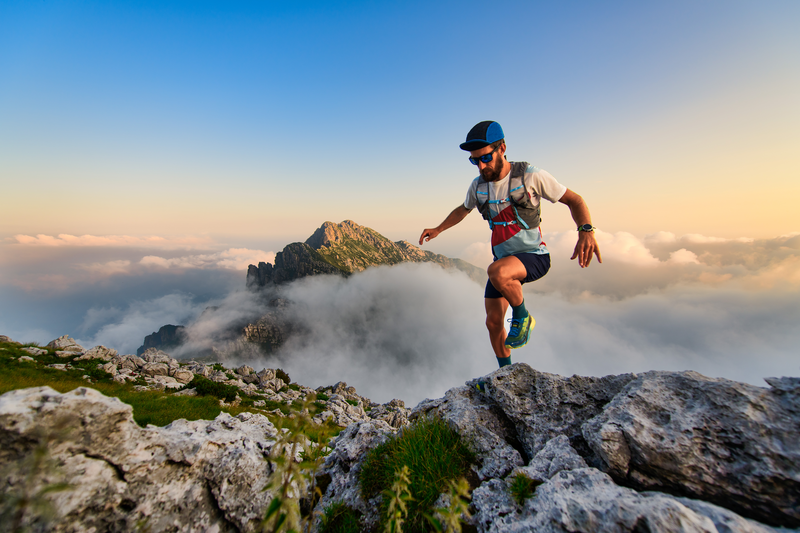
94% of researchers rate our articles as excellent or good
Learn more about the work of our research integrity team to safeguard the quality of each article we publish.
Find out more
SYSTEMATIC REVIEW article
Front. Physiol.
Sec. Exercise Physiology
Volume 16 - 2025 | doi: 10.3389/fphys.2025.1571730
This article is part of the Research Topic Physiological and Pathological Responses to Hypoxia and High Altitude, Volume III View all 6 articles
The final, formatted version of the article will be published soon.
You have multiple emails registered with Frontiers:
Please enter your email address:
If you already have an account, please login
You don't have a Frontiers account ? You can register here
Objective: This study aims to assess the impact of hypoxia training on body composition and glycolipid metabolism in excess body weight or living with obese people through meta-analysis.Methods: Randomized controlled trials investigating the effects of hypoxia training on body composition, glucose, and lipid metabolism in excess body weight or living with obese people were systematically searched from databases including CNKI, PubMed, and Web of Science. The metaanalysis was performed by using Stata 18 and RevMan 5.4 analytic tools. The risk of bias was assessed using the Cochrane evaluation tool, and the level of certainty of evidence was determined by the GRADE framework. Between-study heterogeneity was examined using the I 2 test, and the publication bias was evaluated via the Egger test or funnel plot. Results: A total of 32 RCTs with 1011 participants were included. A meta-analysis of 25 RCTs was performed (499 men and 480 women, Age: 40.25±15.69, BMI: 30.96±3.65). In terms of body composition, the outcome indexes of body fat ratio (MD is -1.16, 95% CI -1.76 to -0.56, P=0.00) in the hypoxia group were better than the normal oxygen group. There was no significant difference in body mass and BMI between the hypoxia group and the normal-oxygen group (P>0.05). In terms of lipid and glucose metabolism, no significant changes were found between the hypoxia group and the normoxia group (P>0.05). Subgroup analysis showed that training in hypoxic environment at altitude 2001-2500m could effectively improve body mass, TG and LDL-C(P<0.05). The effective program to reduce body mass is to carry out moderate intensity training of 45-60min for ≤8 weeks, ≥4 times a week (P<0.05). Conclusion: Hypoxic training is essential for reducing body fat ratio in excess body weight or obese people. It is recommended to carry out 45-60 minutes of moderate-intensity aerobic exercise for ≤8 weeks, ≥ 4 times a week, in a hypoxia environment of 2001-2500 meters to lose body mass. The effects of hypoxia training and normoxia training on lipid and glucose metabolism in excess body weight or obese people are the same.
Keywords: Hypoxia training, Obesity, Body Composition, Metabolism, meta analysis
Received: 06 Feb 2025; Accepted: 17 Feb 2025.
Copyright: © 2025 Liu, Chen, Jiang and Diaz-Cidoncha. This is an open-access article distributed under the terms of the Creative Commons Attribution License (CC BY). The use, distribution or reproduction in other forums is permitted, provided the original author(s) or licensor are credited and that the original publication in this journal is cited, in accordance with accepted academic practice. No use, distribution or reproduction is permitted which does not comply with these terms.
* Correspondence:
Xin Jiang, Dalian University College of Physical Education, Dalian, China
Disclaimer: All claims expressed in this article are solely those of the authors and do not necessarily represent those of their affiliated organizations, or those of the publisher, the editors and the reviewers. Any product that may be evaluated in this article or claim that may be made by its manufacturer is not guaranteed or endorsed by the publisher.
Research integrity at Frontiers
Learn more about the work of our research integrity team to safeguard the quality of each article we publish.