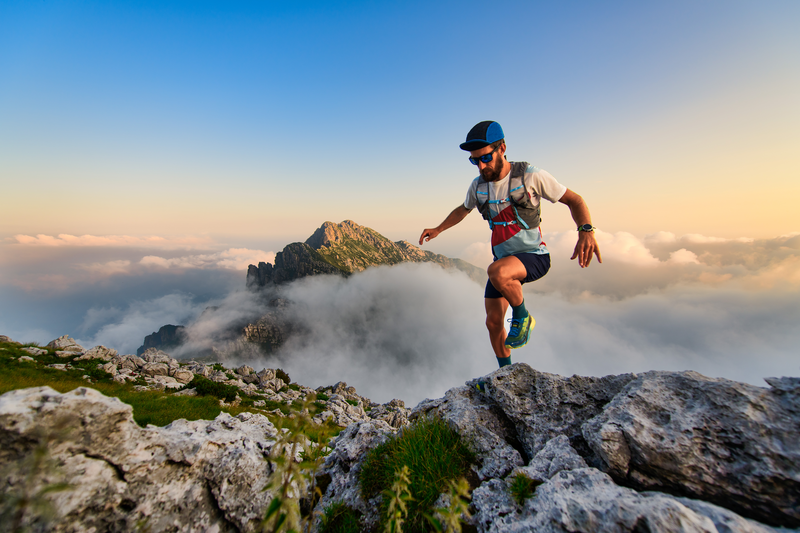
94% of researchers rate our articles as excellent or good
Learn more about the work of our research integrity team to safeguard the quality of each article we publish.
Find out more
EDITORIAL article
Front. Physiol.
Sec. Cell Physiology
Volume 16 - 2025 | doi: 10.3389/fphys.2025.1581282
This article is part of the Research Topic Cell death programs in the pathogenesis of heart disease View all 5 articles
The final, formatted version of the article will be published soon.
You have multiple emails registered with Frontiers:
Please enter your email address:
If you already have an account, please login
You don't have a Frontiers account ? You can register here
Cell death plays an important role in the pathogenesis of multiple forms of heart disease such as myocardial infarction, myocarditis, cardiomyopathies, drug-induced cardiotoxicity, and heart failure of diverse etiologies. Apoptosis is the first identified form of regulated cell death, which is characterized by cell shrinkage, membrane blebbing, chromatin condensation, and DNA fragmentation, without loss of plasma membrane integrity or inflammatory response (Danial and Korsmeyer, 2004). In contrast, necrosis had long been regarded as an unregulated process characterized by loss of plasma membrane integrity, cell swelling and lysis, and marked tissue inflammation and fibrosis (Edinger and Thompson, 2004). However, recent studies have identified multiple regulated necrosis programs, including necroptosis, ferroptosis, pyroptosis, parthanatos, mitochondria-mediated necrosis, and other regulated necrotic processes (Del Re et al., 2019).The discovery of regulated necrosis opens a new avenue to target necrosis for the treatment of heart disease, which was deemed impossible in the past. These cell death programs are often activated by distinct extracellular or intracellular signals under different physiological or pathological settings. The goal of this review series is to provide an overview of recent findings pertaining to the role of various cell death programs in the pathogenesis of heart diseases (Grisanti, 2024;Bhatti, 2024;Rowland, 2024;Fatima, 2024).Apoptosis can be engaged through two primary pathways: the mitochondria-dependent intrinsic pathway or the extrinsic pathway which involves ligand-mediated activation of death receptors on the plasma membrane. While research on cardiac apoptotic death mechanisms have primarily focused on delineating the molecular effectors of intrinsic apoptosis as well as extrinsic apoptosis mediated by the pro-inflammatory cytokines like tumor necrosis factor (TNF)-α in the heart, Grisanti (2024) sheds light on the emerging roles of TRAIL (TNF-related apoptosis-inducing ligand), a member of the TNF superfamily known for its role in cancer cell apoptosis, but is less studied in the context of the heart. TRAIL and its receptors, particularly DR5 (death receptor 5), have been associated with key cardiovascular disease risk factors including smoking, diabetes, and hypertension, and as well as cardiac diseases including arrhythmias, atherosclerosis, acute myocardial infarction, and heart failure. Notably, the relationship between TRAIL and cardiovascular disease may be complex, as human studies suggest both pro-apoptotic and cardioprotective effects. This duality underscores the importance of defining TRAIL's roles in different cardiac cell types and pathologies, which could pave the way for future development of TRAIL-targeted therapies.Beyond apoptosis, this research topic also highlights two emerging forms of regulated necrosis that can also contribute to cardiac disease: ferroptosis (Fatima, 2024) and pyroptosis (Bhatti, 2024). Ferroptosis is a form of regulated cell death characterized by iron-dependent lipid peroxidation. Intracellular iron overload, which can be triggered by dysregulated autophagy of ferritin (ferritinophagy) or aberrant heme degradation, induces lipid peroxidation via the Fenton reaction and cytotoxic damage. Moreover, inactivation of key suppressors of lipid peroxidation, including glutathione peroxidase 4 (GPX4) and ferroptosis suppressor protein-1 (FSP-1), can also induce ferroptotic death. Pyroptosis on the other hand, is a form of regulated necrosis associated that is closely associated with inflammation. In this form of death, activation of inflammationassociated caspases through canonical (caspase-1 dependent) or non-canonical (caspase-4/-5/-11 dependent) pathways lead cleavage and activation of pore-forming gasdermin proteins like GSDMD, which