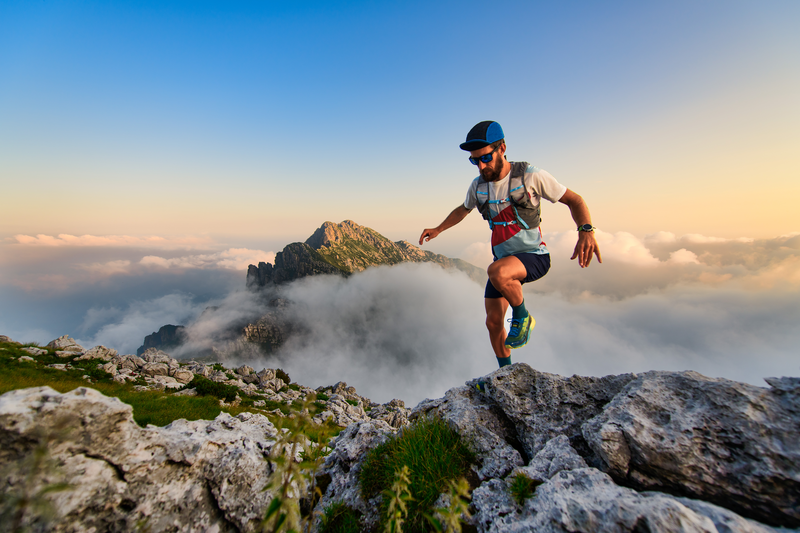
95% of researchers rate our articles as excellent or good
Learn more about the work of our research integrity team to safeguard the quality of each article we publish.
Find out more
MINI REVIEW article
Front. Plant Sci. , 18 March 2014
Sec. Plant Pathogen Interactions
Volume 5 - 2014 | https://doi.org/10.3389/fpls.2014.00101
This article is part of the Research Topic Plant cell wall in pathogenesis, parasitism and symbiosis View all 15 articles
Cell wall pectin forms a matrix around the cellulose–xyloglucan network that is composed of rhamnogalacturonan I, rhamnogalacturonan II, and homogalacturonan (HG), a major pectic polymer consisting of α-1,4-linked galacturonic acids. HG is secreted in a highly methyl-esterified form and selectively de-methyl-esterified by pectin methylesterases (PMEs) during cell growth and pathogen attack. The mechanical damage that often precedes the penetration of the leaf by a pathogen promotes the activation of PME, which in turn leads to the emission of methanol (MeOH), an abundant volatile organic compound, which is quickly perceived by the intact leaves of the damaged plant, and the neighboring plants. The exposure to MeOH may result in a “priming” effect on intact leaves, setting the stage for the within-plant, and neighboring plant immunity. The emission of MeOH by a wounded plant enhances the resistance of the non-wounded, neighboring “receiver” plants to bacterial pathogens and promotes cell-to-cell communication that facilitates the spread of viruses in neighboring plants.
Plant cells are covered with a dense extracellular matrix that prevents direct contact between adjacent cells and pathogens. Damage to the plant epidermis caused by abiotic (wind, hail, and rain) and biotic (insects) factors may allow the penetration of pathogens (bacteria, fungi, oomycetes, and nematodes) into the intercellular space of the leaf and virus particles into the cell. Thus, plant wounding is one of the conditions for pathogen entry. However, mechanical damage to the leaf promotes the emission of volatile organic compounds (VOCs), including the green leaf volatiles (GLVs), and methanol (MeOH), which are quickly perceived by the intact leaves of the damaged plant and the intact neighboring plants (Dorokhov et al., 2014). The transport of VOCs is much faster compared to the transport of infectious viral entities and bacterial effectors through the phloem. Thus, exposure to VOCs may result in a “priming” effect on intact leaves, setting the stage for subsequent plant immunity. GLVs are associated with the smell of a freshly mown lawn and are derived from C18 fatty acids released from damaged membranes. MeOH, quantitatively the most important plant volatile after CO2, is a product of the demethylation of pectin by the pectin methylesterases (PMEs) during cell wall (CW) formation and modification (Pelloux et al., 2007).
