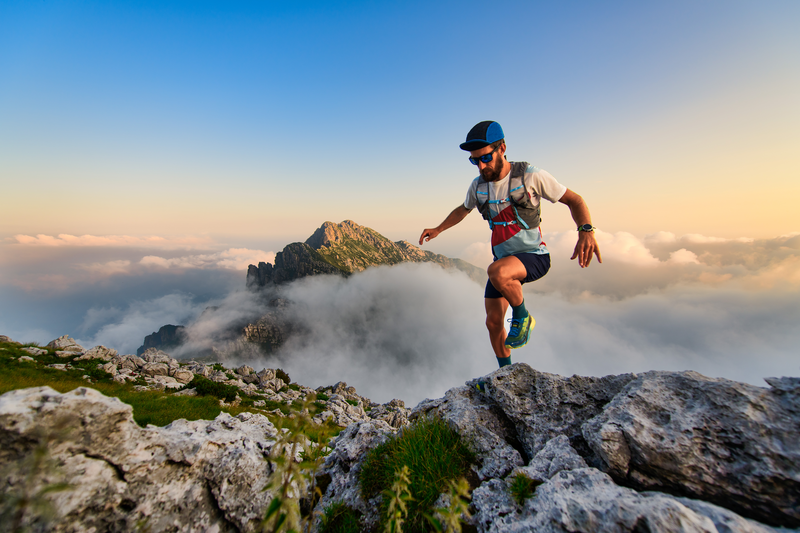
94% of researchers rate our articles as excellent or good
Learn more about the work of our research integrity team to safeguard the quality of each article we publish.
Find out more
ORIGINAL RESEARCH article
Front. Plant Physiol. , 01 February 2024
Sec. Photosynthesis and Metabolism
Volume 2 - 2024 | https://doi.org/10.3389/fphgy.2024.1331421
The combination of low precipitation and high temperature stresses at jointing stage can severely threaten maize production. However, to date, few studies have been conducted on the effects of combined stress on maize plants expression at jointing stage. In the current research, plant growth, root morphology, and yield components were determined after exposure to the single and combined stress of high temperature and drought stress. Leaf gas exchange, malondialdehyde (MDA) content and antioxidant enzymes activities were conducted to identify potential mechanisms of stress responses. The single stress of high temperature and drought significantly reduced the biomass of various organs and the total aboveground biomass, which reduced the yield of maize plants. High temperature substantially decreased aboveground biomass and yield under mild and severe water stress, which indicated that the inhibitory effects of combined stress were more significant than that of high temperature or drought individually. High temperature exacerbated the negative impacts of water stress on plants growth and yield as shown by the reduced leaf photosynthetic rate (Pn), probably related to the increasing MDA content. Leaf-level water use efficiency (WUE) was enhanced as the reduction in leaf transpiration (Tr) was greater than the decrease in leaf photosynthesis under high temperature, even for those plants were suffering water stress. High temperature, drought stress and their combination all greatly increased the activities of superoxide dismutase (SOD), peroxidase (POD), and catalase (CAT), but were ineffective in mitigating oxidative damage. The MDA content and antioxidant enzymes activities showed an increasing trend following 12 days of combined stress. This substantiated the irreversible damage induced by combination of high temperature and desiccation stresses. The combined stress optimized roots length, root volume, root surface area, and thinned the average root diameter, which improved the adaptation of maize to high temperature, drought and combined stress. This study has provided meaningful references for improved understanding the impacts of drought, high temperature, and concurrent events on the physiology and growth of maize plants during the jointing period.
In recent years, the gradual advancement of industrialization and globalization has inevitably resulted in anthropogenic emissions of greenhouse gases (Gultekin et al., 2023), thereby contributing to global warming (Kweku et al., 2018). Globally averaged surface temperatures have risen during 2011 to 2020 by 1.1°C, higher than those observed from 1850 to 1900. If temperatures continue to rise according to this trend, it is estimated that the temperature increases between 2021 and 2040 will reach 1.5°C (IPCC, 2022). The continued rise in global average temperature intensifies evaporation of surface water, changing precipitation patterns, increasing the frequency and duration of meteorological disasters, such as floods and droughts (Ben Ameur et al., 2022). Drought events adversely restrict normal crop growth and development, resulting in yield reduction or harvest loss, putting considerable pressure on global food security and sustainable economic and social development (Islam et al., 2022). Overall, water scarcity from high temperatures has a substantial negative influence on agricultural production, the ecological environment, aquatic resources, and socioeconomic systems (Zhang et al., 2023). Therefore, insight into the underlying mechanisms that control the effects of drought and high temperature on agricultural primary production and global grain yield will aid in maintaining agricultural ecosystem stability and formulating targeted management strategies under climate change scenarios (Chen et al., 2023).
As one of the primary abiotic stresses, drought stress restricts the development and productivity of crops in arid and semi-arid regions by altering stomatal closure, causing injury to the photosystem, and facilitating chlorophyll biodegradation that affects the plant’s pathology and biochemistry in a direct way (Alam et al., 2021). The stomatal closing induced by drought stress usually leads to oxidative stress, resulting in a rapid decline in the internal CO2/O2 ratio (Park et al., 2021). This causes excessive reduction in the photosynthetic electron chains and increased formation of reactive oxygen species (ROS) in chloroplasts and mitochondria (Sachdev et al., 2021). Excess ROS cause oxidative damage, which leads to the increase of membrane lipid hydro peroxidation, malondialdehyde (MDA) levels and additional biological macromolecules, eventually leading to cell loss (Zafari et al., 2020). The production of superoxide free radical in chloroplasts is mainly associated with the degradation of photosynthetic pigments. Therefore, the photosynthetic electron transfer system is destroyed, and the functions of photosynthetic organs are damaged, which eventually lead to the decrease of photosynthetic rate (Chen et al., 2022). To avoid or mitigate oxidative injury, plants developed various sophisticated protection strategies containing effective antioxidative enzymes like superoxide dismutase (SOD), peroxidase (POD), and catalase (CAT), all working in coordination to counteract and scavenge the overproduction of cytotoxic ROS (Han et al., 2022). In addition, reduced leaf photosynthetic rate may inhibit the transport of photosynthetic products to reproductive organs (Sarker et al., 2020), and meanwhile reduce aboveground biomass by increasing assimilate partitioning toward the root system (Avila et al., 2020). This may lead to substantial grain yield reductions (Li et al., 2022; Liu et al., 2022).
High temperature is a limiting factor affecting different development stages of plants, inducing oxidative damage, and restricting the growth, development and productivity of important agricultural crops (Sachdev et al., 2021). High temperature could negatively impact essential physiological processes (e.g., photosynthesis, respiration, and moisture metabolism) of crops (Siddiqui et al., 2022). Extensive research over recent decades has revealed that high temperature stress affects photosynthetic organelles, chlorophyll biosynthesis, CO2 assimilation, chloroplast ultrastructure, electron transport, and photochemical reactions (Chen et al., 2022; Viljevac Vuletic et al., 2022). Specifically, ribulose diphosphate carboxylase/oxygenase (Rubisco) and carbon assimilation rates are considered to confine photosynthesis at high temperatures (Ullah et al., 2022). The photosynthetic system is unable to efficiently convert light energy into chemical energy and fix carbon, leading to the build-up of intracellular ROS (Zhao et al., 2018). Rapid and excessive generation of ROS can impair photosynthesis by lowering the electron transport rate (Iqbal et al., 2021). Similarly, to prevent cellular damage and restore redox homeostasis, plants can trigger high-temperature reactions by hyperactivating enzymatic ROS-scavenging systems (Foyer, 2018). Furthermore, high temperature undermine the function of leaves and lead to stress-induced premature senescence (Rossi et al., 2020). This accelerates senescence and the grain-filling process of the whole plant, which then leads to earlier maturity and shorter reproductive period, eventually resulting in a prominent yield loss (Xiong et al., 2017).
The root system is an important organ for obtaining water and nutrients, adapting to the environment, and achieving high yields. The structural characteristics of the roots are essential to the efficient utilization of moisture and nutrients in the rhizosphere (Xu et al., 2021). The morphologic features of the root system, consisting of root length, surface area, volume, and diameter, are associated with water uptake and aboveground biomass production (Ober et al., 2021). Longer root systems allow plants to obtain water from deeper soil profiles, which is related to the drought tolerance of crops (Prince et al., 2015). It is reported that nutrients and water absorbed from the soil are directly correlated with the surface area of root system in contact with soil. Thus, root surface area is associated positively with yield under drought stress, while root diameter is related to the capacity to penetrate through hard soils, thereby affecting drought tolerance of crops (Xu et al., 2021). High temperature can induce adjustments in C allocation in different plant parts and regulate root morphological traits (Delamare et al., 2023). Raising the ambient temperature is understood to also raise the soil temperature in the root zone of the crop. Therefore, the influence of soil temperature exceeding the optimum value may be harmful to root growth, ultimately resulting in a decline in yield (Chakraborty et al., 2022).
Summer maize (Zea mays L.) is a major cereal grain crop mainly used for animal feed, biofuels and human consumption (FAO, 2022). Maize is very sensitive to high temperature and drought stress (Cohen et al., 2021; Li et al., 2022). Drought and high temperature trigger a range of stressed reactions or phenotypic alterations of maize plants, which can be synergistic or antagonistic to physiology and plant performance (Bheemanahalli et al., 2022). Negative impacts of combined stress on net assimilation, decreased Photosystem II activity coupled with impaired electron transport rate and increased ROS accumulation have been highlighted (Naing and Kim, 2021). Furthermore, individual and combined stress have different effects on physiological processes such as stomatal conductance (Cohen et al., 2021). It is well known that excessive leaf temperature can damage and ultimately kill leaves (Krause et al., 2010). In the short term, transpirational cooling can be an effective means to reduce this risk. However, this requires a lot of water, which is potentially risky for plants under drought conditions (Feng et al., 2023). Despite the adverse impacts of combined drought stress and high temperature on maize yield, few investigations have examined the influences of combined stress on maize physiology and productivity at the jointing stage (Hussain et al., 2019). Even less is known regarding maize root morphology under compound stress (Ru et al., 2022). Therefore, the present research was conducted with a focus on four important aspects: (i) examine the combined effects of high temperature and drought stress on the aboveground growth and grain yield; (ii) analyze maize leaf physiological characteristics in response to compound stress; (iii) elucidate how combined stress influence maize root morphology; and (iv) explore the potential acclimation mechanisms of maize plants to high temperature, drought, and compound stress.
