- 1Tobacco Research Board (TRB), Kutsaga Research Station, Harare, Zimbabwe
- 2Department of Plant Production Sciences and Technologies, University of Zimbabwe, Harare, Zimbabwe
Hemp, which has a wide range of industrial applications, has been marginalized due to its association with marijuana. This stigma has hindered research into improving its resilience to various stressors, resulting in underutilization and neglect. As cultivation expands globally, particularly in hot, dry regions of Africa, understanding drought stress mechanisms in hemp is crucial. This study investigates the drought adaptation mechanisms of three CBD flower hemp genotypes: Cannabis indica (MP) from Switzerland, Cannabis sativa (AQ) from South Africa, and Cannabis sativa (ZB) from Zimbabwe. Conducted under well-watered (WW-75% field capacity [FC]), mild drought (MD-40% FC), and severe drought (SD-0% FC) conditions, the research examines morphophysiological adaptations and proline accumulation in these genotypes, assessed 55 days after transplanting. Results revealed genotype-specific responses to watering regimes. MP demonstrated controlled water use and inherent drought tolerance, maintaining high assimilation rates (A) and superior photosynthetic performance (ΦPSII) under drought conditions. ZB maintained proline levels during drought recovery, suggesting optimized resource allocation and alternative stress-responsive mechanisms, while exhibiting effective morning water use and high non-photochemical quenching (NPQ) for photoprotection. AQ showed conservative water use strategies beneficial in water-limited environments. These findings provide a foundation for breeding programs aimed at developing robust and resilient hemp varieties suited to specific environmental conditions.
1 Introduction
The economic importance of hemp cultivation is evident in its diverse applications in agriculture, industry, and healthcare. Hemp is utilized in various forms such as grain, fiber, and the highly sought-after cannabidiol (CBD) flower (Zheljazkov et al., 2023). Hemp grain is known for being a nutritional powerhouse, providing a rich source of protein and healthy fats. This makes hemp a valuable component in promoting food security and addressing dietary needs (Van Der Werf et al., 1996; Ehrensing, 1998; Small and Marcus, 2002; Salentijn et al., 2015). The robust fibers derived from hemp have versatile applications in the textile, paper, and construction industries, contributing to the development of sustainable and eco-friendly materials.
The CBD flower is associated with medicinal properties, leading to a rising demand in the wellness and pharmaceutical sectors. CBD, a non-psychoactive compound found in hemp, has been extensively studied for its potential therapeutic benefits, including anti-inflammatory, analgesic, and neuroprotective properties. Xiong et al. (2012) conducted research highlighting the neuroprotective potential of CBD, indicating its relevance in mitigating neurological disorders.
While hemp’s contributions are invaluable, there is an ongoing debate about its taxonomic classification within the Cannabis genus. The dispute centers around the monotypic perspective, which recognizes only C. sativa, and the polytypic viewpoint, which acknowledges both C. sativa and C. indica as distinct species (Small and Cronquist, 1976; Russo, 2019). Currently, the widely accepted taxonomy categorizes hemp under a single species, C. sativa L., with subspecies sativa, indica, and ruderalis, which offers some resolution to the taxonomic discourse (Warwick and Rightford, 2020). However, it’s important to note, as highlighted by McPartland (2018), that distinctions between C. sativa and C. indica go beyond taxonomy. Morphological differences, such as C. sativa’s taller, fibrous stalk compared to C. indica’s shorter, woody stalk, and phytochemical variations, such as C. sativa typically exhibiting a cannabinoid ratio with higher Δ9-tetrahydrocannabinol (THC) than CBD, while C. indica shows the opposite, are key factors. Additionally, differences in their original geographic range further differentiate the two species, with C. sativa predominantly found in Europe and C. indica in Asia.
The economic potential of hemp cultivation comes with environmental challenges, especially in drought-prone regions. This type of climate phenomena, marked by increased temperatures in the Pacific Ocean, results in extended dry periods and less rainfall, worsening water scarcity and drought stress in agricultural areas. Southern Africa, in particular, suffers severe consequences during drought events, impacting crop production, food security, and livestock (Baudoin et al., 2017; Sifundza et al., 2019; Mugiyo et al., 2023). Small-scale farmers, who lack sufficient water resources and irrigation systems, are especially vulnerable. Therefore, a better understanding of drought responses and adaptive strategies in hemp and other crops is vital.
Drought stress has a profound effect on plant growth and physiological processes. Researchers have extensively studied strategies that plants use to cope with water deficiency, such as stomatal regulation, osmotic adjustment, and activation of antioxidant defense mechanisms (Verslues et al., 2007). However, there is still much to learn about the complex interaction of these mechanisms, particularly in the case of hemp. Prolonged and severe drought stress can hinder growth, cause changes in plant morphology, and even lead to plant death. Understanding the nuanced responses and underlying mechanisms is crucial for developing strategies to enhance crop productivity and resilience under water-limited conditions (Ahmed et al., 2019).
Recent research has focused on the relationship between leaf chlorophyll fluorescence characteristics, membrane lipid peroxidation, and stress response in plants exposed to drought conditions (Kalaji et al., 2016). The study of photosynthesis, protective enzyme activity, and their interactions provides valuable insights into light energy conversion, utilization, and drought resistance (Zhu et al., 2020). Studies have also emphasized the inhibitory effects of drought on plant growth and photosynthesis and highlighted the importance of understanding these processes under water-limited conditions (Efeoğlu, 2009).
In regions like Zimbabwe, where outdoor hemp cultivation is allowed but irrigation systems are not widespread, vulnerability to drought stress is significant. Insufficient water resources greatly affect the growth and yield of hemp crops, which necessitates conducting comprehensive studies on the physiological and biochemical responses to drought stress. Proline, an amino acid, plays a crucial role in osmotic adjustment and stress mitigation in plants (Hayat et al., 2012a, b). Accumulation and recovery of proline under drought stress conditions act as a protective mechanism, helping to maintain cell turgor and overall cellular integrity (Raza et al., 2023).
This study aims to fill the current research gap by conducting experiments on potted plants. The study will be investigating different hemp genotypes under controlled water conditions. Our goal is to examine how drought stress affects various aspects of hemp, such as photosynthesis, chlorophyll fluorescence parameters, and proline accumulation. By doing this, we hope to gain a holistic understanding of how hemp responds to water-limited environments. Ultimately, our findings will not only contribute to the existing knowledge of hemp physiology but also help in the development of water-saving practices for hemp cultivation. This will promote sustainable and resilient hemp production, particularly in regions prone to drought.
2 Materials and methods
2.1 Plant materials
Three hemp genotypes, sourced from diverse geographical regions, exemplify distinct genetic lineages within the Cannabis species. Given advancements in our understanding of cannabis taxonomy, it’s crucial to provide detailed illustrations of their genetic diversity. Notably, beyond genetic makeup, these genotypes manifest pronounced differences in morphology; for instance, C. sativa exhibits a tall, fibrous stalk, while C. indica displays a shorter stature with a woody stalk, highlighting the multifaceted nature of genetic and phenotypic variation within the Cannabis genus (McPartland, 2018). The genotypes, including a Swiss genotype classified as Cannabis indica (genotype MP), a South African genotype as Cannabis sativa (genotype AQ), and a Zimbabwean genotype also as Cannabis sativa (genotype ZB), were selected to represent genetic diversity, considering their source locations, morphology, and environmental pressure adaptations. To protect intellectual property and prevent unauthorized propagation, the strain names were omitted and focused on describing characteristics. The germplasm used in this study were obtained as feminized seeds from individual suppliers, certified to have a THC content below 1% on a dry weight basis, in accordance with the regulations in Zimbabwe. The experiment was conducted in the 2023 season at the Kutsaga Research Station (-17.9190513, 31.1222), Harare, Zimbabwe, under a rain-out shelter. Sterilization was employed according to Gilbert et al. (2023) with modifications described below to ensure the seeds were devoid of fungal contamination. A surface sterilization method was employed, involving a 5% sodium hypochlorite solution followed by rinsing with distilled water. Sterilized seeds were then sown in Kutsaga float trays® with 242 cells, allowing them to germinate for 8-d. Afterward, well-established and uniform seedlings at day 21 after planting were transplanted into 20 L free-draining pots measuring 31.5 cm in diameter and 38 cm in height, from top to bottom. Each pot accommodated a single seedling and was filled with approximately 32 kg of a homogeneous sandy clay soil mixture, comprising 10% silt, 48% sand, and 42% clay. The soil used had a pH of 4.7 (extraction method with 0.01M CaCl2 solution) and an electrical conductivity (EC) of 13 µS cm-1, determined through soil tests conducted by Kutsaga’s ISO 17025 accredited Analytical Chemistry Laboratory in Harare, Zimbabwe. Throughout the growth period, plants were fertilized weekly with 50 mL of nutritive solution. The solution used was a mixture of COCOS A + B (House and Garden, Van De Zwaan, USA; total N 4%, K2O 9%, P2O5 3%; diluted 1:250) and N27% NITROGEN (House and Garden, Van De Zwaan, USA; total N 27%; diluted 1:100).
