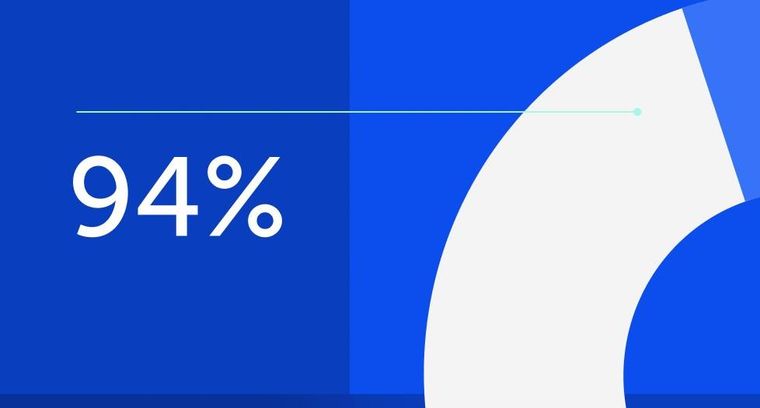
94% of researchers rate our articles as excellent or good
Learn more about the work of our research integrity team to safeguard the quality of each article we publish.
Find out more
ORIGINAL RESEARCH article
Front. Physiol., 26 March 2025
Sec. Integrative Physiology
Volume 16 - 2025 | https://doi.org/10.3389/fphys.2025.1520174
This article is part of the Research TopicPhysiological and Pathological Responses to Hypoxia and High Altitude, Volume IIIView all 8 articles
Acute and chronic hypoxia modulate the expression of inflammatory mediators known as cytokines. However, studies in chronic and intermittent hypobaric or normobaric hypoxia, like those described in miner’s population, are scarce or absent. In this study, we evaluate the effect of chronic intermittent normobaric hypoxia (CINH) on the hematological response and the expression of lymphocyte cytokines IL-1, IL-2, IL-6, and IL-10 in rats. A total of 20 Sprague-Dawley rats were divided into two groups: a) CINH (FiO2 10%, n = 10) and b) Control (normoxic, n = 10). Systolic arterial pressure and heart rate were measured using a tail-cuff sensor. Blood samples were obtained from both groups for hematological studies, and expression of cytokines obtained from lymphocytes was determined by RT-PCR. Hematocrit, hemoglobin, platelet count, and hematological constant were elevated, and leucocyte count decreased in CINH rats. In addition, systolic arterial pressure in CINH rats was significantly increased (over 50%). Cytokine expression from lymphocytes showed that IL-2, and IL-10 increased by 140% and 38%, respectively; IL-6 showed no significant change, while IL-1β expression decreased by 18%. In this regard, CINH could activate an inflammatory response mediated by IL-2. However, this response could be attenuated by increased IL-10 expression, a known anti-inflammatory cytokine, and decreased IL-1β and IL-6 expression, indicative of an adaptation mechanism to CINH.
High altitude exposes people to an environment where factors like hypobaric, cold, low air humidity, and high ultraviolet radiation make adaptation difficult. To date, approximately 23 million people live or work at altitudes between 3,500–5,000 m (Tremblay and Ainslie, 2021), exposing them to an environment with reduced barometric pressure, and hence a reduction in oxygen availability, a condition called hypoxia (Bärtsch and Gibbs, 2007; Parati et al., 2018). Based on the periodicity of exposure, hypoxia can be defined as acute (observed in tourist, climbers, and hikers), chronic (people who live permanently at high altitudes, between 3,000 and 5,000 m), and intermittent (people who alternate exposure to hypoxia and normoxia) (Viscor et al., 2018). However, the term “intermittent hypoxia” can be referred to as Episodic intermittent hypoxia, observed in obstructive sleep apnea (OSA) or chronic intermittent hypobaric hypoxia (CIHH). This condition is observed in people who work a shift system where they work at high altitudes and rest at sea level (Viscor et al., 2018).
