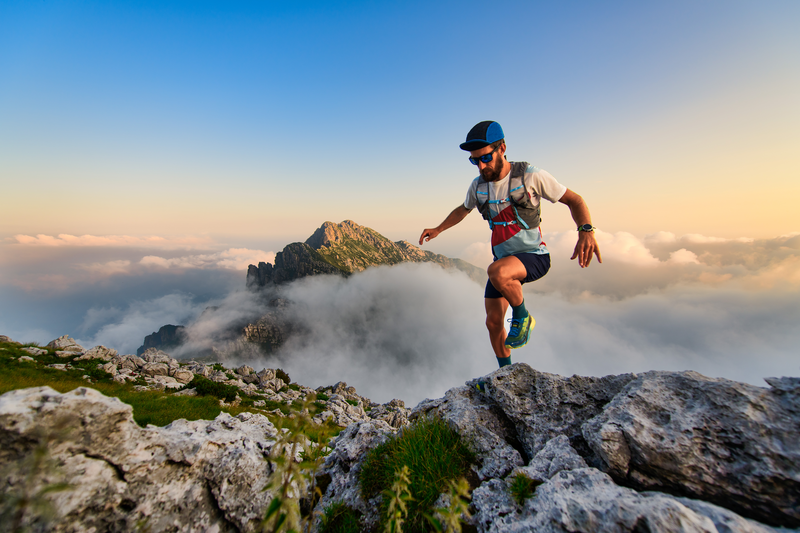
94% of researchers rate our articles as excellent or good
Learn more about the work of our research integrity team to safeguard the quality of each article we publish.
Find out more
ORIGINAL RESEARCH article
Front. Physiol.
Sec. Autonomic Neuroscience
Volume 16 - 2025 | doi: 10.3389/fphys.2025.1529398
The final, formatted version of the article will be published soon.
You have multiple emails registered with Frontiers:
Please enter your email address:
If you already have an account, please login
You don't have a Frontiers account ? You can register here
Objective: This study aims to investigate changes in the autonomic nervous system (ANS) by analyzing the characteristics of heart rate variability (HRV).Methods: A portable 3-lead dynamic electrocardiogram monitoring device was used to collect HRV data from the participants. Based on the inclusion and exclusion criteria, a total of 52 volunteers from the Xinjiang Hetian area (ultra-high plateau group, approximately 5300m altitude) and 56 volunteers from the Sichuan Chengdu area (plain group, approximately 500m altitude) were enrolled for the 24-hour long-term HRV data collection. A cross-sectional comparison was made between the groups in terms of various HRV time-domain, frequencydomain, and nonlinear indices. The diurnal and nocturnal variations in HRV and ANS after prolonged residence in the ultra-high plateau were further explored by dividing the day into daytime and nighttime periods and calculating the ΔHRV values. Additionally, the participants' heart rate and sleep conditions were analyzed.Results: Compared to the plain group, the ultra-high plateau group showed a significant reduction in overall HRV, with decreased indices of vagal activity (RMSSD, NN50, pNN50, HF, HF norm, and SD1) and increased indices of sympathetic activity (LF norm). The ANS balance indices were increased (LF/HF) and decreased (SD1/SD2), respectively. More importantly, although the diurnal and nocturnal trends of various HRV indices in the ultra-high plateau group were consistent with the plain group, the △HRV value analysis indicated that the ultra-high plateau group had increased △LF (P = 0.031) and △LF/HF (P < 0.001), and decreased △HF (P = 0.012) and △S (P = 0.001). Additionally, in the ultra-high plateau group, both the mean and minimum heart rates were elevated compared to the plain group (P < 0.001), while the maximum heart rate was reduced (P = 0.012).Furthermore, the ultra-high plateau group had a significant increase in the number of awakenings (P = 0.046) and the Apnea-Hypopnea Index (AHI) (P < 0.001).Prolonged residence in the ultra-high plateau reduces HRV, cardiac reserve capacity, and sleep quality in healthy young adults, diminishes the diurnal recovery capacity of the vagal nerve, and leads to a shift in ANS balance towards reduced vagal activity and enhanced sympathetic activity.
Keywords: Ultra-high plateau, Heart rate variability, Circadian Rhythm, Heart Rate, Sleep
Received: 16 Nov 2024; Accepted: 24 Feb 2025.
Copyright: © 2025 Zhang, Yang, Gong, Ye, Peng, Liu and Pei. This is an open-access article distributed under the terms of the Creative Commons Attribution License (CC BY). The use, distribution or reproduction in other forums is permitted, provided the original author(s) or licensor are credited and that the original publication in this journal is cited, in accordance with accepted academic practice. No use, distribution or reproduction is permitted which does not comply with these terms.
* Correspondence:
Hao Liu, Southwest Jiaotong University, Chengdu, 610031, Sichuan Province, China
Haifeng Pei, Southwest Medical University, Luzhou, China
Disclaimer: All claims expressed in this article are solely those of the authors and do not necessarily represent those of their affiliated organizations, or those of the publisher, the editors and the reviewers. Any product that may be evaluated in this article or claim that may be made by its manufacturer is not guaranteed or endorsed by the publisher.
Research integrity at Frontiers
Learn more about the work of our research integrity team to safeguard the quality of each article we publish.