2.2 Experiment design and treatments
The experimental design followed a factorial treatment structure, employing a completely randomized design with levels of hemp genotypes (i.e., MP, AQ and ZB), and three water management levels i.e., Well-Watered (WW-75% field capacity [FC] which was adequate water supply), Moderate Drought (MD-40% [FC] which is suboptimal moisture level), and Severe Drought (SD-0% [FC] which is Severe Water Stress), and its treatment combination replicated three times. Following transplanting, all plants were consistently maintained in a well-watered state, receiving 75% FC, a value determined to represent the true field capacity considering the soil’s physical properties. At 40-d after transplanting (DAT), the initiation of water management treatments—WW-75% FC, MD-40% FC, and SD-0% FC—marked a pivotal point in the experimental timeline (Figure 1). The WW-75% FC treatment, functioning as the control, maintained soil water at 75% FC by supplying an average of 30.2 L of water during the subsequent drought stress period, ensuring optimal soil moisture for sustained plant growth. In the MD-40% FC treatment, the soil water level was deliberately reduced to 40% FC for a period of 15-d, creating conditions that mimic moderate water scarcity. Throughout the 15-d drought stress period, an average of 12.1 L of water was supplied, strategically imposing a controlled reduction in water availability to induce a measurable stress response in the hemp genotypes. The SD-0% FC treatment represented the most challenging water stress scenario, with no water supplied during the drought stress period, reducing the soil water level to 0% FC for a period of 15-d. This extreme condition aimed to simulate severe drought stress, thereby challenging the adaptability and resilience of the genotypes to extreme water scarcity. To ensure consistent water levels, all pots were irrigated once every 2-d at 7.30am until they reached the desired field capacity. Soil field capacity for the water managements was maintained by monitoring using a Field scout TDR300 soil moisture meter (Spectrum Technologies, UK), with compensation of the water lost by addition of an equivalent amount of water. At 55 DAT—peak drought stress, the MD-40% FC and SD-0% FC treatments were discontinued, and all plants were transitioned back to the well-watered state, receiving 75% FC until the final harvest at 80 DAT (Figure 1).
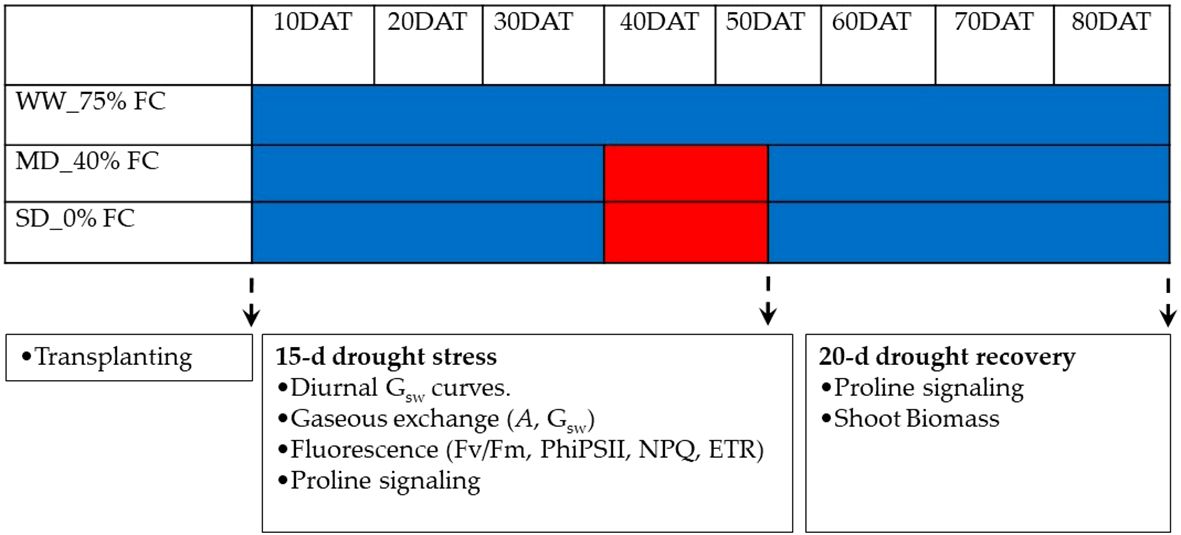
Figure 1. The study design included three treatments: well-watered (WW-75% FC), mild drought (MD-40% FC), and severe drought (SD-0% FC). Well-watered plants received regular irrigation, while mild drought plants had reduced irrigation (40% of field capacity) for 15-d. Severe drought plants received no irrigation for the same period. Visual indicators, such as solid blue bars and red bar inserts, represented the respective treatments. The treatments are depicted by solid blue and red colours, respectively.
2.3 Measurement parameters and methods
2.3.1 Gaseous exchange parameters
Stomatal conductance (gsw) and net assimilation rate (A) readings were obtained from the youngest, fully expanded fan leaves, using the LI-6800 portable photosynthesis system infrared gas analyzer (LI-COR, Lincoln, NE, USA). Leaves were allowed to acclimate to cuvette conditions at 20°C, with the flow rate was set at 500 μmol s-1, CO2 concentration at 400 μmol mol-1, and at photosynthetic photon flux density (PPFD) of 1000 μmol m-2 s-1 PPFD (Barcaccia et al., 2022). Measurements of gsw were conducted every 2-h at 8 am, 10 am, 12 pm, 2 pm, and 4 pm, while A measurements were taken between 10 am and 12 pm, all at 55 DAT—during the peak drought stress period. In addition, a gradient of light intensities ranging from 0 to 2000 μmol m-2 s-1 PPFD was applied for A measurements according to the user’s manual. Intrinsic water use efficiency (iWUE) was also calculated by dividing the A by gsw, for each data point.
2.3.2 Chlorophyll fluorescence parameters
Chlorophyll fluorescence assessments were conducted using the auxiliary equipment integrated into the LI-6800 portable photosynthesis system infrared gas analyzer (LI-COR, Lincoln, NE, USA). Following the nomenclature proposed by Baker and Rosenqvist (2004), measurements of photosystem II (PSII) efficiency were performed to evaluate the conversion of light energy into chemical energy during photosynthesis. The maximal photochemical efficiency of photosystem II (Fv/Fm) ratio, representing the ratio of variable fluorescence (Fv) to maximum fluorescence (Fm) when all PSII reaction centers are open, was utilized for this assessment. Fv, calculated as the difference between Fm (maximum fluorescence) and Fo (minimum fluorescence), was determined for dark-adapted samples at 55 DAT. The readings were taken during the night, commencing at 7.30 pm, ensuring that the plants experienced a period of darkness before measurement. This dark adaptation period considered the sunset time, occurring between 5.30 pm and 6 pm in Zimbabwe. To determine the efficiency of PSII under light adaptation, calculations were made for the quantum yield of PSII (ΦPSII) calculated from fluorescence as the difference between 1 and the steady-state F (Fs) divided by maximal F, light-adapted (Fm’). Additionally, measurements of light adapted electron transport rate (ETR) and non-photochemical quenching (NPQ) were conducted across a gradient of light intensities, ranging from 0 to 2000 μmol m-2 s-1 PPFD at 55 DAT.
2.3.3 Biochemical analysis (proline determination)
Biochemical analysis of proline was done during the last day of drought stress and at 14-d of drought recovery. Analysis was based on Lee et al. (2018) and Gill et al. (2022) with modifications described below. The youngest fully expanded leaves were harvested, and proline accumulation analysis was immediately conducted to minimize changes in proline levels. Proline from 30 mg of fresh leaf material was extracted by homogenization in 200 μL of 1% aqueous sulfosalicylic acid (w/v) using a plastic micro pestle (MRS Scientific, UK) until a paste-like consistency was achieved. Another 800 μL of 1% aqueous sulfosalicylic acid (w/v) was added to the homogenized samples, followed by further grinding for 1 min to make it more efficient in extracting and quantifying proline from the leaf samples. The plant extract was divided into batches, and 66 μL of each extract was transferred to a separate Eppendorf tube, 132 μL of acid ninhydrin reagent (1.25% ninhydrin in 80% glacial acetic acid) was added. The Eppendorf tubes were placed in a 5L general purpose water bath at 95°C and incubated for 60 min to allow the reaction between proline and ninhydrin. After incubation, the reaction was stopped by immersing the tubes in an ice bath for 10 min. Following cooling, the absorbance of each reaction mixture was measured at 510 nm using a spectrophotometer (Thermo Scientific GENESYS 20-Monochromator, UK). A standard curve was obtained and the generated linear equation (R2 = 0.99) was used to calculate proline in plant extracts (Supplementary Figure 1).
2.4 Statistical analysis
Data was analyzed using a two-way analysis of variance (ANOVA) through the General Linear Model procedure in the Statistica Version 13.3 software (TIBCO Software Inc, USA). The effects of genotype (G), and water management (WM), as well as the interaction effects of these two factors (i.e., G × WM) on the various traits measured in the study were tested. To determine significant differences between means, Tukey’s Honestly Significant Difference (HSD) test was applied at a significance level of 5%. Figures representing key photosynthetic parameters were generated across different soil moisture levels (WW-75%FC, MD-40% FC, and SD-0% FC), using Sigma Plot 10.0 software (Systat Software Inc., Chicago, IL, USA). The gsw curve captured the diurnal stomatal dynamics, governing gas exchange, while the A curve revealed the plant’s carbon assimilation performance. These curves were then used to establish a linear correlation between A and gsw. Light curves for ΦPSII, ETR, and NPQ were plotted, showcasing the efficiency of light conversion, highlighting changes in electron flow through the photosynthetic chain, and illustrating plant responses to light stress.
3 Results
3.1 Rainout shelter conditions and visible hemp responses to soil moisture differences
Average maximum temperatures ranged from 24.7°C to 31.6°C, with minimum temperatures varying between 2.9°C and 18.6°C. Evaporation rates ranged from 2.6 mm to 10.5 mm, while sunshine hours fluctuated between 2.4 and 10.5-h (Supplementary Figure 2). Under the WW-75% FC treatment, the hemp plants maintained a healthy morphological status with green leaves. However, in the MD-40% FC treatment, visible signs of stress were observed, as evidenced by slight yellowish discoloration on the bottom leaves. The most noticeable differences in plant response occurred under the SD-0% FC treatment, where severe water stress was evident. The leaves displayed a pronounced yellowish-brown to purple coloration and exhibited a loss of turgor (Supplementary Figure 3).