Cell wall pectin forms a matrix around the cellulose–xyloglucan network that is composed of three main components called rhamnogalacturonan I (RGI), rhamnogalacturonan II (RGA II), and homogalacturonan (HG), a major pectic polymer consisting of α-1,4-linked galacturonic acids (Peaucelle et al., 2012). HG is secreted in a highly methyl-esterified form and selectively de-methyl-esterified by PMEs, resulting in MeOH formation. The PME genes encode a pro-PME precursor with an N-terminal extension of variable length that is essential for protein targeting to the endoplasmic reticulum (Dorokhov et al., 1999). PME maturation requires removal of the PME leader including the transmembrane domain and spacer sequence (Dorokhov et al., 2006a). It was hypothesized that the spacer sequence plays a role in subcellular targeting and acts as an intramolecular chaperone for folding of the mature enzyme or as an autoinhibitor during transport through the endomembrane system (Pelloux et al., 2007). PME participates in CW modulation during general plant growth as it is involved in cell expansion and CW modification (Pelletier et al., 2010). The synthesis of PME is one of the aspects of plant growth that leads to the demethylesterification of the elastic “soft” pectins that accompanies MeOH generation (Komarova et al., 2014) as part of the natural division and maturation of the plant cell. After demethylesterification, pectate can form Ca2+-pectate cross-linked complexes of rigid “hard” pectin, referred to as “egg boxes” (Peaucelle et al., 2012).
The important role of PME in the resistance of plants to fungi and bacteria has been demonstrated (Pelloux et al., 2007). A higher degree of pectin methyl esterification in certain plants induces resistance to pathogenic fungi (Lionetti et al., 2012). CW pectin methyl esterification may have an impact on plant resistance because highly methyl-esterified pectin can be less susceptible to hydrolysis by pectic enzymes such as fungal endopolygalacturonases. This view is supported by experiments performed with plants that were stably transformed with the PME inhibitor (PMEI) gene. The PMEI transgenic Arabidopsis (Lionetti et al., 2007) and durum wheat (Volpi et al., 2011) plants exhibited high levels of resistance to fungal and bacterial pathogens. Moreover, PME-mediated pectin methyl de-esterification may influence the polygalacturonase-mediated release of pectin-derived compounds, which in turn elicits a defense response (Pelloux et al., 2007; Lionetti et al., 2012).
The role of PME in viral infection is more complicated. PME interacts with the movement protein (MP) of the Tobacco mosaic virus (TMV; Dorokhov et al., 1999; Chen et al., 2000), suggesting that PME may be involved in the cell-to-cell movement of plant viruses (Chen and Citovsky, 2003). Interestingly, PMEI also interacts with PME to negatively affect viral infection (Lionetti et al., 2014), most likely by interfering with PME and TMV MP binding. The complex role of PME in viral infection is also underscored by the effects of PME on nuclear protein transport (Komarova et al., 2011) and gene silencing mediated by the activation of siRNA and miRNA production (Dorokhov et al., 2006b).
The PME-mediated conversion of HG methoxyl groups into carboxyl groups results in MeOH release. In humans, MeOH is considered to be a poison because alcohol dehydrogenase metabolizes MeOH into toxic formaldehyde. However, recent data have indicated that MeOH is actually a naturally occurring compound in normal, healthy human individuals. MeOH is not toxic to plant cells and has long been assumed to be a metabolic waste product. Recently, it has been shown that MeOH may regulate plant growth (Komarova et al., 2014) and serve an alarm function (Dorokhov et al., 2012a). The effects of PME-generated MeOH emitted from plants (“emitters”) on the defensive reactions of other plants (“receivers”) were studied (Dorokhov et al., 2012a). The results of this study led to the conclusion that MeOH is a signaling molecule that is involved in within-plant and plant-to-plant communication (Dorokhov et al., 2012a).
