethylene-responsive element binding proteins (EREBPs) which bind to two GCC boxes in osmotin promoter are essential for ethylene response (Raghothama et al., 1997; Xu et al., 1998). Osmotin expression was influenced by the type of promoter used. Osmotin-specific promoter showed higher expression levels compared to CaMV35S promoter in sugar beet cells (Ivic-Haymes and Smigocki, 2005). Osmotin promoter is also induced by wounding in sugar beet and apple (Snyder et al., 1999; Liu et al., 2001). OPBP1 (osmotin promoter binding protein 1), an apetala2/ethylene responsive transcription factor of tobacco displayed salt and disease tolerance in rice (Chen and Guo, 2008). Jasmonate Ethylene Response Factor 1 (JERF1) and GbERF (Gossypium barbadense Ethylene Response Factor) induces osmotin expression by activating the GCC box (Zhang et al., 2004; Qin et al., 2006). Constitutive expression of OLPs was observed due to ethylene responsive elements (AGCCGCC) and ethylene-responsive element binding factor (ERF3) in cultured tobacco cells (Sato et al., 1996; Kitajima et al., 1998), and transcriptional activation of OLP (OSMLl3 and OSML8l) promoters was also studied in potato (Zhu et al., 1995a). Thus, the information is fragmentary and only a few of the plant hormones mentioned above regulate osmotin and OLPs, but we still do not know the comprehensive regulation of osmotin at the transcriptional or post-transcriptional level by other phytohormones.
Singh et al. (1987a) characterized tobacco osmotin protein. It is a 26-kDa cationic protein induced in cultured cells of tobacco adapted to NaCl and low water potential, and accumulates up to 12% of total cell protein. Without salt exposure also, osmotin is accumulated in untreated cells and NaCl-treated cell cultures (Singh et al., 1985, 1987b; Bressan et al., 1987). This indicates that basal levels of osmotin may perhaps be essential for housekeeping in the cells that are not exposed to stress. Osmotin occurs in two forms as osmotin I and II in 2:3 ratios with basic pIs of about 8.2. Osmotin I (aqueous soluble form) and osmotin II (detergent soluble form) have the same first 22 amino acids at N-terminus. But, osmotin II is more resistant than osmotin I to protease digestion and cross reacts with antibodies of osmotin I. Anti-osmotin antibodies cross react with Arabidopsis TLP (ATLP-1) protein also (Hu and Reddy, 1997). Osmotin shows significant sequence homology at amino acid level with the sweet-tasting protein thaumatin and shares several similar characteristic features like molecular weight, lack of sulfhydral residues, basic pI, disulfide bonds, and high proline content. Osmotin is not sweet in taste and the probable reason may be due to lack of lysine residues that are present in thaumatin protein (Richardson et al., 1987). Osmotin contains 16 cysteine residues resulting in the formation of eight disulfide linkages; alanine is present at the cleavage site of the N-terminal leader sequence (Kononowicz et al., 1994). It may also act as a storage protein which is evident by its accumulation in vacuolar inclusion bodies, with the help of C terminal peptide (Melchers et al., 1993). Osmotin activity was completely reduced with increasing concentrations of K+ but, the same was retained even at high concentrations of Ca2+, suggesting that Ca2+ facilitates osmotin binding to the fungal cell surface (Salzman et al., 2004). Interestingly, Izh2p a protein from Saccharomyces cerevisiae also serves as a receptor for osmotin (Kupchak et al., 2008).
Min et al. (2004) analyzed the crystal structure of osmotin. Purified osmotin from salt-adapted cells was used for structural analysis at 2.3 Å resolution and compared with other PR-5 proteins. Osmotin shows two monomers with slight difference in the tertiary structure and presence of a non-crystallographic dimer in the asymmetric unit. Osmotin is composed of three domains and the folds are very much similar to thaumatin, zeamatin, and tobacco PR-5d protein (de Vos et al., 1985; Batalia et al., 1996; Koiwa et al., 1999). Osmotin shows a prominent cleft assembled by domains I and II. Domain I consists of 11 β strands which form the core of the protein, while several loops of domain II extended from domain I are stabilized by four disulfide bonds, and domain III shows a small loop with two disulfide bonds. The acidic cleft of domain I and II is formed by acidic residues Glu84, Asp97, Asp102, and Asp185. But, osmotin lacks thaumatin loop which is responsible for its sweetness in domain II (Slootstra et al., 1995). Homology modeling of Piper colubrinum osmotin2 (PcOSM2) showed domain I with 12-β sheets, an α-helical domain II, and domain III with 2-β sheets, contrarily PcOSM1 exhibited a deformed, unidentical domain III and loss of 4-β sheets in domain I. But, the acidic cleft responsible for antifungal activity was present in both the isoforms (Mani et al., 2012).
The mode of action of osmotin is not well understood, however, osmotin protects the cells from osmotic shock especially during abiotic stresses by compartmentalization of solutes or by structural or metabolic alterations (Singh et al., 1987a; Barthakur et al., 2001). Besides osmotic balance, it plays a crucial role as an antifungal protein. Several hypotheses were proposed to explain the molecular action of osmotin. Firstly, osmotin with the help of cell wall components is involved in permeabilization of membrane and plasmolysed cells become insensitive to osmotin suggesting that the cell wall components are needed for its activity (Abad et al., 1996). In the second mechanism, osmotin subverts cell signal transduction pathway in the target by activating mating pheromones to weaken the cell wall and increases its cytotoxic efficacy (Yun et al., 1998). The third proposed mechanism for antifungal activity is the interaction of osmotin protein with the receptor of cell membrane which initiates mitogen activated protein kinase signal transduction pathway, leading to the formation of a transmembrane pore to cause leakage in membrane and subsequent rupture of the membrane (Bowles, 1990; Roberts and Selitrennikoff, 1990; Cheong et al., 1997; Yun et al., 1997b, 1998; Anzlovar et al., 1998; Narasimhan et al., 2001, 2005). In general, PR-5 proteins function similar to β-1,3-glucanase by hydrolyzing the β-1,3-glucans of the pathogens (Grenier et al., 1999). Osmotin is also involved in apoptosis through the accumulation of reactive oxygen species (ROS) via the RAS2/cAMP pathway as shown in Saccharomyces cerevisiae (Narasimhan et al., 2001).
Plants perceive external signals from environment and manifest mechanisms to acquire stress tolerance through multiple signal transduction pathways. Osmotin (PhOSM) is involved in signal transduction during wound stress (Kim et al., 2002). The mitogen-activated protein kinase (MAPK) cascade is a critical signaling pathway associated in response to external stimuli and contains three consecutively activated kinases. Active MAPKKK (mitogen-activated protein kinase kinase kinase) activates MAPKK (mitogen-activated protein kinase kinase) by phosphorylation, which in turn phosphorylates MAPK. The activated MAPK phosphorylates target proteins and regulates the osmotin gene. MAPKs are activated when exposed to stress conditions like salt, drought, cold, and pathogen attack (Jonak et al., 1996; Mikolajczyk et al., 2000). Overexpression of Gossypium hirsutum MPK2 (GhMPK2), a MAPK in tobacco showed upregulation of osmotin with enhanced tolerance to salt and drought, which suggests that GhMPK2 has a role in signal transduction (Zhang et al., 2011). Based on a bioinformatics study, osmotin confers tolerance against biotic and abiotic stress through its involvement in signal transduction pathway, and not activating a transcription factor. When analyzed with bioinformatics tools, DNA binding motif was not found in osmotin and it has only 0–20% homology with protein sequences from database of Arabidopsis transcription factors which confirms that it has no DNA binding motif. Further, superimposition of 3D-modeled structure of osmotin with Arabidopsis transcription factors also suggests the absence of DNA-binding motifs (Abdin et al., 2011).
Hypersensitive reaction (HR) is a consequence of disease resistance in plants and cell death occurs either by apoptosis or programmed cell death (PCD). PCD plays a crucial role in plant development and host interaction, which is activated by cell wall components or toxins or proteins secreted from pathogens (Aliprantis et al., 1999). P53 is overexpressed in cells treated with toxins and the balance is influenced by hormone or toxin. P53 acts as a link between cell cycle and PCD. Osmotin decreases the pathogenesis by interacting with the cell cycle machinery and overexpresses the cell cycle components which inhibit the cell death pathway components like P53. Brassica juncea calli overexpressing osmotin showed delayed symptoms when treated with Alternaria toxin, suppressed the expression of P53 and the activity of caspase I was not affected which shows that osmotin is involved in P53-mediated PCD pathway (Taj et al., 2004). PCD was observed in Saccharomyces cerevisiae BWG7a cells when treated with different concentrations of osmotin due to suppression of transcription of the stress responsive genes with the accumulation of ROS (Narasimhan et al., 2001).
Osmotin plays an important role in salt stress tolerance by sequestering Na+ ions and compartmentalizing them into vacuoles and intercellular spaces. The association of tobacco osmotin protein with tonoplast (Singh et al., 1987a) and the OLP identified from Mesembryanthemum crystallinum suggests the role of osmotin in the intracellular compartmentation of Na+ ions (Yen et al., 1994). But, whether osmotin upregulates sodium-proton antiporter1 (NHX1) gene to sequester Na+ ions or how it is able to perform this functions is not yet clear. It is also not clear if osmotin has a direct role to play or it stimulates other proteins that are downstream. Transgenics overexpressing osmotin gene exhibited salt tolerance in potato (Evers et al., 1999), tobacco (Barthakur et al., 2001), Triticum aestivum cv. Marvdasht (Noori and Sokhansanj, 2008), strawberry (Husaini and Abdin, 2008), tomatoes (Goel et al., 2010), mulberry (Das et al., 2011), chili pepper (Subramanyam et al., 2011), and soybean (Subramanyam et al., 2012) by retaining chlorophyll, preventing the accumulation of ROS, with an increase in relative water content, proline accumulation, increase in root length, shoot length, plant height, leaf expansion, and improved root growth than controls. Overexpression of OLP lacking short C terminal cDNA also showed such an enhanced salt tolerance in potato (Evers et al., 1999). Transgenic mulberry expressing osmotin driven by CaMV35S promoter displayed better tolerance to salt stress than the transgenics containing osmotin under the influence of rd29A promoter, though the rd29A promoter is responsive to dehydration while the CaMV35S promoter is constitutive (Das et al., 2011). Rice transgenic plants expressing OPBP1 showed salt tolerance with enhanced root length and root growth than the untransformed controls (Chen and Guo, 2008). This suggests that osmotin somehow triggers auxin biosynthesis and improves root biomass under salt stress.
Osmotin and OLPs also accumulate during drought stress. Accumulation of osmotin mRNA and osmotin protein varies in different tissues with different treatments and vice-versa. Osmotin mRNA was observed in different plant tissues when stimulated with water deficit and ABA, whereas osmotin protein was not detected. During water deficit conditions, the number of ribosomes loaded was not affected in apical leaves but, a higher level of messenger RNA was noticed in basal leaves (LaRosa et al., 1992; Kawaguchi et al., 2003). Transgenics expressing osmotin showed an increase in the relative water content, chlorophyll, and leaf expansion than controls and recovered completely after rewatering. This implies that osmotin is able to protect chlorophyll and photosynthetic machinery under water limited conditions. Transgenics are tolerant to water deficit conditions in tobacco (Barthakur et al., 2001) and tomato (Goel et al., 2010). Transgenic mulberry plants expressing osmotin with rd29A promoter are more responsive to drought than mulberry plants expressing osmotin with CaMV35S promoter (Das et al., 2011). Thus, it appears that stress-inducible promoters are better for the overexpression of osmotin gene compared to universal promoters.
Plants are very sensitive to cold stress and results in depolarization and rigidification of cell membrane when exposed to low temperatures (Los and Murata, 2004). Pollen sterility occurs if the plants are exposed to <20°C for few days at young microspore stage in rice. During the cold conditions, the tapetal cell which nourishes the pollen undergoes hypertrophy leading to the formation of sterile pollen grains with little or no starch and it was observed that anthers show more abnormalities than pistils or other floral organs in cold exposed rice plants (Imin et al., 2006). Osmotin also plays a role in cryoprotection during low temperature exposure. During cold stress, osmotin is induced in seed coat and endosperm in olive, and OLP in pollen of Solanum (Volger and Heber, 1975; Zhu et al., 1993; D’Angeli et al., 2013). Increased osmotin promoter activity was also observed in tobacco pollen grains under normal conditions (Kononowicz et al., 1992). Osmotin protein homolog was upregulated when plants are exposed to 12°C in the cold sensitive rice cultivar Doongara (Imin et al., 2006). D’Angeli and Altamura (2007) demonstrated that osmotin is involved in PCD, which is cold inducible in olive trees. The results suggest that osmotin regulates cytoskeleton alterations and mediates calcium signaling under cold stress. A change in Ca2+ concentration may initiate the cold induced PCD (Kratsch and Wise, 2000) which has been elucidated in human cells (Risso et al., 1998) and yeast (Narasimhan et al., 2001, 2005). Overexpression of osmotin enhanced the tolerance to cold in tomato growing at higher altitudes (Sarad et al., 2004). Capsicum, upon treatment with cold stress showed enhanced osmotin transcripts (Patade et al., 2012). OLP was isolated from frozen-thawed protoplasts with a molecular mass of 25 kDa in Solanum dulcamara (Newton and Duman, 2000). Thus, these findings implicate osmotin during cold stress tolerance. However, it is unknown if it induces the biosynthesis of fatty acids that are associated with cell membrane protection under cold stress, conditions or upregulates cold-regulated genes.