The hypoxia-inducible factor (HIF) is a master regulator of the cellular response to low oxygen. HIF is a heterodimeric transcription factor, encompassing a HIF-1α and a HIF-1β subunit. Oxygen sensitivity is accomplished through the action of prolyl hydroxylase domain proteins that hydroxylate proline residues of the HIF-1 subunits, resulting in ubiquitination and proteasome-dependent degradation of HIF-1 when oxygen is present. In response to hypoxia, this prolyl-hydroxylase is inactivated, and HIF-1α dimerizes with HIF-1β and translocate to the cellular nucleus, activating a variety of genes involved in many processes such as glycolysis, angiogenesis, proliferation, migration, autophagy, and apoptosis, amongst others (Semenza, 2020). However, hypoxia conditions also promote an inhibition of mitochondrial oxidative phosphorylation by uncoupling the electron transport chain, causing increased production of reactive oxygen species (ROS) in macrophages, which activates and stabilizes HIF-1α (Semenza, 2020). HIF-1α also increases the formation of neutrophil extracellular traps (Khawaja et al., 2020) and neutrophil survival by inhibiting apoptosis and triggering the nuclear factor NF-kB, which plays a central role in stimulating the release of proinflammatory cytokines such as IL-1β, IL-6 and Tumor Necrosis Factor-alpha (TNFα) (Kiani et al., 2021). In humans, hypoxia induces lymphocytopenia and neutrophilia, causing alterations of specific components of the immune system, such as a reduction in T lymphocyte levels (CD4+) and an increase in natural killer (NK) cells (Mazzeo, 2005). Therefore, the key regulators of transcriptional responses in hypoxia and inflammation are HIF and NF-kB, respectively. However, the precise mechanism remains elusive (D’Ignazio et al., 2016).
Early studies have shown that acute exposure to hypobaric hypoxia (4,300 m) for 12 days produces IL-6 overexpression, mediated by β2-adrenergic stimulation, and remains elevated for several weeks in response to α-adrenergic activation (Mazzeo et al., 2001). Another study conducted in humans showed that acute exposure to hypoxia induces a significant increase in IL-6, while other proinflammatory cytokines are not altered (Klausen et al., 1997). This cytokine was also increased in subjects who passively ascended to an altitude of 3,500 m, reaching its highest concentration after 30 h of permanence at this altitude (Hartmann et al., 2000).
Studies performed in animal models have shown that chronic hypoxia induces strong expression of proinflammatory cytokines IL-6, IL-1β, and TNFα in immune cells of rats subjected to this stress, and after 28 days, IL-6 levels remain elevated, whereas the latter two returned to normal (Liu et al., 2009).
Nowadays, many of these studies are carried out preferably in conditions of acute and chronic hypoxia. However, few reports describe the effect of chronic and intermittent hypoxia on the expression of cytokines in the lymphocytes of animals subjected to this stress. Therefore, the objective of this study was to determine the effect of chronic and intermittent normobaric hypoxia on lymphocyte expression of IL-1β, IL-2, IL-6 and IL-10 in rats.
Twenty young male Sprague-Dawley rats (age: 2.0 months; weight: 250 g, handled in accordance with the Guidelines for Humanitarian Treatment of Laboratory Animals) were used. Two animals per cage were housed and provided with food (10 g/day of pellet per rat) and water using current dispensers. Ambient condition was kept at 22°C along with a 12-h light–dark cycle. All protocols used in the present study were approved by the Ethics Committee of Facultad de Medicina, Universidad Católica del Norte, Coquimbo, Chile (Resolution CEC-FAMED #01/09).
Rats were randomly divided into two groups: A Chronic Intermittent Normobaric Hypoxia group (CINH, N = 10), where animals were exposed to 24 h of hypoxia followed by 24 h of normoxia, for 30 days, and a normoxia group (CONTROL, N = 10). The intermittent hypoxia model was performed by reducing the inspired fraction of oxygen (FiO2) to 10%, using certified mixtures of oxygen and nitrogen, with a tolerance of
Body weight (BW, g), systolic blood pressure (SBP, mmHg), and heart rate (HR, bpm) were measured in rats at basal conditions, every 5 days until protocol was completed. BW was measured using a counting balance (Acculab V-1200), SBP and HR were measured using an inflatable tail-cuff and non-invasive pressure sensor (MLT125 R cuff and transducer, Panlab, AdInstruments), as previously described by our laboratory (Lopez et al., 2009; Moraga et al., 2018). The signal was transmitted through a preamplifier to a data-acquisition system (NIBP controller, Model ML126, AdInstruments), and the average value of six consecutive measurements was calculated. All measurements were performed by placing the animals inside a movement-limiting Plexiglas chamber for 10–20 min at a temperature of 22°C.