The study took place in a synthetic growth chamber (RQS-15; Hengyu Instrument and Equipment Manufacturing Co. Ltd. Nanjing, China) at the Key Laboratory of Agricultural Soil and Water Engineering (108.04°E, 34.17°N; 506 m), Northwest A&F University, Yangling, Shaanxi Province of Northwest China. The experimental material was maize hybrid (Zea mays L. cv. Zhengdan 958). The plastic pots of 15.0 dm3 in value (27.5 cm in height with a top area of 707 cm2 and a bottom area of 397 cm2) with a tiny hole at the bottom were used. The soil was gathered from 0-40 cm deep soil layers in the local experimental field, air-dried, and passed through a 6-mm sieve. Each pot contained 18 kg of screened dry soil, with a field capacity of 27.02% (FC, g/g) and a soil bulk density of 1.35 g/cm3. The soil pH (1:5 soil/water ratio), electrical conductivity (1:5 soil/water ratio), soil NH4+-N, soil NO3–N, exchangeable soil potassium, exchangeable soil phosphorus and organic matter of cultivated soil layer were 8.1, 0.18 ds m-1, 6.9 mg kg-1, 10.1 mg kg-1, 164.5 mg kg-1, 20.5 mg kg-1, and 12.3 g kg-1, respectively. The surface soil of each pot was covered with perlite to minimize soil evaporation and alleviate the impact of irrigation, and the bottom was lined with small stones and fine sand as a filter layer.
Prior to sowing, all the pots were applied with Stanley controlled-release fertilizers (4 g) (18-18-18-N-P-K). On June 15, 2020, three seeds were planted in each pot at a depth of 3-4 cm. After emergence, healthy and uniformly growing maize seedlings were retained by thinning out seedlings, with one seedling per pot at the three-leaf stage. Fifteen pots were randomly set up for each treatment, and the 15 pots were the biological replications in the present study (n = 15). Before the stress treatment, all the maize plants were established in the rain shelter. During jointing stage, all maize pots were moved into an artificial growth chamber on July 25, and then subjected to individual or compound stress of high temperature and drought.
The experiment was conducted using a split-plot design that consisted of two factors (temperature and watering) with temperature as the main plot (two temperature levels) and watering as the subplot with three watering levels, which were full irrigation (F, 70–80%FC), mild water stress (M, 55–65%FC), and severe water stress (S, 40–50%FC). According to the meteorological data of Guanzhong Plain over the past 30 years (China Meteorological Data Service Center, CMDC; http://data.cma.cn/en), the average high temperature near the maize jointing stage has been approximately 35°C, with a relatively stable duration of about 12 days (Figure 1). As a result, the temperature of the high-temperature growth chamber was adjusted to high temperature (H, 36°C/27°C, day/night), and the temperature of the controlled growth chamber was regulated to the control temperature (C, 29°C/20°C). Apart from air temperature, other environmental factors in the growth chambers were maintained at similar levels, including relative humidity (60–70%), photosynthetic photon flux density (1200 μmol m−2 s−1), CO2 concentration (400 μmol mo1−1), and photoperiod (6:00–20:00). In this study, the soil water content was determined by weighting pots and irrigation water was applied to the upper limit whenever the soil moisture approached or fell below the lower limit. After 12 days of stress, all maize pots were moved back to the rain shelter to restore temperature (29°C/20°C) for heat-stressed plants, soil moisture (70-80% FC) for drought-stressed plants, and temperature + soil moisture for combined-stressed plants. Weeds, pests, and diseases were well controlled during the crop growing seasons. Specifically, regular herbicide and insecticide treatments by foliar sprays by foliar sprays can be used to control weeds and vectors, which will help reduce the incidence and severity of disease and pets (Zhan et al., 2022). The specific maize growth and treatment processes is displayed in Figure 2.
Figure 1 Extreme maximum temperature and duration during summer maize jointing in the main production areas on the Guanzhong Plain in China from 1990 to 2020.
Figure 2 The growth processes of maize plants, and the stage exposed to drought, high temperature and combined drought and high temperature as well as the natural condition for each treatment. FC, field capacity. Growth stage definitions: VE, emergence; V6, six leaf; VT, tasseling; R3, milk stage; R6, black layer, physiological maturity.
At V6 (six leaf), VT (tasseling), R3 (milk) and R6 (black layer, physiological maturity) stages of maize, three plants were randomly selected from each treatment and manually cut at ground level. The aboveground parts of the plants were divided into leaves, stems and spikes using scissors, then were oven-dried in paper bags at 75°C until a stable weight was obtained. The plant biomass was then obtained by weighing the plant tissues with electric scales. Three independent replicates were used to determine the aboveground biomass.
The aboveground portion was separated from the belowground portion using a sharp scissor at R6 stage, and the root system as a whole was removed from the pot, and then lightly shook to clear most of the soil attached to roots. The root with soil was placed on a 0.037 mm nylon mesh, and then soaked in water for 20 min to facilitate the separation of the root system from rhizosphere soil. The soil around the roots was thoroughly rinsed with mild deionized water flow, followed by the collection of the roots. The root images were scanned using a high-resolution EPSON V800 scanner. The root analysis system WinRHIZO (Version 2009c; Regent Instruments Inc.) was used to analyze the scanned images to determine root morphology parameters, including root length, root surface area, root volume and average root diameter.
After 12 days of stress, leaf gas exchange was determined using the last completely expanded leaf from one pot, and the measurements were replicated five times on the five pots as five replicates. The selected leaf in a 2 cm × 3 cm cuvette chamber was attached to a portable photosynthesis system (LI-6400XT; LI-COR, Lincoln, NE, USA). Then leaf gas exchange measurements were conducted from 9:00-12:00 to determine the net photosynthesis rate (Pn), stomatal conductance (Gs), and transpiration rate (Tr) at the temperature of 25°C, the saturated light of 1200 μmol photons m−2 s−1 (PPFD), the CO2 concentration of 400 μmol mol−1, and the constant flow rate of 500 ml min-1 for maize plants. The ratio of leaf photosynthesis to transpiration was defined as leaf-level water use efficiency. The cuvette was sealed with plasticine to prevent leakage, and all of the measurements were conducted with VPD lower than 1.5 KPa to eliminate moisture limitation on leaf gas exchange.
Three uniform maize plants were chosen from each treatment group, and the leaves from the labeled plants were measured three times: 3 days (during stress, DAS 3) and 12 days (the end of stress, DAS 12) after individual or compound stress, as well as at the late jointing stage (recovery of control temperature and soil moisture for 7 days, RTM 7). The collected leaves were instantly chilled in liquid nitrogen, then quickly transferred and stored at -80°C for subsequent analyses. According to Du and Bramlage (1992), the degree of lipid peroxidation was determined by measuring the content of malondialdehyde (MDA) in 1.0 g of fresh leaf samples at 532 and 600 nm.
Crude enzymes were extracted employing 50 mmol phosphate buffer (pH 7.8, 5 mmol l−1 MgCl2, 1 mmol l−1 EDTA-2Na, 1% polyvinylpyrrolidone, and 0.3% Triton-X100). Fresh leaf samples (0.5 g) were grounded in a frozen mortar using precooled extraction medium. The homogenates were concentrated by centrifugation at 12000 rpm and 4°C for 20 min and the filtrate was prepared as enzymatic extracts for the determination of dismutase (SOD), peroxidase (POD) and catalase (CAT). As shown by Beauchamp and Fridovich (1971); Zhang (1992), and Aebi (1984), SOD (EC 1.15.1.1), POD (EC 1.11.1.7), and CAT (EC 1.11.1.6) activities were assayed, respectively.
The ear length and ear diameter of maize plants were determined with a tape measure and vernier caliper at R6 stage, respectively. The maize ears were air-dried in the greenhouse conditions for 7 days, and then manually threshed to obtain yield components, recording ear kernel weight and 100-kernel weight. The dry grain was measured and converted to a standard moisture content of 14%. In cereal crops such as maize and rice, harvest index is the quotient of grain yield and total aboveground biomass, viz., harvest index = grain yield/total aboveground biomass (Cui et al., 2000; Fageria, 2007).
Statistical analyses were conducted using two-way analysis of variance (ANOVA) to investigate the main and interactive effects of high temperature and drought stress on aboveground biomass, leaf gas exchanges, antioxidant enzymes activities, root morphological parameters, and grain yield components with General Linear Model Full Factorial Mode. Also, one-way ANOVA was used to investigate the significant differences on aboveground biomass. The statistical analyses all followed by Duncan’s multiple range tests (P < 0.05) by SPSS 26.0 software (IBM, Chicago, IL, USA). The within-group differences between different treatments were clarified using least significant difference tests (LSD). In addition, redundancy analysis (RDA) was conducted to assess the responses of the total aboveground biomass, leaf gas exchange, antioxidant enzymes activities, and ear kernel weight to temperature and watering by Canoco 5.0. In the RDA, the traits were standardized response variables, and high-temperature and drought treatments were explanatory variables. Origin 2023b (OriginLab, USA) and Canoco 5.0 (Microcomputer Power, Ithaca, NY) were employed to construct the figures. Data were expressed as mean ± standard error.