Mechanical damage to plants drastically increases MeOH and GLVs emission. GLVs that are rapidly released from wounded leaves may in turn stimulate PME-generated MeOH production (Dorokhov et al., 2012a). Herbivore attacks also increase MeOH emission levels: Manduca sexta caterpillars enhance wound-induced MeOH emission in Nicotiana attenuate (von Dahl et al., 2006). The over-expression of PME, derived from Arabidopsis thaliana and Aspergillus niger, in transgenic tobacco plants enhances resistance to polyphagous insect pests (Dixit et al., 2013). Transgenic plants with a silenced PME gene exhibited a 50% reduction in PME activity in their leaves and a 70% reduction in herbivore-induced MeOH emissions compared to wild type plants. This result demonstrates that herbivore-induced MeOH emissions originate from pectin demethylation by PME (Körner et al., 2009). The emission of MeOH is very fast and can be detected immediately following mechanical damage. Thus, the MeOH emitted from wounded leaves is produced by two forms of PME: pre-existing PME deposited in the CW before wounding, which allows rapid MeOH release (Körner et al., 2009), and PME that is synthesized de novo after wounding (Dorokhov et al., 2012a), which likely generates MeOH for an extended period.
Unlike longer-chain alcohols, the MeOH emitted by a wounded plant attracts insects and bark beetles. Moreover, mice prefer the odor of MeOH to the odors of other plant volatiles under laboratory conditions, and MeOH exposure alters the accumulation of mRNA in the mouse brain (Dorokhov et al., 2012b). This finding led to the conclusion that the MeOH emitted by wounded plants may have a role in plant-animal signaling.
Investigations demonstrated (Dorokhov et al., 2012a) that increased MeOH emissions from PME-transgenic or mechanically wounded non-transgenic plants retarded the growth of the bacterial pathogen Ralstonia solanacearum in neighboring “receiver” plants. The suppression of R. solanacearum growth observed in the “receiver” plants could be caused by gaseous MeOH or/and by GLVs. Indeed, cis-3-hexen-1-ol evaporated in a desiccator also resulted in decreased bacterial growth in the target plants. However, GLVs rapidly released from wounded leaves stimulated PME-generated MeOH production (Dorokhov et al., 2012a), suggesting that their influence on bacterial growth may be indirect. MeOH-stimulated antibacterial resistance was preceded by the upregulation of genes that control stress response and cell-to-cell communication in the “receiver”. Antibacterial resistance accompanied by MeOH-induced genes (MIGs) upregulation was most likely related to the transcriptional induction of the type II proteinase inhibitor (PI-II) gene. PI-IIs are powerful inhibitors of serine endopeptidases in animals and microorganisms (Turra and Lorito, 2011). The PI-II gene is not expressed in the leaves of healthy plants, but it is induced in leaves that have been subjected to different types of stress, including wounding and bacterial infection. PME-transgenic tobacco with high levels of PI-II expression exhibited increased resistance to R. solanacearum (Dorokhov et al., 2012a). This finding supports the role of PI-II in the suppression of bacterial proteases.
Experiments with gaseous MeOH provided examples of priming in intact plants (Figure 1), which led to conditions conducive for viral infection (Dorokhov et al., 2012a). This effect could be explained by the enhancement of cell-to-cell communication by the MIGs, such as β-1,3-glucanase (BG; Zavaliev et al., 2011) and non-cell-autonomous pathway protein (NCAPP; Lee et al., 2003).
FIGURE 1. The model of the effects of methanol emitted by the damaged plant. Mechanical damage to the leaves of the plant (1), leads to an increase in the expression level of PME (2), and induction of the release of gaseous MeOH (3). Emitted MeOH causes priming of adjacent leaves and neighboring plants, including activation of the MIGs (4), the emergence of bacterial immunity (5), the opening of plasmodesmata, and increased sensitivity to the virus penetration and intercellular distribution of viral pathogens (6).