3.2 Diurnal stomatal conductance dynamics under varying water availability
Highly significant differences (P<0.001; Figures 2A–C), were observed for gsw. Across the three water management treatments, stomatal conductance (gsw) exhibited a diurnal pattern that was specific to each genotype. In the WW-75% FC treatment, the gsw of all three genotypes remains relatively high throughout the day (Figure 2A), with values ranging from approximately 0.3 to 0.5 mol H2O m-2 s−1. At 8 am and 10 am, the gsw is high and statistically similar among the genotypes. As the day progresses to 12 pm, 2 pm, and 4 pm, there is a noticeable decrease in gsw. By 4 pm, a significant reduction is observed, particularly in ZB. This trend suggests that while WW-75% FC treatment allow for higher stomatal activity, diurnal variations still lead to a decline as the day progresses, likely due to increasing temperature and evaporative demand. Under MD-40% FC treatment, the gsw shows a marked decrease compared to the WW-75% FC treatment. At 8 am, all three genotypes have relatively high conductance, but it starts to decline sharply by 10 am and 12 pm (Figure 2B). MP and AQ show similar conductance trends, whereas ZB tends to have slightly lower values at these times. By 2 pm and 4 pm, gsw decreases further for all genotypes, with no significant differences among them at 4 pm. This indicates that moderate drought stress reduces overall stomatal activity and that the response varies slightly among genotypes. In SD-0% FC treatment, the gsw is consistently low throughout the day for all genotypes, reflecting the plants’ response to extreme water deficiency (Figure 2C). The values range from approximately 0.1 to 0.2 mol H2O m-2 s−1, indicating a substantial reduction in stomatal activity. At all times of the day, there are no significant differences among the genotypes, across all time points.
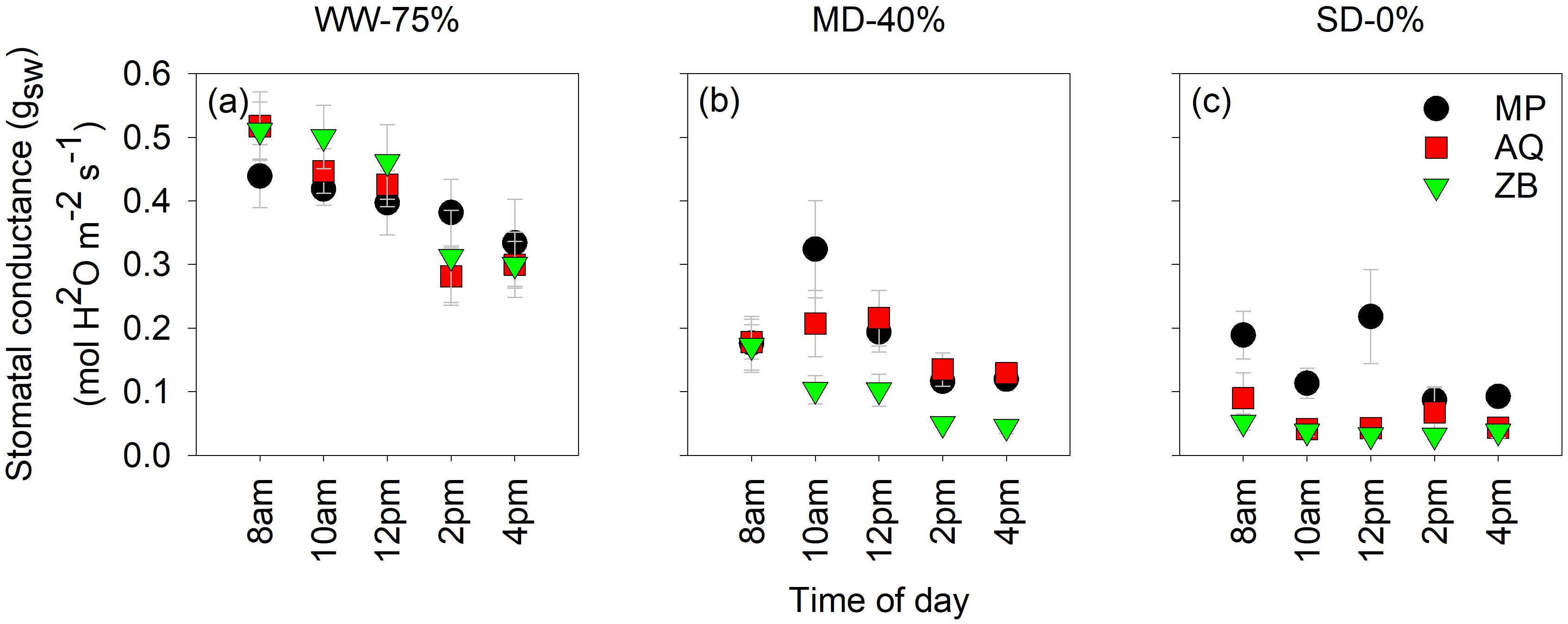
Figure 2. Diurnal curves of leaf stomatal conductance (gsw). Measurements were taken every 2-h from 8am to 4pm on the fourth fan-leaf counting from the top, using LI-6800 LICOR. MP (C. indica), AQ (C. sativa) and ZB (C. sativa). Times are shown in 12 h format, well-watered (WW-75% FC ); (A), moderate drought (MD-40% FC); (B), and severe drought (SD-0% FC); (C). The letters on the plots highlight statistical differences. Values are presented as mean ± se (n = 5).
3.3 Light response of net assimilation rate under drought stress
Across the different water managements, an increase in light intensity corresponded to a highly significant (P<0.001; Figures 3A–C) increase in A across all genotypes. Genotype ZB had the highest A value at a light intensity of 2000 μmol m-2 s-1, reaching a peak of 28.4 μmol·CO2 m-2 s-1. The MP genotype had a lower A value, while genotype AQ had the lowest A value, approximately half of ZB’s value. Interestingly, genotype AQ, showed a unique response characterized by a plateau effect at higher light levels, with the WW-75% FC treatment reaching a peak of 11.7 μmol·CO2 m-2 s-1 at 2000 μmol m-2 s-1 PPFD. Under MD-40% FC treatment, the A rates of all three genotypes are reduced compared to the WW-75% FC treatment (Figure 3B). The response to increasing PPFD is less pronounced, with maximum A rates reaching approximately 20 μmol·CO2 m-2 s-1. MP maintains the highest A rates across all light intensities, while ZB shows the lowest. Under SD-0% FC treatment, the A rates are substantially reduced for all genotypes (Figure 3C). The rates range from 0 to approximately 5 μmol·CO2 m-2 s-1 across the PPFD spectrum, indicating a minimal response to increasing light intensity.
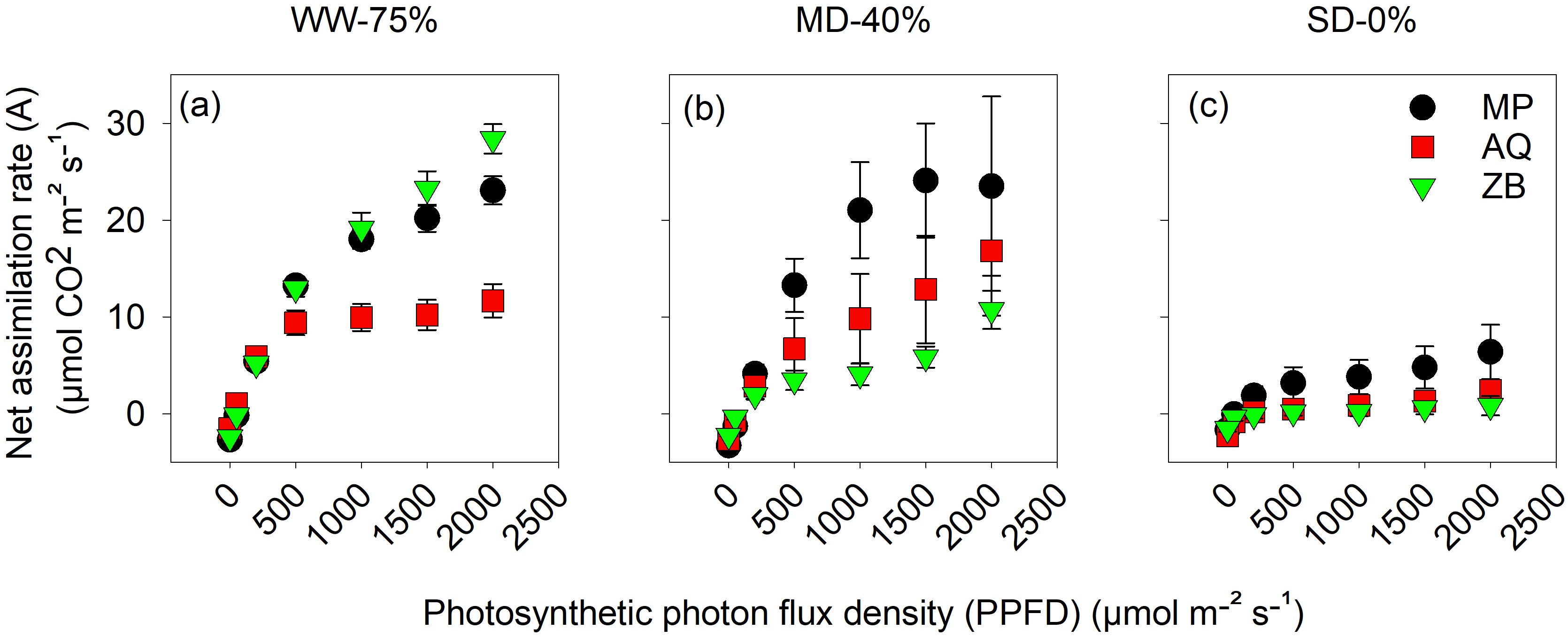
Figure 3. The net assimilation rate (A), measured in μmol CO2 m-2 s−1, plotted against photosynthetic photon flux density (PPFD) ranging from 0 to 2000 μmol•m-2 s -1 on young fully expanded leaves using LI-6800 LICOR at 45-d after transplanting. MP (C. indica), AQ (C. sativa) and ZB (C. sativa). Well-watered (WW-75% FC); (A), moderate drought (MD-40% FC); (B), and severe drought (SD-0% FC); (C). Values are presented as mean ± se (n = 5).