After 30 days of the protocol, the animals were anesthetized using sodium thiopental (100 mg/kg i.p.), blood samples were taken from the vena cava to be processed immediately, and the animals were euthanized by exsanguination.
1 mL of venous blood was taken in EDTA tubes for study of hematological parameters, using a hematological counter (ADVIA 60 Hematology System, Siemens). Hemoglobin concentration (g/dL), hematocrit (%), red blood cell count (RBC, cells/L), mean corpuscular volume (MCV, fL), mean corpuscular hemoglobin (MCH, pg), mean corpuscular hemoglobin concentration (MCHC, g/dL), white blood cells (WBC, cells/mm3) and platelets (cells/mm3) were reported. In addition, 4 mL of venous blood was taken in EDTA tubes for lymphocyte extraction.
Lymphocytes were obtained from 4 mL of whole blood, by centrifugation in a gradient of Ficoll-Paque Plus (Amersham Biosciences) according to the manufacturer’s instructions. Briefly, the blood sample taken with EDTA was diluted 1:1 (vol/vol) in buffered saline solution at pH 7.4. The diluted sample was mixed by gently rotating and subsequently 4 mL of diluted blood was put in a tube with 4 mL Ficoll-Paque Plus (Amersham Biosciences) without mixing. Subsequently, the samples were centrifuged at 400 g by 30 min. To improve lymphocyte extraction, the phase between the plasma and the Ficoll-Paque layer was extracted and centrifuged again at 500 g for 10 min and stored until analysis.
RNA was extracted from purified lymphocytes using the SV Total RNA Isolation kit (Promega, Madison, WI). cDNA was synthesized by reverse transcription using random hexamers and the Superscript First-Strand Synthesis System for RT-PCR kit (Invitrogen Life Technologies). Procedures were carried out according to the manufacturer’s instructions. PCR was performed using the primers indicated in Table 1. The concentration of each RNA was determined by absorbance at 260 nm.
Assay conditions were established for each pair of primers indicated in Table 1. For each sample, two independent preparations were performed to determine the range of cycles between thresholds at which there was no detectable amplification and up to saturation, where there was no greater increase in amplicon intensity with increasing amplification cycles. PCR amplification was carried out from cDNA synthesized from 1 μg of total RNA, with 1 unit of Taq polymerase (Promega), 10 mM Tris·HCl pH 9.0, 50 mM KCl, 0.1% Triton X-100, 1.5 mM MgCl2, 0.2 mM dNTP mix, and 1 μL of each resulting cDNA was used for PCR amplification of IL-1β, IL-2, IL-6, and IL-10. β-actin was used as a housekeeping gene (Table 1). Quantitative real-time PCR assays were performed in a StepOne thermal cycler (Applied Biosystems, CA, United States). The reaction procedure was as follows: initial denaturation step at 95°C for 15 min and 40 cycles (95°C for 30 s; 60°C for 30 s; 72°C for 30 s). The PCR products and molecular weight standard were separated by electrophoresis on 1% agarose gels with ethidium bromide and visualized under UV light. The optical density of the bands of the PCR products were quantified by densitometry using Scion Image Software (Beta 4.02 Win; Scion Image). Relative expression for each gene was expressed as IL-1β, IL-2, IL-6, and IL-10 divided by β-actin expression.
All data were analyzed using Prism 8.4 software (GraphPad Software, San Diego, United States) values are presented as mean ± standard deviation (SD). Changes of body weight, SBP and HR pre and post protocol were analyzed using one-way ANOVA followed by Newman-Keuls post hoc test. Differences between Control and CINH in hematological variables and cytokines expression were analyzed with unpaired t-Test. Finally, significant difference for each analysis was considered when p < 0.05.
Body weight and cardiovascular variables such as SBP and HR are shown in Table 2. We observed that after 30 days, both groups exhibit a significant increase in BW. However, control rats showed a higher and more significant increase in BW compared to rats exposed to CINH. In addition, animals exposed to CINH showed a significant increase in systolic arterial pressure compared to pre-CINH and post-control, without modifications to heart rate.
Hematocrit (Hct), erythrocyte, leucocyte and platelet count, and hematological constant are shown in Table 3. All these parameters increased in rats exposed to CINH, except the leucocyte count, which decreased to 11.5 ± 2.6 × 103/mm3 compared with 14.3 ± 3.9 × 103/mm3 in control animals (p < 0.05).