The various organ biomass and total aboveground biomass of summer maize were significantly reduced by high temperature, drought stress, and the combined stress at any growth stage (P < 0.01; Table 1). Specifically, the stem, leaf, and ear dry weight as well as total aboveground biomass all dramatically declined with the increase of temperature and drought severity. In particular, individual high temperature or drought stress prominently reduced the leaf dry weight (P < 0.001; Table 1), while the combined stress had no significant effect on the leaf dry weight (P > 0.05; Table 1). Among these, the CS treatment decreased the total aboveground biomass by 30.2%, 29.4%, 40.4%, and 31.4%, respectively, compared with that of the CF treatment at V6, VT, R3, and R6 stages (P < 0.05; Figure 3A). Meanwhile, the HF treatment reduced the total aboveground biomass by 47.6%, 51.3%, 48.1%, and 40.7% compared with that of the CF treatment, respectively (P < 0.05). Furthermore, the combined stress (the HS treatment) at any stage reduced the total aboveground biomass by the largest magnitude, which was decreased by 58.5%, 60.8%, 62.1%, and 59.9% (in the order of V6, VT, R3, and R6 stages), respectively, compared with the CF treatment (P < 0.05; Figure 3). In addition, the stem, leaf, and single ear dry weight of maize plants subjected to high temperature or drought stresses applied as single-stress factor displayed a similarly pattern with the total aboveground biomass (P < 0.05; Figures 3B–D). Across the experiment here, both individual high temperature and drought stress generally caused a decrease in the biomass of different organs, and the impact was magnified by the combination of high temperature and drought stress. Overall, drought had the least impact on decreasing the total aboveground biomass of maize plants compared to high temperature or drought and high temperature combination (with drought stress and high temperature combination having the maximal effect). In other words, the combined stress of high temperature and drought reduced the total aboveground biomass more significantly than the single stresses.
Table 1 Interactive effects of temperature and watering on the dry weight of different organs and total aboveground biomass of maize plants at different phenological stages.
Figure 3 Effects of drought stress on the total aboveground biomass (A), stem dry weight (B), leaf dry weight (C), and single ear dry weight (D) of maize plants under different temperature conditions at different phenological stages. Lowercase letters indicate significant differences between six treatments with three watering levels and two temperature levels at P < 0.05. Greek letters indicate significant differences between different temperatures under same soil water conditions at P < 0.05. Uppercase letters indicate significant differences between different growth stages at P < 0.05. CF: control temperature and full irrigation; CM: control temperature and mild water stress; CS: control temperature and severe water stress; HF: high temperature and full irrigation; HM: high temperature and mild water stress; HS: high temperature and severe water stress. Growth stage definitions: V6, six leaf; VT, tasseling; R3, milk stage; R6, black layer, physiological maturity.
High temperature, drought, and their combined stress significantly affected the leaf gas exchange parameters of maize plants (P < 0.05; Figure 4). In detail, the net photosynthetic rates (Pn), stomatal conductance (Gs), and transpiration rate (Tr) all substantially declined with the increment of temperature and drought severity (P < 0.01). The CS treatment prominently decreased the Pn, Gs, and Tr by 36.1%, 49.3%, and 25.8% compared with that of the CF treatment, respectively (P < 0.01). However, under control temperature conditions, the water use efficiency (WUE) first increased and then decreased along with the soil water gradient (P < 0.05), and a sightly c.11.8% lower WUE under the CS treatment compared with that under the CF treatment, which was primarily attributed to the fact that the Tr reduced less than Pn of maize plants subjected to control temperature conditions under severe water stress (i.e., 25.8% < 36.1%). Moreover, the HF treatment significantly decreased the Pn, Gs, Tr, and WUE by 39.8%, 33.3%, 18.8%, and 24.4%, respectively, compared with that of the CF treatment (P < 0.01). Furthermore, the combined stress of drought and high temperature (the HS treatment) dramatically reduced the Pn, Gs, and Tr by 57.1%, 67.3%, and 43.7% compared with that of the CF treatment, respectively (P < 0.01). Particularly, the WUE tended to increase under high temperature conditions with the decline of soil water status, so the HM and HS treatment reduced the WUE by 22.8% and 19.1%, respectively, compared with that of the CF treatment (P < 0.01), which indicated that water stress indeed mitigated the adverse effects of high temperature on the WUE to a certain extent. Interestingly, the Pn of maize plants subjected to the CS treatment or the HF treatment were similar, whereas the Pn of plants subjected to the HS treatment was significantly lower than that of plants subjected to the CS or HF treatment (P < 0.05). In addition, high temperature had the least adverse effects on decreasing the Gs and Tr of maize plants compared to drought or high temperature and drought combination (with high temperature and drought combination having the maximal effect) (P < 0.05). These results suggested that the limitation in CO2 availability resulted from the closure of stomata (decreased stomatal conductance) may cause a further reduction in the Pn in plants subjected to the stress combination (P < 0.05; Figure 4).
Figure 4 Effects of high temperature on the net photosynthetic rates (A), stomatal conductance (B), transpiration rates (C), and water use efficiency (D) of maize plants along a soil water gradient. CF: control temperature and full irrigation; CM: control temperature and mild water stress; CS: control temperature and severe water stress; HF: high temperature and full irrigation; HM: high temperature and mild water stress; HS: high temperature and severe water stress. Uppercase letters indicate significant differences between different watering levels under same temperature conditions at P =0.05 level.* indicates significant differences between different temperatures at P =0.05 level under same soil moisture conditions; ** indicates significant differences between different temperatures at P = 0.01 level under same soil moisture conditions.
High temperature or drought stress alone significantly promoted the malondialdehyde (MDA) synthesis during the stress period (P < 0.01; Figure 5A), and the combined stress dramatically affected the MDA content on DAS 12 and RTM 7 (P < 0.01), which indicated that the combination of high temperature and drought stress had a delayed effect on MDA synthesis. In detail, the MDA content prominently enhanced with the increase of temperature and drought severity (P < 0.01). Among these, the MDA content of control maize plants (the CF treatment) remained essentially unchanged during the stress and recovery periods, which suggested the aging process made no difference on MDA content. Moreover, the CS treatment substantially increased the MDA content by 54.5% and 65.2% on DAS 3 and DAS 12 compared with that of the CF treatment, respectively (P < 0.001). Meanwhile, the HF treatment significantly raised the MDA content by 40.9% and 56.5% on DAS 3 and DAS 12, respectively, compared with that of the CF treatment (P < 0.01). Furthermore, the HM and HS treatment dramatically enhanced the MDA content by 63.6% and 81.8% on DAS 3, as well as 82.6% and 39.1% on DAS 12 compared with that of the CF treatment, respectively. Overall, the MDA content of different treatments generally increased during the stress period, whereas the MDA content of the HS treatment significantly decreased by 20% compared with that on DAS 3. It can be seen that high temperature prominently weakened the synthesis ability of MDA under severe water stress, where the MDA content declined by 15.8% (P < 0.01). Remarkably, high temperature had the least impact on promoting MDA synthesis of maize plants compared to drought or high temperature and drought combination (with the combined stress having the maximal effect). In addition, the MDA content of all the treatments indicated significant decreases on RTM 7, which were lower than those on DAS 3. Following 7 days of relief from high temperature and drought stress, the MDA content of the CM, CS, HF, HM, and HS treatments were 1.05-, 1.24-, 1.14-, 1.34-, and 1.10-fold greater than that of the CF treatment, respectively.
Figure 5 Effects of drought stress on the MDA content (A), SOD activity (B), POD activity (C), and CAT activity (D) of maize plants under different temperature conditions. DAS 3, 3 days after stress; DAS 12, 12 days after stress; RTM 7, recovery of control temperature and soil moisture for 7 days. CF, control temperature and full irrigation; CM, control temperature and mild water stress; CS, control temperature and severe water stress; HF, high temperature and full irrigation; HM, high temperature and mild water stress; HS, high temperature and severe water stress. T, temperature; W, watering. * indicates significant differences between treatments at P =0.05 level; ** indicates significant differences between treatments at P = 0.01 level; *** indicates significant differences between treatments at P = 0.001 level.
High temperature or drought stress applied individually significantly improved the activities of superoxide dismutase (SOD), peroxidase (POD), and catalase (CAT) during the stress period (P < 0.01; Figures 5B–D), and the stress combination substantially affected the activities of antioxidant enzymes on DAS 12 and RTM 7 (P < 0.05). In particular, the effects of high temperature or drought alone on CAT activities were detected on RTM 7 (P < 0.05), while the CAT activities were strongly modified by the stress combination on DAS 3 (P < 0.05). All these indicated that the activities of SOD and POD had delayed responses to the high temperature and drought combination, whereas CAT activities were more sensitive to the combined stress. In detail, the activities of SOD, POD, and CAT markedly improved with the elevation of temperature and drought severity during the stress period (P < 0.01). Among these, the antioxidant enzymes activities in the control treatments displayed such minor fluctuations during the stress and recovery periods that they can be deemed negligible. Moreover, the CS treatment markedly increased the activities of the SOD, POD, and CAT by 20%, 27.3%, and 30.8% on DAS 3, as well as 31.7%, 55.5%, and 69.1% on DAS 12 compared with that of the CF treatment, respectively (P < 0.01). Meanwhile, the HF treatment significantly increased the SOD, POD, and CAT activities by 15.0%, 37.1%, and 78.7% on DAS 3, and 26.8%, 60.7% and 89.4% on DAS 12, respectively, compared with that of the CF treatment (P < 0.001). Furthermore, the HM treatment significantly increased the SOD, POD, and CAT activities by 25.0%, 55.1%, and 67.2% on DAS 3, and 39.0%, 77.4%, and 105.1% on DAS 12 compared with that of the CF treatment, respectively. The HS treatment substantially raised the SOD, POD, and CAT activities by 40.2%, 68.3%, and 98.6% on DAS 3, as well as 22.0%, 80.0%, and 79.2% on DAS 12, respectively, compared with that of the CF treatment. The aforementioned results revealed that the SOD and CAT activities generally increased across the different treatments as the duration of the stress increased during the stress period, whereas SOD and CAT activities in the HS treatments were significantly reduced by 10.8% and 7.9% compared to DAS 3, respectively. It can be seen that severe water stress prominently decreased the SOD and CAT activities under high temperature conditions, where SOD and CAT activities dropped by 12.3% and 12.6%, respectively. In general, high temperature had the least impact on increasing the SOD activity compared to drought or high temperature and drought combination (with stress combination having the maximal effect). By contrast, drought stress had the least impact on increasing the POD and CAT activities compared to high temperature or drought and high temperature combination (with the combined stress having the maximal effect). That is to say that comparing the impact of high temperature and drought stress combination to that of high temperature or drought stresses applied individually, revealed that the combination of high temperature and drought stress had a more severe impact on antioxidant enzymes activities compared to high temperature or drought alone. In addition, the SOD, POD, and CAT activities of the different treatments substantially decreased on RTM 7, which were lower than those on DAS 3. However, the SOD, POD, and CAT of maize plants subjected to high temperature or drought stresses applied as single-stress factor or the stress combination displayed higher activities than those of the control treatment.