Proline, a multifunctional molecule, accumulates during stress and accounts for up to 80% of the total amino acid pool in certain plants. It acts as an osmotic agent and as free radical scavenger that helps plants to withstand drought and salt stress conditions (Kishor and Sreenivasulu, 2014). Proline, when accumulated in cytosol, does not show any detrimental effects but detoxifies ROS and free radicals by forming long living adducts during osmotic stress (Floyd and Nagy, 1984; Lutts et al., 1996; Hong et al., 2000; Vinocur and Altman, 2005). Osmotin triggers the accumulation of osmolytes like proline and glycine betaine (Holmstrom et al., 2000) and the accumulation of proline in osmotin overexpressed transgenics is influenced by both constitutive and inducible promoters. While higher proline accumulation was reported in transgenics overexpressing osmotin such as potato (Evers et al., 1999), tobacco (Barthakur et al., 2001; Sokhansanj et al., 2006), tomato (Goel et al., 2010), mulberry (Das et al., 2011), and chili pepper (Subramanyam et al., 2011), transgenics without proline accumulation were also noticed but with relatively low stress tolerance (Nanjo et al., 1999). Thus, osmotin expression in transgenics confers osmotic tolerance by accumulation of more proline. Nevertheless, it is necessary to find out if there is any upregulation of P5CS or P5CR genes involved in proline biosynthetic pathway by osmotin overexpression.
While the production of ROS increases, the antioxidative system is impaired under many environmental stress conditions (Dhindsa and Matowe, 1981). It has been observed that accumulation of hydrogen peroxide (H2O2) was lower in transgenics overexpressing osmotin than the corresponding controls when treated with different NaCl concentrations implying that osmotin helps in controlling its overaccumulation. Transgenic Capsicum annum L. overexpressing osmotin when treated with salt, showed higher activity of ascorbate peroxidase (APX) and superoxide dismutase (SOD) to detoxify the accumulated H2O2 than the untransformed controls under identical conditions. But, how osmotin activates the APX and SOD activities is not clear. Low levels of malondialdehyde, an indicator of lipid peroxidation levels in transgenics overexpressing osmotin compared to controls, suggests less damage of cell membrane in transformants compared to controls (Subramanyam et al., 2011). Thus, osmotin overexpressing plants neutralize the ROS by producing more compatible solutes or expression of specific antioxidative enzymes.
Fungus results in massive crop losses, crop rotations, and fungicides are not fully effective in controlling fungi (Selitrennikoff, 2001). PR proteins are effective in controlling pathogens and osmotin has specific and broad spectrum activity (Abad et al., 1996; Veronese et al., 2003). Osmotin acts as an antifungal cytotoxic compound with rapid cell death in the yeast (Kupchak et al., 2008). Cell wall components especially oligosaccharides act as elicitors of plant defense (Ebel, 1998). Osmotin requires cell wall components for its action and plasmolysed Trichoderma longibrachiatum fungal cells are resistant to osmotin action (Abad et al., 1996). However, osmotin activity varies with change in the cell wall composition (Ibeas et al., 2000, 2001). The outer layer of the yeast cell wall is composed of mannoproteins, a surface determinant for osmotin. Phosphomannan is an essential polyanion for osmotin binding to the cell wall. Phosphomannoproteins are regulated by MNN1, MNN2, MNN4, or MNN6 which facilitate the binding of osmotin to the cell wall and are responsible for cytotoxicity. MNN1 adds terminal mannose making osmotin unable to bind to cell wall and null mnn1 mutants exhibit enhanced osmotin binding and sensitivity. Salt and carbohydrate disturb the interaction between phosphomannan and osmotin. Overexpression of cell wall protein containing inverted repeats (PIR proteins) results in enhanced resistance to osmotin and deletion results in sensitivity to osmotin in resistant strains (Yun et al., 1997b). Fusarium oxysporum f. sp. Nicotianae overexpressing cell wall glycoprotein PIR2 exhibits increased resistance to osmotin (Narasimhan et al., 2003). Cell walls of pathogens possess proteins that expedite or restrict plant defense proteins to act on the plasma membrane. Specificity exists between osmotin and its target cell. Strains sensitive to tobacco osmotin are resistant to Atriplex nummularia OLP and all spheroplasts of resistant and susceptible strains are equally sensitive to the toxicity of osmotin and not to OLPs. Osmotin binding to the cell wall in Aspergillus nidulans is mediated by a heteromeric G-protein through a signal transduction pathway and its binding is inhibited by guanosine 5′-O-(2-thiodiphosphate) βS (GDPβS) that blocks G-protein. Mutation in FadA (α-subunit) and deletion in SfaD (β subunit) of G-protein blocks the osmotin binding and displays increased resistance to osmotin. These mutants showed enhanced chitin content with decreased sensitivity to osmotin (Coca et al., 2000). Sachharomyces cerevisiae D1 (SSD1) regulates the deposition of glucans and PIR glycoproteins in the cell wall, a major determinant of osmotin resistance in yeast and susceptibility to osmotin in Saccharomyces cerevisiae is encoded by Fusarium osmotin resistance3 (FOR3), a homolog of SSD1. Δfor3 mutants showed high sensitivity to osmotin similar to Δssd1 mutants (Lee et al., 2010a). It was discovered that PH036, an osmotin binding plasma membrane protein is required for full sensitivity of osmotin (Narasimhan et al., 2005).
Osmotin transcript levels vary during growth and decrease with advancing maturity in grapevine berries (Kretschmer et al., 2007). Osmotin transcript accumulation was reported during incompatible plant pathogen interaction with tomato Pto and Pseudomonas syringae pv. Avr Pto genes (Jia and Martin, 1999). In vitro analysis demonstrates that osmotin is effective against different fungal pathogens (Yun et al., 1997a). Osmotin and OLPs are expressed during infection and confer antifungal activity against a broad range of fungal species like Phytophthora infestans (Woloshuk et al., 1991; Vigers et al., 1992; Zhu et al., 1993, 1995b; Liu et al., 1994, 1996; Plessl et al., 2007; Rivero et al., 2012), Candida albicans, Neurospora crassa, and Trichoderma reesei (Vigers et al., 1992), Guignardia bidwellii and Botrytis cinerea (Salzman et al., 1998), Phomopsis viticola, Botrytis cinereamycelia, and Uncinula necator (Monteiro et al., 2003b), Xanthomonas campestris pv. Vesicatoria, Colletotrichum coccodes, and Phytophthora capsici (Hong et al., 2004), X. campestris pv. Vesicatoria (Lee and Hwang, 2005), F. oxysporum f. sp. Lycopersicii (Ouyang et al., 2005), Rhizoctonia batiticola and Sclerotinia sclerotiorum (Jami et al., 2007), pepper mild mottle virus (Elvira et al., 2008), Sclerotinia sclerotiorum (Zhao et al., 2009), Alternaria brassicicola (Mukherjee et al., 2010), F. solani, Neurospora sp., and Colletotrichum gloeosporioides, F. oxysporum, R. solani, Aspergillus niger (de Freitas et al., 2011a,b; Souza et al., 2011; Rivero et al., 2012), Piriformospora indica (Husaini et al., 2012), Phytophthora capsici and F. oxysporum (Mani et al., 2012), Microsphaera diffusa, Septoria glycines and Phakopsora pachyrhizi (Subramanyam et al., 2012) and Phaeoisariopsis personata (Vasavirama and Kirti, 2012). Compared to controls, enhanced activity of osmotin was observed in transgenic mulberry plants and in particular, transgenics expressing osmotin with rd29A promoter showed more tolerance to F. pallidoroseum, Colletotrichum dematium, and Colletotrichum gloeosporioides than transgenics with CaMV35S promoter (Das et al., 2011). Transgenic rice overexpressing OPBP1 exhibited increased disease resistance against Magnaporthe oryzae and R. solani (Chen and Guo, 2008). OLPs are also involved in plant defense and translocate along with actin filaments during cytoplasmic aggregation (Takemoto et al., 1997).
Adiponectin, a mammalian circulating protein produced in adipose tissue is an insulin-sensitizing hormone (Turer and Scherer, 2012). Adiponectin is also referred as Acrp30, AdipoQ, apM1, and GBP28 (Scherer et al., 1995; Hu et al., 1996; Maeda et al., 1996; Nakano et al., 1996). Adiponectin occurs in plasma as high, medium, and low molecular weight forms (HMW, MMW, and LMW). Many studies suggest that the HMW form is closely associated with insulin sensitivity (Bobbert et al., 2005; Salani et al., 2006). Hence, we have considered HMW adiponectin in the present study. It exerts its functions by binding to the plasma membrane receptors called AdipoRs. Adiponectin and its receptors are well reviewed by Kadowaki and Yamauchi (2005). AdipoRs are of two types: AdipoR1 that activates the 5′ adenosine monophosphate-activated protein kinase (AMPK) pathway in skeletal muscles and AdipoR2 that activates peroxisome proliferator-activated receptors (PPARα) pathway in the liver to increase insulin sensitivity and decrease inflammation. Experimental evidence suggests that AdipoR1 and R2 serve as major AdipoRs in in vivo conditions (Yamauchi et al., 2007). The prevalence of obesity has increased sharply in the recent times. Adiponectin levels are negatively correlated with body mass but positively correlated with reduction in body weight. HMW adiponectin is decreased in obesity and type II diabetes and increased in type I diabetes (Yang et al., 2001; Pajvani and Scherer, 2003; Yatagai et al., 2003; Flier, 2004; Wolf et al., 2004; Pereira et al., 2012). It is produced in large quantities by normal fat cells and less by fat cells (Nawrocki et al., 2006). High levels of HMW adiponectin and total adiponectin was observed in children suffering with Prader–Willi syndrome despite of profound obesity and hypoinsulinaemia (Haqq et al., 2007). HMW adiponectin levels are also decreased during gestational diabetes (Retnakaran et al., 2007). In vitro treatment of adipocytes with pioglitazone, an antidiabetic drug, increased HMW adiponectin levels (Bodles et al., 2006). AdipoRon, a small synthetic molecule, acts as agonist of adiponectin. When administered orally in mice, it delivered the same effects of adiponectin in muscle and liver cells and alleviated insulin resistance and type-II diabetes (Okada-Iwabu et al., 2013). Therefore, HMW adiponectin or its agonist can act as novel therapeutic strategy as a treatment to counteract these diseases as suggested by Yamauchi and Kadowaki (2008).
Obesity also results in the development of several other diseases like diabetes, cancer, fatty liver, and cardiovascular disorders which are positively related to angiogenesis. In experimental mice, adiponectin inhibits endothelial cell proliferation and migration, primary tumor growth, and reduces atherosclerosis. Adiponectin alleviates alcoholic and obese induced fatty liver diseases (Xu et al., 2003), acts as anti-inflammatory hormone in the repair of liver injury induced by CCl4 (Yoda-Murakami et al., 2001), and suppresses liver fibrosis (Kamada et al., 2003). Adiponectin plays an important role in energy homeostasis too (Yamauchi et al., 2003; Qi et al., 2004). Hypoadiponectin results in twofold increase in coronary artery diseases in men (Kumada et al., 2003), but development of atherosclerosis was suppressed in mice with increased plasma levels of adiponectin (Okamoto et al., 2002; Trivedi et al., 2012a). It was shown that adiponectin induces antiangiogenesis and antitumor activity via caspase-mediated endothelial cell apoptosis (Brakenhielm et al., 2004). Adiponectin shows anti-inflammatory role in murine colitis also (Arsenescu et al., 2011). Adiponectin can prevent fetal alcohol syndrome by protecting hippocampal neurons against alcohol induced apoptosis (Naseer et al., 2014). Adiponectin alleviates ceramidase activity, helps in the reduction of palmitate-induced cell death and over production of adiponectin decreases caspase-8-mediated death (Holland et al., 2011).
Osmotin, a naturally occurring plant protein mimics human adiponectin. Osmotin shares structural and functional homology with adiponectin and not sequence similarity (Min et al., 2004). Osmotin exerts its action by binding to a seven-transmembrane-domain receptor-like protein encoded by PHO36, while a mammalian homolog of PHO36 receptor is the human hormone AdipoR1. Domain I (core protein) of osmotin resembles the structural homolog of β-barrel domain of adiponectin. Like adiponectin, osmotin binds to AdipoRs and induces AMP kinase phosphorylation in mammalian C2C12 myocytes (Narasimhan et al., 2005). Osmotin activity was studied on in vitro and animal models. When administered intravenously in experimental rats, it acts as adiponectin agonist in obesity and type-II diabetes, and also displayed antiatherosclerotic activity (Trivedi et al., 2012a,b). Like adiponectin, osmotin also exhibited similar functional activity in in vitro cultured human synovial fibroblasts (Miele et al., 2011). Like adiponectin, osmotin exerted similar anti-inflammatory function in murine colitis (Arsenescu et al., 2011). Subcutaneous administration of osmotin, protects rat pups from ethanol induced apoptosis in cortical and hippocampal neurons (Naseer et al., 2014). Osmotin is resistant to pepsin digestion and heat treatment, and shows significant IgE binding and cross reacts with tomato and apple allergens. Allergenicity of osmotin can be reduced by mutations in IgE binding epitopes (Sharma et al., 2011, 2013a). Furthermore, in silico analysis suggests that both osmotin and adiponectin interact with the same drugs.
Osmotin, a multifaceted plant protein confers tolerance to both biotic and abiotic stresses. Adiponectin, an antidiabetic and antiatherosclerotic protein is reduced in obese patients and leads to several diseases including coronary artery disease, inflammation, and liver diseases. Osmotin shows homology with human hormone adiponectin given that osmotin not only induces AMP kinase phosphorylation in mammalian C2C12 myocytes via AdipoRs, but also binds to the AdipoR1 by activating the same signaling path of adiponectin. Osmotin and adiponectin involve in antitumor activity by inhibiting p53 and suppressing caspase activity. In vitro and animal model studies suggest that, like AdipoRon and pioglitazone, osmotin acts as agonist for adiponectin. Due to the multiple activities of osmotin, it can be explored as an attractive option as agonist for adiponectin in treating adiponectin deficiency diseases in humans besides its function in biotic and abiotic stress tolerance in crop plants.
All the authors of the manuscript meet the essential criteria of the publication.