A model (Figure 1) proposing that MeOH-triggered PD dilation should enhance viral spread within the plant was confirmed in experiments in which BG and NCAPP activated cell-to-cell communication and TMV RNA accumulation. Moreover, gaseous MeOH or the vapors from wounded plants increased TMV reproduction in the “receivers” (Dorokhov et al., 2012a).
Thus, MeOH has a contradictory effect on the sensitivity of the leaves of the “receiver” plant to bacteria and viruses. The mechanisms that underlie this phenomenon are not clear; however, we can consider two factors that may explain this inconsistency in the MeOH-induced effects. First, there is a fundamental difference between bacteria and viruses with respect to their modes of intercellular transport. Bacterial pathogens do not cross the plant CW boundaries because they inhabit the intercellular spaces. In contrast, viral pathogens require cell-to-cell movement for local and systemic spread. Second, the most abundant MIGs can be divided into two groups according to their ability to participate in either bacterial or viral pathogenesis. The first, including PI-II and PME inhibitor, are involved in immunity against non-viral pathogens. The second group of genes, including NCAPP and MIG-21 (Dorokhov et al., 2012a), is involved in the PD-mediated intercellular transport and reproduction of viruses. The most abundant MIG, the BG gene, is involved in antibacterial immunity; however, the BG protein also accelerates PD-mediated intercellular transport.
Based on the available data, we can conclude that wounding-stimulated MeOH that is released into the air by damaged plants or plants compromised by herbivorous insects serves as an alarm to help neighboring plants or adjacent leaves prepare for a defense. The MeOH provides protection against herbivorous insects and plant pathogens such as bacteria. However, considering the role of MeOH in the relationship between viruses and plants, we do not find a negative, or even a neutral, influence of MeOH on viruses. On the contrary, the findings described (Dorokhov et al., 2012a) indicate that MeOH sensitizes the plant to allow the entry and spread of a virus through the plant and between plants by insect vectors. Therefore, MeOH promotes viral propagation. The positive impact of MeOH on viral infection may be explained by several factors. First, plant viruses differ from other types of pathogens as they inhabit the symplast. Furthermore, the survival of a virus depends on its ability to move from cell-to-cell exploiting PD to accumulate to sufficient levels and in enough tissues to guarantee survival despite using a very limited amount of genetic material. Thus, a virus, with its small but highly variable genome, spends its entire life in the cell symplast, while other pathogens occupy the apoplast. Second, the symplast is not only the space in which viruses reproduce, but it is also the site of RNA interference mechanisms that serve to eliminate foreign RNA. The specific degradation of RNA by RNA interference allows the host plant to effectively control viruses and other pathogens. It is known that the intracellular and intercellular transport of silencing factors is necessary for effective RNA interference. Therefore, a MeOH-mediated increase in viral replication may be regarded as compensation for the acquisition of antimicrobial resistance.
The authors declare that the research was conducted in the absence of any commercial or financial relationships that could be construed as a potential conflict of interest.
This work was supported by the Russian Science Foundation, the Russian Foundation for Basic Research (grants Nos. 11-04-01152, 12-04-33016, 14-04-00109), and stipend of the President of the Russian Federation for young scientists.