High temperature, drought, and their combined stress significantly affected the root morphology parameters of summer maize (P < 0.01; Figure 6). Specifically, the root length (RL), root volume (RV), and root surface area (RSA) significantly enhanced with the intensification of water stress, whereas the average root diameter (ARD) was significantly reduced (P < 0.001). Consistently, exposure to high temperature conditions demonstrated inhibitory effects on the RL (P < 0.01), ARD (P < 0.01), RV (P < 0.001), and RSA (P < 0.001), which indicated that there were significant differences in the response of root morphology to temperatures regardless of soil water status. The CS treatment dramatically increased the RL, RV, and RSA by 36.8%, 32.0%, and 40.6%, respectively, while decreased the ARD by 12.9% compared with that of the CF treatment (P < 0.001). Meanwhile, the HF treatment prominently decreased the RL, ARD, RV, and RSA by 36.8%, 5.3%, 53.4%, and 47.2% compared with that of the CF treatment, respectively (P < 0.01). Moreover, under high temperature conditions, the root system development of maize plants was much more susceptible to water stress. Taking the RL as an example, the CS and HS treatments increased by 36.8% and 88.6% compared with the CF and HF treatments, respectively (P < 0.001). As the degree of water stress intensified, the inhibitory effects of high temperature on root morphological parameters diminished. For instance, high temperature substantially reduced the RL by 36.8%, 27.7%, and 12.9% under full irrigation, mild water stress, and severe water stress, respectively (P < 0.001). Furthermore, the HS treatment significantly decreased the ARD by 15.6% compared with that of the CF treatment (P < 0.001). It can be seen that high temperature had the least impact on reducing the ARD of maize plants compared to drought or high temperature and drought combination (with temperature and drought combination having the maximal effect). That is to say the combined stress of drought and high temperature reduced the ARD more significantly than the single stresses.
Figure 6 Effects of high temperature on the root length (A), average root diameter (B), root volume (C), and root surface area (D) of maize plants along the soil water gradient. Full irrigation: 70%-80%FC; Mild water stress: 55%-65%FC; Severe water stress: 40%-50%FC. FC, field capacity; T, temperature; W, watering.
High temperature, drought, and their combined stress significantly decreased the yield components of summer maize (P < 0.001; Table 2). In detail, the ear weight (EW), 100-grain weight (GW), ear length (EL), ear diameter (ED), and harvest index (HI) all dramatically decreased with the intensification of temperature and drought severity (P < 0.001). Compared to controls, maize plants subjected to high temperature or drought stresses applied as single-stress factor displayed a 40.3% and 34.3% EW reduction, respectively (P < 0.001), while the average EW reduction in response to a combination of high temperature and drought stress was 58.1% (P < 0.001). This impact of stress combination on EW could manifest itself in a number of different ways. We therefore compared the impact of stress combination on different yield components to that of each of the stresses applied individually.
Table 2 The effects of drought stress on the yield components of maize plants under different temperature conditions and the interactive effects of temperature and watering on the yield components.
HI is a key indicator of biomass partitioning between vegetative and reproductive organs in crop plants. It is defined as the total weight of grain divided by the total weight of aboveground biomass. As highlighted in Table 2, when maize plants were exposed to high temperature or drought stress, the HI decreased by 24.2% and 18.2%, respectively (P < 0.001). Interestingly, the combination of high temperature and drought stress further impacted HI and reduced it by 39.4% (P < 0.001). This result suggested that high temperature and drought stress combination may shorten the life cycle of maize plants and drive them to produce seeds earlier, and mostly likely result in reducing numbers and/or size, with less vegetative tissues produced in the process. Indeed, as shown in Table 2, the combination of high temperature and drought stress decreased the GW, EL, and ED of maize plants by an average of 44.7%, 58.2%, 41.4%, respectively (P < 0.001). Intriguingly, the impacts of drought on the GW, EL, and ED (the reductions of 21.2%, 21.9%, and 26.2%) were different than that of high temperature (the reductions of 29.7%, 31.1%, and 30.9%) revealing that drought had the least impact on decreasing the GW, EL, and ED compared to high temperature or drought and high temperature combination (with drought and high temperature combination having the maximal effect). In conclusion, comparing the impact of high temperature and drought stress combination to that of high temperature or drought stress applied individually for maize plants, revealed that the combined stress had a more severe impact on yield components compared to high temperature or drought alone. Remarkably, the combined stress acted more similarly to the high temperature on the yield components (Table 2).
To investigate the relationships among growth, physiological and biochemical characteristics as well as maize yield, we analyzed the total aboveground biomass, leaf gas exchange, MDA content, antioxidant enzymes activities, root morphology, and yield components by using Redundancy and Pearson correlation analyses (Figures 7, 8). The Redundancy Analysis (RDA) for the effects of temperature and watering on the traits of maize plants is shown in Figure 7. The temperature and watering were considered as explanatory variables, while MDA content, antioxidant enzymes activities, leaf gas exchange, total aboveground biomass and maize yield were response variables. The first and second axes interpreted 49.11% and 48.41% of the maize plants traits, respectively. The projection length of the ray in which the response variables were located on both axes and the arrow direction illustrated the correlation between the explanatory variables and response variables. RDA 1 corresponded to leaf gas exchange, MDA content, antioxidant enzymes activities, total aboveground biomass, and maize yield, which could account for the majority of the variations within the dataset. This indicated that antioxidant enzymes contributed more significantly to the development of heat tolerance in maize plants, whereas high temperature suppressed Pn, Tr, Gs and WUE, thus resulting in the decreased total aboveground biomass and yield of maize plants. RDA 2 predominantly corresponded to leaf gas exchange and maize yield, which indicated that water stress decreased the Pn, Tr, and Gs, but enhanced WUE of maize plants, ultimately leading to yield reductions. The magnitude of the angles between the rays represents the correlation between the variables. Leaf gas exchange had positive effects on the total aboveground biomass and maize yield. On the contrary, MDA was detrimental to the production of total aboveground biomass and maize yield (Figures 7, 8). Furthermore, strongly positive correlations were observed between leaf gas exchange and root morphology parameters, as well as between MDA content and antioxidant enzymes activities (Figure 8). MDA and SOD showed weakly positive correlations with ARD, whereas POD and CAT were weakly negatively correlated with RL, RV, and RSA. Strong linear relationships were observed between root morphological parameters and total aboveground biomass as well as yield. In addition, significantly positive relationship was detected between total aboveground biomass and maize yield (Figure 8).
Figure 7 Redundancy analysis for high temperature or drought stress on maize traits. SOD, superoxide dismutase; CAT, catalase; POD, guaiacol peroxidase; MDA, malondialdehyde; Pn, net photosynthetic rate; Tr, leaf transpiration rate; Gs, stomatal conductance; WUE, water use efficiency; TBM, total aboveground biomass; EW, ear grain weight.
Figure 8 Relationships among growth, physiological and biochemical characteristics as well as yield components of maize plants. Pn, net photosynthetic rate; Tr, leaf transpiration rate; Gs, stomatal conductance; WUE, water use efficiency; SOD, superoxide dismutase; CAT, catalase; POD, guaiacol peroxidase; MDA, malondialdehyde; RL, root length; ARD, average root diameter; RV, root volume; RSA, root surface area; TBM, total aboveground biomass; EW, ear grain weight. The numbers represent the Pearson correlation coefficients between the parameters. The circles represent the degree of correlation between the parameters, with wider circles representing lower correlations and narrower circles representing higher correlations. Red represents positive correlations and blue represents negative correlations. Darker colors represent higher correlations and lighter colors represent lower correlations.
The aboveground biomass of crops is a basic agro-ecological indicator for studying environmental processes and precision agriculture, which comprehensively reflects crops growth, light utilization efficiency, and carbon stocks in agricultural ecosystems, so it’s closely associated with crop yield production and human living (Li et al., 2020). Water shortages and high temperatures pose considerable threats to crops growth and development, which will be further exacerbated by climate changes (Avila et al., 2020; Rossi et al., 2020). Plant leaves are among the most important plant tissues that significantly affect plant growth and yield production. In the current study, drought stress alone dramatically decreased the leaf dry weight at V6, VT, R3, and R6 stages, which could be due to the fact that drought stress reduced the leaf area and its expansion rate (Khan et al., 2023). On one hand, the limitation of leaf area expansion is to reduce water loss, while on the other hand, it constrains the photosynthetic efficiency of plants, which indicated a reason for suppressed growth under drought stress (Karimi et al., 2018). Leaf area is reduced as a result of a reduction in cell division and cell expansion (Koch et al., 2019). Avramova et al. (2015) also showed leaf growth inhibition in maize under drought stress due to inhibition in cell expansion. In addition, drought during the jointing stage of maize will lead to hindered differentiation of the female ears, affecting the normal growth and development of filaments and forming empty poles or ears (Guo et al., 2023). Therefore, in our study, both stem and single ear dry weight significantly decreased with the intensification of drought severity at any growth stage. Overall, water stress led to a substantial reduction in the total aboveground biomass as well. This conclusion is consistent with the result reported by Rahman et al. (2024) under high temperature and drought combination.