3.4 Intrinsic water use efficiency under drought stress
There was a highly significant interaction (P<0.001; Figures 4A–C) between water management and genotype for intrinsic water use efficiency (iWUE). In Figure 4A, which represents the WW-75% FC treatment, the A rate shows a positive correlation with gsw. As gsw increases from approximately 0.0 to 0.4 mol·H2O m-2 s-1, the A rate also increases, reaching up to about 28 μmol·CO2 m-2 s-1 for the ZB genotype. The AQ and MP genotypes display lower A rates compared to ZB but still follow a similar trend of increasing A rate with increasing gsw. Under MD-40% FC treatment, distinct patterns emerge among the different genotypes (Figure 4B). The MP genotype shows a tendency to shift toward the far right of the graph. The data points for MP extend to higher gsw values (up to 0.4 mol·H2O m-2 s-1) and demonstrate a relatively higher A rate compared to the other genotypes. The AQ and ZB genotypes exhibit clustering in the lower to mid-range gsw values (approximately 0.0 to 0.2 mol·H2O m-2 s-1). Their A rates are more varied but generally lower than those of MP, indicating that these genotypes do not maintain as high gsw under MD-40% FC treatment as MP. Figure 4C, representing SD-0% FC treatment, the A rate is significantly reduced across all genotypes. The A rates do not exceed 10 μmol·CO2 m-2 s-1, and most data points cluster around lower values of gsw (0.0 to 0.2 mol·H2O m-2 s-1). This suggests a substantial impact of SD-0% FC treatment on photosynthetic activity, regardless of the genotype.
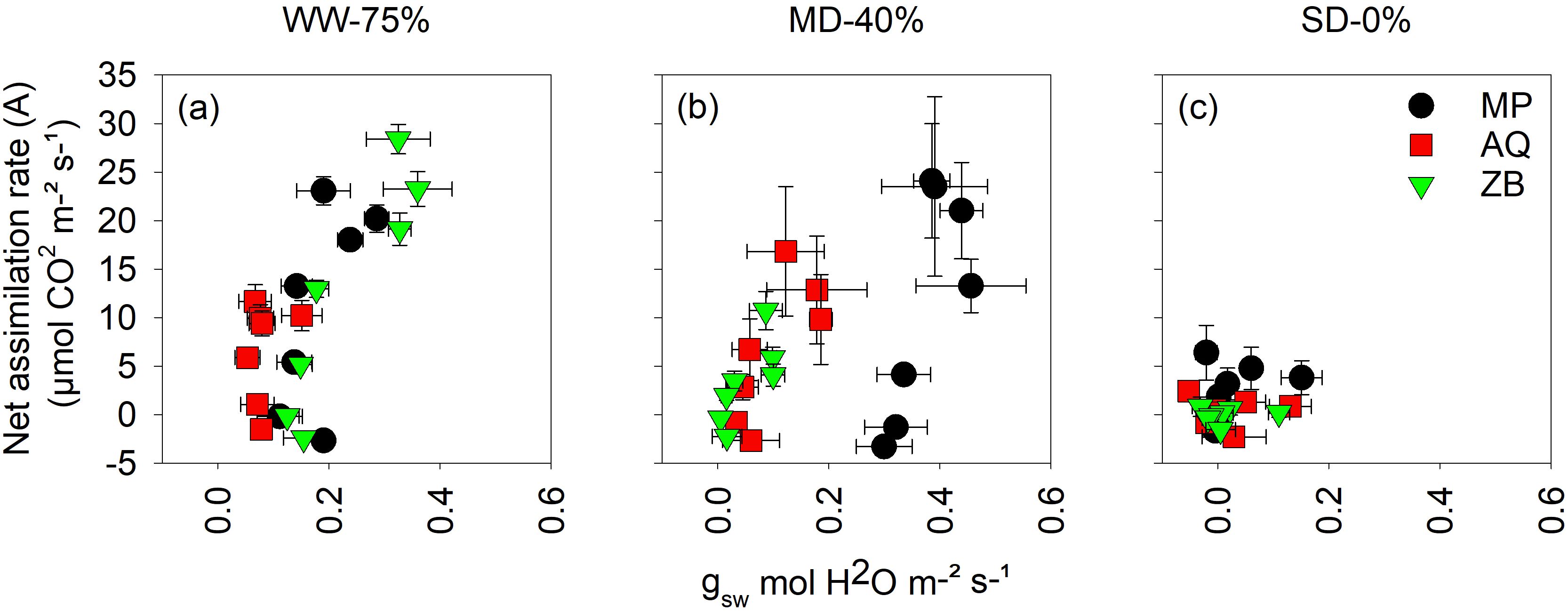
Figure 4. Intrinsic water-use efficiency (iWUE) taken on young fully expanded leaves using LI-6800 LICOR at 55-d after transplanting. MP (C. indica), AQ (C. sativa) and ZB (C. sativa). Well-watered (WW-75% FC); (A), moderate drought (MD-40% FC); (B), and severe drought (SD-0% FC); (C). Values are presented as mean ± se (n = 5).
3.5 Light response of chlorophyll fluorescence parameters under drought stress
A highly significant interaction (P<0.001; Figures 5A–I) between water management and genotype for ΦPSII, NPQ, and ETR. Genotype MP and ZB both showed slightly higher ΦPSII under the WW-75% FC treatment (Figure 5A), compared to AQ at lower PPFD values. The decline in ΦPSII suggests light saturation effects as PPFD increases. Under MD-40% FC treatment, ΦPSII still decreases with increasing PPFD, but the values are generally lower compared to the WW-75% FC treatment (Figure 5B). MP maintains higher ΦPSII at lower PPFD, while AQ and ZB exhibit similar declines. Under SD-0% FC treatment, ΦPSII is substantially lower across all genotypes and PPFD levels, indicating significant photoinhibition or stress (Figure 5C). MP, AQ, and ZB show minimal differences, with ΦPSII values clustering closely together.
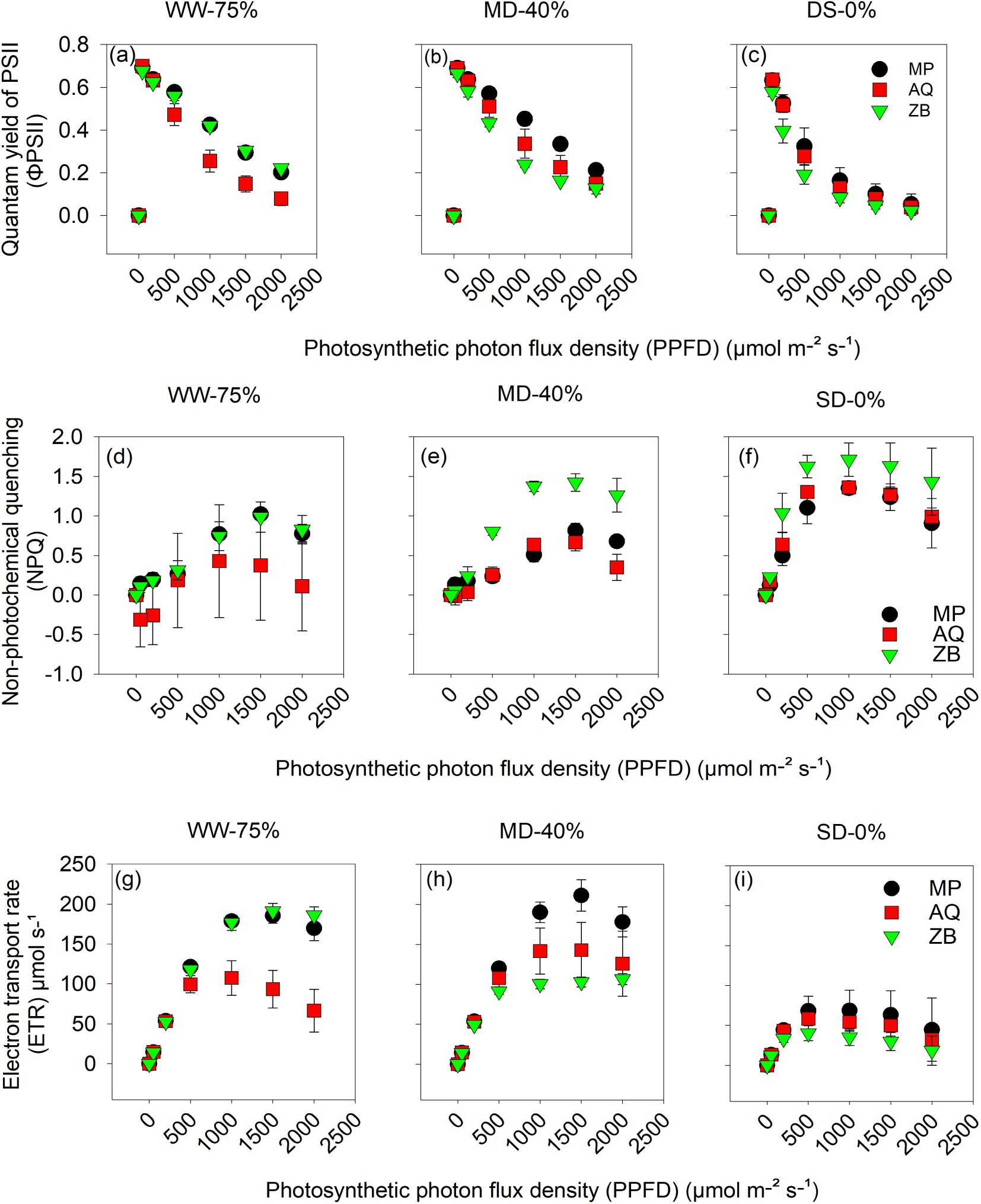
Figure 5. Quantum yield of PSII (ΦPSII); (A–C) calculated from fluorescence as the difference between 1 and the steady-state F (Fs) divided by maximal F light-adapted (Fm')., non-photochemical quenching (NPQ); (D–F) and electron transport rate (ETR); (G–I) taken at different light intensities (0, 500, 1000, 1500 and 2000 μmol•mol-1 PPFD) on young fully expanded leaves at 55-d after transplanting. MP (C. indica), AQ (C. sativa) and ZB (C. sativa). Well-watered (WW-75% FC), moderate drought (MD-40% FC), and severe drought (SD-0% FC). Values are presented as mean ± se (n = 5).