The authors declare that the research was conducted in the absence of any commercial or financial relationships that could be construed as a potential conflict of interest.
SK and PK thank the University Grants Commission, New Delhi, India for financial assistance. PK thanks the CSIR, New Delhi, for sanctioning CSIR-Emeritus Scientist fellowship. CM thanks Bioclues, Hyderabad for providing lab space.
Abad, L. R., D’Urzo, M. P., Liu, D., Narasimhan, M. L., Reuveni, M., Zhu, J. K.,et al. (1996). Antifungal activity of tobacco osmotin has specificity and involves plasma membrane permeabilization. Plant Sci. 118, 11–23. doi: 10.1016/0168-9452(96)04420-2
Abdin, M. Z., Kiran, U., and Alam, A. (2011). Analysis of osmotin, a PR protein as metabolic modulator in plants. Bioinformation 5, 336–340. doi: 10.6026/97320630005336
PubMed Abstract | Full Text | CrossRef Full Text | Google Scholar
Agaoglu, Y. S., Ergül, A., and Aras, S. (2004). Molecular characterization of salt stress in grapevine cultivars (Vitis vinifera L.) and root stocks. Vitis 43, 107–110.
Aghaei, K., Ehsanpour, A. A., and Komatsu, S. (2008). Proteome analysis of potato under salt stress. J. Proteome Res. 7, 4858–4868. doi: 10.1021/pr800460y
PubMed Abstract | Full Text | CrossRef Full Text | Google Scholar
Aliprantis, A. O., Yang, R. B., Mark, M. R., Suggett, S., Devaux, B., Radolf, J. D.,et al. (1999). Cell activation and apoptosis by bacterial lipoproteins through toll-like receptor-2. Science 285, 736–739. doi: 10.1126/science.285.5428.736
Anssour, S., and Baldwin, I. T. (2010). Variation in antiherbivore defense responses in synthetic Nicotiana allopolyploids correlates with changes in uniparental patterns of gene expression. Plant Physiol. 153, 1907–1918. doi: 10.1104/pp.110.156786
PubMed Abstract | Full Text | CrossRef Full Text | Google Scholar
Anzlovar, S., Serra, M. D., Dermastia, M., and Menestrina, G. (1998). Membrane permeabilizing activity of pathogenesis-related protein linusit in from flax seed. Mol. Plant Microbe Interact. 11, 610–617. doi: 10.1094/MPMI.1998.11.7.610
Apse, M. P., and Blumwald, E. (2002). Engineering salt tolerance in plants. Curr. Opin. Biotechnol. 13, 146–150. doi: 10.1016/S0958-1669(02)00298-7
Arsenescu, V., Narasimhan, M. L., Halide, T., Bressan, R. A., Barisione, C., Cohen, D. A.,et al. (2011). Adiponectin and plant-derived mammalian adiponectin homolog exert a protective effect in murine colitis. Dig. Dis. Sci. 56, 2818–2832. doi: 10.1007/s10620-011-1692-0
PubMed Abstract | Full Text | CrossRef Full Text | Google Scholar
Aslam, M., Singh, R., Anandhan, S., Pande, V., and Ahmed, Z. (2009). Development of a transformation protocol for Tecomella undulate (Smith) Seem from cotyledonary node explants. Sci. Horticult. 121, 119–121. doi: 10.1016/j.scienta.2009.01.007
Atkinson, N. J., and Urwin, P. E. (2012). The interaction of plant biotic and abiotic stresses: from genes to the field. J. Exp. Bot. 63, 3523–3543. doi: 10.1093/jxb/ers100
PubMed Abstract | Full Text | CrossRef Full Text | Google Scholar
Barthakur, S., Babu, V., and Bansal, K. C. (2001). Over-expression of osmotin induces proline accumulation and confers tolerance to osmotic stress in transgenic tobacco. J. Plant Biochem. Biotechnol. 10, 31–37. doi: 10.1007/BF03263103
Batalia, M. A., Monzingo, A. F., Ernst, S., Roberts, W., and Robertus J. D. (1996). The crystal structure of the antifungal protein zeamatin, a member of the thaumatin-like, PR-5 protein family. Nat. Struct. Biol. 3, 19–23. doi: 10.1038/nsb0196-19
Bhattacharya, A., Saini, U., Sharma, P., Nagar, P. K., and Ahuja P. S. (2006). Osmotin-regulated reserve accumulation and germination in genetically transformed tea somatic embryos: a step towards regulation of stress tolerance and seed recalcitrance. Seed Sci. Res. 16, 203–211. doi: 10.1079/SSR2006252
Bobbert, T., Rochlitz, H., Wegewitz, U., Akpulat, S., Mai, K., Weickert, M. O.,et al. (2005). Changes of adiponectin oligomer composition by moderate weight reduction. Diabetes Metab. Res. Rev. 54, 2712–2719. doi: 10.2337/diabetes.54.9.2712
PubMed Abstract | Full Text | CrossRef Full Text | Google Scholar
Bodles, A. M., Banga, A., Rasouli, N., Ono, F., Kern, P. A., and Owens, R. J. (2006). Pioglitazone increases secretion of high-molecular-weight adiponectin from adipocytes. Am. J. Physiol. Endocrinol. Metab. 291, E1100–E1105. doi: 10.1152/ajpendo.00187.2006
PubMed Abstract | Full Text | CrossRef Full Text | Google Scholar
Bol, J. F., Linthorst, H. J. M., and Cornelissen, B. J. C. (1990). Plant pathogenesis-related proteins induced by virus infection. Annu. Rev. Phytopathol. 28, 113–138. doi: 10.1146/annurev.py.28.090190.000553
Bowles, D. J. (1990). Defense-related proteins in higher plants. Annu. Rev. Biochem. 59, 873–907. doi: 10.1146/annurev.bi.59.070190.004301
Boyer, J. S. (1982). Plant productivity and environment. Science 218, 443–448. doi: 10.1126/science.218.4571.443
PubMed Abstract | Full Text | CrossRef Full Text | Google Scholar
Brakenhielm, E., Veitonmaki, N., Cao, R., Kihara, S., Matsuzawa, Y., Zhivotovsky, B.,et al. (2004). Adiponectin-induced antiangiogenesis and antitumor activity involve caspase-mediated endothelial cell apoptosis. Proc. Natl. Acad. Sci. U.S.A. 101, 2476–2481. doi: 10.1073pnas.0308671100
PubMed Abstract | Full Text | CrossRef Full Text | Google Scholar
Bressan, R. A., Singh, N. K., Handa, A. K., Mount, R., Clithero, J., and Hasegawa, P. M. (1987). “Stability of altered genetic expression in cultured plant cells adapted to salt,” in Drought Resistance in Plants, Physiological and Genetic Aspects, eds L. Monti and E. Porceddu (Brussels: EEC), 41–58.
Brinker, M., Brosché, M., Vinocur, B., Abo-Ogiala, A., Fayyaz, P., Janz, D.,et al. (2010). Linking the salt transcriptome with physiological responses of a salt-resistant Populus species as a strategy to identify genes important for stress acclimation. Plant Physiol. 154, 1697–1709. doi: 10.1104/pp.110.164152
PubMed Abstract | Full Text | CrossRef Full Text | Google Scholar
Brune, A., Urbach, W., and Dietz, K. J. (1995). Differential toxicity of heavy metals is partly related to a loss of preferential extraplasmic compartmentation: a comparison of Cd-, Mo-, Ni-, and Zn-stress. New Phytol. 129, 404–409. doi: 10.1111/j.1469-8137.1995.tb04310.x
Capelli, N., Diogon, T., Greppin, H., and Simon, P. (1997). Isolation and characterization of cDNA clone encoding an osmotin-like protein from Arabidopsis thaliana. Gene 191, 51–56. doi: 10.1016/S0378-1119(97)00029-2
PubMed Abstract | Full Text | CrossRef Full Text | Google Scholar
Casas, A. M., Nelson, D. E., Raghothama, K. G., Paino, D. M., Singh, N. K., Bressan, R. A.,et al. (1992). Expression of osmotin-like genes in the halophyte Atriplex nummularia L. Plant Physiol. 99, 329–337. doi: 10.1104/pp.99.1.329
PubMed Abstract | Full Text | CrossRef Full Text | Google Scholar
Chen, D. (2012). Aspergillus niger Control on Strawberries by Recombinant Tobacco Osmotin for Extending Shelf-Life. Dissertation, Auburn University, Auburn, AL.
Chen, R., Wang, F., and Smith, A. G. (1996). A flower-specific gene encoding an osmotin-like protein from Lycoperiscon esculentum. Gene 179, 302–310. doi: 10.1016/S0378-1119(96)00399-X
Chen, X., and Guo, Z. (2008). Tobacco OPBP1 enhances salt tolerance and disease resistance of transgenic rice. Int. J. Mol. Sci. 9, 2601–2613. doi: 10.3390/ijms9122601
PubMed Abstract | Full Text | CrossRef Full Text | Google Scholar
Cheong, N. E., Choi, Y. O., Kim, W. Y., Bae, I. S., Cho, M. J., Hwang, I.,et al. (1997). Purification and characterization of an antifungal PR-5 protein from pumpkin leaves. Mol. Cells 7, 214–219.
Chisholm, S. T., Coaker, G., Day, B., and Staskawicz, B. J. (2006). Host-microbe interactions: shaping the evolution of the plant immune response. Cell 124, 803–814. doi: 10.1016/j.cell.2006.02.008
PubMed Abstract | Full Text | CrossRef Full Text | Google Scholar
Choi, D. S., Hong, J. K., and Hwan, B. K. (2013). Pepper osmotin-like protein 1 (CaOSM1) is an essential component for defense response, cell death, and oxidative burst in plants. Planta 238, 1113–1124. doi: 10.1007/s00425-013-1956-3
PubMed Abstract | Full Text | CrossRef Full Text | Google Scholar
Coca, M. A., Damsz, B., Yun, D.-J., Hasegawa, P. M., Bressan, R. A., and Narasimhan, M. L. (2000). Heterotrimeric G-proteins of a filamentous fungus regulate cell wall composition and susceptibility to a plant PR-5 protein. Plant J. 22, 61–69. doi: 10.1046/j.1365-313x.2000.00718.x
PubMed Abstract | Full Text | CrossRef Full Text | Google Scholar
Cornelissen, B. J., Hooft van Huijsduijnen, R. A., and Bol, J. F. (1986). A tobacco mosaic virus-induced protein is homologous to the sweet-tasting protein thaumatin. Nature 321, 531–532. doi: 10.1038/321531a0
PubMed Abstract | Full Text | CrossRef Full Text | Google Scholar
D’Angeli, S., and Altamura, M. M. (2007). Osmotin induces cold protection in olive trees by affecting programmed cell death and cytoskeleton organization. Planta 225, 1147–1163. doi: 10.1007/s00425-006-0426-6
PubMed Abstract | Full Text | CrossRef Full Text | Google Scholar
D’Angeli, S., Falasca, G., Matteucci, M., and Altamura, M. M. (2013). Cold perception and gene expression differ in Olea europaea seed coat and embryo during drupe cold acclimation. New Phytol. 197, 123–138. doi: 10.1111/j.1469-8137.2012.04372.x
PubMed Abstract | Full Text | CrossRef Full Text | Google Scholar
Das, M., Chauhan, H., Chhibbar, A., Haq, Q. M. R., and Khurana, P. (2011). High-efficiency transformation and selective tolerance against biotic and abiotic stress in mulberry, Morus indica cv. K2, by constitutive and inducible expression of tobacco osmotin. Transgenic Res. 20, 231–246. doi: 10.1007/s11248-010-9405-6
PubMed Abstract | Full Text | CrossRef Full Text | Google Scholar
de Freitas, C. D. T., Nogueira, F. C. S., Vasconcelos, I. M., Oliveira, J. T. A., Domont, G. B., and Ramos, M. V. (2011a). Osmotin purified from the latex of Calotropis procera: biochemical characterization, biological activity and role in plant defense. Plant Physiol. Biochem. 49, 738–743. doi: 10.1016/j.plaphy.2011.01.027
PubMed Abstract | Full Text | CrossRef Full Text | Google Scholar
de Freitas, C. D. T., Lopes, J. L. D. S., Beltramini, L. M., de Oliveira, R. S. B., Oliveira, J. T. A., and Ramos, M. V. (2011b). Osmotin from Calotropis procera latex: new insights into structure and antifungal properties. Biochim. Biophys. Acta 1808, 2501–2507. doi: 10.1016/j.bbamem.2011.07.014
PubMed Abstract | Full Text | CrossRef Full Text | Google Scholar
de Vos, A. M., Hatada, M., van der Wel, H., Krabbendam, H., Peerdeman, A. F., and Kim, S. H. (1985). Three-dimensional structure of thaumatin I, an intensely sweet protein. Proc. Natl. Acad. Sci. U.S.A. 82, 1406–1409. doi: 10.1073/pnas.82.5.1406
Dhindsa, R. S., and Matowe, W. (1981). Drought tolerance in two mosses correlated with enzymatic defense against lipid peroxidation. J. Exp. Bot. 22, 79–91. doi: 10.1093/jxb/32.1.79
Ebel, J. (1998). Oligoglucoside elicitor-mediated activation of plant defense. Bioessays 20, 569–576. doi: 10.1002/(SICI)1521-1878(199807)20
PubMed Abstract | Full Text | CrossRef Full Text | Google Scholar
Edens, L., Heslinga, L., Klok, R., Ledeboer, A. M., Maat, J., Toonen, M. Y.,et al. (1982). Cloning of cDNA encoding the sweet-tasting plant protein thaumatin and its expression in Escherichia coli. Gene 18, 1–12. doi: 10.1016/0378-1119(82)90050-6
PubMed Abstract | Full Text | CrossRef Full Text | Google Scholar
El-Komy, M. H., Abou-taleb, E. M., Aboshosha, S. M., and El-sherif, E. M. (2010). Differential expression of potato pathogenesis-related proteins upon infection with late blight pathogen: a case study expression of potato osmotin-like protein. Int. J. Agric. Biol. 12, 179–186.