Biomass accumulation is one of the most sensitive growth processes of crops, so this process is susceptible to the negative effects of high temperature stress. High temperature usually accelerates the degradation of chlorophyll in leaves, thereby weakens leaf photosynthetic capacity and reduces green leaf area (Viljevac Vuletic et al., 2022). In the present study, high temperature applied individually prominently reduced the leaf dry weight of maize plants at any growth stage. The declines of photosynthetic rate and green leaf area index caused by high temperature ultimately combine to reduce biomass production (Wu et al., 2019). In another study, the authors demonstrated that heat at the jointing stage will affects the synthesis and transport of organic matter in maize plants and the differentiation of male and female ears (Guo et al., 2023). According to our results, the stem and single ear dry weight dramatically reduced with the elevation of temperature at V6, VT, R3, and R6 stages. As a result, maize plants subjected to high temperature applied as single-stress factor displayed a significant reduction in total aboveground biomass. Previous literature have showed that reduced photosynthesis, decreased leaf area index and shortened grain filling duration are all potential pivotal factors leading to biomass decline under high temperature (Xiong et al., 2017; Rossi et al., 2020). In addition, the impacts of high temperature on biomass production could be modified by other environmental conditions, like water stress (Liu et al., 2017). Hu et al. (2023) have shown that the stress combination inhibited the dry matter production to a similar degree or even a lower degree with high temperature, indicating that there was a positive interaction between high temperature and drought stress. Similarly, it can be seen that high temperature and drought combination had a synergistic impact on aboveground biomass in our study. In detail, the stress combination reduced the aboveground biomass more significantly than the single stress, which indicated that high temperature exacerbated the negative effects of drought stress on the growth and development of maize plants, thereby reducing the accumulation of aboveground biomass.
As an adaptation to drought stress, plants adjust the relationship between water, transpiration, photosynthesis, and water use efficiency through stomatal changes in order to maximize CO2 assimilation (Li et al., 2017). When plants sense drought stress and the consequences demand saving water, rapid stomatal closure is induced to prevent water loss (Ji et al., 2018). Our data suggested that maize seedlings under drought treatment showed stomatal closure, thereby reducing transpirational water loss, which indicated that stomatal closure was an active process to maintain the plant water status (Schachtman and Goodger, 2008). In this scenario, stomatal closure results in less CO2 entering the mesophyll cells, so oxygen becomes the electron acceptor in the chloroplasts, which leads to the rapid generation of reactive oxygen species (ROS) and induces lipid peroxidation in the cell membrane (Orek et al., 2020; Zafari et al., 2020). As a result, intracellular production of superoxide free radicals leads to the degradation of photosynthetic pigments and the disruption of photosynthetic electron transfer system, which ultimately result in decreased photosynthetic capacity, stunted plant development, and lower yield production (Chen et al., 2022; Li et al., 2022). Our results thus indicated that drought stress dramatically reduced the Pn of maize plants and yield components including EW, GW, EL, ED, and HI. Therefore, drought as single stress can negatively impact each yield component, which is consistent with the result reported by Fard et al. (2023) under drought stress. Water use efficiency (WUE) is considered an appropriate parameter generally used to study plant water status to drought stress (Hatfield and Dold, 2019). Our results indicated that WUE first increased and then decreased along the soil water status under control temperature. In agreement with the previous study of Karimi et al. (2020), we found a significant increase in WUE under mild water stress. This is because stomatal closure under drought stress results in a simultaneous decrease in CO2 uptake and water loss. Thus, WUE increased due to lower Gs combined with sustained Pn. However, severe water stress reduced WUE by about 12.0% compared to the control treatment, which was primarily attributed to the fact that the reduction in Tr was less than that in Pn under severe water stress.
The leaf anatomical changes caused by high temperature are similar to those under drought stress, which were characterized by the stomatal closure, transpirational water loss, with severe damage to mesophyll cells caused in a reduced cell size and increased plasma membrane leakage (Zhang et al., 2005). Some studies report that high temperature increased Gs (Urban et al., 2017), while others have found that high temperature induced stomatal closure (Lahr et al., 2015), or that high temperature had no significant effect on Gs (Sage and Sharkey, 1987). In the present study, maize plants could not effectively mitigate high temperature through increasing transpiration when soil water was limited under warming condition. Hence, high temperature might induce stomatal closure and have a down-regulation effect on leaf photosynthesis. The study of Ruiz-Vera et al. (2013) also demonstrated that high temperature reduced the net photosynthetic rate of soybean in dry land, and this was associated with reductions in stomatal conductance and intercellular CO2 concentration which caused stomatal limitation. High temperature stress results in protein denaturation (altering protein conformation), cell membrane damage, and chlorophyll degradation (Larkindale et al., 2005). The degradation of photosynthetic pigments is closely associated with a severe and precipitous decline in the photosynthetic capacity of stressed plants (Wang et al., 2022), which further leads to an increase in spikelet sterility and yield loss in crops (Prasad et al., 2017). According to our results, high temperature significantly decreased Pn of maize plants, thereby reducing the EW, GW, EL, ED, and HI. Thus, high temperature as single stress can negatively impact each yield component, which is consistent with the findings reported by Chandarak et al. (2023) under heat stress. In addition, high temperature prominently decreased WUE due to stomatal closure, which is an effective strategy that plants use to adapt to high temperature.
Generally, exposure of plants to two or more factors can result in additive, synergistic, or antagonistic impacts on various functional and hierarchical levels (Jansen et al., 2019; Abdelhakim et al., 2021). Notably, our current results showed that the maize plants experiencing combined high temperature and drought exhibited compromised photosynthetic and gas exchange parameters. Remarkably, the combined stress produced the maximal detrimental effects on Gs and Tr compared to single stress, followed by drought stress. Our study thus demonstrated that maize plants prioritize drought-dependent modulation of stomatal conductance over high temperature-modulated stomatal responses under the stress combination. Moreover, a strong reduction of WUE was observed under combined stress of high temperature and drought stress which implied that the response of WUE to drought is further modulated by temperatures. Our results revealed strong interactive effects of high temperature and drought on WUE manifested by a further decline of WUE with increasing temperature in maize plants exposed to drought stress. In addition, in this study, all yield components displayed synergistic negative responses to combined high temperature and drought stress. Although both high temperature and drought as single stress can negatively impact each yield component, the impact on these traits was magnified by the stress combination. As expected, based on the impacts of high temperature and drought combination on aboveground biomass and yield components, the combined stress caused a further decline in HI.
The overproduction of ROS in instigated by high temperature or drought stress is intricately associated with photooxidative damage in stressed plants (Zhou et al., 2019), and this overabundance of ROS also elevates the levels of MDA content, which further indicate oxidative damage to cellular membranes (Xia et al., 2023). In the current investigation, maize plants subjected to individual and combined high temperature and drought stress exhibited the increased oxidative damage as evidenced by the accumulations of MDA in stressed plants. Specifically, the effects of drought stress on MDA content were more significant than that of high temperature, and the effect of combined stress was the greatest. As a result, high temperature and drought combination had a synergistic impact on MDA synthesis. However, the interactive effects of combined stress at an early stage on MDA content were not obvious, implying that high temperature and drought combination had delayed effects on MDA synthesis, which has rarely been demonstrated in previous research.
In response to the damage inflicted on organisms by high temperature or drought stress, most crops mitigate oxidative damage by promoting the synthesis of related enzymes such as SOD, POD, and CAT, thereby alleviating the negative effects to crops (Xue et al., 2012; Han et al., 2022). With the aggravation of heat or drought stress, the maize plants have suffered a certain extent of cellular damage, and upregulation of antioxidant enzymes activities can rapidly scavenge ROS to improve membrane stability, thus reducing the damage to cells and maintaining the normal growth of plants (Xue et al., 2012; Ahanger et al., 2021). In the current study, we observed both individual high temperature and drought stress caused an improvement in SOD, POD, and CAT activity. It was noted that this study showed that the effects of high temperature on POD and CAT activities of maize leaves were more significant than those of drought stress, while the effect of drought stress on SOD activity was more obvious than that of high temperature. Although both high temperature and drought stress as single stress can positively affect antioxidant enzymes activities, the examined literature indicates a shared trend among crops which display a synergistic positive response to high temperature and drought stress combination. It can be seen that combined stress magnified the positive effects of single stress on antioxidant enzymes activities. Overall, the effects of high temperature or combined stress increased POD and CAT activities to a similar degree, whereas the effect of drought stress or stress combination increased SOD activities to a similar extent. At the later stage of stress, SOD and CAT activities first increased and then decreased along the water gradient under high temperature, which may be due to the accumulation of ROS exceeding the damage threshold caused by drought stress, leading to the disruption of the cell membrane integrity. However, POD activities consistently raised with the decline of soil water status regardless of temperature conditions, suggesting that SOD and CAT activities may be more pressure sensitive than POD, which had a more significant role in eliminating ROS from plants under severe drought stress. Huang et al. (2021) demonstrated that SOD, POD, and CAT activities showed different behaviors from 0 to 7 h stress, indicating that their activities changed and differed from each other within a period of time. This may be the main reason for the changes in antioxidant enzymes activities over time during stress. In addition, the interactive effects of combined stress at an early stage on SOD and POD activities were not significant, while CAT activity was strongly modified by the combined stress upon stress initiation. This indicated that SOD and POD activities had delayed responses to high temperature and drought combination, whereas CAT activity was more sensitive to the combined stress, which has rarely been demonstrated in previous research.
The root is a crucial plant organ that plays a decisive role in the absorption of water and mineral nutrients (Xu et al., 2021). The growth and development of plants are directly influenced by the degree of root development, which is one of the criteria used to evaluate drought tolerance (Ober et al., 2021). Many researches have reported that more photosynthetic product is transported to the root system of crops under drought stress with the aim of improving root growth and enhancing water absorption through producing higher root length, more finer roots, and deeper root distribution in the soil profile (Wang et al., 2021). In our current investigation, we observed that the RL, RV, and RSA of maize plants were continuously enhanced as the decline of soil water status, which is in line with previous outcomes (Wang et al., 2021). Inversely, the ARD dramatically declined with the intensification of drought severity, which facilitates space occupation or reduces hydraulic resistance of the cortex (Freschet et al., 2021). As a thermophilic crop, appropriately raised temperature is conducive to the maize growth, while excessively high temperatures beyond a certain range will markedly disrupt the absorption and synthesis functions of the root systems, thereby ultimately restricting the growth and development of the maize plants (Xia et al., 2021). In this study, high temperature stress was maintained on maize plants throughout the growth period, and as a result, the RL, RV, and RSA all decreased by more than 35.0%, whereas the ARD only reduced by 5.3%. This conclusion is similar to the results reported by Xia et al. (2021) under high temperature in different maize genotypes. Overall, high temperature and drought as single stress can negatively and positively impact the RL, RV, and RSA of maize plants, respectively. Thus, the RL, RV, and RSA displayed an antagonistic response to high temperature and drought combination. In detail, the intensification of drought stress alleviated the inhibitory effects of high temperature on the RL, RV, and RSA of maize plants. Remarkably, although both high temperature and drought as single stress can negatively affect each root morphology parameters, the examined literature demonstrated a shared trend among maize plants which display a synergistic negative response to the combined stress. That is to say, both individual high temperature and drought stress generally caused a decreased in the root morphology parameters, and the impact on these traits was magnified by the stress combination.