can permeabilize the plasma membrane leading to the release of proinflammatory signals. While both ferroptosis and pyroptosis have independently been implicated in a host of cardiac pathologies, notably, they both contribute to myocardial infarction/reperfusion injury, doxorubicin-induced cardiomyopathy, and hypertrophic cardiomyopathy, suggesting that these pathways may interact. Indeed, Fatima et al discuss the highlight the crosstalk between ferroptosis and pyroptosis, as deletion of GPX4, a central regulator of ferroptosis, can engage pyroptosis through the activation of caspase 11, leading to the cleavage and activation of GSDMD. This potential crosstalk between ferroptosis and pyroptosis suggests a complex interplay of death pathways in cardiac disease and future work aimed at delineating how different death mechanisms intersect and defining key regulatory nodes may hold the key to limiting cardiomyocyte cell death in cardiovascular disease.Cell death pathways in supporting cells of the heart also play important roles in disease pathogenesis. For example, fibroblasts are involved not only in maintenance of the cardiac extracellular matrix (ECM) but are also activated in response to stress and injury. Myocardial infarction results in fibroblast differentiation into myofibroblasts (Ivey, 2018) that proliferate to provide the heart with mechanical support and prevent rupture (Davis, 2014). Hypertrophic remodeling and subsequent decompensation are also associated with the development of fibrosis (Ivey, 2018;Xia, 2009). This places cardiac fibroblasts in an environmental context that may activate unfolded protein response (UPR) signaling that can initiate cell death pathways under prolonged stress (Rowland, 2024). However cardiac fibroblasts also demonstrate resistance to apoptosis and fibroblast overactivation is a major contributor to adverse cardiac remodeling and heart failure progression (Meng, 2018). Thus, future studies investigating how cardiac fibroblasts evade cell death may suggest strategies to limit pathological remodeling and disease progression.Recent studies clearly demonstrate that multiple cell death programs, including apoptosis, necroptosis, ferroptosis, pyroptosis, and other cell death modalities, contribute to the pathogenesis of heart diseases. Genetic or pharmacologic inhibition of these cell death programs showed beneficial effects in experimental models of heart disease such as myocardial infarction and heart failure. Therefore, targeting cell death represents a promising therapeutic strategy for various forms of heart disease, although these approaches warrant further investigation in clinical settings. Of note, accumulating evidence suggests that besides cardiomyocytes, cell death in other cell types, including endothelial cells, smooth muscle cells and fibroblasts, also contributes the pathogenesis of heart disease. Moreover, the relative contributions of various cell death programs under specific disease conditions remain unclear, partly due to the lack of reliable and specific cell death markers and other diagnostic tools, which warrants further investigation. The crosstalk between various cell death mechanisms further increases the complexity of these cell
Keywords: Cell Death, Heart disease, Apoptosis, ferroptosis, pyroptosis
Received: 21 Feb 2025; Accepted: 04 Mar 2025.
Copyright: © 2025 Kwong, Correll and Liu. This is an open-access article distributed under the terms of the Creative Commons Attribution License (CC BY). The use, distribution or reproduction in other forums is permitted, provided the original author(s) or licensor are credited and that the original publication in this journal is cited, in accordance with accepted academic practice. No use, distribution or reproduction is permitted which does not comply with these terms.
* Correspondence:
Jennifer Kwong, Emory University, Atlanta, 30322, Georgia, United States
Robert Correll, University of Alabama, Tuscaloosa, 35487-0166, Alabama, United States
Qinghang Liu, University of Washington, Seattle, United States
Disclaimer: All claims expressed in this article are solely those of the authors and do not necessarily represent those of their affiliated organizations, or those of the publisher, the editors and the reviewers. Any product that may be evaluated in this article or claim that may be made by its manufacturer is not guaranteed or endorsed by the publisher.
Research integrity at Frontiers
Learn more about the work of our research integrity team to safeguard the quality of each article we publish.