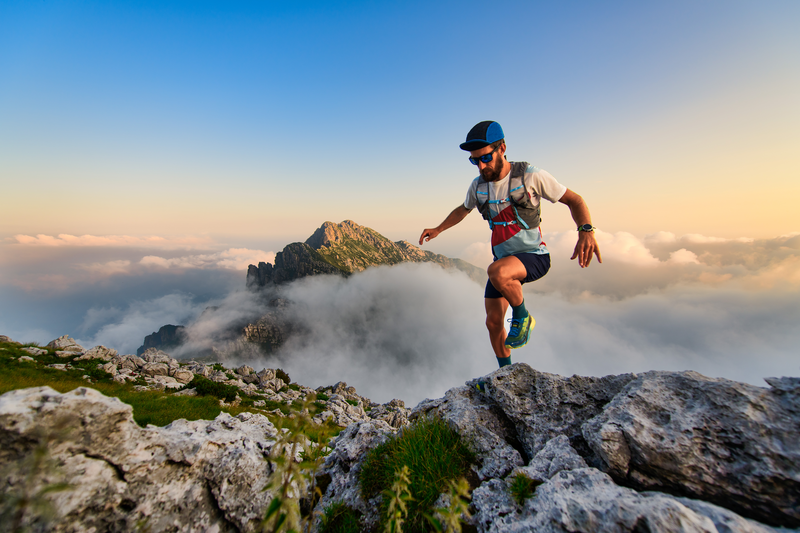
94% of researchers rate our articles as excellent or good
Learn more about the work of our research integrity team to safeguard the quality of each article we publish.
Find out more
ORIGINAL RESEARCH article
Front. Physiol.
Sec. Exercise Physiology
Volume 16 - 2025 | doi: 10.3389/fphys.2025.1574439
This article is part of the Research Topic Acute and Chronic Physiological Adaptations to Resistance Exercises Across Various Populations: Mechanisms and Practical Applications View all 7 articles
The final, formatted version of the article will be published soon.
You have multiple emails registered with Frontiers:
Please enter your email address:
If you already have an account, please login
You don't have a Frontiers account ? You can register here
The effect of inspiratory muscle training on upper limbs One Rep Maximum (1RM) in professional natural bodybuilders is still unclear. Our aim of this study is to investigate the effects of a 6 week inspiratory muscle training on 1RM results. This study included 14 athletes, that had participated in international bodybuilding competitions. Each athlete had been training for minimum of five years and at least five hours per week. The participants were randomly divided into two groups: inspiratory muscle training (IMT) and control (CON) groups. The IMT group and CON group consisted of 7 subjects. While the control group continued with the normal training regime, the IMT group additionally performed inspiratory muscle training with the resistance setting of the PowerBreathe® Classic device set to 40% of the participant's MIP. Prior to and during the six-week training period, the bench press 1 RM, anthropometry, pulmonary function tests, and maximal inspiratory (MIP) and expiratory (MEP) pressure measurements were obtained. A nutrition protocol developed by a qualified dietician was implemented for each athlete.The mean maximal strength of the respiratory muscle training group changed by 14.39%, whereas that of the control group changed by 9.43% (p=.012). The changes in the mean FVC (p = .000), FEV1 (p = .001), PEF (p = .064), MIP (p = .001), and MEP (p = .017) were greater in the IMT group. The mean circumferences of the shoulder (p = .004), chest (p = .008), arm (p = .004), 1 and neck (p = .003) improved more in the IMT group than in the CON group. A reduction in abdominal measurement was observed in the IMT group (p = 0.039), whereas no notable discrepancy was identified in body fat percentage (p = 0.295).In conclusion we identified that the addition of progressive loading inspiratory muscle training for competition preparation programs in professional natural bodybuilders will further improve their respiratory function, respiratory muscle strength, maximal strength, and muscle development. This research provides valuable insights into how IMT influences bodybuilding training outcomes, potentially guiding future interventions and program designs to better support strength development and body composition in bodybuilding training contexts.
Keywords: inspiratory muscle training1, bodybuilding2, resistance training3, muscle hypertrophy4, post-activation performance enhancement5, physical performance6
Received: 10 Feb 2025; Accepted: 07 Mar 2025.
Copyright: © 2025 Çelikel, Yılmaz, Demir, Sezer, CEYLAN, CEYLAN and Tan. This is an open-access article distributed under the terms of the Creative Commons Attribution License (CC BY). The use, distribution or reproduction in other forums is permitted, provided the original author(s) or licensor are credited and that the original publication in this journal is cited, in accordance with accepted academic practice. No use, distribution or reproduction is permitted which does not comply with these terms.
* Correspondence:
Coşkun Yılmaz, Gümüşhane University; Kelkit Aydın Dogan VOCATIONAL SCHOOL, Gümüşhane, Türkiye
Levent CEYLAN, Faculty of Sport Sciences, Hitit University, Corum, Türkiye
Disclaimer: All claims expressed in this article are solely those of the authors and do not necessarily represent those of their affiliated organizations, or those of the publisher, the editors and the reviewers. Any product that may be evaluated in this article or claim that may be made by its manufacturer is not guaranteed or endorsed by the publisher.
Research integrity at Frontiers
Learn more about the work of our research integrity team to safeguard the quality of each article we publish.