Irrigation is a potentially useful method to reduce both heat and water stress in crops simultaneously for crops (Zhang et al., 2015). Although irrigation is beneficial for crop production, enhancing the sustainability of local water resources is a key consideration for the wise development and expansion of irrigation infrastructure. The extensive groundwater extraction for agriculture has impacted the water resources in the study area. Alternative methods are available to reduce crop water stress without significantly increasing irrigation withdrawals. For instance, adopting higher-efficiency irrigation technologies could reduce or eliminate the need for additional irrigation withdrawals, but would still require finding alternative sources for dwindling water supplies (McDonald and Girvetz, 2013). Alternative crop production practices, such as conservation tillage or use of cover crops, could also reduce irrigation water consumption (Grassini et al., 2011). By limiting irrigation applications to critical periods of crop growth and yield formation, the total amount of irrigation water could also be reduced through deficit irrigation (Fereres and Soriano, 2007). Also, the combination of planting dates and phenology allows the plants to avoid the coincidence between the critical developmental phases and drought and heat stresses determining yield (Yang et al., 2020).
Two-thirds of the yield reduction can be mitigated by irrigation, and the remaining one-third of the yield loss is due to heat stress that cannot be alleviated by irrigation (Zhang et al., 2015). Thus, development of maize cultivars with greater heat tolerance should be considered the fundamental approach to adapt future yield to climate for this crop. Stress response genes and proteins such as antioxidant enzymes, heat stress transcription factors, high- and low-molecular-weight HSPs, LEA proteins, and dehydrins are important for maize plants to acquire tolerance to drought, heat, and the combined stress (Notununu et al., 2022). Therefore, it should be a priority to investigate how these stress response genes are altered under the stress combination. This in turn will have a positive impact on agricultural productivity by alleviating the negative effects of climate change over the coming decades.
Individual high temperature and drought stress had strongly inhibitory effects on maize growth and yield, and this negative impacts generally enhanced with the decline of soil water status. This suggested that high temperature may aggravate the detrimental impacts of drought stress to plant development and production. High temperature, drought stress and their combined stress all decreased the photosynthetic capacity of summer maize, thus impeding dry matter accumulation and yield production. Both single high temperature and drought caused an improvement in antioxidant enzymes activities, and the impacts on these traits were magnified by stress combination. Nevertheless, stimulating antioxidant enzymes activities cannot effectively decrease oxidative damage of maize plants. Therefore, the increase in MDA content was likely the main reason for the decrease in net photosynthetic rate under individual and combined stress. Single high temperature or drought and their combined stress also significantly influenced the root morphology parameters for the improvement of plant tolerance.
The original contributions presented in the study are included in the article/supplementary material. Further inquiries can be directed to the corresponding author.
XF: Writing – original draft. YM: Data curation, Writing – review & editing. YP: Software, Writing – review & editing. JS: Conceptualization, Writing – review & editing. PH: Software, Writing – review & editing. XH: Funding acquisition, Project administration, Writing – review & editing.
The author(s) declare financial support was received for the research, authorship, and/or publication of this article. This study was funded by the Natural Science Foundation of Shaanxi Province (2022JZ-24); the National Key Research and Development Program of China (2017YFD0201508).
The authors would like to acknowledge all the team members of the Key Laboratory of Agricultural Soil and Water Engineering in Arid and Semiarid Areas, Ministry of Education.
The authors declare that the research was conducted in the absence of any commercial or financial relationships that could be construed as a potential conflict of interest.
All claims expressed in this article are solely those of the authors and do not necessarily represent those of their affiliated organizations, or those of the publisher, the editors and the reviewers. Any product that may be evaluated in this article, or claim that may be made by its manufacturer, is not guaranteed or endorsed by the publisher.
Abdelhakim L. O. A., Palma C. F. F., Zhou R., Wollenweber B., Ottosen C. O., Rosenqvist E. (2021). The effect of individual and combined drought and heat stress under elevated CO2 on physiological responses in spring wheat genotypes. Plant Physiol. Biochem. 162, 301–314. doi: 10.1016/j.plaphy.2021.02.015
Ahanger M. A., Qi M. D., Huang Z. G., Xu X. D., Begum N., Qin C., et al. (2021). Improving growth and photosynthetic performance of drought stressed tomato by application of nano-organic fertilizer involves up-regulation of nitrogen, antioxidant and osmolyte metabolism. Ecotoxicol. Environ. Saf. 216, 112195. doi: 10.1016/j.ecoenv.2021.112195
Alam H., Khattak J. Z. K., Ksiksi T. S., Saleem M. H., Fahad S., Sohail H., et al. (2021). Negative impact of long-term exposure of salinity and drought stress on native Tetraena mandavillei L. Physiol. Plant 172, 1336–1351. doi: 10.1111/ppl.13273
Avila R. T., de Almeida W. L., Costa L. C., MaChado K. L. G., Barbosa M. L., de Souza R. P. B., et al. (2020). Elevated air [CO2] improves photosynthetic performance and alters biomass accumulation and partitioning in drought-stressed coffee plants. Environ. Exp. Bot. 177, 104137. doi: 10.1016/j.envexpbot.2020.104137
Avramova V., Abdelgawad H., Zhang Z. F., Fotschki B., Casadevall R., Vergauwen L., et al. (2015). Drought induces distinct growth response, protection, and recovery mechanisms in the maize leaf growth zone. Plant Physiol. 169 (2), 1382–1396. doi: 10.1104/pp.15.00276
Beauchamp C., Fridovich I. (1971). Superoxide dismutase: improved assays and an assay applicable to acrylamide gels. Anal. Biochem. 44 (1), 276–287. doi: 10.1016/0003-2697(71)90370-8
Ben Ameur H., Han X. Y., Liu Z. Y., Peillex J. (2022). When did global warming start? A new baseline for carbon budgeting. Econ Model. 116, 106005. doi: 10.1016/j.econmod.2022.106005
Bheemanahalli R., Vennam R. R., Ramamoorthy P., Reddy K. R. (2022). Effects of post-flowering heat and drought stresses on physiology, yield, and quality in maize (Zea mays L.). Plants Stress. 6, 100106. doi: 10.1016/j.stress.2022.100106
Chakraborty P. K., Banerjee S., Nath R., Samanta S. (2022). Assessing congenial soil temperature and its impact on root growth, grain yield of summer rice under varying water stress condition in Lower Gangetic Plain of India. J. Saudi Soc Agric. Sci. 21 (2), 98–107. doi: 10.1016/j.jssas.2021.07.001
Chandarak N., Somjinda P., Phoncharoen P., Banterng P., Taratima W., Theerakulpisut P., et al. (2023). Booting heat stress alters leaf photosynthesis, growth rate, phenology and yield in rice. Plant Stress. 10, 100226. doi: 10.1016/j.stress.2023.100226
Chen Q., Cao X., Nie X., Li Y., Liang T., Ci L. (2022). Alleviation role of functional carbon nanodots for tomato growth and soil environment under drought stress. J. Hazard. Mater. 423, 127260. doi: 10.1016/j.jhazmat.2021.12726
Chen L. J., Li Z. Z., Zhou X. W., Xing X. Y., Lv B. (2023). Integrated transcriptome and metabolome analysis reveals molecular responses of spider to single and combined high temperature and drought stress. Environ. pollut. 317, 120763. doi: 10.1016/j.envpol.2022.120763
Cohen I., Huck C., Fritschi F. B., Mittler R., Zandalinas S. I. (2021). Meta-analysis of drought and heat stress combination impact on crop yield and yield components. Physiol. Plant 171 (1), 66–76. doi: 10.1111/ppl.13203
Cui J., Kusutani A., Toyota M., Asanuma K. I. (2000). Studies on the varietal difference of harvest index in rice: relationship between harvest index and dry matter production. Jpn. J. Crop Sci. 69 (3), 359–364. doi: 10.1626/jcs.69.359
Delamare J., Brune-Muguet S., Morvan-Bertrand A., Cantat O., Firmin S., Trinsoutrot-Gattin I., et al. (2023). Thermopriming effects on root morphological traits and root exudation during the reproductive phase in two species with contrasting strategies: Brassica napus (L.) and Camelina sativa (L.) Crantz. Environ. Exp. Bot. 210, 105318. doi: 10.1016/j.envexpbot.2023.105318
Du Z., Bramlage W. J. (1992). Modifified thiobarbituric acid assay for measuring lipid oxidation in sugar-rich plant tissue extracts. J. Agric. Food Chem. 40, 1566–1570. doi: 10.1021/jf00021a018
Fageria N. K. (2007). Yield physiology of rice. J. Plant Nutr. 30, 843–879. doi: 10.1080/15226510701374831