A representative blot of the expression of cytokines is shown in Figure 1A and analysis of expression is observed in Figure 1B. Our results show a significant increase in the IL-2 and IL-10 at 140% and 38%, respectively, in CINH conditions compared to the expression from control lymphocytes (p < 0.05). In contrast, IL-1β decreased its expression by 18% compared with control condition (p < 0.05). However, no significant change in IL-6 expression was observed.
Figure 1. (A) RT-PCR products for IL-1β, IL-2 IL-6, IL-10 and β-actin, obtained from lymphocytes in normoxic rats (Control) and exposed to chronic and intermittent normobaric hypoxia (CINH). MW, Molecular Weight. (B) Relative change of expression of cytokines. Data expressed as mean ± SD. Open bars, Control; Close bars, CINH. *p < 0.05, ***p < 0.0005 and ****p < 0.0001.
This study provides new insights regarding the expression of interleukins by lymphocytes in rats exposed to CINH.
In our model of CINH, the period of normobaric hypoxia of 10% was maintained for 24 h followed by 24 h in normoxia, for a total of 30 days. This protocol aims to emulate the periods of exposure to which miners are subjected in northern Chile. Previous models using rats exposed to chronic intermittent hypobaric hypoxia (CIHH) for periods of 48 h or chronic hypobaric exposure considered those animals intolerant to hypoxia since they reached hematocrit values of approximately 70%, in addition to loss of body weight and increased vascular resistance, contributing to pulmonary and systemic hypertension (Siqués et al., 2006; Lopez et al., 2009; Moraga et al., 2018). In contrast, our model of CINH demonstrates an increase in the hematological response (nearly 9%) with an increase in body weight, supporting the definition of tolerant animals to hypoxia (Lopez et al., 2009; Moraga et al., 2018), and similar observations were previously described in other models of CIHH (Germack et al., 2002). These data support that changes in blood parameters depend on the duration of exposure to hypoxia, and the increase in hematocrit described in our study (near 9%), can be related to an increase in blood viscosity and therefore explain in part the elevation in systemic blood pressure (Merrill, 1969). However, no change in hematocrit was found in episodic hypoxia associated with very short repetitive exposure in previous model of obstructive sleep apnea in rats (Fletcher et al., 1992). This model has been related with a potentiation in the activity of carotid body, causing an overflow of sympathetic activity with expression of hypertensive mechanisms mediated by proinflammatory cytokines such as IL-1β, IL-6 and TNFα, and this response was abolished by use of anti-inflammatory ibuprofen (Del Rio et al., 2012; Iturriaga et al., 2015; 2022). In addition, the increase in systolic blood pressure observed agrees with results obtained in models of chronic and CIHH exposure (Siqués et al., 2006; Lopez et al., 2009; Moraga et al., 2018; López et al., 2021).
In contrast with the increased number of erythrocytes in response to exposure to CINH, we observed that leucocyte count was decreased by 19.6% in those rats subjected to CINH compared to normoxic rats. This data agrees with previous studies showing leucopenia induced by hypoxia, with a considerable decrease in CD4+ T lymphocyte count (Pedersen and Steensberg, 2002; Thake et al., 2004; Mazzeo, 2005). In addition, lymphocytes respond and adapt to hypoxic stimulation by expressing and releasing various cytokines (Facco et al., 2005). One of the cytokines evaluated in this work was IL-1β, which is a proinflammatory molecule with pleiotropic effects on various cells, including an inhibitory effect that determines tumor regression (Dinarello, 1996; Naldini and Carraro, 2005). In the cascade of cytokines related to inflammation, IL-1β together with TNFα are early released from the inflammatory site, which in turn induces the release of IL-6 (another proinflammatory cytokine) and IL-10, which has an anti-inflammatory role, reduces the production of IL-1β and TNFα. In this regard, a study conducted in humans subjected to chronic and intermittent hypoxia showed an increase in IL-6 and IL-10 levels, associated with no changes in systemic pro-inflammatory cytokines TNFα, IL-1β, and IL-8 levels in plasma (Wang et al., 2007), supporting our present findings, where we observed that lymphocytes from rats exposed to CINH have an 18% decrease in IL-1β expression, with an associated increase in IL-10 levels. In contrast, previous reports showed that cultured human mononuclear leukocytes subjected to 40 h of continuous hypoxia dramatically increased IL-1β expression (Naldini et al., 2001). Additionally, a study in Sprague-Dawley rats showed that models of episodic intermittent hypoxia (OSA model, using 15 s of 5% O2, followed by 45 s in normoxia, for 3 h daily) induced a marked increase in serum levels of IL-1β and TNFα (Nácher et al., 2009). These differences are probably due to the different intermittency times of hypoxia, which would suggest that interleukin-producing cells adapt to the hypoxic stimulus by reducing the pro-inflammatory stimulus against chronic and intermittent hypoxia, probably through the expression of another systemic anti-inflammatory cytokine such as IL-10.