In Figure 5D, under the WW-75% FC treatment, NPQ increases with PPFD, indicating enhanced energy dissipation as heat to protect the photosystem. ZB shows the highest NPQ values, suggesting a robust photoprotective mechanism, followed by MP and AQ. Under MD-40% FC treatment, NPQ still increases with PPFD, but the differences between genotypes are less pronounced (Figure 5E). ZB maintains slightly higher NPQ, indicating effective energy dissipation under moderate drought. In Figure 5F, under SD-0% FC treatment, NPQ values are highest under severe drought across all genotypes, reflecting increased stress and the need for photoprotection. ZB continues to show higher NPQ, followed by MP and AQ, but the differences are minimal.
In Figure 5G, WW-75% FC treatment, ETR increases with PPFD for all genotypes, with MP and ZB showing higher rates compared to AQ, indicating more efficient electron transport under the WW-75% FC treatment. Under MD-40% FC treatment, ETR increases with PPFD but at a reduced rate compared to the WW-75% FC treatment (Figure 5H). MP maintains a higher ETR than AQ and ZB, suggesting better electron transport efficiency under stress. In Figure 5I, ETR values are significantly reduced under SD-0% FC treatment across all PPFD levels. MP, AQ, and ZB exhibit similar ETR values, indicating severe limitations in electron transport due to extreme drought stress.
After dark adaptation, Fv/Fm was significantly (P<0.05; Table 1) affected by the interaction between water management and genotype. Genotype MP showed remarkable stability, with Fv/Fm values of 0.83 under WW-75% FC treatment, increasing by 1.2% under MD-40% FC treatment, and remaining stable with no change under SD-0% FC treatment. Genotype AQ exhibited an increase of 3.7% under MD-40% FC treatment, but a significant decrease of 2.4% under SD-0% FC treatment. Genotype ZB maintained a stable Fv/Fm value of 0.85 under WW-75% FC treatment and MD-40% FC treatment, but experienced a 5.9% decrease to 0.80 under SD-0% FC treatment.
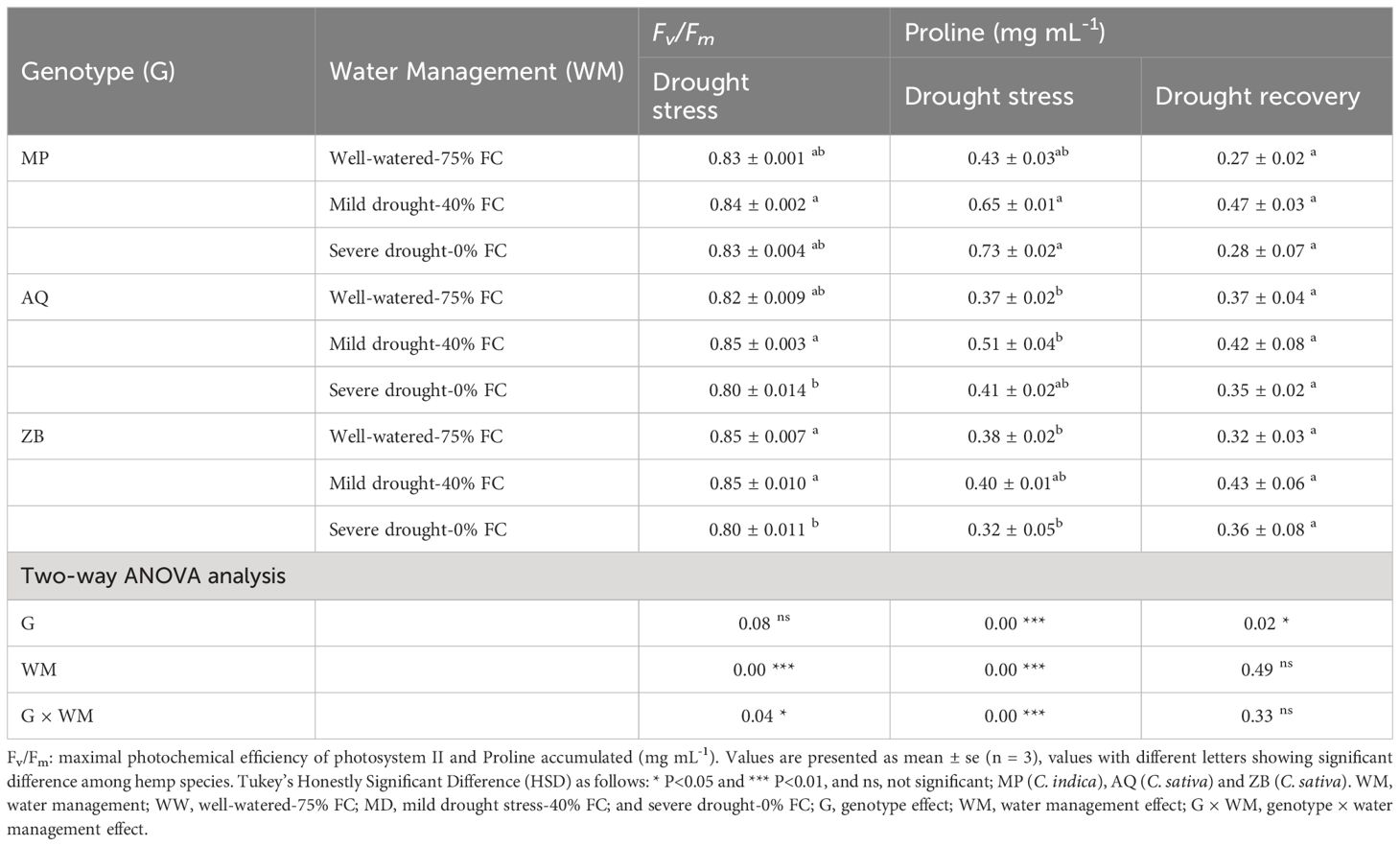
Table 1. Fv/Fm and proline content across three genotypes (MP, AQ, ZB) under different water management treatments.
3.6 Influence of drought on proline accumulation
There was a significant interaction (P<0.001; Table 1), involving both water management and genotype. Genotype MP shows a significant increase in proline content under both MD-40% FC treatment (51.2%) and SD-0% FC treatment (69.8%) compared to WW-75% FC treatment. Genotype AQ exhibits a moderate increase in proline content under MD-40% FC treatment (37.8%) and a slight increase under SD-0% FC treatment (10.8%). Genotype ZB has a minimal increase in proline content under MD-40% FC treatment (5.3%) and a decrease under SD-0% FC treatment (15.8%), Throughout the drought recovery period, there was a general decline in proline concentrations, with significant differences (P<0.05; Table 1) observed only among the three genotypes (Figure 6). It is important to highlight that genotype ZB showed significant increases in proline levels during drought recovery phases, especially under MD-40% FC treatment recovery (34.4% increase).
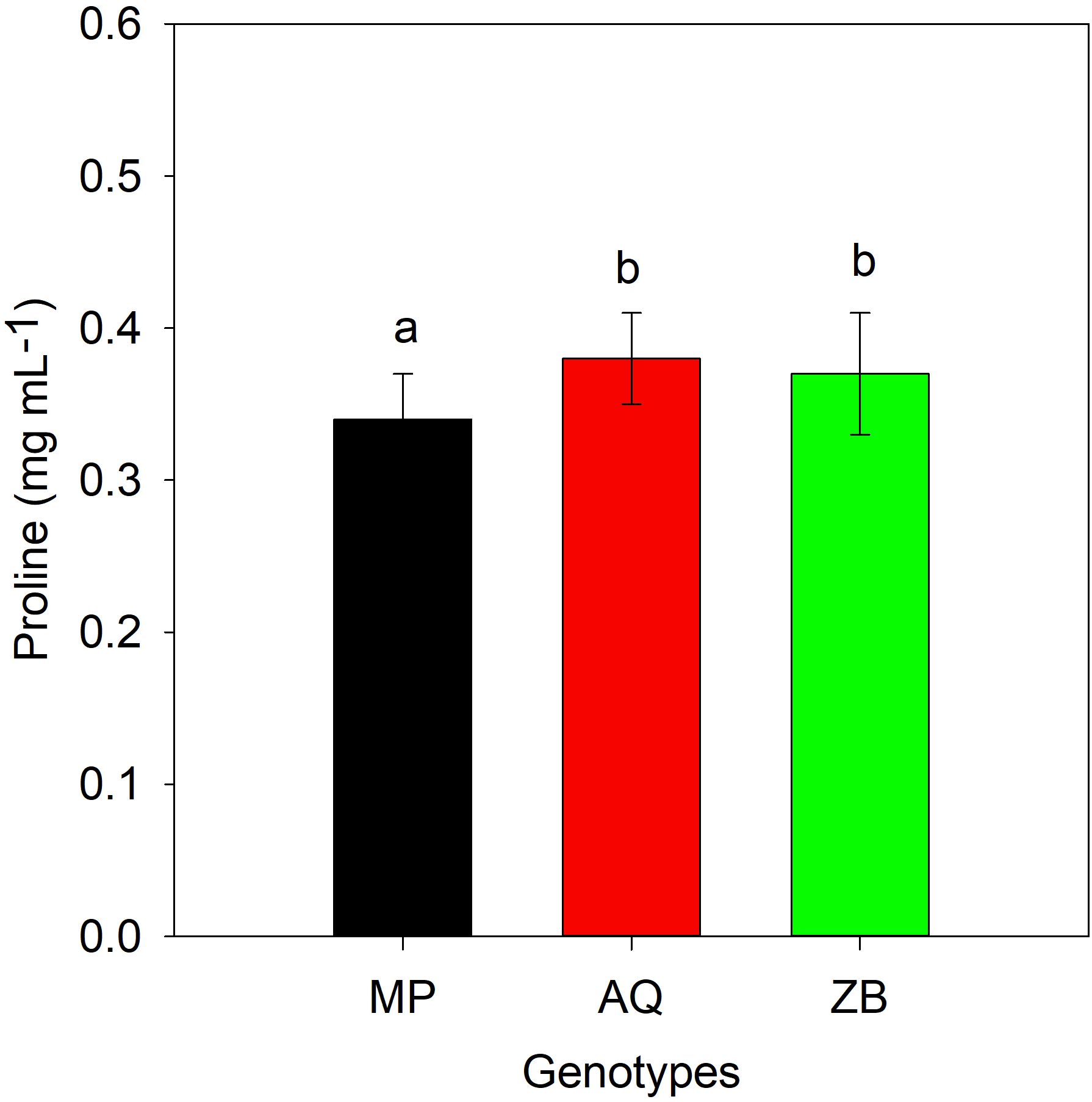
Figure 6. Proline accumulated (mg mL-1). Values are presented as mean ± se (n = 3), values with different letters showing significant difference among hemp species, after 20 days drought recovery period using Tukey's Honestly Significant Difference (HSD). MP (C. indica), AQ (C. sativa) and ZB (C. sativa).