Fard S. K., Soleymani A., Javanmard H. (2023). Plant growth regulators affecting maize leaf senescence and area index impact yield under drought. Biocatal. Agr. Biotech. 51, 102749. doi: 10.1016/j.bcab.2023.102749
Feng X. L., Liu R., Li C. J., Zhang H., Slot M. (2023). Contrasting responses of two C4 desert shrubs to drought but consistent decoupling of photosynthesis and stomatal conductance at high temperature. Environ. Exp. Bot. 209, 105295. doi: 10.1016/j.envexpbot.2023.105295
Fereres E., Soriano M. A. (2007). Deficit irrigation for reducing agricultural water use. J. Exp. Bot. 58 (2), 147–159. doi: 10.1093/jxb/erl165
Foyer (2018). Reactive oxygen species, oxidative signaling and the regulation of photosynthesis. Environ. Exp. Bot. 154, 134–142. doi: 10.1016/j.envexpbot.2018.05.003
Freschet G. T., Roumet C., Comes L. H., Weemstra M., Bengough A. G., Rewald B., et al. (2021). Root traits as drivers of plant and ecosystem functioning: current understanding, pitfalls and future research needs. New Phytol. 232, 1123–1158. doi: 10.1111/nph.17072
Grassini P., Yang H. S., Irmak S., Thorburn J., Burr C., Cassman K. G. (2011). High-yield irrigated maize in the Western U.S. Corn Belt: II. Irrigation management and crop water productivity. Field Crop Res. 120 (1), 133–141. doi: 10.1016/j.fcr.2010.09.013
Gultekin R., Avag K., Görgisen C., Öztürk Ö., Yeter T., Bahceci Alsan P. (2023). Effect of deficit irrigation practices on greenhouse gas emissions in drip irrigation. Sci. Hortic. 310, 111757. doi: 10.1016/j.scienta.2022.111757
Guo Y., Zhang J. Q., Li K. W., Aru H., Feng Z., Liu X. P., et al. (2023). Quantifying hazard of drought and heat compound extreme events during maize (Zea mays L.) growing season using Magnitude Index and Copula. Weather Clim. Extreme. 40, 100566. doi: 10.1016/j.wace.2023.100566
Han Y. X., Hou Z. N., Zhang X. M., Yan K. J., Liang Z. S., He Q. L. (2022). Important changes in germination, seedling tolerance, and active components content due to drought stress on three licorice (Glycyrrhiza) species. Ind. Crop Prod. 175, 114240. doi: 10.1016/j.indcrop.2021.114240
Hatfield J. L., Dold C. (2019). Water-use efficiency: advances and challenges in a changing climate. Front. Plant Sci. 10. doi: 10.3389/fpls.2019.00103
Hu J., Zhao X. Y., Gu L. M., Liu P., Zhao B., Zhang J. W., et al. (2023). The effects of high temperature, drought, and their combined stresses on the photosynthesis and senescence of summer maize. Agr. Water Manage. 289, 108525. doi: 10.1016/j.agwat.2023.108525
Huang J. M., Chen Q., Rong Y., Tang B., Zhu L., Ren R., et al. (2021). Transcriptome analysis revealed gene regulatory network involved in PEG-induced drought stress in Tartary buckwheat (Fagopyrum Tararicum). Peerj. 9, e11136. doi: 10.7717/peerj.11136
Hussain H. A., Men S., Hussain S., Chen Y., Ali S., Zhang S., et al. (2019). Interactive effects of drought and heat stresses on morpho-physiological attributes, yield, nutrient uptake and oxidative status in maize hybrids. Sci. Rep. 9 (1), 1–12. doi: 10.1038/s41598-019-40362-7
IPCC (2022). Climate change 2022: impacts, adaptation and vulnerability. Contribution of working group II to the sixth assessment report of the intergovernmental panel on climate change. Eds. Portner H.-O., Roberts D. C., Tignor M., Poloczanska E. S., Mintenbeck K., Alegría A., Craig M., Langsdorf S., Loschke S., Moller V., Okem A., Rama B. (Cambridge, UK and New York, NY, USA: Cambridge University Press), 3056. doi: 10.1017/9781009325844
Iqbal N., Umar S., Khan N. A., Corpas F. J. (2021). Nitric oxide and hydrogen sulfide coordinately reduce glucose sensitivity and decrease oxidative stress via ascorbate-glutathione cycle in heat-stressed wheat (Triticum aestivum L.) plants. Antioxidants. 10, 108. doi: 10.3390/antiox10010108
Islam S. M. S., Islam K. M. A., Mullick M. R. A. (2022). Drought hot spot analysis using local indicators of spatial autocorrelation: An experience from Bangladesh. Environ. Chall. 6, 100410. doi: 10.1016/j.envc.2021.100410
Jansen M. A. K., Bilger W., Hideg É., Strid Å., Participants U. W., Urban O. (2019). Editorial: Interactive effects of UV-B radiation in a complex environment. Plant Physiol. Biochem. 134, 1–8. doi: 10.1016/j.plaphy.2018.10.021
Ji T., Li S. Z., Li L. J., Huang M. L., Wang X. F., Wei M., et al. (2018). Cucumber phospholipase D alpha gene overexpression in tobacco enhanced drought stress tolerance by regulating stomatal closure and lipid peroxidation. BMC. Plant Biol. 18, 355. doi: 10.1186/s12870-018-1592-y
Karimi S., Karami H., Vahdati K., Mokhtassi-Bidgoli A. (2020). Antioxidative responses to short-term salinity stress induce drought tolerance in walnut. Sci. Horrtic. 267, 109322. doi: 10.1016/j.scienta.2020.109322
Karimi S., Rahemi M., Rostami A. A., Sedaghat S. (2018). Drought effects on growth, water content and osmoprotectants in four olive cultivars with different drought tolerance. Int. J. Fruit Sci. 18 (3), 254–267. doi: 10.1080/15538362.2018.1438328
Khan R., Ma X. H., Hussain Q., Chen K., Farooq S., Asim M., et al. (2023). Transcriptome and anatomical studies reveal alterations in leaf thickness under long-term drought stress in tobacco. J. Plant Physiol. 281, 153920. doi: 10.1016/j.jplph.2023.153920
Koch G., Rolland G., Dauzat M., Bédiée A., Baldzzi V., Bertin N., et al. (2019). Leaf production and expansion: a generalized response to drought stresses from cells to whole leaf biomass-a case study in the tomato compound leaf. Plants. 8 (10), 409. doi: 10.3390/plants8100409
Krause G. H., Winter K., Krause B., Jahns P., García M., Aranda J. (2010). High-temperature tolerance of a tropical tree, Ficus insipida: methodological reassessment and climate change considerations. Funct. Plant Biol. 37 (9), 890–900. doi: 10.1071/FP10034
Kweku D. W., Bismark O., Maxwell A., Desmond K. A., Danso K. B., Oti-Mensah E. A., et al. (2018). Greenhouse effect: greenhouse gases and their impact on global warming. J. Sci. Res. Rep. 17 (6), 1–9. doi: 10.9734/JSRR/2017/39630
Lahr E. C., SChade G. W., Crossett C. C., Watson M. R. (2015). Photosynthesis and isoprene emission from trees along an urban-rural gradient in Texas. Glob. Chang Biol. 21, 4221–4236. doi: 10.1111/gcb.13010
Larkindale J., Hall J. D., Knight M. R., Vierling E. (2005). Heat stress phenotypes of Arabidopsis mutants implicate multiple signaling pathways in the acquisition of thermotolerance. Plant Physiol. 138 (2), 882–897. doi: 10.1104/pp.105.062257
Li Y. P., Li H. B., Li Y. Y., Zhang S. Q. (2017). Improving water-use efficiency by decreasing stomatal conductance and transpiration rate to maintain higher ear photosynthetic rate in drought-resistant wheat. Crop J. 5 (3), 231–239. doi: 10.1016/j.cj.2017.01.001
Li B., Xu X., Zhang L., Han J., Bian C., Li G., et al. (2020). Above-ground biomass estimation and yield prediction in potato by using UAV-based RGB and hyperspectral imaging. ISPRS J. Photogramm. 162, 161–172. doi: 10.1016/j.isprsjprs.2020.02.013
Li E., Zhao J., Pullens J. W. M., Yang X. G. (2022). The compound effects of drought and high temperature stresses will be the main constraints on maize yield in Northeast China. Sci. Total. Environ. 812, 152461. doi: 10.1016/j.scitotenv.2021.152461
Liu B., Asseng S., Wang A. N., Wang S. H., Tang L., Cao W. X., et al. (2017). Modelling the effects of post-heading heat stress on biomass growth of winter wheat. Agr. For. Meteorol. 247, 476–490. doi: 10.1016/j.agrformet.2017.08.018
Liu S. L., Xiao L. J., Sun J., Yang P., Yang X. G., Wu W. B. (2022). Probability of maize yield failure increases with drought occurrence but partially depends on local conditions in China. Eur. J. Agron. 139, 126552. doi: 10.1016/j.eja.2022.126552
McDonald R. I., Girvetz E. H. (2013). Two challenges for U.S. irrigation due to climate change: Increasing irrigated area in wet states and increasing irrigation rates in dry states. PloS One 8, e65589. doi: 10.1371/journal.pone.0065589
Naing A. H., Kim C. K. (2021). Abiotic stress-induced anthocyanins in plants: their role in tolerance to abiotic stresses. Physiol. Plant 172 (3), 1711–1723. doi: 10.1111/ppl.13373
Notununu I., Moleleki L., Roopnarain A., Adeleke R. (2022). Effects of plant growth-promoting rhizobacteria on the molecular responses of maize under drought and heat stresses: A review. Pedosphere. 32 (1), 90–106. doi: 10.1016/S1002-0160(21)60051-6
Ober E. S., Alahmad S., Cockram J., Forestan C., Hickey L. T., Kant J., et al. (2021). Wheat root systems as a breeding target for climate resilience. Theor. Appl. Genet. 134, 1645–1662. doi: 10.1007/s00122-021-03819-w
Orek C., Gruissem W., Ferguson M., Vanderschuren H. (2020). Morpho-physiological and molecular evaluation of drought tolerance in cassava (Manihot esculenta Crantz). Field Crop Res. 255, 107861. doi: 10.1016/j.fcr.2020.107861
Park S. H., Lee B. R., La V. H., Mamun M. A., Bae D. W., Kim T. H. (2021). Characterization of salicylic acid- and abscisic acid-mediated photosynthesis, Ca2+ and H2O2 accumulation in two distinct phases of drought stress intensity in Brassica napus. Environ. Exp. Bot. 186, 104434. doi: 10.1016/j.envexpbot.2021.104434
Prasad P. V. V., Bheemanahalli R., Jagadish S. V. K. (2017). Field crops and the fear of heat stress-opportunities, challenges and future directions. Field Crop Res. 200, 114–121. doi: 10.1016/j.fcr.2016.09.024