Elvira, M. I., Galdeano, M. M., Gilardi, P., García-Luque, I., and Serra, M. T. (2008). Proteomic analysis of pathogenesis-related proteins (PRs) induced by compatible and incompatible interactions of pepper mild mottle virus (PMMoV) in Capsicum chinense L3 plants. J. Exp. Bot. 59, 1253–1265. doi: 10.1093/jxb/ern032
PubMed Abstract | Full Text | CrossRef Full Text | Google Scholar
Evers, D., Overney, S., Simon, P., Greppin, H., and Hausman, J. F. (1999). Salt tolerance of Solanum tuberosum L. overexpressing an heterologous osmotin-like protein. Biol. Plant 42, 105–112. doi: 10.1023/A:1002131812340
Fatima, T., Teasdale, J. R., Bunce, J., Autar, K., and Mattoo, A. K. (2012). Tomato response to legume cover crop and nitrogen: differing enhancement patterns of fruit yield, photosynthesis and gene expression. Funct. Plant Biol. 39, 246–254. doi: 10.1071/FP1124
Flier, J. S. (2004). Obesity wars: molecular progress confronts an expanding epdemic. Cell 116, 337–350. doi: 10.1016/S0092-8674(03)01081-X
PubMed Abstract | Full Text | CrossRef Full Text | Google Scholar
Floyd, R. A., and Nagy, Z. S. (1984). Formation of long lived hydroxyl free radical adducts of proline and hydroxyl-proline in a fenton reaction. Biochim. Biophys. Acta 790, 94–97. doi: 10.1016/0167-4838(84)90337-6
Friedrich, C. L., Moyles, D., Beveridge, T. J., and Hancock, R. E. W. (2000). Antibacterial action of structurally diverse cationic peptides on gram-positive bacteria. Antimicrob. Agents Chemother. 44, 2086–2092. doi: 10.1128/AAC.44.8.2086-2092.2000
Fujita, T., Kouchi, H., Ichikawa, T., and Syonol, K. (1994). Cloning of cDNAs for genes that are specifically or preferentially expressed during the development of tobacco genetic tumors. Plant J. 5, 645–654. doi: 10.1111/j.1365-313X.1994.00645.x
Gill, S. S., and Tuteja, N. (2010). Reactive oxygen species and antioxidant machinery in abiotic stress tolerance in crop plants. Plant Physiol. Biochem. 48, 909–930. doi: 10.1016/j.plaphy.2010.08.016
PubMed Abstract | Full Text | CrossRef Full Text | Google Scholar
Gimeno, J., Gadea, J., Forment, J., Pérez-Valle, J., Santiago, J., Martínez-Godoy, M. A.,et al. (2009). Shared and novel molecular responses of mandarin to drought. Plant Mol. Biol. 70, 403–420. doi: 10.1007/s11103-009-9481-2
PubMed Abstract | Full Text | CrossRef Full Text | Google Scholar
Goel, D., Singh, A. K., Yadav, V., Babbar, S. B., and Bansal, K. C. (2010). Overexpression of osmotin gene confers tolerance to salt and drought stresses in transgenic tomato (Solanum lycopersicum L.). Protoplasma 245, 133–141. doi: 10.1007/s00709-010-0158-0
PubMed Abstract | Full Text | CrossRef Full Text | Google Scholar
Grenier, J., Potvin, C., Trudel, J., and Asselin, A. (1999). Some thaumatin-like proteins hydrolyze polymeric beta-1,3-glucans. Plant J. 19, 473–480. doi: 10.1046/j.1365-313X.1999.00551.x
Grillo, S., Leone, A., Xu, Y., Tucci, M., Francione, R., Hasegawa, P. M.,et al. (1995). Control of osmotin gene expression by ABA and osmotic stress in vegetative tissues of wild-type and ABA-deficient mutants of tomato. Physiol. Plant. 93, 498–504. doi: 10.1111/j.1399-3054.1995.tb06849.x
Grosset, J., Meyer, Y., Chartier, Y., Kauffmann, S., Legrand, M., and Fritig, B. (1990). Tobacco mesophyll protoplasts synthesize 1,3-beta-glucanase, chitinases, and “osmotins” during in vitro culture. Plant Physiol. 92, 520–527. doi: 10.1104/pp.92.2.520
PubMed Abstract | Full Text | CrossRef Full Text | Google Scholar
Hajheidari, M., Abdollahian-Noghabi, M., Askari, H., Heidari, M., Sadeghian, S. Y., Ober, E. S.,et al. (2005). Proteome analysis of sugar beet leaves under drought stress. Proteomics 5, 950–960. doi: 10.1002/pmic.200401101
Haqq, A. M., Muehlbauer, M., Svetkey, L. P., Newgard, C. B., Purnell, J. Q., Grambow, S. C.,et al. (2007). Altered distribution of adiponectin isoforms in children with Prader-Willi syndrome (PWS): association with insulin sensitivity and circulating satiety peptide hormones. Clin. Endocrinol. 6, 944–951. doi: 10.1111/j.1365-2265.2007.02991.x
PubMed Abstract | Full Text | CrossRef Full Text | Google Scholar
Harada, E., Kim, J. A., Meyer, A. J., Hell, R., Clemens, S., and Choi, Y. E. (2010). Expression profiling of tobacco leaf trichomes identifies genes for biotic and abiotic stresses. Plant Cell Physiol. 51, 1627–1637. doi: 10.1093/pcp/pcq118
PubMed Abstract | Full Text | CrossRef Full Text | Google Scholar
Helleboid, S., Hendriks, T., Bauw, G., Inze, D., Vasseur, J., and Hilbert, J. L. (2000). Three major somatic embryogenesis related proteins in Cichorium identified as PR proteins. J. Exp. Bot. 51, 1189–1200. doi: 10.1093/jexbot/51.348.1189
PubMed Abstract | Full Text | CrossRef Full Text | Google Scholar
Holland, W. L., Miller, R. A., Wang, Z. V., Sun, K., Barth, B. M., Bui, H. H.,et al. (2011). Receptor-mediated activation of ceramidase activity initiates the pleiotropic actions of adiponectin. Nat. Med. 17, 55–63. doi: 10.1038/nm.2277
PubMed Abstract | Full Text | CrossRef Full Text | Google Scholar
Holland, W. L., and Scherer, P. E. (2013). Ronning after the adiponectin receptors. Science 342, 1460–1461. doi: 10.1126/science.1249077
PubMed Abstract | Full Text | CrossRef Full Text | Google Scholar
Holmstrom, K. O., Somersalo, S., Mandal, A., Palva, T. E., and Welin, B. (2000). Improved tolerance to salinity and low temperature in transgenic tobacco producing glycine betaine. J. Exp. Bot. 51, 177–185. doi: 10.1093/jexbot/51.343.177
PubMed Abstract | Full Text | CrossRef Full Text | Google Scholar
Hong, J. K., Jung, H. W., Lee, B. K., Lee, S. C., Lee, J. Y., and Hwang, B. K. (2004). An osmotin-like protein gene, CAOSM1, from pepper: differential expression and in situ localization of its mRNA during pathogen infection and abiotic stress. Physiol. Mol. Plant Pathol. 64, 301–310. doi: 10.1016/j.pmpp.2004.10.004
Hong, Z., Lakkineni, K., Zhang, Z., and Verma, D. P. S. (2000). Removal of feedback inhibition of delta(1)-pyrroline-5-carboxylate synthetase results in increased proline accumulation and protection of plants from osmotic stress. Plant Physiol. 122, 1129–1136. doi: 10.1104/pp.122.4.1129
Hu, E., Liang, P., and Spiegelman, B. M. (1996). AdipoQ is a novel adipose-specific gene dysregulated in obesity. J. Biol. Chem. 271, 10697–10703. doi: 10.1074/jbc.271.18.10697
PubMed Abstract | Full Text | CrossRef Full Text | Google Scholar
Hu, X., and Reddy, A. S. N. (1997). Cloning and expression of a PR5-like protein from Arabidopsis: inhibition of fungal growth by bacterially expressed protein. Plant Mol. Biol. 34, 949–959. doi: 10.1023/A:1005893119263
PubMed Abstract | Full Text | CrossRef Full Text | Google Scholar
Huang, J., Wang, H. M., Jiang, Y., Wang, Q. H., Huang, X., and Zhang, H. S. (2008). Stress repressive expression of rice SRZ1and characterization of plant SRZ gene family. Plant Sci. 174, 227–235. doi: 10.1016/j.plantsci.2007.11.010
Husaini, A. M., and Abdin, M. Z. (2008). Overexpression of tobacco osmotin gene leads to salt stress tolerance in strawberry (Fragaria x ananassa Duch.) plants. Indian J. Biotechnol. 7, 465–471.
Husaini, A. M., Abdin, M. Z., Khan, S., Xu, Y. W., Aquil, S., and Anis, M. (2012). Modifying strawberry for better adaptability to adverse impact of climate change. Curr. Sci. 102, 1660–1673.
Husaini, A. M., and Rafiqi, A. M. (2012). Role of osmotin in strawberry improvement. Plant Mol. Biol. Rep. 30, 1055–1064. doi: 10.1007/s11105-011-0394-2
Ibeas, J. I., Lee, H., Damsz, B., Prasad, D. T., Pardo, J. M., Hasegawa, P. M.,et al. (2000). Fungal cell wall phosphomannans facilitate the toxic activity of a plant PR-5 protein. Plant J. 23, 375–383. doi: 10.1046/j.1365-313x.2000.00792.x
PubMed Abstract | Full Text | CrossRef Full Text | Google Scholar
Ibeas, J. I., Yun, D.-J., Damsz, B., Narasimhan, M. L., Uesono, Y., Ribas, J. C.,et al. (2001). Resistance to the plant PR-5 protein osmotin in the model fungus Saccharomyces cerevisiae is mediated by the regulatory effects of SSD1 on cell wall composition. Plant J. 25, 271–280. doi: 10.1046/j.1365-313x.2001.00967.x
PubMed Abstract | Full Text | CrossRef Full Text | Google Scholar
Imin, N., Kerim, T., Weinman, J. J., and Rolfe, B. G. (2006). Low temperature treatment at the young microspore stage induces protein changes in rice anthers. Mol. Cell. Proteomics 5, 274–292. doi: 10.1074/mcp.M500242-MCP200
Ivic-Haymes, S. D., and Smigocki, A. C. (2005). Biolistic transformation of highly regenerative sugar beet (Beta vulgaris L.) leaves. Plant Cell Rep. 23, 699–704. doi: 10.1007/s00299-004-0873-0
PubMed Abstract | Full Text | CrossRef Full Text | Google Scholar
Jami, S. K., Anuradha, T. S., Guruprasad, L., and Kirti, P. B. (2007). Molecular, biochemical and structural characterization of osmotin-like protein from black nightshade (Solanum nigrum). J. Plant Physiol. 164, 238–252. doi: 10.1016/j.jplph.2006.01.006
PubMed Abstract | Full Text | CrossRef Full Text | Google Scholar
Jia, Y., and Martin, G. B. (1999). Rapid transcript accumulation of pathogenesis-related genes during an incompatible interaction in bacterial speck disease-resistant tomato plants. Plant Mol. Biol. 40, 455–465. doi: 10.1023/A:1006213324555
PubMed Abstract | Full Text | CrossRef Full Text | Google Scholar
Jonak, C., Kiegerl, S., Ligterink, W., Barker, P. J., Huskisson, N. S., and Hirt, H. (1996). Stress signaling in plants: a mitogen-activated protein kinase pathway is activated by cold and drought. Proc. Natl. Acad. Sci. U.S.A. 93, 11274–11279. doi: 10.1073/pnas.93.20.11274
Jones, J. D. G., and Dangl, J. L. (2006). The plant immune system. Nature 444, 323–329. doi: 10.1038/nature05286
PubMed Abstract | Full Text | CrossRef Full Text | Google Scholar
Jung, H. W., and Hwang, B. K. (2000). Isolation, partial sequencing, and expression of pathogenesis-related cDNA genes from pepper leaves infected by Xanthomonas campestris pv. Vesicatoria. Mol. Plant Microbe Interact. 13, 136–142. doi: 10.1094/MPMI.2000.13.1.136
PubMed Abstract | Full Text | CrossRef Full Text | Google Scholar
Kadowaki, T., and Yamauchi, T. (2005). Adiponectin and adiponectin receptors. Endocr. Rev. 26, 439–451. doi: 10.1210/er.2005-0005
PubMed Abstract | Full Text | CrossRef Full Text | Google Scholar
Kamada, Y., Tamura, S., Kiso, S., Matsumoto, H., Saji, Y., Yoshida, Y.,et al. (2003). Enhanced carbon tetrachloride-induced liver fibrosis in mice lacking adiponectin. Gastroenterology 125, 1796–1807. doi: 10.1053/j.gastro.2003.08.029
PubMed Abstract | Full Text | CrossRef Full Text | Google Scholar
Kancharla, J. R. (2011). Generation of Transgenic Medicago sativa Overexpressing “Osmotin-Chitinase” Gene Chimera. Dissertation, Western Kentucky University, Bowling Green, KY.