In addition, IL-10 can inhibit T cell activation, preventing tissue damage mediated by hypoxia. IL-10 is produced primarily by lymphocytes Th2 and Th0 CD4+, lymphocytes B, and macrophages (Grilli et al., 2000). The inflammatory reaction triggered by hypoxia requires activation of NF-κB, which in turn induces the expression of chemokines and cytokines. IL-10 induces the attenuation of NF-κB and the consequent decrease of chemical mediators induced by this factor (Miguel-Carrasco et al., 2010). In our work, CINH induced a 38% increase in the expression of IL-10 in lymphocytes of exposed rats, compared to lymphocytes isolated from normoxic rats, which would indicate a protective mechanism against inflammation mediated by other cytokines, inhibiting the expression of IL-6 and decreasing the expression of IL-1β in these cells. Our results agree with those previously reported, where a group of individuals was subjected to 12% O2, 1 h daily, 5 days a week, for 8 weeks, after which it was determined that plasma levels of IL-10 increased (Wang et al., 2007).
IL-2 is a 15 kDa cytokine produced almost exclusively by activated T cells and promotes the proliferation of lymphocytes, macrophages, and NK cells. It also participates in the differentiation of CD4+ T lymphocytes into Th1 and Th2 effector cells while inhibiting the differentiation of Th17 cells (Hoyer et al., 2008). In our study, we showed that CINH induces a marked increase in this proinflammatory cytokine, which correlates with previous studies, where intermittent exposure to hypoxia (OSA model) stimulates the synthesis of IL-2, IL-8, and TNFα among other cytokines (Naldini and Carraro, 1999; Ryan and McNicholas, 2008). On the other hand, in a study carried out on T lymphocytes, a drastic reduction in the expression of IL-2 was observed when these cells were exposed to 45 min of a pO2 < 40 Torr, even after 18 h of recovery in normoxia (Zuckerberg et al., 1994), suggesting again that the cells adapt to different hypoxic conditions. In our model, we speculate that increased IL-2 expression is not related to an increase in lymphocyte proliferation. This is supported by previous studies, where despite inducing the release of IL-2 in lymphocytes exposed to hypoxia during 40 h, IL-2 levels did not reach the necessary concentration to induce cell proliferation, since immune cells subjected to hypoxia express less mitotic cyclins with the consequent inhibition of their growth (Naldini and Carraro, 1999). In addition, unpublished studies performed in rats exposed to CINH of 4,600 m show decreased expression of pro-inflammatory IL-1β and preserved expression of IL-6 measured in lymphocytes, which could be associated with increased anti-inflammatory interleukin-10 levels. In the same line, unpublished data from our group performed in humans supports evidence of an increase in anti-inflammatory IL-10 production with a reduction in the expression of IL-1α and IL-1β, a low expression of IL-2, IL-6 and TNFα in acclimatized workers exposed to CIHH at 4,500 m respect of a similar population working at sea level. Nevertheless, the reduced number of cytokines studied, and the lack of information regarding plasmatic levels would be consider important limitations of the present study that must be considered in future studies, as well as expression of different transcription factors related to inflammatory pathways and their possible contribution in response to CIHH. In this sense, work in progress from our group are focused on study the expression of cytokines and transcription factors related to inflammatory response mediated by T lymphocytes in a similar animal model of CINH presented here.
In conclusion, the results presented in this work show that lymphocytes of rats exposed to CINH activate an inflammatory response mediated by IL-2. However, this response tends to be attenuated since these cells also increase IL-10 expression and hence inhibit IL-6 expression and decrease IL-1β expression, indicating an adaptative mechanism to hypoxia. This suggests that prolonged hypobaric exposure results in the sequential induction of differentially mediated anti-inflammatory activity, most likely due to the contemporary repression of the pro-inflammatory role associated to NF-κB, TGFβ and TNFα. Future studies focused on other cytokines and transcription factors related to inflammatory response are required to elucidate the pathways involved and the long-term implications of these process in people exposed to CIHH.
