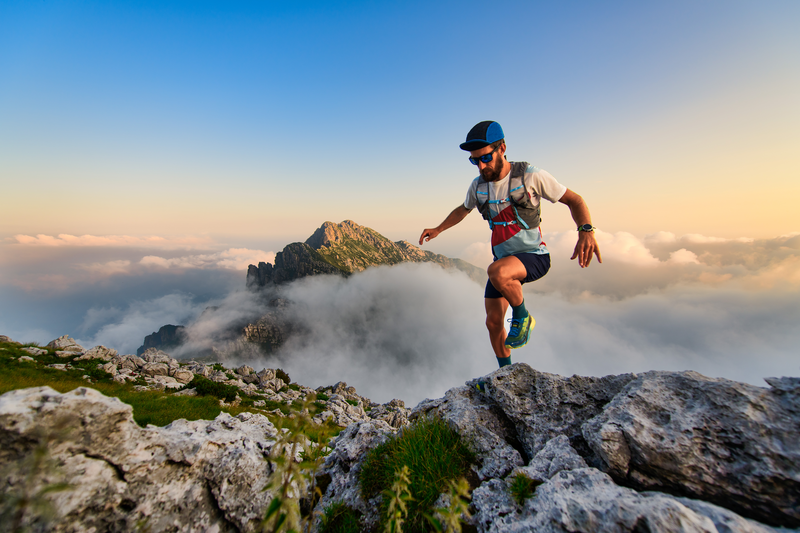
95% of researchers rate our articles as excellent or good
Learn more about the work of our research integrity team to safeguard the quality of each article we publish.
Find out more
ORIGINAL RESEARCH article
Front. Physiol.
Sec. Striated Muscle Physiology
Volume 16 - 2025 | doi: 10.3389/fphys.2025.1588084
The final, formatted version of the article will be published soon.
You have multiple emails registered with Frontiers:
Please enter your email address:
If you already have an account, please login
You don't have a Frontiers account ? You can register here
Introduction: Spasticity and altered muscle tone are key features in children with neurodevelopmental disorders, particularly cerebral palsy (CP). They impact movement, range of motion (ROM), and pain perception, influencing functional abilities and quality of life.Understanding the intrinsic muscle differences in children with CP can help improve clinical assessment and therapeutic interventions. This study aims to evaluate differences in muscle tone, stiffness, ROM, and pain perception between children with CP and typically developing peers using objective biomechanical measures.Methods: An observational, cross-sectional study was conducted with 40 participants of both sexes (20 children with CP, 20 typically developing peers). Muscle tone and stiffness of the lower limb muscles were measured using the Myoton PRO device. ROM was assessed by goniometry, and pain perception was evaluated using the Visual Analog Scale during a Straight Leg Raise (SLR) test. A generalized linear mixed model was used to detect differences in myotonometry, ROM, and pain perception measurements. In participants with CP, the Pearson product-moment correlation coefficient analysis was used to explore possible associations between clinical features and muscle tone and stiffness.Results: Children with CP exhibited reduced ROM, with a significant group effect for hip flexion (P < 0.001; η 2 = 0.843), knee extension (P < 0.001; η 2 = 0.355), and ankle flexion (P < 0.001; η 2 = 0.959). and higher pain perception during the SLR test (P < 0.001; η 2 = 0.831), compared to controls. Myotonometry revealed significantly increased muscle stiffness of the rectus femoris (P = 0.004; η 2 = 0.112) and adductor muscles (P = 0.019; η2 = 0.074) in the CP group, with no differences in muscle tone between the groups. Sex-related differences were found for muscle tone and stiffness, with males showing higher values. Correlation analyses indicated that adductor muscles stiffness was associated with CP severity.Conclusions: Children with CP demonstrate significant changes in ROM, pain perception, and muscle stiffness, emphasizing the need for targeted therapeutic interventions. These findings support the use of objective biomechanical tools for assessing muscle properties in clinical settings, contributing to better management strategies for spasticity-related impairments.
Keywords: Cerebral Palsy, Muscle stiffness, Spasticity, Pain Perception, Myotonometry
Received: 05 Mar 2025; Accepted: 27 Mar 2025.
Copyright: © 2025 González-Matilla, Abuín-Porras, Mínguez-Esteban and Heredia-Rizo. This is an open-access article distributed under the terms of the Creative Commons Attribution License (CC BY). The use, distribution or reproduction in other forums is permitted, provided the original author(s) or licensor are credited and that the original publication in this journal is cited, in accordance with accepted academic practice. No use, distribution or reproduction is permitted which does not comply with these terms.
* Correspondence:
Vanesa Abuín-Porras, Department of Physiotherapy, Universidad Europea de Madrid. Faculty of Medicine, Health and Sports,, Villaviciosa de Odón, Spain
Disclaimer: All claims expressed in this article are solely those of the authors and do not necessarily represent those of their affiliated organizations, or those of the publisher, the editors and the reviewers. Any product that may be evaluated in this article or claim that may be made by its manufacturer is not guaranteed or endorsed by the publisher.
Research integrity at Frontiers
Learn more about the work of our research integrity team to safeguard the quality of each article we publish.