Chen, M. H., and Citovsky, V. (2003). Systemic movement of a tobamovirus requires host cell pectin methylesterase. Plant J. 35, 386–392. doi: 10.1046/j.1365-313X.2003.01818.x
Chen, M. H., Sheng, J., Hind, G., Handa, A. K., and Citovsky, V. (2000). Interaction between the tobacco mosaic virus movement protein and host cell pectin methylesterases is required for viral cell-to-cell movement. EMBO J. 19, 913–920. doi: 10.1093/emboj/19.5.913
Dixit, S., Upadhyay, S. K., Singh, H., Sidhu, O. P., Verma, P. C., and K, C. (2013). Enhanced methanol production in plants provides broad spectrum insect resistance. PLoS ONE 8:e79664. doi: 10.1371/journal.pone.0079664
Dorokhov, Y. L., Komarova, T. V., Petrunia, I. V., Frolova, O. Y., Pozdyshev, D. V., and Gleba, Y. Y. (2012a). Airborne signals from a wounded leaf facilitate viral spreading and induce antibacterial resistance in neighboring plants. PLoS Pathog. 8:e1002640. doi: 10.1371/journal.ppat.1002640
Dorokhov, Y. L., Komarova, T. V., Petrunia, I. V., Kosorukov, V. S., Zinovkin, R. A., Shindyapina, A. V., et al. (2012b). Methanol may function as a cross-kingdom signal. PLoS ONE 7:e36122. doi: 10.1371/journal.pone.0036122
Dorokhov, Y. L., Komarova, T. V., and Sheshukova, E. V. (2014). “Volatile organic compounds and plant virus-host interaction,” in Plant Virus-Host Interaction, eds R. K. Gaur, T. Hohn, and P. Sharma (Amsterdam: Elsevier).
Dorokhov, Y. L., Makinen, K. M., Frolova, O. Y., Merits, A., Kalkkinen, N., Saarinen, J., et al. (1999). A novel function for a ubiquitous plant enzyme pectin methylesterase: the host-cell receptor for the tobacco mosaic virus movement protein. FEBS Lett. 461, 223–228. doi: 10.1016/S0014-5793(99)01447-7
Dorokhov, Y. L., Skurat, E. V., Frolova, O. Y., Gasanova, T. V., Ivanov, P. A., Ravin, N. V., et al. (2006a). Role of the leader sequences in tobacco pectin methylesterase secretion. FEBS Lett. 580, 3329–3334. doi: 10.1016/j.febslet.2006.04.090
Dorokhov, Y. L., Frolova, O. Y., Skurat, E. V., Ivanov, P. A., Gasanova, T. V., Sheveleva, A.S., et al. (2006b). A novel function for a ubiquitous plant enzyme pectin methylesterase: the enhancer of RNA silencing. FEBS Lett. 580, 3872–3878. doi: 10.1016/j.febslet.2006.06.013
Komarova, T. V., Citovsky, V., and Dorokhov, Y. L. (2011). “Pectin methylesterase enhances tomato bushy stunt virus P19 RNA silencing suppressor effects,” in RNAi Technology, eds R. K. Gaur, Y. Gafni, P. Sharma, and V. K. Gupta (Boca Raton, FL: CRC Press, Taylor & Francis Group), 125–134.
Komarova, T. V., Pozdyshev, D. V., Petrunia, I. V., Sheshukova, E. V., and Dorokhov, Y. L. (2014). Pectin methylesterase-generated methanol may be involved in tobacco leaf growth. Biochemistry (Mosc.) 79, 102–110. doi: 10.1134/S0006297914020035
Körner, E., von Dahl, C. C., Bonaventure, G., and Baldwin, I. T. (2009). Pectin methylesterase NaPME1 contributes to the emission of methanol during insect herbivory and to the elicitation of defence responses in Nicotiana attenuata. J. Exp. Bot. 60, 2631–2640. doi: 10.1093/jxb/erp106
Lee, J. Y., Yoo, B. C., Rojas, M. R., Gomez-Ospina, N., Staehelin, L. A., and Lucas, W. J. (2003). Selective trafficking of non-cell-autonomous proteins mediated by NtNCAPP1. Science 299, 392–326. doi: 10.1126/science.1077813
Lionetti, V., Cervone, F., and Bellincampi, D. (2012). Methyl esterification of pectin plays a role during plant-pathogen interactions and affects plant resistance to diseases. J. Plant Physiol. 169, 1623–1630. doi: 10.1016/j.jplph.2012.05.006