Kaplan, F., Kopka, J., Haskell, D. W., Zhao, W., Schiller, K. C., Gatzke, N.,et al. (2004). Exploring the temperature stress metabolome of Arabidopsis. Plant Physiol. 136, 4159–4168. doi: 10.1104/pp.104.052142
PubMed Abstract | Full Text | CrossRef Full Text | Google Scholar
Kawaguchi, R., Williams, A. J., Bray, E. A., and Bailey-serres, J. (2003). Water-deficit-induced translational control in Nicotiana tabacum. Plant Cell Environ. 26, 221–229. doi: 10.1046/j.1365-3040.2003.00952.x
Kessler, A., and Baldwin, I. T. (2002). Plant responses to insect herbivory: the emerging molecular analysis. Annu. Rev. Plant Biol. 53, 299–328. doi: 10.1146/annurev.arplant.53.100301.135207
PubMed Abstract | Full Text | CrossRef Full Text | Google Scholar
Kim, H., Mun, J.-H., Byun, B. H., Hwang, H. J., Kwon, Y. M., and Kim, S. G. (2002). Molecular cloning and characterization of the gene encoding osmotin protein in Petunia hybrida. Plant Sci. 162, 745–752. doi: 10.1016/S0168-9452(02)00016-X
Kishor, P. B. K., and Sreenivasulu, N. (2014). Is proline accumulation per se correlated with stress tolerance or is proline homeostasis a more critical issue? Plant Cell Environ. 37, 300–311. doi: 10.1111/pce.12157
PubMed Abstract | Full Text | CrossRef Full Text | Google Scholar
Kitajima, S., Koyama, T., Yamada, Y., and Sato, F. (1998). Constitutive expression of the neutral PR-5 (OLP, PR-5d) gene in roots and cultured cells of tobacco is mediated by ethylene-responsive cis-element AGCCGCC sequences. Plant Cell Rep. 18, 173–179. doi: 10.1007/s002990050552
Koiwa, H., Kato, H., Nakatsu, T., Oda, J., Yamada, Y., and Sato, F. (1999). Crystal structure of tobacco PR-5d protein at 1.8 Å resolution reveals a conserved acidic cleft structure in antifungal thaumatin-like proteins. J. Mol. Biol. 286, 1137–1145. doi: 10.1006/jmbi.1998.2540
PubMed Abstract | Full Text | CrossRef Full Text | Google Scholar
Koiwa, H., Sato, F., and Yamada, Y. (1994). Characterization of accumulation of tobacco PR-5 proteins by IEF-immunoblot analysis. Plant Cell Physiol. 35, 821–827.
Kononowicz, A. K., Nelson, D. E., Singh, N. K., Hasegawa, P. M., and Bressan, R. A. (1992). Regulation of the osmotin gene promoter. Plant Cell 4, 513–524. doi: 10.1105/tpc.4.5.513
Kononowicz, A. K., Raghothama, K. G., Casas, A. M., Nelson, D. E., Liu, D., Narasimhan, M. L.,et al. (1994). “Structure, regulation and function of the osmotin gene,” in Biochemical and Cellular Mechanisms of Stress Tolerance in Plants, ed. J. H. Cherry (Berlin: Springer-Verlag), 381–414. doi: 10.1007/978-3-642-79133-8_24
Koyama, T., Kitajima, S., and Sato, F. (2001). Expression of PR-5d and ERF genes in cultured tobacco cells and their NaCl stress-response. Biosci. Biotechnol. Biochem. 65, 1270–1273. doi: 10.1271/bbb.65.1270
PubMed Abstract | Full Text | CrossRef Full Text | Google Scholar
Kratsch, H. A., and Wise, R. R. (2000). The ultrastructure of chilling stress. Plant Cell Environ. 23, 337–350. doi: 10.1046/j.1365-3040.2000.00560.x
Kretschmer, M., Kassemeyer, H. H., and Hahn, M. (2007). Age-dependent grey mould susceptibility and tissue-specific defence gene activation of grapevine berry skins after infection by Botrytis cinerea. Phytopathology 155, 258–263. doi: 10.1111/j.1439-0434.2007.01216.x
Kumada, M., Kihara, S., Sumitsuji, S., Kawamoto, T., Matsumoto, S., Ouchi, N.,et al. (2003). Association of hypoadiponectinemia with coronary artery disease in men. Arterioscler. Thromb. Vasc. Biol. 23, 85–89. doi: 10.1161/01.ATV.0000048856.22331.50
Kumar, V., and Spencer, M. E. (1992). Nucleotide sequence of an osmotin cDNA from the Nicotiana tabacum cv. White Burley generated by the polymerase chain reaction. Plant Mol. Biol. 18, 621–622. doi: 10.1007/BF00040683
PubMed Abstract | Full Text | CrossRef Full Text | Google Scholar
Kupchak, B. R., Villa, N. Y., Kulemina, L. V., and Lyons, T. J. (2008). Dissecting the regulation of yeast genes by the osmotin receptor. Biochem. Biophys. Res. Commun. 374, 210–213. doi: 10.1016/j.bbrc.2008.07.002
PubMed Abstract | Full Text | CrossRef Full Text | Google Scholar
LaRosa, P. C., Chen, Z., Nelson, D. E., Singh, N. K., Hasegawa, P. M., and Bressan, R. A. (1992). Osmotin gene expression is posttranscriptionally regulated. Plant Physiol. 100, 409–415. doi: 10.1104/pp.100.1.409
PubMed Abstract | Full Text | CrossRef Full Text | Google Scholar
LaRosa, P. C., Hasegawa, P. M., Rhodes, D., Clithero, J. M., Watad, A. A., and Bressan, R. A. (1987). Abscisic acid stimulated osmotic adjustment and its involvement in adaptation of tobacco cells to NaCl. Plant Physiol. 85, 174–181. doi: 10.1104/pp.85.1.174
LaRosa, P. C., Singh, N. K., Hasegawa, P. M., and Bressan, R. A. (1989). Stable NaCl tolerance of tobacco cells is associated with enhanced accumulation of osmotin. Plant Physiol. 91, 855–861. doi: 10.1104/pp.91.3.855
PubMed Abstract | Full Text | CrossRef Full Text | Google Scholar
Lee, H., Damsz, B., Woloshuk, C. P., Bressan, R. A., and Narasimhan, M. L. (2010a). Use of the plant defense protein osmotin to identify Fusarium oxysporum genes that control cell wall properties. Eukaryot. Cell 9, 558–568. doi: 10.1128/EC.00316-09
PubMed Abstract | Full Text | CrossRef Full Text | Google Scholar
Lee, S. C., Choi, D. S., Hwang, I. S., and Hwang, B. K. (2010b). The pepper oxidoreductase CaOXR1 interacts with the transcription factor CaRAV1 and is required for salt and osmotic stress tolerance. Plant Mol. Biol. 73, 409–424. doi: 10.1007/s11103-010-9629-0
PubMed Abstract | Full Text | CrossRef Full Text | Google Scholar
Lee, J. H., and Kim, W. K. (2003). Molecular and biochemical characterization of VR-EILs encoding mung bean ethylene insensitive3-like proteins. Plant Physiol. 132, 1475–1488. doi: 10.1104/pp.103.022574
PubMed Abstract | Full Text | CrossRef Full Text | Google Scholar
Lee, S. C., and Hwang, B. K. (2005). Induction of some defense related genes and oxidative burst is required for the establishment of systemic acquired resistance in Capsicum annuum. Planta 221, 790–800. doi: 10.1007/s00425-005-1488-6
PubMed Abstract | Full Text | CrossRef Full Text | Google Scholar
Li, R., Wu, N., Fan, Y., and Song, B. (1999). Transgenic potato plants expressing osmotin gene inhibits fungal development in inoculated leaves. Chin. J. Biotechnol. 15, 71–75.
Linthotst, H. J. M., and Van Loon, L. C. (1991). Pathogenesis-related proteins of plants. Rev. Plant Sci. 10, 123–150. doi: 10.1080/07352689109382309
Liu, D., Raghothama, K. G., Hasegawa, P. M., and Bressan, R. A. (1994). Osmotin overexpression in potato delays development of disease symptoms. Proc. Natl. Acad. Sci. U.S.A. 91, 1888–1892. doi: 10.1073/pnas.91.5.1888
PubMed Abstract | Full Text | CrossRef Full Text | Google Scholar
Liu, D., Rhodes, D., D’Urzo, M. P., Xu, Y., Narsimhan, M. L., Hasegawa, P. M.,et al. (1996). In vivo and in vitro activity of truncated osmotin that is secreted into the extracellular matrix. Plant Sci. 121, 123–131. doi: 10.1016/S0168-9452(96)04514-1
Liu, Q., Ingersoll, J., Owens, L., Salih, S., Meng, R., and Hammerschlag, F. (2001). Response of transgenic royal gala apple (Malus × domestica Borkh.) shoots carrying a modified cecropin MB39gene, to Erwinia amylovora. Plant Cell Rep. 20, 306–312. doi: 10.1007/s002990100333
Lodge, J. K., Kaniewski, W. K., and Tumer, N. E. (1993). Broad-spectrum virus resistance in transgenic plants ex-pressing pokeweed antiviral protein. Proc. Natl. Acad. Sci. U.S.A. 90, 7089–7093. doi: 10.1073/pnas.90.15.7089
Los, D. A., and Murata, N. (2004). Membrane fluidity and its roles in the perception of environmental signals. Biochim. Biophys. Acta 1666, 142–157. doi: 10.1016/j.bbamem.2004.08.002
PubMed Abstract | Full Text | CrossRef Full Text | Google Scholar
Lutts, S., Kinet, J. M., and Bouharmont, J. (1996). Effects of salt stress on growth, mineral nutrition and proline accumulation in relation to osmotic adjustment in rice (Oryza sativa L.) cultivars differing in salinity resistance. Plant Growth Regul. 19, 207–218. doi: 10.1007/BF00037793
Maeda, K., Okubo, K., Shimomura, I., Funahashi, T., Matsuzawa, Y., and Matsubara, K. (1996). cDNA cloning and expression of a novel adipose specific collagen-like factor, apM1 (AdiPose most abundant gene transcript 1). Biochem. Biophys. Res. Commun. 221, 286–289. doi: 10.1006/bbrc.1996.0587
PubMed Abstract | Full Text | CrossRef Full Text | Google Scholar
Malehorn, D. E., Borgmeyer, J. R., Smith, C. E., and Shah, D. M. (1994). Characterization and expression of an antifungal zeamatin-like protein (Zlp) gene from Zea mays. Plant Physiol. 106, 1471–1481. doi: 10.1104/pp.106.4.1471
PubMed Abstract | Full Text | CrossRef Full Text | Google Scholar
Mani, T., Sivakumar, K. C., and Manjula, S. (2012). Expression and functional analysis of two osmotin (PR5) isoforms with differential antifungal activity from Piper colubrinum: prediction of structure–function relationship by bioinformatics approach. Mol. Biotechnol. 52, 251–261. doi: 10.1007/s12033-011-9489-0
PubMed Abstract | Full Text | CrossRef Full Text | Google Scholar
Margaria, P., and Palmano, S. (2011). Response of the Vitis vinifera L. cv. ‘Nebbiolo’ proteome to Flavescence dore’e phytoplasma infection. Proteomics 11, 212–224. doi: 10.1002/pmic.201000409
PubMed Abstract | Full Text | CrossRef Full Text | Google Scholar
Melchers, L. S., Sela-Buurlage, M. B., Vloemans, S. A., Woloshuk, C. P., Roekel, J. S. C. V., Pen, J.,et al. (1993). Extracellular targeting of the vacuolar tobacco proteins AP24, chitinase and β-1,3-glucanase in transgenic plants. Plant Mol. Biol. 21, 583–593. doi: 10.1007/bf00014542
Miele, M., Costantini, S., and Colonna, G. (2011). Structural and functional similarities between osmotin from Nicotiana tabacum seeds and human adiponectin. PLoS ONE 6:e16690. doi: 10.1371/journal.pone.0016690
PubMed Abstract | Full Text | CrossRef Full Text | Google Scholar
Mikolajczyk, M., Awotunde, O. S., Muszynska, G., Klessig, D. F., and Dobrowolska, G. (2000). Osmotic stress induces rapid activation of a salicylic acid-induced protein kinase and a homolog of protein kinase ASK1 in tobacco cells. Plant Cell 12, 165–178. doi: 10.1105/tpc.12.1.165
PubMed Abstract | Full Text | CrossRef Full Text | Google Scholar
Min, K., Ha, S. C., Hasegawa, P. M., Bressan, R. A., Yun, D. J., and Kim, K. K. (2004). Crystal structure of osmotin, a plant antifungal protein. Proteins 54, 170–173. doi: 10.1002/prot.10571
PubMed Abstract | Full Text | CrossRef Full Text | Google Scholar
Miyama, M., and Tada, Y. (2011). Expression of Bruguiera gymnorhizaBgARP1 enhances salt tolerance in transgenic Arabidopsis plants. Euphytica 177, 383–392. doi: 10.1007/s10681-010-0264-2
Monteiro, S., Picarra-Pereira, M. A., Teixeira, A. R., Loureiro, V. B., and Ferreira, R. B. (2003a). Environmental conditions during vegetative growth determine the major proteins that accumulate in mature grapes. J. Agric. Food Chem. 51, 4046-4053. doi: 10.1021/jf020456v
PubMed Abstract | Full Text | CrossRef Full Text | Google Scholar
Monteiro, S., Barakat, M., Piçarra-Pereira, M. A., Teixeira, A. R., and Ferreira, R. B. (2003b). Osmotin and thaumatin from grape: a putative general defense mechanism against pathogenic fungi. Phytopathology 93, 1505–1512. doi: 10.1094/phyto.2003.93.12.1505