3.7 Shoot fresh during drought recovery
The interaction effects between water management and genotype on shoot fresh weight (SFW) were not statistically significant (P>0.05, Table 2). Interestingly, Genotype ZB shows minimal reduction in SFW under drought conditions, with only a 2.4% decrease from well-watered to severe drought. Genotype AQ exhibits resilience with a slight increase in SFW under mild drought and a stable SFW under severe drought, showing only a 0.3% decrease from mild to severe drought. Genotype MP is more affected by drought, with an 8.8% decrease in SFW from WW-75% FC treatment to SD-0% FC treatment.
4 Discussion
In this study, the major morphophysiological adaptations and proline signaling of three distinct hemp genotypes—MP (C. indica), AQ (C. sativa), and ZB (C. sativa)—originating from Switzerland, South Africa, and Zimbabwe, respectively were investigated. During the study, these genotypes were exposed to water management stress in a generally warm to hot climate, characterized by occasional temperature fluctuations. The evaporation rates indicated varying degrees of water loss. When water was abundant, hemp plants displayed resilience with green leaves, demonstrating their ability to adapt to well-watered conditions. However, when subjected to moderate water deficit, signs of stress were observed across all genotypes, highlighting the genotype-specific responses to this level of water scarcity. Severe water deficit triggered pronounced stress responses, such as yellowish-brown to purple discoloration in the AQ genotype and turgor loss in leaves, which was particularly noticeable in the ZB genotype. These observations emphasize the impact of severe water deficit conditions on hemp genotypes, and underscore the need for a nuanced understanding of their responses to different water management strategies.
The study also examined the morphological differences and drought tolerance mechanisms among different hemp genotypes. The environmental conditions in Zimbabwe and South Africa, characterized by warm climates and diverse soil compositions, may contribute to C. sativa’s adaptability to arid conditions. On the other hand, the cooler climate in Switzerland may influence the growth of C. indica. Morphological differences, such as taller structures in C. sativa and a more compact appearance in C. indica, could affect water utilization efficiency and drought response. Previous studies on other crops suggest that leaf shape, size, and stomatal density are crucial factors in drought tolerance. These findings align with observations by Mateva et al. (2023) on bambara groundnut (Vigna subterranea (L.) Verdc.). They identified differential deep root foraging and density patterns in genotypes sourced from dry and wet regions, highlighting the significant influence of environmental factors on plant traits and unintentional selection by farmers (Mateva, 2022). With these findings in mind, future research on hemp genotypes could benefit from a holistic approach that incorporates both above and below-ground phenotyping to develop more resilient varieties.
4.1 Diurnal stomatal dynamics and genotypic responses to water deficit: adaptability and water use strategies
Distinctive patterns in gsw, a key parameter influencing water loss and overall plant water status, were observed. Stomatal behavior exhibited diurnal variation, consistent across different water treatments, indicating dynamic gaseous exchange throughout the day (Farooq et al., 2009). Under optimal water conditions, ZB consistently exhibited higher gsw throughout most of the day compared to MP and AQ. However, in the afternoon, ZB showed a decline in gsw while MP recorded higher values. This afternoon data is valuable for distinguishing stress-sensitive and stress-insensitive genotypes. This genotype-specific variation in stomatal regulation aligns with previous research highlighting the significance of stomatal conductance (gsw) in plant responses to water availability (Seleiman et al., 2021). As explained by Farooq et al. (2009) and Gill et al. (2022), stomatal closure is associated with reduced leaf turgor, water potential, and overall biomass accumulation.
4.2 Interplay of assimilation rates, stomatal conductance, under varying light, and drought stress
In the investigation, it was found that under scenarios of increased light intensity (2000 μmol m-2s-1 PPFD) combined with sufficient water availability, the ZB and MP genotypes demonstrated significantly higher A compared to the AQ genotype. However, when exposed to stress-inducing conditions characterized by drought stress and intense light, a noticeable trade-off emerged. The MP genotype exhibited elevated A under both moderate and severe water deficit conditions, while the ZB genotype had the lowest A in both treatments. This suggests a dynamic utilization of resources, highlighting the adaptability of each genotype to varying environmental challenges. Reduced A in plants serves as a reliable indicator of compromised growth, yield, and metabolic processes, often associated with environmental stress such as drought (Jones et al., 2018). This decline reflects the plant’s impaired ability to effectively capture and convert carbon dioxide, disrupting the synthesis of essential molecules crucial for growth and development (Smith et al., 2020). Furthermore, the subsequent shift in the energy budget may result in altered resource allocation, further impacting overall plant resilience in challenging conditions (García-Tejero et al., 2019).
Further investigation was conducted to explore the relationship between A and gsw to calculate iWUE (Rouhi et al., 2007; Herppich et al., 2020; Li et al., 2021). iWUE reflects a plant’s efficiency in utilizing water for photosynthetic carbon assimilation, indicating its ability to optimize carbon gain while minimizing water loss. The study highlighted the varied responses among genotypes to drought stress. The genotypes ZB and AQ demonstrated a conservative water use strategy, while MP showed a potential trade-off between water use efficiency and carbon gain under mild drought stress. This emphasizes that different hemp genotypes, landraces, or species may have disparate responses. Some may prioritize water conservation through significant stomatal closure, while others use adaptive strategies to balance photosynthetic needs with water conservation.
4.3 Insights from chlorophyll fluorescence and biochemical variations among genotypes
Drought stress poses considerable challenges to plant growth, especially impacting ΦPSII (Sapeta et al., 2023). Our study aligns with existing research, supporting a significant decrease in ΦPSII activity under severe drought stress. Notably, the MP genotype consistently exhibited superior photosynthetic performance, maintaining high ΦPSII values even under drought stress, in contrast to the responses of ZB and AQ genotypes. This robust performance in MP correlated with elevated ETR, reflecting higher A and growth, as suggested by (Wang et al., 2021). Higher Fv/Fm values were observed in MP, attributed to its ability to maintain cellular hydration under drought stress, preventing photoinhibition and preserving the D1 protein associated with PSII (Abid et al., 2018; Makonya et al., 2020). While ZB and AQ genotypes generally exhibited healthy plants with Fv/Fm values close to the theoretical maximum under well-watered and moderate drought stress conditions, severe drought stress led to a decline in Fv/Fm values, indicating compromised ETR. ZB and AQ recorded Fv/Fm values of 0.8 under severe drought stress, a threshold considered indicative of stress in many plant species (Makonya, 2019). The reduction in Fv/Fm is often associated with an increase in the efficiency of NPQ (Guan et al., 2015; Możdżeń et al., 2015; Bano et al., 2021).
Notably, the ZB genotype demonstrated pronounced NPQ under well-watered conditions, as well as during moderate and severe drought stress, spanning diverse light intensities. The impact of light intensities or photon flux densities on photosynthesis is noteworthy (Huang et al., 2021). In conditions of low light, plants tend to augment the antenna size of photosystems, enhancing the capture and transfer of excitation energy to the photosynthetic reaction centers (Green and Parson, 2003). Conversely, when exposed to excessive light, as observed in our study, plants dissipate surplus excitation energy through NPQ of the excited state of Chlorophyll a, releasing it as heat (Miu, 2012; Saglam et al., 2014; Liu et al., 2019; Bano et al., 2021; Hu et al., 2023). Our study underscores this dynamic interplay between drought stress, light intensities and the plant’s photoprotective mechanisms, with the ZB genotype serving as a noteworthy example.
Proline accumulation is indicative of osmotic regulation, has been reported in numerous studies (Marin et al., 2010; Abid et al., 2018; Gill et al., 2022). The MP genotype consistently showed increased proline levels under both moderate and severe drought stress, aligning with trends observed in drought-tolerant germplasms of peanut (Furlan et al., 2020) and rice (Dien et al., 2019). This emphasizes the importance of up-regulating P5CS, a key enzyme in proline biosynthesis, as a significant factor influencing proline production, as highlighted in the study by Parkash and Singh (2020). As expected, proline concentrations generally decreased during the drought recovery period. However, the ZB genotype-maintained proline concentrations without decline, suggesting optimized resource allocation or alternative stress-responsive mechanisms. This ability to moderate proline levels after stress, avoiding excessive energy expenditure (Forlani et al., 2019), implies preparedness for recurring stress, acting as a preventive measure (Wang et al., 2018). ZB’s resilience may also involve enhanced antioxidant production or specific gene activation (Seok et al., 2023), contributing to its strong capacity to sustain growth and biomass production under water stress. This suggests that ZB possesses physiological or genetic traits that confer drought resilience. Similarly, AQ demonstrates effective adaptation to fluctuating water availability, adjusting its physiological processes to cope with both moderate and severe water stress without significant biomass loss.