Lionetti, V., Raiola, A., Camardella, L., Giovane, A., Obel, N., Pauly, M., et al. (2007). Overexpression of pectin methylesterase inhibitors in Arabidopsis restricts fungal infection by Botrytis cinerea. Plant Physiol. 143, 1871–1880. doi: 10.1104/pp.106.090803
Lionetti, V., Raiola, A., Cervone, F., and Bellincampi, D. (2014). Transgenic expression of pectin methylesterase inhibitors limits tobamovirus spread in tobacco and Arabidopsis. Mol. Plant Pathol. 15, 265–274. doi: 10.1111/mpp.12090
Peaucelle, A., Braybrook, S., and Höfte, H. (2012). Cell wall mechanics and growth control in plants: the role of pectins revisited. Front. Plant Sci. 3:121. doi: 10.3389/fpls.2012.00121
Pelletier, S., Van Orden, J., Wolf, S., Vissenberg, K., Delacourt, J., Ndong, Y. A. et al. (2010). A role for pectin de-ethylesterification in a developmentally regulated growth acceleration in dark-grown Arabidopsis hypocotyls. New Phytol. 188, 726–739. doi: 10.1111/j.1469-8137.2010.03409.x
Pelloux, J., Rusterucci, C., and Mellerowicz, E. J. (2007). New insights into pectin methylesterase structure and function. Trends Plant Sci. 12, 267–277. doi: 10.1016/j.tplants.2007.04.001
Turra, D., and Lorito, M. (2011). Potato type I and II proteinase inhibitors: modulating plant physiology and host resistance. Curr. Protein Pept. Sci. 12, 374–385. doi: 10.2174/138920311796391151
Volpi, C., Janni, M., Lionetti, V., Bellincampi, D., Favaron, F., and D’Ovidio, R. (2011). The ectopic expression of a pectin methyl esterase inhibitor increases pectin methylesterification and limits fungal diseases in wheat. Mol. Plant Microbe Interact. 24, 1012–1019. doi: 10.1094/MPMI-01-11-0021
von Dahl, C. C., Hävecker, M., Schlögl, R., and Baldwin, I. T. (2006). Caterpillar-elicited methanol emission: a new signal in plant-herbivore interactions? Plant J. 46, 948–960. doi: 10.1111/j.1365-313X.2006.02760.x
Keywords: cell wall, methanol, pectin, pectin methylesterase, plant immunity, priming
Citation: Komarova TV, Sheshukova EV and Dorokhov YL (2014) Cell wall methanol as a signal in plant immunity. Front. Plant Sci. 5:101. doi: 10.3389/fpls.2014.00101
Received: 31 January 2014; Accepted: 02 March 2014;
Published online: 18 March 2014.
Edited by:
Vincenzo Lionetti, Sapienza Università di Roma, ItalyReviewed by:
Simone Ferrari, Sapienza Università di Roma, ItalyCopyright © 2014 Komarova, Sheshukova and Dorokhov. This is an open-access article distributed under the terms of the Creative Commons Attribution License (CC BY). The use, distribution or reproduction in other forums is permitted, provided the original author(s) or licensor are credited and that the original publication in this journal is cited, in accordance with accepted academic practice. No use, distribution or reproduction is permitted which does not comply with these terms.
*Correspondence: Yuri L. Dorokhov, A. N. Belozersky Institute of Physico-Chemical Biology, Moscow State University, Moscow 119991, Russia e-mail:ZG9yb2tob3ZAZ2VuZWJlZS5tc3UucnU=
Disclaimer: All claims expressed in this article are solely those of the authors and do not necessarily represent those of their affiliated organizations, or those of the publisher, the editors and the reviewers. Any product that may be evaluated in this article or claim that may be made by its manufacturer is not guaranteed or endorsed by the publisher.
Research integrity at Frontiers
Learn more about the work of our research integrity team to safeguard the quality of each article we publish.