PubMed Abstract | Full Text | CrossRef Full Text | Google Scholar
Mukherjee, A. K., Carp, M. J., Zuchman, R., Ziv, T., Horwitz, B. A., and Gepstein, S. (2010). Proteomics of the response of Arabidopsis thaliana to infection with Alternaria brassicicola. J. Proteom. 73, 709–720. doi: 10.1016/j.jprot.2009.10.005
PubMed Abstract | Full Text | CrossRef Full Text | Google Scholar
Nakano, Y., Tobe, T., Choi-Miura, N. H., Mazda, T., and Tomita, M. (1996). Isolation and characterization of GBP28, a novel gelatin-binding protein purified from human plasma. J. Biochem. 120, 803–812. doi: 10.1093/oxfordjournals.jbchem.a021483
Nanjo, T., Kobayashi, M., Yoshiba, Y., Sanada, Y., Wada, K., Tsukaya, H.,et al. (1999). Biological functions of proline in morphogenesis and osmotolerance revealed in antisense transgenic Arabidopsis thaliana. Plant J. 18, 185–193. doi: 10.1046/j.1365-313X.1999.00438.x
Narasimhan, M. L., Coca, M. A., Jin, J., Yamauchi, T., Ito, Y., Kadowaki, T.,et al. (2005). Osmotin is a homolog of mammalian adiponectin and controls apoptosis in yeast through a homolog of mammalian adiponectin receptor. Mol. Cell. 17, 171–180. doi: 10.1016/j.molcel.2004.11.050
PubMed Abstract | Full Text | CrossRef Full Text | Google Scholar
Narasimhan, M. L., Damsz, B., Coca, M. A., Ibeas, J. I., Yun, D. J., Pardo, J. M.,et al. (2001). A plant defense response effector induces microbial apoptosis. Mol. Cell. 8, 921–930. doi: 10.1016/S1097-2765(01)00365-3
PubMed Abstract | Full Text | CrossRef Full Text | Google Scholar
Narasimhan, M. L., Lee, H., Damsz, B., Singh, N. K., Ibeas, J. I., Matsumoto, T. K.,et al. (2003). Overexpression of a cell wall glycoprotein in Fusarium oxysporum increases virulence and resistance to a plant PR-5 protein. Plant J. 36, 390–400. doi: 10.1046/j.1365-313X.2003.01886.x
PubMed Abstract | Full Text | CrossRef Full Text | Google Scholar
Naseer, M. I., Ullah, I., Narasimhan, M. L., Lee, H. Y., Bressan, R. A., Yoon, G. H.,et al. (2014). Neuroprotective effect of osmotin against ethanol-induced apoptotic neurodegeneration in the developing rat brain. Cell Death Dis. 5, e1150. doi: 10.1038/cddis.2014.53
PubMed Abstract | Full Text | CrossRef Full Text | Google Scholar
Nawrocki, A. R., Rajala, M. W., Tomas, E., Pajvani, U. B., Saha, A. K., Trumbauer, M. E.,et al. (2006). Mice lacking adiponectin show decreased hepatic insulin sensitivity and reduced responsiveness to peroxisome proliferator-activated receptor agonists. J. Biol. Chem. 281, 2654–2660. doi: 10.1074/jbc.M505311200
PubMed Abstract | Full Text | CrossRef Full Text | Google Scholar
Neale, A. D., Wahleithner, J. A., Lund, M., Bonnett, H. T., Kelly, A., Meekswagner, D. R.,et al. (1990). Chitinase, beta-1,3-glucanase, osmotin, and extensin are expressed in tobacco explants during flower formation. Plant Cell 2, 673–684. doi: 10.1105/tpc.2.7.673
PubMed Abstract | Full Text | CrossRef Full Text | Google Scholar
Nelson, D. E., Raghothama, K. G., Singh, N. K., Hasegawa, P. M., and Bressan, R. A. (1992). Analysis of structure and transcriptional activation of an osmotin gene. Plant Mol. Biol. 19, 577–588. doi: 10.1007/BF00026784
PubMed Abstract | Full Text | CrossRef Full Text | Google Scholar
Newton, S. S., and Duman, J. G. (2000). An osmotin-like cryoprotective protein from the bitter sweet nightshade Solanum dulcamara. Plant Mol. Biol. 44, 581–589. doi: 10.1023/A:1026599028063
Niu, X., Li, X., Veronese, P., Bressan, R. A., Weller, S. C., and Hasegawa, P. M.(2000). Factors affecting Agrobacterium tumefaciens-mediated transformation of peppermint. Plant Cell Rep. 19, 304–310. doi: 10.1007/s002990050017
Noori, S. A. S., and Sokhansanj, A. (2008). Wheat plants containing an osmotin gene show enhanced ability to produce roots at high NaCl concentration. Russ. J. Plant Physiol. 55, 256–258. doi: 10.1134/S1021443708020143
Oerke, E. C. (2006). Crop losses to pests. J. Agric. Sci. 144, 31–43. doi: 10.1017/S0021859605005708
Ogata, C. M., Gordon, P. F., deVos, A. M., and Kim, S. H. (1992). Crystal structure of a sweet tasting protein thaumatin I, at 1.65 A resolution. J. Mol. Biol. 228, 893–908. doi: 10.1016/0022-2836(92)90873-I
PubMed Abstract | Full Text | CrossRef Full Text | Google Scholar
Okada-Iwabu, M., Yamauchi, T., Iwabu, M., Honma, T., Hamagami, K.-I., Matsuda, K.,et al. (2013). A small-molecule AdipoR agonist for type 2 diabetes and short life in obesity. Nature 503, 493–499. doi: 10.1038/nature12656
PubMed Abstract | Full Text | CrossRef Full Text | Google Scholar
Okamoto, Y., Kihara, S., Ouchi, N., Nishida, M., Arita, Y., Kumada, M.,et al. (2002). Adiponectin reduces atherosclerosis in apolipoprotein E-deficient mice. Circulation 106, 2767–2770. doi: 10.1161/01.CIR.0000042707.50032.19
Okuda, T., Fukui, M., Takayana, T., and Yokotsuka, K. (2006). Characterization of major stable proteins in Chardonnay Wine. Food Sci. Technol. Res. 12, 131–136. doi: 10.3136/fstr.12.131
Onishi, M., Tachi, H., Kojima, T., and Takahara, H. (2006). Molecular cloning and characterization of a novel salt-inducible gene encoding an acidic isoform of PR-5 protein in soybean (Glycine max [L.]Merr.). Plant Physiol. Biochem. 44, 574–580. doi: 10.1016/j.plaphy.2006.09.009
PubMed Abstract | Full Text | CrossRef Full Text | Google Scholar
Ouyang, B., Chen, Y. H., Li, H. X., Qian, C. J., Huang, S. L., and Ye, Z. B. (2005). Transformation of tomatoes with osmotin and chitinase genes and their resistance to Fusarium wilt. J. Horticult. Sci. Biotechnol. 80, 517–522.
Pajvani, U. B., and Scherer, P. E. (2003). Adiponectin: systemic contributor to insulin sensitivity. Curr. Diab. Rep. 3, 207–213. doi: 10.1007/s11892-003-0065-2
Parent, C., Berger, A., Folzer, H., Dat, J., Crevecoeur, M., Badot, P. M.,et al. (2008). A novel nonsymbiotic hemoglobin from oak: cellular and tissue specificity of gene expression. New. Phytol. 177, 142–154. doi: 10.1111/j.1469-8137.2007.02250.x
PubMed Abstract | Full Text | CrossRef Full Text | Google Scholar
Parkhi, V., Kumar, V., Sunilkumar, G., Campbell, L. A. M., Singh, N. K., and Rathore, K. S. (2009). Expression of apoplastically secreted tobacco osmotin in cotton confers drought tolerance. Mol. Breed. 23, 625–639. doi: 10.1007/s11032-009-9261-3
Patade, V. Y., Khatri, D., Manoj, K., Kumari, M., and Ahmed, Z. (2012). Cold tolerance in thiourea primed Capsicum seedlings is associated with transcript regulation of stress responsive genes. Mol. Biol. Rep. 39, 10603–10613. doi: 10.1007/s11033-012-1948-6
PubMed Abstract | Full Text | CrossRef Full Text | Google Scholar
Pereira, R. I., Snell-Bergeon, J. K., Erickson, C., Schauer, I. E., Bergman, B. C., Rewers, M.,et al. (2012). Adiponectin dysregulation and insulin resistance in type 1 diabetes. J. Clin. Endocrinol. Metab. 97, E642–E647. doi: 10.1210/jc.2011-2542
PubMed Abstract | Full Text | CrossRef Full Text | Google Scholar
Pla, M., Huguet, G., Verdaguer, D., Puigderrajols, P., Llompart, B., Nadal, A.,et al. (1998). Stress proteins co-expressed in suberized and lignified cells and in apical meristems. Plant Sci. 139, 49–57. doi: 10.1016/S0168-9452(98)00169-1
Plessl, M., Elstner, E. F., Rennenberg, H., Habermeyer, J., and Heiser, I. (2007). Influence of elevated CO2 and ozone concentrations on late blight resistance and growth of potato plants. Environ. Exp. Bot. 60, 447–457. doi: 10.1016/j.envexpbot.2007.01.003
Poupard, P., Parisi, L., Campion, C., Ziadi, S., and Simo-neau, P. (2003). A wound and ethephon inducible PR-10 gene subclass from apple is differentially expressed during infection with a compatible and incompatible race of Venturiainaequalis. Physiol. Mol. Plant Pathol. 62, 3–12. doi: 10.1016/S0885-5765(03)00008-0
Qi, Y., Takahashi, N., Hileman, S. M., Patel, H. R., Berg, A. H., Pajvani, U. B.,et al. (2004). Adiponectin acts in the brain to decrease body weight. Nat. Med. 10, 524–529. doi: 10.1038/nm1029
Qin, J., Zuo, K., Zhao, J., Ling, H., Cao, Y., Qiu, C.,et al. (2006). Overexpression of GbERF confers alteration of ethylene-responsivegene expression and enhanced resistance to Pseudomonas syringae in transgenic tobacco. J. Biosci. 31, 255–263. doi: 10.1007/BF02703918
PubMed Abstract | Full Text | CrossRef Full Text | Google Scholar
Qureshi, M. I., Qadir, S., and Zoll, L. (2007). Proteomics based dissection of stress-responsive pathways in plants. Plant Physiol. 164, 1239–1260. doi: 10.1016/j.jplph.2007.01.013
PubMed Abstract | Full Text | CrossRef Full Text | Google Scholar
Raghothama, K. G., Liu, D., Nelson, D. E., Hasegawa, P. M., and Bressan, R. A. (1993). Analysis of an osmotically regulated pathogenesis-related osmotin gene promoter. Plant Mol. Biol. 23, 117–1128. doi: 10.1007/BF00042346
PubMed Abstract | Full Text | CrossRef Full Text | Google Scholar
Raghothama, K. G., Maggio, A., Narasimhan, M. L., Kononowicz, A. K., Wang, G. L., Durzo, M. P.,et al. (1997). Tissue-specific activation of the osmotin gene by ABA, C2H4 and NaCl involves the same promoter region. Plant Mol. Biol. 34, 393–402. doi: 10.1023/A:1005812217945
PubMed Abstract | Full Text | CrossRef Full Text | Google Scholar
Randhawa, G. J., Singh, M., Chhabra, R., Guleria, S., and Sharma, R. (2009). Molecular diagnosis of transgenic tomato with osmotin gene using multiplex polymerase chain reaction. Curr. Sci. 96, 689–694.
Rao, M. V. R., Parameswari, C., Sripriya, R., and Veluthambi, K. (2011). Transgene stacking and marker elimination in transgenic rice by sequential Agrobacterium-mediated co-transformation with the same selectable marker gene. Plant Cell Rep. 30, 1241–1252. doi: 10.1007/s00299-011-1033-y
PubMed Abstract | Full Text | CrossRef Full Text | Google Scholar
Retnakaran, R., Connelly, P. W., Maguire, G., Sermer, M., Zinman, B., and Hanley, A. J. (2007). Decreased high-molecular-weight adiponectin in gestational diabetes: implications for the pathophysiology of type 2 diabetes. Diabet. Med. 3, 245–252. doi: 10.1111/j.1464-5491.2007.02077.x
PubMed Abstract | Full Text | CrossRef Full Text | Google Scholar
Richardson, M., Valdes-rodriguez, S., and Blanco-labia, A. (1987). A possible function for thaumatin and a TMV-induced protein suggested by homology to a maize inhibitor. Nature 327, 432–434. doi: 10.1038/327432a0
Risso, A., Zanetti, M., and Gennaro, R. (1998). Cytotoxicity and apoptosis mediated by two peptides of innate immunity. Cell Immunol. 189, 107–115. doi: 10.1006/cimm.1998.1358
Rivero, M., Furman, N., Mencaccia, N., Picca, P., Toum, L., Lentz, E.,et al. (2012). Stacking of antimicrobial genes in potato transgenic plants confers increased resistance to bacterial and fungal pathogens. J. Biotechnol. 157, 334–343. doi: 10.1016/j.jbiotec.2011.11.005
PubMed Abstract | Full Text | CrossRef Full Text | Google Scholar
Roberts, W. K., and Selitrennikoff, C. P. (1990). Zeamatin, an antifungal protein from maize with membrane-permeabilizing activity. J. Gen. Microbiol. 136, 1771–1778. doi: 10.1099/00221287-136-9-1771
Rodriguez, M., Canales, E., and Borras-Hidalgo, O. (2005). Molecular aspects of abiotic stress in plants. Biotecnol. Apl. 22, 1–10.