Prince S. J., Song L., Qiu D., Maldonado Dos Santos J. V., Chai C., Joshi T., et al. (2015). Genetic variants in root architecture-related genes in a Glycine soja accession, a potential resource to improve cultivated soybean. BMC Genom. 16, 132. doi: 10.1186/s12864-015-1334-6
Rahman M., Mostofa M. G., Keya S. S., Ghosh P. K., Abdelrahman M., Anik T. R. (2024). Jasmonic acid priming augments antioxidant defense and photosynthesis in soybean to alleviate combined heat and drought stress effects. Plant Physiol. Biochem. 206, 108193. doi: 10.1016/j.plaphy.2023.108193
Rossi S., Chapman C., Huang B. (2020). Suppression of heat-induced leaf senescence by γ-aminobutyric acid, proline, and ammonium nitrate through regulation of chlorophyll degradation in creeping bentgrass. Environ. Exp. Bot. 177, 104116. doi: 10.1016/j.envexpbot.2020.104116
Ru C., Hu X. T., Chen D. Y., Wang W. E., Song T. Y. (2022). Heat and drought priming induce tolerance to subsequent heat and drought stress by regulating leaf photosynthesis, root morphology, and antioxidant defense in maize seedlings. Environ. Exp. Bot. 202, 105010. doi: 10.1016/j.envexpbot.2022.105010
Ruiz-Vera U. M., Siebers M., Gray S. B., Drag D. W., Rosenthal D. M., Kimball B. A., et al. (2013). Global warming can negate the expected CO2 stimulation in photosynthesis and productivity for soybean grown in the Midwestern United States. Plant Physiol. 162 (1), 410–423. doi: 10.1104/pp.112.211938
Sachdev S., Ansari S. A., Ansari M. I., Fujita M., Hasanuzzaman M. (2021). Abiotic stress and reactive oxygen species: generation signaling, and defense mechanisms. Antioxidants. 10 (2), 277. doi: 10.3390/antiox10020277
Sage R. F., Sharkey T. D. (1987). The effect of temperature on the occurrence of O2 and CO2 insensitive photosynthesis in field grown plants. Plant Physiol. 84 (3), 658–664. doi: 10.2307/4270692
Sarker K. K., Hossain A., Timsina J., Biswas S. K., Malone S. L., Alam M. K., et al. (2020). Alternate furrow irrigation can maintain grain yield and nutrient content, and increase crop water productivity in dry season maize in sub-tropical climate of South Asia. Agr. Water Manage. 238, 106229. doi: 10.1016/j.agwat.2020.106229
Schachtman D. P., Goodger J. Q. D. (2008). Chemical root to shoot signaling under drought. Trends Plant Sci. 13 (6), 281–287. doi: 10.1016/j.tplants.2008.04.003
Siddiqui M. H., Mukherjee S., Kumar R., Alansi S., Shah A. A., Kalaji H. M., et al. (2022). Potassium and melatonin-mediated regulation of fructose-1, 6-bisphosphatase (FBPase) and sedoheptulose-1, 7-bisphosphatase (SBPase) activity improve photosynthetic efficiency, carbon assimilation and modulate glyoxalase system accompanying tolerance to cadmium stress in tomato seedlings. Plant Physiol. Biochem. 171, 49–65. doi: 10.1016/j.plaphy.2021.12.018
Ullah A., Nadeem F., Nawaz A., Siddique K. H. M., Farooq M. (2022). Heat stress effects on the reproductive physiology and yield of wheat. J. Agron. Crop Sci. 208, 1–17. doi: 10.1111/jac.12572
Urban J., Ingwers M. W., Mcguire M. A., Teskey R. O. (2017). Increase in leaf temperature opens stomata and decouples net photosynthesis from stomatal conductance in Pinus taeda and Populus deltoides x nigra. J. Exp. Bot. 68 (7), 1757–1767. doi: 10.1093/jxb/erx052
Viljevac Vuletic M., Mihaljević I., Tomaš V., Horvat D., Zdunić Z., Vuković D. (2022). Physiological response to short-term heat stress in the leaves of traditional and modern plum (Prunus domestica L.) cultivars. Horticulturae. 8 (1), 72. doi: 10.3390/horticulturae8010072
Wang R., Cavagnaro T. R., Jiang Y., Dijkstra F. A. (2021). Carbon allocation to the rhizosphere is affected by drought and nitrogen addition. J. Ecol. 109, 3699–3709. doi: 10.1111/1365-2745.13746
Wang G. J., Zeng F. L., Song P., Sun B., Wang Q., Wang J. Y. (2022). Effects of reduced chlorophyll content on photosystem functions and photosynthetic electron transport rate in rice leaves. J. Plant Physiol. 272, 153669–153611. doi: 10.1016/j.jplph.2022.153669
Wu A., Hammer G. L., Doherty A., von Caemmerer S., Farquhar G. D. (2019). Quantifying impacts of enhancing photosynthesis on crop yield. Nat. Plants. 5 (4), 380–388. doi: 10.1038/s41477-019-0398-8
Xia Z. Q., Wu M. K., Bai J. X., Zhang S. B., Zhang G. X., Gong Y. X., et al. (2023). Root zone temperature regulates potassium absorption and photosynthesis in maize (Zea mays). Plant Physiol. Biochem. 198, 107694. doi: 10.1016/j.plaphy.2023.107694
Xia Z. Q., Zhang G. X., Zhang S. B., Wang Q., Fu Y. F., Lu H. D. (2021). Efficacy of root zone temperature increase in root and shoot development and hormone changes in different maize genotypes. Agriculture-Basel. 11 (6), 477. doi: 10.3390/agriculture11060477
Xiong D., Ling X., Huang J., Peng S. (2017). Meta-analysis and dose-response analysis of high temperature effects on rice yield and quality. Environ. Exp. Bot. 141, 1–9. doi: 10.1016/j.envexpbot.2017.06.007
Xu F., Chen S., Yang X., Zhou S., Wang J., Zhang Z., et al. (2021). Genome-wide association study on root traits under different growing environments in wheat (Triticum aestivum L.). Front. Genet. 12. doi: 10.3389/fgene.2021.646712
Xue D., Jiang H., Hu J., Zhang X., Guo L., Zeng D., et al. (2012). Characterization of physiological response and identification of associated genes under heat stress in rice seedlings. Plant Physiol. Biochem. 61, 46–53. doi: 10.1016/j.plaphy.2012.08.011
Yang C. Y., Fraga H., Ieperen W. V., Santos J. A. (2020). Assessing the impacts of recent-past climatic constraints on potential wheat yield and adaptation options under Mediterranean climate in southern Portugal. Agric. Syst. 182, 102844. doi: 10.1016/j.agsy.2020.102844
Zafari M., Ebadi A., Jahanbakhsh S., Sedghi M. (2020). Safflower (Carthamus tinctorius) biochemical properties, yield, and oil content affected by 24-epibrassinosteroid and genotype under drought stress. J. Agric. Food Chem. 68, 6040–6047. doi: 10.1021/acs.jafc.9b06860
Zhan B. H., Yang X. L., Steven A. L., Zhou X. P. (2022). Recent progress in maize lethal necrosis disease: From pathogens to integrated pest management. J. Integr. Agr. 21 (12), 3445–3455. doi: 10.1016/j.jia.2022.08.050
Zhang X. Z. (1992). “The measurement and mechanism of lipid peroxidation and SOD, POD and CAT activities in biological system,” in Research methodology of crop physiology (Beijing, China: Agriculture Press), 208–211.
Zhang J. H., Huang W. D., Liu Y. P., Pan Q. H. (2005). Effects of temperature acclimation pretreatment on the ultrastructure of mesophyll cells in young grape plants (Vitis vinifera L. cv. Jingxiu) under cross-temperature stresses. J. Integr. Biol. 47, 959–970. doi: 10.1111/j.1744-7909.2005.00109.x
Zhang T. Y., Lin X. M., Sassenrath G. F. (2015). Current irrigation practices in the central United States reduce drought and extreme heat impacts for maize and soybean, but not for wheat. Sci. Total. Environ. 508, 331–342. doi: 10.1016/j.scitotenv.2014.12.004
Zhang Y. L., Wu Z. Y., Singh V. P., Lin Q. X., Ning S. W., Zhou Y. L., et al. (2023). Agricultural drought characteristics in a typical plain region considering irrigation, crop growth, and water demand impacts. Agr. Water. Manage. 282, 108266. doi: 10.1016/j.agwat.2023.108266
Zhao Y., Yu W., Hu X., Shi Y., Liu Y., Zhong Y., et al. (2018). Physiological and transcriptomic analysis revealed the involvement of crucial factors in heat stress response of Rhododendron hainanense. Gene. 660, 109–119. doi: 10.1016/j.gene.2018.03.082
Keywords: high temperature, drought, plant growth, leaf photosynthesis, antioxidant activity, yield components
Citation: Fan X, Hu X, Ma Y, Pang Y, Sun J and Hou P (2024) Influence of high temperature and drought stress at jointing stage on crop physiological responses and growth in summer maize plants (Zea mays L.). Front. Plant Physiol. 2:1331421. doi: 10.3389/fphgy.2024.1331421
Received: 01 November 2023; Accepted: 16 January 2024;
Published: 01 February 2024.
Edited by:
Giles Nicholas Johnson, The University of Manchester, United KingdomReviewed by:
Alexandra Jacquelyn Burgess, University of Nottingham, United KingdomCopyright © 2024 Fan, Hu, Ma, Pang, Sun and Hou. This is an open-access article distributed under the terms of the Creative Commons Attribution License (CC BY). The use, distribution or reproduction in other forums is permitted, provided the original author(s) and the copyright owner(s) are credited and that the original publication in this journal is cited, in accordance with accepted academic practice. No use, distribution or reproduction is permitted which does not comply with these terms.
*Correspondence: Xiaotao Hu, aHV4aWFvdGFvMTFAbndzdWFmLmVkdS5jbg==
Disclaimer: All claims expressed in this article are solely those of the authors and do not necessarily represent those of their affiliated organizations, or those of the publisher, the editors and the reviewers. Any product that may be evaluated in this article or claim that may be made by its manufacturer is not guaranteed or endorsed by the publisher.
Research integrity at Frontiers
Learn more about the work of our research integrity team to safeguard the quality of each article we publish.