The raw data supporting the conclusions of this article will be made available by the authors, without undue reservation.
The animal study was approved by the Comité de Bioética, Facultad de Medicina, Universidad Catolica del Norte. The study was conducted in accordance with the local legislation and institutional requirements.
RC-J: Conceptualization, Data curation, Formal Analysis, Investigation, Methodology, Writing–original draft. GB: Conceptualization, Data curation, Formal Analysis, Funding acquisition, Investigation, Methodology, Project administration, Resources, Writing–original draft. DM: Conceptualization, Data curation, Formal Analysis, Methodology, Writing–original draft. FM: Conceptualization, Data curation, Formal Analysis, Funding acquisition, Investigation, Methodology, Project administration, Resources, Supervision, Validation, Visualization, Writing–original draft, Writing–review and editing.
The author(s) declare that financial support was received for the research and/or publication of this article. This work was funded by Innova-Corfo Project 07CN13ISM-152 “Producción, Aplicación y Validación de Biomarcadores de Exposición laboral en trabajadores de Empresas Mineras del Norte de Chile”, executed at Facultad de Medicina, Universidad Católica del Norte.
We thank Mr. Hervis Galleguillos for his permanent support in the laboratory during the development of this project.
The authors declare that the research was conducted in the absence of any commercial or financial relationships that could be construed as a potential conflict of interest.
The author(s) declare that no Generative AI was used in the creation of this manuscript.
All claims expressed in this article are solely those of the authors and do not necessarily represent those of their affiliated organizations, or those of the publisher, the editors and the reviewers. Any product that may be evaluated in this article, or claim that may be made by its manufacturer, is not guaranteed or endorsed by the publisher.
Bärtsch P., Gibbs J. S. R. (2007). Effect of altitude on the heart and the lungs. Circulation 116, 2191–2202. doi:10.1161/CIRCULATIONAHA.106.650796
Del Rio R., Moya E. A., Parga M. J., Madrid C., Iturriaga R. (2012). Carotid body inflammation and cardiorespiratory alterations in intermittent hypoxia. Eur. Respir. J. 39, 1492–1500. doi:10.1183/09031936.00141511
D’Ignazio L., Bandarra D., Rocha S. (2016). NF-κB and HIF crosstalk in immune responses. FEBS J. 283, 413–424. doi:10.1111/FEBS.13578
Dinarello C. A. (1996). Biologic basis for interleukin-1 in disease. Blood 87, 2095–2147. doi:10.1182/BLOOD.V87.6.2095.BLOODJOURNAL8762095
Facco M., Zilli C., Siviero M., Ermolao A., Travain G., Baesso I., et al. (2005). Modulation of immune response by the acute and chronic exposure to high altitude. Med. Sci. Sports Exerc 37, 768–774. doi:10.1249/01.MSS.0000162688.54089.CE
Fletcher E. C., Lesske J., Qian W., Miller C. C., Unger T. (1992). Repetitive, episodic hypoxia causes diurnal elevation of blood pressure in rats. Hypertension 19, 555–561. doi:10.1161/01.HYP.19.6.555
Germack R., Leon-Velarde F., Valdes De La Barra R., Farias J., Soto G., Richalet J. P. (2002). Effect of intermittent hypoxia on cardiovascular function, adrenoceptors and muscarinic receptors in Wistar rats. Exp. Physiol. 87, 453–460. doi:10.1111/J.1469-445X.2002.TB00058.X
Grilli M., Barbieri I., Basudev H., Brusa R., Casati C., Lozza G., et al. (2000). Interleukin-10 modulates neuronal threshold of vulnerability to ischaemic damage. Eur. J. Neurosci. 12, 2265–2272. doi:10.1046/J.1460-9568.2000.00090.X
Hartmann G., Tschöp M., Fischer R., Bidlingmaier C., Riepl R., Tschöp K., et al. (2000). High altitude increases circulating interleukin-6, interleukin-1 receptor antagonist and C-reactive protein. Cytokine 12, 246–252. doi:10.1006/CYTO.1999.0533