Future studies should validate these hypotheses to enhance our understanding of ZB’s complex stress adaptation strategies. Addressing the non-significant water management effects and G × WM interaction by increasing sample size and replicating under different environmental conditions would provide more robust conclusions. Given the significant genotype effect, research should include a broader range of genotypes to capture the full scope of genetic variability in drought response. Incorporating physiological and biochemical parameters such as root depth, leaf water potential, pigment composition, photosynthetic complex stoichiometry, and the presence of reactive oxygen species would offer deeper insights into drought tolerance mechanisms.
5 Conclusion
In this study, the major morphophysiological adaptations and proline signaling of three hemp genotypes—MP (C. indica), AQ (C. sativa), and ZB (C. sativa)—were investigated under varying water management conditions. ZB maintained proline levels during drought recovery, suggesting optimized resource allocation and alternative stress-responsive mechanisms, while MP showed increased proline levels under drought, indicating reliance on osmotic regulation. ZB exhibited dynamic stomatal conductance and high non-photochemical quenching (NPQ), highlighting its effective water use and photoprotective strategies. MP maintained high assimilation rates (A) and superior photosynthetic performance (ΦPSII) under drought stress. These findings underscore the importance of proline accumulation, gsw, A, and photoprotective mechanisms in drought resilience. Future research should include a broader range of genotypes and additional physiological and biochemical parameters to fully understand the genetic variability in drought response and enhance the development of drought-tolerant hemp varieties.
Data availability statement
The original contributions presented in the study are included in the article/Supplementary Material. Further inquiries can be directed to the corresponding author.
Author contributions
KM: Conceptualization, Data curation, Formal analysis, Investigation, Methodology, Software, Supervision, Validation, Visualization, Writing – original draft, Writing – review & editing. EM: Formal analysis, Investigation, Writing – original draft, Writing – review & editing. TM: Investigation, Supervision, Writing – review & editing. CK: Conceptualization, Investigation, Supervision, Writing – review & editing. FM: Conceptualization, Funding acquisition, Investigation, Project administration, Resources, Supervision, Validation, Writing – review & editing. SD: Conceptualization, Funding acquisition, Investigation, Project administration, Resources, Supervision, Validation, Writing – review & editing.
Funding
The author(s) declare financial support was received for the research, authorship, and/or publication of this article. The support of the Tobacco Research Board t/a Kutsaga in funding this research is gratefully acknowledged.
Acknowledgments
We would like to express our gratitude to the Plant Breeding Division at Kutsaga for providing the genotypes used in this study. Special thanks go to Mr. Hillary Zharo from the Department of Biotechnology at the University of Zimbabwe for his valuable assistance in proline quantification. Our gratitude is extended to Dr. Givemore Munashe Makonya from Washington State University (WSU) and Kutsaga’s editorial team for their dedicated efforts in assisting with the internal review of the manuscript. Their valuable contributions and feedback have greatly improved the quality of this work.
Conflict of interest
The authors declare that the research was conducted in the absence of any commercial or financial relationships that could be construed as a potential conflict of interest.
Publisher’s note
All claims expressed in this article are solely those of the authors and do not necessarily represent those of their affiliated organizations, or those of the publisher, the editors and the reviewers. Any product that may be evaluated in this article, or claim that may be made by its manufacturer, is not guaranteed or endorsed by the publisher.
Supplementary material
The Supplementary Material for this article can be found online at: https://www.frontiersin.org/articles/10.3389/fphgy.2024.1441262/full#supplementary-material
References
Abid M., Ali S., Qi L. K., Zahoor R., Tian Z., Jiang D., et al. (2018). Physiological and biochemical changes during drought and recovery periods at tillering and jointing stages in wheat (Triticum aestivum L.). Sci. Rep. 8, 1–15. doi: 10.1038/s41598-018-21441-7
Ahmed M., Aslam M. A., Hassan F. U. L., Hayat R., Ahmad S., Ahmed M., et al. (2019). Biochemical, physiological and agronomic response of wheat to changing climate of rainfed areas of Pakistan. Pakistan Botanical 51, 2, 535–551. doi: 10.30848/PJB2019
Baker N. R., Rosenqvist E. (2004). Applications of chlorophyll fluorescence can improve crop production strategies: An examination of future possibilities. J. Exp. Bot. 55, 1607–1621. doi: 10.1093/jxb/erh196
Bano H., Athar H.ur R., Zafar Z. U., Ogbaga C. C., Ashraf M. (2021). Peroxidase activity and operation of photo-protective component of NPQ play key roles in drought tolerance of mung bean [Vigna radiata (L.) Wilcziek]. Physiologia Plantarum 172, 603–614. doi: 10.1111/ppl.13337
Barcaccia G., Palumbo F., Scariolo F., Vannozzi A., Borin M., Bona S., et al. (2022). Cannabis sativa: an optimization study for ROI by bachelor of science in mechanical engineering Cannabis sativa : an optimization study for ROI. Front. Plant Sci. 13, 1–29. doi: 10.3390/plants10010185
Baudoin M. A., Vogel C., Nortje K., Naik M. (2017). Living with drought in South Africa: lessons learnt from the recent El Niño drought period. Int. J. Disaster Risk Reduction 23, 128–137. doi: 10.1016/j.ijdrr.2017.05.005
Dien D. C., Thu T. T. P., Moe K., Yamakawa T. (2019). Proline and carbohydrate metabolism in rice varieties (Oryza sativa L.) under various drought and recovery conditions. Plant Physiol. Rep. 24, 376–387. doi: 10.1007/s40502-019-00462-y
Efeoğlu B. (2009). Heat shock proteins and heat shock response in plants. Gazi Univ. J. Sci. 22, 67–75.
Ehrensing D. T. (1998). Feasibility of Industrial Hemp Production in the United States Pacific Northwest (OSU Agricultural Experiment Station Bulletin), 1–46. Available at: https://catalog.extension.oregonstate.edu/sites/catalog.extension.oregonstate.edu/files/project/pdf/sb681.pdf%5Cnhttp://hdl.handle.net/1957/13306.
Farooq M., Basra S. M. A., Wahid A., Ahmad N., Saleem B. A. (2009). Improving the drought tolerance in rice ( Oryza sativa L.) by exogenous application of salicylic acid. Journal of Agronomy and Crop Science 195 (4), 237–246. doi: 10.1111/j.1439-037X.2009.00365.x
Forlani G., Bertazzini M., Cagnano G. (2019). Stress-driven increase in proline levels, and not proline levels themselves, correlates with the ability to withstand excess salt in a group of 17 Italian rice genotypes. Plant Biol. J. 21, 336–342. doi: 10.1111/plb.12916
Furlan A. L., Bianucci E., Giordano W., Castro S., Becker D. F. (2020). Proline metabolic dynamics and implications in drought tolerance of peanut plants. Plant Physiol. biochemistry: PPB 151, 566–578. doi: 10.1016/j.plaphy.2020.04.010
García-Tejero I. F., Durán Zuazo V. H., Sánchez-Carnenero C., Hernández A., Ferreiro-Vera C., Casano S. (2019). Seeking suitable agronomical practices for industrial hemp (Cannabis sativa L.) cultivation for biomedical applications. Ind. Crops Products 139. doi: 10.1016/j.indcrop.2019.111524
Gilbert G. S., Diaz A., Bregoff H. A. (2023). Seed disinfestation practices to control seed-borne fungi and bacteria in home production of sprouts. Foods 12, 747. doi: 10.3390/foods12040747
Gill A. R., Loveys B. R., Cowley J. M., Hall T., Cavagnaro T. R., Burton R. A. (2022). Physiological and morphological responses of industrial hemp (Cannabis sativa L.) to water deficit. Ind. Crops Products 187, 115331. doi: 10.1016/j.indcrop.2022.115331
Green B., Parson W. (2003). Light-harvsting antennas in photosynthesis. Photosynthesis Research 82, 109. doi: 10.1007/978-94-017-2087-8
Guan X. K., Song L., Wang T. C., Turner N. C., Li F. M. (2015). Effect of drought on the gas exchange, chlorophyll fluorescence and yield of six different-era spring wheat cultivars. J. Agron. Crop Sci. 201, 253–266. doi: 10.1111/jac.12103
Hayat S., Hayat Q., AlYemeni M. N., Wani A. S., Pichtel J., Ahmad A. (2012a). Role of proline under changing environments: A review. Plant Signaling Behav. 7, 1456–1466. doi: 10.4161/psb.21949
Hayat S., Khalique G., Irfan M., Wani A. S., Tripathi B. N., Ahmad A. (2012b). Physiological changes induced by chromium stress in plants: an overview. Protoplasma 249, 599–611. doi: 10.1007/s00709-011-0331-0
Herppich W. B., Gusovius H. J., Flemming I., Drastig K. (2020). Effects of drought and heat on photosynthetic performance, water use and yield of two selected fiber hemp cultivars at a poor-soil site in brandenburg (Germany). Agronomy 10, 8–10. doi: 10.3390/agronomy10091361
Hu F., Zhang Y., Guo J. (2023). Effects of drought stress on photosynthetic physiological characteristics, leaf microstructure, and related gene expression of yellow horn. Plant Signaling Behav. 18. doi: 10.1080/15592324.2023.2215025
Huang M. Y., Wong S. L., Weng J. H. (2021). Rapid light-response curve of chlorophyll fluorescence in terrestrial plants: Relationship to CO2 exchange among five woody and four fern species adapted to different light and water regimes. Plants 10, 1–13. doi: 10.3390/plants10030445
Jones Z. L., Mikkelson K. M., Nygren S., Sedlak D. L., Jonathan O. (2018). Establishment and convergence of photosynthetic microbial biomats in shallow unit process open-water wetlands. Water Res. doi: 10.1016/j.watres.2018.01.021
Kalaji H. M., Jajoo A., Oukarroum A., Brestic M. (2016). Chlorophyll a fluorescence as a tool to monitor physiological status of plants under abiotic stress conditions Chlorophyll a fluorescence as a tool to monitor physiological status of plants under abiotic stress conditions. Acta Physiologiae Plantarum, 38. doi: 10.1007/s11738-016-2113-y
Lee M. R., Kim C. S., Park T., Choi Y. S., Lee K. H. (2018). Optimization of the ninhydrin reaction and development of a multiwell plate-based high-throughput proline detection assay. Analytical Biochem. 556, 57–62. doi: 10.1016/j.ab.2018.06.022
Li H., Yang Y., Wang H., Liu S., Jia F., Su Y., et al. (2021). The receptor-like kinase erecta confers improved water use efficiency and drought tolerance to poplar via modulating stomatal density. Int. J. Mol. Sci. 22. doi: 10.3390/ijms22147245
Liu B., Liang J., Tang G., Wang X., Liu F., Zhao D. (2019). Drought stress affects on growth, water use efficiency, gas exchange and chlorophyll fluorescence of Juglans rootstocks. Scientia Hortic. 250, 230–235. doi: 10.1016/j.scienta.2019.02.056
Makonya G. M. (2019). Thermo and drought tolerance markers and regulation of heat stress proteins for chickpea (Cicer arietinum L.; Fabaceae) production in NE South Africa (Faculty of Science, Department of Biological Sciences). Available at: http://hdl.handle.net/11427/32397.