Rossel, J. B., Wilson, I. W., and Pogson, B. J. (2002). Global changes in gene expression in response to high light in Arabidopsis. Plant Physiol. 130, 1109–1120. doi: 10.1104/pp.005595
PubMed Abstract | Full Text | CrossRef Full Text | Google Scholar
Salani, B., Briatore, L., Andraghetti, G., Adami, G. F., Maggi, D., and Cordera, R. (2006). High-molecular weight adiponectin isoforms increase after biliopancreatic diversion in obese subjects. Obesity 14, 1511–1514. doi: 10.1038/oby.2006.174
PubMed Abstract | Full Text | CrossRef Full Text | Google Scholar
Salzman, R. A., Koiwa, H., Ibeas, J. I., Pardo, J. M., Hasegawa, P. M., and Bressan, R. A. (2004). Inorganic cations mediate plant PR5 protein antifungal activity through fungal Mnn1- and Mnn4-regulated cell surface glycans. Mol. Plant Microb. Interact. 17, 780–788. doi: 10.1094/MPMI.2004.17.7.780
PubMed Abstract | Full Text | CrossRef Full Text | Google Scholar
Salzman, R. A., Tikhonova, I., Bordelon, B. P., Hasegawa, P. M., and Bressan, R. A. (1998). Coordinate accumulation of antifungal proteins and hexoses constitutes a developmentally controlled defense response during fruit ripening in grape. Plant Physiol. 117, 465–472. doi: 10.1104/pp.117.2.465
PubMed Abstract | Full Text | CrossRef Full Text | Google Scholar
Sanchez, D. H., Siahpoosh, M. R., Roessner, U., Ud-vardi, M., and Kopka, J. (2008). Plant metabolomics reveals conserved and divergent metabolic responses to salinity. Physiol. Plant. 132, 209–219. doi: 10.1111/j.1399-3054.2007.00993.x
PubMed Abstract | Full Text | CrossRef Full Text | Google Scholar
Sarad, N., Rathore, M., Singh, N. K., and Kumar, N. (2004). “Genetically engineered tomatoes: new vista for sustainable agriculture in high altitude regions,” in Proceedings of the 4th International Crop Science Congress (Brisbane, QLD), 234–238.
Sato, F., Kitajima, S., Koyama, T., and Yamada, Y. (1996). Ethylene-induced gene expression of osmotin-like protein, a neutral isoform of tobacco PR-5, is mediated by the AGCCGCC cis-sequence. Plant Cell Physiol. 37, 249–255. doi: 10.1093/oxfordjournals.pcp.a028939
PubMed Abstract | Full Text | CrossRef Full Text | Google Scholar
Scherer, P. E., Williams, S., Fogliano, M., Baldini, G., and Lodish, H. F. (1995). A novel serum protein similar to C1q, produced exclusively in adipocytes. J. Biol. Chem. 270, 26746–26749. doi: 10.1074/jbc.270.45.26746
PubMed Abstract | Full Text | CrossRef Full Text | Google Scholar
Selitrennikoff, C. (2001). Antifungal proteins. Appl. Environ. Microbiol. 67, 2883–2894. doi: 10.1128/AEM.67.7.2883-2894.2001
PubMed Abstract | Full Text | CrossRef Full Text | Google Scholar
Sharma, P., Gaur, S. N., Arora, N. (2013a). In silico identification of IgE-binding epitopes of osmotin protein. PLoS ONE 8:e54755. doi: 10.1371/journal.pone.0054755
PubMed Abstract | Full Text | CrossRef Full Text | Google Scholar
Sharma, S., Lin, W., Villamor, J. G., and Verslues, P. E. (2013b). Divergent low water potential response in Arabidopsis thaliana accessions Landsberg erecta and Shahdara. Plant Cell Environ. 36, 994–1008. doi: 10.1111/pce.12032
PubMed Abstract | Full Text | CrossRef Full Text | Google Scholar
Sharma, P., Singh, A. K., Singh, B. P., Gaur, S. N., and Arora, N. (2011). Allergenicity assessment of osmotin, a pathogenesis-related protein, used for transgenic crops. J. Agric. Food Chem. 59, 9990–9995. doi: 10.1021/jf202265d
PubMed Abstract | Full Text | CrossRef Full Text | Google Scholar
Shih, C.-Y. T., Wu, J., Jia, S., Khan, A. A., Ting, K.-L. H., and Shih, D. S. (2001). Purification of an osmotin-like protein from the seeds of Benincasa hispida and cloning of the gene encoding this protein. Plant Sci. 160, 817–826. doi: 10.1016/S0168-9452(00)00450-7
PubMed Abstract | Full Text | CrossRef Full Text | Google Scholar
Siahsar, B. A., Sarani, S., and Allahdoo, M. (2011). Polypeptide electrophoretic pattern of Matricaria chamomilla and Anthemis nobilis under salt and Fe deficiency stress. African J. Biotechnol. 10, 11182–11185. doi: 10.5897/AJB11.476
Singh, N. K., Bracker, C. A., Hasegawa, P. M., Handa, A. K., Buckel, S., Hermodson, M. A.,et al. (1987a). Characterization of osmotin: a thaumatin-like protein associated with osmotic adaptation in plant cells. Plant Physiol. 85, 529–536. doi: 10.1104/pp.85.2.529
Singh, N. K., LaRosa, P. C., Handa, A. K., Hasegawa, P. M., and Bressan, R. A. (1987b). Hormonal regulation of protein synthesis associated with salt tolerance in plant cells. Proc. Natl. Acad. Sci. U.S.A. 84, 739–743. doi: 10.1073/pnas.84.3.739
PubMed Abstract | Full Text | CrossRef Full Text | Google Scholar
Singh, N. K., Handa, A. K., Hasegawa, P. M., and Bressan, R. A. (1985). Proteins associated with adaptation of cultured tobacco cells to NaCl. Plant Physiol. 79, 126–137. doi: 10.1104/pp.79.1.126
Singh, N. K., Nelson, D. E., Kuhn, D., Hasegawa, P. M., and Bressan, R. A. (1989). Molecular cloning of osmotin and regulation of its expression by ABA and adaptation to low water potential. Plant Physiol. 90, 1096–1101. doi: 10.1104/pp.90.3.1096
PubMed Abstract | Full Text | CrossRef Full Text | Google Scholar
Skriver, K., and Mundy, J. (1990). Gene expression in response to abscisic acid and osmotic stress. Plant Cell 2, 503–512. doi: 10.1105/tpc.2.6.503
PubMed Abstract | Full Text | CrossRef Full Text | Google Scholar
Slootstra, J. W., De Geus, P., Haas, H., Verrips, C. T., and Meloen, R. H. (1995). Possible active site of the sweet-tasting protein thaumatin. Chem. Senses. 20, 535–543. doi: 10.1093/chemse/20.5.535
PubMed Abstract | Full Text | CrossRef Full Text | Google Scholar
Snyder, G. W., Ingersoll, J. C., Smigocki, A. C., and Owens, L. D. (1999). Introduction of pathogen defense genes and a cytokinin biosynthesis gene into sugarbeet (Beta vulgaris L.) by Agrobacterium or particle bombardment. Plant Cell Rep. 18, 829–834. doi: 10.1007/s002990050669
Sokhansanj, A., Noori, S. A. S., and Niknam, V. (2006). Comparison of bacterial and plant genes participating in proline biosynthesis with osmotin gene, with respect to enhancing salinity tolerance of transgenic tobacco plants. Russ. J. Plant Physiol. 53, 110–115. doi: 10.1134/S1021443706010146
Souza, D. P., Freitas, C. D. T., Pereira, D. A., Nogueira, F. C., Silva, F. D. A., Salas, C. E.,et al. (2011). Laticifer proteins play a defensive role against hemibiotrophic and necrotrophic phytopathogens. Planta 234, 183–193. doi: 10.1007/s00425-011-1392-1
PubMed Abstract | Full Text | CrossRef Full Text | Google Scholar
Sripriya, R., Sangeetha, M., Parameswari, C., Veluthambi, B., and Veluthambi, K. (2011). Improved Agrobacterium-mediated cotransformation and selectable marker elimination in transgenic rice by using a high copy number pBin19-derived binary vector. Plant Sci. 180, 766–774. doi: 10.1016/j.plantsci.2011.02.010
PubMed Abstract | Full Text | CrossRef Full Text | Google Scholar
Stintzi, A., Heitz, T. S., Kauffmann, S., Legrand, M., and Fritig, B. (1991). Identification of a basic pathogenesis-related, thaumatin-like protein of virus-infected tobacco as osmotin. Physiol. Mol. Plant Pathol. 38, 137–146. doi: 10.1016/S0885-5765(05)80131-6
Stintzi, A., Heitz, T., Prasad, V., Wiedemann-Merdinoglu, S., Kauffmann, S., Geoffroy, P.,et al. (1993). Plant ‘pathogenesis-related’ proteins and their role in defense against pathogens. Biochimie 75, 687–706. doi: 10.1016/0300-9084(93)90100-7
Subramanyam, K., Arun, M., Mariashibu, T. S., Theboral, J., Rajesh, M., Singh, N. K.,et al. (2012). Overexpression of tobacco osmotin (Tbosm) in soybean conferred resistance to salinity stress and fungal infections. Planta 236, 1909–1925. doi: 10.1007/s00425-012-1733-8
PubMed Abstract | Full Text | CrossRef Full Text | Google Scholar
Subramanyam, K., Sailaja, K. V., Subramanyam, K., Rao, D. M., and Lakshmidevi, K. (2011). Ectopic expression of an osmotin gene leads to enhanced salt tolerance in transgenic chilli pepper (Capsicum annum L.). Plant Cell Tissue Organ. Cult. 105, 181–192. doi: 10.1007/s11240-010-9850-1
Tachi, H., Fukuda-Yamada, K., Kojima, T., Shiraiwa, M., and Takahara, H. (2009). Molecular characterization of a novel soybean gene encoding a neutral PR-5 protein induced by high-salt stress. Plant Physiol. Biochem. 47, 73–79. doi: 10.1016/j.plaphy.2008.09.012
PubMed Abstract | Full Text | CrossRef Full Text | Google Scholar
Tada, Y., and Kashimura, T. (2009). Proteomic analysis of salt-responsive proteins in the mangrove plant, Bruguiera gymnorhiza. Plant Cell Physiol. 50, 439–446. doi: 10.1093/pcp/pcp002
PubMed Abstract | Full Text | CrossRef Full Text | Google Scholar
Taj, G., Anil, K., Bansal, K. C., and Garg, G. K. (2004). Introgression of osmotin gene for creation of resistance against Alternaria blight by perturbation of cell cycle machinery. Indian J. Biotechnol. 3, 291–298.
Takeda, S., Sato, F., Ida, K., and Yamada, Y. (1991). Nucleotide sequence of a cDNA for osmotin-like protein from cultured tobacco Cells. Plant Physiol. 97, 844–846. doi: 10.1104/pp.97.2.844
Takemoto, D., Furuse, K., Doke, N., and Kawakita, K. (1997). Identification of chitinase and osmotin-like protein as actin-binding proteins in suspension-cultured potato cells. Plant Cell Physiol. 38, 441–448. doi: 10.1093/oxfordjournals.pcp.a029187
Tanaka, N., Matsuoka, M., Kitano, H., Asano, T., Kaku, H., and Komatsu, S. (2006). gid1, a gibberellin-insensitive dwarf mutant, shows altered regulation of probenazole-inducible protein (PBZ1) in response to cold stress and pathogen attack. Plant Cell Environ. 29, 619–631. doi: 10.1111/j.1365-3040.2005.01441.x
PubMed Abstract | Full Text | CrossRef Full Text | Google Scholar
Tang, Y. T., Hu, T., Arterburn, M., Boyle, B., Bright, J. M., Emtage, P. C.,et al. (2005). PAQR proteins: a novel membrane receptor family defined by an ancient 7-transmembrane pass motif. J. Mol. Evol. 61, 372–380. doi: 10.1007/s00239-004-0375-2
PubMed Abstract | Full Text | CrossRef Full Text | Google Scholar
Thomas, J. C., and Bohnert, H. J. (1993). Salt stress perception and plant growth regulators in the halophyte Mesembryanthemum crystallinum. Plant Physiol. 103, 1299–1304.
Trivedi, V. R., Chorawala, M. R., and Shah, G. B. (2012a). Antiatherosclerotic activity of osmotin, an adiponectin agonist in atherogenic diet induced hypertriglyceridemia and hypercholesterolemia in wistar rats. Adv. Res. Pharmaceuticals Biol. 2, 196–207.
Trivedi, V. R., Chorawala, M. R., and Shah, G. B. (2012b). Osmotin: a new adiponectin agonist, in type-II diabetes and obesity. Int. J. Pharm. Sci. Rev. Res. 16, 70–74.
Turer, A. T., and Scherer, P. E. (2012). Adiponectin: mechanistic insights and clinical implications. Diabetologia 55, 2319–2326. doi: 10.1007/s00125-012-2598-x
PubMed Abstract | Full Text | CrossRef Full Text | Google Scholar
Tzou, Y. M., Huang, T. S., Huggins, K. W., Chin, B. A., Simonne, A. H., and Singh, N. K. (2011). Expression of truncated tobacco osmotin in Escherichia coli: purification and antifungal activity. Biotechnol. Lett. 33, 539–543. doi: 10.1007/s10529-010-0453-z
PubMed Abstract | Full Text | CrossRef Full Text | Google Scholar
Van Loon, L. C. (1997). Induced resistance in plants and the role of pathogenesis-related proteins. Eur. J. Plant Pathol. 103, 753–765. doi: 10.1023/A:1008638109140
Van Loon, L. C., and Kammen, A. V. (1970). Polyacrylamide disc electrophoresis of the soluble leaf proteins from Nicotiana tabacum var. “Samsun” and “Samsun NN” II. Changes in protein constitution after infection with tobacco mosaic virus. Virology 40, 199–211. doi: 10.1016/0042-6822(70)90395-8
Van Loon, L. C., and Van Strien, E. A. (1999). The families of pathogenesis related proteins, their activities, and comparative analysis of PR-1 type proteins. Physiol. Mol. Plant. Pathol. 55, 85–97. doi: 10.1006/pmpp.1999.0213
Vander, W. H., and Loeve, K. (1972). Isolation and characterization of thaumatin I and II, the sweet-tasting proteins from Thaumatococcus daniellii Benth. Eur. J. Biochem. 31, 221–225. doi: 10.1111/j.1432-1033.1972.tb02522.x
PubMed Abstract | Full Text | CrossRef Full Text | Google Scholar
Vasavirama, K., and Kirti, P. B. (2012). Increased resistance to late leaf spot disease in transgenic peanut using a combination of PR genes. Funct. Integr. Genomics 12, 625–634. doi: 10.1007/s10142-012-0298-8
PubMed Abstract | Full Text | CrossRef Full Text | Google Scholar
Velazhahan, R., Datta, S. K., and Muthukrishnan, S. (1999). “The PR-5 family: thaumatin-like proteins in plants,” in Pathogenesis-Related Proteins in Plants, eds S. K. Datta and S. Muthukrishnan (Boca Raton, FL: CRC press), 107–129.