Hoyer K. K., Dooms H., Barron L., Abbas A. K. (2008). Interleukin-2 in the development and control of inflammatory disease. Immunol. Rev. 226, 19–28. doi:10.1111/J.1600-065X.2008.00697.X
Iturriaga R., Del Rio R., Alcayaga J. (2022). Carotid body inflammation: role in hypoxia and in the anti-inflammatory reflex. Physiol. (Bethesda) 37, 128–140. doi:10.1152/PHYSIOL.00031.2021
Iturriaga R., Moya E. A., Del Rio R. (2015). Inflammation and oxidative stress during intermittent hypoxia: the impact on chemoreception. Exp. Physiol. 100, 149–155. doi:10.1113/expphysiol.2014.079525
Khawaja A. A., Chong D. L. W., Sahota J., Mikolasch T. A., Pericleous C., Ripoll V. M., et al. (2020). Identification of a novel HIF-1α-αMβ2 Integrin-NET axis in fibrotic interstitial lung disease. Front. Immunol. 11, 2190. doi:10.3389/FIMMU.2020.02190
Kiani A. A., Elyasi H., Ghoreyshi S., Nouri N., Safarzadeh A., Nafari A. (2021). Study on hypoxia-inducible factor and its roles in immune system. Immunol. Med. 44, 223–236. doi:10.1080/25785826.2021.1910187
Klausen T., Olsen N. V., Poulsen T. D., Richalet J. P., Pedersen B. K. (1997). Hypoxemia increases serum interleukin-6 in humans. Eur. J. Appl. Physiol. Occup. Physiol. 76, 480–482. doi:10.1007/S004210050278
Liu X., He L., Stensaas L., Dinger B., Fidone S. (2009). Adaptation to chronic hypoxia involves immune cell invasion and increased expression of inflammatory cytokines in rat carotid body. Am. J. Physiol. Lung Cell Mol. Physiol. 296, 158–166. doi:10.1152/AJPLUNG.90383.2008
Lopez V., Siques P., Brito J., Vallejos C., Naveas N., Carvallo C., et al. (2009). Upregulation of arginase expression and activity in hypertensive rats exposed to chronic intermittent hypobaric hypoxia. High. Alt. Med. Biol. 10, 373–381. doi:10.1089/HAM.2008.1072
López V., Uribe E., Moraga F. A. (2021). Activation of arginase II by asymmetric dimethylarginine and homocysteine in hypertensive rats induced by hypoxia: a new model of nitric oxide synthesis regulation in hypertensive processes? Hypertens. Res. 44, 263–275. doi:10.1038/s41440-020-00574-1
Mazzeo R. S., Donovan D., Fleshner M., Butterfield G. E., Zamudio S., Wolfel E. E., et al. (2001). Interleukin-6 response to exercise and high-altitude exposure: influence of α-adrenergic blockade. J. Appl. Physiol. 91, 2143–2149. doi:10.1152/JAPPL.2001.91.5.2143
Merrill E. W. (1969). Rheology of blood. Physiol. Rev. 49, 863–888. doi:10.1152/PHYSREV.1969.49.4.863
Miguel-Carrasco J. L., Zambrano S., Blanca A. J., Mate A., Vázquez C. M. (2010). Captopril reduces cardiac inflammatory markers in spontaneously hypertensive rats by inactivation of NF-kB. J. Inflamm. 7, 21–29. doi:10.1186/1476-9255-7-21
Moraga F. A., Miranda G., López V., Vallejos C., Silva D. (2018). Chronic intermittent hypobaric hypoxia (4600 M) attenuates pulmonary vasodilation induced by acetylcholine or sodium nitroprusside. High. Alt. Med. Biol. 19, 149–155. doi:10.1089/HAM.2017.0012
Nácher M., Farré R., Montserrat J. M., Torres M., Navajas D., Bulbena O., et al. (2009). Biological consequences of oxygen desaturation and respiratory effort in an acute animal model of obstructive sleep apnea (OSA). Sleep. Med. 10, 892–897. doi:10.1016/J.SLEEP.2008.09.014
Naldini A., Carraro F. (1999). Hypoxia modulates cyclin and cytokine expression and inhibits peripheral mononuclear cell proliferation. J. Cell Physiol. 181, 448–454. doi:10.1002/(SICI)1097-4652(199912)181:3<448::AID-JCP8>3.0.CO;2-F
Naldini A., Carraro F. (2005). Role of inflammatory mediators in angiogenesis. Curr. Drug Targets Inflamm. Allergy 4, 3–8. doi:10.2174/1568010053622830
Naldini A., Pucci A., Carraro F. (2001). Hypoxia induces the expression and release of interleukin 1 receptor antagonist in mitogen-activated mononuclear cells. Cytokine 13, 334–341. doi:10.1006/CYTO.2001.0842