Makonya G. M., Ogola J. B., Muasya A. M., Crespo O., Maseko S., Valentine A. J., et al. (2020). Intermittent moisture supply induces drought priming responses in some heat-tolerant chickpea genotypes. Crop Sci. 60, 2527–2542. doi: 10.1002/csc2.20228
Marin J. A., Andreu P., Carrasco A., Arbeloa A. (2010). Determination of proline concentration, an abiotic stress marker, in root exudates of excised root cultures of fruit tree rootstocks under salt stress 24, 722–727. http://hdl.handle.net/10261/255659
Mateva K. I. (2022). Root trait variation and its contribution to drought tolerance in bambara groundnut (Vigna subterranea (L.) Verdc.). PhD thesis (University of Nottingham).
Mateva K. I., Tan X. L., Halimi R. A., Chai H. H., Makonya G. M., Gao X. (2023). Bambara groundnut (Vigna subterranea (L.) Verdc.). 557–615. doi: 10.1016/B978-0-323-90537-4.00021-1
McPartland J. M. (2018). Cannabis systematics at the levels of family, genus, and species Vol. 3 (Cannabis and Cannabinoid Research), 203–212. doi: 10.1089/can.2018.0039
Miu L. (2012). Response of photosynthesis and chlorophyll fluorescence to drought stress in two maize cultivars. Afr. J. Agric. Reseearch 7, 4751–4760. doi: 10.5897/AJAR
Możdżeń K., Bojarski B., Rut G., Migdałek G., Repka P., Rzepka A. (2015). Effect of drought stress induced by Mannitol on physiological parameters of maize (Zea mays L.) seedlings and plants. J. Microbiology Biotechnol. Food Sci. 4(special issue 2, 86–91. doi: 10.15414/jmbfs.2015.4.special2.86-91
Mugiyo H., Magadzire T., Choruma D. J., Chimonyo V. G. P., Manzou R., Jiri O., et al. (2023). El Niño’s effects on southern African agriculture in 2023/24 and anticipatory action strategies to reduce the impacts in Zimbabwe. Atmosphere 14, 1692. doi: 10.3390/atmos14111692
Parkash V., Singh S. (2020). A review on potential plant-based water stress indicators for vegetable crops. Sustainability 12, 3945. doi: 10.3390/su12103945
Raza A., Charagh S., Corpas F. J., Jin W. (2023). Assessment of proline function in higher plants under extreme temperatures. Plant biology 25, 379–395. doi: 10.1111/plb.13510
Rouhi V., Samson R., Lemeur R., van Damme P. (2007). Photosynthetic gas exchange characteristics in three different almond species during drought stress and subsequent recovery. Environ. Exp. Bot. 59, 117–129. doi: 10.1016/j.envexpbot.2005.10.001
Russo E. B. (2019). The case for the entourage effect and conventional breeding of clinical cannabis: No “Strain,” no gain. Front. Plant Sci. 9. doi: 10.3389/fpls.2018.01969
Saglam A., Terzi R., Demiralay M. (2014). Effect of polyethylene glycol induced drought stress on photosynthesis in two chickpea genotypes with different drought tolerance. Acta Biologica Hungarica 65, 178–188. doi: 10.1556/ABiol.65.2014.2.6
Salentijn E. M. J., Zhang Q., Amaducci S., Yang M., Trindade L. M. (2015). New developments in fiber hemp (Cannabis sativa L.) breeding. Ind. Crops Products 68, 32–41. doi: 10.1016/j.indcrop.2014.08.011
Sapeta H., Yokono M., Takabayashi A., Ueno Y., Cordeiro A. M., Hara T., et al. (2023). Reversible down-regulation of photosystems I and II leads to fast photosynthesis recovery after long-term drought in Jatropha curcas. J. Exp. Bot. 74, 336–351. doi: 10.1093/jxb/erac423
Seleiman M. F., Al-suhaibani N., Ali N., Akmal M., Alotaibi M., Refay Y., et al. (2021). Alleviate Its Adverse Effects. 1–25.
Seok H.-Y., Lee S.-Y., Sarker S., Bayzid Md, Moon Y.-H. (2023). Genome-wide analysis of stress-responsive genes and alternative splice variants in Arabidopsis roots under osmotic stresses. Int. J. Mol. Sci. 24, 14580. doi: 10.3390/ijms241914580
Sifundza L. S., van der Zaag P., Masih I. (2019). Evaluation of the responses of institutions and actors to the 2015/2016 el niño drought in the komati catchment in Southern Africa: Lessons to support future drought management. Water SA 45, 547–559. doi: 10.17159/wsa/2019.v45.i4.7535
Small E., Cronquist A. (1976). “A practical and natural taxonomy for cannabis author (s): ernest small and arthur cronquist,” in Taxon, vol. 25.
Small E., Marcus D. (2002). Hemp: a new crop with new uses for North America. Eds. Janick J., Whipkey A. (Alexandria, VA: ASHS Press), 284–326. Available at: http://www.hort.purdue.edu/newcrop/ncnu02/v5284.html%0Ahttp://www.hemphasis.com/files/publications/newuses.htm.
Smith M. N., Taylor T. C., van Haren J., Rosolem R., Restrepo-Coupe N., Adams J., et al. (2020). Empirical evidence for resilience of tropical forest photosynthesis in a warmer world. Nat. Plants 6, 1225–1230. doi: 10.1038/s41477-020-00780-2
Van Der Werf H. M. G., Mathijssen E. W. J. M., Haverkort A. J. (1996). The potential of hemp (Cannabis sativa L.) for sustainable fibre production: A crop physiological appraisal. Ann. Appl. Biol. 129. doi: 10.1111/j.1744-7348.1996.tb05736.x
Verslues P. E., Kim Y.-S., Zhu J.-K. (2007). Altered ABA, proline and hydrogen peroxide in an Arabidopsis glutamate: glyoxylate aminotransferase. Plant Mol. Biol. 64, 205–217. doi: 10.1007/s11103-007-9145-z
Wang A., Ma C., Ma H., Qiu Z., Wen X. (2021). Physiological and proteomic responses of pitaya to PEG-induced drought stress. Agric. (Switzerland) 11. doi: 10.3390/agriculture11070632
Wang C., Zhou L., Zhang G., Xu Y., Gao X., Jiang N., et al. (2018). Effects of drought stress simulated by polyethylene glycol on seed germination, root and seedling growth, and seedling antioxidant characteristics in job ‘ s tears. Agricultural sciences 09, 991–1006. doi: 10.4236/as.2018.98069
Warwick B., Rightford B. (2020). Exploring hemp farming as a sustainable agriculture in South Africa, using a social- ecological systems approach. (Doctoral dissertation, Stellenbosch: Stellenbosch University). http://hdl.handle.net/10019.1/108267
Xiong W., Cui T., Cheng K., Yang F., Chen S., Willenbring D., et al. (2012). Cannabinoids suppress inflammatory and neuropathic pain by targeting α3 glycine receptors. The Journal of Experimental Medicine 209 (6), 1121–1134. doi: 10.1084/jem.20120242
Zheljazkov V. D., Sikora V., Noller J., Latkovi D., Ocamb C. M., Koren A. (2023). Industrial hemp ( Cannabis sativa L.) agronomy and utilization : A review. Agronomy 13 (3), 931. doi: 10.3390/agronomy13030931
Keywords: chlorophyll fluorescence, drought stress, industrial hemp, neglected/underutilized species, photosynthesis, proline accumulation
Citation: Mateva KI, Mahenga E, Matibiri T, Kamutando CN, Magama F and Dimbi S (2024) Genotype-specific morphophysiological adaptations and proline accumulation uncover drought adaptation complexity in hemp (Cannabis sativa and Cannabis indica). Front. Plant Physiol. 2:1441262. doi: 10.3389/fphgy.2024.1441262
Received: 30 May 2024; Accepted: 22 July 2024;
Published: 09 September 2024.
Edited by:
Giles Nicholas Johnson, The University of Manchester, United KingdomReviewed by:
Sorin Vatca, University of Agricultural Sciences and Veterinary Medicine of Cluj-Napoca, RomaniaOded Liran, University of Haifa, Israel
Copyright © 2024 Mateva, Mahenga, Matibiri, Kamutando, Magama and Dimbi. This is an open-access article distributed under the terms of the Creative Commons Attribution License (CC BY). The use, distribution or reproduction in other forums is permitted, provided the original author(s) and the copyright owner(s) are credited and that the original publication in this journal is cited, in accordance with accepted academic practice. No use, distribution or reproduction is permitted which does not comply with these terms.
*Correspondence: Kumbirai Ivyne Mateva, a21hdGV2YUBrdXRzYWdhLmNvLnp3