Veronese, P., Ruiz, M. T., Coca, M. A., Hernandez-Lopez, A., Lee, H., Ibeas, J. I.,et al. (2003). In defense against pathogens. Both plant sentinels and foot soldiers need to know the enemy. Plant Physiol. 131, 1580–1590. doi: 10.1104/pp.102.013417
PubMed Abstract | Full Text | CrossRef Full Text | Google Scholar
Vigers, A. J., Roberts, W. K., and Selitrennikoff, C. P. (1991). A new family of plant antifungal proteins. Mol. Plant Microbe Interact. 4, 315–323. doi: 10.1094/MPMI-4-315
Vigers, A. J., Weidmann, S., Roberts, W. K., Legrand, M., Selitrennikoff, C. P., and Fritig, B. (1992). Thaumatin-like pathogenesis related proteins are antifungal. Plant Sci. 83, 155–161. doi: 10.1016/0168-9452(92)90074-V
Viktorova, J., Krasny, L., Kamlar, M., Novakova, M., Mackova, M., and Macek, T. (2012). Osmotin, a pathogenesis-related protein. Curr. Protein Pept. Sci. 13, 672–681. doi: 10.2174/138920312804142129
Vinocur, B., and Altman, A. (2005). Recent advances in engineering plant tolerance to abiotic stress: achievements and limitations. Curr. Opin. Biotechnol. 16, 123–132. doi: 10.1016/j.copbio.2005.02.001
PubMed Abstract | Full Text | CrossRef Full Text | Google Scholar
Volger, H. G., and Heber, U. (1975). Cryoprotective leaf proteins. Biochim. Biophys. Acta 412, 335–349. doi: 10.1016/0005-2795(75)90048-3
Wang, W., Vinocur, B., and Altman, A. (2003). Plant responses to drought, salinity and extreme temperatures: towards genetic engineering for stress tolerance. Planta 218, 1–14. doi: 10.1007/s00425-003-1105-5
PubMed Abstract | Full Text | CrossRef Full Text | Google Scholar
Whan, J. A., Dann, E. K., Smith, L. J., and Aitken, E. A. B. (2009). Acibenzolar-S-methyl-induced alteration of defence gene expression and enzyme activity in cotton infected with Fusarium oxysporum f. sp. Vasinfectum. Physiol. Mol. Plant Pathol. 73, 175–182. doi: 10.1016/j.pmpp.2009.06.003
Wilkinson, J. R., Spradling, K. D., Yoder, D. W., Pirtle, I. L., and Pirtle, R. M. (2005). Molecular cloning and analysis of a cotton gene cluster of two genes and pseudogenes for the PR5 protein osmotin. Physiol. Mol. Plant Pathol. 67, 68–82. doi: 10.1016/j.pmpp.2005.09.006
Wolf, A. M., Wolf, D., Rumpold, H., Enrich, B., and Tilg, H. (2004). Adiponectin induces the anti-inflammatory cytokines IL-10 and IL-1RA in human leukocytes. Biochem. Biophys. Res. Commun. 323, 630–635. doi: 10.1016/j.bbrc.2004.08.145
PubMed Abstract | Full Text | CrossRef Full Text | Google Scholar
Woloshuk, C. P., Meulenhoff, S. J., Sela-Burlage, M., Van den Eltzen, P. J. M., and Cornelissen, B. J. (1991). Pathogen-induced proteins with inhibitory activity toward Phytophthora infestans. Plant Cell 3, 619–628. doi: 10.1105/tpc.3.6.619
PubMed Abstract | Full Text | CrossRef Full Text | Google Scholar
Xu, A., Wang, Y., Keshaw, H., Xu, L. Y., Lam, K. S., and Cooper, G. J. (2003). The fat-derived hormone adiponectin alleviates alcoholic and nonalcoholic fatty liver diseases in mice. J. Clin. Invest. 112, 91–100. doi: 10.1172/JCI17797
PubMed Abstract | Full Text | CrossRef Full Text | Google Scholar
Xu, P., Narasimhan, M. L., Samson, T., Coca, M. A., Huh, G. H., Zhou, J.,et al. (1998). A nitrilase-like protein interacts with GCC box DNA-binding proteins involved in ethylene and defense responses. Plant Physiol. 118, 867–874. doi: 10.1104/pp.118.3.867
PubMed Abstract | Full Text | CrossRef Full Text | Google Scholar
Xu, Y., Chang, P. F. L., Liu, D., Narasimhan, M. L., Raghothama, K. G., Hasegawa, P. M.,et al. (1994). Plant defense genes are synergistically induced by ethylene and methyl jasmonate. Plant Cell 6, 1077–1085. doi: 10.1105/tpc.6.8.1077
Yamaguchi-Shinozaki, K., and Shinozaki, K. (2006). Transcriptional regulatory networks in cellular responses and tolerance to dehydration and cold stresses. Annu. Rev. Plant Biol. 57, 781–803. doi: 10.1146/annurev.arplant.57.032905.105444
PubMed Abstract | Full Text | CrossRef Full Text | Google Scholar
Yamauchi, T., Hara, K., Kubota, N., Terauchi, Y., Tobe, K., Froguel, P.,et al. (2003). Dual roles of adiponectin/Acrp30 in vivo as an anti-diabetic and anti-atherogenic adipokine. Curr. Drug Targets Immune Endocr. Metabol. Disord. 3, 243–253. doi: 10.2174/1568008033340090
PubMed Abstract | Full Text | CrossRef Full Text | Google Scholar
Yamauchi, T., and Kadowaki, T. (2008). Physiological and pathophysiological roles of adiponectin and adiponectin receptors in the integrated regulation of metabolic and cardiovascular diseases. Int. J. Obes. 32, S13–S18. doi: 10.1038/ijo.2008.233
PubMed Abstract | Full Text | CrossRef Full Text | Google Scholar
Yamauchi, T., Nio, Y., Maki, T., Kobayashi, M., Takazawa, T., Iwabu, M.,et al. (2007). Targeted disruption of AdipoR1 and AdipoR2 causes abrogation of adiponectin binding and metabolic actions. Nat. Med. 13, 332–339. doi: 10.1038/nm1557
PubMed Abstract | Full Text | CrossRef Full Text | Google Scholar
Yang, W. S., Lee, W. J., Funahashi, T., Tanaka, S., Matsuzawa, Y., Chao, C. L.,et al. (2001). Weight reduction increases plasma levels of an adipose-derived anti-inflammatory protein, adiponectin. J. Clin. Endocrinol. Metab. 86, 3815–3819. doi: 10.1210/jc.86.8.3815
PubMed Abstract | Full Text | CrossRef Full Text | Google Scholar
Yatagai, T., Nagasaka, S., Taniguchi, A., Fukushima, M., Nakamura, T., Kuroe, A.,et al. (2003). Hypoadiponectinemia is associated with visceral fat accumulation and insulin resistance in Japanese men with type 2 diabetes mellitus. Metabolism 52, 1274–1278. doi: 10.1016/S0026-0495(03)00195-1
PubMed Abstract | Full Text | CrossRef Full Text | Google Scholar
Yen, H. E., Edward, G. E., and Grimes, H. D. (1994). Characterization of salt-responsive 24-Kilodalton glycoprotein in Mesembryanthemum crystallinum. Plant Physiol. 105, 1179–1187. doi: 10.1104/pp.105.4.1179
PubMed Abstract | Full Text | CrossRef Full Text | Google Scholar
Yoda-Murakami, M., Taniguchi, M., Takahashi, K., Kawamata, S., Saito, K., Choi-Miura, N. H.,et al. (2001). Change in expression of GBP28/adiponectin in carbon tetrachloride-administrated mouse liver. Biochem. Biophys. Res. Commun. 285, 372–377. doi: 10.1006/bbrc.2001.5134
Yun, D. J., Bressan, R. A., and Hasegawa, P. M. (1997a). “Plant antifungal proteins,” in Plant Breeding Reviews, ed. J. Janick (New York: John Wiley & Sons, Inc.), 39–88.
Yun, D. J., Zhao, Y., Pardo, J. M., Narasimhan, M. L., Damsz, B., Lee, H.,et al. (1997b). Stress proteins on the yeast cell surface determine resistance to osmotin, a plant antifungal protein. Proc. Natl. Acad. Sci. U.S.A. 94, 7082–7087. doi: 10.1073/pnas.94.13.7082
PubMed Abstract | Full Text | CrossRef Full Text | Google Scholar
Yun, D. J., Ibeas. J. I., Lee, H., Coca, M. A., Narsimhan, M. L., Uesono, Y.,et al. (1998). Osmotin, a plant antifungal protein, subverts signal transduction to enhance fungal cell susceptibility. Mol. Cell 1, 807–817. doi: 10.1016/S1097-2765(00)80080-5
PubMed Abstract | Full Text | CrossRef Full Text | Google Scholar
Zemanek, E., and Wasserman, B. P. (1995). Issues and advances on the use of transgenic organisms for the production of thaumatin, the intensely sweet protein from Thaumatococcus daniellii. Crit. Rev. Food Sci. Nutr. 35, 455–466. doi: 10.1080/10408399509527709
PubMed Abstract | Full Text | CrossRef Full Text | Google Scholar
Zhang, H., Huang, Z., Chen, B. X. Q., Tian, X., Zhang, X., Zhang, H.,et al. (2004). The ethylene-, jasmonate-, abscisic acid- and NaCl-responsive tomato transcription factor JERF1 modulates expression of GCC box-containing genes and salt tolerance in tobacco. Planta 220, 262–270. doi: 10.1007/s00425-004-1347-x
PubMed Abstract | Full Text | CrossRef Full Text | Google Scholar
Zhang, L., Xi, D., Li, S., Gao, Z., Zhao, S., Shi, J.,et al. (2011). A cotton group C MAP kinase gene, GhMPK2, positively regulates salt and drought tolerance in tobacco. Plant Mol. Biol. 77, 17–31. doi: 10.1007/s11103-011-9788-7
PubMed Abstract | Full Text | CrossRef Full Text | Google Scholar
Zhang, Y., and Shih, D. S. (2007). Isolation of an osmotin-like protein gene from strawberry and analysis of the response of this gene to abiotic stresses. J. Plant Physiol. 164, 68–77. doi: 10.1016/j.jplph.2006.02.002
PubMed Abstract | Full Text | CrossRef Full Text | Google Scholar
Zhao, J., Buchwaldt, L., Rimmer, S. R., Sharpe, A., Mcgregor, L., Bekkaoui, D.,et al. (2009). Patterns of differential gene expression in Brassica napus cultivars infected with Sclerotinia sclerotiorum. Mol. Plant Pathol. 10, 635–649. doi: 10.1111/J.1364-3703.2009.00558.X
PubMed Abstract | Full Text | CrossRef Full Text | Google Scholar
Zhu, B., Chen, T. H. H., and Li, P. H. (1993). Expression of an ABA-responsive osmotin-like gene during the induction of freezing tolerance in Solanum commersonii. Plant Mol. Biol. 21, 729–735. doi: 10.1007/BF00014558
Zhu, B., Chen, T. H. H., and Li, P. H. (1995a). Activation of two osmotin-like protein genes by abiotic stimuli and fungal pathogen in transgenic potato plants. Plant Physiol. 108, 929–937. doi: 10.1104/pp.108.3.929
PubMed Abstract | Full Text | CrossRef Full Text | Google Scholar
Zhu, B., Chen, T. H. H., and Li, P. H. (1995b). Expression of three osmotin-like protein genes in response to osmotic stress and fungal infection in potato. Plant Mol. Biol. 28, 17–26. doi: 10.1007/BF00042034
Zhu, B., Chen, T. H. H., and Li, P. H. (1996). Analysis of late-blight disease resistance and freezing tolerance in transgenic potato plants expressing sense and antisense genes for an osmotin-like protein. Planta 198, 70–77. doi: 10.1007/BF00197588
PubMed Abstract | Full Text | CrossRef Full Text | Google Scholar
Keywords: abiotic stress, adiponectin, biotic stress, OLPs, osmotin, protein–protein interactions
Citation: Anil Kumar S, Hima Kumari P, Shravan Kumar G, Mohanalatha C and Kavi Kishor PB (2015) Osmotin: a plant sentinel and a possible agonist of mammalian adiponectin. Front. Plant Sci. 6:163. doi: 10.3389/fpls.2015.00163
Received: 10 June 2014; Accepted: 01 March 2015;
Published online: 16 March 2015
Edited by:
Sagadevan G. Mundree, Queensland University of Technology, AustraliaReviewed by:
Paulo Arruda, Universidade Estadual de Campinas, BrazilCopyright © 2015 Anil Kumar, Hima Kumari, Shravan Kumar, Mohanalatha and Kavi Kishor. This is an open-access article distributed under the terms of the Creative Commons Attribution License (CC BY). The use, distribution or reproduction in other forums is permitted, provided the original author(s) or licensor are credited and that the original publication in this journal is cited, in accordance with accepted academic practice. No use, distribution or reproduction is permitted which does not comply with these terms.
*Correspondence: P. B. Kavi Kishor, Department of Genetics, Osmania University, Hyderabad 500007, IndiacGJrYXZpQHlhaG9vLmNvbQ==
Disclaimer: All claims expressed in this article are solely those of the authors and do not necessarily represent those of their affiliated organizations, or those of the publisher, the editors and the reviewers. Any product that may be evaluated in this article or claim that may be made by its manufacturer is not guaranteed or endorsed by the publisher.
Research integrity at Frontiers
Learn more about the work of our research integrity team to safeguard the quality of each article we publish.