Parati G., Agostoni P., Basnyat B., Bilo G., Brugger H., Coca A., et al. (2018). Clinical recommendations for high altitude exposure of individuals with pre-existing cardiovascular conditions: a joint statement by the European society of cardiology, the council on hypertension of the European society of cardiology, the European society of hypertension, the international society of mountain medicine, the Italian society of hypertension and the Italian society of mountain medicine. Eur. Heart J. 39, 1546–1554. doi:10.1093/EURHEARTJ/EHX720
Pedersen B. K., Steensberg A. (2002). Exercise and hypoxia: effects on leukocytes and interleukin-6-shared mechanisms? Med. Sci. Sports Exerc 34, 2004–2013. doi:10.1097/00005768-200212000-00022
Ryan S., McNicholas W. T. (2008). Intermittent hypoxia and activation of inflammatory molecular pathways in OSAS. Arch. Physiol. Biochem. 114, 261–266. doi:10.1080/13813450802307337
Semenza G. L. (2020). The genomics and genetics of oxygen homeostasis. Annu. Rev. Genomics Hum. Genet. 21, 183–204. doi:10.1146/ANNUREV-GENOM-111119-073356
Siqués P., Brito J., León-Velarde F., Barrios L., de la Cruz J. J., López V., et al. (2006). Time course of cardiovascular and hematological responses in rats exposed to chronic intermittent hypobaric hypoxia (4600 m). High. Alt. Med. Biol. 7, 72–80. doi:10.1089/HAM.2006.7.72
Thake C. D., Mian T., Garnham A. W., Mian R. (2004). Leukocyte counts and neutrophil activity during 4 h of hypocapnic hypoxia equivalent to 4000 m. Aviat. Space Environ. Med. 75, 811–817.
Tremblay J. C., Ainslie P. N. (2021). Global and country-level estimates of human population at high altitude. Proc. Natl. Acad. Sci. U. S. A. 118, e2102463118. doi:10.1073/PNAS.2102463118
Viscor G., Torrella J. R., Corral L., Ricart A., Javierre C., Pages T., et al. (2018). Physiological and biological responses to short-term intermittent hypobaric hypoxia exposure: from sports and mountain medicine to new biomedical applications. Front. Physiol. 9, 814–820. doi:10.3389/fphys.2018.00814
Wang J. S., Lin H. Y., Cheng M. L., Wong M. K. (2007). Chronic intermittent hypoxia modulates eosinophil- and neutrophil-platelet aggregation and inflammatory cytokine secretion caused by strenuous exercise in men. J. Appl. Physiol. 103, 305–314. doi:10.1152/JAPPLPHYSIOL.00226.2007
Keywords: interleukins, lymphocytes, chronic intermittent hypobaric hypoxia, hematological response, inflammation
Citation: Calderon-Jofre R, Bernal G, Moraga D and Moraga FA (2025) Interleukins expression by rat Lymphocytes exposed to chronic intermittent normobaric hypoxia. Front. Physiol. 16:1520174. doi: 10.3389/fphys.2025.1520174
Received: 30 October 2024; Accepted: 07 March 2025;
Published: 26 March 2025.
Edited by:
Tatum S. Simonson, University of California, San Diego, United StatesCopyright © 2025 Calderon-Jofre, Bernal, Moraga and Moraga. This is an open-access article distributed under the terms of the Creative Commons Attribution License (CC BY). The use, distribution or reproduction in other forums is permitted, provided the original author(s) and the copyright owner(s) are credited and that the original publication in this journal is cited, in accordance with accepted academic practice. No use, distribution or reproduction is permitted which does not comply with these terms.
*Correspondence: Fernando A. Moraga, Zm1vcmFnYUB1Y24uY2w=
Disclaimer: All claims expressed in this article are solely those of the authors and do not necessarily represent those of their affiliated organizations, or those of the publisher, the editors and the reviewers. Any product that may be evaluated in this article or claim that may be made by its manufacturer is not guaranteed or endorsed by the publisher.
Research integrity at Frontiers
Learn more about the work of our research integrity team to safeguard the quality of each article we publish.